- 1Parasitology Research Laboratory (PRL), Institute of Molecular Biosciences, Mahidol University, Nakhon Pathom, Thailand
- 2Department of Parasitology, Faculty of Medicine Siriraj Hospital, Mahidol University, Bangkok, Thailand
- 3Department of Microbiology, Faculty of Science, Mahidol University, Bangkok, Thailand
Babesia bovis and B. bigemina are the most common tick-borne parasites that cause bovine babesiosis which effects livestock production, leading to economic losses in tropical and subtropical areas of the world. The aims of this study were to determine the molecular detection, genetic diversity and antigenicity prediction of B. bovis based on spherical body protein 2 (sbp-2) gene and B. bigemina based on rhoptry-associated protein 1a (rap-1a) gene in cattle in Thailand. By PCR assay, the molecular detection of B. bovis and B. bigemina infection revealed levels of 2.58% (4/155) and 5.80% (9/155), respectively. The phylograms showed that B. bovis sbp-2 and B. bigemina rap-1a sequences displayed 5 and 3 clades with similarity ranging between 85.53 to 100% and 98.28 to 100%, respectively, when compared within Thailand strain. Diversity analysis of sbp-2 and rap-1a sequences showed 18 and 4 haplotypes, respectively. The entropy analysis illustrated 104 and 7 polymorphic sites of sbp-2 and rap-1a nucleic acid sequences, respectively, while those of sbp-2 and rap-1a amino acid sequences showed 46 and 4 high entropy peaks, respectively. Motifs analysis exhibited the distribution and conservation among sbp-2 and rap-1a sequences. The continuous and discontinuous B-cell epitopes have also been evaluated in this work. Therefore, our findings may be used to ameliorate the understanding inputs of molecular phylogeny, genetic diversity and antigenicity of B. bovis and B. bigemina Thailand stains.
Highlights
● The rhoptry-associated protein-1a sequences of B. bigemina Thailand strain exhibited a phylogenetic proximity.
● TCS network showed 18 and 4 haplotypes of the B. bovis spherical body protein 2 and B. bigemina rhoptry-associated protein-1a genes, respectively.
● Amino acid sequence entropy showed 46 and 4 polymorphic sites of the spherical body protein 2 and rhoptry-associated protein-1a, respectively.
● The B. bovis spherical body protein 2 sequence motif was more diverse than the B. bigemina rhoptry-associated protein-1a sequence motif.
● B-cell epitopes of B. bigemina rhoptry-associated protein-1a sequences were conserved, while that of B. bovis spherical body protein 2 sequences were diverse.
Introduction
Bovine babesiosis is an important tick-borne hemoparasitic disease caused by Babesia bovis and B. bigemina, and transmitted by ticks (Silva et al., 2009; Sayed and Elshahawy, 2018). This disease leads to economic losses in livestock production in tropical and subtropical areas including Thailand (Uilenberg, 2006; Yusuf, 2017), with estimated losses of US$13.9-18.7 billion globally each year (Hurtado and Giraldo-Ríos, 2018; Marques et al., 2020). B. bovis and B. bigemina cause morbidity and mortality resulting in losses in milk and meat production, and other livestock by-products. Although B. bigemina is more widespread, B. bovis infection is the most critical and fatal because of its clinical manifestations including hyperthermia, anorexia, hemoglobinuria and neurological symptoms (Bock et al., 2004; Uilenberg, 2006; Sayed and Elshahawy, 2018). The diagnostic methods of bovine babesiosis have relied mostly on clinical signs (Wagner et al., 2002; Sayed and Elshahawy, 2018), microscopic examination of the parasites in Giemsa-stained blood smears (Almeria et al., 2001; Bock et al., 2004; Kumar et al., 2018; Mendes et al., 2019) and serological testing for antibody detection (Bono et al., 2008; Singh et al., 2009; Li et al., 2014; Lira-Amaya et al., 2021). The molecular method by polymerase chain reaction (PCR) is reliable and employed in diagnosing the infection (Kumar et al., 2018; Mendes et al., 2019). The occurrence of bovine babesiosis in different parts of Thailand has been reported in previous studies (Cao et al., 2012; Nagano et al., 2013; Simking et al., 2014; Tattiyapong et al., 2016; Jirapattharasate et al., 2016; Jirapattharasate et al., 2017).
The spherical body proteins (SBPs) secreted by spherical bodies are identified to belong to a family consisting of sbp-1, sbp-2, sbp-3 and sbp-4, which have been characterized in B. bovis (Yokoyama et al., 2006; Guo et al., 2018). The B. bovis sbp-2 protein is encoded by sbp-2 gene and has been identified as an immunostimulatory protein released into the host erythrocyte post-invasion and localized to the cytoplasmic side of the infected-erythrocyte membrane (Ruef et al., 2000; Yokoyama et al., 2006; Terkawi et al., 2011b; Lobo et al., 2012; Ganzinelli et al., 2018). The rhoptry-associated protein-1a is secreted by rhoptries organelles that participate in the success of invasion and establishment of intracellular parasitic viability (Cao et al., 2012; Kemp et al., 2013). The B. bigemina rap-1a gene is conserved and used for discriminating against field B. bigemina (Petrigh et al., 2008; Cao et al., 2012). Thus, both of B. bovis sbp-2 and B. bigemina rap-1a genes were candidates for molecular markers and potent in identifying specific B. bovis and B. bigemina, respectively (AbouLaila et al., 2010; Moumouni et al., 2015).
The scarcity of the genotyping and epidemiology information which might be used to control the disease is not available. And this includes the fact that little is known regarding the genetic diversity of B. bovis and B. bigemina isolates in Thailand (Sivakumar et al., 2018). Therefore, this study was done with the main objective of investigating molecular detection and genetic diversity of B. bovis sbp-2 and B. bigemina rap-1a genes in cattle from Northern, Western and Southern regions of Thailand. Additionally, in silico bioinformatic analyses among the isolated sequences identified in this work and those from other countries are presented. The results will provide further information on the genetic structure of these parasite populations.
Materials and methods
Collection of blood samples
The present study was carried out in three areas of Thailand. A total of 155, blood samples from apparently healthy cattle (Holstein Friesian cross-bred) aged between 1 and 5 years were randomly collected in Muang district (18°44′22″ N, 100°41′4″ E) of Nan province (n = 50) in northern area, in Photharam district (13°44′20″ N, 99°55′25″ E) of Ratchaburi province (n = 54) in western area as well as in Don-sak district (9°7′8″ N, 99°42′20″ E) of Surat Thani province (n = 15), Cha-wang district (8°28′10″ N, 99°32′3″ E) of Nakhon Si Thammarat province (n = 16) and Pa-phayom district (7°48′11″ N, 99°55′29″ E) of Phatthalung province (n = 20) in Southern area. Approximately 5 ml of whole blood samples were collected directly from the middle caudal coccygeal vein of each animal. They were transferred to a collection tube with Ethylene Diamine Tetraacetic Acid (EDTA) (BD Vacutainer®, USA), and stored at -20°C until the DNA extraction took place. The procedures of animal restraint and blood collection were performed by a licensed veterinarian.
DNA extraction
Genomic DNA (gDNA) of B. bovis and B. bigemina was extracted from cattle blood samples using a Tissue DNA Extraction Kit (OMEGA, bio-tex, USA) following the methods described by Junsiri et al. (2020) and Watthanadirek et al. (2021) with some modifications. Briefly, 250 µl of blood sample was transferred into a 1.5 ml microcentrifuge tube, and 25 µl of Omega Bio-tek (OB) protease solution and 250 µl of BL buffer (lysis buffer) were added. They were mixed and incubated for 10 min at 70°C. Then, 250 µl of absolute ethanol was added, and the sample was transferred to a HiBind® DNA Mini Column (OMEGA, bio-tex, USA), and centrifuged at maximum speed for 1 min at room temperature. After removing the filtrate, 500 µl of HBC binding buffer (High Salt Wash Buffer) was added and centrifuged at maximum speed for 30 sec at room temperature. After discarding the filtrate, 700 µl of DNA washing buffer was added and centrifuged at maximum speed for 30 sec at room temperature. Then, the column was transferred to a new microcentrifuge tube, and the DNA samples were eluted in 50 µl of MiliQ water. Finally, the purity and concentration of DNA samples were determined using NanoDrop™ 2000 Spectrophotometers (Thermo Scientific™) at the 260/280 and 260/230 ratios, and stored at -20°C until further use.
Molecular amplification and cloning of the Babesia bovis sbp-2 gene and Babesia bigemina rap-1a gene DNA
All specific primer pairs according to sequences from the previous studies (AbouLaila et al., 2010; Moumouni et al., 2015) were used for amplification of the B. bovis sbp-2 and B. bigemina rap-1a genes. The B. bovis sbp-2 and B. bigemina rap-1a genes were amplified by nested PCR assay using the specific primers. In the first step of amplification, outer forward F (5′-CTGGAAGTGGATCTCATGCAACC-3′) and outer reverse R (5′-TCACGAGCACTCTACGGCTTTGCAG-3′) were used for B. bovis sbp-2 gene, while outer forward F (5′-GAGTCTGCCAAATCCTTAC-3′) and outer reverse R (5′-TCCTCTACAGCTGCTTCG-3′) were used for B. bigemina rap-1a gene. In the second step, 2 µl (350 ng/µl) of the amplified PCR product was used for the subsequent nested PCR. The specific primers: inner forward F1 (5′-GAATCTAGGCATATAAGGCAT-3′) and inner reverse R1 (5′-CCCCTCCTAAGGTTGGCTAC-3′) were used for B. bovis sbp-2 gene, while inner forward F2 (5′-AGCTTGCTTTCACAACTCGCC-3′) and inner reverse R2 (5′-TTGGTGCTTTGACCGACGACAT-3′) were used for B. bigemina rap-1a gene. The PCR reaction containing 50 ng of DNA template, 0.2 µM each of the primers, 200 µM of each deoxynucleotide triphosphate (dNTPs), 1x standard Taq reaction buffer, nuclease-free water and 1.25 U Taq DNA polymerase (BioLabs®, USA), were performed in a thermal cycler (Bio-Rad, USA) with the following conditions: 35 cycles of denaturation at 94°C for 2 min, annealing at 60°C and 50°C for 1 min for the 1st and 2 nd steps of B. bovis sbp-2 gene, at 47°C and 56°C for 30 sec for 1st and 2 nd steps of B. bigemina rap-1a gene, extension at 68°C for 90 sec, and a final extension at 68°C for 5 min. The PCR products were stained with FluoroStain™ DNA Fluorescent Staining Dye (SMOBIO, Taiwan), analyzed by 1% agarose gels and visualized under ultraviolet (UV) transilluminator. A 100 bp DNA Ladder M (MolBio™ HIMEDIA®, India) was used as standard for determining the molecular mass of PCR products. Positive PCR products were purified using GenepHlow™ Gel/PCR Kit (Geneaid, Taiwan) following the manufacturer’s protocols for DNA cloning. The purified PCR products were quantified and cloned into the pGEM®-T Easy Vector (Promega, USA). Chemically competent Escherichia coli host strain TOP10 cells (Invitrogen, USA) were then transformed with the ligation products. Subsequently, 200 µl of transformed E. coli culture was spread on the Luria-Bertani (LB)-ampicillin agar plates after 100µl of 100mM IPTG, and 20µl of 50mg/ml X-Gal were spread on LB-ampicillin plate, then the plates were incubated at 37°C for overnight. The white colonies were selected and grown in LB medium containing ampicillin for overnight. The recombinant plasmids were extracted from the competent cells using Presto™ Mini Plasmid Kit (Geneaid, Taiwan) following the manufacturer’s instructions. The plasmid DNA products were amplified using the specific primers and the correct size of the inserts was analyzed by agarose gel electrophoresis.
Sequence and in silico analysis
Purified plasmid DNA products containing the B. bovis sbp-2 and B. bigemina rap-1a genes were confirmed by the Sanger method of DNA sequencing. All sequences were analyzed by BLAST (The National Center for Biotechnology Information, NCBI, http://www.ncbi.nlm.nih.gov/BLAST). All DNA sequences generated in the present study were submitted and deposited in GenBank database; accession numbers are provided in Table 1.
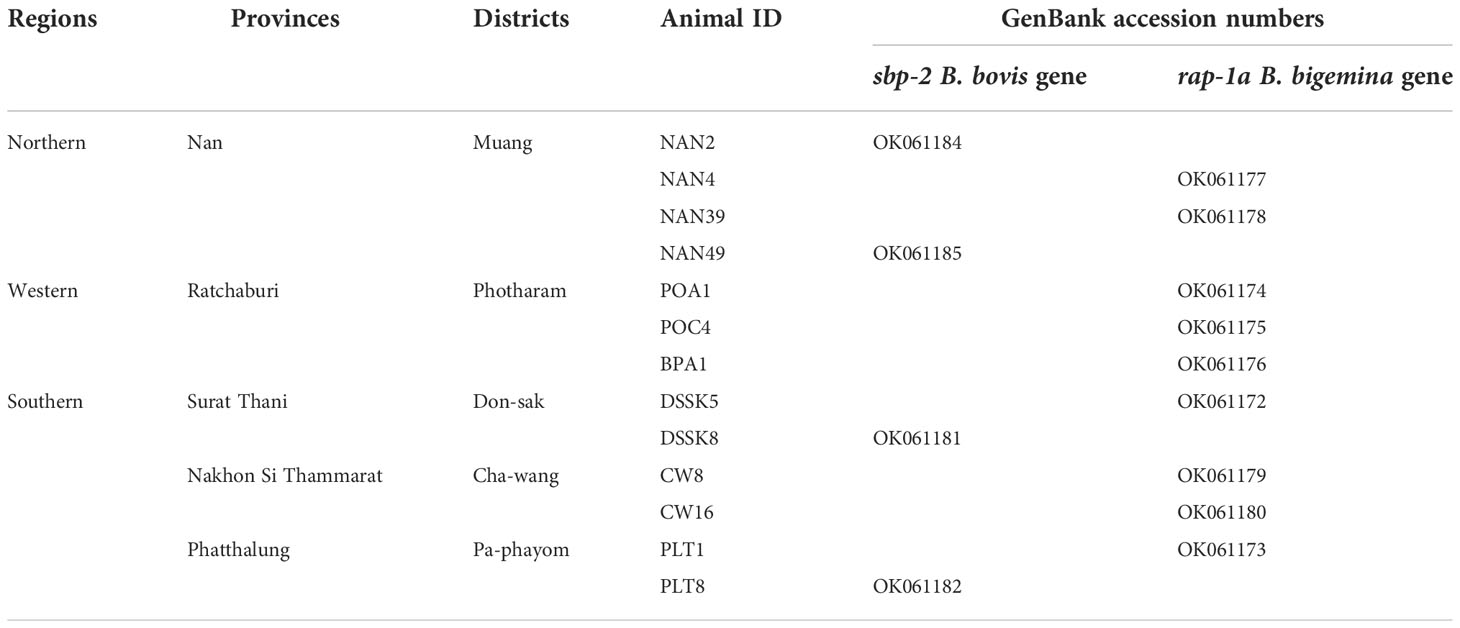
Table 1 The Babesia spp. nucleotide sequences amplified in Thailand strain were deposited in the GenBank database.
Phylogenetic tree analysis
The B. bovis sbp-2 and B. bigemina rap-1a gene sequences were utilized for sequence alignment and phylogenetic analysis. Multiple sequences alignments were carried out with the Clustal W algorithm, and then molecular phylogenetic trees were reconstructed using the maximum likelihood (ML) as implemented in the MEGA software v.7.0.26 (Kumar et al., 2016). Bootstrap analysis with 1000 repetitions was used to evaluate the confidence of the branching pattern of the trees. The evolutionary distances were computed using the Kimura 2-parameter method (Kimura, 1980). The sbp-4 and rap-1 sequences of B. bovis were used as appropriate outgroups for the construction of the phylogenetic trees of B. bovis sbp-2 and B. bigemina rap-1a sequences, respectively. The similarity of nucleic acid was also defined with a sequence identity matrix in Bioedit software v.7.0.5.3 (Hall, 1999).
Haplotype diversity analysis
The alignment of B. bovis sbp-2 and B. bigemina rap-1a sequences were used to assess the nucleotide diversity (π), diversity of haplotypes (Dh), number of haplotypes, and average number of nucleotide differences (K), using DNA Sequence Polymorphism (DnaSP) version 6.12.03 software (Rozas et al., 2017). In addition, all sequences were subjected to the Population Analysis with the Reticulate Trees (popART) program in order to analyze the Templeton, Crandall, and Sing (TCS) Network (Clement et al., 2002; Leigh and Bryant, 2015).
Entropy and motif analysis
The variability between the nucleic and amino acid sequences was analyzed by entropy estimation. The B. bovis sbp-2 and B. bigemina rap-1a nucleotide sequences were converted to amino acid sequences, aligned and analyzed by Shannon’s entropy (H(x)) plot using Bioedit software version 7.2.5 (Hall, 1999). Also, the motif analysis of nucleic and amino acid was performed in multiple expectation maximizations for motif elicitation (MEME) suite tools (https://meme-suite.org/meme/) with E-values less than 0.05 (Bailey and Elkan, 1994).
B-cell epitopes prediction
Nucleotide sequences of B. bovis sbp-2 and B. bigemina rap-1a genes were translated to amino acid sequences and then submitted to the VaxiJen v.2.0 Server and the Immune Epitope Database and Analysis Resource (IEDB-AR, http://tools.iedb.org/bcell/) for B-cell epitopes analysis (Vita et al., 2019). The antigenic determinants on protein antigens were predicted by a semi-empirical method (Kolaskar and Tongaonkar, 1990). Linear sequences-based analysis of the B. bovis sbp-2 and B. bigemina rap-1a was used to predict the continuous B-cell epitopes, BepiPred linear epitope, Parker hydrophilicity, Emini surface accessibility, Chou & Fasman Beta-turn and Karplus & Schulz flexibility. The 3D structure of antigen was evaluated using the Ellipro Server for prediction of the discontinuous B-cell epitopes
Results
Molecular detection of Babesia infection in cattle blood samples
The Babesia spp. detected by nested PCR was demonstrated, and the size of PCR products of B. bovis and B. bigemina were 584 and 412 bp, respectively. The representative positive PCR products for each of the parasites tested were exhibited in Supplementary Figure 1. The PCR results showed that 4/155 (2.58%) and 9/155 (5.80%) of cattle blood samples were infected with B. bovis and B. bigemina, respectively. B. bovis infections were found in Nan, Surat Thani and Phatthalung provinces, whilst B. bigemina infections were mainly detected in Ratchaburi, followed by Nan, Surat Thani, Nakhon Si Thammarat and Phatthalung provinces as shown in Table 1.
Phylogenetic, similarity and sequence analysis of the Babesia bovis sbp-2 and Babesia bigemina rap-1a gene sequences
The phylogenetic tree based on the sequence alignment of the B. bovis sbp-2 gene obtained in this study along with the other sequences retrieved from the GenBank was divided into 5 clades (designated as clade 1-5). The Thailand sequences are positioned in the 2nd and 3rd clade together with the sequences from other countries (Figure 1A) The percentage similarity of Thailand B. bovis sequences within each clade was 85.53-100% (2nd clade) and 95.40-100% (3rd clade), while the similarity of the sequences within other clades was 85.57-100% (1st clade), 89.07-100% (4th clade) and 100% (5th clade) as shown in Table 2. For phylogenetic tree of the B. bigemina rap-1a gene, the sequences were classified as 3 clades in the phylogram. Our nine sequences were found in clade 1 together with the sequences from Philippines, Indonesia and South Africa. The Thailand sequences showed phylogenetic proximity representing the genetic variability of B. bigemina rap-1a sequence from this study (Figure 1B). The total similarity among Thailand B. bigemina sequences was 100% (1st clade), whereas the similarity of the remaining sequences within other clades was 98.53-100% (2nd clade) and 100% (3rd clade) as demonstrated in Table 3.
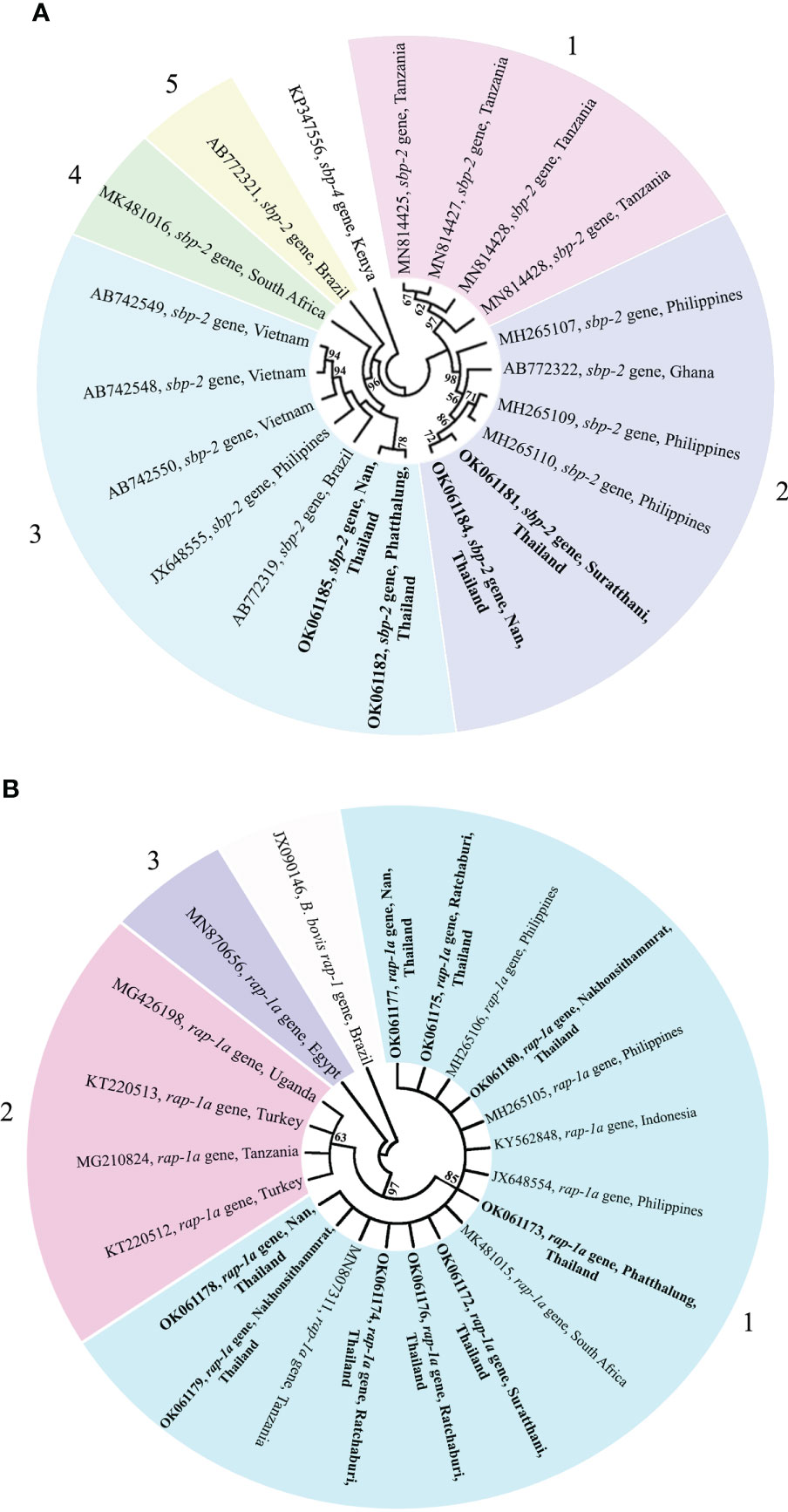
Figure 1 A maximum likelihood phylogenetic cladogram of B bovis sbp-2 (A) and B bigemina rap-1a (B) gene sequences in this study (boldface) and those taken from GenBank. The numbers on each node are consistent with the bootstrap analysis of 1000 replicates (only percentage greater than 60% were represented). The GenBank accession numbers of the sequences used in the phylogenetic analysis are exhibited. Two sequences of B bovis sbp-4 (A) and rap-1 (B) gene are used as outer groups. Different colors represent the different clades.
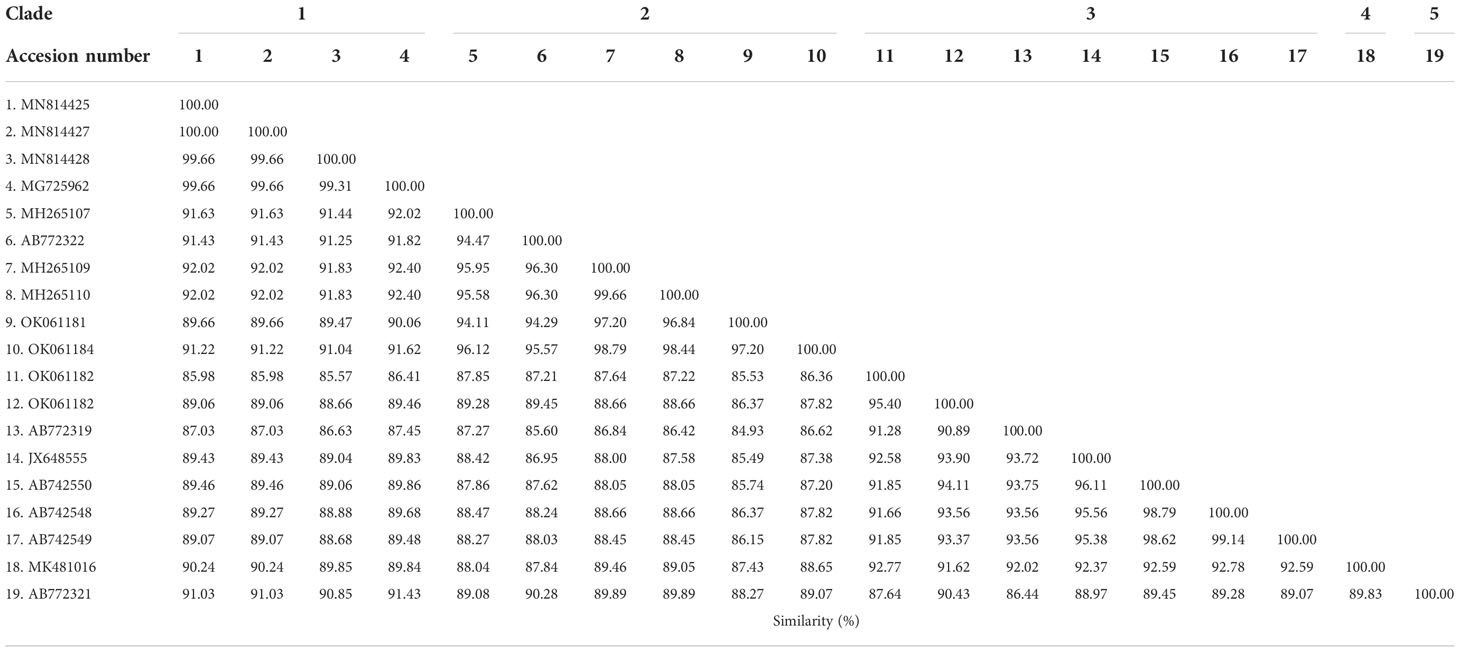
Table 2 Similarity of the B. bovis sbp-2 gene sequences as examined in cattle samples in Thailand and other countries.
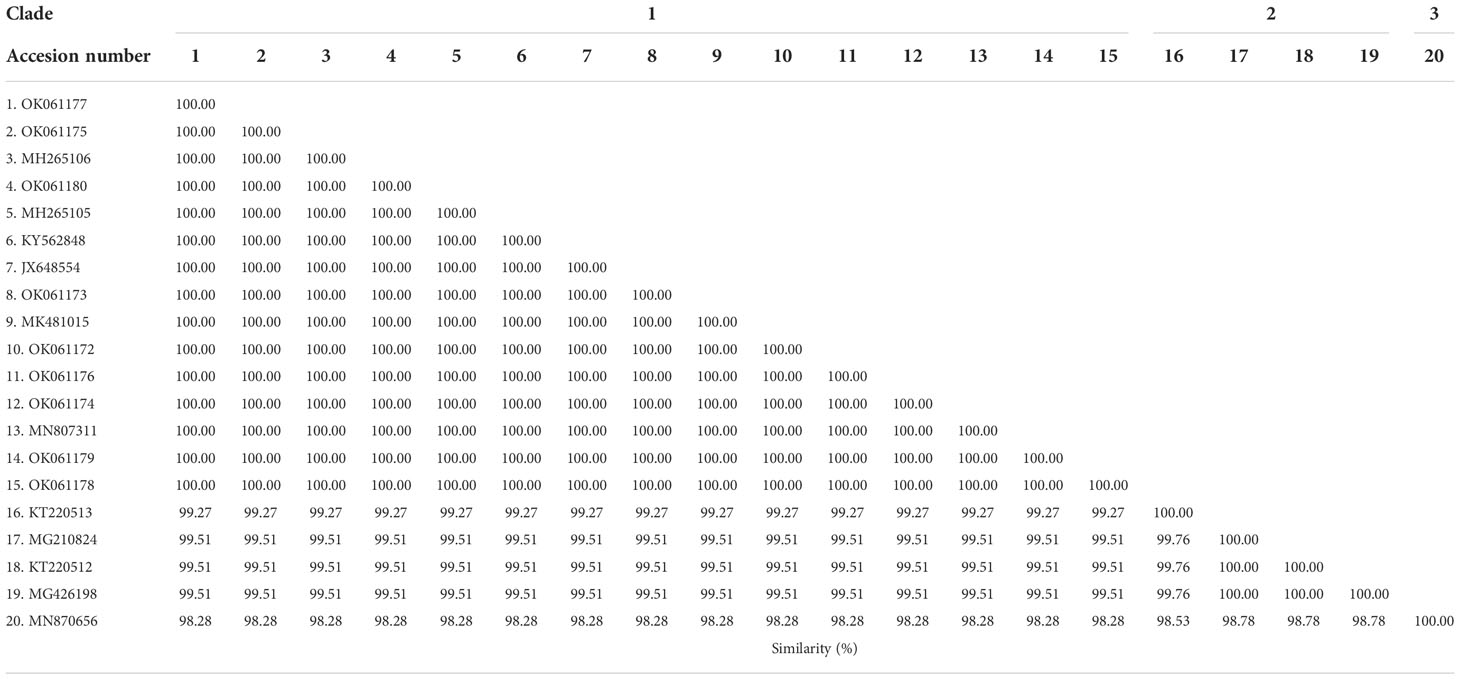
Table 3 Similarity of the B. bigemina rap-1a gene sequences as detected in cattle samples in Thailand and other countries.
In addition, the nucleic acid substitution rate in sbp-2 sequences among B. bovis and rap-1a sequences among B. bigemina was evaluated under the Tamura and Nei (1993) mode. The different transitional and transversional substitutions of B. bovis sbp-2 sequences were 14.06 and 5.47, respectively, while those of B. bigemina rap-1a sequences were 18.79 and 3.11, respectively (Supplementary Table 1). The alignment of B. bovis sbp-2 sequence of Thailand strains and other strains revealed the 420 and 162 positions of consensus and non-consensus, respectively. The consensus and non-consensus of B. bigemina rap-1a sequences alignment were 404 and 8 positions, respectively, as demonstrated in Supplementary Figures 2 and 3. In addition, the consensus and non-consensus of B. bovis sbp-2 amino acid sequence alignments were 121 and 72 positions, respectively, while the alignment of B. bigemina rap-1a amino acid sequences revealed 132 and 4 positions of the consensus and non-consensus, respectively (Supplementary Figure 4). Seventy-two positions of the amino acid (aa) sequences revealed high genetic variation among B. bovis strains (Supplementary Table 2), whereas four aa were found in the genetic variation among B. bigemina strains (Supplementary Table 3). The highest variation of rap-1a aa position among 20 sequences was aa 130 [from Asparagine (Asn; N) to Aspartic acid (Asp; D)], as shown in Supplementary Table 3.
Haplotype diversity
The haplotype network of B. bovis sbp-2 and B. bigemina rap-1a gene sequences was established by the TCS Network tool. The haplotype of each gene was estimated and displayed high variation from multiple sequence alignments. The B. bovis sbp-2 gene showed a greater number of nucleotide variations and higher diversity than B. bigemina rap-1a gene as demonstrated in Table 4 and Figure 2. For haplotype analysis of B. bovis sbp-2 gene, 18 haplotypes shown in TCS network displayed that haplotype #9 and #11 were obtained from Nan province, haplotype #8 was found in Surat Thani province, and haplotype #10 was observed from Phatthalung province of Thailand. Our haplotypes from Thailand formed the nearest branch to the haplotype from the Philippines. The rest of the haplotypes from the Philippines, Ghana, Tanzania, Brazil, Vietnam, South Africa were exhibited in Figure 2A. In addition, a total of 4 different haplotypes were analyzed based on the B. bigemina rap-1a gene. Our nine sequences from Nan, Ratchaburi, Surat Thani, Nakhon Si Thammarat and Phatthalung provinces of Thailand were grouped together in haplotype #1 with the Philippines, Tanzania, South Africa, Indonesia. The rest of the haplotypes were obtained from other countries as shown in Figure 2B.
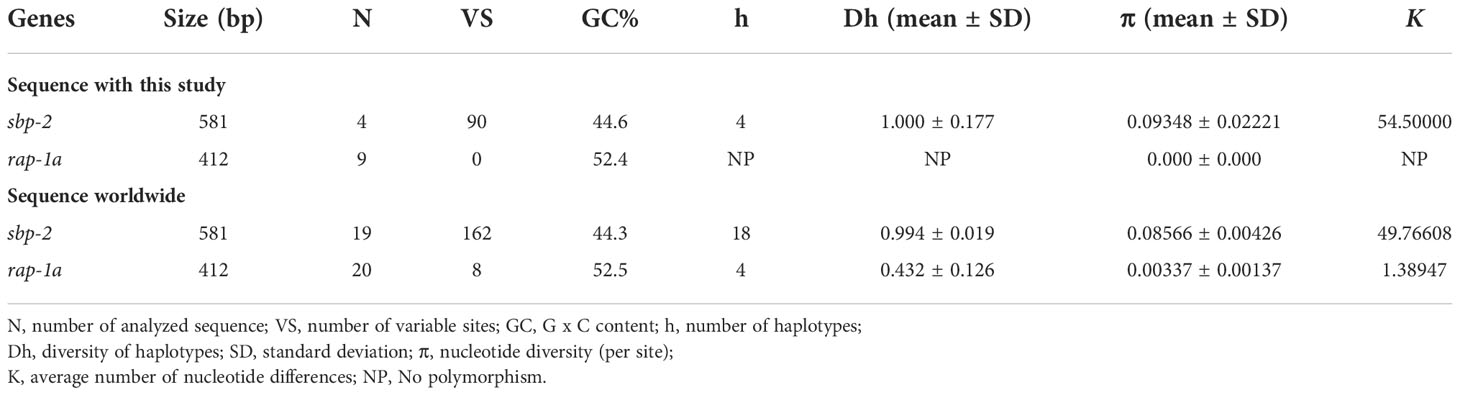
Table 4 Polymorphism and genetic diversity of the B. bovis sbp-2 and B. bigemina rap-1a gene sequences as examined in cattle samples in Thailand and other countries.
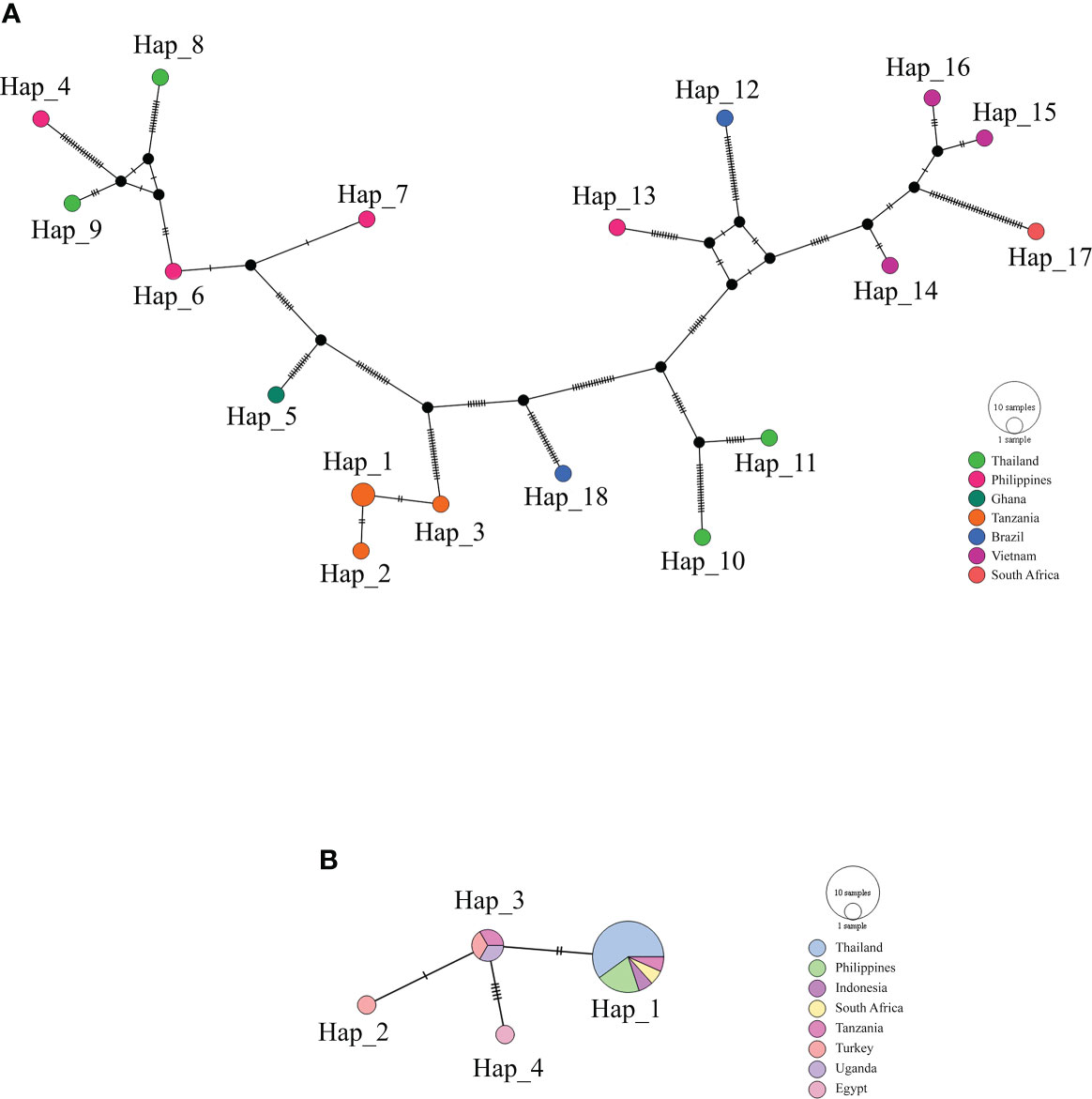
Figure 2 TCS network of haplotypes based on the B bovis sbp-2 (A) and B bigemina rap-1a (B) gene sequences detected in Thailand and worldwide countries. Small traits between a haplotype and another stand for mutational occurrence. The black circles are the intermediated traits caused by the single nucleotide polymorphism (SNP).
Entropy and motif analyses
To evaluate nucleic acid entropy, the B. bovis sbp-2 gene sequences showed 104 polymorphic sites with entropy values ranging from 0.20619 to 1.02809 (Figure 3A), while the B. bigemina rap-1a gene sequences exhibited 7 polymorphic sites with entropy values ranged between 0.19852 and 0.56234 (Figure 3B). Entropy analysis of amino acid sequences was conducted using the sbp-2 and rap-1a amino acid sequences alignments. The charts exhibited 46 and 4 high entropy peaks for the sbp-2 (Figure 3C) and rap-1a (Figure 3D) amino acid sequences, respectively. The entropy values were observed with the range of 0.20619 to 1.2064 for sbp-2 (Figure 3C) and 0.19852 to 0.56234 for rap-1a (Figure 3D) amino acid sequences, respectively.
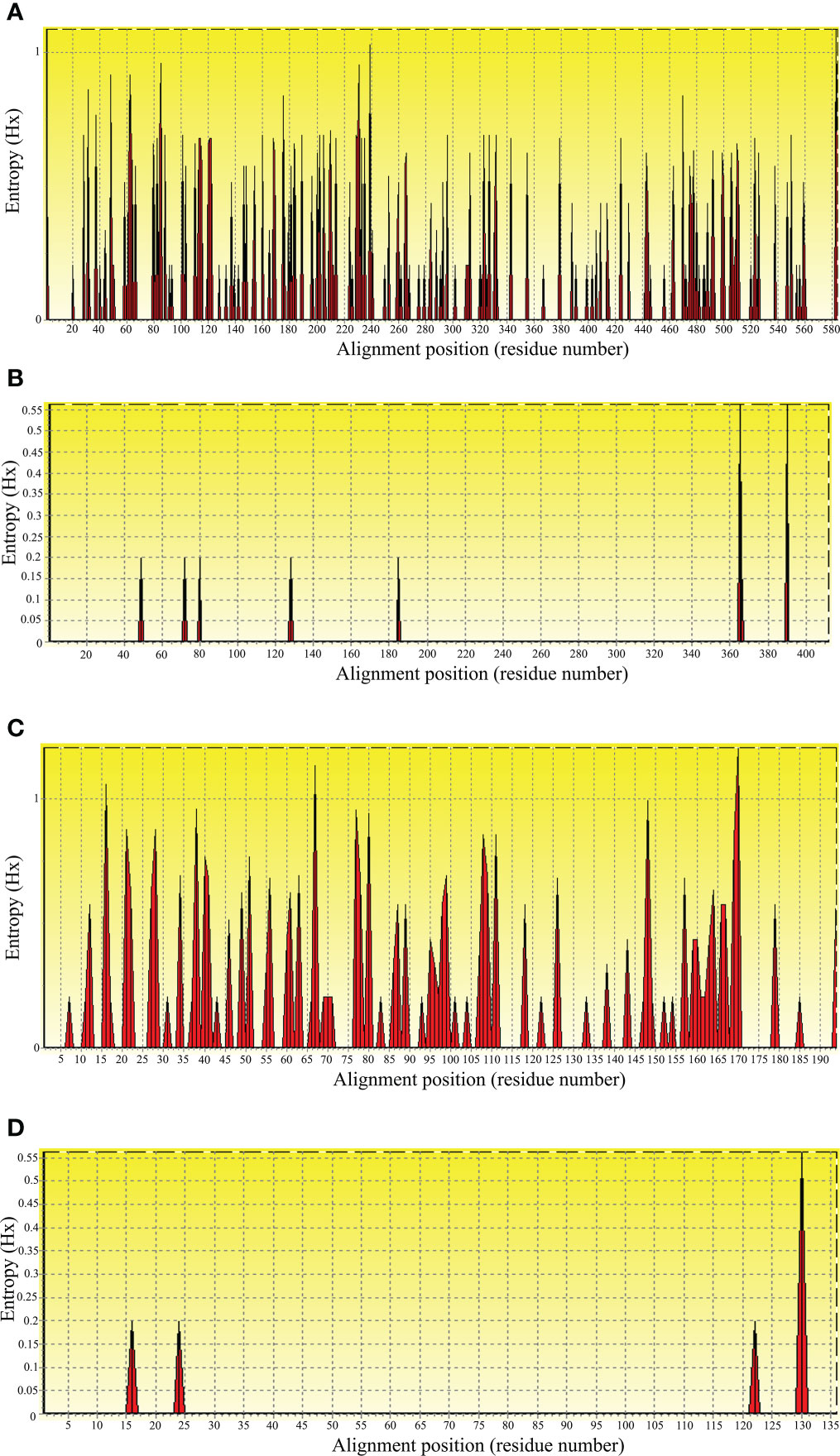
Figure 3 Entropy analysis of Babesia gene sequences. Entropy plot of multiple nucleic acid sequence alignment of B bovis sbp-2 (A) and B bigemina rap-1a (B) genes. Entropy plot of multiple amino acid sequence alignment of sbp-2 (C) and rap-1a (D). The red peaks indicate the high variation at each position of the nucleic (A, B) and amino (C, D) acid sequences.
The motifs analysis of B. bovis sbp-2 and B. bigemina rap-1a gene sequences showed three conserved motifs in each sequence. These motifs are distributed and conserved among the sbp-2 and rap-1a gene sequences. Although the DNA motif was highly conserved, the sbp-2 DNA motif was more diverse than the rap-1a DNA motif (Figures 4A, C). Also, the motif analysis of the sbp-2 and rap-1a amino acid sequences exhibited three conserved motifs among each amino acid sequence (Figures 4B, D). Our motif analysis results were consistent with other results including the genetic diversity, similarity, haplotype, entropy and the sequence alignment, which is the B. bovis sbp-2 has more diversity than B. bigemina rap-1a in both of DNA and amino acid sequences.
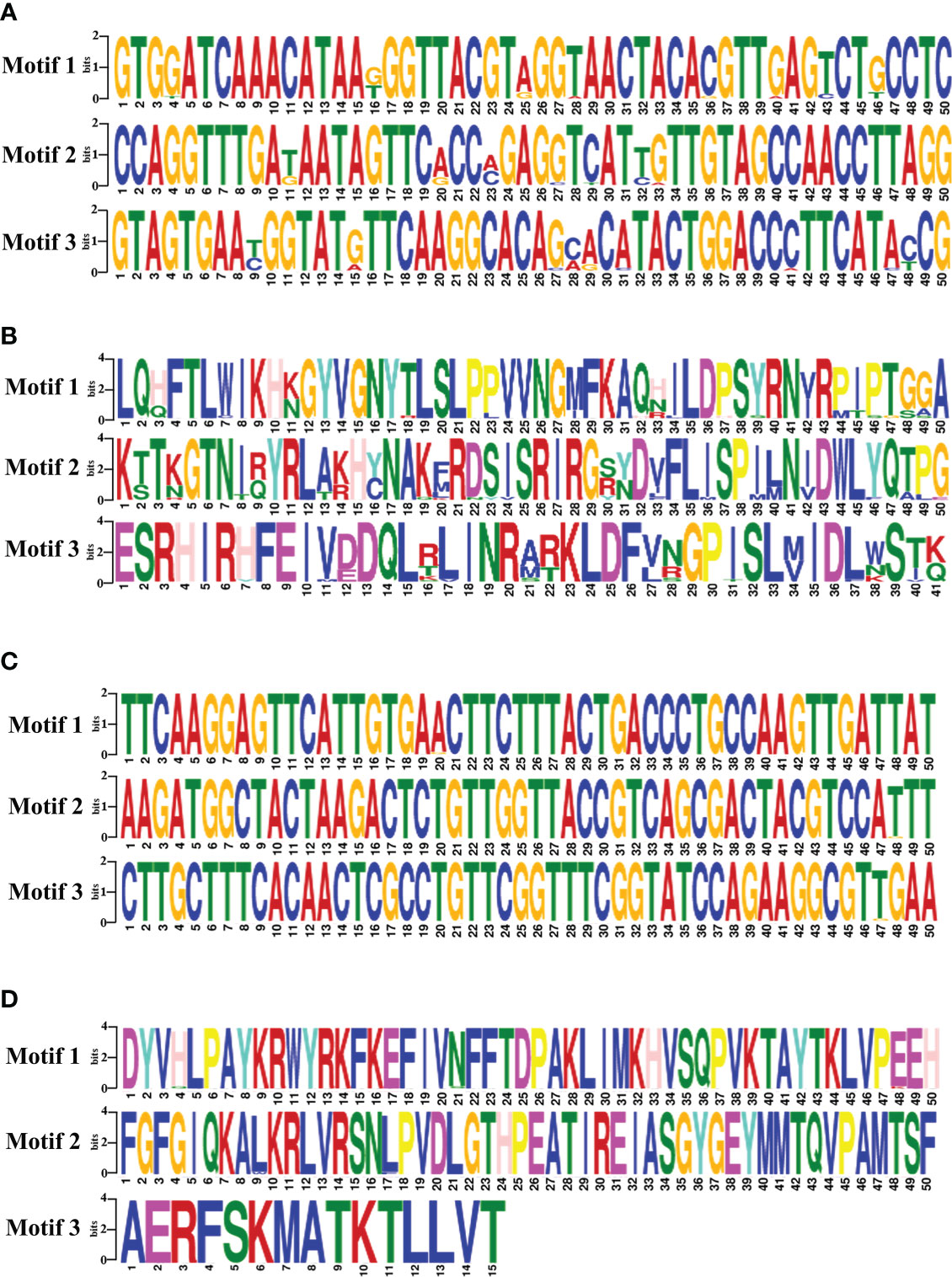
Figure 4 The motifs analysis of B bovis sbp-2 nucleic (A) and amino (B) acid sequences as well as B bigemina rap-1a nucleic (C) and amino (D) acid sequences.
B-cell epitopes analysis
The B-cell epitopes predicted among Thailand B. bovis sbp-2 and B. bigemina rap-1a amino acid sequences were conserved, and all others were observed to be highly polymorphic (Table 5). The continuous B-cell epitopes were anticipated for antigenic properties along with amino acid sequences by the Kolaskar & Tongaonkar antigenicity algorithm. The average antigenicity of sbp-2 (Figure 5A) and rap-1a (Figure 6A) amino acid sequences were 1.041 (minimum = 0.891; maximum = 1.250) and 1.035 (minimum = 0.923; maximum = 1.170), respectively. In addition, the physicochemical properties of continuous B-cell epitopes were analyzed by BepiPred linear epitope, Chou & Fasman Beta-turn, Emini surface accessibility, Karplus & Schulz flexibility and Parker hydrophilicity predictions. Our findings exhibited an overlapping amino acid region that could be a candidate for B-cell epitopes recognition and accessible for antibody binding (Figures 5B–F and 6B–F). However, the discontinuous B-cell epitopes were predicted based on the 3D structure of sbp-2 (Supplementary Table 4 and Figures 7A–D) and rap-1a (Supplementary Table 4 and Figure 8A–C) amino acid sequences using the ElliPro Server, which overlapped with the linear sequence of B-cell epitopes. The yellow color of the Ellipro graphs indicated the presence of these antigenic properties in that region of sbp-2 (Figure 7E) and rap-1a (Figure 8D) correlating the BepiPred linear epitope prediction.
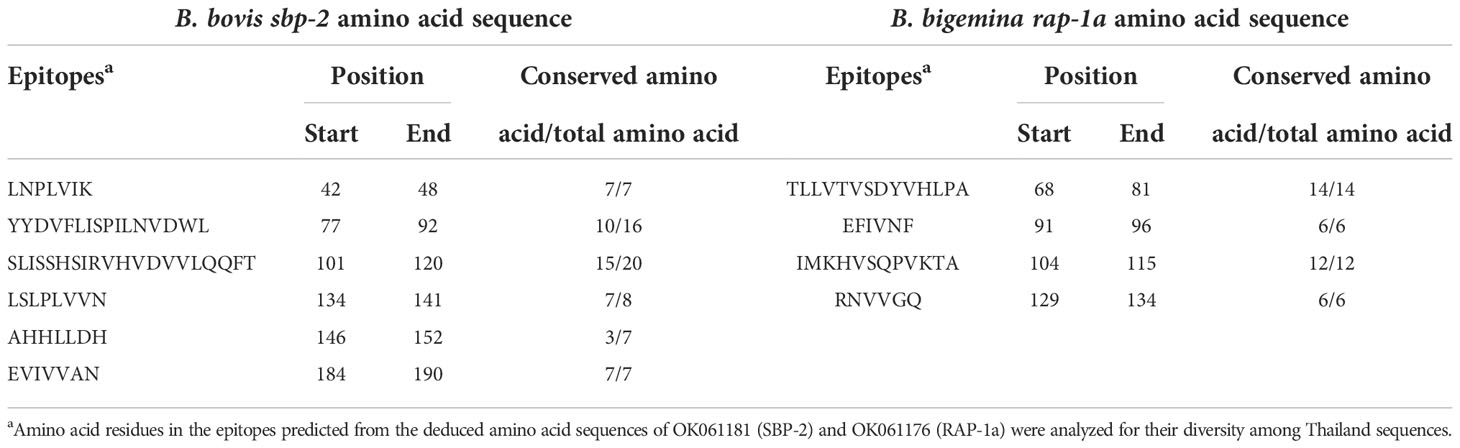
Table 5 Antigenicity of B-cell epitopes predicted from sbp-2 and rap-1a amino acid sequences determined in the present study.
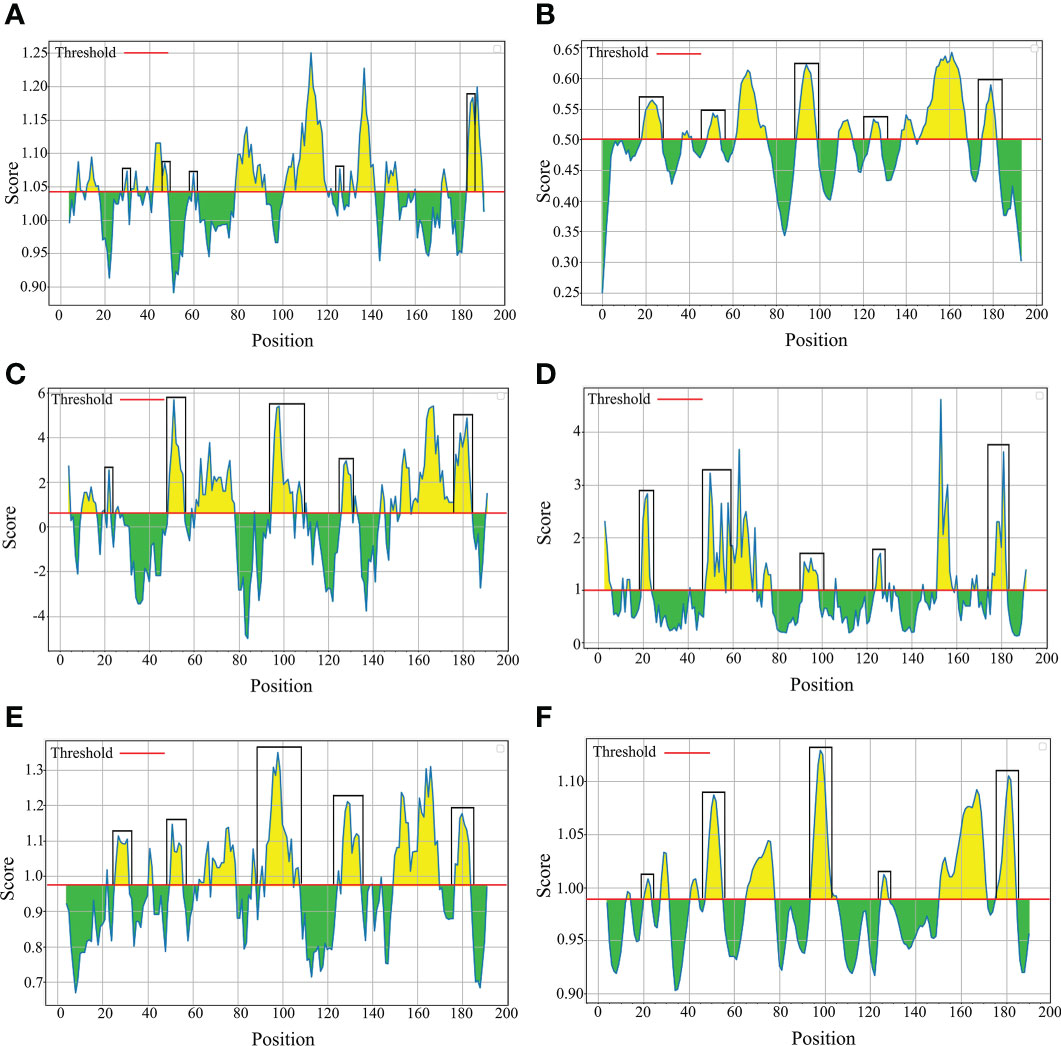
Figure 5 The physicochemical properties of B bovis sbp-2 protein were assessed based on Kolaskar & Tongaonkar antigenicity (A), BepiPred linear epitope (B), Parker hydrophilicity (C), Emini surface accessibility (D), Chou & Fasman Beta-turn (E) and Karplus & Schulz flexibility prediction (F).
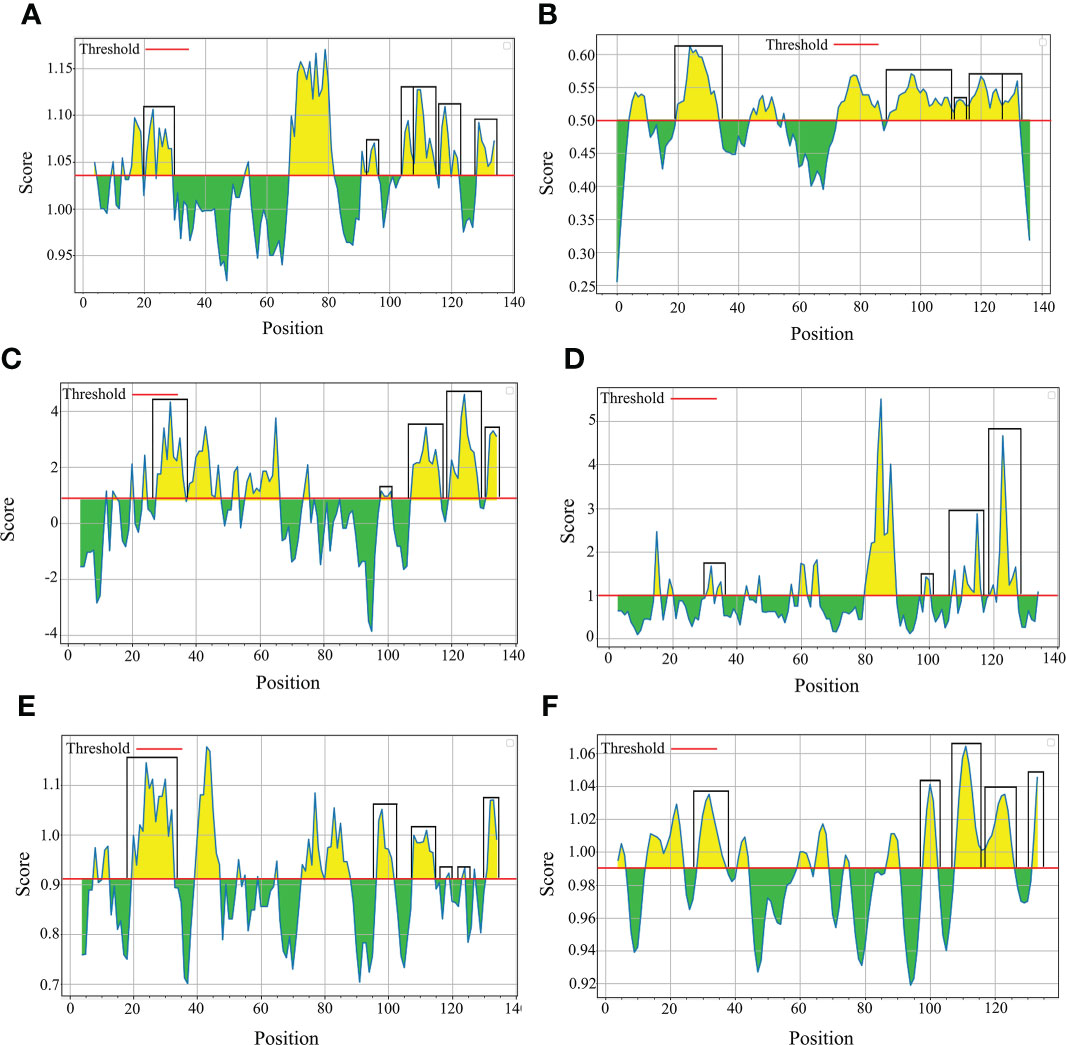
Figure 6 The physicochemical properties of B bigemina rap-1a protein were assessed based on Kolaskar & Tongaonkar antigenicity (A), BepiPred linear epitope (B), Parker hydrophilicity (C), Emini surface accessibility (D), Chou & Fasman Beta-turn (E) and Karplus & Schulz flexibility prediction (F).
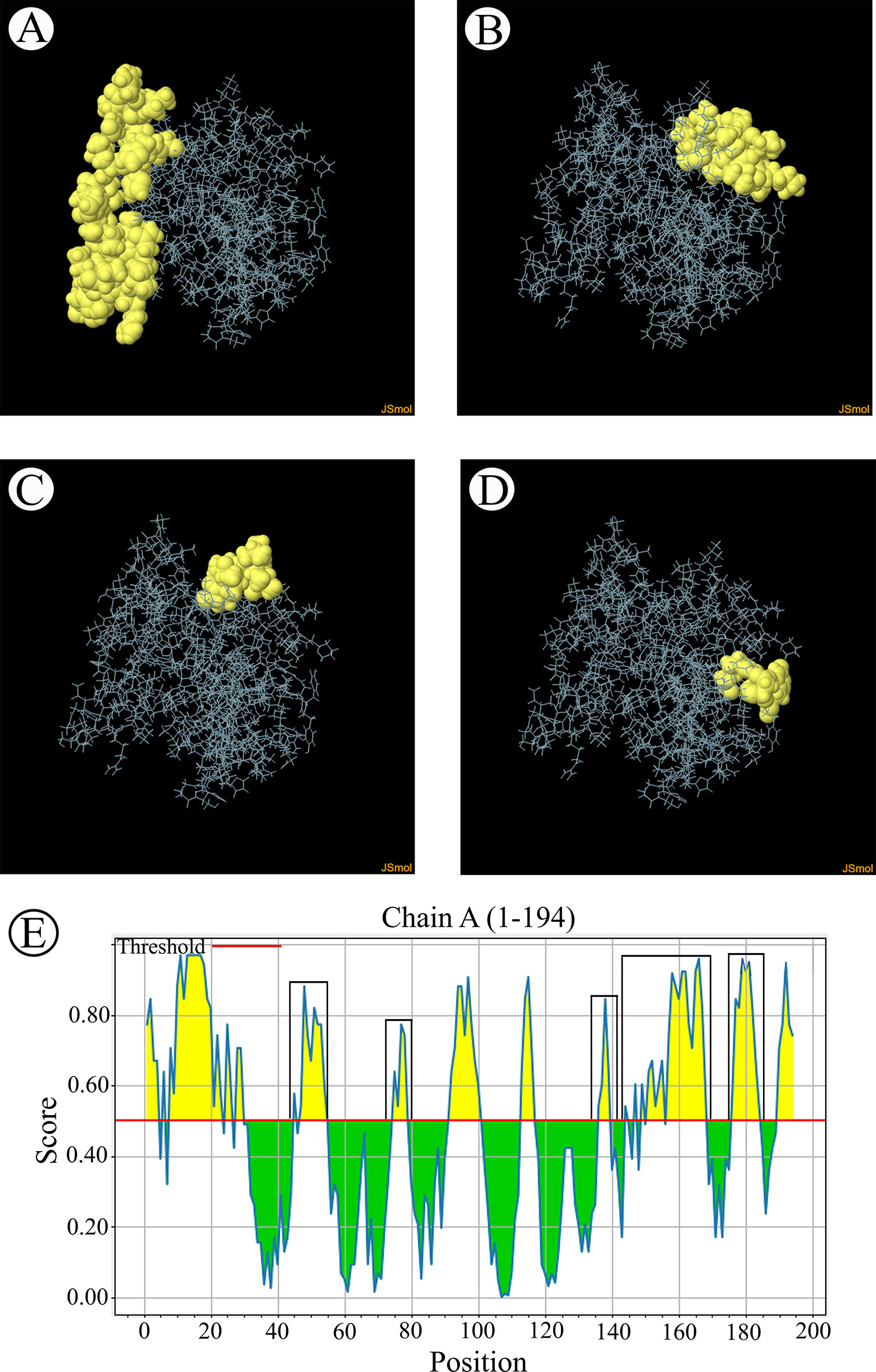
Figure 7 The predicted discontinuous B-cell epitopes based on the 3D structure of B bovis sbp-2 protein (A–D) displayed the overlapping region in the same position as the B-cell linear structure prediction (E).
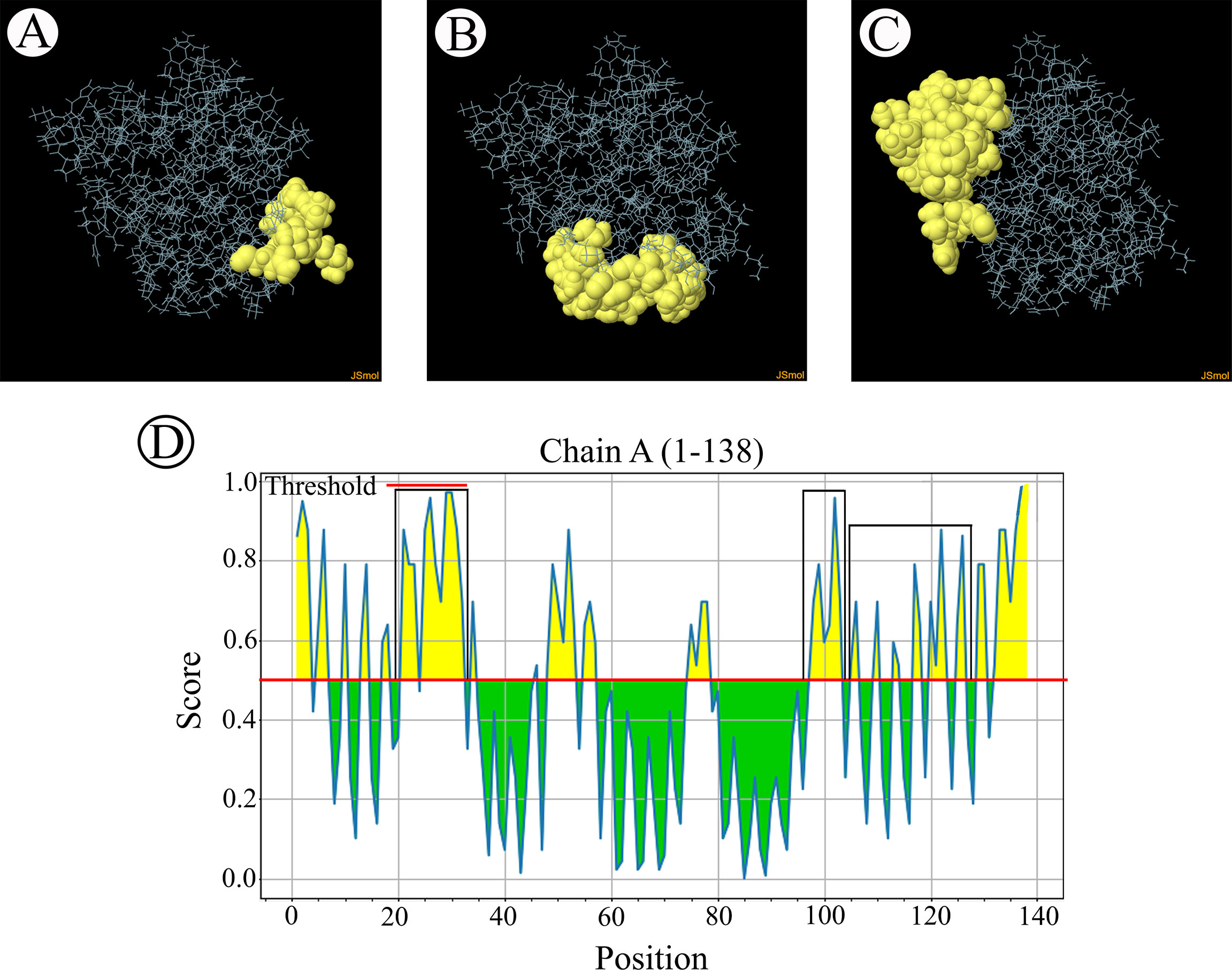
Figure 8 The predicted discontinuous B-cell epitopes based on the 3D structure of B bigemina rap-1a protein (A–C) displayed the overlapping region in the same position as the B-cell linear structure prediction (D).
Discussion
In Thailand, bovine babesiosis is a serious disease that causes clinical infection in cattle resulting in decreased livestock production and economic losses (Bock et al., 2004; Uilenberg, 2006; Simking et al., 2014). Currently, a molecular assay such as nested PCR is a sensitive diagnostic method and employed for detection and characterization of the parasites during the low level of parasitemia in infected animals (Smeenk et al., 2000; Altay et al., 2008; AbouLaila et al., 2010; Mosqueda et al., 2012). Our results showed a molecular detection of Babesia spp. in cattle blood sample in three regions (northern, western, and southern) of Thailand. The molecular assay revealed that of the cattle sampled, 2.58% (4/155) and 5.80% (9/155) were positive for B. bovis sbp-2 and B. bigemina rap-1a genes, respectively. Both sbp-2 and rap-1a genes have been employed as good markers for establishing phylogeographic patterns on the large scale and beneficial for epidemiological study of babesiosis (AbouLaila et al., 2010; Terkawi et al., 2011a; Cao et al., 2012; Moumouni et al., 2015; Zhou et al., 2016). The infection rate of B. bovis and B. bigemina in this study is lower than those reported by the epidemiology studies in Thailand that showed 5.3% (Simking et al., 2014) and 12.9% (Cao et al., 2012). In addition, the low occurrence of B. bovis compared to B. bigemina in the present study also agrees with previous reports (Awad et al., 2011; Terkawi et al., 2011a; Cao et al., 2012; Ibrahim et al., 2013; Simking et al., 2014; Jirapattharasate et al., 2016). Therefore, the chance of transmission of B. bovis is lower than that of B. bigemina in cattle in Thailand (Jirapattharasate et al., 2016). Moreover, the occurrence of B. bovis using sbp-4 gene was found to be 11.2% in the northeast region of Thailand (Terkawi et al., 2011a), and the ITS1-5.8s rRNA gene has been used to determine the genetic diversity of B. bigemina-infected beef cattle in Thailand (Jirapattharasate et al., 2017). This is the first study that revealed a molecular detection of Babesia spp. in cattle in 5 provinces (Nan, Ratchaburi, Surat Thani, Nakhon Si Thammarat and Phatthalung) of Thailand. From our results, it is possible that the increase in livestock industry and urbanization might lead to migration of animals in these provinces and also a risk of transboundary disease might be increased. Additionally, the seasonal sampling and population density of ticks and cattle raising might be the risk factors for Babesia infections (Ahantarig et al., 2008; Iseki et al., 2010; Awad et al., 2011; Terkawi et al., 2011a; Simking et al., 2014).
The genetic diversity of B. bovis and B. bigemina strains based on the sequences of sbp-2 and rap-1a genes, respectively, has been evaluated in several countries (Nagano et al., 2013; Moumouni et al., 2015; Guswanto et al., 2017; Herrera et al., 2017; Galon et al., 2019). Unfortunately, a little is known regarding the diversity of B. bovis and B. bigemina Thailand strains. In this work, the sbp-2 and rap-1a genes in cattle population in the northern, western and southern regions of Thailand were used to assess the genetic diversity of B. bovis and B. bigemina in these areas, respectively. The phylogenetic analysis of B. bovis sbp-2 gene Thailand strain showed 2 clades with other strains, while B. bigemina rap-1a gene Thailand isolate revealed a clade which showed phylogenetic proximity. Our bootstraps on the phylogenetic tree were 60-98% of bootstrap values, which are consistent with the of a majority-rule consensus tree of 1000 replicates for each alignment (Buckley and Cunningham, 2002; Soltis and Soltis, 2003). The results exhibited that the genetic diversity observed in the phylogram was confirmed by the high similarity values for B. bovis sbp-2 gene (85.53-100%). This finding showed that the genetic diversity among B. bovis populations has been varied according to the geographical areas with further diversification resulting from local selection pressure. Furthermore, the high similarity value was detected for B. bigemina rap-1a gene (100%). This finding indicated the phylogenetic proximity of B. bigemina rap-1a gene circulating in both different countries and Thailand.
In this study, the genotype of B. bovis sbp-2 and B. bigemina rap-1a sequences were established in the haplotype TCS networks. They were conducted with the sequences observed in this work together with other sequences obtained from GenBank database that was found in other regions of the world. Our finding revealed that there was a high genetic diversity of B. bovis sbp-2 and B. bigemina rap-1a genes detected in the different haplotype networks in Thailand and worldwide when compared to the earlier study of Mendes and colleagues (2019) who showed the diversity of haplotypes of B. bovis msa-2b gene (1.000 ± 0.027) and B. bovis msa-2c gene (0.9794 ± 0.039) detected in Brazilian cattle (Mendes et al., 2019). In addition, sequences observed in this work were clustered with sequences from different countries, indicating that there has been no unique haplotype in Thailand. These two sequences shared genetic traits with all sequences previously investigated worldwide. These indicated that the genetic diversity among Babesia populations varied in accordance with the geographical areas (Homer et al., 2000; Bock et al., 2004; Uilenberg, 2006). Notably, it is possible that the B. bovis and B. bigemina strains acquainted by importing cattle from other countries might have contributed to the observed genetic diversity of sbp-2 and rap-1a gene sequences in Thailand (Iseki et al., 2010; Lau et al., 2010; Tattiyapong et al., 2016; Sivakumar et al., 2018).
Regarding the analysis of Babesia nucleic and amino acid sequences, the sbp-2 genes have higher levels of sequence polymorphism than those of rap-1a genes. For codon degeneration, most codon degeneracies of B. bovis sbp-2 gene showed the synonymous (Ks) and non-synonymous (Kn) substitutions values were 0.1875 and 0.0708, respectively, while the B. bigemina rap-1a gene showed the Ks and Kn values were 0.0129 and 0.0064, respectively. The non-synonymous to synonymous mutations ratio (Kn/Ks) of B. bovis sbp-2 and B. bigemina rap-1a genes were 0.3776 and 0.4961 which interpreted as neutral selection (Niu et al., 2015; Rittipornlertrak et al., 2017). This indicated that the evolutionary substitution of mutations in coding sequences might not affect the protein sequence modification and could not impact change the biological function effect of a protein (Goymer, 2007; Choi and Chan, 2015).
In this study, our results exhibited the polymorphism with 104 entropy peaks reaching up to 1.02809 for B. bovis sbp-2 gene sequences and with 7 entropy peaks reaching up to 0.56234 for B. bigemina rap-1a gene sequences. In addition, the analysis of amino acid sequences of B. bovis sbp-2 and B. bigemina rap-1a sequences showed the polymorphism with 46 and 4 entropy peaks continuing up to 1.2064 and 0.56234, respectively. These findings indicated that different genotypes might involve being a genetic diversity of Babesia distribution in Thailand. Notably, our results were in line with the previous report of Mendes et al. (2019) who exhibited the entropy values of B. bovis msa-2b gene reaching up to 1.53, and B. bovis msa-2c gene with entropy values reaching up to 1.09. The high entropy values indicate a high number of variations of single-nucleotide polymorphisms (SNP), which is associated with a high number of nucleotide and amino acid variables (Mendes et al., 2019). The low entropy values indicate a few numbers of variations of SNP in each sequence (Pham, 2010). Furthermore, motif analysis gains information for determining the functional properties of biopolymers (nucleotide and protein subunits) and representing the roles in biological networks, such as DNA binding sites and protein interaction domains (Bailey et al., 2009; Nystrom and McKay, 2021). The analysis of the conserved motifs was correlated with our findings, and these motifs were essential for the parasite in evading the host immune system (Dijkstra et al., 2018).
In the present study, the results showed that the predicted B-cell epitopes of sbp-2 and rap-1a amino acid sequences among Thailand isolates were relatively conserved. Although many epitopes of sbp-2 and rap-1a amino acid sequences were conserved as functional peptides, they are not located within the signal peptide. In addition, the sbp-2 and rap-1 proteins were identified as the conserved peptide containing B-cell epitopes in B. bovis that involved Th1 immune response in an in vivo study (Gallego-Lopez et al., 2019; Hidalgo-Ruiz et al., 2022). However, further research into the functional properties of the rap-1a protein in B. bigemina was required. The high polymorphism investigated among the epitope prediction of sbp-2 amino acid sequences might exhibit the highest evolutionarily variability, suggesting that the antigenicity of variable sequence and evolutionarily conserved regions could induce immunological tolerance (Benjamin et al., 1984). Furthermore, B. bovis sbp-2 protein has been associated to increased parasite virulence and severe disease outcomes (Gallego-Lopez et al., 2019). This implies that the sbp-2 protein may help parasites to evade the host defense mechanism, and it is believed that B. bovis infection is a severe disease because of this. (Suarez et al., 2000; Bock et al., 2004; Yusuf, 2017; Gallego-Lopez et al., 2019).
Conclusions
The present study importantly indicates a molecular detection and genetic diversity of B. bovis and B. bigemina in cattle samples from Thailand’s northern, western, and southern regions. Our results revealed that the diversity of B. bovis spb-2 gene is genetically diverse, whereas that of B. bigemina rap-1a gene is conserved in Thailand and worldwide. These findings could be used to improve the insight of molecular phylogenetics and diversity among sbp-2 of B. bovis and rap-1a of B. bigemina Thailand strains. Therefore, it is possible that our findings will be useful in development of immunodiagnostic tests and vaccine strategies. However, we still encourage further in vivo validation studies for further prevention and control of bovine babesiosis throughout the country in order to mitigate the infection of cattle vector-borne parasites.
Data availability statement
The datasets presented in this study can be found in online repositories. The names of the repository/repositories and accession number(s) can be found in the article/Supplementary Material.
Ethics statement
All experimental procedures involving animals were approved by the Animal Care and Use Committee (IMBMU-ACUC), Institute of Molecular Biosciences, Mahidol University, Thailand. All suitable international, national and/or institutional guidelines for animal care and use were followed. As well, we have received consent to collect the cattle blood sample at the animal farm.
Author contributions
NS: Conceptualization, Methodology, Investigation, Writing original draft version, Data Curation, Visualization and Project administration. PN: Visualization and Resources. NP: Visualization and Resources. SM: Resources. AW: Resources. WJ: Resources. SS: Resources. RC: Resources. PA: Conceptualization, Investigation, Methodology, Visualization, Data Curation, Project administration, Validation, Writing-Review & Editing, Supervision, Resources and Funding acquisition. All authors contributed to the article and approved the submitted version.
Funding
This research paper is supported by Specific League Funds and Basic Research Fund: fiscal year 2022 from Mahidol University to PA.
Acknowledgments
We are also grateful to Surat Thani artificial insemination and biotechnology center, Surat Thani province, Thailand and Mrs. Pacharaporn Khumpim for their providing some cattle blood samples. Likewise, we would like to thank Prof. Dr. Peter Quantick, Research Director, RedKnight Consultancy Ltd, UK for assistance in correcting the manuscript.
Conflict of interest
The authors declare that the research was conducted in the absence of any commercial or financial relationships that could be construed as a potential conflict of interest.
Publisher’s note
All claims expressed in this article are solely those of the authors and do not necessarily represent those of their affiliated organizations, or those of the publisher, the editors and the reviewers. Any product that may be evaluated in this article, or claim that may be made by its manufacturer, is not guaranteed or endorsed by the publisher.
Supplementary material
The Supplementary Material for this article can be found online at: https://www.frontiersin.org/articles/10.3389/fcimb.2022.1065963/full#supplementary-material
Supplementary Figure 1 | PCR products of B. bovis sbp-2 and B. bigemina rap-1a genes of Thailand strain show a 584 bp fragment of sbp-2 gene (A) from Muang (lane 1-2), Don-Sak (lane 3) and Pa-phayom (lane 4) districts, and a 412 bp fragment of rap-1a gene (B) from Muang (lane 1), Photharam (lane 2), Don-sak (lane 3), Cha-wang (lane 4) and Pa-phayom (lane 5) districts. Lanes N and P indicate negative and positive controls, respectively. The molecular size standard (M) is a 100-bp ladder.
Supplementary Figure 2 | Multiple sequence alignment of the sbp-2 gene sequences among B. bovis different strains (visualized with JalView). The sequences obtained in the present study are shown in boldface latter. The green letter indicates identical consensus. The red rectangle indicates non-consensus.
Supplementary Figure 3 | Multiple sequence alignment of the rap-1a gene sequences among B. bigemina different strains (visualized with JalView). The sequences detected in the present study are exhibited in boldface latter. The green letter represents identical consensus. The red rectangle represents non-consensus.
Supplementary Figure 4 | Alignment of amino acid sequences of B. bovis sbp-2 (A) and B. bigemina rap-1a (B) from Thailand strain (boldface letter) and other strains (visualized with JalView). The identical residues of SBP-2 and RAP-1a are shown in yellow letters. The red rectangle indicates non-conserved residues.
References
AbouLaila, M., Yokoyama, N., Igarashi, I. (2010). Development and evaluation of a nested PCR based on spherical body protein 2 gene for the diagnosis of Babesia bovis infection. Vet. Parasitol. 169, 45–50. doi: 10.1016/j.vetpar.2009.12.013
Ahantarig, A., Trinachartvanit, W., Milne, J. (2008). Tick-borne pathogens and diseases of animals and humans in thailand. Southeast Asian J. Trop. Med. Public Health 39, 1015–1032.
Almeria, S., Castellà, J., Ferrer, D., Ortuño, A., Estrada-Peña, A., Gutiérrez, J. F. (2001). Bovine piroplasms in minorca (Balearic islands, spain): a comparison of PCR-based and light microscopy detection. Vet. Parasitol. 99, 249–259. doi: 10.1016/s0304-4017(01)00464-2
Altay, K., Aydin, F. M., Dumamli, N., Aktas, M. (2008). Molecular detection of Theileria and Babesia infections in cattle. Vet. Parasitol. 158, 295–301. doi: 10.1016/j.vetpar.2008.09.025
Awad, H., Antunes, S., Galindo, R. C., Rosário, V. E., Fuente, J., Domingos, A. (2011). Prevalence and genetic diversity of Babesia and Anaplasma species in cattle in Sudan. Vet. Parasitol. 181, 146–152. doi: 10.1016/j.vetpar.2011.04.007
Bailey, T. L., Boden, M., Buske, F. A., Frith, M., Grant, C. E., Clementi, L., et al. (2009). MEME SUITE: tools for motif discovery and searching. Nucleic Acids Res. 37, W202–W208. doi: 10.1093/nar/gkp335
Bailey, T. L., Elkan, C. (1994). Fitting a mixture model by expectation maximization to discover motifs in biopolymers. Proc. Int. Conf Intell. Syst. Mol. Biol. 2, 28–36.
Benjamin, D. C., Berzofsk, J. A., East, I. J., Gurd, F. R. N., Hannum, C., Leach, S. J., et al. (1984). The antigenic structure of proteins: a reappraisal. Ann. Rev. Immunol. 2, 67–101. doi: 10.1146/annurev.iy.02.040184.000435
Bock, R., Jackson, L., Vos, D. A., Jorgensen, W. (2004). Babesiosis of cattle. Parasitology 129, S247–S269. doi: 10.1017/s0031182004005190
Bono, M. F., Mangold, A. J., Baravalle, M. E., Valentini, B. S., Thompson, C. S., Wilkowsky, S. E., et al. (2008). Efficiency of a recombinant MSA-2c-based ELISA to establish the persistence of antibodies in cattle vaccinated with Babesia bovis. Vet. Parasitol. 157, 203–210. doi: 10.1016/j.vetpar.2008.07.025
Buckley, T. R., Cunningham, C. W. (2002). The effects of nucleotide substitution model assumptions on estimates of nonparametric bootstrap support. Mol. Biol. Evol. 19, 394–405. doi: 10.1093/oxfordjournals.molbev.a004094
Cao, S., Aboge, G. O., Terkawi, M. A., Yu, L., Kamyingkird, K., Luo, Y., et al. (2012). Molecular detection and identification of Babesia bovis and Babesia bigemina in cattle in northern Thailand. Parasitol. Res. 111, 1259–1266. doi: 10.1007/s00436-012-2960-4
Choi, Y., Chan, A. P. (2015). PROVEAN web server: a tool to predict the functional effect of amino acid substitutions and indels. Bioinformatics 31, 2745–2747. doi: 10.1093/bioinformatics/btv195
Clement, M., Snell, Q., Walker, P., Posada, D., Crandall, K. (2002). “TCS: Estimating gene genealogies,” in Proceedings 16th International Parallel and Distributed Processing Symposium, Institute of Electrical and Electronics Engineers (Lauderdale, FL, USA: IEEE Publisher)) Vol. 1, 1–7. doi: 10.1109/IPDPS.2002.1016585
Dijkstra, M., Bawono, P., Abeln, S., Feenstra, A., Fokkink, W., Heringa, J. (2018). Motif-aware PRALINE: Improving the alignment of motif regions. PloS Comput. Biol. 14, e1006547. doi: 10.1371/journal.pcbi.1006547
Gallego-Lopez, G., Lau, A., O'Connor, R. M., Ueti, M. W., Cooke, B. M., Laughery, J. M., et al. (2019). Up-regulated expression of spherical body protein 2 trucated copy 11 in Babesia bovis is associated with reduced cytoadhesion to vascular endothelial cells. Int. J. Parasitol. 49, 127–137. doi: 10.1016/j.ijpara.2018.05.015
Galon, E. M., Moumouni, P. F. A., Ybanez, R. H. D., Ringo, A. E., Efstratiou, A., Lee, S. H., et al. (2019). First molecular detection and characterization of tick-borne pathogens in water buffaloes in bohol, Philippines. Ticks Tick Borne Dis. 10, 815–821. doi: 10.1016/j.ttbdis.2019.03.016
Ganzinelli, S., Rodríguez, A., Schnittger, L., Florin-Christensen, M. (2018). “Babesia in domestic ruminants,” in Parasitic Protozoa of farm animals and pets. Eds. Florin-Christensen, M., Schnittger, L. (Cham: Springer), 215–239.
Goymer, P. (2007). Synonymous mutations break their silence. Nat. Rev. Genet. 8, 92. doi: 10.1038/nrg2056
Guo, J., Li, M., Sun, Y., Yu, L., He, P., Nie, Z., et al. (2018). Characterization of a novel secretory spherical body protein in Babesia orientalis and Babesia orientalis-infected erythrocytes. Parasit Vectors. 11, 433. doi: 10.1186/s13071-018-3018-y
Guswanto, A., Allamanda, P., Mariamah, E. S., Munkjargal, T., Tuvshintulga, B., Takemae, H., et al. (2017). Evaluation of immunochromatographic test (ICT) strips for the serological detection of Babesia bovis and Babesia bigemina infection in cattle from Western Java, Indonesia. Vet. Parasitol. 239, 76–79. doi: 10.1016/j.vetpar.2017.04.010
Hall, T. A. (1999). BioEdit: A user-friendly biological sequence alignment editor and analysis program for windows 95/98/NT. Nucl. Acids Symp. Ser. 41, 95–98.
Herrera, P. C. T., Viloria, V. V., Balbin, M. M., Mingala, C. N. (2017). Prevalence of babesiosis (Babesia bovis and Babesia bigemina) in cattle and water buffalo in nueva ecija, Philippines using nested polymerase chain reaction. Ann. Parasitol. 63, 309–316. doi: 10.17420/ap6304.117
Hidalgo-Ruiz, M., Mejia-López, S., Pérez-Serrano, R. M., Zaldívar-Lelo de Larrea, G., Ganzinelli, S., Florin-Christensen, M., et al. (2022). Babesia bovis AMA-1, MSA-2c and RAP-1 contain conserved b and T-cell epitopes, which generate neutralizing antibodies and a long-lasting Th1 immune response in vaccinated cattle. Vaccine 40, 1108–1115. doi: 10.1016/j.vaccine.2022.01.023
Homer, M. J., Irma, A. D., Sam, R. T., Krause, P. J., Persing, D. H. (2000). Babesiosis. Clin. Microbiol. Rev. 13, 451–469. doi: 10.1128/CMR.13.3.451
Hurtado, O. J. B., Giraldo-Ríos, C. (2018). “Economic and health impact of the ticks in production animals,” in Ticks and tick-borne pathogens. Eds. Abubakar, M., Perera, P. K. (London, United Kingdom: IntechOpen Inc.), 1–19. doi: 10.5772/intechopen.81167
Ibrahim, H. M., Moumouni, P. F. A., Mohammed-Geba, K., Sheir, S. K., Hashem, I. S. Y., Cao, S., et al. (2013). Molecular and serological prevalence of Babesia bigemina and Babesia bovis in cattle and water buffalos under small-scale dairy farming in beheira and faiyum provinces, Egypt. Vet. Parasitol. 198, 187–192. doi: 10.1016/j.vetpar.2013.08.028
Iseki, H., Zhou, L., Kim, C., Inpankaew, T., Sununta, C., Yokoyama, N., et al. (2010). Seroprevalence of Babesia infections of dairy cows in northern Thailand. Vet. Parasitol. 170, 193–196. doi: 10.1016/j.vetpar.2010.02.038
Jirapattharasate, C., Moumouni, P. F. A., Cao, S., Iguchi, A., Liu, M., Wang, G., et al. (2016). Molecular epidemiology of bovine babesia spp. and Theileria orientalis parasites in beef cattle from northern and northeastern Thailand. Parasitol. Int. 65, 62–69. doi: 10.1016/j.parint.2015.10.005
Jirapattharasate, C., Moumouni, P. F. A., Cao, S., Iguchi, A., Liu, M., Wang, G., et al. (2017). Molecular detection and genetic diversity of bovine babesia spp., Theileria orientalis, and Anaplasma marginale in beef cattle in Thailand. Parasitol. Res. 116, 751–762. doi: 10.1007/s00436-016-5345-2
Junsiri, W., Watthanadirek, A., Poolsawat, N., Kaewmongkol, S., Jittapalaponga, S., Chawengkirttikul, R., et al. (2020). Molecular detection and genetic diversity of Anaplasma marginale based on the major surface protein genes in Thailand. Acta Trop. 205, 105338. doi: 10.1016/j.actatropica.2020.105338
Kemp, L. E., Yamamoto, M., Soldati-Favre, D. (2013). Subversion of host cellular functions by the apicomplexan parasites. Microbiol. Rev. 37, 607–631. doi: 10.1111/1574-6976.12013
Kimura, M. (1980). A simple method for estimating evolutionary rate of base substitutions through comparative studies of nucleotide sequences. J. Mol. Evol. 16, 111–120. doi: 10.1007/BF01731581
Kolaskar, A. S., Tongaonkar, P. C. (1990). A semi-empirical method for prediction of antigenic determinants on protein antigens. FEBS Lett. 276, 172–174. doi: 10.1016/0014-5793(90)80535-q
Kumar, B., Mondal, D. B., Jithin, M. V. (2018). Prevalence of babesiosis in cattle in Patna region, India. Int. J. Curr. Microbiol. App Sci. 7, 5167–5174.
Kumar, S., Stecher, G., Tamura, K. (2016). MEGA7: Molecular evolutionary genetics analysis version 7.0 for bigger datasets. Mol. Biol. Evol. 33, 1870–1874. doi: 10.1093/molbev/msw054
Lau, A. O. T., Cereceres, K., Palmer, G. H., Fretwell, D. L., Pedroni, M. J., Mosqueda, J., et al. (2010). Genotypic diversity of merozoite surface antigen 1 of Babesia bovis within an endemic population. Mol. Biochem. Parasitol. 172, 107–112. doi: 10.1016/j.molbiopara.2010.03.017
Leigh, J. W., Bryant, D. (2015). POPART: full-feature software for haplotype network construction. Methods Ecol. Evol. 6, 1110–1116. doi: 10.1111/2041-210X.12410
Li, Y., Luo, Y., Cao, S., Terkawi, M. A., Lan, D. T. B., Long, P. T., et al. (2014). Molecular and seroepidemiological survey of Babesia bovis and Babesia bigemina infections in cattle and water buffaloes in the central region of Vietnam. Trop. Biomed. 33, 406–413.
Lira-Amaya, J. J., Martines-Garcia, G., Santamaria-Espinosa, R. M., Castaneda-Arriola, R. O., Ojeda-Carrasco, J. J., Avila-Ramirez, G., et al. (2021). Comparative study of indirect fluorescent antibody, ELISA, and immunochromatography tests for serological diagnosis of bovine babesiosis caused by Babesia bovis. Animals 11, 3358. doi: 10.3390/ani11123358
Lobo, C. A., Rodriguez, M., Cursino-Santos, J. R. (2012). Babesia and red cell invasion. Curr. Opin. Hematol. 19, 170–175. doi: 10.1097/MOH.0b013e328352245a
Marques, R., Krüger, R. F., Peterson, A. T., de Melo, L. F., Vicenzi, N., Jiménez−García, D. (2020). Climate change implications for the distribution of the babesiosis and anaplasmosis tick vector, Rhipicephalus (Boophilus) microplus. Vet. Res. 51, 81. doi: 10.1186/s13567-020-00802-z
Mendes, N. S., Ramos, I. A. S., Herrera, H. M., Campos, J. B. V., Alves, J. V. A., Macedo, G. C., et al. (2019). Genetic diversity of Babesia bovis in beef cattle in a large wetland in Brazil. Parasitol. Res. 118, 2027–2040. doi: 10.1007/s00436-019-06337-3
Mosqueda, L., Olvera-Ramírez, A., Aguilar-Tipacamú, G., Cantó, G. J. (2012). Current advances in detection and treatment of babesiosis. Curr. Med. Chem. 19, 1504–1518. doi: 10.2174/092986712799828355
Moumouni, P. F. A., Aboge, G. O., Terkawi, M. A., Masatani, T., Cao, S., Kamyingkird, K., et al. (2015). Molecular detection and characterization of Babesia bovis, Babesia bigemina, Theileria species and Anaplasma marginale isolated from cattle in Kenya. Parasit Vectors. 8, 496. doi: 10.1186/s13071-015-1106-9
Nagano, D., Sivakumar, T., Maceso, A. C. C., Inpankaew, T., Alhassan, A., Igarashi, I., et al. (2013). The genetic diversity of merozoite surface antigen 1 (MSA-1) among Babesia bovis detected from cattle populations in Thailand, Brazil and Ghana. J. Vet. Med. Sci. 75, 1463–1470. doi: 10.1292/jvms.13-0251
Niu, Q., Liu, Z., Yu, P., Yang, J., Abdallah, M. O., Guan, G., et al. (2015). Genetic characterization and molecular survey of Babesia bovis, Babesia bigemina and Babesia ovata in cattle, dairy cattle and yaks in China. Parasites Vectors. 8, 518. doi: 10.1186/s13071-015-1110-0
Nystrom, S. L., McKay, D. J. (2021). Memes: a motif analysis environment in r using tools from the MEME suite. PloS Comput. Biol. 17 (9), e1008991. doi: 10.1371/journal.pcbi.1008991
Petrigh, R., Ruybal, P., Thompson, C., Neumann, R., Moretta, R., Wilkowsky, S., et al. (2008). Improved molecular tools for detection of Babesia bigemina. Ann. N Y Acad. Sci. 1149, 155–157. doi: 10.1196/annals.1428.038
Pham, T. D. (2010). GeoEntropy: A measure of complexity and similarity. Pattern Recognit. 43, 887–896. doi: 10.1016/j.patcog.2009.08.015
Rittipornlertrak, A., Nambooppha, B., Simking, P., Punyapornwithaya, V., Tiwananthagorn, S., Jittapalapong, S., et al. (2017). Low levels of genetic diversity associated with evidence of negative selection on the Babesia bovis apical membrane antigen 1 from parasite populations in Thailand. Infect. Genet. Evol. 54, 447–454. doi: 10.1016/j.meegid.2017.08.009
Rozas, J., Ferrer-Mata, A., S´anchez-DelBarrio, J. C., Guirao-Rico, S., Librado, P., Ramos-Onsins, S. E., et al. (2017). DnaSP 6: DNA sequence polymorphism analysis of large data sets. Mol. Biol. Evol. 34, 3299–3302. doi: 10.1093/molbev/msx248
Ruef, B. J., Dowling, S. C., Conley, P. G., Perryman, L. E., Brown, W. C., Jasmer, D. P., et al. (2000). A unique Babesia bovis spherical body protein is conserved among geographic isolates and localizes to the infected erythrocyte membrane. Mol. Biochem. Parasitol. 105, 1–12. doi: 10.1016/s0166-6851(99)00167-x
Sayed, E. M., Elshahawy, I. (2018). The current prevalence of bovine babesiosis and theileriosis infection in Egypt. J. Bacteriol Mycol. 6, 306–311. doi: 10.15406/jbmoa.2018.06.00224
Silva, M. G., Henriques, G., Sanchez, C., Marques, P. X., Suarez, C. E., Oliva, A. (2009). First survey for Babesia bovis and Babesia bigemina infection in cattle from central and southern regions of Portugal using serological and DNA detection methods. Vet. Parasitol. 166, 66–72. doi: 10.1016/j.vetpar.2009.07.031
Simking, P., Yatbantoong, N., Saetiew, N., Saengow, S., Yodsri, W., Chaiyarat, R., et al. (2014). Prevalence and risk factors of Babesia infections in cattle trespassing natural forest areas in salakpra wildlife sanctuary, kanchanaburi province. J. Trop. Med. Parasitol. 37, 10–19.
Singh, H., Mishra, A. K., Rao, J. R., Tewari, A. K. (2009). Comparison of indirect fluorescent antibody test (IFAT) and slide enzyme linked immunosorbent assay (SELISA) for diagnosis of Babesia bigemina infection in bovines. Trop. Anim. Health Prod. 41, 153–159. doi: 10.1007/s11250-008-9170-1
Sivakumar, T., Lan, D. T. B., Long, P. T., Viet, L. Q., Weerasooriya, G., Kume, A., et al. (2018). Serological and molecular surveys of babesia bovis and Babesia bigemina among native cattle and cattle imported from Thailand in hue, Vietnam. J. Vet. Med. Sci. 80, 333–336. doi: 10.1292/jvms.17-0549
Smeenk, I., Kelly, P. J., Wray, K., Musuka, G., Trees, A. J., Jongejan, F. (2000). Babesia bovis and B. bigemina DNA detected in cattle and ticks from Zimbabwe by polymerase chain reaction. J. S Afr Vet. Assoc. 71, 21–24. doi: 10.4102/jsava.v71i1.671
Soltis, P. S., Soltis, D. E. (2003). Applying the bootstrap in phylogeny reconstruction. Stat. Sci. 18, 256–267. doi: 10.1214/ss/1063994980
Suarez, C. E., Florin-Christensen, M., Hines, S. A., Palmer, G. H., Brown, W. C., McElwain, T. F. (2000). Characterization of allelic variation in the Babesia bovis merozoite surface antigen 1 (MSA-1) locus and identification of a cross-reactive inhibition-sensitive MSA-1 epitope. Infect. Immun. 68, 6865–6870. doi: 10.1128/IAI.68.12.6865-6870.2000
Tamura, K., Nei, M. (1993). Estimation of the number of nucleotide substitutions in the control region of mitochondrial DNA in humans and chimpanzees. Mol. Biol. Evol. 103, 512–526. doi: 10.1093/oxfordjournals.molbev.a040023
Tattiyapong, M., Sivakumar, T., Takemae, H., Simking, P., Jittapalapong, S., Igarashi, I. (2016). Genetic diversity and antigenicity variation of Babesia bovis merozoite surface antigen-1 (MSA-1) in Thailand. Infect. Genet. Evol. 41, 255–261. doi: 10.1016/j.meegid.2016.04.021
Terkawi, M. A., Huyen, N. X., Shinuo, C., Inpankaew, T., Maklon, K., AbouLaila, M., et al. (2011a). Molecular and serological prevalence of Babesia bovis and Babesia bigemina in water buffaloes in the northeast region of Thailand. Vet. Parasitol. 178, 201–207. doi: 10.1016/j.vetpar.2011.01.041
Terkawi, M. A., Seuseu, F. J., Eko-Wibowo, P., Huyen, N. X., Minoda, Y., AbouLaila, M., et al. (2011b). Secretion of a new spherical body protein of Babesia bovis into the cytoplasm of infected erythrocytes. Mol. Biochem. Parasitol. 178, 40–45. doi: 10.1016/j.molbiopara.2011.02.006
Uilenberg, G. (2006). Babesia-a historical overview. Vet. Parasitol. 138, 3–10. doi: 10.1016/j.vetpar.2006.01.035
Vita, R., Mahajan, S., Overton, J. A., Dhanda, S. K., Martini, S., Cantrell, J. R., et al. (2019). The immune epitope database (IEDB): 2018 update. Nucleic Acids Res. 47, D339–D343. doi: 10.1093/nar/gky1006
Wagner, G. G., Holman, P., Waghela, S. (2002). Babesiosis and heartwater: threats without boundaries. Vet. Clin. Food Anim. 18, 417–430. doi: 10.1016/s0749-0720(02)00027-0
Watthanadirek, A., Junsiri, W., Minsakorn, S., Poolsawat, N., Srionrod, N., Khumpim, P., et al. (2021). Molecular and recombinant characterization of major surface protein 5 from Anaplasma marginale. Acta Trop. 220, 105933. doi: 10.1016/j.actatropica.2021.105933
Yokoyama, N., Okamura, M., Igarashi, I. (2006). Erythrocyte invasion by Babesia parasites: current advances in the elucidation of the molecular interactions between the protozoan ligands and host receptors in the invasion stage. Vet. Parasitol. 138, 22–32. doi: 10.1016/j.vetpar.2006.01.037
Yusuf, J. J. (2017). Review on bovine babesiosis and its economical importance. J. Vet. Med. Res. 4, 1090.
Zhou, M., Cao, S., Sevinc, F., Sevinc, M., Ceylan, O., Moumouni, P. F. A., et al. (2016). Molecular detection and genetic identification of Babesia bigemina, Theileria annulata, Theileria orientalis and Anaplasma marginale in Turkey. Ticks Tick Borne Dis. 7, 126–134. doi: 10.1016/j.ttbdis.2015.09.008
Keywords: Babesia bovis, Babesia bigemina, Babesiosis, nested PCR, genetic diversity, cattle, Thailand
Citation: Srionrod N, Nooroong P, Poolsawat N, Minsakorn S, Watthanadirek A, Junsiri W, Sangchuai S, Chawengkirttikul R and Anuracpreeda P (2022) Molecular characterization and genetic diversity of Babesia bovis and Babesia bigemina of cattle in Thailand. Front. Cell. Infect. Microbiol. 12:1065963. doi: 10.3389/fcimb.2022.1065963
Received: 10 October 2022; Accepted: 14 November 2022;
Published: 29 November 2022.
Edited by:
Ehsan Ahmadpour, Tabriz University of Medical Sciences, IranReviewed by:
Shimaa El-Sayed, Mansoura University, EgyptMartina Paoletta, Instituto Nacional de Tecnología Agropecuaria, Argentina
Copyright © 2022 Srionrod, Nooroong, Poolsawat, Minsakorn, Watthanadirek, Junsiri, Sangchuai, Chawengkirttikul and Anuracpreeda. This is an open-access article distributed under the terms of the Creative Commons Attribution License (CC BY). The use, distribution or reproduction in other forums is permitted, provided the original author(s) and the copyright owner(s) are credited and that the original publication in this journal is cited, in accordance with accepted academic practice. No use, distribution or reproduction is permitted which does not comply with these terms.
*Correspondence: Panat Anuracpreeda, cGFuYXQuYW51QG1haGlkb2wuYWMudGg=; cGFuYXQuYW51cmFjcHJlZWRhQGdtYWlsLmNvbQ==