- 1Laboratory of Genetic Regulators in the Immune System, Henan Collaborative Innovation Center of Molecular Diagnosis and Laboratory Medicine, School of Laboratory Medicine, Xinxiang Medical University, Xinxiang, China
- 2Henan Key Laboratory of Immunology and Targeted Therapy, Xinxiang Medical University, Xinxiang, China
- 3Institute of Psychiatry and Neuroscience, Xinxiang Medical University, Xinxiang, China
Schistosomiasis has been widely disseminated around the world, and poses a significant threat to human health. Schistosoma eggs and soluble egg antigen (SEA) mediated inflammatory responses promote the formation of egg granulomas and liver fibrosis. With continuous liver injuries and inflammatory stimulation, liver fibrosis can develop into liver cirrhosis and liver cancer. Therefore, anti-fibrotic therapy is crucial to increase the survival rate of patients. However, current research on antifibrotic treatments for schistosomiasis requires further exploration. In the complicated microenvironment of schistosome infections, it is important to understand the mechanism and pathology of schistosomiasis-associated liver fibrosis(SSLF). In this review, we discuss the role of SEA in inhibiting liver fibrosis, describe its mechanism, and comprehensively explore the role of host-derived and schistosome-derived microRNAs (miRNAs) in SSLF. Inflammasomes and cytokines are significant factors in promoting SSLF, and we discuss the mechanisms of some critical inflammatory signals and pro-fibrotic cytokines. Natural killer(NK) cells and Natural killer T(NKT) cells can inhibit SSLF but are rarely described, therefore, we highlight their significance. This summarizes and provides insights into the mechanisms of key molecules involved in SSLF development.
Introduction
Schistosomiasis is a zoonotic parasitic disease caused by schistosomes and infects more than 200 million people in 78 countries worldwide (Huang et al., 2020). Schistosomiasis is a major threat to human health and economic development, with more than 200,000 deaths and nearly 800 million people exposed to schistosomiasis infections every year (Huang et al., 2020; Chen et al., 2022). There are six subtypes of schistosomes, though only Schistosoma japonicum, Schistosoma mansoni, and Schistosoma haematobium are responsible for human diseases (Anthony et al., 2010). In the lifecycle of schistosomes, the oncomelania is the unique intermediate host of schistosomes. The oncomelania releases mature schistosoma cercariae into the water, which invades the skin and enters the circulatory system of the host (Colley et al., 2014). As schistosome larvae mature, adult schistosomes attach to the inner walls of the hepatic portal and mesenteric veins, with the aid of suction cups in the mouth and abdomen, to avoid being displaced by high-speed blood flow (Wang J. et al., 2020). The liver is the center of energy metabolism, and the presence of schistosomes in the liver and mesenteric veins is likely related to adequate energy supply. Schistosomes are dioecious, the sexual maturation and egg laying of females are completely controlled by males, and the mating of males and females promotes the production of eggs by females (Chen et al., 2022). The average lifetime of schistosomes in human hosts is 3-10 years, though in certain cases can reach 40 years. A single female schistosome can produce more than a million eggs during its entire life cycle (Colley et al., 2014; Chen et al., 2022). The eggs produced by female schistosomes in the mesenteric vein cause an inflammatory response, and an excessive egg burden can damage the intestinal wall (Clerinx and Van Gompel, 2011). Schistosoma eggs migrate from the damaged intestinal wall into the intestine and are excreted in the feces (Carson et al., 2018). Schistosoma eggs can hatch into miracidia in a suitable natural environment, and the miracidia can infect the oncomelania and develop into mother sporocysts and daughter sporocysts in their bodies, and finally release mature cercariae (Sokolow et al., 2018). Mature cercariae reinfect susceptible hosts and perpetuate the schistosome life cycle.
The large number of eggs produced by female schistosomes is extremely harmful to the host, and over 50% of these eggs are deposited in the liver sinusoids (Anthony et al., 2010). Schistosome eggs and SEA mediate the formation of egg granulomas and liver fibrosis, which are the main pathological features of schistosomiasis (Zheng et al., 2020). In the early stages of schistosome infections, the toxins and antigenic substances released by schistosomes trigger a strong T-helper 1 (Th1) type immune response in the host organism, which mainly manifests by increased expression of Th1-type cytokines, such as IFN-γ, TNF-α, and IL-1, IL-2 (Stadecker et al., 2004). The th1-type immune response has a beneficial killing effect on Schistosoma. However, hosts appear to lack the capacity to eliminate schistosomes, which is also related to the specific immune escape mechanism of schistosomes (Trottein et al., 1999). Continuous inflammatory stimulation leading to excessive Th1 response can accelerate host death due to a cytokine storm (Hoffmann et al., 2000). After 4 weeks of Schistosoma japonicum infection, along with the production of schistosome eggs, the host’s immune response transforms into a T-helper 2 (Th2) type immune response, which is marked by increased secretion of Th2 type cytokines, such as IL4, IL-5, and IL-13 (Stadecker et al., 2004). Th2-type cytokines promote the polarization of macrophages to M2-type macrophages, while M2-type macrophages and Th2 maintain a high level of type II immune response in their microenvironment (Mei et al., 2022). Type II cytokines inhibit excessive activation of the inflammatory response and promote the formation of egg granulomas (Chen et al., 2014). Egg granulomas are formed by a massive number of eosinophils, neutrophils, macrophages, lymphocytes, monocytes, and aggregates around the eggs (Li L. et al., 2017). The formation of egg granulomas enables the isolation of eggs and toxins, which helps facilitate host survival (Chen D. et al., 2013). However, egg granulomas and type II immune responses inhibit host killing and clearance of schistosomes and lead to the formation of severe liver fibrosis (Hoffmann et al., 2000).
SSLF is a characteristic pathological transformation of the liver caused by schistosome infection, and liver fibrosis is an excessive deposition of extracellular matrix(ECM), which is characterized by increased production and decreased degradation of ECM (Huang et al., 2014). This results in the transformation of the ECM into a mesenchymal matrix composed of type I and type III collagen (Chen et al., 2019b). Egg granulomas and liver fibrosis occur in the hepatic sinusoids and portal veins causing increased pressure in the hepatic sinusoids, which can enlarge the liver and spleen, portal hypertension, and varices (Wang et al., 2022). The development of SSLF aggravates hepatic circulation disorders, which aggravates portal hypertension and gastroesophageal varices. The bleeding caused by varices in the lateral circulation of the gastro esophagus is the main cause of death in patients with schistosomiasis (Chatterjee et al., 2004). The proliferation and activation of hepatic stellate cells(HSCs) is a critical factor in the progression of SSLF. Resting HSCs are located in the Disse region of the subendothelial space and their major function is to stock vitamin A and erythropoietin (Carson et al., 2018; Wang et al., 2022). HSCs are activated to myofibroblasts during liver injuries or inflammatory stimulation, which express α-smooth muscle actin (α-SMA) and overproduce hydroxyproline-containing collagen leading to massive deposition of ECM (Yu et al., 2016; Zhu et al., 2018). Schistosoma infections induce differentiation of HSCs into two functionally distinct cell subtypes, while MHC II- HSCs exhibit a myofibroblast phenotype, and MHC II+ HSCs are associated with regulatory T cell development (Zhou et al., 2017). Inhibiting HSC activity or directly killing HSCs is the main goal of treating liver fibrosis while promoting senescence and apoptosis of HSCs could effectively relieve liver fibrosis (Chen et al., 2016).
Schistosome vaccines have been extensively researched and have a positive effect on the prevention of schistosomiasis (McManus et al., 2020). However, the treatment of schistosomiasis patients has always been highly dependent on praziquantel (Huang et al., 2020). Praziquantel can kill mature schistosomes but is not effective in schistosome eggs, and patients who are reinfected with schistosomes in endemic areas will gradually develop a resistance phenotype to praziquantel (Li Y. Q. et al., 2017). Liver fibrosis is reversible in quiescent circumstances; however, continued damage and inflammatory stimulation will transform liver fibrosis into cirrhosis and cancer (Kamdem et al., 2018). Therefore, the study of antifibrotic treatments for schistosomiasis is significant for improving the treatment and prognosis of schistosomiasis patients.
Previous reviews have extensively discussed the host regulators of SSLF and the genetics of human schistosomiasis (Andrade, 2008; Kamdem et al., 2018; Mewamba et al., 2021). However, there is insufficient discussion in SSLF regarding the role of SEA, schistosome-derived miRNAs and critical pro-fibrotic cytokines. This review outlines some important mechanisms of cytokines in SSLF.
SEA suppresses the proliferation and activation of HSCs
The activation of HSCs is suppressed by SEA, and is accompanied by decreased levels of a-SMA and Collagen 1A1(Col1A1) and increased levels of Matrix Metalloproteinase 9 (MMP-9) (Anthony et al., 2013). This also explains why HSCs tend to be located at the margins of egg granulomas. Collagen deposition in the area close to the eggs is likely detrimental to the survival of the eggs. The composition of SEA considerably varies at different stages of egg development. The first release of Schistosoma japonicum eggs is immature, and the inner envelope of the mature eggs after one week is considered to be the main source of SEA (De Marco Verissimo et al., 2019). SEA is a complex mixture of multiple egg antigens, of which Schistosoma japonicum egg antigen p40(Sjp40) is one of the components after six weeks of Schistosoma japonicum infection (Zhu et al., 2018; Chen et al., 2019b). Sjp40 is a marker used for early schistosomiasis diagnosis, and sjp40 inhibits the activation and proliferation of HSCs (Zhu et al., 2018). Mechanistically, sjp40 inhibits STAT5 activity to promote MicroRNA-155 expression (Figure 1), which down-regulates FOXO3a to inhibit the proliferation and activation of HSCs (Zhu et al., 2018). Lethal-7b (Let-7b) can specifically inhibit type I collagen expression, upregulation of Let-7b in HSCs by Sjp40 inhibits type I collagen expression, and ECM deposition (Sun X. et al., 2021). SKP2 is an important E3 ubiquitin ligase that targets P27 (an inhibitor of cyclin-dependent kinases) and participates in regulating cellular senescence and apoptosis (Wang et al., 2021). Sjp40 promotes cellular senescence in HSCs by upregulating the SKP2/P27 signaling pathway (Figure 1), and senescent HSCs show reduced collagen production and increased degradation (Xu et al., 2017). STAT3 is a transcription factor that activates proliferation-related cytokines involved in cell survival and growth, however, STAT3 promotes egg granulomas and liver fibrosis in Schistosoma infections (Zhao et al., 2021). JQ-1 is a small-molecule bromodomain inhibitor that relieves liver fibrosis caused by Schistosoma japonicum infections by inhibiting JAK2/STAT3 signaling (Ding et al., 2021). Interestingly, STAT3 signaling also promotes cellular senescence and growth arrest, and phosphorylated-STAT3(p-STAT3) promotes the P53/P21 signaling axis to inhibit cell proliferation (Santarelli et al., 2019). Sjp40 promotes cell cycle arrest in HSCs by activating the STAT3/P53/P21 signaling pathway (Chen et al., 2016). STAT3 is a double-edged sword in schistosome infections and is involved in the activation and senescence of HSCs, which could be related to different sources of signaling with stimulation (Chen et al., 2016; Ding et al., 2021). Bone morphogenic protein (BMP-7) is an antifibrotic protein that inhibits the activity of HSCs, and Sjp40 promotes Y-Box protein-1 (YB1) expression to initiate BMP-7 transcription (Chen B. L. et al., 2013; Chen et al., 2019b). P53 promotes nuclear translocation of YB-1, and STAT3/P53 signaling could regulate HSC activation by regulating nuclear translocation of YB-1 (Figure 1). SEA promotes increased production of reactive oxygen species (ROS) and induces apoptosis in HSCs, which could be associated with increased ROS-mediated caspase-1 apoptosis (Kong et al., 2019). However, increased apoptosis of HSCs could play an anti-fibrotic role, but the apoptosis of HSCs aggravates the inflammation and damage caused by schistosome infections. IL-34 promotes M2 macrophage polarization and inhibits NK cell killing of HSCs to promote liver fibrosis; however, SEA inhibits IL-34 expression (Chen et al., 2019a). Peroxisome proliferator-activated receptor γ(PPAR-γ) signaling inhibits HSC differentiation into myofibroblasts, and SEA inhibits HSC activation by increasing the expression of PPAR-γ (Anthony et al., 2010). SEA induces endothelial cell (EC) proliferation and upregulates vascular endothelial growth factor (VEGF) to promote HSC activation (Luo et al., 2017).
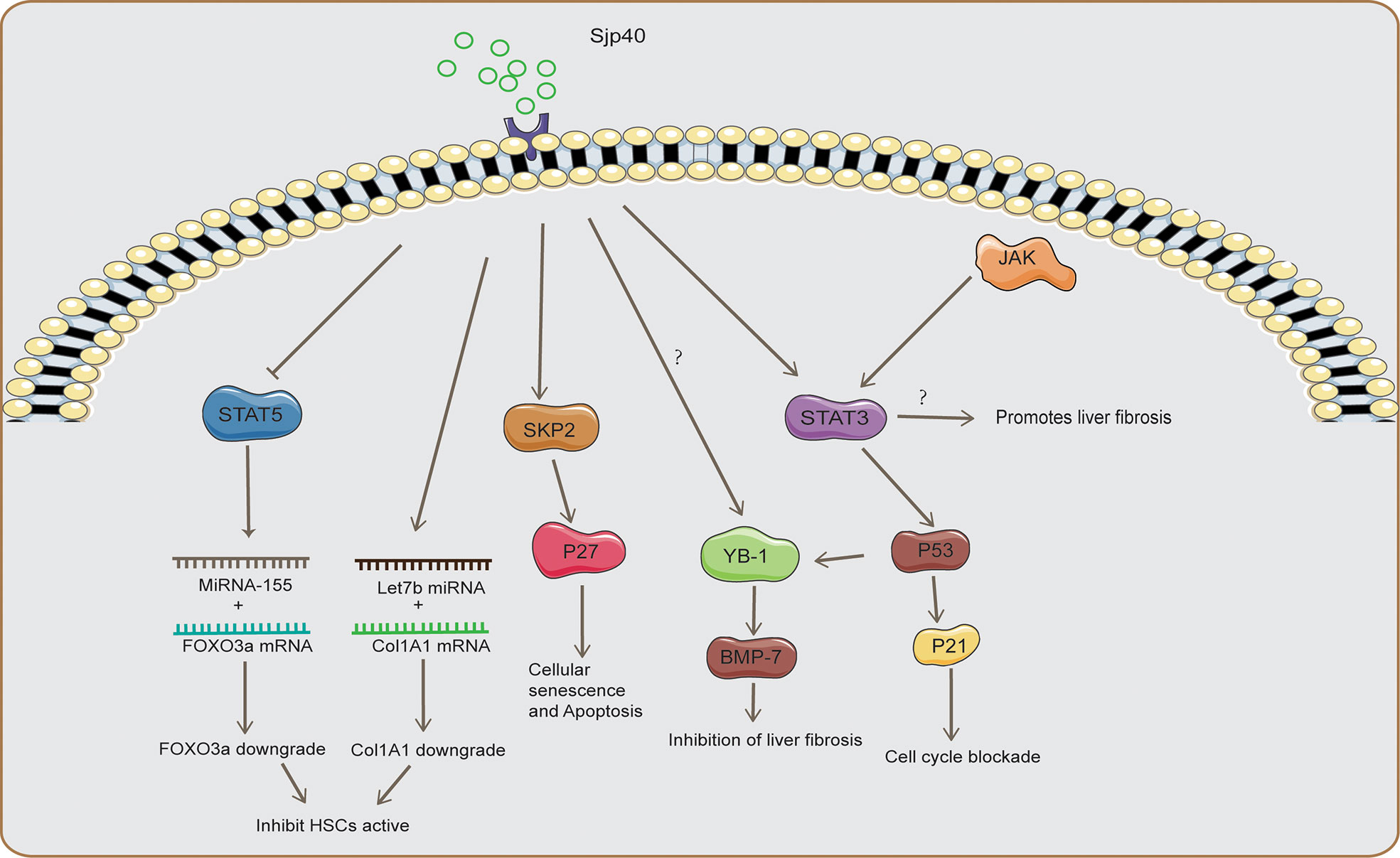
Figure 1 The mechanism of SSLF inhibition by Sjp40. Sjp40 inhibited STAT5 activity to promote MicroRNA-155 expression, which down-regulated FOXO3a to inhibit the proliferation and activation of HSCs and upregulation of Let-7b in HSCs to inhibit type I collagen expression. Sjp40 promotes cellular senescence in HSCs by upregulating the SKP2/P27 signaling pathway. Sjp40 promotes Y-Box protein-1 (YB1) expression to initiate BMP-7 transcription. Sjp40 promoted the P53/P21 signaling to inhibit cell proliferation, and P53 promotes nuclear translocation of YB-1. Inhibition of JAK2/STAT3 signaling could inhibit SSLF.
The schistosome infection microenvironment is highly complex and diverse, consisting of various parenchymal and non-parenchymal cells (Zhan et al., 2019). Under in vitro SEA stimulation conditions, HSCs are quiescent and suppressed, however, in the complex environment in vivo, SEA has a bidirectional effect on the development of liver fibrosis (Anthony et al., 2013). HSCs are usually absent from the core area of egg granulomas, and HSC-induced ECM deposition could be detrimental to the survival of the eggs. However, schistosome eggs and SEA construct a type II immune microenvironment, and some critical cytokines have potent pro-fibrotic effects, such as transforming growth factor-beta (TGF-β) and IL-13 (Li et al., 2010; Wang S. et al., 2020). This also explains the collagen deposition at the margins of the egg granuloma but the lack of collagen deposition in the core area. Schistosoma eggs and SEA can inhibit the activation and collagen deposition of HSCs, which could be related to the survival of Schistosoma eggs in the host.
MicroRNA
MiRNAs are a group of highly conserved endogenous non-coding single-stranded RNA molecules that play an important role in regulating gene expression (He et al., 2018a; Wang et al., 2022). miRNAs combine with the 3’UTR region of target mRNAs to inhibit gene expression by promoting mRNA degradation and translation blockage. More than 60% of protein-coding genes contain miRNA target sequences (Huang et al., 2018; Liu et al., 2021a; Sun X. et al., 2021). The miRNA in the liver of schistosome-infected mice originated from the host and schistosome eggs (Wang L. et al., 2020; Xu et al., 2021).
Exosomes of schistosome egg-derived origin transport contents to the receptor cells in the form of secretory vesicles and regulate the functional activity of the receptor cells. These exosomes also contain large amounts of proteins, lipids, and nucleic acids for long-range signal transmission (Wang L. et al., 2020; Wang et al., 2022). Generally, miRNAs promote the degradation of target genes after combining with their mRNAs (Wang J. et al., 2020). Interestingly, Schistosoma japonicum egg-derived miRNA-33 upregulates TGF-β receptor expression in HSCs, and miRNA-33 can promote TGF-β receptor expression by enhancing mRNA stability (Wang et al., 2022). Another schistosome egg-derived miRNA-71a targets semaphorin 4D(Sema4D) to play an inhibitory role in liver fibrosis (Wang L. et al., 2020). Wnt/β-catenin signaling is involved in protein construction and modification, and the Wnt/β-catenin signaling pathway can activate HSCs and promote the expression of connective tissue growth factor (CTGF) and TGF-β (Wang Q. et al., 2017). Secreted frizzed-related protein 1 (SFRP1) is an extracellular homologous protein of the Wnt receptor that exerts negative regulation by competing with the wnt receptor to bind Wnt ligands (Zhang H. et al., 2019). Schistosoma-derived miRNA-1 promotes SSLF by targeting SFRP1 in HSCs and upregulating Wnt/β-catenin signaling (Wang Y. et al., 2020). MiRNAs originating from schistosome-infected hosts are also involved in regulating SSLF. FOXO1 is a main downstream effector of the PI3K/AKT signaling pathway, and FOXO1 is involved in regulating the cell cycle, apoptosis, autophagy, and other physiological processes (Xing et al., 2018). MiRNA-182 promotes the activation and proliferation of HSCs by targeting and inhibiting FOXO1 expression (Huang et al., 2018). Let-7b is a member of the Let miRNA family, and Let-7b targets the 3’ UTR of type I collagen mRNA to inhibit collagen deposition in the ECM (Sun X. et al., 2021). Overexpression of Let-7b by recombinant lentivirus transfection dramatically relieves SSLF and suppresses Th1 and Th2 type immune responses (Tang et al., 2017). IL-33 is an important pro-SSLF cytokine. miRNA-203-3p can target and inhibit IL-33 expression, while overexpression of miRNA-203-3p inhibited SSLF by downregulation of IL-33 levels (He et al., 2018b). KH-type splicing regulatory protein (KSRP) is an RNA-binding protein that targets the 3’UTR-rich AU region of TGF-β1 mRNA and promotes degradation by reducing its stability (Sundaram et al., 2013). miRNA-27b increased the mRNA stability of TGF-β by inhibiting KSRP expression and miRNA-27b knockdown could significantly inhibit SSLF (Wang S. et al., 2020). Vitamin D receptor (VDR) signaling promotes the dissociation of the Smad proteome from DNA to inhibit liver fibrosis (Ding et al., 2013). miRNA-351 inhibits VDR signaling and reduces the inhibitory effect of VDR signaling on the Samd proteasome (He et al., 2018a). MiRNA-21 inhibits Smad7 expression to promote TGF-β/Smad signaling-mediated liver fibrosis, and chlorogenic acid inhibits miRNA-21 expression to suppress SSLF (Wang Y. et al., 2017; Cui et al., 2021). miR-130a-3p inhibits the expression of the TGF-β receptor, which suppresses the TGF-β signaling-mediated expression of fibrosis-related genes (Liu et al., 2021a). miR-200a can negatively regulate SSLF, which could be related to the downregulation of TGF-β2 levels, but the target genes of miRNA-200a in SSLFs are unclear (Xu et al., 2021). TLR2 signaling plays an important role in SSLF development, and overexpression of miR-92a-2-5p could block TLR2 signaling (Zhao et al., 2019). miRNAs are widely involved in the post-transcriptional regulation of genes and provide interesting protein molecules for researching SSLF (Table 1).
NLRP3 inflammasome and TLR2/4 signaling pathway
NLRP3 inflammasome
NLRP3 is a member of the NOD-like receptors (NLRs) family, which is a multiprotein complex that plays an important role in SSLF (Lu et al., 2017; Mangan et al., 2018). The NLRP3 inflammasome consists of NLRP3, adaptor protein apoptosis-associated speck-like protein (ASC), and pro-caspase-1, which is activated by various agonists (Meng et al., 2016; Zhang W. J. et al., 2019). The three main molecular mechanisms of NLRP3 activation are ROS production, increased lysosomal membrane permeability, potassium efflux, and caspase-1 shearing of precursors of IL-1β and IL-18 to promote their activation (Meng et al., 2016; Lu et al., 2017; Zhang W. J. et al., 2019; Zhen and Zhang, 2019). IL-1β promotes the activation and proliferation of HSCs and recruits high levels of inflammatory cytokines, and also induces the activation of T cells and macrophages to promote worm egg granulomas and liver fibrosis (Palacios-Macapagal et al., 2017; Liu et al., 2019).
There are high levels of IL-1β and IL-18 in the liver of schistosome-infected mice, and in HSC cells with highly activated NLRP3 inflammasome (Meng et al., 2016). Dendritic cell-associated C-type lectin-1 (Dectin-1) is a C-type lectin-like pattern receptor that recruits and phosphorylates spleen tyrosine kinase (Syk) (Sun W. et al., 2021). Activation of NLRP3 in HSCs caused by schistosome infections depends on the Dectin-1/Syk signaling pathway (Figure 2), while inhibition of NLRP3 inflammasome activation significantly reduces egg granulomas and liver fibrosis (Lu et al., 2017). Phosphorylated syk(p-syk) promotes activation of the NLRP3 inflammasome by phosphorylated ASC, and promotes NADPH oxidase2 (NOX2) signaling producing ROS to indirectly activate the NLRP3 inflammasome (Figure 2) (Lu et al., 2017; Yu et al., 2021). Induction of caspase-1-dependent apoptosis by ROS mediates extensive inflammatory damage in schistosome-infected mice, and ROS blockades inhibit inflammation and promote M1-type polarization of macrophages (Kong et al., 2019). Oxidative stress promotes the dissociation of thioredoxin-interacting protein (TXNIP) from thioredoxin and TXNIP can activate the NLRP3 inflammasome, but taurine inhibits inflammation by inhibiting the TXNIP/NLRP3 signaling pathway (Liu et al., 2019). Treatment of schistosome-infected mice with vitamin E could reduce liver fibrosis and egg granulomas (Wang et al., 2012). Vitamin E could be involved in regulating SSLF by inhibiting ROS production and relieving inflammatory damage, though its regulatory mechanism is unclear (Figure 2).
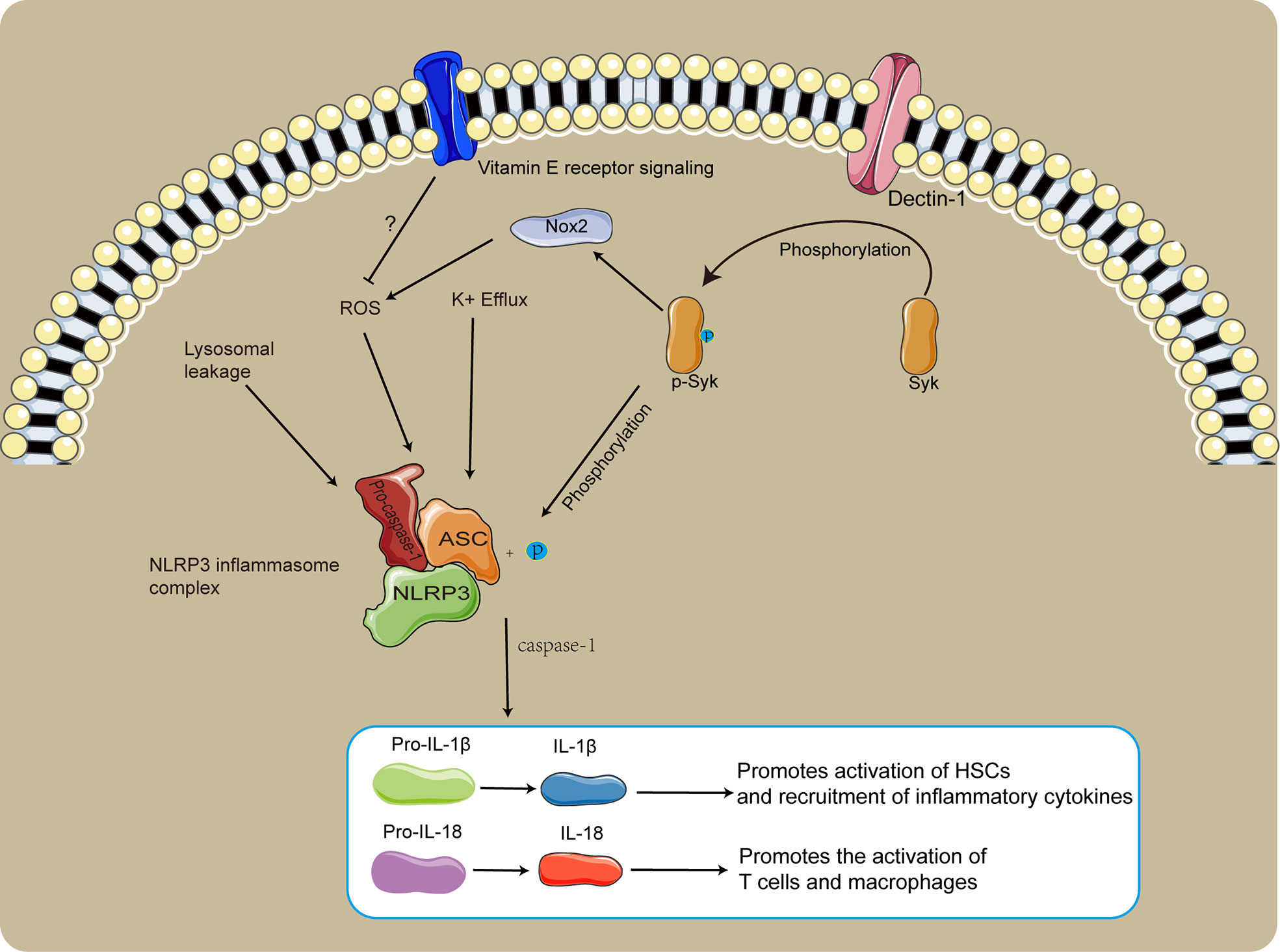
Figure 2 The role of NLRP3 in SSLF. The Dectin-1/Syk signaling pathway promotes NLRP3 inflammasome activation via phosphorylation of ASC. p-syk dependent NOX2 induced production of ROS facilitates NLRP3 inflammasome activation. Vitamin E likely inhibits SSLF by suppressing ROS production and relieving inflammatory injury.
High activation of NLRP3 in the liver of schistosome-infected mice promoted extensive tissue damage and inflammatory responses, which induced connective tissue proliferation promoting SSLF development (Meng et al., 2016; Carson et al., 2018; Zhang W. J. et al., 2019). Inhibiting excessive activation of the inflammatory response and tissue damage by inhibiting NLRP3 signaling has a positive significance for SSLF therapy.
TLR2/4 signaling pathway
The toll-like receptor (TLR) is a pattern recognition receptor that senses endogenous and exogenous dangerous molecules to produce high levels of pro-inflammatory cytokines, which activate lymphocytes to generate adaptive immune responses and destroy invading pathogens or damaged cells (Lim and Staudt, 2013; Chen L. et al., 2021). The TLR family consists of 13 molecules that are widely expressed on immune and non-immune cells in mice. Of these, TLR2/4 is the most important receptor for the recognition of schistosome components (Zhang et al., 2011; Liu et al., 2020). However, TLR2 and TLR4 have different effects on schistosome infection. TLR2 deficiency reduces egg burden and promotes T cell activation, and TLR4 deficiency aggravates schistosome immunopathology and liver fibrosis (Zhang et al., 2011; Liu et al., 2020). TLR2 deficiency upregulates IFN-γ and IL-4, which could be related to TLR2 signaling-induced regulatory T cell differentiation (Layland et al., 2007; Burton et al., 2010). TLR2 signaling in dendritic cells promotes PD-L2 expression to maintain immunosuppression (Gao et al., 2013). TLR2 signaling induced by SEA stimulation promotes M2 polarization in macrophages, which is beneficial for developing liver fibrosis (Gong et al., 2018). TLR2 signaling can contribute to maintaining the immunosuppressive state of the schistosome-infected microenvironment and suppressing T cell activation (Zhang et al., 2011). TLR4 signaling promotes HSC activation and ECM deposition by activating the COX2/PGE2 axis to upregulate Prostaglandin E2 (PGE2) expression (Chen L. et al., 2021). TLR4 signaling promotes M1-type polarization of macrophages, which has the opposite effect of TLR2 (Xu et al., 2014). TLR2/4 plays an important role in regulating the Th1/Th2 balance, which is highly correlated with the development of SLLF. However, the role of TLR2/4 in schistosome infections requires further study.
TGF-β/Smad axis and IL-13 signing promote SSLF
TGF-β/Smad axis
There are three subtypes of TGF-β with partially overlapping functions. In liver fibrosis, the most extensively researched is TGF-β1, which is the main pro-fibrotic cytokine driving HSC activation and proliferation (Dewidar et al., 2019). Chronic liver injuries induced by schistosome infections provoke a continuous inflammatory response, and liver sinusoidal endothelial cells (LSECs) are the first cell type to be affected after liver injuries (Parola and Pinzani, 2019; Ruart et al., 2019). Chronic inflammation is maintained by the increased production of reactive oxygen radicals (ROS) and other oxidative stress products (Ruart et al., 2019). Kupffer cells also play an important role in sensing liver injuries and triggering inflammation. With the continued depletion of Kupffer cells, the liver recruits mononuclear-derived macrophages to maintain chronic inflammation and liver fibrosis (Tacke and Zimmermann, 2014; Wang et al., 2018). Persistent liver injuries and chronic inflammatory responses mediated by schistosome infections are important factors in the development of liver fibrosis. The type II immune microenvironment created by schistosome infections promotes TGF-β1 secretion by macrophages, hepatic stellate cells, and hepatocytes in the liver (Li Y. Q. et al., 2017; Wang S. et al., 2020). Inflammatory and injurious stimulants induce high levels of TGF-β, and TGF-β binding to TGF-β receptor complexes promotes SMAD2/SMAD3 phosphorylation (Dewidar et al., 2019). Phosphorylated Smad2/Smad3 combines with Smad4 to form a protein complex, and the Smad complex performs nuclear translocation to promote transcription of target genes, with the expression of many fibrosis-related genes dependent on Smad3 (Chen B. L. et al., 2013; Chen et al., 2019b; Wang et al., 2022). SMAD3 has a low affinity for DNA; therefore, the Smad2/3/4 protein complex recruits transcriptional co-activators to stabilize the activation complex (Dewidar et al., 2019). Smad protein complexes promote collagen deposition by enhancing the expression of liver fibrosis-related genes and matrix metalloproteinase inhibitors (He et al., 2018a). Phosphorylated Smad1/5/8 competes with phosphorylated Smad2/3 to bind Smad4 to suppress the transcription of liver fibrosis-related genes, and BMP-7 promotes the phosphorylation of Smad1/5/8 in hepatic stellate cells (Chen et al., 2019b). Intense TGF-β signaling induces excessive inflammatory responses and collagen deposition, while also activating the negative feedback regulation of TGF-β signaling by Smad7 (Yang et al., 2020). Smad7 is an interesting target for anti-SSLF therapy, and Smad7 overexpression in HSCs significantly relieves liver fibrosis (Dooley et al., 2008). Sedum sarmentosum Total Flavonoids (SSTF) have anti-inflammatory and antioxidant effects, which induce high expression of Smad7 in HSCs to inhibit TGF-β signaling in Schistosoma infection (Yang et al., 2020). However, the mechanism behind SSTF-induced high expression of Smad7 has not yet been researched. Previous studies have demonstrated that Sema4Dis constitutively expressed on T cells and is involved in regulating T cell activation (Kuklina et al., 2017). However, schistosome infections induce HSCs highly expressing Sema4D and Sema4D receptors, and Sema4D signaling enhances TGF-β signaling, while inhibition of Sema4D significantly reduces SSLF (Wang L. et al., 2020). As a natural flavonoid with anti-inflammatory and antioxidant effects, myricetin can inhibit TGF-β1/smad signaling in HSCs to inhibit SSLF (Huang et al., 2020). TGF-β1/Samd signaling promotes increased expression of CTGF, which promotes HSC activation and ECM deposition (Kamdem et al., 2018). TGF-β has various biological functions, and the inhibition of TGF-β signaling has a positive effect during antifibrotic therapy for schistosomiasis (Dewidar et al., 2019; Larson et al., 2020). Current research indicates that TGF-β/Smad is an important pro-fibrotic signal in schistosome infections. The targeted regulation of TGF-β/Smad signaling could provide a breakthrough for antifibrotic therapy of schistosomiasis (Table 2).
IL-13
Both parenchymal and immune cells can produce IL-13 in the liver of schistosome-infected mice, and IL-13 signaling induces the expression of type I collagen and other fibrosis-related genes in HSCs (Liu et al., 2012; Zheng et al., 2015). The IL-13 signaling receptor complex consists of IL-4Rα, IL-13Rα1, and IL13Rα2 (Figueiredo et al., 2016). IL-13Rα1 combines with IL-4Rα1 to constitute a functional receptor for IL-13 signaling, and IL-13 Rα2 is a bait receptor capable of blocking IL-13 signaling (Rahaman et al., 2002; Zheng et al., 2015). However, schistosome infections induced high levels of IL-13 Rα2 expression by macrophages, which are positively correlated with the development of liver fibrosis (Wang et al., 2009). Gadolinium chloride (GdCl3) relieves SSLF by scavenging macrophages and decreasing IL13Rα2 levels in the liver, which is likely related to the reduction of TGF-β production by macrophages induced by IL13Rα2 (Zheng et al., 2015). Macrophage IL-13Rα2 inhibits Matrix metalloproteinase 13(MMP-13) expression and thus ECM degradation, and Matrix metalloproteinase 12(MMP-12) deficient inhibition of IL-13Rα2 expression recovers the activity of MMP-13 (Madala et al., 2010). Specific knockdown of IL-13 Rα2 in macrophages could result in important discoveries relevant to SSLF research, however, none have yet been reported.
IL-13 combines with the IL-13 functional receptor to promote JAK/STAT6 phosphorylation, and phosphorylated STAT6 to constitute homodimers and nuclear translocations to induce the expression of fibrosis-related genes (Du et al., 2016; Li et al., 2016; Wang L. et al., 2020). IL-13 activates the ERK-MAPK signaling pathway to promote Smad1/2 phosphorylation and increase CTGF expression to induce HSC activation; however, this must be confirmed in SSLF (Liu et al., 2011; Wang Y. et al., 2017). Many antifibrotic drugs inhibit IL-13 signaling to inhibit the progression of SSLF, such as paeoniflorin, corilagin, and chlorogenic acid (Li et al., 2010; Li et al., 2016; Wang Y. et al., 2017). C/EBP Homologous Protein (CHOP) is an endoplasmic reticulum stress-induced transcriptional regulator, which inhibits the IL-13/STAT6 signaling pathway to suppress M2 macrophage polarization and SSLF (Duan et al., 2019). Sema4D combined with its receptor upregulates IL-13 signaling to promote SSLF, and targeted inhibition of Sema4D significantly inhibits SSLF (Wang L. et al., 2020). IL-13 signaling plays an important role in the development of SSLF (Table 2). However, numerous cell types secrete IL-13 in the liver. Therefore, we recommend analyzing total IL-13 levels in the liver or researching IL-13 downstream signaling regulation in specific cell types.
IL-17 and IL-33 promote SSLF
IL-17
IL-17 is a pro-inflammatory cytokine involved in many inflammatory and infectious diseases, including schistosomiasis, and blocking IL-17 significantly reduces egg granuloma and liver fibrosis (Zhang et al., 2015). T Helper 17 (Th17) cells, natural killer T (NKT) cells, and gamma delta (γδ) T cells are important sources of IL-17 (Webster et al., 2014; Sun et al., 2020). In the schistosome-infected liver, γδ T cells account for 1-2% of all T lymphocytes, however, the most important source of IL-17 is γδ T cells (Chen D. et al., 2013). γδ T cell deficiency reduces IL-17 levels in the liver, and IL-17 levels tend to be positively correlated with the progression of SSLF (Sun et al., 2020). The γδ T cells in the schistosome-infected liver are mainly composed of Vγ1 and Vγ2, with Vγ1 γδ T cells secreting IFN-γ and Vγ2 γδ T cells secreting both IFN-γ and IL-17A (Zheng et al., 2017). IL-17 receptors are highly expressed on the surface of neutrophils, while IL-17 secreted by Vγ2 γδ T cells recruited neutrophils to migrate to the area of egg granulomas and TGF-β secreted by neutrophils promotes the progression of SSLF (Zheng et al., 2017; Sun et al., 2020). IL-17 induces Type I collagen gene expression in HSC cells by phosphorylating STAT3 to promote liver fiber (Zhou et al., 2019). ApoE-deficient mice have a severe lipid metabolism disorder and hyperactivated inflammatory response. High levels of IL-17 in the liver of schistosome-infected ApoE-deficient mice aggravate egg granulomas and fibrosis (Guan et al., 2020). The role of IL-17 in promoting schistosome immunopathology does not need to be emphasized; however, the mechanism of IL-17 regulation of SSLF requires further research (Table 2).
IL-33
IL-33 is a part of the IL-1 family and is normally present in the nucleus and released into the extracellular compartment in response to tissue damage (Bai et al., 2021). IL-33 plays an important role in immunity to schistosome infection, and IL-33 levels are positively correlated with the progression of egg granuloma and liver fibrosis (Zhang et al., 2021). IL-33 induces macrophage M2-type polarization and releases IL-5 and IL-13 to promote liver fibrosis (Li et al., 2019). IL-33 promotes the recruitment of Group 2 innate lymphoid cells (ILC2s) in the liver, and secretion of IL-13 by ILC2s promotes the development of liver fibrosis (He et al., 2018b). IL-33 contributes to the maintenance of type II immune microenvironments in the liver of schistosome-infected mice. During chronic infections, Hepatic progenitor cells (HPCs) inhibit the secretion of IL-33 to promote tissue restoration and inhibit the development of liver fibrosis (Zhang et al., 2021). Tissue Transglutaminase (tTG) regulates cell death and cytoskeletal rearrangement, however, tTG is also involved in the regulation of SSLF. The tTG upregulation of IL-33 promotes liver fibrosis and worm egg granuloma (Tang et al., 2015; Li et al., 2019). ST2 is a unique IL-33 receptor, and appropriate inhibition of IL-33/ST2 reduces the immunopathology of schistosomes; however, knockdown of IL-33 or ST2 exacerbates schistosomiasis liver pathology (Li et al., 2019; Bai et al., 2021). IL-33 deficiency could activate excessive Th17 immune response. The IL-33/ST2 axis is influenced by multiple immune cells and cytokines during immunity to Schistosoma infection. There could be additional receptors for IL-33 besides ST2. Therefore, we recommend exploring additional receptors for IL-33 and lateral regulation of the IL-33/ST2 axis (Table 2).
The role of HMGB1 in SSLF
High mobility group box-1 protein (HMGB1) is a highly conserved nuclear DNA binding protein that plays a regulatory role in gene transcription as a structural cofactor (Chen et al., 2004). HMGB1 can be released from dead cells or secreted from intrinsic immune cells, and HMGB1 promotes the secretion of inflammatory cytokines (Rauvala and Rouhiainen, 2007). HMGB1 promotes the proliferation and activation of HSCs to promote liver fibrosis during SSLF progression, and high levels of HMGB1 at the injury site recruit fibroblasts, endothelial cells, and smooth muscle cells to promote tissue injury repair (Vicentino et al., 2018; Chen H. et al., 2021). However, this decompensation repair has resulted in liver fibrosis due to persistent inflammation and injuries, and HMGB1 promotes the migration of immune cells to the area of egg granulomas (Vicentino et al., 2018). HMGB1 inhibitors significantly inhibit SSLF, which indicates that HMGB1 plays a role in promoting liver fibrosis, though its mechanism is unknown (Vicentino et al., 2018; Chen H. et al., 2021). HMGB1 is derived from passive leakage from dead cells and primary release from intrinsic immune cells, and the primary source and mechanism of HMGB1 in SSLF must be clarified. In conclusion, high levels of HMGB1 were positively correlated with SLLF, HMGB1 was extensively post-translationally modified, and the role of HMGB1 in SSLF must be further investigated (Chen et al., 2004).
NK cells and NKT cells
NK cells are natural immune cells that directly lyse or induce apoptosis of target cells by secreting granzyme, perforin, and FasL/Fas pathways (Liu et al., 2021b). The natural killer group 2, member D(NKG2D), is an essential NK cell activation and function-related molecule, and retinoic acid early induction 1 (RAE 1) is one of the ligands of NKG2D (Hou et al., 2012a). At 2 to 4 weeks of schistosome infection, HSC cells are highly expressive of RAE 1 and tumor necrosis factor-related apoptosis-inducing ligand (TRAIL), which facilitate NK cell recognition and the killing of HSCs (Hou et al., 2012a; Hu et al., 2020). Murine UL16-binding protein-like transcript 1 (MULT1) is the strongest ligand for NKG2D, and overexpression of MULT1 enhances NK cell activity to inhibit SSLF (Yang et al., 2022). NK cells express a variety of TLRs, and schistosome infection induces high TLR3 expression in NK cells (Qu et al., 2018). Polyinosinic-polycytidylic acid (poly I:C)-TLR3 signaling post-translationally promotes NKG2D expression and IFN-g secretion in NK cells (Hou et al., 2012b). NK cells secrete IFN-γ to induce HSC apoptosis and block the cell cycle, and the absence of NK cells promotes SSLF (Hou et al., 2012a). In general, IFN-g secretion by NK cells and direct killing of HSCs inhibited SSLF in the early stages of schistosome infections. However, with schistosome egg production, the number of NK cells in the liver increased but their activities were dramatically inhibited (Hu et al., 2020). Therefore, it is important to identify how to restore NK cell activity to resist SSLF.
NKT is an innate immune cell subset that expresses both NK and T cell markers but is essentially a specialized T cell subset (Faveeuw et al., 2008). NKT cells can be classified as either invariant NKT(iNKT) or non-invariant NKT(non-iNKT) based on the variability of their TCR (Noma et al., 2015). CD1d is a highly conserved MHC class I molecule, and NKT cell recognition of APC-presented antigenic peptides depends on CD1d (Nishioka et al., 2018). Antigen-peptide processing by antigen-presenting cells stimulates NKT cells to produce high levels of IFN-γ and IL-4; however, this conflicting effect is undertaken by different NKT subsets (Lei et al., 2021). iNKT cells promote upregulated levels of IFN-γ, while non-iNKT cells promote upregulated levels of IL-4, which indicates that these two cell types could play opposite roles in the development of SSLF (Mallevaey et al., 2007). Schistosoma haematobium infections lead to increased numbers of NKT cells in the liver and decreased CD1d expression in hepatocytes, which could be associated with CD1d-mediated apoptosis of NKT cells (Lei et al., 2021). IL-30 promotes the recruitment of NKT cells in the liver and upregulates the expression of NKG2D, promoting the killing of HSCs by NKT cells (Mitra et al., 2014). However, the role of IL-30 in SSLF has yet to be validated. The major cell subsets of NKT cells could play opposite roles in SSLF, and research targeting NKT cell subsets could lead to breakthroughs for SSLF therapy.
Conclusion
Liver fibrosis caused by schistosomiasis is still a problem when treating schistosomiasis, and anti-fibrosis therapy are important for improving the quality of life of patients and restoring their liver function. The main reasons for liver fibrosis are continuous liver injury and inflammatory stimulation. The most important difference between SSLF and other types of liver fibrosis is the persistent damage and inflammatory response mediated by eggs and SEA, which is unique to SSLF. SEA inhibits the proliferation and activation of HSCs, and schistosome-derived miRNAs are involved in regulating SSLF. The levels of expression of some critical pro-fibrotic cytokines could be affected by schistosomes. The schistosome infection microenvironment is composed of extremely complex components that could play opposing roles in the progression of liver fibrosis. Therefore, we must understand the functions and mechanisms of the molecules involved in regulating SSLF. This paper reviews the mechanisms of the major molecules or signaling pathways involved in the development of SSLF and discusses potential future research topics.
Author contributions
ZL and LZ drafted and revised the manuscript, and LL and YL reviewed and revised the manuscript for publication. All authors contributed to the article and approved the submitted version.
Funding
This work was supported by the National Natural Science Foundation of China (32070898 to YL, 81471595 to YL, 32170879 to LZ, 81601360 to LZ, 81501342 to LL, and U1904157 to LL).
Conflict of interest
The authors declare that the research was conducted in the absence of any commercial or financial relationships that could be construed as a potential conflict of interest.
Publisher’s note
All claims expressed in this article are solely those of the authors and do not necessarily represent those of their affiliated organizations, or those of the publisher, the editors and the reviewers. Any product that may be evaluated in this article, or claim that may be made by its manufacturer, is not guaranteed or endorsed by the publisher.
References
Andrade, Z. A. (2008). Schistosomiasis and hepatic fibrosis regression. Acta Trop. 108 (2-3), 79–82. doi: 10.1016/j.actatropica.2008.04.003
Anthony, B. J., James, K. R., Gobert, G. N., Ramm, G. A., McManus, D. P. (2013). Schistosomajaponicum eggs induce a proinflammatory, anti-fibrogenic phenotype in hepatic stellate cells. PloS One 8 (6), e68479. doi: 10.1371/journal.pone.0068479
Anthony, B., Mathieson, W., de Castro-Borges, W., Allen, J. (2010). Schistosoma mansoni: egg-induced downregulation of hepatic stellate cell activation and fibrogenesis. Exp. Parasitol. 124 (4), 409–420. doi: 10.1016/j.exppara.2009.12.009
Bai, Y., Guan, F., Zhu, F., Jiang, C., Xu, X., Zheng, F., et al. (2021). IL-33/ST2 axis deficiency exacerbates hepatic pathology by regulating treg and Th17 cells in murine schistosomiasis japonica. J. Inflamm. Res. 14, 5981–5998. doi: 10.2147/JIR.S336404
Burton, O. T., Gibbs, S., Miller, N., Jones, F. M., Wen, L., Dunne, D. W., et al. (2010). Importance of TLR2 in the direct response of T lymphocytes to schistosoma mansoni antigens. Eur. J. Immunol. 40 (8), 2221–2229. doi: 10.1002/eji.200939998
Carson, J. P., Ramm, G. A., Robinson, M. W., McManus, D. P., Gobert, G. N. (2018). Schistosome-induced fibrotic disease: The role of hepatic stellate cells. Trends Parasitol. 34 (6), 524–540. doi: 10.1016/j.pt.2018.02.005
Chatterjee, S., Mbaye, A., Alfidja, A. T., Weyler, J., Scott, J. T., Van Damme, P., et al. (2004). Circulating levels of the neuropeptide hormone somatostatin may determine hepatic fibrosis in schistosoma mansoni infections. Acta Trop. 90 (2), 191–203. doi: 10.1016/j.actatropica.2003.12.002
Chen, Q. R., Guan, F., Song, S. M., Jin, J. K., Lei, D. S., Chen, C. M., et al. (2014). Allograft inflammatory factor-1 alleviates liver disease of BALB/c mice infected with schistosoma japonicum. Parasitol. Res. 113 (7), 2629–2639. doi: 10.1007/s00436-014-3915-8
Chen, L., Ji, X., Wang, M., Liao, X., Liang, C., Tang, J., et al. (2021). Involvement of TLR4 signaling regulated-COX2/PGE2 axis in liver fibrosis induced by schistosoma japonicum infection. Parasit. Vectors 14 (1), 279. doi: 10.1186/s13071-021-04790-7
Chen, H., Li, G., Zhang, J., Zheng, T., Chen, Q., Zhang, Y., et al. (2021). Sodium butyrate ameliorates schistosoma japonicum-induced liver fibrosis by inhibiting HMGB1 expression. Exp. Parasitol. 231, 108171. doi: 10.1016/j.exppara.2021.108171
Chen, D., Luo, X., Xie, H., Gao, Z., Fang, H., Huang, J. (2013). Characteristics of IL-17 induction by schistosoma japonicum infection in C57BL/6 mouse liver. Immunology 139 (4), 523–532. doi: 10.1111/imm.12105
Chen, B. L., Peng, J., Li, Q. F., Yang, M., Wang, Y., Chen, W. (2013). Exogenous bone morphogenetic protein-7 reduces hepatic fibrosis in schistosoma japonicum-infected mice via transforming growth factor-beta/Smad signaling. World J. Gastroenterol. 19 (9), 1405–1415. doi: 10.3748/wjg.v19.i9.1405
Chen, R., Wang, J., Gradinaru, I., Vu, H. S., Geboers, S., Naidoo, J., et al. (2022). A male-derived nonribosomal peptide pheromone controls female schistosome development. Cell. 185 (9), 1506–1520.e17. doi: 10.1016/j.cell.2022.03.017
Chen, G., Ward, M., Sama, A. E., Wang, H. (2004). Extracellular HMGB1 as a proinflammatory cytokine. Off. J. Int. Soc. Interferon Cytokine Res. 24 (6), 329–333. doi: 10.1089/107999004323142187
Chen, J., Xu, T., Zhu, D., Wang, J., Huang, C., Lyu, L., et al. (2016). Egg antigen p40 of schistosoma japonicum promotes senescence in activated hepatic stellate cells by activation of the STAT3/p53/p21 pathway. Cell Death Dis. 7 (7), e2315. doi: 10.1038/cddis.2016.228
Chen, L., Yu, Y., Liu, E., Duan, L., Zhu, D., Chen, J., et al. (2019a). Schistosoma japonicum soluble egg antigen inhibits TNF-alpha-induced IL-34 expression in hepatic stellate cells. Parasitol. Res. 118 (2), 551–557. doi: 10.1007/s00436-018-6165-3
Chen, L., Zhou, Q., Liu, E., Zhang, J., Duan, L., Zhu, D., et al. (2019b). rSjp40 inhibits activated hepatic stellate cells by promoting nuclear translocation of YB1 and inducing BMP-7/Smad1/5/8 pathway. Parasit. Vectors 12 (1), 279. doi: 10.1186/s13071-019-3539-z
Chiaramonte, M. G., Mentink-Kane, M., Jacobson, B. A., Cheever, A. W., Whitters, M. J., Goad, M. E., et al. (2003). Regulation and function of the interleukin 13 receptor alpha 2 during a T helper cell type 2-dominant immune response. J. Exp. Med. 197 (6), 687–701. doi: 10.1084/jem.20020903
Clerinx, J., Van Gompel, A. (2011). Schistosomiasis in travellers and migrants. Travel Med. Infect. Dis. 9 (1), 6–24. doi: 10.1016/j.tmaid.2010.11.002
Colley, D. G., Bustinduy, A. L., Secor, W. E., King, C. H. (2014). Human schistosomiasis. Lancet 383 (9936), 2253–2264. doi: 10.1016/s0140-6736(13)61949-2
Cui, W., Zhou, S., Wang, Y., Shi, X., Liu, H. (2021). Cadmium exposure activates the PI3K/AKT signaling pathway through miRNA-21, induces an increase in M1 polarization of macrophages, and leads to fibrosis of pig liver tissue. Ecotoxicol. Environ. Saf. 228, 113015. doi: 10.1016/j.ecoenv.2021.113015
De Marco Verissimo, C., Potriquet, J., You, H., McManus, D. P., Mulvenna, J., Jones, M. K. (2019). Qualitative and quantitative proteomic analyses of schistosoma japonicum eggs and egg-derived secretory-excretory proteins. Parasit. Vectors 12 (1), 173. doi: 10.1186/s13071-019-3403-1
Dewidar, B., Meyer, C., Dooley, S., Meindl-Beinker, A. N. (2019). TGF-beta in hepatic stellate cell activation and liver fibrogenesis-updated 2019. Cells 8 (11), 1419. doi: 10.3390/cells8111419
Ding, H., Yang, X., Tian, J., Wang, X., Ji, Y., El-Ashram, S., et al. (2021). JQ-1 ameliorates schistosomiasis liver fibrosis by suppressing JAK2 and STAT3 activation. BioMed. Pharmacother. 144, 112281. doi: 10.1016/j.biopha.2021.112281
Ding, N., Yu, R. T., Subramaniam, N., Sherman, M. H., Wilson, C., Rao, R., et al. (2013). A vitamin d receptor/SMAD genomic circuit gates hepatic fibrotic response. Cell 153 (3), 601–613. doi: 10.1016/j.cell.2013.03.028
Dooley, S., Hamzavi, J., Ciuclan, L., Godoy, P., Ilkavets, I., Ehnert, S., et al. (2008). Hepatocyte-specific Smad7 expression attenuates TGF-beta-mediated fibrogenesis and protects against liver damage. Gastroenterology 135 (2), 642–659. doi: 10.1053/j.gastro.2008.04.038
Duan, M., Yang, Y., Peng, S., Liu, X., Zhong, J., Guo, Y., et al. (2019). C/EBP homologous protein (CHOP) activates macrophages and promotes liver fibrosis in schistosoma japonicum-infected mice. J. Immunol. Res. 2019, 5148575. doi: 10.1155/2019/5148575
Du, P., Ma, Q., Zhu, Z. D., Li, G., Wang, Y., Li, Q. Q., et al. (2016). Mechanism of corilagin interference with IL-13/STAT6 signaling pathways in hepatic alternative activation macrophages in schistosomiasis-induced liver fibrosis in mouse model. Eur. J. Pharmacol. 793, 119–126. doi: 10.1016/j.ejphar.2016.11.018
Faveeuw, C., Mallevaey, T., Trottein, F. (2008). Role of natural killer T lymphocytes during helminthic infection. Parasite 15 (3), 384–388. doi: 10.1051/parasite/2008153384
Figueiredo, A. L., Domingues, A. L., Melo, W. G., Tashiro, T., de Lorena, V. M., Montenegro, S. M., et al. (2016). Receptor antagonist of IL-13 exerts a potential negative regulation during early infection of human schistosomiasis. Scand. J. Immunol. 84 (5), 284–290. doi: 10.1111/sji.12472
Gao, Y., Chen, L., Hou, M., Chen, Y., Ji, M., Wu, H., et al. (2013). TLR2 directing PD-L2 expression inhibit T cells response in schistosoma japonicum infection. PloS One 8 (12), e82480. doi: 10.1371/journal.pone.0082480
Gong, W., Huang, F., Sun, L., Yu, A., Zhang, X., Xu, Y., et al. (2018). Toll-like receptor-2 regulates macrophage polarization induced by excretory-secretory antigens from schistosoma japonicum eggs and promotes liver pathology in murine schistosomiasis. PloS Negl. Trop. Dis. 12 (12), e0007000. doi: 10.1371/journal.pntd.0007000
Guan, F., Zhang, C., Jiang, C., Jacques, M. L., Bai, Y., Lu, S., et al. (2020). ApoE deficiency promotes hepatic pathology by aggravating Th17/Treg imbalance in murine schistosomiasis japonica. Parasite Immunol. 42 (12), e12785. doi: 10.1111/pim.12785
He, X., Sun, Y., Lei, N., Fan, X., Zhang, C., Wang, Y., et al. (2018a). MicroRNA-351 promotes schistosomiasis-induced hepatic fibrosis by targeting the vitamin d receptor. Proc. Natl. Acad. Sci. U.S.A. 115 (1), 180–185. doi: 10.1073/pnas.1715965115
He, X., Xie, J., Wang, Y., Fan, X., Su, Q., Sun, Y., et al. (2018b). Down-regulation of microRNA-203-3p initiates type 2 pathology during schistosome infection via elevation of interleukin-33. PloS Pathog. 14 (3), e1006957. doi: 10.1371/journal.ppat.1006957
Hoffmann, K. F., Cheever, A. W., Wynn, T. A. (2000). IL-10 and the dangers of immune polarization: excessive type 1 and type 2 cytokine responses induce distinct forms of lethal immunopathology in murine schistosomiasis. J. Immunol. 164 (12), 6406–6416. doi: 10.4049/jimmunol.164.12.6406
Hou, X., Yu, F., Man, S., Huang, D., Zhang, Y., Liu, M., et al. (2012a). Negative regulation of schistosoma japonicum egg-induced liver fibrosis by natural killer cells. PloS Negl. Trop. Dis. 6 (1), e1456. doi: 10.1371/journal.pntd.0001456
Hou, X., Yu, F., Man, S., Huang, D., Zhang, Y., Liu, M., et al. (2012b). Polyinosinic-polycytidylic acid attenuates hepatic fibrosis in C57BL/6 mice with schistosoma japonicum infection. Acta Trop. 121 (2), 99–104. doi: 10.1016/j.actatropica.2011.10.008
Huang, Y., Fan, X., Tao, R., Song, Q., Wang, L., Zhang, H., et al. (2018). Effect of miR-182 on hepatic fibrosis induced by schistosomiasis japonica by targeting FOXO1 through PI3K/AKT signaling pathway. J. Cell Physiol. 233 (10), 6693–6704. doi: 10.1002/jcp.26469
Huang, J. Q., Tao, R., Li, L., Ma, K., Xu, L., Ai, G., et al. (2014). Involvement of heat shock protein 47 in schistosoma japonicum-induced hepatic fibrosis in mice. Int. J. Parasitol. 44 (1), 23–35. doi: 10.1016/j.ijpara.2013.08.009
Huang, P., Zhou, M., Cheng, S., Hu, Y., Gao, M., Ma, Y., et al. (2020). Myricetin possesses anthelmintic activity and attenuates hepatic fibrosis via modulating TGFbeta1 and akt signaling and shifting Th1/Th2 balance in schistosoma japonicum-infected mice. Front. Immunol. 11. doi: 10.3389/fimmu.2020.00593
Hu, Y., Wang, X., Wei, Y., Liu, H., Zhang, J., Shen, Y., et al. (2020). Functional inhibition of natural killer cells in a BALB/c mouse model of liver fibrosis induced by schistosoma japonicum infection. Front. Cell Infect. Microbiol. 10. doi: 10.3389/fcimb.2020.598987
Kamdem, S. D., Moyou-Somo, R., Brombacher, F., Nono, J. K. (2018). Host regulators of liver fibrosis during human schistosomiasis. Front. Immunol. 9. doi: 10.3389/fimmu.2018.02781
Kong, D. L., Kong, F. Y., Liu, X. Y., Yan, C., Cui, J., Tang, R. X., et al. (2019). Soluble egg antigen of schistosoma japonicum induces pyroptosis in hepatic stellate cells by modulating ROS production. Parasit. Vectors 12 (1), 475. doi: 10.1186/s13071-019-3729-8
Kuklina, E. M., Nekrasova, I. V., Valieva, Y. V. (2017). Involvement of semaphorin (Sema4D) in T-dependent activation of b cells. Bull. Exp. Biol. Med. 163 (4), 447–450. doi: 10.1007/s10517-017-3825-8
Larson, C., Oronsky, B., Carter, C. A., Oronsky, A., Knox, S. J., Sher, D., et al. (2020). TGF-beta: a master immune regulator. Expert Opin. Ther. Targets 24 (5), 427–438. doi: 10.1080/14728222.2020.1744568
Layland, L. E., Rad, R., Wagner, H., da Costa, C. U. (2007). Immunopathology in schistosomiasis is controlled by antigen-specific regulatory T cells primed in the presence of TLR2. Eur. J. Immunol. 37 (8), 2174–2184. doi: 10.1002/eji.200737063
Lei, Z., Tang, R., Qi, Q., Gu, P., Wang, J., Xu, L., et al. (2021). Hepatocyte CD1d protects against liver immunopathology in mice with schistosomiasis japonica. Immunology 162 (3), 328–338. doi: 10.1111/imm.13288
Li, Y. Q., Chen, Y. F., Dang, Y. P., Wang, Y., Shang, Z. Z., Ma, Q., et al. (2017). Corilagin counteracts IL-13Ralpha1 signaling pathway in macrophages to mitigate schistosome egg-induced hepatic fibrosis. Front. Cell Infect. Microbiol. 7. doi: 10.3389/fcimb.2017.00443
Li, H. R., Li, G., Li, M., Zhang, S. L., Wang, H., Luo, T., et al. (2016). Corilagin ameliorates schistosomiasis hepatic fibrosis through regulating IL-13 associated signal pathway in vitro and in vivo. Parasitology 143 (12), 1629–1638. doi: 10.1017/S0031182016001128
Lim, K. H., Staudt, L. M. (2013). Toll-like receptor signaling. Cold Spring Harb. Perspect. Biol. 5 (1), a011247. doi: 10.1101/cshperspect.a011247
Li, X., Shen, J., Zhong, Z., Peng, J., Wen, H., Li, J., et al. (2010). Paeoniflorin ameliorates schistosomiasis liver fibrosis through regulating IL-13 and its signalling molecules in mice. Parasitology 137 (8), 1213–1225. doi: 10.1017/S003118201000003X
Liu, S., Galat, V., Galat, Y., Lee, Y. K. A., Wainwright, D., Wu, J. (2021). NK cell-based cancer immunotherapy: from basic biology to clinical development. J. Hematol. Oncol. 14 (1), 7. doi: 10.1186/s13045-020-01014-w
Liu, Y., Meyer, C., Muller, A., Herweck, F., Li, Q., Mullenbach, R., et al. (2011). IL-13 induces connective tissue growth factor in rat hepatic stellate cells via TGF-beta-independent smad signaling. J. Immunol. 187 (5), 2814–2823. doi: 10.4049/jimmunol.1003260
Liu, Y., Munker, S., Mullenbach, R., Weng, H. L. (2012). IL-13 signaling in liver fibrogenesis. Front. Immunol. 3. doi: 10.3389/fimmu.2012.00116
Liu, L., Wang, P., Wang, Y. S., Zhang, Y. N., Li, C., Yang, Z. Y., et al. (2021). MiR-130a-3p alleviates liver fibrosis by suppressing HSCs activation and skewing macrophage to Ly6C(lo) phenotype. Front. Immunol. 12. doi: 10.3389/fimmu.2021.696069
Liu, X., Zhang, Y. R., Cai, C., Ni, X. Q., Zhu, Q., Ren, J. L., et al. (2019). Taurine alleviates schistosoma-induced liver injury by inhibiting the TXNIP/NLRP3 inflammasome signal pathway and pyroptosis. Infect. Immun. 87 (12), e00732–19. doi: 10.1128/IAI.00732-19
Liu, C., Zhang, Y. S., Chen, F., Wu, X. Y., Zhang, B. B., Wu, Z. D., et al. (2020). Immunopathology in schistosomiasis is regulated by TLR2,4- and IFN-gamma-activated MSC through modulating Th1/Th2 responses. Stem Cell Res. Ther. 11 (1), 217. doi: 10.1186/s13287-020-01735-2
Li, Z. Y., Xiao, L., Lin, G., Tang, J., Chen, Y., Chen, L., et al. (2019). Contribution of tissue transglutaminase to the severity of hepatic fibrosis resulting from schistosoma japonicum infection through the regulation of IL-33/ST2 expression. Parasit. Vectors 12 (1), 302. doi: 10.1186/s13071-019-3542-4
Li, L., Xie, H., Wang, M., Qu, J., Cha, H., Yang, Q., et al. (2017). Characteristics of IL-9 induced by schistosoma japonicum infection in C57BL/6 mouse liver. Sci. Rep. 7 (1), 2343. doi: 10.1038/s41598-017-02422-8
Luo, J., Liang, Y., Kong, F., Qiu, J., Liu, X., Chen, A., et al. (2017). Vascular endothelial growth factor promotes the activation of hepatic stellate cells in chronic schistosomiasis. Immunol. Cell Biol. 95 (4), 399–407. doi: 10.1038/icb.2016.109
Lu, Y. Q., Zhong, S., Meng, N., Fan, Y. P., Tang, W. X. (2017). NLRP3 inflammasome activation results in liver inflammation and fibrosis in mice infected with schistosoma japonicum in a syk-dependent manner. Sci. Rep. 7 (1), 8120. doi: 10.1038/s41598-017-08689-1
Madala, S. K., Pesce, J. T., Ramalingam, T. R., Wilson, M. S., Minnicozzi, S., Cheever, A. W., et al. (2010). Matrix metalloproteinase 12-deficiency augments extracellular matrix degrading metalloproteinases and attenuates IL-13-dependent fibrosis. J. Immunol. 184 (7), 3955–3963. doi: 10.4049/jimmunol.0903008
Mallevaey, T., Fontaine, J., Breuilh, L., Paget, C., Castro-Keller, A., Vendeville, C., et al. (2007). Invariant and noninvariant natural killer T cells exert opposite regulatory functions on the immune response during murine schistosomiasis. Infect. Immun. 75 (5), 2171–2180. doi: 10.1128/IAI.01178-06
Mangan, M. S. J., Olhava, E. J., Roush, W. R., Seidel, H. M., Glick, G. D., Latz, E. (2018). Targeting the NLRP3 inflammasome in inflammatory diseases. Nat. Rev. Drug Discovery 17 (8), 588–606. doi: 10.1038/nrd.2018.97
McManus, D. P., Bergquist, R., Cai, P., Ranasinghe, S., Tebeje, B. M., You, H. (2020). Schistosomiasis-from immunopathology to vaccines. Semin. Immunopathol. 42 (3), 355–371. doi: 10.1007/s00281-020-00789-x
Mei, C., Yang, Y., Dong, P., Song, L., Zhou, Y., Xu, Y., et al. (2022). Deficiency of PKClambda/iota alleviates the liver pathologic impairment of schistosoma japonicum infection by thwarting Th2 response. Parasit. Vectors 15 (1), 154. doi: 10.1186/s13071-022-05283-x
Meng, N., Xia, M., Lu, Y. Q., Wang, M., Boini, K. M., Li, P. L., et al. (2016). Activation of NLRP3 inflammasomes in mouse hepatic stellate cells during schistosoma j. infection. Oncotarget 7 (26), 39316–39331. doi: 10.18632/oncotarget.10044
Mewamba, E. M., Nyangiri, O. A., Noyes, H. A., Egesa, M., Matovu, E., Simo, G. (2021). The genetics of human schistosomiasis infection intensity and liver disease: A review. Front. Immunol. 12. doi: 10.3389/fimmu.2021.613468
Mitra, A., Satelli, A., Yan, J., Xueqing, X., Gagea, M., Hunter, C. A., et al. (2014). IL-30 (IL27p28) attenuates liver fibrosis through inducing NKG2D-rae1 interaction between NKT and activated hepatic stellate cells in mice. Hepatology 60 (6), 2027–2039. doi: 10.1002/hep.27392
Nishioka, Y., Masuda, S., Tomaru, U., Ishizu, A. (2018). CD1d-restricted type II NKT cells reactive with endogenous hydrophobic peptides. Front. Immunol. 9. doi: 10.3389/fimmu.2018.00548
Noma, H., Eshima, K., Satoh, M., Iwabuchi, K. (2015). Differential dependence on nuclear factor-kappaB-inducing kinase among natural killer T-cell subsets in their development. Immunology 146 (1), 89–99. doi: 10.1111/imm.12484
Palacios-Macapagal, D., Connor, J., Mustelin, T., Ramalingam, T. R., Wynn, T. A., Davidson, T. S. (2017). Cutting edge: Eosinophils undergo caspase-1-Mediated pyroptosis in response to necrotic liver cells. J. Immunol. 199 (3), 847–853. doi: 10.4049/jimmunol.1601162
Parola, M., Pinzani, M. (2019). Liver fibrosis: Pathophysiology, pathogenetic targets and clinical issues. Mol. Aspects Med. 65, 37–55. doi: 10.1016/j.mam.2018.09.002
Qu, J., Li, L., Xie, H., Zhang, X., Yang, Q., Qiu, H., et al. (2018). TLR3 modulates the response of NK cells against schistosoma japonicum. J. Immunol. Res. 2018, 7519856. doi: 10.1155/2018/7519856
Rahaman, S. O., Sharma, P., Harbor, P. C., Aman, M. J., Vogelbaum, M. A., Haque, S. J. (2002). IL-13R(alpha)2, a decoy receptor for IL-13 acts as an inhibitor of IL-4-dependent signal transduction in glioblastoma cells. Cancer Res. 62 (4), 1103–1109.
Rauvala, H., Rouhiainen, A. (2007). RAGE as a receptor of HMGB1 (Amphoterin): roles in health and disease. Curr. Mol. Med. 7 (8), 725–734. doi: 10.2174/156652407783220750
Ruart, M., Chavarria, L., Camprecios, G., Suarez-Herrera, N., Montironi, C., Guixe-Muntet, S., et al. (2019). Impaired endothelial autophagy promotes liver fibrosis by aggravating the oxidative stress response during acute liver injury. J. Hepatol. 70 (3), 458–469. doi: 10.1016/j.jhep.2018.10.015
Santarelli, R., Carillo, V., Romeo, M. A., Gaeta, A., Nazzari, C., Gonnella, R., et al. (2019). STAT3 phosphorylation affects p53/p21 axis and KSHV lytic cycle activation. Virology 528, 137–143. doi: 10.1016/j.virol.2018.12.015
Sokolow, S. H., Wood, C. L., Jones, I. J., Lafferty, K. D., Kuris, A. M., Hsieh, M. H., et al. (2018). To reduce the global burden of human schistosomiasis, use ‘Old fashioned’ snail control. Trends Parasitol. 34 (1), 23–40. doi: 10.1016/j.pt.2017.10.002
Stadecker, M. J., Asahi, H., Finger, E., Hernandez, H. J., Rutitzky, L. I., Sun, J. (2004). The immunobiology of Th1 polarization in high-pathology schistosomiasis. Immunol. Rev. 201, 168–179. doi: 10.1111/j.0105-2896.2004.00197.x
Sundaram, G. M., Common, J. E., Gopal, F. E., Srikanta, S., Lakshman, K., Lunny, D. P., et al. (2013). ‘See-saw’ expression of microRNA-198 and FSTL1 from a single transcript in wound healing. Nature 495 (7439), 103–106. doi: 10.1038/nature11890
Sun, L., Gong, W., Shen, Y., Liang, L., Zhang, X., Li, T., et al. (2020). IL-17A-producing gammadelta T cells promote liver pathology in acute murine schistosomiasis. Parasit. Vectors 13 (1), 334. doi: 10.1186/s13071-020-04200-4
Sun, W., Wang, H., Hu, H., Ma, X., Zhang, H., Chen, J., et al. (2021). Cutting edge: EPHB2 is a coreceptor for fungal recognition and phosphorylation of syk in the dectin-1 signaling pathway. J. Immunol. 206 (7), 1419–1423. doi: 10.4049/jimmunol.2001373
Sun, X., Zhang, L., Jiang, Y., Li, A., Zhu, D., Wu, J., et al. (2021). The role of let-7b in the inhibition of hepatic stellate cell activation by rSjP40. PloS Negl. Trop. Dis. 15 (6), e0009472. doi: 10.1371/journal.pntd.0009472
Tacke, F., Zimmermann, H. W. (2014). Macrophage heterogeneity in liver injury and fibrosis. J. Hepatol. 60 (5), 1090–1096. doi: 10.1016/j.jhep.2013.12.025
Tang, N., Wu, Y., Cao, W., Liang, Y., Gao, Y., Hu, L., et al. (2017). Lentivirus-mediated over-expression of let-7b microRNA suppresses hepatic fibrosis in the mouse infected with schistosoma japonicum. Exp. Parasitol. 182, 45–53. doi: 10.1016/j.exppara.2017.09.024
Tang, J., Zhu, X., Zhao, J., Fung, M., Li, Y., Gao, Z., et al. (2015). Tissue transglutaminase-regulated transformed growth factor-beta1 in the parasite links schistosoma japonicum infection with liver fibrosis. Mediators Inflamm. 2015, 659378. doi: 10.1155/2015/659378
Trottein, F., Nutten, S., Angeli, V., Delerive, P., Teissier, E., Capron, A., et al. (1999). Schistosoma mansoni schistosomula reduce e-selectin and VCAM-1 expression in TNF-alpha-stimulated lung microvascular endothelial cells by interfering with the NF-kappaB pathway. Eur. J. Immunol. 29 (11), 3691–3701. doi: 10.1002/(SICI)1521-4141(199911)29:11<3691::AID-IMMU3691>3.0.CO;2-L
Vicentino, A. R. R., Carneiro, V. C., Allonso, D., Guilherme, R. F., Benjamim, C. F., Dos Santos, H. A. M., et al. (2018). Emerging role of HMGB1 in the pathogenesis of schistosomiasis liver fibrosis. Front. Immunol. 9. doi: 10.3389/fimmu.2018.01979
Wang, J., Aldahamsheh, O., Ferrena, A., Borjihan, H., Singla, A., Yaguare, S., et al. (2021). The interaction of SKP2 with p27 enhances the progression and stemness of osteosarcoma. Ann. N Y Acad. Sci. 1490 (1), 90–104. doi: 10.1111/nyas.14578
Wang, C., Chen, W. J., Wu, Y. F., You, P., Zheng, S. Y., Liu, C. C., et al. (2018). The extent of liver injury determines hepatocyte fate toward senescence or cancer. Cell Death Dis. 9 (5), 575. doi: 10.1038/s41419-018-0622-x
Wang, Q., Chou, X., Guan, F., Fang, Z., Lu, S., Lei, J., et al. (2017). Enhanced wnt signalling in hepatocytes is associated with schistosoma japonicum infection and contributes to liver fibrosis. Sci. Rep. 7 (1), 230. doi: 10.1038/s41598-017-00377-4
Wang, Y., Fan, X., Lei, N., He, X., Wang, X., Luo, X., et al. (2020). A MicroRNA derived from schistosoma japonicum promotes schistosomiasis hepatic fibrosis by targeting host secreted frizzled-related protein 1. Front. Cell Infect. Microbiol. 10. doi: 10.3389/fcimb.2020.00101
Wang, Y., Gong, W., Zhou, H., Hu, Y., Wang, L., Shen, Y., et al. (2022). A novel miRNA from egg-derived exosomes of schistosoma japonicum promotes liver fibrosis in murine schistosomiasis. Front. Immunol. 13. doi: 10.3389/fimmu.2022.860807
Wang, L., Liao, Y., Yang, R., Yu, Z., Zhang, L., Zhu, Z., et al. (2020). Sja-miR-71a in schistosome egg-derived extracellular vesicles suppresses liver fibrosis caused by schistosomiasis via targeting semaphorin 4D. J. Extracell. Vesicles 9 (1), 1785738. doi: 10.1080/20013078.2020.1785738
Wang, S., Li, M., Zhao, X., Wang, H., Zhu, J., Wang, C., et al. (2020). Upregulation of KSRP by miR-27b attenuates schistosomiasis-induced hepatic fibrosis by targeting TGF-beta1. FASEB J. 34 (3), 4120–4133. doi: 10.1096/fj.201902438R
Wang, J., Paz, C., Padalino, G., Coghlan, A., Lu, Z., Gradinaru, I., et al. (2020). Large-Scale RNAi screening uncovers therapeutic targets in the parasite schistosoma mansoni. Science 369 (6511), 1649–1653. doi: 10.1126/science.abb7699
Wang, W., Shen, Y., Li, J., Zhang, S. H., Luo, Q. L., Zhong, Z. R., et al. (2009). Enhanced expression of the decoy receptor IL-13Ralpha2 in macrophages of schistosoma japonicum-infected mice. Chin. Med. J. 122 (14), 1650–1654. doi: 10.3760/cma.j.issn.0366-6999.2009.14.009
Wang, Y., Yang, F., Xue, J., Zhou, X., Luo, L., Ma, Q., et al. (2017). Antischistosomiasis liver fibrosis effects of chlorogenic acid through IL-13/miR-21/Smad7 signaling interactions In vivo and In vitro. Antimicrob. Agents Chemother. 61 (2), e01347–16. doi: 10.1128/AAC.01347-16
Wang, X., Zhang, R., Du, J., Hu, Y., Xu, L., Lu, J., et al. (2012). Vitamin e reduces hepatic fibrosis in mice with schistosoma japonicum infection. Mol. Med. Rep. 5 (2), 465–468. doi: 10.3892/mmr.2011.654
Webster, K. E., Kim, H. O., Kyparissoudis, K., Corpuz, T. M., Pinget, G. V., Uldrich, A. P., et al. (2014). IL-17-producing NKT cells depend exclusively on IL-7 for homeostasis and survival. Mucosal Immunol. 7 (5), 1058–1067. doi: 10.1038/mi.2013.122
Xing, Y. Q., Li, A., Yang, Y., Li, X. X., Zhang, L. N., Guo, H. C. (2018). The regulation of FOXO1 and its role in disease progression. Life Sci. 193, 124–131. doi: 10.1016/j.lfs.2017.11.030
Xu, T., Chen, J., Zhu, D., Chen, L., Wang, J., Sun, X., et al. (2017). Egg antigen p40 of schistosoma japonicum promotes senescence in activated hepatic stellate cells via SKP2/P27 signaling pathway. Sci. Rep. 7 (1), 275. doi: 10.1038/s41598-017-00326-1
Xu, J., Zhang, H., Chen, L., Zhang, D., Ji, M., Wu, H., et al. (2014). Schistosoma japonicum infection induces macrophage polarization. J. Biomed. Res. 28 (4), 299–308. doi: 10.7555/jbr.27.20130072
Xu, A., Zhong, G., Wang, J., Liu, C., Liu, Y., Wang, W. (2021). MicroRNA 200a inhibits liver fibrosis of schistosoma. Bioengineered 12 (1), 4736–4746. doi: 10.1080/21655979.2021.1950441
Yang, P. C., Bai, W. Z., Wang, J., Yan, C. H., Huang, W. F., Jiang, S. Z. (2020). Sedum sarmentosum total flavonoids alleviate schistosomiasis-induced liver fibrosis by altering TGF-beta1 and Smad7 expression. Evid. Based Complement Alternat. Med. 2020, 2083697. doi: 10.1155/2020/2083697
Yang, L., Sun, L., Cao, Y., Wang, Q., Song, A., Zhu, R., et al. (2022). MULT1-encoding DNA alleviates schistosomiasis-associated hepatic fibrosis via modulating cellular immune response. J. Inflammation Res. 15, 4027–4045. doi: 10.2147/JIR.S354224
Yu, Y. R., Ni, X. Q., Huang, J., Zhu, Y. H., Qi, Y. F. (2016). Taurine drinking ameliorates hepatic granuloma and fibrosis in mice infected with schistosoma japonicum. Int. J. Parasitol. Drugs Drug Resist. 6 (1), 35–43. doi: 10.1016/j.ijpddr.2016.01.003
Yu, Y., Wang, J., Wang, X., Gu, P., Lei, Z., Tang, R., et al. (2021). Schistosome eggs stimulate reactive oxygen species production to enhance M2 macrophage differentiation and promote hepatic pathology in schistosomiasis. PloS Negl. Trop. Dis. 15 (8), e0009696. doi: 10.1371/journal.pntd.0009696
Zhang, W. J., Fang, Z. M., Liu, W. Q. (2019). NLRP3 inflammasome activation from kupffer cells is involved in liver fibrosis of schistosoma japonicum-infected mice via NF-kappaB. Parasit. Vectors 12 (1), 29. doi: 10.1186/s13071-018-3223-8
Zhang, M., Gao, Y., Du, X., Zhang, D., Ji, M., Wu, G. (2011). Toll-like receptor (TLR) 2 and TLR4 deficiencies exert differential in vivo effects against schistosoma japonicum. Parasite Immunol. 33 (4), 199–209. doi: 10.1111/j.1365-3024.2010.01265.x
Zhang, Y., Huang, D., Gao, W., Yan, J., Zhou, W., Hou, X., et al. (2015). Lack of IL-17 signaling decreases liver fibrosis in murine schistosomiasis japonica. Int. Immunol. 27 (7), 317–325. doi: 10.1093/intimm/dxv017
Zhang, H., Sun, D., Qiu, J., Yao, L. (2019). SFRP1 inhibited the epithelial ovarian cancer through inhibiting wnt/beta-catenin signaling. Acta Biochim. Pol. 66 (4), 393–400. doi: 10.18388/abp.2019_2757
Zhang, B., Wu, X., Li, J., Ning, A., Zhang, B., Liu, J., et al. (2021). Hepatic progenitor cells promote the repair of schistosomiasis liver injury by inhibiting IL-33 secretion in mice. Stem Cell Res. Ther. 12 (1), 546. doi: 10.1186/s13287-021-02589-y
Zhan, T., Ma, H., Jiang, S., Zhong, Z., Wang, X., Li, C., et al. (2019). Interleukin-9 blockage reduces early hepatic granuloma formation and fibrosis during schistosoma japonicum infection in mice. Immunology 158 (4), 296–303. doi: 10.1111/imm.13111
Zhao, Y., Dang, Z., Chong, S. (2019). Mmu-miR-92a-2-5p targets TLR2 to relieve schistosoma japonicum-induced liver fibrosis. Int. Immunopharmacol. 69, 126–135. doi: 10.1016/j.intimp.2019.01.007
Zhao, J., Liu, X., Chen, Y., Zhang, L. S., Zhang, Y. R., Ji, D. R., et al. (2021). STAT3 promotes schistosome-induced liver injury by inflammation, oxidative stress, proliferation, and apoptosis signal pathway. Infect. Immun. 89 (3), e00309–e00320. doi: 10.1128/IAI
Zheng, L., Hu, Y., Wang, Y., Huang, X., Xu, Y., Shen, Y., et al. (2017). Recruitment of neutrophils mediated by Vgamma2 gammadelta T cells deteriorates liver fibrosis induced by schistosoma japonicum infection in C57BL/6 mice. Infect. Immun. 85 (8), e01020–16. doi: 10.1128/IAI.01020-16
Zheng, S., Lu, Q., Xu, Y., Wang, X., Shen, J., Wang, W. (2015). GdCl3 attenuates schistosomiasis japonicum egg-induced granulomatosis accompanied by decreased macrophage infiltration in murine liver. PloS One 10 (8), e0132222. doi: 10.1371/journal.pone.0132222
Zheng, B., Zhang, J., Chen, H., Nie, H., Miller, H., Gong, Q., et al. (2020). T Lymphocyte-mediated liver immunopathology of schistosomiasis. Front. Immunol. 11. doi: 10.3389/fimmu.2020.00061
Zhen, Y., Zhang, H. (2019). NLRP3 inflammasome and inflammatory bowel disease. Front. Immunol. 10. doi: 10.3389/fimmu.2019.00276
Zhou, C. L., Kong, D. L., Liu, J. F., Lu, Z. K., Guo, H. F., Wang, W., et al. (2017). MHC II(-), but not MHC II(+), hepatic stellate cells contribute to liver fibrosis of mice in infection with schistosoma japonicum. Biochim. Biophys. Acta Mol. Basis Dis. 1863 (7), 1848–1857. doi: 10.1016/j.bbadis.2017.05.002
Zhou, W., Yang, Y., Mei, C., Dong, P., Mu, S., Wu, H., et al. (2019). Inhibition of rho-kinase downregulates Th17 cells and ameliorates hepatic fibrosis by schistosoma japonicum infection. Cells 8 (10), 1261. doi: 10.3390/cells8101262
Keywords: schistosomiasis, liver fibrosis, molecular mechanisms, soluble egg antigen, cytokines
Citation: Liu Z, Zhang L, Liang Y and Lu L (2022) Pathology and molecular mechanisms of Schistosoma japonicum-associated liver fibrosis. Front. Cell. Infect. Microbiol. 12:1035765. doi: 10.3389/fcimb.2022.1035765
Received: 03 September 2022; Accepted: 13 October 2022;
Published: 28 October 2022.
Edited by:
Renyong Lin, Xinjiang Medical University, ChinaCopyright © 2022 Liu, Zhang, Liang and Lu. This is an open-access article distributed under the terms of the Creative Commons Attribution License (CC BY). The use, distribution or reproduction in other forums is permitted, provided the original author(s) and the copyright owner(s) are credited and that the original publication in this journal is cited, in accordance with accepted academic practice. No use, distribution or reproduction is permitted which does not comply with these terms.
*Correspondence: Yinming Liang, eWlubWluZy5saWFuZ0BncmlzLm9yZy5jbg==; Liaoxun Lu, bHVsaWFveHVuQGdyaXMub3JnLmNu