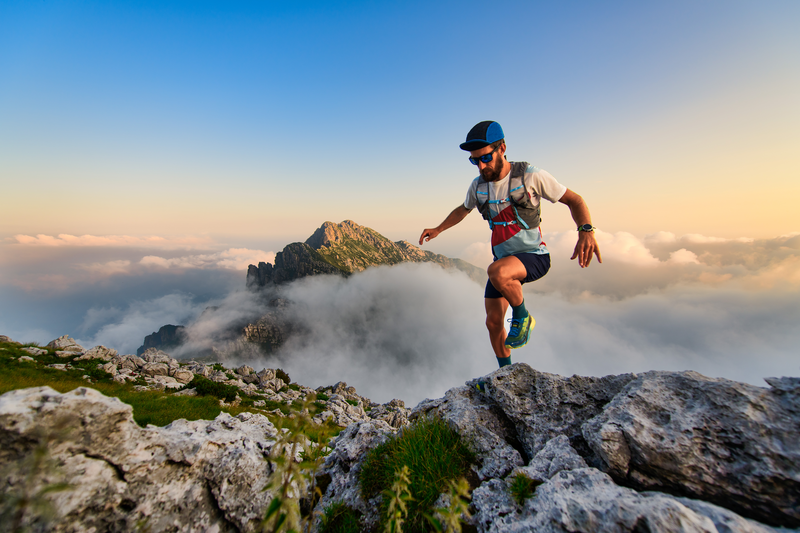
95% of researchers rate our articles as excellent or good
Learn more about the work of our research integrity team to safeguard the quality of each article we publish.
Find out more
ORIGINAL RESEARCH article
Front. Cell. Infect. Microbiol. , 28 November 2022
Sec. Clinical Microbiology
Volume 12 - 2022 | https://doi.org/10.3389/fcimb.2022.1023243
This article is part of the Research Topic Brucella: intracellular survival, epidemiology, prevention and therapy View all 5 articles
Introduction: Brucellosis is a highly prevalent zoonotic disease caused by Brucella spp. Brucella suis S2 vaccination is an effective strategy to prevent animal brucellosis. However, S2 induces antibodies against the smooth lipopolysaccharide,making it challenging to distinguish field infected from vaccinated livestock. Early and accurate diagnosis is essential for infection control and prevention. In this study, we aimed to develop a quick and accurate assay to distinguish the BrucellaS2 vaccine strain from closely related B. abortus and B. melitensis.
Methods: Whole-genome sequencing of B. suis S2 was performed, and the sequence was compared with that of the genomes of B. abortus and B. melitensis. One specific gene, GL_0002189, was selected as a marker to differentiate the BrucellaS2vaccine strain from B. abortus and B. melitensis. A loop-mediated isothermal amplification (LAMP) assay was developed, based on the GL_0002189 gene, and then assessed for target specificity, lower limit of detection, and repeatability.
Results: Our results revealed that there was no cross-reaction with other strains, and the LAMP assay displayed high sensitivity for detecting S2 with a minimum detection limit of 18.9×103 copies/µL DNA input, it is nearly 100 times higher than conventional PCR technology. Concordance between the LAMP assay and a conventional polymerase chain reaction method was assessed using 54 blood samples collected from sheep with suspected brucellosis. Total concordance between the two assays was 92.6%, without a significant difference (p > 0.05) in the test results.
Conclusion: This is the first report of a LAMP assay for the detection of the B. suis S2vaccine strain. Our approach can be helpful for the control and eradication of brucellosis, and its simplicity in requiring no specialized equipment or personnel makes it useful for implementation in resource-limited settings as well as for field use.
Brucellosis, caused by Brucella spp., is a serious zoonotic disease, which is prevalent worldwide (Kamga et al., 2020). It causes serious economic loses for farmers through the reduction of productivity (El-Sayed and Awad, 2018). The clinical symptoms caused by brucellosis include abortion, infertility, decreased production, and undulating fever with arthralgia in humans (Cao et al., 2018). In some economically developed countries, brucellosis in domestic animals is mainly purified by detection and positive killing to achieve prevention and control. In China, in recent years, brucellosis in cattle, sheep, and humans has increased to varying degrees since low prevalence areas do not carry out vaccine immunization, and the positive animals are eliminated to purify the herds. In areas with severe numbers of brucellosis cases, the infection in cattle and sheep can be prevented and controlled by vaccination. According to the survey, cattle, sheep and caprine brucellosis is predominantly related to B. melitensis, as well as B. abortus (Dadar and Alamian, 2021). The live attenuated vaccine strains play an important role in the prevention and control of animal and human brucellosis (Goodwin and Pascual, 2016). Although attenuated vaccines do not completely protect against Brucella spp. infection, vaccination is currently the most effective prevention and control strategy against brucellosis (Wang et al., 2020). In China, live attenuated Brucella suis vaccine (Brucella suis S2) is the most widely used brucellosis vaccine. The Brucella S2 vaccine is quite effective in preventing livestock brucellosis, such as in sheep, goats, cattle and pigs (Zhu et al., 2016). It has many advantages, including high safety, efficacy, and multiple vaccination routes. Nevertheless, S2 induces antibodies against the smooth lipopolysaccharide, making it challenging to distinguish field infected from vaccinated sheep, cattle and pigs.
Diagnosis of brucellosis is mainly based on bacteriological and immunological tests, which include Rose-Bengal plate agglutination test, standard tube agglutination test, Fluorescence polarization assay, complement fixation test, and enzyme-linked immunosorbent assay (Ducrotoy et al., 2018). Therefore, under the reality of vaccination against brucellosis, it is urgent to develop a differential diagnosis approach for commonly used vaccines. The advantages of Loop Mediated Isothermal Amplification (LAMP) analysis are that it is easy to operate, requires less special equipment, has high accuracy, can be directly interpreted by naked eye, and can be used in places with limited instrument resources (Trangoni et al., 2015). The colorimetric LAMP assay is rapid and can be used in the field, such as farm sites, as it does not require specialized equipment and personnel (Sathish Kumar et al., 2021).
The qPCR method was used to detect the target nucleic acid in the peripheral blood of 54 sheep. The target gene encoded the Brucella ABC transporter permease (NCBI Reference Sequence: WP_002965788.1), which is a common gene of the Brucella wild strain and B.suis S2 vaccine strain. The B.suis S2 vaccine is a live attenuated vaccine, after inoculation, nucleic acid of the B.suis S2 vaccine strain can be detected. Therefore, qPCR test results cannot determine whether 54 sheep are infected with the Brucella wild strain or B.suis S2 attenuated vaccine, and further differentiation is needed. In this study, comparative genomics research methods were used to sequence the whole genome of the widely used Brucella S2 vaccine in China, analyze and compare it with the genomes of six classical species of Brucella published by GenBank, and screen out several differential genes, including GL_0002189 which was selected as a marker gene to distinguish Brucella S2 vaccine from Brucella melitensis and Brucella abortus. Therefore, this study is based on the LAMP detection method using GL_0002189 marker gene for the distinguishing of Brucella S2 vaccine strain from the aforementioned strains. In addition, we evaluated the specificity, lower detection limit and repeatability of LAMP (GL_0002189). Lastly, we planned to compare concordance between the LAMP assay and a conventional PCR-based approach in order to investigate the clinical utility of the LAMP assay in low-resource settings as well as for field use. Our methods help prevent and control brucellosis.
B. abortus S19, B. abortus A19, B. melitensis Rev.1, B. melitensis M28, and B. suis S2 were tested in this study along with three non-Brucella species, namely Streptococcus, Salmonella, and Staphylococcus aureus. Genomic DNA was extracted from the isolates using the Genomic Template Extraction Kit (Tian gen Biotech Co. Ltd, Beijing, China), according to the manufacturer’s protocol, and quantified using Nanodrop (Gene Company Limited).
Whole-genome sequencing for B. suis S2 was performed using Illumina HiSeq 2000. A comparative genomics investigation was carried out with the whole-genome sequences of the field epidemic strains, B. melitensis and B. abortus, using the Basic Local Alignment Search Tool (BLAST) of the National Center for Biotechnology Information (NCBI) (https://blast.ncbi.nlm.nih.gov/Blast.cgi). The essential data of Brucella spp. are shown in Table 1.
A BLAST alignment of the target gene was performed against Brucella spp. genomes published on the NCBI database to investigate percentage of identity. Simultaneously, designed PCR primers for target gene. The forward and backward primers utilized were 5′-CCTACAACCCATCGAAGTGG-3′ and 5′-TCTTGAGCAAGAACCAGCAC-3′, respectively. The one-step assay, which takes approximately 3 h to complete, was composed of one denaturing cycle at 95°C for 5 min, followed by 30 cycles at 94°C for 45 s, 56°C for 45 s, and 72°C for 45 s, and the final extension step was performed at 72°C for 10 min. The PCR amplification products were analyzed using electrophoresis on 1% agarose gel. The reaction components for the PCR included 12.5 μL Premix Taq (Takara Biomedical Technology Co., Ltd., Beijing, China), 1μL of forward and backward primers (final concentration was 10 µmol/L), 1 μL of the DNA template, and then made up to 25 μL with DNase/RNase-free water.
The LAMP primers based on the target gene were designed using Primer Explorer V5 software (http://primerexplorer.jp/e/). The primer information is shown in Table 2.
The LAMP assay was optimized in a 25 μL reaction system, which included MgSO4 (New England Biolabs Co., Ltd, Beijing, China), dNTPs (Takara Biomedical Technology Co., Ltd., Beijing, China), and LAMP primers (Sangon Biotech Shanghai Co., Ltd., Shanghai, China). To improve the LAMP conditions, the reactions were assessed at various concentrations of MgSO4 (2–10 mmol/L), dNTPs (0.6–3.0 mmol/L), outer primers F3/B3 (0.1–0.5 µmol/L), inner primers FIP/BIP (0.8–2.8 µmol/L), loop primers LF/LB (0.1–1.0 µmol/L), and reaction temperatures (59–69°C). End-point analysis of the products of the assay was performed using agarose gel electrophoresis and a visual test, using SYBR Green I dye (Beijing Solarbio Science & Technology, Beijing, China). For the visual test, under visible light, fluorescent green indicated the presence of the amplicon, while orange indicated.
The specificity of the LAMP assay target gene, was analyzed using 10 ng/µL genomic DNA from the five Brucella strains and three non-Brucella strains. A 1 μL aliquot of genomic DNA was used as a template for LAMP reactions, and each sample was tested in triplicate.
Following the optimization of the LAMP assay, the sensitivity of the test was analyzed using 10-fold serial dilutions of 18.9×1010 copies/µL B. suis S2 DNA. For comparison, the 10-fold dilutions were further tested using PCR and the sensitivities of the two assays were compared.
The repeatability of the LAMP assay was assessed by repeating the experiment using three different batches of reagents and following the optimized reaction conditions. The main components of the three batches of reagents are identical, but they are produced at different times to test the stability of this method in different batches of reagents. Each sample was tested in triplicate.
Fifty-four blood samples were collected from sheep that were suspected of being infected with wild strains in April 2022, the samples were stored in EDTA anticoagulation tubes. The sheep had been vaccinated with the Brucella S2 vaccine the previous year. DNA was extracted from the blood samples using the Genomic Template Extraction Kit (Tian gen Biotech Co. Ltd), according to the manufacturer’s protocol. The initial testing was performed using quantitative PCR, which confirmed Brucella spp. infection in 52 of the samples. However, the qPCR target gene encoded the Brucella ABC transporter permease (NCBI Reference Sequence: WP_002965788.1), which is a common gene of the Brucella wild strain and B.suis S2 vaccine strain. Therefore, qPCR test results cannot determine whether 54 sheep are infected with the Brucella wild strain or B.suis S2 attenuated vaccine, and further differentiation is needed. Using this gene as a molecular detection target, the nucleic acid of the B. suis S2 vaccine strain was positively detected, while that of B. melitensis, B. abortus, and other control strains were negatively detected. Therefore, all 54 samples were tested using the LAMP and conventional PCR methods established in this study to distinguish between the strains, so as to assess the clinical practicability of the LAMP assay as a diagnostic tool. The animal study was reviewed and approved by the Animal Ethics and Welfare Committee of Inner Mongolia Agricultural University.
The genome of Brucella S2 vaccine strain was compared with that of six strains of B. abortus and B. melitensis. The results revealed a total of 20 B. suis S2-specific genes (Table 3), of which 7 genes with functional annotation were as follows: arginine ABC transporter permeability enzyme gene (GL000288), carmine transport permeability enzyme gene (GL000289), DNA replication and recombination gene (GL002175), phage gene (GL002176), protein binding gene (GL002179), type IV binding protein gene (GL002181), and endonuclease gene (GL002186). The remaining 13 genes are not functionally annotated. The GL_0002189 gene was viewed as a marker that can differentiate the Brucella S2 vaccine strain from B. abortus and B. melitensis. Analysis indicates that there are some genome-level differences among the different genotypes of Brucella.
The length of the GL_0002189 gene was 660 bp and the molecular weight was 24.1 kDa. The gene contained a 660bp complete open reading frame, encoding 219 amino acids. The protein encoded by this gene is hydrophilic, lacks transmembrane region, and is located in the outer bacterial membrane (https://trace.ncbi.nlm.nih.gov/Traces/index.html?view=study&acc=SRP394076). Based on the BLAST analysis, the GL_0002189 gene sequence showed high percentage of identity with B. suis, B. canis, and B. microti, but it was not homologous with B. abortus, B. melitensis, and B. ovis (Table 4). The PCR amplification products were analyzed using electrophoresis on 1% agarose gel. It was found that the expected sizes (201 bp) were amplified only in the S2 vaccine strain, but not amplified in the B. abortus and B. melitensis strains tested in this study (Figure 1).
Figure 1 Polymerase chain reaction results of the GL_0002/89 gene. It was fotmd that the expected sizes (201 bp) were amplified only in the S2 vaccine strain, but not amplified in the B. abortus and B.melitensis strains tested in this study.
Single-factor optimization of the LAMP reaction conditions was carried out for MgSO4, dNTPs, outer primers (F3/B3), inner primers (FIP/BIP), loop primers (LF/LB), and reaction temperature. Based on gel electrophoresis results, the amplicons that had the brightest bands on the agarose gel were produced using MgSO4 at 4 mmol/L, dNTPs at 1.8 mmol/L, F3/B3 at 0.2 µmol/L, FIP/BIP at 2.0 µmol/L, and LF/LB at 0.6 µmol/L. Therefore, these were considered optimal reaction conditions for the assay. Furthermore, while amplification was observed from 63–69°C, 65°C was chosen as the optimum temperature for the LAMP assay.
Figure 2 shows the visual assay used to determine the target specificity of the LAMP assay. Amplification was detected only for the B. suis S2 vaccine strain, indicated in fluorescent green, whereas no amplification was noted for the B. abortus and B. melitensis strains as well as the other three non-Brucella spp., indicated in orange. The results were also confirmed using agarose gel electrophoresis (Figure 3).
Figure 2 Target specificity of the LAMP assay. Fluorescent green indicates presence of the amplicon while orange indicates no amplification. Cross reaction was not observed when 10 ng/µL of DNA from B. suis S2, B. abortus Sl9, B.abortus Al9, B.melitensis Rev.1, B.melite11sis M28 strains, Staphylococcus aureus, Salmonella, Streptococcus DNA and negative control were used.
Figure 3 Target specificity of the L-\MP assay. Assay products were visualized using gel electrophoresis. Cross reaction was not observed when 10 ng/µL of DNA from B. suis S2, B. abortus SI9, B. abortus A19, B.melitensis Rev.1, B.melitensis M28 strains, Staphylococcus aureus, Salmonella, Streptococcus DNA and negative control were used.
The sensitivities of the LAMP and conventional PCR assays were evaluated and compared using serially diluted (10-fold) B. suis S2 DNA. The LAMP assay could detect as low as 18.9×10 (Goodwin and Pascual, 2016) copies/µL and 18.9×103 copies/µL of S2 genomic DNA based on the visual and electrophoretic methods (Figures 4, 5). Similarly, the conventional PCR assay could detect as low as 18.9×105 copies/µL, based on agarose gel analysis (Figure 6). Therefore, the LAMP method is nearly 100 times higher than conventional PCR technology.
Figure 4 Lower limit of detect ion of the LAMP assay for the detection of Brucella suis S2 vaccine strain. Assay products were visualized using color change to detect different DNA concentrations. Fluorescent green indicates presence of the amplicon while orange indicates no amplification.
Figure 5 Lower limit of detection of the LAMP assay for the detect ion of Brucella suis S2 vaccine strain. Assay products were visualized using gel electrophoresis.
Figure 6 Lower limit of detection of the PCR assay for the detection of Brucella suis S2 vaccine strain. Assay products were visualized using gel electrophoresis.
Repeatability of the LAMP assay was assessed by repeating the experiment using different batches of reagents. Based on the visual and electrophoretic methods, amplification was detected after using the three distinct batches of reagents, indicating the repeatability of the LAMP assay (Figures 7, 8).
Figure 7 Repeatability detection of the LAMP assay for the detection of Brucella suis 52 vaccine strain. Assay products were visualized using gel electrophoresis. M, DNA marker DlL_2000; Lanes 1-3 are different batches of reagents and Lanes 4 is a negative control. The main components of the three batches of reagents are identical, but they are produced at different times.
Figure 8 Repeatability detection of the LAMP assay for the detection of Brucella suis 52 vaccine strain. Assay products were visualized using color change. Pipe 1-3, three different batches of reagents. Fluorescent green indicates presence of the amplicon while orange indicates no amplification. The main components of the three batches of reagents are identical, but they are produced at different times.
The information provided in Table 5 compares detection of B.suis S2 vaccine strain reliability of the established LAMP method targeting the GL_0002189 gene with the conventional PCR method. The results showed B.suis S2 36 positive samples and 18 negative samples were successfully detected by LAMP, whereas B.suis S2 34 positive samples and 20 negative samples were successfully detected by conventional PCR. The positive coincidence rate of the two detection methods was 94.4% (34/36), the negative coincidence rate was 88.9%, and the total coincidence rate was 92.6%. There was no significant difference between the detection performance of the two methods, indicating that the LAMP method established in this study can be used to distinguish the B.suis S2 vaccine strain. The other 18 negative samples may be B.suis S2 vaccine strain or wild strains because the concentration of nucleic acid in their blood is lower than the detection limit of LAMP method. Therefore, to determine whether the 18 sheep were infected with the B.suis S2 vaccine strain or wild Brucella strains, a more sensitive detection method is needed.
Brucellosis is a highly prevalent infectious disease and poses a major public health threat worldwide (Saber Marouf et al., 2021). The disease is caused by Brucella spp. and can cause multiple organ damage in humans and animals with serious health complications (Elfaki et al., 2015; López-Santiago et al., 2019). Vaccination of animals against the disease, using the B. suis S2 live-attenuated vaccine, has played an important role in reducing the incidence of brucellosis in humans and animals in the past decades (Masjedian Jezi et al., 2019). An effective way to eliminate brucellosis in livestock is to timely diagnose, identify, and eliminate brucellosis positive cattle and sheep. In countries where vaccination is widely used to prevent and control brucellosis, an effective way to eliminate brucellosis in herds in a short time period is to adopt detection methods that can diagnose and identify brucellosis natural infection and the immune response of animals to the vaccine. However, it can be a challenge to distinguish field infected from vaccinated livestock using current laboratory methods (Wang et al., 2014).
For nearly half a century, many economically developed countries have adopted quarantine and culling to purify livestock, which has achieved the control and eradication of brucellosis. China has adopted regional prevention and control approach. In areas where brucellosis is seriously prevalent, the method of detection and killing of positive animals was also used. However, the continuous emergence of positive animals has led to the necessity of preventing and controlling brucellosis infection in cattle and sheep through vaccination. Infection can be latent, and if field infected livestock are not culled from production, they can act as reservoirs of the pathogen for future infections. Thus, the ability to differentiate between wild type and vaccine strains is essential for the implementation of effective infection control and prevention protocols.
Based on some conserved genes and antigens of Brucella, such as OMP25, BP26, BCSP31, it is not possible to distinguish B.suis S2 vaccine strains from wild strains using fluorescence polarization and ultrasensitive detection technologies such as qPCR. However, few studies have been conducted to identify genes that differ between vaccine strains and wild strains. The target gene GL_0002189 used in this study was screened by comparing the whole genome of B.suis S2 vaccine with that of the most prevalent B. melitensis and B. abortus strains. Therefore, using GL_0002189 gene knot combined with qPCR, or using this gene to prepare a standard antigen combined with fluorescence polarization, could achieve the goal of ultrasensitive differentiation between vaccine strains and wild strains. Therefore, this study emphasizes the specificity of the GL_0002189 gene, which could provide an important molecular target for many ultrasensitive diagnostic techniques. Fluorescence polarization, qPCR, ELISA, and other methods have shown excellent performance in disease detection, such as strong specificity and high sensitivity, but the instruments and equipment are generally expensive. In China and many developing countries, there are many small farms that do not have enough cost to buy instruments and equipment. The reason why LAMP technology was selected in this study is that it has low requirements for test instruments. Only a constant temperature device is needed, and the test results can be interpreted with the naked eye. This is a very convenient and low-cost detection method, the loop-mediated isothermal amplification strategy could be more widely used in regions with limited resources. However, this technology has some limitations. For example, the detection results are easily affected by aerosols due to the high sensitivity of the test (Notomi et al., 2015).
Investigation shows that B. melitensis and B. abortus are the main pathogens of Brucella infection in sheep, goats and cattle. Therefore, we analyzed the whole genome sequence of S2, compared it with the genome of B. melitensis and B. abortus, and screened some differential genes between the strain contained in the S2 vaccine natural virulent strains of B. melitensis and B. abortus. S2 vaccine specific GL_ 0002189 gene research LAMP detection method was used to distinguish B. melitensis, B. abortus, and S2 vaccine strains. However, this method still has several limitations. For example, it cannot detect B. suis field strain infections, although a large number of studies have reported that livestock are mainly infected with B. melitensis and B. abortus.
As shown in the results of this study, 36 out of 54 clinical samples were detected as the B.suis S2 vaccine strain. The other 18 negative samples may be negative because the concentration of nucleic acid in their blood is lower than the detection limit of this method. Therefore, to determine whether the 18 sheep were infected with the B. suis S2 vaccine strain or other Brucella strains, a more sensitive detection method is needed. Studies by Cheville NF and others have shown that in goats inoculated with B. Melitensis 16M in the first 4 days of infection, the concentration of Brucella in milk was 104–107 CFU/mL. Furthermore, a bacterial culture of the superficial cervical lymph nodes produced a large number of bacteria in post-inoculation day 3 (PID3). Finally, at PID 7, the concentration of bacterial culture remained at a high level, but decreased significantly at 14-28 days. Bacteria can be isolated from blood in PID 1-2. Bacteriemia was detected in PID 3, but not in PID 116 (Cheville et al., 1996). In a study by Araya LN et al., BALB/c mice were infected intravenously with B. abortus strain 19. Subsequently, bacteria proliferated in large numbers in the spleen, the concentration reached a peak 2 weeks after infection (pi), and then the body gradually cleared the infection. After 8 weeks, the number of bacteria decreased by >10,000-fold (Araya et al., 1989). S I Muhammed et al. found that 20 ewes were inoculated with Brucella ovis on the vaginal mucosa from 5 to 40 days of pregnancy. Subsequent studies found that bacteremia was temporary or intermittent. After 98 days of infection, Brucella ovis could not be cultured from the blood or other tissues (Muhammed et al., 1975). It is not clear how long the B.susi S2 vaccine strain can exist in the blood after vaccination because it has not been studied. However, according to our research results, nucleic acids of the B. suis S2 vaccine strain can still be detected one year after vaccination.
In conclusion, we have found some differential genes that can distinguish B. suis S2 from B. melitensis and B. abortus through comparative genomics. Based on one of the genes (GL_0002189 gene), LAMP detection method, based on a marker gene (GL_0002189 gene), was successfully developed to quickly distinguish between the vaccine and the causative pathogen strains. In addition, we report the first LAMP trial using comparative genomics designed to distinguish between animals infected with the naturally occurring Brucella strains and those that were vaccine immunized. The analysis method is simple and fast, can detect low content DNA in samples, and has high target specificity. It provides an effective detection method for the prevention and control of brucellosis.
The datasets presented in this study can be found in online repositories. The name of the repository and accession number can be found below: NCBI; SRP394076.
The animal study was reviewed and approved by the Animal Ethics and Welfare Committee of Inner Mongolia Agricultural University. Written informed consent was obtained from the owners for the participation of their animals in this study.
WW contributed to study design, laboratory supervision, and manuscript editing. CL contributed to study design and manuscript editing. JM contributed to study design, doing experiments, data analysis and manuscript drafting, editing, and writing. QL have contributed equally to this work and share first authorship. XY, XM, YS, and YQ contributed to laboratory quality control and data collection and helped perform the analysis with constructive discussions. All authors contributed to the article and approved the submitted version.
This study was supported by Scientific Research Project of Colleges and Universities in Inner Mongolia Autonomous Region (NJZZ19041) and Science and Technology Planning Project of Inner Mongolia Autonomous Region (20140174, 20120244).
The authors declare that the research was conducted in the absence of any commercial or financial relationships that could be construed as a potential conflict of interest.
All claims expressed in this article are solely those of the authors and do not necessarily represent those of their affiliated organizations, or those of the publisher, the editors and the reviewers. Any product that may be evaluated in this article, or claim that may be made by its manufacturer, is not guaranteed or endorsed by the publisher.
Araya, L. N., Elzer, P. H., Rowe, G. E., Enright, F. M., Winter, A. J. (1989). Temporal development of protective cell-mediated and humoral immunity in BALB/c mice infected with brucella abortus. J. Immunol. 143 (10), 3330–3337.
Cao, X., Li, S., Li, Z., Liu, Z., Ma, J., Lou, Z., et al. (2018). Enzootic situation and molecular epidemiology of brucella in livestock from 2011 to 2015 in qingyang, China. Emerg. Microbes Infect. 7 (1), 58. doi: 10.1038/s41426-018-0060-y
Cheville, N. F., Olsen, S. C., Jensen, A. E., Stevens, M. G., Florance, A. M., Houng, H. S., et al. (1996). Bacterial persistence and immunity in goats vaccinated with a purE deletion mutant or the parental 16M strain of brucella melitensis. Infect. Immun. 64 (7), 2431–2439. doi: 10.1128/iai.64.7.2431-2439.1996
Dadar, M., Alamian, S. (2021). Identification of main brucella species implicated in ovine and caprine abortion cases by molecular and classical methods. Arch. Razi Inst. 76 (1), 51–60. doi: 10.22092/ari.2019.128003.1398
Ducrotoy, M. J, Muñoz, P. M., Conde-Álvarez, R., Blasco, J. M., Moriyón, I. (2018). A systematic review of current immunological tests for the diagnosis of cattle brucellosis. Prev. Vet. Med. 151, 57–72. doi: 10.1016/j
Elfaki, M. G., Alaidan, A. A., Al-Hokail, A. A. (2015). Host response to brucella infection: review and future perspective. J. Infect. Dev. Ctries. 9 (7), 697–701. doi: 10.3855/jidc.6625
El-Sayed, A., Awad, W. (2018). Brucellosis: Evolution and expected comeback. Int. J. Vet. Sci. Med. 6 (Suppl), S31–S35. doi: 10.1016/j.ijvsm.2018.01.008
Goodwin, ZI, Pascual, D. W. (2016). Brucellosis vaccines for livestock. Vet. Immunol. Immunopathol. 181, 51–58. doi: 10.1016/j
Kamga, R. M. N., Silatsa, B. A., Farikou, O., Kuiate, J. R., Simo, G. (2020). Detection of brucella antibodies in domestic animals of southern Cameroon: Implications for the control of brucellosis. Vet. Med. Sci. 6 (3), 410–420. doi: 10.1002/vms3.264
López-Santiago, R., Sánchez-Argáez, A. B., De Alba-Núñez, L. G., Baltierra-Uribe, S. L., Moreno-Lafont, M. C. (2019). Immune response to mucosal brucella infection. Front. Immunol. 10. doi: 10.3389/fimmu.2019.01759
Masjedian Jezi, F., Razavi, S., Mirnejad, R., Zamani, K. (2019). Immunogenic and protective antigens of brucella as vaccine candidates. Comp. Immunol. Microbiol. Infect. Dis. 65, 29–36. doi: 10.1016/j.cimid.2019.03.015
Muhammed, S. I., Lauerman, L. H., Jr., Mesfin, G. M., Otim, C. P. (1975). Duration of brucella ovis infection in ewes. Cornell Vet. 65 (2), 221–227.
Notomi, T., Mori, Y., Tomita, N., Kanda, H. (2015). Loop-mediated isothermal amplification (LAMP): principle, features, and future prospects. J. Microbiol. 53 (1), 1–5. doi: 10.1007/s12275-015-4656-9
Saber Marouf, A., Hanifian, S., Shayegh, J. (2021). Prevalence of brucella spp. in raw milk and artisanal cheese tested via real-time qPCR and culture assay. Int. J. Food Microbiol. 347, 109192. doi: 10.1016/j.ijfoodmicro.2021.109192
Sathish Kumar, T., Radhika, K., Joseph Sahaya Rajan, J., Makesh, M., Alavandi, S. V., Vijayan, K. K. (2021). Closed-tube field-deployable loop-mediated isothermal amplification (LAMP) assay based on spore wall protein (SWP) for the visual detection of enterocytozoon hepatopenaei (EHP). J. Invertebr Pathol. 183, 107624. doi: 10.1016/j.jip.2021.107624
Trangoni, M. D, Gioffré, A. K., Cerón Cucchi, M. E., Caimi, K. C., Ruybal, P., Zumárraga, M. J., et al. (2015). LAMP technology: Rapid identification of brucella and mycobacterium avium subsp. paratuberculosis. Braz. J. Microbiol. 46 (2), 619–626. doi: 10.1590/S1517-838246220131206
Wang, S., Wang, W., Sun, K., Bateer, H., Zhao, X. (2020). Comparative genomic analysis between newly sequenced brucella abortus vaccine strain A19 and another brucella abortus vaccine S19. Genomics. 112 (2), 1444–1453. doi: 10.1016/j.ygeno
Wang, J. Y., Wu, N., WH, L., JJ, R., Tang, P., YH, Q., et al. (2014). A repA-based ELISA for discriminating cattle vaccinated with brucella suis 2 from those naturally infected with brucella abortus and brucella melitensis. Mol. Cell Probes. 28 (5-6), 251–254. doi: 10.1016/j.mcp.2014.06.002
Keywords: Brucellosis, Brucella spp., LAMP assay, Brucella S2 vaccine, differential diagnosis
Citation: Mu J, Li Q, Yan X, Mao X, Shi Y, Qin Y, Liu C and Wang W (2022) Detection of Brucella S2 vaccine strain by a loop-mediated isothermal amplification (LAMP) method. Front. Cell. Infect. Microbiol. 12:1023243. doi: 10.3389/fcimb.2022.1023243
Received: 02 September 2022; Accepted: 10 November 2022;
Published: 28 November 2022.
Edited by:
Michael Marceau, Université Lille Nord de France, FranceReviewed by:
Maryam Dadar, Razi Vaccine and Serum Research Institute, IranCopyright © 2022 Mu, Li, Yan, Mao, Shi, Qin, Liu and Wang. This is an open-access article distributed under the terms of the Creative Commons Attribution License (CC BY). The use, distribution or reproduction in other forums is permitted, provided the original author(s) and the copyright owner(s) are credited and that the original publication in this journal is cited, in accordance with accepted academic practice. No use, distribution or reproduction is permitted which does not comply with these terms.
*Correspondence: Wenlong Wang, d3dsLmltYXVAMTYzLmNvbQ==; Chunxia Liu, bGN4LmltYXVAaW1hdS5lZHUuY24=
†These authors have contributed equally to this work and share first authorship
Disclaimer: All claims expressed in this article are solely those of the authors and do not necessarily represent those of their affiliated organizations, or those of the publisher, the editors and the reviewers. Any product that may be evaluated in this article or claim that may be made by its manufacturer is not guaranteed or endorsed by the publisher.
Research integrity at Frontiers
Learn more about the work of our research integrity team to safeguard the quality of each article we publish.