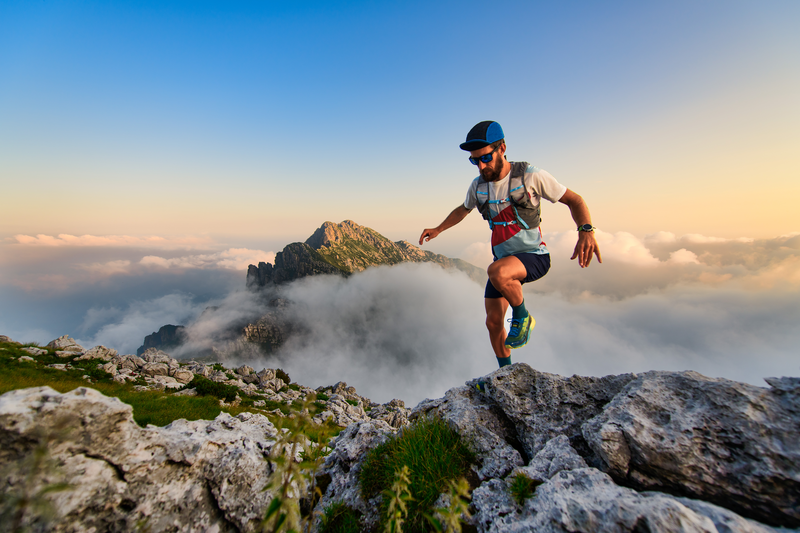
94% of researchers rate our articles as excellent or good
Learn more about the work of our research integrity team to safeguard the quality of each article we publish.
Find out more
REVIEW article
Front. Cell. Infect. Microbiol. , 27 December 2022
Sec. Molecular Bacterial Pathogenesis
Volume 12 - 2022 | https://doi.org/10.3389/fcimb.2022.1060810
This article is part of the Research Topic Reviews in Molecular Bacterial Pathogenesis and Host Immunity-Pathogens Interactions View all 6 articles
Despite meritorious attempts, a S. aureus vaccine that prevents infection or mitigates severity has not yet achieved efficacy endpoints in prospective, randomized clinical trials. This experience underscores the complexity of host-S. aureus interactions, which appear to be greater than many other bacterial pathogens against which successful vaccines have been developed. It is increasingly evident that S. aureus employs strategic countermeasures to evade or exploit human immune responses. From entering host cells to persist in stealthy intracellular reservoirs, to sensing the environmental milieu and leveraging bacterial or host metabolic products to reprogram host immune responses, S. aureus poses considerable challenges for the development of effective vaccines. The fact that this pathogen causes distinct types of infections and can undergo transient genetic, transcriptional or metabolic adaptations in vivo that do not occur in vitro compounds challenges in vaccine development. Notably, the metabolic versatility of both bacterial and host immune cells as they compete for available substrates within specific tissues inevitably impacts the variable repertoire of gene products that may or may not be vaccine antigens. In this respect, S. aureus has chameleon phenotypes that have alluded vaccine strategies thus far. Nonetheless, a number of recent studies have also revealed important new insights into pathogenesis vulnerabilities of S. aureus. A more detailed understanding of host protective immune defenses versus S. aureus adaptive immune evasion mechanisms may offer breakthroughs in the development of effective vaccines, but at present this goal remains a very high bar. Coupled with the recent advances in human genetics and epigenetics, newer vaccine technologies may enable such a goal. If so, future vaccines that protect against or mitigate the severity of S. aureus infections are likely to emerge at the intersection of precision and personalized medicine. For now, the development of S. aureus vaccines or alternative therapies that reduce mortality and morbidity must continue to be pursued.
This review of the human immune response to Staphylococcus aureus covers large amounts of new information that have become available since our last relatively recent review (Miller et al., 2020). In the previous review, evidence for increased numbers of human infections was linked to defects in innate immunity of keratinocytes, mucosal epithelial cells, and phagocytes (including neutrophils, monocytes/macrophages and dendritic cells). These genetic defects emphasized the role of these cells in preventing S. aureus infections. Also considered is how staphylococcal exotoxins (e.g. superantigens and pore-forming toxins) impair immune functions by lysing or dysregulating innate cells, further highlighting the crucial role of innate immunity in staphylococcal defense. Toxin-neutralizing antibodies have been hypothesized to improve outcomes in invasive S. aureus infections by mitigating such virulence and promoting immune function. This concept derived in-part from considerations of the cytokine response to invasive infections, especially in S. aureus bacteremia (SAB), and is further explored in the current review.
Most previous vaccine trials have been aimed at improving opsonophagocytic activity or promoting Th17 mediated adaptive immunity. New insights discussed herein provide perspectives on the challenges of these approaches. Moreover, previous vaccine trials did not fully appreciate the complexity of S. aureus-host immune interactions, thereby contributing to their limited efficacy. The lack of clear determinants of protective immunity against S. aureus infections, and direct or surrogate biomarkers thereof, also greatly present challenges to successful vaccine development vs. this pathogen.
This review examines a substantial body of recent data on human genetic variation and the crucial role of host-bacterial metabolic crosstalk in contributing to outcomes of S. aureus infections. The new information encompasses several areas, including: 1) the adaptive evolution of S. aureus within the host; 2) how S. aureus evades immune efficacy within the host; 3) how human genetic variability can be linked to outcomes of infections; 4) how the interplay between host and pathogen metabolism shapes the immune response to intracellular S. aureus, including the evolution of S. aureus metabolic genes over time within the host; 5) how vitamins enhance the innate immune response to S. aureus; and 6) how anti-toxin antibody levels and anti-glucosaminidase levels may be biomarkers for outcomes of S. aureus infections.
S. aureus has co-evolved with mammalian hosts for millennia, which has produced multiple mechanisms for evasion of the host immune response. Indeed, rates of invasive infections and deaths from S. aureus are similar if not higher compared to other severe respiratory pathogens such as N. meningitidis, H. influenzae, and S. pneumoniae for which vaccines have been developed (Proctor, 2021; Collaborators, 2022). [Incidence of invasive infections/100,000 human cases/year: S. pneumoniae = 15; H. influenzae = 23; S. aureus = 32]. Further complicating vaccine development is the kinetic relationship of in vivo host-staphylococcal interactions (discussed below). Moreover, over forty immune evasion molecules produced by S. aureus that disarm the immune system (e.g., complement inactivation, antibody subversion, TLR2 inhibition) have been described (de Jong et al., 2019), and these will not be covered in this review. Rather, this review will focus on key concepts regarding the evolution of S. aureus within the host.
During nasal colonization, S. aureus strains that caused invasive infections evolve within the host. Such genotypic variation is termed adaptive mutation. In a study by Young et al. (2017), genomes from 1163 strains were examined from 105 patients wherein 72% of the infections emerged from the nasal colonizing strain. There was a 2.8 to 3.6-fold enrichment of adaptive mutations in genes including rsp and agr. These gene products regulate the expression of surface proteins and toxins, which stimulate host-derived anti-microbial peptides. The adaptive mutations in pathogenesis-associated genes occurred in the strains that became invasive (3.1-fold enriched) as compared to those that only resided in the nose. Young et al. noted that “whole-genome sequencing case studies add weight to the idea that within-host evolution plays an important role in infection”. Enrichment of adaptive mutations was not observed in asymptomatic carriers, nor within unrelated bacteria.” This relationship indicates that some patients have selection pressures that place them at risk of invasive disease due to strains of S. aureus that may be uniquely advantaged in a given human host. In another study, when colonizing strains were compared to standard laboratory strains of S. aureus, colonizing strains showed a delay in inducing tlr2 expression in cultured human nasal epithelial cells (NECs) (Quinn and Cole, 2007). Moreover, human β-defensin-2 and human β-defensin-3 were negligibly induced in NECs by nasal carrier strain in comparison to the non-carrier strain of S. aureus. These data suggest that carrier strains are able to suppress the normal TLR-2 pathway, thereby reducing innate immune responses and imposing risks of more serious invasive disease as well as decreasing the barrier immunity given by cationic antimicrobial peptides. Finally, 15 SAB MRSA isolates from a single patient with persistent bacteremia were fully sequenced and shown to harbor a total of 37 sequence polymorphisms (Gao et al., 2015). This study identified how organisms evolved during treatment. Of note, the chromosome expanded to include a 20 kb tandem repeats encompassing mprF and parC, as well as transient mutations in genes such as relA and rplC, which can play a role in resistance to endogenous host defense peptides and peptide-based antibiotics and contribute to small colony variant (SCV) formation. A more recent study by Giulieri et al. (2022) examined the evolution of 2590 genomes in 396 S. aureus strains during infection in vivo. Results demonstrated a distinctive evolutionary pattern within the invasive S. aureus populations (early and late adapted) compared to those colonizing the nares. Late infection-adapted strains were enriched for variants predicted to interfere with intergenic regulatory regions through point mutations or insertions. However, several genes with the most significant enrichment (agrA, agrC, stp1, and sucA) were recurrently mutated across multiple host in vivo evolutionary scenarios, implying a global role in S. aureus adaptation during colonization and invasion. The mutations in succinate metabolism are consistent with adaptations seen in persistence (discussed below). Adaptation within the invasive population was strongly driven by antibiotics, e.g., enhanced MprF production following daptomycin pressure. This is a small sampling of the genes identified and the reader is referred to this paper. Together, understanding how these factors promote colonization vs. infection will allow novel strategies for vaccines or immunotherapeutics to prevent invasion or infection.
S. aureus employs many methods to adapt to the host environment including adaptive mutations in essential genes involved in pathogenesis and covert chromosomal rearrangements that promote invasive and/or persistent infections. S. aureus has surface proteins that bind fibronectin: fibronectin binding protein A (FnbpA) and FnbpB. Early studies correlated the numbers of fibronectin receptors expressed on S. aureus with overall invasive disease (Proctor et al., 1984). In these studies, 22 isolates from patients with invasive infections were compared to 19 isolates in patients with non-invasive infections. The differences were highly significant (p < 0.00025). Moreover, fibronectin was shown to enhance uptake of S. aureus by cultured endothelial cells (Hamill et al., 1986). Further studies refined these concepts. Two polymorphisms in S. aureus FnbpA were identified that promoted pathogenesis. One polymorphism increased adhesion (VDREED) to host fibronectin (Lower et al., 2011), which resulted in enhanced binding to endovascular devices, while another polymorphism (GIDRVED) enhanced uptake into host cells (Xiong et al., 2015). Isolates that had higher binding affinities were more likely to cause device-related infections in patients (Lower et al., 2011; Hos et al., 2015). Additional work examined five strains isolated from patients with persistent S. aureus bacteremia versus five strains with resolving S. aureus bacteremia (SAB) (Xiong et al., 2015). Isolates with increased affinity for host fibronectin were better able to initiate endovascular infections whereas other variants showed reduced affinity but increased invasion of host cells. Thus, binding to fibronectin does not always mean more intracellular uptake, as it is cell-type specific (Niemann et al., 2021). For endothelial cells, this appears to be true, but for osteoblasts large amounts of fibronectin can reduce uptake, via formation of fibrillar networks that inhibit uptake.
Additionally, staphylococcal adaptation for persistence also includes phenotype switching mechanisms that occur as a result of chromosomal rearrangements without any apparent genetic mutations (Guérillot et al., 2019). The inversion that was described involved half of the chromosome. This inversion occurred via recombination between methylase genes, and the activation of a conserved prophage harboring the Immune Evasion Cluster (IEC). IEC contains three genes (chi, scn, sak), which promote evasion of neutrophils and has been associated with persistent infections. Moreover, this inversion led to slow growth, enhanced host cell invasion, low hemolysin and pigment production, and reduced agr expression, phenotypes which are typical of SCVs. Cui et al. (2012) have also identified large symmetrical inversion (1.26 Mb) impacting two inverted copies of the S. aureus pathogenicity island (SaPl) that also resulted in the SCV phenotype, but this inversion does not appear as often as the one described by Guérillot et al. (2019). Thus, there is increasing recognition of S. aureus strain-specific characteristics that temporospatially exploit host cells or tissues that may differ considerably from human to human.
Colonized humans seem to have better outcomes in invasive infection than those who are not colonized (Wertheim et al., 2004). Nevertheless, the fact that the large majority of invasive infections are caused by the strain of S. aureus colonizing the nares and can evolve over time supports a need to revise conventional thinking on “commensalism” (Proctor, 2021). S. aureus is increasingly being referred to as a human pathobiont, especially given its ability to undergo adaptive mutations in response to host-mediated stresses and antibiotic exposure. S. aureus strains undergoing adaptive changes within the nose may make them more likely to invade the host, cause more severe disease and result in persistent infections. Hence, nasal colonization has serious impacts which imply a non-commensal interplay between the bacterium and the host.
Previously, we had reviewed data suggesting that staphylococcal toxins may suppress the immune response, especially in the context of the T cell—phagocyte axis (Miller et al., 2020). This concept has been further explored wherein each step in the interactions between antigen-presenting cells and T cells can be impacted by toxins (Teymournejad and Montgomery, 2021). Examples of toxin interference include LukAB killing of dendritic cells (Berends et al., 2019), LukED killing of dendritic and T cells when binding to CCR5 (Alonzo et al., 2013), alpha-toxin (α-hemolysin or Hla) inducing naïve T cell apoptosis (Nygaard et al., 2012), and TSST-1 interfering with differentiation of naïve T cells is given as a single example of the many examples of enterotoxins and superantigens altering T cell development. Or course, there is also the broader dysregulation of cytokine response caused by these toxins (Kametani et al., 2008). Strong evidence for these events impacting host responses to S. aureus has been presented in a prospective study of 76 children (31 had invasive infections and 45 non-invasive infections) wherein T cell and antibody responses were measured over time (Li et al., 2022). One specific rationale behind this study was the observation that S. aureus infections recurred in 50% of neonates within 12 months of birth. Major findings included globally impaired T-cell function in infected babies, with 5- to 12-fold reduction in IL-17A and IFNγ in response to S. aureus, while anti-LukE, -LukS-PV (but not Hla) increased following infections in those less than 5 years old. This raises questions regarding whether children with suppressed T-cells get S. aureus infections, or if S. aureus toxins suppress T-cells, or both?
Further evidence for S. aureus impairment of T cell-mediated immunity was provided by Sanchez et al. (Sanchez et al., 2017), in which humans failed to develop protective immunity after S. aureus bacteremia (SAB). The investigators proposed that the mechanism underlying this result was O-acetylation of peptidoglycan (PG), limiting Th17 response via pattern recognition receptors (PRRs). If so, it would follow that such immune subversion strategies would be strain-dependent. However, there are numerous other mechanisms by which S. aureus may subvert or deviate immune responses that would otherwise be protective against recurrent infection.
Recent evidence suggests that skin-resident T cells are involved in host defense against S. aureus skin infections (Clegg et al., 2021). For instance, healthy human skin is typically replete with S. aureus-specific tissue-resident memory CD4+ T cells that produce IL-17A, IL-22, IFNγ, and TNFα; in response to heat-killed S. aureus (Hendriks et al., 2021). Such responses indicate that adaptive immunity does develop against S. aureus in the skin.
However, whether these responses are protective in the presence of S. aureus toxins or can be targeted by vaccines for durable protection against recurrent infections warrants further investigation.
While T cell immunity has been emphasized as important for protection against invasive S. aureus infections (Armentrout et al., 2020), mispolarized, profusive or protracted T cell responses have also been implicated in adverse responses (Proctor, 2012). For example, the V710 vaccine (recombinant IsdB) stimulated protection via Th17 pathways that were implicated in adverse outcomes in clinical trials (Joshi et al., 2012). The data monitoring committee stopped the V710 vaccine trial because of such hyperimmune responses in subjects who had become infected with invasive S. aureus infections. A follow-up study to examine possible explanations for these results showed that patients who died after receiving V710 vaccine had constitutively low IL-2 levels before vaccination. Clearly, the vaccine disclosed this immune dysregulation correlate, substantiating the broader hypothesis that a protective but restrained immune response is necessary for vaccine safety and efficacy. Because IL-2 is needed for regulatory T cell (Treg) development, it is conceivable that patients with low IL-2 levels were deficient for generation of Treg cells that control systemic inflammatory response. As with most S. aureus immune responses, the Treg response is complex. Hence, more work is needed to sort out their exact role in patients with low IL-2 (Gaborit et al., 2020; Gao et al., 2022). Similarly, IL-17-producing T cells that are associated with hyperactive and pathogenic immune responses are induced in the presence of commensals such as staphylococcal species specifically upon the use of immune checkpoint inhibitors (Hu et al., 2022). This raised concerns over whether all commensal-specific immune responses are pathogenic in the context of anti-tumor therapy and how cancer patients would fare on Th17 stimulating vaccines. An additional observation comes from the V710 clinical trial. Infections in the vein graft donor site were significantly reduced in those subjects receiving the vaccine (Fowler et al., 2013). It is known that Th17 plays a very important role in skin infections (O'Brien and McLoughlin, 2019), hence this suggests that vaccination outcomes may be very location dependent. This concept has been termed contextual immunity and represents distinct temporospatial signatures of molecular and cellular response to infection (Yeaman et al., 2014; Chan et al., 2017). Thus, while T cell immunity is likely critical in protective efficacy, a balance between T cell subsets and their polarization paradigms that is optimal for protective immunity and minimizes off-target effects is necessary for safe and effective vaccines (Proctor, 2012; Yeaman and Hennessey, 2017).
Beyond the above observations, new information continues to emerge regarding the ways through which S. aureus appears to subvert the human immune system. For example, Uebele et al. (Uebele et al., 2020) reported that S. aureus protein A induces Treg numbers through interactions with antigen presenting cells. Askarian et al. (Askarian et al., 2018) reviewed the mechanisms through which S. aureus modulates innate immune responses via toll-like (TLR), NOD-like (NLR) and C-type lectin (CLR) receptor interactions. These are pattern recognition receptors that bind S. aureus lipoproteins and peptidoglycan, which results in transcriptional upregulation of inflammatory pathways in macrophages. This interaction provides an early activation system of innate immunity that results in bacterial clearance. However, S. aureus has developed ways to evade these systems. For example, S. aureus deficient in lipoproteins fail to activate TLR2 pathways. Moreover, S. aureus is able to inhibit heterodimer formation, use structural mimicry of the TIR domain to prevent activation of TLR2, and activate inhibitory receptor pathways [reviewed in (Askarian et al., 2018)]. Similarly, 12% of S. aureus produce a protein, TirS, which down-regulates NF-κB pathways that are critical for production of pro-inflammatory cytokines via NOD2 (Askarian et al., 2018). Staphylococcal evasion factors for CLR recognition have not been described to date.
Recent reports from Medie et al. (2019) and Chang et al. (2021) provide compelling evidence that poor outcomes in vancomycin treatment of S. aureus bacteremia (SAB; e.g. severity, persistence, etc.) are associated with host epigenetic signatures driving immune polarization. Interestingly, anti-IsdB antibodies serve as a negative biomarker as they correlate with poor outcome (IsdB is also highly immunogenic). Hence, it is conceivable or even probable that S. aureus may induce antibody subclass switching for evasion or exploitation of immune responses. Collectively, such findings suggest that prior experience (colonization, infection, invasion) with S. aureus may shape subsequent immune memory and protective efficacy. However, an outstanding question relating to these findings focuses on how S. aureus has evolved to attenuate or exploit host immune responses to its advantage.
S. aureus is well known for deploying an arsenal of immune evasion strategies including the formation of biofilms and SCVs. SCVs have been extensively reviewed (Proctor et al., 2014; Ricciardi et al., 2018; Masters et al., 2019; Tuchscherr et al., 2020) and will not be covered in detail in this review. S. aureus biofilms are particularly difficult to eradicate in light of their ability to limit antibiotic diffusion and immune cell penetration to bacterial cells. Biofilm evasion of the immune system occurs in three steps. First, bacteria attach to host cell surface via microbial surface components recognizing adhesive matrix molecules (MSCRAMMs; e.g. FnbpA/B and clumping factor A/B (ClfA/B)). Moreover, S. aureus FnbpB facilitates evasion of neutrophil extracellular traps (NETs) by neutralizing the bactericidal activity of histones. Next, staphylococci form an extracellular matrix consisting of polysaccharides, proteins and extracellular DNA. This three-dimensional structure also blocks the activity of NETs on growing bacteria contained within the biofilm. Finally, the biofilm bacterial population produce planktonic cells that disseminate and facilitate new foci of infection within the host (Schilcher and Horswill, 2020; Speziale and Pietrocola, 2021).
In addition to biofilm formation, S. aureus can also evade the host through metabolic changes, resulting in SCV formation. S. aureus SCVs are phenotypically characterized by slow growth, pinpoint colony size as well as reduced pigmentation, hemolysis and virulence factor expression. These cells develop within biofilms and intracellularly in host cells (von Eiff et al., 2001; Tuchscherr et al., 2020). Due to their slow growing and resistant nature, SCVs are a source of bacterial persistent infection despite administration of gold standard therapy.
Some of the basic beliefs about S. aureus were that its shape was fixed and that it was non-motile. However, recent studies show that S. aureus is able to change its shape to invade and persist within the osteocyte lacuno-canalicular network (OLCN) of cortical bone during osteomyelitis (Masters et al., 2020). S. aureus invasion of the OLCN is mediated by a combination of penicillin-binding protein 4 (PBP4), a non-essential cell wall transpeptidase involved in the final stages of cell wall synthesis, plus the major autolysin, Atl. Transmission electron microscopy (TEM) revealed that S. aureus is capable of deforming, invading and colonizing the submicron sized networks of canaliculi, connecting the lacunar spaces of osteocytes within cortical bone (de Mesy Bentley et al., 2017; de Mesy Bentley et al., 2018). The OLCN is too narrow to allow neutrophil entry, thereby creating a privileged site for the bacteria. Of note, antibodies against the Alt or its glucosaminidase subunit (Gmd) and mutations of atl result in the formation of megaclusters of S. aureus that are unable to enter the OLCN (Biswas et al., 2006; Varrone et al., 2014).
Our previous review detailed the imbalances in cytokine release in those patients that did well versus those with a poor outcome in SAB (Miller et al., 2020). High IL-10 (anti-inflammatory cytokine) and low IL-1 and IL-6 (critically important cytokines early in the immune response) are associated with worse outcome. A more detailed examination of the cytokine response to S. aureus infections can be found in our previous review (Miller et al., 2020), and newer data on human genetic susceptibility is considered here. Some of the genetic variability is related to methylation patterns of the human genome that relate to the control of these cytokines.
Among the first studies aiming to identify the role of human genetic variability in SAB outcomes was one reported by Nelson in 2014. This study used a genome-wide association study (GWAS) approach and found more complicated SAB associated with single nucleotide polymorphisms (SNPs) located within CDON (cell surface receptor in the immunoglobulin family) genes (Nelson et al., 2014). GWAS was studied in 361 cases of SAB and 699 controls (hospitalized patients with no SAB). Causal relationships could not be established due to the relatively small size, but this study showed that future analyses would likely produce important leads.
Subsequent studies aiming to identify genes involved in predisposition to invasive S. aureus infection have been described. In a large French and Danish study of 139 patients with SAB, genetic predisposition for the development of native valve endocarditis was examined using whole genome sequencing (Moreau et al., 2018). Patients with SNPs in SLC7A14 (Solute Carrier Family 7, Membere 14; possibly a cationic amino acid transporter) suggested protection against acquiring infective endocarditis. Ex vivo analysis of aortic valve tissues showed higher expression levels of SLC7A14 mRNA for people that were protected against acquiring infective endocarditis. No mechanism has been established for how this genotype protects.
Recently, Spaan et al. (2022) performed a GWAS study on 105 cases of life-threatening S. aureus infection vs. 1157 controls. The authors reported that haploinsufficiency (heterozygous deleterious mutations) of otulin genes represents a significant correlate of worsened outcomes in human skin infection due to S. aureus. Otulin limits host cell death in context of inflammation by deubiquinating the linear ubiquitin chain assembly complex (LUBAC). Human otulin haploinsufficiency is hypothesized to limit cell-intrinsic immunity of dermal fibroblasts to S. aureus α-toxin, resulting in greater host cell vulnerability to inflammatory death due to this virulence factor.
Population studies also linked outcomes of SAB to family clusters in first-degree relatives in Denmark (Oestergaard et al., 2016). Fifty-four patients with SAB were found amongst first-degree relatives who had prior hospitalization with microbiologically verified S. aureus bacteremia. Thus, having a first-degree relative with SAB significantly increased one’s risk for SAB. While no genes were studied in these patients, other groups have reported genes associated with bacterial sepsis caused by other pathogens, including TLR2, TLR4, TIRAP, IRAK4, TRAF6, NOD2, and CISH. Patients with SAB were found to have SNPs in the haplotypes of TLR2, TLR4, TIRAP (Toll/IL-1R domain-containing adapter protein), NOD2, and CISH. SNPs associated with persistent or more complicated SAB were found in TIRAP’s, GLS2 (glutamine synthase) (Cyr et al., 2017; Scott et al., 2018; Carter et al., 2020).
Using a directed approach to the study of the human genome variability, Chong et al., 2014 looked at the role of mannose-binding lectin (MBL) in SAB, as MBL was known to play a role in controlling S. aureus infection in animals (Chong et al., 2014). MBL is a circulating C-type lectin that selectively binds patterns of glycans on many organisms, including S. aureus. Polymorphisms in the human MBL2 gene were linked to persistent SAB. This is consistent with previous studies which found that MBL2 deficiency was associated with worse outcomes in patients with cystic fibrosis (CF) (Carlsson et al., 2005).
Since the first human GWAS study in 2014, several studies have linked individual differences in human DNA methylation patterns with persistent SAB (Mba Medie et al., 2019; Chang et al., 2021). Whole genome sequencing was performed on whole blood of patients with vancomycin-treated persistent versus resolving SAB who were matched for important clinical variables (Mba Medie et al., 2019). Genetic variants were identified and differences in epigenetic signatures, gene expression and serum cytokine levels were examined in 34 patients in each group of resolving (RB) versus persistent SAB (PB). A single nucleotide polymorphism in DNA methyltransferase-3A (DNMT3A), which likely resulted in lower methylation activity, correlated with persistent SAB and increased serum IL-10 levels in patients. In contrast, a gain-in-function polymorphism in DNMT3A correlates with protection against persistence and lower IL-10 levels, wherein A/C heterozygotes were much more likely to resolve SAB (p = 7.8 x10-6) than A/A homozygotes (Mba Medie et al., 2019; Chang et al., 2021). Thus, genotypically A/A patients had higher serum levels of IL-10, skewing immune context to an anti-inflammatory bias, in turn correlating with persistent SAB and poor outcomes (Mba Medie et al., 2019). High levels of IL-10 have been reported in several other studies of persistent SAB (Miller et al., 2020). The mechanisms corresponding to methylation-driven differences in immune efficacy for resolving versus persisting SAB are under investigation.
In a follow-up study of 142 patients from the same cohort (RB = 70, PB = 72), the persistent SAB group exhibited hypomethylation of the CCAAT enhancer binding protein-β (C/EBPβ) promoter (Chang et al., 2021). C/EBPβ is a key transcription factor in emergency granulopoiesis, rapidly mobilizing bone marrow progenitors during systemic infection. This finding was consistent with earlier observations reported by Hirai et al. (Hirai et al., 2006), and corresponded to higher neutrophil counts in the peripheral blood of patients with persistent SAB. This observation suggests that the quantity of neutrophils may not be as important as their anti-staphylococcal readiness in response to S. aureus infection. For example, immature neutrophils (e.g. band cells) generated by emergency granulopoiesis may be less efficient at killing S. aureus, thereby facilitating persistence. This counterintuitive relationship between greater numbers of neutrophils but less effective clearance of SAB is an interesting line of investigation in progress.
Persistent vs. resolving outcomes in SAB also appear to involve differential T cell polarization. For example, patients with persistent SAB showed hypomethylation of the STAT1 promoter that activates the Th1 pathway, but suppresses Th17 activation. Concordantly, other studies have shown that S. aureus activates human macrophages via STAT1 (Sun et al., 2018). In this scenario, greater IFNγ response would be anticipated to promote macrophage function, but reduced IL-17 would be anticipated to limit neutrophil function. Given that STAT3 induction is integral to Th17 polarization and IL-17 expression that promotes neutrophil influx into infected sites (Yeaman et al., 2014), the coordination of T cell responses provides contextual immunity essential for resolution of S. aureus infection. Finally, in patients with resolving SAB, hypomethylation of the promoter for the glucocorticoid receptor (GR) and its signaling partner, histone acetyltransferase p300, suggested that glucocorticoids may play a role in SAB resolution (Chang et al., 2021). Activated GR can directly interact with p300 to regulate signaling. Together, these findings suggest GR-p300 signaling can promote immune responses in RB and facilitate bacteremia resolution. Human genetic variabilities have also been observed in relation to susceptibility and outcomes in S. aureus infections other than SAB, including endocarditis and soft tissue infections (Messina et al., 2016; Moreau et al., 2018; Scott et al., 2018).
Rates of invasive S. aureus infections and severity are significantly higher among African Americans than European-descended populations (Klevens et al., 2007; Kallen et al., 2010). In white subjects, two single-nucleotide polymorphisms (SNPs) in the human leukocyte antigen (HLA) class II region on chromosome 6 have been significantly associated with susceptibility to S. aureus infection at a genome-wide level (DeLorenze et al., 2016). This finding suggests that differences in antigen processing or presentation may affect immune responses to S. aureus infection. A study of SAB in African Americans revealed significant evidence of increased European ancestry among SAB cases in the same 5′ of the HLA-DRA region (Cyr et al., 2017). This study identified the same region previously reported in our GWAS and extends the association of HLA class II to SAB in multiple ethnic groups. Furthermore, a recent study by Rani et al. (2022) found HLA-DR-DQ gene polymorphisms determined outcomes of S. aureus infections. These results provide further support for association of SAB susceptibility relative to polymorphisms in the HLA class II region, which was introduced into African Americans in the USA.
Sex hormones have been demonstrated to influence susceptibility and outcomes in experimental models as well as human S. aureus infection. Numerous studies including that of Stensen et al. (Stensen et al., 2022) have shown that circulating sex-steroids influence nasal S. aureus carriage, with male sex associated with higher risk of colonization and infection by the organism. Interestingly, an inverse relationship has been observed between lower testosterone levels and increased persistent nasal carriage in males. Castleman et al. (2018) demonstrated that greater protection in skin of female mice is correlated with a sex-specific response to alpha hemolysin secreted by S. aureus. Likewise, sex-specific differences in neutrophil staphylocidal activity was also observed in relation to outcomes of experimental skin infection. Further investigation found that complement protein 3 and its receptor in neutrophils contributed to this innate sex bias to S. aureus infections in mice (Pokhrel et al., 2020). These findings implicated a role for estrogen in the induction of protective innate immune responses of female skin.
Studies using multivariate analysis identified gender differences as a correlate for developing bacteremia and endovascular infection due to S. aureus in humans. For example, Humphreys et al. (Humphreys et al., 2015) reported that human males have a greater incidence of SAB than females, while females appear to experience worse outcomes of SAB than males. This report is consistent with hazard ratios of 1.63 and 1.72 for MSSA and MRSA bacteremia respectively in males (Laupland et al., 2013). Likewise, studies by Kaasch et al. (Kaasch et al., 2014), Lessa et al. (Lessa et al., 2010), Gasch et al. (Gasch et al., 2013) and others have observed higher incidence of SAB in males than females. In contrast, human females have been suggested to have a somewhat higher mortality rate than males as a result of SAB (Lamagni et al., 2011; Barry et al., 2021). Similarly, Bansal et al. (Bansal et al., 2021) and Barry et al. recently reported that males have a higher incidence of infective endocarditis (Barry et al., 2021). However, this is a common theme of sepsis (Sunden-Cullberg et al., 2020) where sepsis in females is associated with higher mortality, is recognized later and females receive treatment later than males.
Langerhans cells (LCs) are important for human immunity to S. aureus, as they are the professional antigen-presenting cell type unique to the epidermis. They sense S. aureus through their pattern-recognition receptor langerin (CD207), which promotes uptake of S. aureus as a C-type lectin receptor for surface components triggering a proinflammatory response. Langerin recognizes β-1,4-linked N-acetylglucosamine (Hendriks et al., 2021). Mutations have been defined which cause defects in Langerhans cells that correlated with increased skin infections and with poor resolution (Stojadinovic et al., 2013; van Dalen et al., 2020; Hendriks et al., 2021). SNPs are commonly found in langerin, which changes specificity for glycan ligands and can alter specificity for certain staphylococcal antigens (Feinberg et al., 2013). Decreased numbers of CD207+ LC have been correlated with failure to heal diabetic foot ulcers (Stojadinovic et al., 2013), which is prevalently caused by S. aureus.
In addition to LCs, numerous studies have shown that NOD-like receptor P3 (NLRP3) inflammasomes of neutrophils and macrophages are key for clearing S. aureus from the skin. NOD-like receptors are intracellular sensors that detect intracellular microbial antigens and cell injury that activates the innate immune inflammatory responses to S. aureus infection. NLRP3 inflammasome is activated through the detection of staphylococcal antigens (e.g. Panton-Valentine leukocidin, α-hemolysin and leukocidin A/B) (Craven et al., 2009; Holzinger et al., 2012; Melehani et al., 2015). NLRP3 Q705K/C10X polymorphisms are associated with delayed apoptosis of neutrophils (Blomgran et al., 2012), which likely mitigates intracellular clearance of S. aureus. Moreover, analysis of NLRP3 29940G>C SNPs in clinical sepsis populations revealed that this gain-of-function mutation suppressed NLRP3 expression and downstream inflammatory cytokine responses, which protected patients against poor clinical outcomes (Lu et al., 2021). Thus, studies of anti-S. aureus vaccines could be impacted by the presence of these mutations in the subjects in vaccine trails.
In summary, human genetic variability that impacts outcome has major relevance for the design of clinical trials. If the trial is large enough, then genetic variation should be negligible between vaccine and control groups. Conversely, such variations could impact outcomes of the trials in small sample populations. Such an event may have impacted the Merck V710 trial (recombinant IsdB) wherein subjects that developed a fatal systemic inflammatory response following vaccination and invasive S. aureus infection (Fowler et al., 2013) had abnormally low IL-2 levels prior to vaccination (McNeely et al., 2014). Therefore, genetic variability correlates of poor outcomes need to be assessed to interpret results of vaccine trials.
Staphylococcal infection involves adaptation to numerous host selective pressures within specific niches. The metabolic microenvironment directs activity of both the host immune response as well as that of the bacteria that must compete for favored substrates and micronutrients. Many of the so-called S. aureus virulence factors are gene products that are regulated by the abundance of specific metabolites. Such regulatory systems enable bacteria that have disseminated to various tissues to rapidly adapt, often using metabolic pathways that are distinct from those expressed during bloodstream infection. The concept that central metabolism serves as a signaling pathway in S. aureus (Somerville and Proctor, 2009) is now being substantiated by multiple studies reviewed below.
Isolates that have undergone in vivo adaption often harbor loss of function mutations in the master virulence regulator agr (Traber et al., 2008; Suligoy et al., 2018), yet these strains are often better able to persist [reviewed in (Tuchscherr et al., 2020)]. Other adaptive mutations (gain or loss of function) occur in metabolic genes (McAdam et al., 2011; Acker et al., 2019; Gabryszewski et al., 2019; Giulieri et al., 2022), highlighting the crucial role of S. aureus metabolic flexibility in bacterial survival and persistence. For example, in glucose-replete conditions, the catabolite control protein A (CcpA) enables S. aureus to utilize the preferred carbon source glucose over secondary carbon sources such as amino acids (Seidl et al., 2009). However, when glucose is depleted, as is often the case in infected tissues such as the lung, as host and bacterial cells compete, catabolite repression is alleviated to enable rapid bacterial consumption of available substrates. In this section, we will discuss the metabolic crosstalk between bacterial and innate immune cells and how the dynamics of this interaction influences the infection outcome, often to the detriment of the host.
Upon bacterial stimulation, immune cells are activated and undergo profound metabolic changes that dictate their immune function, a process termed metabolic reprograming (reviewed in (O'Neill et al., 2016) (Figure 1). This includes an increase in glycolysis, which is accompanied by the accumulation of the tricarboxylic acid cycle (TCA) metabolites including succinate and itaconate. Succinate stabilizes the host transcription factor, hypoxia-inducible factor-1α (HIF1-α), promoting the production of the pro-inflammatory cytokine IL-1β. In contrast, itaconate restores homeostatic balance following succinate-driven inflammation via its anti-inflammatory and anti-oxidative roles (Lampropoulou et al., 2016; Qin et al., 2019; Hooftman et al., 2020). There have been multiple demonstrations that metabolically active S. aureus stimulates macrophage and keratinocyte glycolysis and HIF1-α activation (Wickersham et al., 2017; Acker et al., 2019; Wong Fok Lung et al., 2020). This was shown to be dependent on the ability of S. aureus itself to perform glycolysis, highlighting host-bacterial cell competition for glucose. Immunometabolites are increasingly appreciated as drivers of bacterial adaptation and thus have an important role in pathogenesis. Moreover, a better understanding of how S. aureus adapts to specific microenvironments may provide novel, tissue-targeted strategies for staphylococcal eradication.
Figure 1 Metabolic reprogramming of macrophage. During infection with live bacteria or upon stimulation with PAMPs such as LPS, macrophages become activated for pathogen clearance (pro-inflammatory M1 phenotype) by undergoing metabolic changes. These include increased glycolysis and accumulation of TCA cycle metabolites such as citrate, succinate and itaconate. Succinate stabilizes the transcription factor HIF-1α, which promotes the production of proinflammatory cytokines. Itaconate dampens the succinate-induced inflammation via its anti-inflammatory properties.
A component of the TCA cycle generated by Acod1 or Irg1, itaconate has a well-established role in modulating the proinflammatory response to LPS. Its importance in host defenses against S. aureus is being increasingly appreciated, as this is one of the major metabolites found in the airway in S. aureus pneumonia (Tomlinson et al., 2021). Whilst itaconate suppresses inflammation in the host by inhibiting the NLRP3 inflammasome, glycolytic enzymes and succinate dehydrogenase (SDH/mitochondrial complex II) (Lampropoulou et al., 2016; Qin et al., 2019; Hooftman et al., 2020), it has several bacterial targets. Itaconate inhibits the glyoxylate cycle of Salmonella typhimurium and Pseudomonas aeruginosa as well as glycolysis of S. aureus (McFadden and Purohit, 1977; Hersch and Navarre, 2020; Tomlinson et al., 2021), crucial pathways for the survival of these pathogens. While the former two pathogens can degrade itaconate (Sasikaran et al., 2014; Wang et al., 2019), S. aureus lacks these degradative enzymes, yet can tolerate it by actively altering its metabolic activity. It was recently shown that the inhibition of the staphylococcal glycolytic enzyme aldolase by itaconate led to redirecting of carbon flux to pathways that promote biofilm synthesis (Figure 2) (Tomlinson et al., 2021). Longitudinal isolates of S. aureus that have adapted to the itaconate-replete cystic fibrosis (CF) airway (Riquelme et al., 2020) displayed enhanced biofilm forming capacity (Tomlinson et al., 2021), a response that can be replicated by exposure of S. aureus to itaconate in vitro.
Figure 2 S. aureus adaptation to specific immunometabolites impact host immunity. Left panel: Itaconate produced during infection inhibits S. aureus glycolysis. This rewires staphylococcal metabolism, promoting carbon flux through metabolic pathways that synthesize biofilms. S. aureus biofilms promote OXPHOS in macrophages and an anti-inflammatory phenotype. Middle panel: Lactate produced by S. aureus biofilm inhibits HDAC11 in macrophages and promotes anti-inflammatory IL-10 production. Right panel: Fumarate also inhibits glycolysis. In order to relieve the inhibition of glycolysis and ensure their survival, S. aureus SCVs increase their expression of fumC, which encodes the enzyme fumarate hydratase to degrade fumarate. SCV degradation of fumarate inhibits trained immunity through decreased methylation at the promoters of genes encoding proinflammatory cytokines such as IL-6. This impairs swift cytokine production upon re-stimulation.
Changes in bacterial metabolism affect the metabolic activity of host immune cells. S. aureus that produce biofilm were shown to skew monocyte metabolism towards oxidative phosphorylation (OXPHOS) in a murine prosthetic joint infection (PJI) model (Yamada et al., 2020), although the exact mechanism is still unknown. This metabolic bias promoted an anti-inflammatory phenotype, as evidenced by the production of the anti-inflammatory cytokine IL-10, and bacterial persistence (Figure 2). IL-10 has also been associated with poor outcomes in human S aureus infections (Miller et al., 2020). Of note, OXPHOS is also a preferred metabolic pathway of immunosuppressive myeloid derived suppressor cells (MDSCs), which have been shown to promote S. aureus persistence in biofilm, a phenotype that could be attenuated by the adoptive transfer of pro-inflammatory monocytes (Heim et al., 2014; Heim et al., 2015). Importantly, the delivery of nanoparticles containing the OXPHOS inhibitor, oligomycin, enhanced bacterial clearance by inducing inflammation, indicating the potential of immunometabolic therapy to treat such persistent staphylococcal infections. Whether a comparable bias toward OXPHOS also happens in the lungs upon itaconate-induced biofilm formation remains to be determined. In contrast, in a model of murine eye infection, itaconate promotes S. aureus clearance (Singh et al., 2021), indicating that itaconate may have distinct effects in different tissues/sites.
Other mechanisms through which itaconate promotes staphylococcal persistence are likely. Itaconate can covalently modify available cysteine residues in both bacteria and host cells (Qin et al., 2019; Zhang and Cao, 2019; Zhang et al., 2021). In Salmonella, itaconation targets isocitrate lyase and given its accumulation at sites of infection, may also modify S. aureus metabolic targets other than aldolase. S. aureus induction of itaconate likely has multiple effects on anti-staphylococcal immune responses by affecting both the microorganism and the host (Chang et al., 2013; Jeon et al., 2020). In addition to biofilm formation and rewiring host immune metabolism, S. aureus activates several metabolite-associated epigenetic changes as discussed below.
Lactate is a common metabolite generated during infection that induces epigenetic changes in immune cells (Latham et al., 2012). While mammalian cells predominantly produce L-lactate, S. aureus in biofilm produces both L- and D-stereoisomers (Stockland and San Clemente, 1969; Kondoh et al., 1992; Fuller et al., 2011). These forms of lactate are transported into monocytes, macrophages and MDSCs via the transporter MCT1 to inhibit the histone deacetylase, HDAC11. The decreased HDAC11 activity enables unchecked HDAC6 activity, a positive regulator of Il-10, thereby increasing the production of the anti-inflammatory cytokine IL-10 and promoting bacterial persistence (Figure 2) (Heim et al., 2020). This demonstrates the role of active bacterial metabolism in shaping the host immune response. Interestingly, the synovial fluid of patients with PJI had increased levels of D-lactate and IL-10 compared to control subjects, confirming the clinical significance of lactate in the modulation of host immunity. There are undoubtedly other sites of S. aureus infection in which staphylococcal lactate similarly alters immune responses.
Fumarate, which is structurally similar to itaconate, is another major component of the TCA cycle that is generated during S. aureus infection. Like itaconate, fumarate also can inhibit glycolysis (Kornberg et al., 2018). S. aureus SCVs rely heavily on glycolysis for survival and persistence (Proctor et al., 1995). The SCVs, which are phenotypically distinct from normal S. aureus colonies, typically have mutations in genes associated with electron transport chain components such as heme and menadione, that alter their metabolism (Proctor et al., 2006). In addition, these mutations render them resistant to aminoglycoside antibiotics, and clinically problematic in settings of chronic infections. SCVs have decreased TCA cycle activity and OXPHOS, thereby relying on increased glycolysis to meet their energy requirements (Kriegeskorte et al., 2014). In order to relieve the inhibition of glycolysis and ensure their survival, prototypic SCVs notably increase their expression of fumC, which encodes the enzyme fumarate hydratase to degrade fumarate (Figure 2) (Wong Fok Lung et al., 2020).
While the local accumulation of fumarate affects staphylococcal metabolic activity, there are also important effects on the host. S. aureus expression of fumC depletes fumarate from the local environment and has serious detrimental ramifications on host innate immunity. SCV degradation of fumarate derails innate immune memory (trained immunity) in a mouse model of skin infection, allowing recurrent infections (Figure 2). Trained immunity refers to increased innate immune protection against a secondary challenge following primary infection and relies on the active interplay between both metabolic and epigenetic reprogramming. Specifically, trained macrophages undergo metabolic changes including a hallmark increase in glycolysis and the accumulation of metabolites that drive epigenetic rewiring of cellular function (Arts et al., 2016). The accumulation of fumarate inhibits KDM5 histone demethylases promoting histone methylation (H3K4me3) at the promoters of genes encoding proinflammatory cytokines such as TNF-α and IL-6. This induces swift cytokine production upon re-stimulation. In a model of skin infection, prior priming with wild type (WT) S. aureus USA300 reduced skin lesion severity and bacterial burden upon secondary challenge (Chan et al., 2017; Chan et al., 2018; Wong Fok Lung et al., 2020), illustrating the ability of the host to fight recurrent infection by WT S. aureus. This highly localized protection was mediated by macrophages (Chan et al., 2017; Chan et al., 2018) and adoptive transfer of WT S. aureus-primed macrophages into naïve mouse skin conferred protection in vivo (Chan et al., 2018). However, priming by a S. aureus SCV prototype, ∆hemB, or a host-adapted isolate from a patient with atopic dermatitis did not confer protection from secondary infection in a fumC-dependent manner (Acker et al., 2019; Wong Fok Lung et al., 2020). Increased fumC expression was also observed in numerous S. aureus isolates from patients with atopic dermatitis and cystic fibrosis (Acker et al., 2019; Gabryszewski et al., 2019). However, it remains to be determined if the modulation of trained immunity by host-adapted S. aureus strains occurs in other organs such as the lungs.
There are a wealth of in vitro data delineating the exceptional diversity of S. aureus metabolic activity with well characterized strains and many defined mutants. The increased availability of genomic sequencing, transcriptomic and metabolomic data generated from in vivo models and clinical isolates facilitate a much more nuanced understanding of how these organisms adjust their metabolic activity in the setting of clinically important infections. The immunometabolic consequences of S. aureus infection affect both the host and the microorganism and are a major factor in the selection of variants best suited for infection in a specific microenvironment.
Mucosal-associated Invariant T (MAIT) cells are a highly conserved unconventional T cell subset, which are abundant in humans, and important in mucocutaneous immunity against S. aureus. Activation of MAIT cells requires interaction with vitamin B2 metabolites, which recognize vitamin B-based antigens presented by the non-polymorphic MHC class I related-1 molecule (MR1) of antigen-presenting cells (Corbett et al., 2014; Eckle et al., 2015; Soudais et al., 2015). S. aureus riboflavin synthesis pathway releases riboflavin intermediates that bind the MR1-presentation pathway to activate MAIT cells. MAIT cells can be viewed as “emergency responders” to invasion of mucocutaneous surfaces (Shaler et al., 2017) as they are capable of producing pro- and/or anti-inflammatory cytokines (e.g., IFN-γ, TNF-α, IL-4, IL-10) readily after invariant T cell receptor (iTCR) stimulation. MAIT cells are an important source for IL-17 production in the mucosa and play a protective role in bacterial pneumonia (Hannaway et al., 2020) and skin infections (Cox et al., 2021). MAIT cells are much more predominant in human skin and mucosa than in mice. Co-culture of MAIT and dendritic cells infected with S. aureus enhances IFN-γ and granzyme B production and degranulation. Granzyme B has direct anti-staphylococcal activity and promotes neutrophil-mediated clearance of SA infected cells, reducing intracellular persistence of S. aureus (Cooper et al., 2022). Hence, vitamins and MAIT may play a key role in resolving S. aureus infections.
However, S. aureus can hijack MAIT cells, especially during cytokine storm induced by superantigens (SAgs), as they are the most powerful source of pro-inflammatory cytokines after exposure to SAgs (Shaler et al., 2017). When MAIT cells are hyperactivated by SAgs, the ability of the host to clear bacteria is reduced (Shaler et al., 2017). Moreover, MAIT cells are susceptible to leukocidin ED-mediated lysis, which was mediated through CCR5 binding (Boulouis et al., 2022). S. aureus also can avoid recognition by MAIT cells via SAg activation of MAIT cells in a non-specific manner leaving them functionally impaired to stimulation with bacterial antigen (Sandberg et al., 2017).
Vitamins D and A enhance innate immunity against S. aureus in keratinocytes, which was previously reviewed in Miller et al. (2020), but new information about a potential role for vitamin D has been reported by Zheng Q et al. (Zheng et al., 2022). In this study of differentially expressed genes in 31 patients with SAB as compared to 43 healthy controls, IFI44 was identified as an immune evasion biomarker. IFI44 negatively regulates the IFN signaling pathway in dendritic cells and was found to be increased in patients with SAB as compared to healthy controls. Of note, increased IFI44 is seen in patients with vitamin D deficiency, and it can be suppressed by administering vitamin D3. Hence, vitamin D therapy may be an option is patients with persistent SAB. However, vitamin D therapy recently failed in a trial of persistent MRSA carriage even though all included patients were deficient in vitamin D (Björkhem-Bergman et al., 2018).
Among the key mysteries needing to be solved to accelerate the development of safe and effective vaccines targeting S. aureus is the identification of specific determinant(s) of protective immune efficacy. As can be surmised from the above sections, no single cellular subset or molecular class is solely responsible for protective immunity to this elusive pathogen. Furthermore, neither adaptive cellular immune mechanisms nor antibodies are sufficient for protection against in patients who face recurring S. aureus infection.
Vaccines demonstrating success in experimental models have provided hints regarding biomarkers for protection that remain to be fully explored in humans. Anti-toxin antibody levels against Hla, TSST-1, PVL, and SEA correlate with less severe disease including pneumonia, skin infections, osteomyelitis, and pyomyositis in the majority of studies (Miller et al., 2020). However, a direct quantitative correlation cannot always be made between presence of antibody and the infection outcome. For example, antibody subclasses IgG1 and IgG3 in humans have greater opsonophagocytic potential and likely contribute to more effective cell-mediated immune clearance of the pathogen than do IgG2 and IgG4. The opposite may be true for the neutralizing capacity of S. aureus exotoxins. Affinity maturation of antibody subclasses further complicates the specificity of antibody response. Of course, B cell production of antibody, particularly IgG, is governed by T cell recognition and crosstalk with B cells identifying cognate epitopes. Thus, the net protective efficacy of immune response to S. aureus infection or mitigation of severity likely results from a complex intersection of host and pathogen factors, including host genetic make-up, history of colonization or infection (immune memory), as well as S. aureus strain-specific combinations of immune evasion factors, antigenic epitopes, exotoxin signature and expression of these virulence factors. Moreover, neither the host immune response nor the adaptive pathogen virulence is static; rather, there is considerable dynamic interplay of host and pathogen in distinct temporospatial contexts.
Of the SA toxins, pore-forming toxins (PFTs) and superantigens (SAgs) have the most profound effect on the immune system. PFTs disrupt the skin barrier by killing keratinocytes (Soong et al., 2012), the first line of defense against SA i.e., polymorphonuclear neutrophils (PMNs), macrophages, and to lesser extent T cells (LukED) (Vandenesch et al., 2012; Aman and Adhikari, 2014). Uncontrolled lysis of PMNs leads to release of cytotoxic and proinflammatory molecules with pivotal consequences to host health and can also promote the dissemination of bacteria contained within phagosomes. Toxins are the only class of vaccine targets backed by strong human epidemiological and clinical data (Humphreys et al., 1989; Lina et al., 1999; Gillet et al., 2002; Barretti et al., 2009; Jacobsson et al., 2010; Rasigade et al., 2011; Adhikari et al., 2012; Bachert and Zhang, 2012; Fritz et al., 2013; Tong et al., 2015). Presence of pvl gene is associated with abscesses and furuncles (OR=10.5 (95% CI, 7.4 to 14.9) (Shallcross et al., 2013). The role of Hla in SSTI has been widely reported (Berube and Bubeck Wardenburg, 2013; Chua et al., 2014) (Inoshima et al., 2012). SAg-induced cytokine storm causes toxic shock (McCormick et al., 2001) and SAg expression has been related to septicemia (Humphreys et al., 1989; Jacobsson et al., 2010). PFTs and SAgs are implicated in SA skin colonization and exacerbation of atopic dermatitis (AD) that predisposes patients to recurrent SA SSTIs (Humphreys et al., 1989; Jacobsson et al., 2010; Brauweiler et al., 2013; Brauweiler et al., 2014). Most AD isolates produce SAgs (McFadden et al., 1993). SAgs are likely a major factor in the Th2 inflammatory response in AD patients (McFadden et al., 1993) and facilitate epithelial presentation of allergens to Th2 cells (Ardern-Jones et al., 2007; Forbes-Blom et al., 2012). Adhesion molecules that bind to SA in the skin like fibronectin and laminin are upregulated via the Th2 cytokine IL-4 and suppress IFNγ or TNFα induced release of antimicrobial peptides by keratinocytes (Cho et al., 2001). Thus, neutralization of PFTs and SAgs in the skin can change the immunological environment to unfavorable conditions for colonization and infection.
A large number of human clinical and epidemiological studies provide evidence for a protective role of antibodies against toxins in S. aureus disease. Lower levels of antitoxin antibodies in patients correlates with increased probability of complications (Jacobsson et al., 2010; Adhikari et al., 2012; Fritz et al., 2013). Skin infections with bacteria that produce PVL cause a higher frequency of abscess and need for incision and drainage (Gubbay et al., 2008; Jahamy et al., 2008; Munckhof et al., 2008; Kaltsas et al., 2011). Higher level of antibodies to Hla correlate with reduced rate of recurrent skin infection (Fritz et al., 2013). Hla production also correlates with poor resolution of staphylococcal peritonitis in continuous ambulatory peritoneal dialysis (CAPD) patients (Barretti et al., 2009). Children with the lowest antibody levels against Hla and the PVL component LukF are more likely to develop invasive disease (Fritz et al., 2013). Menstrual TSS patients that do not seroconvert are more likely to experience recurring bouts (Kansal et al., 2007). Humphreys et al. characterized 52 strains from septic patients and 27 strains from healthy SA nasal carriers. Of the septic isolates, 63% produced SEA, SEB or SEC, whereas 11% of the nasal isolates produced a SAg (Humphreys et al., 1989). Azuma et al. detected SAgs in the plasma of 31% of septic patients without septic shock and 41% of those with septic shock (Azuma et al., 2004). It is important to note that to-date studies have primarily evaluated total antibody titers (mostly total IgG) rather than toxin neutralizing titers. Functional antibody titers are more likely to be informative biomarkers than total IgG.
A series of recent studies in animal models highlight the importance of staphylococcal protein A (SpA) as a key virulence factor. Protein A (SpA) is expressed on the surface of SA and as secreted protein, and best known for its interaction with the Fc domain of IgG, preventing Ig hexamer formation required for C1q binding and subsequent bacterial clearance (Sharma-Kuinkel et al., 2019), and reducing interaction of IgG with the neonatal Fc receptor (FcRn) at pH6 shortening antibody half-life (Stapleton et al., 2011). Furthermore, SpA binds indiscriminately to the Fab portion of VH3 lineage antibodies and causes polyclonal expansion of these clones (Goodyear and Silverman, 2003). These SpA-mediated immune evasion mechanisms are believed to be critical for SA pathogenesis based on a series of animal studies showing that anti-SpA neutralizing antibodies as well as vaccination against SpA broadens the antibody response to other SA antigens and protects from lethal disease (Thammavongsa et al., 2015). A recent study showed that anti-IsdB antibodies in IsdB-immunized mice form complexes with SpA, haemoglobin and haptoglobin on the surface of SA (Nishitani et al., 2020). The complex is phagocytosed along with SA by a CD163 dependent mechanism into macrophages. Macrophages then act as trojan horse disseminating bacteria systemically leading to sepsis, suggesting a role for SpA in the failure of IsdB vaccine in the Merck clinical trial. However, clear evidence for the role of SpA in human disease remains to be explored.
Finally, a biomarker for good outcome has been identified that is not associated with toxin neutralization. Low levels of anti-glucosaminidase (Gmd) levels in patients with S. aureus osteomyelitis had a 2.7-fold increased chance of poor outcome. Gmd is the lytic portion of AltA (major autolysin) (Kates et al., 2020). Anti-Gmd antibodies that neutralize autolysin activity improve outcomes of invasive S. aureus infection (Sherchand et al., 2022).
There have been multiple attempts at producing a S. aureus vaccine that will prevent serious infections or mitigate their severity. Despite earnest efforts, none of these has yet to demonstrate safety and efficacy in human clinical trials. This result is in no small measure due to the fact that the complexities of both the virulence strategies of S. aureus and the human immune response are incompletely understood. In many respects, constitutive colonization with intermittent infection distinguishes this microorganism from many other bacterial pathogens. This host-pathogen relationship of course relates to the long evolutionary time span over which S. aureus has resided within or cultivated niches such as the nares of mammalian hosts. We are now learning that S. aureus enters human cells to subvert host defenses, and changes the basic metabolism of the host cells, which in turn has repercussions on the nature of the host immune response elicited. This is a two-way street as inflammatory immune cells, which sense the presence of intracellular S. aureus via its metabolic products or via competition for available substrates, also impose a strong selective pressure on the bacteria. This immunometabolic pressure is exemplified by the direct effects of metabolites such as itaconate on the bacteria that drive staphylococcal adaptation for survival. Of note, these host-staphylococcal metabolic cross-talks emphasize the importance of using live bacteria in infection models as opposed to bacterial proxies such as pathogen-associated molecular patterns (PAMPs). These complex interactions are just beginning to be understood.
Detailed information about the human genetic susceptibility to S. aureus infection is in its infancy, but we can already see that some humans seem predisposed for worsened outcomes, persistence or recurrent infection. For example, prior to recombinant IsdB vaccine administration, patients that developed a systemic inflammatory response to S. aureus invasion had pre-existing low IL-2 levels. This example raises key questions regarding potential dysregulation of Treg responses, which may fail to limit adverse inflammatory responses. There is also a chicken-versus-egg mystery remaining to be solved regarding host-pathogen relationships if we are to develop safe and effective vaccines for this pathogen. Surprisingly, a large proportion of children who become infected with S. aureus demonstrated impaired T-cell function. Further, patients with SNPs in CD207 of Langerhans cells or NLRP3 of phagocytes may be more prone to skin infections. Such correlates point to potential heritable as well as acquired dysfunction in protective immunity to S. aureus.
Because S. aureus and mammals have lived together for so long, preventing invasive disease presents unique and complex challenges for vaccine development that have yet to be solved. More detailed understanding of protective determinants of immunity to S. aureus and new experimental models in which to test candidate vaccines may provide new insights to overcome current hurdles. Even though these represent a very high bar, the continuous development of cutting-edge techniques and the potential leveraging of integrated omics data as a more holistic approach bring us closer to our goal. Alternatively, because S. aureus infections represent myriad forms of disease reflecting distinct temporospatial contexts, it may prove necessary to create vaccines aimed at protecting specific tissues such as skin or in persons with individual genetic make-up—truly precision meets personalized medicine.
In looking to the future, we may be able to pair conventional vaccine targets with novel host-directed strategies with the aim of decreasing the selective pressure on the bacteria while promoting immune clearance (Figure 3). The field is currently on the threshold of testing multivalent anti-toxin vaccines with the hopes that recurrent skin infections can be reduced. Future vaccine attempts should also consider addressing potential immune imbalances such as low IL-2 levels/reduced Tregs to avoid the production of over exuberant immune responses in subgroups of vaccinated patients. Also, consideration will need to be given to the enhancement of M1 polarization of macrophages whose activities are down-regulated by the metabolic tricks used by S. aureus to disarm the immune response. Compounds such as oligomycin (OXPHOS inhibitor), which showed promise following nanoparticle delivery in a mouse model of S. aureus prosthetic joint infection, might be considered as adjuvants to the natural immune response. However, a deeper understanding of immunometabolic interactions within specific tissues is required. Because multiple studies have reported that increased IL-10 levels correlate with poor outcomes, perhaps anti-IL-2 therapies may improve outcomes. Finally, the novel anti-Gmd antibodies, while opsonic, are also directed at the fission plane, resulting in mega-clusters of S. aureus that are unable to disseminate. While anti-Gmd antibody levels have not yet been validated as a biomarker in human clinical trials, it serves as a potential example biomarker candidate of successful outcome and therefore a vaccine directed at Gmd may hold promise to reduce mortality in S. aureus infections.
Figure 3 Model summarizing the concepts covered and their links to vaccine development and alternative therapies. During infection, S. aureus employs several strategies to evade immune clearance. This includes increased binding to host fibronectin and uptake by host epithelial or endothelial cells. S. aureus also impairs immune responses through toxin-mediated immune cell damage. This may be counteracted through the use of multivalent anti-toxin vaccine. S. aureus fails to activate immune cells for pathogen clearance via immunometabolic subversion, such as skewing the host cell metabolism away from glycolysis and inflammatory signaling towards OXPHOS. Alternatively, S. aureus escapes immune cells and antibiotics through the formation of biofilms, or small colony variants (SCVs) that survive inside non-professional phagocytes. In addition, during bone infections, S. aureus undergoes shape shifting to invade the canaliculi of osteocytes, which cannot be reached by neutrophil pseudopods. Anti-Gmd antibodies may be the only effective anti-surface structure protein to be associated with reduced infection given that these antibodies make S. aureus form megaclusters.
All authors listed have made a substantial, direct, and intellectual contribution to the work and approved it for publication.
AP was supported by NIH grant R35 HL 135800 and the Cystic Fibrosis Foundation CFF PRINCE18G0. TWFL was supported by NIH grant K99 HL157550. MY was supported by NIH grant U01 AI-124319-01, R21 AI-111661-02, and R33 AI-111661-01. LC was supported by grant KL2TR001882 from the UCLA CTSI (UL1TR001881). NA was supported by NIH grant R01 AI146177, R01 AR073665, and R01 AI111205. MA receives support via NIH grant R01 AI111205.
Figures were created with Biorender.com.
RP is a consultant for IBT which is producing a multi-valent antitoxin vaccine and is a member of the review board for the University of Rochester which is involved in an anti-Gmd vaccine effort. MY is a consultant for Genentech-Roche, Alexion/AstraZeneca and Horizon Pharmaceuticals, which are involved in discovery and development of immunotherapeutic agents and strategies. NA is a consultant for Janssen Pharmaceuticals and has received previous grant support from Pfizer and Boehringer Ingelheim, which are involved in discovery and development of immunotherapeutic agents and strategies. MA has stocks in Integrated Biotherapeutics, a company engaged in development of vaccines and antibody therapies for bacterial infections.
The remaining authors declare that the research was conducted in the absence of any commercial or financial relationships that could be construed as a potential conflict of interest.
All claims expressed in this article are solely those of the authors and do not necessarily represent those of their affiliated organizations, or those of the publisher, the editors and the reviewers. Any product that may be evaluated in this article, or claim that may be made by its manufacturer, is not guaranteed or endorsed by the publisher.
Acker, K. P., Wong Fok Lung, T., West, E., Craft, J., Narechania, A., Smith, H., et al. (2019). Strains of Staphylococcus aureus that colonize and infect skin harbor mutations in metabolic genes. iScience 19, 281–290. doi: 10.1016/j.isci.2019.07.037
Adhikari, R. P., Ajao, A. O., Aman, M. J., Karauzum, H., Sarwar, J., Lydecker, A. D., et al. (2012). Lower antibody levels to Staphylococcus aureus exotoxins are associated with sepsis in hospitalized adults with invasive s. aureus infections. J. Infect. Dis. 206 (6), 915–923. doi: 10.1093/infdis/jis462
Alonzo, F., 3rd, Kozhaya, L., Rawlings, S. A., Reyes-Robles, T., DuMont, A. L., Myszka, D. G., et al. (2013). CCR5 is a receptor for Staphylococcus aureus leukotoxin ED. Nature 493 (7430), 51–55. doi: 10.1038/nature11724
Aman, M. J., Adhikari, R. P. (2014). Staphylococcal bicomponent pore-forming toxins: targets for prophylaxis and immunotherapy. Toxins (Basel). 6 (3), 950–972. doi: 10.3390/toxins6030950
Ardern-Jones, M. R., Black, A. P., Bateman, E. A., Ogg, G. S. (2007). Bacterial superantigen facilitates epithelial presentation of allergen to T helper 2 cells. Proc. Natl. Acad. Sci. U.S.A. 104 (13), 5557–5562. doi: 10.1073/pnas.0700733104
Armentrout, E. I., Liu, G. Y., Martins, G. A. (2020). T Cell immunity and the quest for protective vaccines against staphylococcus aureus infection. Microorganisms 8 (12), 1936. doi: 10.3390/microorganisms8121936
Arts, R. J., Novakovic, B., Ter Horst, R., Carvalho, A., Bekkering, S., Lachmandas, E., et al. (2016). Glutaminolysis and fumarate accumulation integrate immunometabolic and epigenetic programs in trained immunity. Cell Metab. 24 (6), 807–819. doi: 10.1016/j.cmet.2016.10.008
Askarian, F., Wagner, T., Johannessen, M., Nizet, V. (2018). Staphylococcus aureus modulation of innate immune responses through toll-like (TLR), (NOD)-like (NLR) and c-type lectin (CLR) receptors. FEMS Microbiol. Rev. 42 (5), 656–671. doi: 10.1093/femsre/fuy025
Azuma, K., Koike, K., Kobayashi, T., Mochizuki, T., Mashiko, K., Yamamoto, Y. (2004). Detection of circulating superantigens in an intensive care unit population. Int. J. Infect. Dis. 8 (5), 292–298. doi: 10.1016/j.ijid.2003.12.005
Bachert, C., Zhang, N. (2012). Chronic rhinosinusitis and asthma: novel understanding of the role of IgE 'above atopy'. J. Intern. Med. 272 (2), 133–143. doi: 10.1111/j.1365-2796.2012.02559.x
Bansal, A., Cremer, P. C., Jaber, W. A., Rampersad, P., Menon, V. (2021). Sex differences in the utilization and outcomes of cardiac valve replacement surgery for infective endocarditis: Insights from the national inpatient sample. J. Am. Heart Assoc. 10 (20), e020095. doi: 10.1161/JAHA.120.020095
Barretti, P., Montelli, A. C., Batalha, J. E., Caramori, J. C., Cunha Mde, L. (2009). The role of virulence factors in the outcome of staphylococcal peritonitis in CAPD patients. BMC Infect. Dis. 9, 212. doi: 10.1186/1471-2334-9-212
Barry, M., Bari, S. A., Akhtar, M. Y., Al Nahdi, F., Erlandez, R., Al Khushail, A., et al. (2021). Clinical and microbiological characteristics of infective endocarditis at a cardiac center in Saudi Arabia. J. Epidemiol. Glob Health 11 (4), 435–443. doi: 10.1007/s44197-021-00013-5
Berends, E. T. M., Zheng, X., Zwack, E. E., Ménager, M. M., Cammer, M., Shopsin, B., et al. (2019). Staphylococcus aureus impairs the function of and kills human dendritic cells. via LukAB Toxin. mBio. 10 (1), e01918–18. doi: 10.1128/mBio.01918-18
Berube, B. J., Bubeck Wardenburg, J. (2013). Staphylococcus aureus α-toxin: nearly a century of intrigue. Toxins (Basel). 5 (6), 1140–1166. doi: 10.3390/toxins5061140
Biswas, R., Voggu, L., Simon, U. K., Hentschel, P., Thumm, G., Götz, F. (2006). Activity of the major staphylococcal autolysin atl. FEMS Microbiol. Lett. 259 (2), 260–268. doi: 10.1111/j.1574-6968.2006.00281.x
Björkhem-Bergman, L., Missailidis, C., Karlsson-Valik, J., Tammelin, A., Ekström, L., Bottai, M., et al. (2018). Vitamin d supplementation to persistent carriers of MRSA-a randomized and placebo-controlled clinical trial. Eur. J. Clin. Microbiol. Infect. Dis. 37 (9), 1735–1744. doi: 10.1007/s10096-018-3306-7
Blomgran, R., Patcha Brodin, V., Verma, D., Bergström, I., Söderkvist, P., Sjöwall, C., et al. (2012). Common genetic variations in the NALP3 inflammasome are associated with delayed apoptosis of human neutrophils. PLoS One 7 (3), e31326. doi: 10.1371/journal.pone.0031326
Boulouis, C., Leeansyah, E., Mairpady Shambat, S., Norrby-Teglund, A., Sandberg, J. K. (2022). Mucosa-associated invariant T cell hypersensitivity to Staphylococcus aureus leukocidin ED and its modulation by activation. J. Immunol. 208 (5), 1170–1179. doi: 10.4049/jimmunol.2100912
Brauweiler, A. M., Bin, L., Kim, B. E., Oyoshi, M. K., Geha, R. S., Goleva, E., et al. (2013). Filaggrin-dependent secretion of sphingomyelinase protects against staphylococcal α-toxin-induced keratinocyte death. J. Allergy Clin. Immunol. 131 (2), 421–7.e1-2. doi: 10.1016/j.jaci.2012.10.030
Brauweiler, A. M., Goleva, E., Leung, D. Y. M. (2014). Th2 cytokines increase Staphylococcus aureus alpha toxin-induced keratinocyte death through the signal transducer and activator of transcription 6 (STAT6). J. Invest. Dermatol. 134 (8), 2114–2121. doi: 10.1038/jid.2014.43
Carlsson, M., Sjöholm, A. G., Eriksson, L., Thiel, S., Jensenius, J. C., Segelmark, M., et al. (2005). Deficiency of the mannan-binding lectin pathway of complement and poor outcome in cystic fibrosis: bacterial colonization may be decisive for a relationship. Clin. Exp. Immunol. 139 (2), 306–313. doi: 10.1111/j.1365-2249.2004.02690.x
Carter, T. C., Ye, Z., Ivacic, L. C., Budi, N., Rose, W. E., Shukla, S. K. (2020). Association of variants in selected genes mediating host immune response with duration of Staphylococcus aureus bacteremia. Genes Immun. 21 (4), 240–248. doi: 10.1038/s41435-020-0101-0
Castleman, M. J., Pokhrel, S., Triplett, K. D., Kusewitt, D. F., Elmore, B. O., Joyner, J. A., et al. (2018). Innate sex bias of Staphylococcus aureus skin infection is driven by α-hemolysin. J. Immunol. 200 (2), 657–668. doi: 10.4049/jimmunol.1700810
Chan, L. C., Chaili, S., Filler, S. G., Miller, L. S., Solis, N. V., Wang, H., et al. (2017). Innate immune memory contributes to host defense against recurrent skin and skin structure infections caused by methicillin-resistant staphylococcus aureus. Infect. Immun. 85 (2), e00876–16. doi: 10.1128/IAI.00876-16
Chang, C. H., Curtis, J. D., Maggi, L. B., Jr., Faubert, B., Villarino, A. V., O'Sullivan, D., et al. (2013). Posttranscriptional control of T cell effector function by aerobic glycolysis. Cell 153 (6), 1239–1251. doi: 10.1016/j.cell.2013.05.016
Chang, Y. L., Rossetti, M., Gjertson, D. W., Rubbi, L., Thompson, M., Montoya, D. J., et al. (2021). Human DNA methylation signatures differentiate persistent from resolving MRSA bacteremia. Proc. Natl. Acad. Sci. U.S.A. 118 (10), e2000663118. doi: 10.1073/pnas.2000663118
Chan, L. C., Rossetti, M., Miller, L. S., Filler, S. G., Johnson, C. W., Lee, H. K., et al. (2018). Protective immunity in recurrent Staphylococcus aureus infection reflects localized immune signatures and macrophage-conferred memory. Proc. Natl. Acad. Sci. U.S.A. 115 (47), E11111–E111e9. doi: 10.1073/pnas.1808353115
Chong, Y. P., Park, K. H., Kim, E. S., Kim, M. N., Kim, S. H., Lee, S. O., et al. (2014). Association of mannose-binding lectin 2 gene polymorphisms with persistent Staphylococcus aureus bacteremia. PloS One 9 (3), e89139. doi: 10.1371/journal.pone.0089139
Cho, S. H., Strickland, I., Tomkinson, A., Fehringer, A. P., Gelfand, E. W., Leung, D. Y. (2001). Preferential binding of Staphylococcus aureus to skin sites of Th2-mediated inflammation in a murine model. J. Invest. Dermatol. 116 (5), 658–663. doi: 10.1046/j.0022-202x.2001.01331.x
Chua, K. Y., Monk, I. R., Lin, Y. H., Seemann, T., Tuck, K. L., Porter, J. L., et al. (2014). Hyperexpression of α-hemolysin explains enhanced virulence of sequence type 93 community-associated methicillin-resistant. Staphylococcus aureus. BMC Microbiol. 14, 31. doi: 10.1186/1471-2180-14-31
Clegg, J., Soldaini, E., Bagnoli, F., McLoughlin, R. M. (2021). Targeting skin-resident memory T cells via vaccination to combat Staphylococcus aureus infections. Trends Immunol. 42 (1), 6–17. doi: 10.1016/j.it.2020.11.005
Collaborators, A. R. (2022). Global burden of bacterial antimicrobial resistance in 2019: a systematic analysis. Lancet 399 (10325), 629–655. doi: 10.1016/s0140-6736(21)02724-0
Cooper, A. J. R., Clegg, J., Cassidy, F. C., Hogan, A. E., McLoughlin, R. M. (2022). Human MAIT cells respond to staphylococcus aureus with enhanced anti-bacterial activity. Microorganisms 10 (1), 148. doi: 10.3390/microorganisms10010148
Corbett, A. J., Eckle, S. B., Birkinshaw, R. W., Liu, L., Patel, O., Mahony, J., et al. (2014). T-Cell activation by transitory neo-antigens derived from distinct microbial pathways. Nature 509 (7500), 361–365. doi: 10.1038/nature13160
Cox, J. R., Cruickshank, S. M., Saunders, A. E. (2021). Maintenance of barrier tissue integrity by unconventional lymphocytes. Front. Immunol. 12, 670471. doi: 10.3389/fimmu.2021.670471
Craven, R. R., Gao, X., Allen, I. C., Gris, D., Bubeck Wardenburg, J., McElvania-Tekippe, E., et al. (2009). Staphylococcus aureus alpha-hemolysin activates the NLRP3-inflammasome in human and mouse monocytic cells. PloS One 4 (10), e7446. doi: 10.1371/journal.pone.0007446
Cui, L., Neoh, H. M., Iwamoto, A., Hiramatsu, K. (2012). Coordinated phenotype switching with large-scale chromosome flip-flop inversion observed in bacteria. Proc. Natl. Acad. Sci. U. S. A. 109 (25), E1647–E1656. doi: 10.1073/pnas.1204307109
Cyr, D. D., Allen, A. S., Du, G. J., Ruffin, F., Adams, C., Thaden, J. T., et al. (2017). Evaluating genetic susceptibility to Staphylococcus aureus bacteremia in African americans using admixture mapping. Genes Immun. 18 (2), 95–99. doi: 10.1038/gene.2017.6
de Jong, N. W. M., van Kessel, K. P. M., van Strijp, J. A. G. (2019). Immune evasion by staphylococcus aureus. Microbiol. Spectr. 7 (2), 1–27. doi: 10.1128/microbiolspec.GPP3-0061-2019
DeLorenze, G. N., Nelson, C. L., Scott, W. K., Allen, A. S., Ray, G. T., Tsai, A. L., et al. (2016). Polymorphisms in HLA class II genes are associated with susceptibility to Staphylococcus aureus infection in a white population. J. Infect. Dis. 213 (5), 816–823. doi: 10.1093/infdis/jiv483
de Mesy Bentley, K. L., MacDonald, A., Schwarz, E. M., Oh, I. (2018). Chronic osteomyelitis with staphylococcus aureus deformation in submicron canaliculi of osteocytes: A case report. JBJS Case Connect. 8 (1), e8. doi: 10.2106/JBJS.CC.17.00154
de Mesy Bentley, K. L., Trombetta, R., Nishitani, K., Bello-Irizarry, S. N., Ninomiya, M., Zhang, L., et al. (2017). Evidence of staphylococcus aureus deformation, proliferation, and migration in canaliculi of live cortical bone in murine models of osteomyelitis. J. Bone Miner Res. 32 (5), 985–990. doi: 10.1002/jbmr.3055
Eckle, S. B., Corbett, A. J., Keller, A. N., Chen, Z., Godfrey, D. I., Liu, L., et al. (2015). Recognition of vitamin b precursors and byproducts by mucosal associated invariant T cells. J. Biol. Chem. 290 (51), 30204–30211. doi: 10.1074/jbc.R115.685990
Feinberg, H., Rowntree, T. J., Tan, S. L., Drickamer, K., Weis, W. I., Taylor, M. E. (2013). Common polymorphisms in human langerin change specificity for glycan ligands. J. Biol. Chem. 288 (52), 36762–36771. doi: 10.1074/jbc.M113.528000
Forbes-Blom, E., Camberis, M., Prout, M., Tang, S. C., Le Gros, G. (2012). Staphylococcal-derived superantigen enhances peanut induced Th2 responses in the skin. Clin. Exp. Allergy 42 (2), 305–314. doi: 10.1111/j.1365-2222.2011.03861.x
Fowler, V. G., Allen, K. B., Moreira, E. D., Moustafa, M., Isgro, F., Boucher, H. W., et al. (2013). Effect of an investigational vaccine for preventing Staphylococcus aureus infections after cardiothoracic surgery: a randomized trial. JAMA 309 (13), 1368–1378. doi: 10.1001/jama.2013.3010
Fritz, S. A., Tiemann, K. M., Hogan, P. G., Epplin, E. K., Rodriguez, M., Al-Zubeidi, D. N., et al. (2013). A serologic correlate of protective immunity against community-onset Staphylococcus aureus infection. Clin. Infect. Dis. 56 (11), 1554–1561. doi: 10.1093/cid/cit123
Fuller, J. R., Vitko, N. P., Perkowski, E. F., Scott, E., Khatri, D., Spontak, J. S., et al. (2011). Identification of a lactate-quinone oxidoreductase in Staphylococcus aureus that is essential for virulence. Front. Cell Infect. Microbiol. 1, 19. doi: 10.3389/fcimb.2011.00019
Gaborit, B. J., Roquilly, A., Louvet, C., Sadek, A., Tessoulin, B., Broquet, A., et al. (2020). Regulatory T cells expressing tumor necrosis factor receptor type 2 play a major role in CD4+ T-cell impairment during sepsis. J. Infect. Dis. 222 (7), 1222–1234. doi: 10.1093/infdis/jiaa225
Gabryszewski, S. J., Wong Fok Lung, T., Annavajhala, M. K., Tomlinson, K. L., Riquelme, S. A., Khan, I. N., et al. (2019). Metabolic adaptation in methicillin-resistant Staphylococcus aureus pneumonia. Am. J. Respir. Cell Mol. Biol. 61 (2), 185–197. doi: 10.1165/rcmb.2018-0389OC
Gao, W., Monk, I. R., Tobias, N. J., Gladman, S. L., Seemann, T., Stinear, T. P., et al. (2015). Large Tandem chromosome expansions facilitate niche adaptation during persistent infection with drug-resistant staphylococcus aureus. Microb. Genom. 1 (2), e000026. doi: 10.1099/mgen.0.000026
Gao, Y. L., Yao, Y., Zhang, X., Chen, F., Meng, X. L., Chen, X. S., et al. (2022). Regulatory T cells: Angels or demons in the pathophysiology of sepsis? Front. Immunol. 13, 829210. doi: 10.3389/fimmu.2022.829210
Gasch, O., Camoez, M., Dominguez, M. A., Padilla, B., Pintado, V., Almirante, B., et al. (2013). Predictive factors for mortality in patients with methicillin-resistant Staphylococcus aureus bloodstream infection: impact on outcome of host, microorganism and therapy. Clin. Microbiol. Infect. 19 (11), 1049–1057. doi: 10.1111/1469-0691.12108
Gillet, Y., Issartel, B., Vanhems, P., Fournet, J. C., Lina, G., Bes, M., et al. (2002). Association between Staphylococcus aureus strains carrying gene for panton-valentine leukocidin and highly lethal necrotising pneumonia in young immunocompetent patients. Lancet 359 (9308), 753–759. doi: 10.1016/s0140-6736(02)07877-7
Giulieri, S. G., Guérillot, R., Duchene, S., Hachani, A., Daniel, D., Seemann, T., et al. (2022). Niche-specific genome degradation and convergent evolution shaping staphylococcus aureus adaptation during severe infections. Elife 11, e77195. doi: 10.7554/eLife.77195.sa2
Goodyear, C. S., Silverman, G. J. (2003). Death by a b cell superantigen: In vivo VH-targeted apoptotic supraclonal b cell deletion by a staphylococcal toxin. J. Exp. Med. 197 (9), 1125–1139. doi: 10.1084/jem.20020552
Gubbay, J. B., Gosbell, I. B., Barbagiannakos, T., Vickery, A. M., Mercer, J. L., Watson, M. (2008). Clinical features, epidemiology, antimicrobial resistance, and exotoxin genes (including that of panton-valentine leukocidin) of gentamicin-susceptible methicillin-resistant Staphylococcus aureus (GS-MRSA) isolated at a paediatric teaching hospital in new south Wales, Australia. Pathology 40 (1), 64–71. doi: 10.1080/00313020701716276
Guérillot, R., Kostoulias, X., Donovan, L., Li, L., Carter, G. P., Hachani, A., et al. (2019). Unstable chromosome rearrangements in Staphylococcus aureus cause phenotype switching associated with persistent infections. Proc. Natl. Acad. Sci. U.S.A. 116 (40), 20135–20140. doi: 10.1073/pnas.1904861116
Hamill, R. J., Vann, J. M., Proctor, R. A. (1986). Phagocytosis of Staphylococcus aureus by cultured bovine aortic endothelial cells: model for postadherence events in endovascular infections. Infect. Immun. 54 (3), 833–836. doi: 10.1128/iai.54.3.833-836.1986
Hannaway, R. F., Wang, X., Schneider, M., Slow, S., Cowan, J., Brockway, B., et al. (2020). Mucosal-associated invariant T cells and Vδ2(+) γδ T cells in community acquired pneumonia: association of abundance in sputum with clinical severity and outcome. Clin. Exp. Immunol. 199 (2), 201–215. doi: 10.1111/cei.13377
Heim, C. E., Bosch, M. E., Yamada, K. J., Aldrich, A. L., Chaudhari, S. S., Klinkebiel, D., et al. (2020). Lactate production by Staphylococcus aureus biofilm inhibits HDAC11 to reprogramme the host immune response during persistent infection. Nat. Microbiol. 5 (10), 1271–1284. doi: 10.1038/s41564-020-0756-3
Heim, C. E., Vidlak, D., Kielian, T. (2015). Interleukin-10 production by myeloid-derived suppressor cells contributes to bacterial persistence during Staphylococcus aureus orthopedic biofilm infection. J. Leukoc. Biol. 98 (6), 1003–1013. doi: 10.1189/jlb.4VMA0315-125RR
Heim, C. E., Vidlak, D., Scherr, T. D., Kozel, J. A., Holzapfel, M., Muirhead, D. E., et al. (2014). Myeloid-derived suppressor cells contribute to Staphylococcus aureus orthopedic biofilm infection. J. Immunol. 192 (8), 3778–3792. doi: 10.4049/jimmunol.1303408
Hendriks, A., Mnich, M. E., Clemente, B., Cruz, A. R., Tavarini, S., Bagnoli, F., et al. (2021). Staphylococcus aureus-specific tissue-resident memory CD4(+) T cells are abundant in healthy human skin. Front. Immunol. 12, 642711. doi: 10.3389/fimmu.2021.642711
Hendriks, A., van Dalen, R., Ali, S., Gerlach, D., van der Marel, G. A., Fuchsberger, F. F., et al. (2021). Impact of glycan linkage to Staphylococcus aureus wall teichoic acid on langerin recognition and langerhans cell activation. ACS Infect. Dis. 7 (3), 624–635. doi: 10.1021/acsinfecdis.0c00822
Hersch, S. J., Navarre, W. W. (2020). The salmonella LysR family regulator RipR activates the SPI-13-Encoded itaconate degradation cluster. Infect. Immun. 88 (10), e00303-20. doi: 10.1128/IAI.00303-20
Hirai, H., Zhang, P., Dayaram, T., Hetherington, C. J., Mizuno, S., Imanishi, J., et al. (2006). C/EBPbeta is required for 'emergency' granulopoiesis. Nat. Immunol. 7 (7), 732–739. doi: 10.1038/ni1354
Holzinger, D., Gieldon, L., Mysore, V., Nippe, N., Taxman, D. J., Duncan, J. A., et al. (2012). Staphylococcus aureus panton-valentine leukocidin induces an inflammatory response in human phagocytes via the NLRP3 inflammasome. J. Leukoc. Biol. 92 (5), 1069–1081. doi: 10.1189/jlb.0112014
Hooftman, A., Angiari, S., Hester, S., Corcoran, S. E., Runtsch, M. C., Ling, C., et al. (2020). The immunomodulatory metabolite itaconate modifies NLRP3 and inhibits inflammasome activation. Cell Metab. 32 (3), 468–478.e7. doi: 10.1016/j.cmet.2020.07.016
Hos, N. J., Rieg, S., Kern, W. V., Jonas, D., Fowler, V. G., Higgins, P. G., et al. (2015). Amino acid alterations in fibronectin binding protein a (FnBPA) and bacterial genotype are associated with cardiac device related infection in Staphylococcus aureus bacteraemia. J. Infect. 70 (2), 153–159. doi: 10.1016/j.jinf.2014.09.005
Hu, Z. I., Link, V. M., Lima-Junior, D. S., Delaleu, J., Bouladoux, N., Han, S.-J., et al. (2022). Immune checkpoint inhibitors unleash pathogenic immune responses against the microbiota. Proc. Natl. Acad. Sci. 119 (26), e2200348119. doi: 10.1073/pnas.2200348119
Humphreys, H., Fitzpatick, F., Harvey, B. J. (2015). Gender differences in rates of carriage and bloodstream infection caused by methicillin-resistant staphylococcus aureus: Are they real, do they matter and why? Clin. Infect. Diseases. 61 (11), 1708–1714. doi: 10.1093/cid/civ576
Humphreys, H., Keane, C. T., Hone, R., Pomeroy, H., Russell, R. J., Arbuthnott, J. P., et al. (1989). Enterotoxin production by Staphylococcus aureus isolates from cases of septicaemia and from healthy carriers. J. Med. Microbiol. 28 (3), 163–172. doi: 10.1099/00222615-28-3-163
Inoshima, N., Wang, Y., Bubeck Wardenburg, J. (2012). Genetic requirement for ADAM10 in severe Staphylococcus aureus skin infection. J. Invest. Dermatol. 132 (5), 1513–1516. doi: 10.1038/jid.2011.462
Jacobsson, G., Colque-Navarro, P., Gustafsson, E., Andersson, R., Möllby, R. (2010). Antibody responses in patients with invasive Staphylococcus aureus infections. Eur. J. Clin. Microbiol. Infect. Dis. 29 (6), 715–725. doi: 10.1007/s10096-010-0919-x
Jahamy, H., Ganga, R., Al Raiy, B., Shemes, S., Nagappan, V., Sharma, M., et al. (2008). Staphylococcus aureus skin/soft-tissue infections: the impact of SCCmec type and panton-valentine leukocidin. Scand. J. Infect. Dis. 40 (8), 601–606. doi: 10.1080/00365540701877312
Jeon, J. H., Hong, C. W., Kim, E. Y., Lee, J. M. (2020). Current understanding on the metabolism of neutrophils. Immune Netw. 20 (6), e46. doi: 10.4110/in.2020.20.e46
Joshi, A., Pancari, G., Cope, L., Bowman, E. P., Cua, D., Proctor, R. A., et al. (2012). Immunization with Staphylococcus aureus iron regulated surface determinant b (IsdB) confers protection via Th17/IL17 pathway in a murine sepsis model. Hum. Vaccin Immunother. 8 (3), 336–346. doi: 10.4161/hv.18946
Kaasch, A. J., Barlow, G., Edgeworth, J. D., Fowler, V. G., Jr., Hellmich, M., Hopkins, S., et al. (2014). Staphylococcus aureus bloodstream infection: a pooled analysis of five prospective, observational studies. J. Infect. 68 (3), 242–251. doi: 10.1016/j.jinf.2013.10.015
Kallen, A. J., Mu, Y., Bulens, S., Reingold, A., Petit, S., Gershman, K., et al. (2010). Health care-associated invasive MRSA infections, 2005-2008. JAMA 304 (6), 641–648. doi: 10.1001/jama.2010.1115
Kaltsas, A., Guh, A., Mediavilla, J. R., Varshney, A. K., Robiou, N., Gialanellia, P., et al. (2011). Frequency of panton-valentine leukocidin-producing methicillin-sensitive Staphylococcus strains in patients with complicated skin and soft tissue infection in bronx, new york. J. Clin. Microbiol. 49 (8), 2992–2995. doi: 10.1128/JCM.00704-11
Kametani, Y., Wang, L., Koduka, K., Sato, T., Katano, I., Habu, S. (2008). Rapid histone deacetylation and transient HDAC association in the IL-2 promoter region of TSST-1-stimulated T cells. Immunol. Lett. 119 (1-2), 97–102. doi: 10.1016/j.imlet.2008.05.006
Kansal, R., Davis, C., Hansmann, M., Seymour, J., Parsonnet, J., Modern, P., et al. (2007). Structural and functional properties of antibodies to the superantigen TSST-1 and their relationship to menstrual toxic shock syndrome. J. Clin. Immunol. 27 (3), 327–338. doi: 10.1007/s10875-007-9072-4
Kates, S. L., Owen, J. R., Beck, C. A., Xie, C., Muthukrishnan, G., Daiss, J. L., et al. (2020). Lack of humoral immunity against glucosaminidase is associated with postoperative complications in Staphylococcus aureus osteomyelitis. J. Bone Joint Surg. Am. 102 (21), 1842–1848. doi: 10.2106/JBJS.20.00029
Klevens, R. M., Morrison, M. A., Nadle, J., Petit, S., Gershman, K., Ray, S., et al. (2007). Invasive methicillin-resistant Staphylococcus aureus infections in the united states. JAMA 298 (15), 1763–1771. doi: 10.1001/jama.298.15.1763
Kondoh, Y., Kawase, M., Kawakami, Y., Ohmori, S. (1992). Concentrations of d-lactate and its related metabolic intermediates in liver, blood, and muscle of diabetic and starved rats. Res. Exp. Med. (Berl). 192 (6), 407–414. doi: 10.1007/BF02576298
Kornberg, M. D., Bhargava, P., Kim, P. M., Putluri, V., Snowman, A. M., Putluri, N., et al. (2018). Dimethyl fumarate targets GAPDH and aerobic glycolysis to modulate immunity. Science 360 (6387), 449–453. doi: 10.1126/science.aan4665
Kriegeskorte, A., Grubmüller, S., Huber, C., Kahl, B. C., von Eiff, C., Proctor, R. A., et al. (2014). Staphylococcus aureus small colony variants show common metabolic features in central metabolism irrespective of the underlying auxotrophism. Front. Cell Infect. Microbiol. 4, 141. doi: 10.3389/fcimb.2014.00141
Lamagni, T. L., Potz, N., Powell, D., Pebody, R., Wilson, J., Duckworth, G. (2011). Mortality in patients with meticillin-resistant Staphylococcus aureus bacteraemia, England 2004-2005. J. Hosp Infect. 77 (1), 16–20. doi: 10.1016/j.jhin.2010.07.015
Lampropoulou, V., Sergushichev, A., Bambouskova, M., Nair, S., Vincent, E. E., Loginicheva, E., et al. (2016). Itaconate links inhibition of succinate dehydrogenase with macrophage metabolic remodeling and regulation of inflammation. Cell Metab. 24 (1), 158–166. doi: 10.1016/j.cmet.2016.06.004
Latham, T., Mackay, L., Sproul, D., Karim, M., Culley, J., Harrison, D. J., et al. (2012). Lactate, a product of glycolytic metabolism, inhibits histone deacetylase activity and promotes changes in gene expression. Nucleic Acids Res. 40 (11), 4794–4803. doi: 10.1093/nar/gks066
Laupland, K. B., Lyytikäinen, O., Søgaard, M., Kennedy, K. J., Knudsen, J. D., Ostergaard, C., et al. (2013). The changing epidemiology of Staphylococcus aureus bloodstream infection: a multinational population-based surveillance study. Clin. Microbiol. Infect. 19 (5), 465–471. doi: 10.1111/j.1469-0691.2012.03903.x
Lessa, F. C., Mu, Y., Davies, J., Murray, M., Lillie, M., Pearson, A., et al. (2010). Comparison of incidence of bloodstream infection with methicillin-resistant Staphylococcus aureus between England and united states, 2006-2007. Clin. Infect. Dis. 51 (8), 925–928. doi: 10.1086/656414
Li, Z., Beesetty, P., Gerges, G., Kleinhenz, M., Moore-Clingenpeel, M., Yang, C., et al. (2022). Impaired T-lymphocyte responses during childhood Staphylococcus aureus infection. J. Infect. Dis. 225 (1), 177–185. doi: 10.1093/infdis/jiab326
Lina, G., Piémont, Y., Godail-Gamot, F., Bes, M., Peter, M. O., Gauduchon, V., et al. (1999). Involvement of panton-valentine leukocidin-producing Staphylococcus aureus in primary skin infections and pneumonia. Clin. Infect. Dis. 29 (5), 1128–1132. doi: 10.1086/313461
Lower, S. K., Lamlertthon, S., Casillas-Ituarte, N. N., Lins, R. D., Yongsunthon, R., Taylor, E. S., et al. (2011). Polymorphisms in fibronectin binding protein a of Staphylococcus aureus are associated with infection of cardiovascular devices. Proc. Natl. Acad. Sci. U.S.A. 108 (45), 18372–18377. doi: 10.1073/pnas.1109071108
Lu, F., Chen, H., Hong, Y., Lin, Y., Liu, L., Wei, N., et al. (2021). A gain-of-function NLRP3 3'-UTR polymorphism causes miR-146a-mediated suppression of NLRP3 expression and confers protection against sepsis progression. Sci. Rep. 11 (1), 13300. doi: 10.1038/s41598-021-92547-8
Masters, E. A., de Mesy Bentley, K. L., Gill, A. L., Hao, S. P., Galloway, C. A., Salminen, A. T., et al. (2020). Identification of penicillin binding protein 4 (PBP4) as a critical factor for staphylococcus aureus bone invasion during osteomyelitis in mice. PloS Pathog. 16 (10), e1008988. doi: 10.1371/journal.ppat.1008988
Masters, E. A., Trombetta, R. P., de Mesy Bentley, K. L., Boyce, B. F., Gill, A. L., Gill, S. R., et al. (2019). Evolving concepts in bone infection: redefining "biofilm", "acute vs. chronic osteomyelitis", "the immune proteome" and "local antibiotic therapy". Bone Res. 7, 20. doi: 10.1038/s41413-019-0061-z
Mba Medie, F., Sharma-Kuinkel, B. K., Ruffin, F., Chan, L. C., Rossetti, M., Chang, Y. L., et al. (2019). Genetic variation of DNA methyltransferase-3A contributes to protection against persistent MRSA bacteremia in patients. Proc. Natl. Acad. Sci. U. S. A. 116 (40), 20087–20096. doi: 10.1073/pnas.1909849116
McAdam, P. R., Holmes, A., Templeton, K. E., Fitzgerald, J. R. (2011). Adaptive evolution of Staphylococcus aureus during chronic endobronchial infection of a cystic fibrosis patient. PloS One 6 (9), e24301. doi: 10.1371/journal.pone.0024301
McCormick, J. K., Yarwood, J. M., Schlievert, P. M. (2001). Toxic shock syndrome and bacterial superantigens: an update. Annu. Rev. Microbiol. 55, 77–104. doi: 10.1146/annurev.micro.55.1.77
McFadden, J. P., Noble, W. C., Camp, R. D. (1993). Superantigenic exotoxin-secreting potential of staphylococci isolated from atopic eczematous skin. Br. J. Dermatol. 128 (6), 631–632. doi: 10.1111/j.1365-2133.1993.tb00257.x
McFadden, B. A., Purohit, S. (1977). Itaconate, an isocitrate lyase-directed inhibitor in Pseudomonas indigofera. J. Bacteriol. 131 (1), 136–144. doi: 10.1128/jb.131.1.136-144.1977
McNeely, T. B., Shah, N. A., Fridman, A., Joshi, A., Hartzel, J. S., Keshari, R. S., et al. (2014). Mortality among recipients of the Merck V710 Staphylococcus aureus vaccine after postoperative s. aureus infections: an analysis of possible contributing host factors. Hum. Vaccin Immunother. 10 (12), 3513–3516. doi: 10.4161/hv.34407
Melehani, J. H., James, D. B., DuMont, A. L., Torres, V. J., Duncan, J. A. (2015). Staphylococcus aureus leukocidin A/B (LukAB) kills human monocytes via host NLRP3 and ASC when extracellular, but not intracellular. PLoS Pathog. 11 (6), e1004970. doi: 10.1371/journal.ppat.1004970
Messina, J. A., Thaden, J. T., Sharma-Kuinkel, B. K., Fowler, V. G., Jr. (2016). Impact of bacterial and human genetic variation on Staphylococcus aureus infections. PLoS Pathog. 12 (1), e1005330. doi: 10.1371/journal.ppat.1005330
Miller, L. S., Fowler, V. G., Shukla, S. K., Rose, W. E., Proctor, R. A. (2020). Development of a vaccine against Staphylococcus aureus invasive infections: Evidence based on human immunity, genetics and bacterial evasion mechanisms. FEMS Microbiol. Rev. 44 (1), 123–153. doi: 10.1093/femsre/fuz030
Moreau, K., Clemenceau, A., Le Moing, V., Messika-Zeitoun, D., Andersen, P. S., Bruun, N. E., et al. (2018). Human genetic susceptibility to native valve staphylococcus aureus endocarditis in patients with s. aureus bacteremia: Genome-wide association study. Front. Microbiol. 9, 640. doi: 10.3389/fmicb.2018.00640
Munckhof, W. J., Nimmo, G. R., Carney, J., Schooneveldt, J. M., Huygens, F., Inman-Bamber, J., et al. (2008). Methicillin-susceptible, non-multiresistant methicillin-resistant and multiresistant methicillin-resistant Staphylococcus aureus infections: a clinical, epidemiological and microbiological comparative study. Eur. J. Clin. Microbiol. Infect. Dis. 27 (5), 355–364. doi: 10.1007/s10096-007-0449-3
Nelson, C. L., Pelak, K., Podgoreanu, M. V., Ahn, S. H., Scott, W. K., Allen, A. S., et al. (2014). A genome-wide association study of variants associated with acquisition of Staphylococcus aureus bacteremia in a healthcare setting. BMC Infect. Dis. 14, 83. doi: 10.1186/1471-2334-14-83
Niemann, S., Nguyen, M. T., Eble, J. A., Chasan, A. I., Mrakovcic, M., Böttcher, R. T., et al. (2021). More is not always better-the double-headed role of fibronectin in Staphylococcus aureus host cell invasion. mBio 12 (5), e0106221. doi: 10.1128/mBio.01062-21
Nishitani, K., Ishikawa, M., Morita, Y., Yokogawa, N., Xie, C., de Mesy Bentley, K. L., et al. (2020). IsdB antibody-mediated sepsis following. S. aureus Surg. site infection. JCI Insight 5 (19), e141164. doi: 10.1172/jci.insight.141164
Nygaard, T. K., Pallister, K. B., DuMont, A. L., DeWald, M., Watkins, R. L., Pallister, E. Q., et al. (2012). Alpha-toxin induces programmed cell death of human T cells, b cells, and monocytes during USA300 infection. PloS One 7 (5), e36532. doi: 10.1371/journal.pone.0036532
O'Brien, E. C., McLoughlin, R. M. (2019). Considering the 'Alternatives' for next-generation anti-staphylococcus aureus vaccine development. Trends Mol. Med. 25 (3), 171–184. doi: 10.1016/j.molmed.2018.12.010
O'Neill, L. A., Kishton, R. J., Rathmell, J. (2016). A guide to immunometabolism for immunologists. Nat. Rev. Immunol. 16 (9), 553–565. doi: 10.1038/nri.2016.70
Oestergaard, L. B., Christiansen, M. N., Schmiegelow, M. D., Skov, R. L., Andersen, P. S., Petersen, A., et al. (2016). Familial clustering of Staphylococcus aureus bacteremia in first-degree relatives: A Danish nationwide cohort study. Ann. Intern. Med. 165 (6), 390–398. doi: 10.7326/M15-2762
Pokhrel, S., Triplett, K. D., Daly, S. M., Joyner, J. A., Sharma, G., Hathaway, H. J., et al. (2020). Complement receptor 3 contributes to the sexual dimorphism in neutrophil killing of. Staphylococcus aureus. J. Immunol. 205 (6), 1593–1600. doi: 10.4049/jimmunol.2000545
Proctor, R. A. (2012). Is there a future for a Staphylococcus aureus vaccine? Vaccine 30 (19), 2921–2927. doi: 10.1016/j.vaccine.2011.11.006
Proctor, R. A. (2021). Have we outlived the concept of commensalism for Staphylococcus aureus? Clin. Infect. Dis. 73 (1), e267–e2e9. doi: 10.1093/cid/ciaa1431
Proctor, R. A., Christman, G., Mosher, D. F. (1984). Fibronectin-induced agglutination of Staphylococcus aureus correlates with invasiveness. J. Lab. Clin. Med. 104 (4), 455–469.
Proctor, R. A., Kriegeskorte, A., Kahl, B. C., Becker, K., Löffler, B., Peters, G. (2014). Staphylococcus aureus small colony variants (SCVs): a road map for the metabolic pathways involved in persistent infections. Front. Cell. Infection Microbiol. 4. doi: 10.3389/fcimb.2014.00099
Proctor, R. A., van Langevelde, P., Kristjansson, M., Maslow, J. N., Arbeit, R. D. (1995). Persistent and relapsing infections associated with small-colony variants of Staphylococcus aureus. Clin. Infect. Dis. 20 (1), 95–102. doi: 10.1093/clinids/20.1.95
Proctor, R. A., von Eiff, C., Kahl, B. C., Becker, K., McNamara, P., Herrmann, M., et al. (2006). Small colony variants: a pathogenic form of bacteria that facilitates persistent and recurrent infections. Nat. Rev. Microbiol. 4 (4), 295–305. doi: 10.1038/nrmicro1384
Qin, W., Qin, K., Zhang, Y., Jia, W., Chen, Y., Cheng, B., et al. (2019). S-glycosylation-based cysteine profiling reveals regulation of glycolysis by itaconate. Nat. Chem. Biol. 15 (10), 983–991. doi: 10.1038/s41589-019-0323-5
Quinn, G. A., Cole, A. M. (2007). Suppression of innate immunity by a nasal carriage strain of Staphylococcus aureus increases its colonization on nasal epithelium. Immunology 122 (1), 80–89. doi: 10.1111/j.1365-2567.2007.02615.x
Rani, L., Singh, J., Sharma, A., Singh, H., Verma, I., Panda, N. K., et al. (2022). Anti-staphylococcal responses and their relationship with HLA-DR-DQ polymorphism in granulomatosis with polyangiitis: a preliminary evidence of association with disease outcome. Clin. Exp. Med. doi: 10.1007/s10238-022-00865-6
Rasigade, J. P., Sicot, N., Laurent, F., Lina, G., Vandenesch, F., Etienne, J. (2011). A history of panton-valentine leukocidin (PVL)-associated infection protects against death in PVL-associated pneumonia. Vaccine 29 (25), 4185–4186. doi: 10.1016/j.vaccine.2011.04.033
Ricciardi, B. F., Muthukrishnan, G., Masters, E., Ninomiya, M., Lee, C. C., Schwarz, E. M. (2018). Staphylococcus aureus evasion of host immunity in the setting of prosthetic joint infection: Biofilm and beyond. Curr. Rev. Musculoskelet Med. 11 (3), 389–400. doi: 10.1007/s12178-018-9501-4
Riquelme, S. A., Wong Fok Lung, T., Prince, A. (2020). Pulmonary pathogens adapt to immune signaling metabolites in the airway. Front. Immunol. 11, 385. doi: 10.3389/fimmu.2020.00385
Sanchez, M., Kolar, S. L., Müller, S., Reyes, C. N., Wolf, A. J., Ogawa, C., et al. (2017). O-Acetylation of peptidoglycan limits helper T cell priming and permits Staphylococcus aureus reinfection. Cell Host Microbe 22 (4), 543–51.e4. doi: 10.1016/j.chom.2017.08.008
Sandberg, J. K., Norrby-Teglund, A., Leeansyah, E. (2017). Bacterial deception of MAIT cells in a cloud of superantigen and cytokines. PloS Biol. 15 (7), e2003167. doi: 10.1371/journal.pbio.2003167
Sasikaran, J., Ziemski, M., Zadora, P. K., Fleig, A., Berg, I. A. (2014). Bacterial itaconate degradation promotes pathogenicity. Nat. Chem. Biol. 10 (5), 371–377. doi: 10.1038/nchembio.1482
Schilcher, K., Horswill, A. R. (2020). Staphylococcal biofilm development: Structure, regulation, and treatment strategies. Microbiol. Mol. Biol. Rev. 84 (3), e00026-19. doi: 10.1128/MMBR.00026-19
Scott, W. K., Medie, F. M., Ruffin, F., Sharma-Kuinkel, B. K., Cyr, D. D., Guo, S., et al. (2018). Human genetic variation in GLS2 is associated with development of complicated Staphylococcus aureus bacteremia. PloS Genet. 14 (10), e1007667. doi: 10.1371/journal.pgen.1007667
Seidl, K., Müller, S., François, P., Kriebitzsch, C., Schrenzel, J., Engelmann, S., et al. (2009). Effect of a glucose impulse on the CcpA regulon in Staphylococcus aureus. BMC Microbiol. 9, 95. doi: 10.1186/1471-2180-9-95
Shaler, C. R., Choi, J., Rudak, P. T., Memarnejadian, A., Szabo, P. A., Tun-Abraham, M. E., et al. (2017). MAIT cells launch a rapid, robust and distinct hyperinflammatory response to bacterial superantigens and quickly acquire an anergic phenotype that impedes their cognate antimicrobial function: Defining a novel mechanism of superantigen-induced immunopathology and immunosuppression. PloS Biol. 15 (6), e2001930. doi: 10.1371/journal.pbio.2001930
Shallcross, L. J., Fragaszy, E., Johnson, A. M., Hayward, A. C. (2013). The role of the panton-valentine leucocidin toxin in staphylococcal disease: a systematic review and meta-analysis. Lancet Infect. Dis. 13 (1), 43–54. doi: 10.1016/S1473-3099(12)70238-4
Sharma-Kuinkel, B. K., Tkaczyk, C., Bonnell, J., Yu, L., Tovchigrechko, A., Tabor, D. E., et al. (2019). Associations of pathogen-specific and host-specific characteristics with disease outcome in patients with Staphylococcus aureus bacteremic pneumonia. Clin. Transl. Immunol. 8 (7), e01070. doi: 10.1002/cti2.1070
Sherchand, S. P., Adhikari, R. P., Muthukrishnan, G., Kanipakala, T., Owen, J. R., Xie, C., et al. (2022). Evidence of neutralizing and non-neutralizing anti-glucosaminidase antibodies in patients with s. aureus osteomyelitis and their association with clinical outcome following surgery in a clinical pilot. Front. Cell. Infection Microbiol. 12. doi: 10.3389/fcimb.2022.876898
Singh, S., Singh, P. K., Jha, A., Naik, P., Joseph, J., Giri, S., et al. (2021). Integrative metabolomics and transcriptomics identifies itaconate as an adjunct therapy to treat ocular bacterial infection. Cell Rep. Med. 2 (5), 100277. doi: 10.1016/j.xcrm.2021.100277
Somerville, G. A., Proctor, R. A. (2009). At The crossroads of bacterial metabolism and virulence factor synthesis in staphylococci. Microbiol. Mol. Biol. Rev. 73 (2), 233–248. doi: 10.1128/MMBR.00005-09
Soong, G., Chun, J., Parker, D., Prince, A. (2012). Staphylococcus aureus activation of caspase 1/calpain signaling mediates invasion through human keratinocytes. J. Infect. Dis. 205 (10), 1571–1579. doi: 10.1093/infdis/jis244
Soudais, C., Samassa, F., Sarkis, M., Le Bourhis, L., Bessoles, S., Blanot, D., et al. (2015). In vitro and In vivo analysis of the gram-negative bacteria-derived riboflavin precursor derivatives activating mouse MAIT cells. J. Immunol. 194 (10), 4641–4649. doi: 10.4049/jimmunol.1403224
Spaan, A. N., Neehus, A. L., Laplantine, E., Staels, F., Ogishi, M., Seeleuthner, Y., et al. (2022). Human OTULIN haploinsufficiency impairs cell-intrinsic immunity to staphylococcal α-toxin. Science 376 (6599), eabm6380. doi: 10.1126/science.abm6380
Speziale, P., Pietrocola, G. (2021). Staphylococcus aureus induces neutrophil extracellular traps (NETs) and neutralizes their bactericidal potential. Comput. Struct. Biotechnol. J. 19, 3451–3457. doi: 10.1016/j.csbj.2021.06.012
Stapleton, N. M., Andersen, J. T., Stemerding, A. M., Bjarnarson, S. P., Verheul, R. C., Gerritsen, J., et al. (2011). Competition for FcRn-mediated transport gives rise to short half-life of human IgG3 and offers therapeutic potential. Nat. Commun. 2, 599. doi: 10.1038/ncomms1608
Stensen, D. B., Småbrekke, L., Olsen, K., Grimnes, G., Nielsen, C. S., Ericson, J. U., et al. (2022). Circulating sex-steroids and Staphylococcus aureus nasal carriage in a general male population. Epidemiol. Infect. 150, e93. doi: 10.1017/S0950268822000735
Stockland, A. E., San Clemente, C. L. (1969). Multiple forms of lactate dehydrogenase in. Staphylococcus aureus. J. Bacteriol. 100 (1), 347–353. doi: 10.1128/jb.100.1.347-353.1969
Stojadinovic, O., Yin, N., Lehmann, J., Pastar, I., Kirsner, R. S., Tomic-Canic, M. (2013). Increased number of langerhans cells in the epidermis of diabetic foot ulcers correlates with healing outcome. Immunol. Res. 57 (1-3), 222–228. doi: 10.1007/s12026-013-8474-z
Suligoy, C. M., Lattar, S. M., Noto Llana, M., González, C. D., Alvarez, L. P., Robinson, D. A., et al. (2018). Mutation of Agr is associated with the adaptation of Staphylococcus aureus to the host during chronic osteomyelitis. Front. Cell Infect. Microbiol. 8, 18. doi: 10.3389/fcimb.2018.00018
Sunden-Cullberg, J., Nilsson, A., Inghammar, M. (2020). Sex-based differences in ED management of critically ill patients with sepsis: a nationwide cohort study. Intensive Care Med. 46 (4), 727–736. doi: 10.1007/s00134-019-05910-9
Sun, A., Zhang, H., Pang, F., Niu, G., Chen, J., Chen, F., et al. (2018). Essential genes of the macrophage response to Staphylococcus aureus exposure. Cell Mol. Biol. Lett. 23, 25. doi: 10.1186/s11658-018-0090-4
Teymournejad, O., Montgomery, C. P. (2021). Evasion of immunological memory by s. aureus infection: Implications for vaccine design. Front. Immunol. 12, 633672. doi: 10.3389/fimmu.2021.633672
Thammavongsa, V., Rauch, S., Kim, H. K., Missiakas, D. M., Schneewind, O. (2015). Protein a-neutralizing monoclonal antibody protects neonatal mice against. Staphylococcus aureus. Vaccine 33 (4), 523–526. doi: 10.1016/j.vaccine.2014.11.051
Tomlinson, K. L., Prince, A. S., Wong Fok Lung, T. (2021). Immunometabolites drive bacterial adaptation to the airway. Front. Immunol. 12, 790574. doi: 10.3389/fimmu.2021.790574
Tong, S. Y., Davis, J. S., Eichenberger, E., Holland, T. L., Fowler, V. G., Jr. (2015). Staphylococcus aureus infections: epidemiology, pathophysiology, clinical manifestations, and management. Clin. Microbiol. Rev. 28 (3), 603–661. doi: 10.1128/CMR.00134-14
Traber, K. E., Lee, E., Benson, S., Corrigan, R., Cantera, M., Shopsin, B., et al. (2008). Agr function in clinical staphylococcus aureus isolates. Microbiol. (Reading) 154 (Pt 8), 2265–2274. doi: 10.1099/mic.0.2007/011874-0
Tuchscherr, L., Löffler, B., Proctor, R. A. (2020). Persistence of staphylococcus aureus: Multiple metabolic pathways impact the expression of virulence factors in small-colony variants (SCVs). Front. Microbiol. 11, 1028. doi: 10.3389/fmicb.2020.01028
Uebele, J., Habenicht, K., Ticha, O., Bekeredjian-Ding, I. (2020). Staphylococcus aureus protein a induces human regulatory T cells through interaction with antigen-presenting cells. Front. Immunol. 11. doi: 10.3389/fimmu.2020.581713
van Dalen, R., Fuchsberger, F. F., Rademacher, C., van Strijp, J. A. G., van Sorge, N. M. (2020). A common genetic variation in langerin (CD207) compromises cellular uptake of Staphylococcus aureus. J. Innate Immun. 12 (2), 191–200. doi: 10.1159/000500547
Vandenesch, F., Lina, G., Henry, T. (2012). Staphylococcus aureus hemolysins, bi-component leukocidins, and cytolytic peptides: a redundant arsenal of membrane-damaging virulence factors? Front. Cell Infect. Microbiol. 2, 12. doi: 10.3389/fcimb.2012.00012
Varrone, J. J., de Mesy Bentley, K. L., Bello-Irizarry, S. N., Nishitani, K., Mack, S., Hunter, J. G., et al. (2014). Passive immunization with anti-glucosaminidase monoclonal antibodies protects mice from implant-associated osteomyelitis by mediating opsonophagocytosis of staphylococcus aureus megaclusters. J. Orthop Res. 32 (10), 1389–1396. doi: 10.1002/jor.22672
von Eiff, C., Becker, K., Metze, D., Lubritz, G., Hockmann, J., Schwarz, T., et al. (2001). Intracellular persistence of Staphylococcus aureus small-colony variants within keratinocytes: a cause for antibiotic treatment failure in a patient with darier's disease. Clin. Infect. Dis. 32 (11), 1643–1647. doi: 10.1086/320519
Wang, H., Fedorov, A. A., Fedorov, E. V., Hunt, D. M., Rodgers, A., Douglas, H. L., et al. (2019). An essential bifunctional enzyme in Mycobacterium tuberculosis for itaconate dissimilation and leucine catabolism. Proc. Natl. Acad. Sci. U. S. A. 116 (32), 15907–15913. doi: 10.1073/pnas.1906606116
Wertheim, H. F., Vos, M. C., Ott, A., van Belkum, A., Voss, A., Kluytmans, J. A., et al. (2004). Risk and outcome of nosocomial Staphylococcus aureus bacteraemia in nasal carriers versus non-carriers. Lancet 364 (9435), 703–705. doi: 10.1016/s0140-6736(04)16897-9
Wickersham, M., Wachtel, S., Wong Fok Lung, T., Soong, G., Jacquet, R., Richardson, A., et al. (2017). Metabolic stress drives keratinocyte defenses against Staphylococcus aureus infection. Cell Rep. 18 (11), 2742–2751. doi: 10.1016/j.celrep.2017.02.055
Wong Fok Lung, T., Monk, I. R., Acker, K. P., Mu, A., Wang, N., Riquelme, S. A., et al. (2020). Staphylococcus aureus small colony variants impair host immunity by activating host cell glycolysis and inducing necroptosis. Nat. Microbiol. 5 (1), 141–153. doi: 10.1038/s41564-019-0597-0
Xiong, Y. Q., Sharma-Kuinkel, B. K., Casillas-Ituarte, N. N., Fowler, V. G., Jr., Rude, T., DiBartola, A. C., et al. (2015). Endovascular infections caused by methicillin-resistant Staphylococcus aureus are linked to clonal complex-specific alterations in binding and invasion domains of fibronectin-binding protein a as well as the occurrence of fnbB. Infect. Immun. 83 (12), 4772–4780. doi: 10.1128/IAI.01074-15
Yamada, K. J., Heim, C. E., Xi, X., Attri, K. S., Wang, D., Zhang, W., et al. (2020). Monocyte metabolic reprogramming promotes pro-inflammatory activity and Staphylococcus aureus biofilm clearance. PloS Pathog. 16 (3), e1008354. doi: 10.1371/journal.ppat.1008354
Yeaman, M. R., Filler, S. G., Chaili, S., Barr, K., Wang, H., Kupferwasser, D., et al. (2014). Mechanisms of NDV-3 vaccine efficacy in MRSA skin versus invasive infection. Proc. Natl. Acad. Sci. U. S. A. 111 (51), E5555–E5563. doi: 10.1073/pnas.1415610111
Yeaman, M. R., Hennessey, J. P., Jr (2017). Innovative approaches to improve anti-infective vaccine efficacy. Annu. Rev. Pharmacol. Toxicol. 57, 189–222. doi: 10.1146/annurev-pharmtox-010716-104718
Young, B. C., Wu, C. H., Gordon, N. C., Cole, K., Price, J. R., Liu, E., et al. (2017). Severe infections emerge from commensal bacteria by adaptive evolution. Elife 6, e30637. doi: 10.7554/eLife.30637.066
Zhang, Q., Cao, X. (2019). Epigenetic regulation of the innate immune response to infection. Nat. Rev. Immunol. 19 (7), 417–432. doi: 10.1038/s41577-019-0151-6
Zhang, Y., Qin, W., Liu, D., Liu, Y., Wang, C. (2021). Chemoproteomic profiling of itaconations in. Salmonella. Chem. Sci. 12 (17), 6059–6063. doi: 10.1039/D1SC00660F
Keywords: S. aureus, immunity, vaccine, immunometabolites, human genetics, human epigenetics
Citation: Wong Fok Lung T, Chan LC, Prince A, Yeaman MR, Archer NK, Aman MJ and Proctor RA (2022) Staphylococcus aureus adaptive evolution: Recent insights on how immune evasion, immunometabolic subversion and host genetics impact vaccine development. Front. Cell. Infect. Microbiol. 12:1060810. doi: 10.3389/fcimb.2022.1060810
Received: 03 October 2022; Accepted: 16 November 2022;
Published: 27 December 2022.
Edited by:
Edwin Leeansyah, Shenzhen International Graduate School, Tsinghua University, ChinaReviewed by:
Wilmara Salgado Pabón, University of Wisconsin-Madison, United StatesCopyright © 2022 Wong Fok Lung, Chan, Prince, Yeaman, Archer, Aman and Proctor. This is an open-access article distributed under the terms of the Creative Commons Attribution License (CC BY). The use, distribution or reproduction in other forums is permitted, provided the original author(s) and the copyright owner(s) are credited and that the original publication in this journal is cited, in accordance with accepted academic practice. No use, distribution or reproduction is permitted which does not comply with these terms.
*Correspondence: Richard A. Proctor, cmFwQHdpc2MuZWR1
†These authors have contributed equally to this work
Disclaimer: All claims expressed in this article are solely those of the authors and do not necessarily represent those of their affiliated organizations, or those of the publisher, the editors and the reviewers. Any product that may be evaluated in this article or claim that may be made by its manufacturer is not guaranteed or endorsed by the publisher.
Research integrity at Frontiers
Learn more about the work of our research integrity team to safeguard the quality of each article we publish.