- 1Aix Marseille Univ, Institut de recherche pour le développement (IRD), Assistance Publique-Hôpitaux de Marseille (APHM), Microbes, Evolution, Phylogénie et Infection (MEPHI), Faculté de Médecine et de Pharmacie, Marseille, France
- 2Institut hospitalo-universitaire (IHU) Méditerranée Infection, Marseille, France
- 3Service de Maladies infectieuses et tropicales, Centre Hospitalier de Quimper, Quimper, France
- 4Department of Thoracic Oncology, Pleural Diseases, and Interventional Pulmonology, North University Hospital, Marseille, France
- 5Aix-Marseille University, Marseille, France
Background: Carbapenemase-producing Enterobacteriaceae (CPE) represent an increasing threat to public health, especially in hospitals.
Objectives: To investigate an outbreak of CPE in a thoracic-oncology unit by using whole genome sequencing (WGS) and to describe the control measures taken to limit the epidemic, including fecal microbiota transplantation (FMT).
Methods: A retrospective study between December 2016 and October 2017 was performed to investigate an outbreak of CPE in a thoracic-oncology unit at the North Hospital in Marseille, France. The isolates were identified, and antimicrobial susceptibility tests were performed. All CPE were sequenced using MiSeq and/or MinIon technologies. Nucleotide variations between plasmids and similarity within the same species were investigated. The origin of this outbreak, its spread, and the decolonization of patients in the ward were also studied.
Results: Four Citrobacter freundii, one Enterobacter cloacae and four E. hormaechei OXA-48 carbapenemase producers were isolated in eight patients hospitalized the same year in a thoracic-oncology ward. The blaOXA-48 gene was present in a Tn1999.2 transposon located in IncL/M plasmids, with single nucleotide variants (SNV) ranging from 0 to 5. All C. freundii strains belonged to the same ST22 and had more than 99.6% similarity between them. Two strains of E. hormaechei ST1007 were almost identical at 99.98%, while the others belonged to a different ST (ST98, ST114, ST133). No single source was identified. FMT resulted in decolonization in 4/6 patients.
Conclusions: WGS demonstrated the dissemination of the blaOXA-48 gene by both clonal (C. freundii ST22 and E. hormaechei ST1007) and plasmid spread (pOXA-48 IncL/M). The origin of this outbreak appeared to be both external and internal to the ward. This evidence of cross-infection supports the urgent need for the implementation of infection control measures to prevent CPE dissemination.
Introduction
Carbapenems are broad-spectrum antibiotics that play a major role in the treatment of severe infections caused by Gram-negative bacteria. The global spread of carbapenem-resistant Enterobacteriaceae is becoming a public health issue (Jamal et al., 2020). The rise of carbapenem resistance in Enterobacteriaceae is mainly due to the acquisition of carbapenem-hydrolyzing enzymes (carbapenemases) (Tilahun et al., 2021). Genes encoding carbapenemases may be incorporated into the bacterial chromosome, but are mostly located on mobile elements, such as plasmids or transposons that are transferable between bacterial strains and species (San Millan, 2018). Hence, clinical outbreaks are usually complex, involving various factors of gene propagation by clones, plasmids, or transposons (Brehony et al., 2019).
Carbapenemase type OXA-48 first appeared in the mid-2000s in Turkey and has since been found in many European countries and worldwide (Hidalgo et al., 2019). In France, it is the most common enzyme among carbapenemase-producing Enterobacteriaceae (CPE) (Emeraud et al., 2020). The blaOXA-48 gene is thought to originate from the chromosome of environmental Shewanella strains (Tacão et al., 2018). Its rapid dissemination between species is due to its nesting in a transposon (Tn1999) that is carried primarily by IncL/M type plasmids (Shankar et al., 2020).
Controlling outbreaks in hospital wards is necessary to limit the spread of multidrug-resistant bacteria. The colonization of patients by CPE can interfere with proper care. CPE colonization can also have an impact on the initiation of chemotherapy in cancer patients, as it has been associated with a lower survival rate in patients undergoing induction chemotherapy (Ballo et al., 2019). Thus, a strategy to restore a healthy gut microbiota and to eliminate the CPE reservoir such as fecal microbiota transplantation (FMT) has been implemented. FMT is a validated therapy that is highly effective against recurrent Clostridium difficile infections (Yoon et al., 2019). In addition, recent studies have shown that FMT was an efficient strategy for sustained CPE eradication. (Decraene et al., 2018; Saïdani et al., 2019). However, there could be other reservoirs of CPE. For instance, the survival of CPE on surfaces also allows the resumption of epidemics months after the initial case (Mateos et al., 2020).
During nosocomial outbreaks, the transmission of pathogens in a ward can be studied by whole genome sequencing of the bacteria of interest, a highly discriminatory typing technique. The genetic relationship between strains, as well as the presence of antibiotic resistance genes and their genetic support, can be determined very accurately and completely (Jamal et al., 2020). However, short-read sequencing (Illumina technology) is not always suitable for locating antibiotic resistance genes on plasmids, whereas long-read sequencing (Nanopore technology) can solve this problem. Therefore, a hybrid assembly of both highly accurate Illumina data and Nanopore data allow the reconstitution of plasmids and their in-depth analysis (George et al., 2017). In our study, these technologies were used to investigate the source and mode of dissemination of the blaOXA-48 gene in different Enterobacteriaceae during an outbreak in an adult thoracic-oncology unit in Marseille, France. The management of this epidemic by clinicians and the decolonization of patients are also described.
Methods
Study design and bacterial strains
This retrospective study was conducted to investigate an outbreak of CPE in six patients that occurred between October and December 2017 in a thoracic-oncology center at the North hospital of Marseille, France. To perform this investigation, we also extracted medical records from two other patients that had been positive for CPE in the same ward, one in December 2016 and one between August and October 2017 (Figure 1; Table 1). From these eight patients, nine strains were isolated, including eight from rectal swabs and one from a urine sample. Patient 2 carried two different CPE isolates. An epidemiological investigation was conducted to understand the source of this outbreak. The mode of dissemination of the blaOXA-48 gene and the relatedness between isolates were studied using whole-genome sequencing.
Decolonization by fecal microbiota transplantation
As previously noted, FMT was performed in six patients (Patients 2 to 7) (Saïdani et al., 2019). Briefly, patients who underwent FMT were previously sampled for various colonization sites (urine, pharynx, nasopharynx, and rectum) and additional potential sites (gastrostomy, skin, wounds, etc.). This mapping of CPE-colonized sites was established over three consecutive days prior to the FMT protocol. Eight days before FMT, a 3-day nasopharyngeal decolonization (in case of nasopharyngeal carriage) was performed using 0.12% chlorhexidine gluconate as local treatment of the mouth (gargling) and nasal cavities (swab applications). Five days before FMT, patients received an initial bowel lavage (until stools became watery and clear). An oral non-absorbable combination of antibiotics comprising colistin 6 MIU every 6h and amikacin 200 mg every 6h was then administered, replaced by other antibiotics in case of resistance, according to isolated CPE antibiotic susceptibility. One day prior to FMT, the patient received a second bowel lavage (until stools became watery and clear) and was given a proton-pump inhibitor (pantoprazole 40 mg twice a day for 48h).
Fecal microbiota transplantation was only considered for CPE-colonized patients for whom rehabilitation, surgery or chemotherapies were indicated and were likely to be delayed, based on the argument that CPE carriage would lead to a consequent loss of opportunity.
Phenotypic and molecular analyses
All strains were isolated on chromID® CARBA SMART agar (bioMérieux, Marcy-l’Etoile, France), except for P7700 on Columbia agar with 5% sheep blood (bioMérieux). Isolates were identified by matrix-assisted laser desorption and ionization time-of-flight mass spectrometry (MALDI-TOF) (Microflex, Brüker Daltonics, Bremen, Germany) (Seng et al., 2009). Antimicrobial susceptibility testing (AST) was performed using the disc diffusion method on a panel of 13 antibiotics (i2a, Montpellier, France): amoxicillin, amoxicillin-clavulanic acid, cefepime, ceftriaxone, piperacillin-tazobactam, ertapenem, imipenem, fosfomycin, doxycycline, trimethoprim-sulfamethoxazole, amikacin, gentamicin and ciprofloxacin, according to EUCAST recommendations (version 3.1). The minimum inhibitory concentration (MIC) of ertapenem and imipenem was determined by the E-test method (bioMérieux, Marcy l’Etoile, France). The β-CARBA test (Bio-Rad, Hercules, CA, USA) was used to detect carbapenemase production.
Real-time PCR (RT-PCR) was performed in all strains to confirm the presence of genes encoding carbapenem hydrolyzing enzymes (blaNDM, blaKPC, blaOXA-48, blaVIM) using the CFX96 Touch Real-Time PCR Detection System (Bio-Rad, Hercules, CA, USA). Targeted genes were detected using specific primers and Taqman probes (Yousfi et al., 2019).
Genomic analyses
Total genomic DNA (gDNA) was extracted using a EZ1 DNA kit and the BioRobot EZ1 (Qiagen, Courtaboeuf, France) according to the manufacturer’s instructions. Then, the gDNA was quantified by a Qubit assay (Life Technologies, Carlsbad, CA, USA) and its quality was controlled by Bioanalyser systems (Agilent, Santa Clara, CA, USA). All CPEs were sequenced in 2x250 bp paired-end reads in MiSeq (Illumina Inc., San Diego, CA, USA), of which two Citrobacter freundii strains (P7697, P7699) and two Enterobacter sp. isolates (P7536, P7538) were also sequenced using MinIon technology (Oxford Nanopore Technologies Inc., United Kingdom). The runs performed by the MinION technology were done using Ligation Sequencing Kit (Oxford Nanopore Technologies Inc., United Kingdom) and the libraries were loaded on a flow cell R9.4.1. Spades software (Bankevich et al., 2012) was used to assemble the Illumina generated sequencing data and also for the assembly of the mixed Nanopore-Illumina data. Genome annotation, antibiotic resistance gene, and plasmid screening were performed with RAST (Aziz et al., 2008), Resfinder (Bortolaia et al., 2020) and PlasmidFinder (Carattoli et al., 2014), respectively. The CPE sequence type (ST) was performed in silico using multilocus sequence typing (MLST) analysis on the Center for Genomic Epidemiology website (https://www.genomicepidemiology.org/).
The genetic environment of the blaOXA-48 genes was reconstructed by comparing the sequences of the genes surrounding this gene to the NCBI database using the blastX parameter. The complete plasmid sequences of strains P7697, P7699, P7536 and P7538 carrying blaOXA-48 genes were compared with the reference plasmid CP027039.1 using CGViewServer software (Stothard et al., 2017). Variant calling on the snippy tool version 4.6.0 was used to detect single nucleotides variants (SNV), both between blaOXA-48 plasmids and between strains of the same species. Pangenome analysis was performed with Roary (Page et al., 2015) and visualized using Phandango software (Hadfield et al., 2018). The percent similarity of the C. freundii strains and Enterobacter sp. strains was calculated by pairwise comparison of their average nucleotide identity based on Blast (ANIb) and using JSpecies (Richter et al., 2016).
Genomes of P7535, P7536, P7537, P7538, P7697, P7698, P7699, P7700 and P7702 strains have been submitted to GenBank under accession numbers JAGDEG000000000, CP071788- CP071792, JAGDEH000000000, CP071830-CP071833, CP071834-CP071838, JAGDEI000000000, CP071907-CP071913, JAGDEJ000000000, and JAGDEK000000000, respectively (Table 2).
Ethics
No sampling was performed for research purposes. Phenotypic, molecular and genomic analyses were performed on bacteria isolated for diagnostic as routine care for epidemiological investigation of the outbreak and infection control intervention. According to European General Data Protection Regulation No. 2016/679, the study was registered under N° 2022-28 in the APHM register.
Results
Outbreak control and decolonization by FMT
Overall, eight CPE carriers in a thoracic-oncology ward were identified (Table 1; Figure 1). The detection of CPE in patient 3 led to the implementation of measures to control the transmission of the outbreak according to current French recommendations (Lepelletier et al., 2015). Initially, the patient was placed on contact isolation precautions, associated with enhanced environmental disinfection, and a training for the healthcare staff on reduction of the risk of cross-transmission was done. In addition, screening of 49 contact patients hospitalized in the thoracic-oncology ward was performed. Five (5/49, 10.2%) new secondary cases (patients 4 to 8) were detected in the thoracic-oncology unit (Table 1; Figure 1). Isolation and prevention policies were also applied to these patients.
Before this outbreak, two patients carried a CPE at different times. The detection of CPE in patient 1 was confirmed after he died of his oncological illness on the ward, one year before the outbreak. Consequently, no isolation measures were taken. Patient 2 was hospitalized two months before the outbreak and was still hospitalized when patient 3 was detected positive. Patient 2 had been hospitalized abroad within 12 months, so he was placed on contact isolation precautions on arrival in the ward and was evaluated for the carriage of CPE according to the recommendations of the French High Committee for Public Health (Lepelletier et al., 2015). Isolation measures were maintained after the finding of C. freundii OXA-48 carriage.
In addition to hand hygiene and contact isolation policies, treatment by selective personalized decolonization and FMT was performed in 6/8 patients so that they could receive chemotherapy. Four out of six patients had a successful FMT (patient 2, 3, 4, 7) confirmed by three negative consecutive CPE controls after transplantation. Two successive FMTs were required to decolonize patient 2 (Table 1; Figure 1).
A 12-month follow-up did not detect additional CPE carriers in this thoracic-oncology ward.
Isolates and antibiotic susceptibility testing
Four C. freundii strains and five E. cloacae strains were isolated from eight clinical samples. All CPE were sensitive to fosfomycin and amikacin but resistant to amoxicillin, amoxicillin-clavulanic acid, ceftriaxone, piperacillin-tazobactam, and ciprofloxacin. All strains of C. freundii had the same resistance phenotype (doxycycline, gentamicin, trimethoprim-sulfamethoxazole resistant and cefepime sensitive). Concerning the Enterobacter strains, two phenotypes were observed. The isolates P7535 and P7537 were sensitive to cefepime, doxycycline, trimethoprim-sulfamethoxazole and gentamicin, while the remaining (P7536, P7538, P7698) were not (Table 1).
Ertapenem and imipenem MICs of C. freundii strains varied from 0.5 to 1 µg/mL and 0.5 to 1 µg/mL respectively, while ertapenem and imipenem MICs of Enterobacter isolates ranged respectively from 1 to >32 µg/mL and 1 to 6µg/mL (Table 1). The β-CARBA test was positive for all isolates that carried only the blaOXA-48 carbapenemase gene.
Resistome
All strains of C. freundii had a median length of 5.2Mb and a median GC of 51.7% while the median length and GC of Enterobacter isolates ranged between 4.98 to 5.1Mb and between 55 to 55.1%, respectively. RT-PCR and genome analysis confirmed that OXA-48 carbapenemase was produced by all strains. Resistome analysis showed the presence of genes encoding resistance to different families of antibiotics such as β-lactams, quinolones, aminoglycosides, sulfonamides, cyclins, and chloramphenicol (Table 2).
The gene encoding the OXA-48 enzyme was found in a 63kb IncL/M plasmid, whereas the other antibiotic resistance genes were present on other plasmid types or in the chromosome (Table 2). In all CPE, the blaOXA-48 gene was in a Tn1999.2 transposon (IS10A-lysR-blaOXA-48-IS1-IS10A) (Figure 2). Comparison of the complete OXA-48 plasmids with all CPE showed the quasi-similarity of the outbreak plasmids, with presence of 0 to 5 SNV.
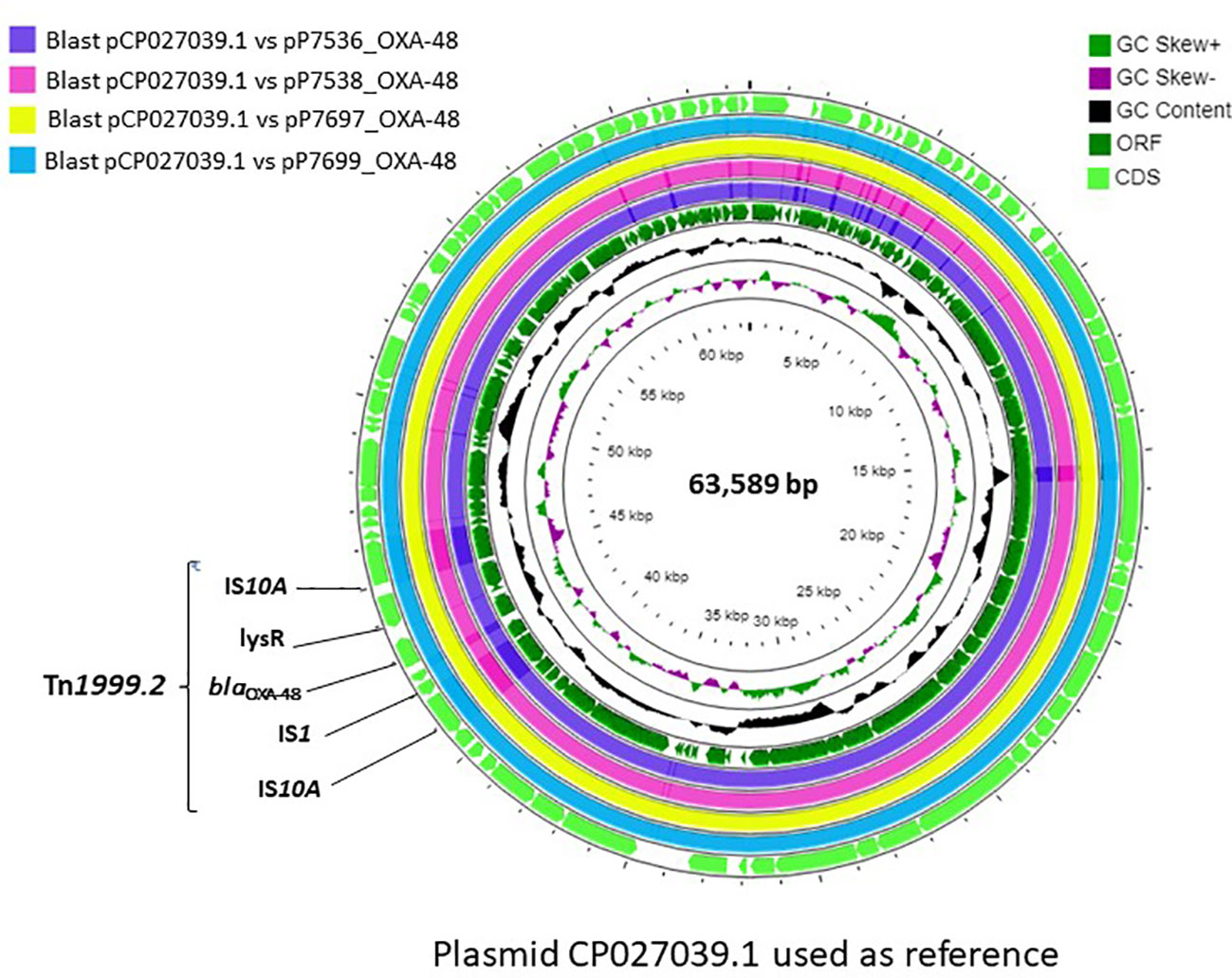
Figure 2 Genetic environment of the blaOXA-48 gene and circular representation of the complete OXA-48 plasmids.
Annotation of genomes on NCBI allowed reclassification of P7535, P7537, P7538, P7698 isolates as Enterobacter hormaechei strains (Table 2). According to MLST analysis, all C. freundii strains belonged to the same ST22 sequence type, while Enterobacter isolates had different ST, except P7535 and P7537, which were ST1007 (Figure 3; Table 2). E. hormaechei strains P7535 and P7537 had 99.98% ANIb similarity, while the similarity between the other Enterobacter isolates varied from 96.1 to 98.5%. The C. freundii strains also shared high similarity rates, from 99.64 to 99.98%. Pangenome and SNV analysis confirmed the same origin for E. hormaechei isolates P7535 and P7537, whereas the distance of C. freundii isolates indicated that they were related but appear to have diverged over time (Figure 3).
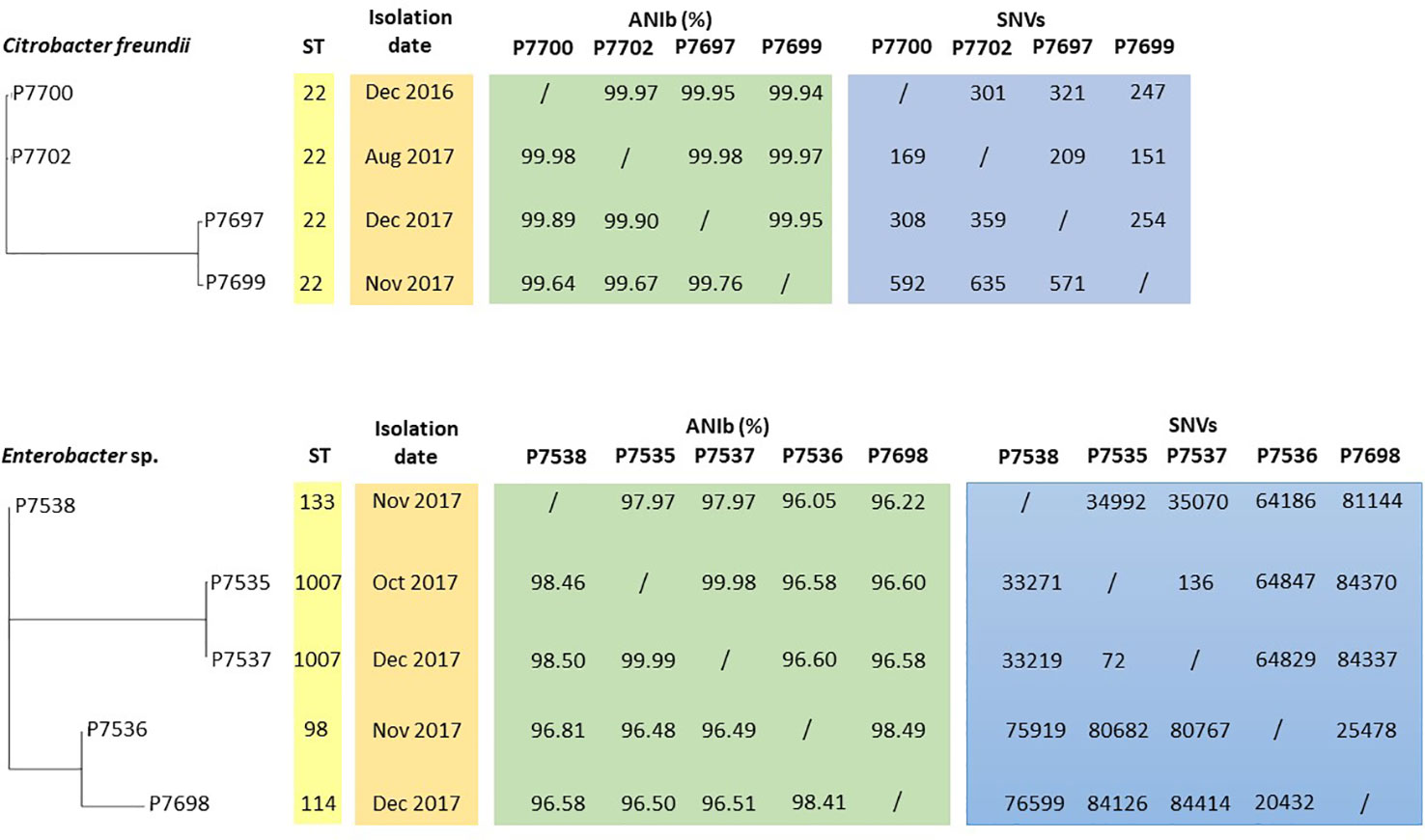
Figure 3 Phylogenetic tree based on the pangenome of C. freundii and Enterobacter sp. strains associated with ST, isolation date, ANIb and SNV data.
Discussion
The emergence and spread of CPE in the hospital setting are a major concern for clinicians. In this study, among the CPE, we isolated C. freundii and E. hormaechei, which belong to the E. cloacae complex and are identified as E. cloacae by most biochemical methods (Mateos et al., 2020). The presence of C. freundii producing OXA-48 carbapenemase is emerging in clinical settings where recent reports from Spain warned of an increase in its incidence. In Germany, the ST22 clone of OXA-48 carbapenemase producing C. freundii is increasingly noted in the hospital setting (Villa et al., 2017; Lalaoui et al., 2019; Yao et al., 2021). Recently in the north of France, an outbreak of OXA-48 carbapenemase -producing Enterobacteriaceae species, including Enterobacter sp. and C. freundii ST22, has been reported in a haematological ward (Jolivet et al., 2021).
IncL/M plasmids are known to be major carriers of the blaOXA-48 gene, which is very commonly inserted into a Tn1999-type transposon (Giani et al., 2012). Tn1999.2, present in all of our strains, has been found in different species of Enterobacteriaceae in Europe (Hidalgo et al., 2019). In our case, the same IncL/M OXA-48 plasmid was propagated among the different strains of C. freundii and Enterobacter sp. The horizontal transfer of conjugative plasmid is a key factor in the spread of antibiotic resistance genes in different clones and species of Enterobacteriaceae. It allows the evolution of resistance in certain bacterial clones due to an efficient bacterial-plasmid association (San Millan, 2018). This phenomenon was observed in a Spanish hospital in which a Klebsiella pneumoniae ST11 clone carrying OXA-48 carbapenemase was identified in 44 patients (Branas et al., 2015). In our case, similar clones of C. freundii circulated in the ward for one year, and the same strain of E. hormaechei spread between patients 3 and 7.
In our study, all patients colonized by CPE were hospitalized in the thoracic-oncology ward. Rectal or urinary colonization with CPE and prolonged hospital stays are risk factors for infections by these pathogens (Mateos et al., 2020). Spontaneous decolonization of intestinal carriage in patients colonized with CPE is a common but slow event (Zimmerman et al., 2013). FMT is a validated procedure that allows reduction of the time of colonization and thus accelerates medical management for patients (Saïdani et al., 2019). In our case, CPE colonization of cancer patients led to the postponement of chemotherapy treatment due to a higher risk of CPE infections in immunocompromised patients. Therefore, the benefits of FMT was to reduce the delay in the management of these patients, thus reducing their loss of opportunity (Saïdani et al., 2019).
The source of initial contamination of patient 1, at the origin of this outbreak, has not been identified to date, but an environmental track is probable and should not be neglected. The hospital environment and especially inanimate surfaces have often been identified as a reservoir for multidrug-resistant bacterial outbreaks (Decraene et al., 2018). Many Gram-negative species can survive on these surfaces for as long as several months (Pantel et al., 2016). One reason for the persistent transmission of OXA-48 C. freundii in a hematology department was the presence of contaminated toilets which constituted a potential reservoir (Jolivet et al., 2021). In our case, the same clone of C. freundii was present in four different patients, hospitalized in different rooms, at different times, which is worrying. Therefore, the origins of this clone seem to be both external and internal to the unit. On the one hand, it could be due to the persistence of CPE in the department after the first patient died in 2016. Before the outbreak, patients were not systematically screened on this ward, unless they came from another hospital. On the other hand, the outbreak could have started with patient 2, despite the implementation of isolation precaution procedures. In particular, hand hygiene, the use of gloves, protective clothing and single-use sterile consumables, the excreta management as well as reinforced disinfection of the environment and technical areas are essential in the reduction of the cross-transmission risk (Lepelletier et al., 2015). Systematic screening and isolation of patients upon entry to the unit may be an efficient way to solve this issue and to allow the best possible control of CPE dissemination, although these measures are cumbersome to implement. (Pantel et al., 2014). These procedures are even more important in wards caring for immunocompromised patients (Nicolas-Chanoine et al., 2019). The spread of the same OXA-48 plasmid through different bacterial species and clones has been established. Nevertheless, the measures of control, implemented in accordance with the recommendations of the French High Committee for Public Health after the detection of this outbreak, together with the implementation of an FMT procedure for CPE carriers, promptly controlled this outbreak (Lepelletier et al., 2015).
Conclusion
Whole genome sequencing demonstrated several modes of transmission of OXA-48 carbapenemase through specific clones, plasmids, and transposons. The emergence and spread of CPE over a period of one year in our hospital is a worrisome development. This study highlights the necessity of investigating the source of contamination and controlling it as soon as possible to avoid the persistence of risky clones within a unit. Despite the precautions taken in the thoracic-oncology ward, this outbreak occurred, demonstrating the difficulty in prevention and control. Decolonization by FMT is an effective procedure that allowed a rapid resumption of oncological treatments. Nevertheless, it is necessary to remain vigilant and to continue epidemiological surveillance, especially when new patients are admitted to a unit, particularly in wards with immunosuppressed patients.
Data availability statement
The datasets presented in this study can be found in online repositories. The names of the repository/repositories and accession number(s) can be found below: https://www.ncbi.nlm.nih.gov/genbank/, JAGDEG000000000, https://www.ncbi.nlm.nih.gov/genbank/, CP071788-CP071792, https://www.ncbi.nlm.nih.gov/genbank/, JAGDEH000000000, https://www.ncbi.nlm.nih.gov/genbank/, CP071830-CP071833, https://www.ncbi.nlm.nih.gov/genbank/, CP071834-CP071838, https://www.ncbi.nlm.nih.gov/genbank/, JAGDEI000000000, https://www.ncbi.nlm.nih.gov/genbank/, CP071907-CP071913, https://www.ncbi.nlm.nih.gov/genbank/, JAGDEJ000000000, https://www.ncbi.nlm.nih.gov/genbank/, JAGDEK000000000.
Ethics statement
No sampling was performed for research purposes. Phenotypic, molecular and genomic analyses were performed on bacteria isolated for diagnostic as routine care for epidemiological investigation of the outbreak and infection control intervention. According to European General Data Protection Regulation No. 2016/679, the study was registered under N° 2022-28 in the APHM register.
Author contributions
LH wrote the manuscript, performed the experiments, and analyzed the data. NS, CH, PA and PB performed medical examinations, collected and analyzed data. NC performed medical examinations and helped draft the manuscript. J-MR reviewed the manuscript. SB designed the study, drafted, and revised the manuscript. All authors read and approved the final version of the manuscript.
Funding
This work was supported by the French Government under the program “Investissement d’avenir” managed by the “Agence Nationale de la Recherche ANR”, (Méditerranée Infection 10-IAHU-03). This work was supported by Région Provence-Alpes-Côte d’Azur and European funding FEDER PRIMI.
Acknowledgments
The authors thank TradOnline for proofreading the text.
Conflict of interest
The authors declare that the research was conducted in the absence of any commercial or financial relationships that could be construed as a potential conflict of interest.
Publisher’s note
All claims expressed in this article are solely those of the authors and do not necessarily represent those of their affiliated organizations, or those of the publisher, the editors and the reviewers. Any product that may be evaluated in this article, or claim that may be made by its manufacturer, is not guaranteed or endorsed by the publisher.
References
Aziz, R. K., Bartels, D., Best, A. A., DeJongh, M., Disz, T., Edwards, R. A., et al. (2008). The RAST server: Rapid annotations using subsystems technology. BMC Genomics 9, 75. doi: 10.1186/1471-2164-9-75
Ballo, O., Tarazzit, I., Stratmann, J., Reinheimer, C., Hogardt, M., Wichelhaus, T. A., et al. (2019). Colonization with multidrug resistant organisms determines the clinical course of patients with acute myeloid leukemia undergoing intensive induction chemotherapy. PLoS One 14, e0210991. doi: 10.1371/JOURNAL.PONE.0210991
Bankevich, A., Nurk, S., Antipov, D., Gurevich, A. A., Dvorkin, M., Kulikov, A. S., et al. (2012). SPAdes: A new genome assembly algorithm and its applications to single-cell sequencing. J. Comput. Biol. 19, 455–477. doi: 10.1089/cmb.2012.0021
Bortolaia, V., Kaas, R. S., Ruppe, E., Roberts, M. C., Schwarz, S., Cattoir, V., et al. (2020). ResFinder 4.0 for predictions of phenotypes from genotypes. J. Antimicrob. Chemother. 75, 3491–3500. doi: 10.1093/jac/dkaa345
Branas, P., Villa, J., Viedma, E., Mingorance, J., Orellana, M. A., Chaves, F. (2015). Molecular epidemiology of carbapenemase-producing klebsiella pneumoniae in a hospital in Madrid: Successful establishment of an OXA-48 ST11 clone. Int. J. Antimicrob. Agents 46, 111–116. doi: 10.1016/J.IJANTIMICAG.2015.02.019
Brehony, C., McGrath, E., Brennan, W., Tuohy, A., Whyte, T., Brisse, S., et al. (2019). An MLST approach to support tracking of plasmids carrying OXA-48-like carbapenemase. J. Antimicrob. Chemother. 74, 1856–1862. doi: 10.1093/jac/dkz136
Carattoli, A., Zankari, E., Garcia-Fernandez, A., Voldby Larsen, M., Lund, O., Villa, L., et al. (2014). In silico detection and typing of plasmids using PlasmidFinder and plasmid multilocus sequence typing. Antimicrob. Agents Chemother. 58, 3895–3903. doi: 10.1128/AAC.02412-14
Decraene, V., Phan, H. T. T., George, R., Wyllie, D. H., Akinremi, O., Aiken, Z., et al. (2018). A Large, refractory nosocomial outbreak of Klebsiella pneumoniae carbapenemase-producing escherichia coli demonstrates carbapenemase gene outbreaks involving sink sites require novel approaches to infection control. Antimicrob. Agents Chemother. 62 (12), e01689-18. doi: 10.1128/AAC.01689-18
Emeraud, C., Biez, L., Girlich, D., Jousset, A. B., Naas, T., Bonnin, R. A., et al. (2020). Screening of OXA-244 producers, a difficult-to-detect and emerging OXA-48 variant? J. Antimicrob. Chemother. 75, 2120–2123. doi: 10.1093/jac/dkaa155
George, S., Pankhurst, L., Hubbard, A., Votintseva, A., Stoesser, N., Sheppard, A. E., et al. (2017). Resolving plasmid structures in Enterobacteriaceae using the MinION nanopore sequencer: Assessment of MinION and MinION/illumina hybrid data assembly approaches. Microb. Genomics 3 (8), e000118. doi: 10.1099/mgen.0.000118
Giani, T., Conte, V., Di Pilato, V., Aschbacher, R., Weber, C., Larcher, C., et al. (2012). Escherichia coli from Italy producing OXA-48 carbapenemase encoded by a novel Tn1999 transposon derivative. Antimicrob. Agents Chemother. 56, 2211–2213. doi: 10.1128/AAC.00035-12
Hadfield, J., Croucher, N. J., Goater, R. J., Abudahab, K., Aanensen, D. M., Harris, S.R. (2018). Phandango: an interactive viewer for bacterial population genomics. Bioinformatics 34, 292–293. doi: 10.1093/BIOINFORMATICS/BTX610
Hidalgo, L., de Been, M., Rogers, M. R. C., Schürch, A. C., Scharringa, J., van der Zee, A., et al. (2019). Sequence-based epidemiology of an OXA-48 plasmid during a hospital outbreak. Antimicrob. Agents Chemother. 63 (12), e01204-19. doi: 10.1128/AAC.01204-19
Jamal, A. J., Mataseje, L. F., Brown, K. A., Katz, K., Johnstone, J., Muller, M. P., et al. (2020). Carbapenemase-producing enterobacterales in hospital drains in southern Ontario, Canada. J. Hosp. Infect. 106, 820–827. doi: 10.1016/j.jhin.2020.09.007
Jolivet, S., Couturier, J., Vuillemin, X., Gouot, C., Nesa, D., Adam, M., et al. (2021). Outbreak of OXA-48-producing enterobacterales in a haematological ward associated with an uncommon environmental reservoir, francs 2016 To 2019. Eurosurveillance 26, 1. doi: 10.2807/1560-7917.ES.2021.26.21.2000118
Lalaoui, R., Djukovic, A., Bakour, S., Hadjadj, L., Sanz, J., Salavert, M., et al. (2019). Genomic characterization of citrobacter freundii strains coproducing OXA-48 and VIM-1 carbapenemase enzymes isolated in leukemic patient in Spain. Antimicrob. Resist. Infect. Control 8, 167. doi: 10.1186/s13756-019-0630-3
Lepelletier, D., Berthelot, P., Lucet, J. C., Fournier, S., Jarlier, V., Grandbastien, B., et al. (2015). French Recommendations for the prevention of “emerging extensively drug-resistant bacteria” (eXDR) cross-transmission. J. Hosp. Infect. 90, 186–195. doi: 10.1016/J.JHIN.2015.04.002
Mateos, M., Hernández-García, M., del Campo, R., Martínez-García, L., Gijón, D., Morosini, M. I., et al. (2020). Emergence and persistence over time of carbapenemase-producing enterobacter isolates in a Spanish university hospital in Madrid, spain, (2005–2018). Microb. Drug Resist 27 (7), 895–903. doi: 10.1089/mdr.2020.0265
Nicolas-Chanoine, M. H., Vigan, M., Laouénan, C., Robert, J., Laurans, C., Vachée, A., et al. (2019). Risk factors for carbapenem-resistant enterobacteriaceae infections: a French case-control-control study. Eur. J. Clin. Microbiol. Infect. Dis. 38, 383–393. doi: 10.1007/S10096-018-3438-9/TABLES/6
Page, A. J., Cummins, C. A., Hunt, M., Wong, V. K., Reuter, S., Holden, M. T. G., et al. (2015). Roary: rapid large-scale prokaryote pan genome analysis. Bioinformatics 31, 3691–3693. doi: 10.1093/BIOINFORMATICS/BTV421
Pantel, A., Marchandin, H., Prère, M. F., Boutet-Dubois, A., Brieu-Roche, N., Gaschet, A., et al. (2014). Faecal carriage of carbapenemase-producing gram-negative bacilli in hospital settings in southern France. Eur. J. Clin. Microbiol. Infect. Dis. 34, 899–904. doi: 10.1007/S10096-014-2298-1
Pantel, A., Richaud-Morel, B., Cazaban, M., Bouziges, N., Sotto, A., Lavigne, J. P. (2016). Environmental persistence of OXA-48–producing klebsiella pneumoniae in a French intensive care unit. Am. J. Infect. Control 44, 366–368. doi: 10.1016/J.AJIC.2015.09.021
Richter, M., Rosselló -Móra, R., Oliver Glöckner, F., Peplies, J. (2016). JSpeciesWS: a web server for prokaryotic species circumscription based on pairwise genome comparison. Bioinformatics 32, 929–931. doi: 10.1093/bioinformatics/btv681
Saïdani, N., Lagier, J. C., Cassir, N., Million, M., Baron, S., Dubourg, G., et al. (2019). Faecal microbiota transplantation shortens the colonisation period and allows re-entry of patients carrying carbapenamase-producing bacteria into medical care facilities. Int. J. Antimicrob. Agents 53, 355–361. doi: 10.1016/J.IJANTIMICAG.2018.11.014
San Millan, A. (2018). Evolution of plasmid-mediated antibiotic resistance in the clinical context. Trends Microbiol. 26, 978–985. doi: 10.1016/j.tim.2018.06.007
Seng, P., Drancourt, M., Gouriet, F., La Scola, B., Fournier, P.-E., Rolain, J. M., et al. (2009). Ongoing revolution in bacteriology: routine identification of bacteria by matrix-assisted laser desorption ionization time-of-flight mass spectrometry. Clin. Infect. Dis. 49, 543–551. doi: 10.1086/600885
Shankar, C., Muthuirulandi Sethuvel, D. P., Neeravi, A. R., Venkatesan, M., Devanga Ragupathi, N. K., Anandan, S., et al. (2020). Identification of plasmids by PCR based replicon typing in bacteremic Klebsiella pneumoniae. Microb. Pathog. 148, 104429. doi: 10.1016/j.micpath.2020.104429
Stothard, P., Grant, J. R., Van Domselaar, G. (2017). Visualizing and comparing circular genomes using the CGView family of tools. Brief. Bioinform 20 (4), 1576–1582. doi: 10.1093/bib/bbx081
Tacão, M., Araújo, S., Vendas, M., Alves, A., Henriques, I. (2018). Shewanella species as the origin of blaOXA-48 genes: insights into gene diversity, associated phenotypes and possible transfer mechanisms. Int. J. Antimicrob. Agents 51, 340–348. doi: 10.1016/J.IJANTIMICAG.2017.05.014
Tilahun, M., Kassa, Y., Gedefie, A., Ashagire, M. (2021). Emerging carbapenem-resistant enterobacteriaceae infection, its epidemiology and novel treatment options: A review. Infect. Drug Resist. 14, 4363–4374. doi: 10.2147/IDR.S337611
Villa, J., Arana, D. M., Viedma, E., Perez-Montarelo, D., Chaves, F. (2017). Characterization of mobile genetic elements carrying VIM-1 and KPC-2 carbapenemases in citrobacter freundii isolates in Madrid. Int. J. Med. Microbiol. 307, 340–345. doi: 10.1016/j.ijmm.2017.07.001
Yao, Y., Falgenhauer, L., Falgenhauer, J., Hauri, A. M., Heinmüller, P., Domann, E., et al. (2021). Carbapenem-resistant citrobacter spp. as an emerging concern in the hospital-setting: Results from a genome-based regional surveillance study. Front. Cell. Infect. Microbiol. 11. doi: 10.3389/FCIMB.2021.744431
Yoon, Y. K., Suh, J. W., Kang, E. J., Kim, J. Y. (2019). Efficacy and safety of fecal microbiota transplantation for decolonization of intestinal multidrug-resistant microorganism carriage: beyond clostridioides difficile infection. Ann. Med. 51, 379. doi: 10.1080/07853890.2019.1662477
Yousfi, H., Hadjadj, L., Dandachi, I., Lalaoui, R., Merah, A., Amoura, K., et al. (2019). Colistin- and carbapenem-resistant Klebsiella pneumoniae Clinical_Isolates: Algeria. Microb. Drug Resist. 25, 258–263. doi: 10.1089/mdr.2018.0147
Keywords: whole genome sequencing, carbapenemase, blaOXA-48 gene, IncL/M plasmid, fecal microbiota transplantation
Citation: Hadjadj L, Cassir N, Saïdani N, Hoffman C, Brouqui P, Astoul P, Rolain J-M and Baron SA (2022) Outbreak of carbapenem-resistant enterobacteria in a thoracic-oncology unit through clonal and plasmid-mediated transmission of the blaOXA-48 gene in Southern France. Front. Cell. Infect. Microbiol. 12:1048516. doi: 10.3389/fcimb.2022.1048516
Received: 19 September 2022; Accepted: 23 November 2022;
Published: 07 December 2022.
Edited by:
Maria Teresa Mascellino, Sapienza University of Rome, ItalyReviewed by:
Kohar Annie B. Kissoyan, General Reinsurance AG, GermanyFarah Al Marzooq, United Arab Emirates University, United Arab Emirates
Copyright © 2022 Hadjadj, Cassir, Saïdani, Hoffman, Brouqui, Astoul, Rolain and Baron. This is an open-access article distributed under the terms of the Creative Commons Attribution License (CC BY). The use, distribution or reproduction in other forums is permitted, provided the original author(s) and the copyright owner(s) are credited and that the original publication in this journal is cited, in accordance with accepted academic practice. No use, distribution or reproduction is permitted which does not comply with these terms.
*Correspondence: Sophie Alexandra Baron, c29waGllLmJhcm9uQGFwLWhtLmZy