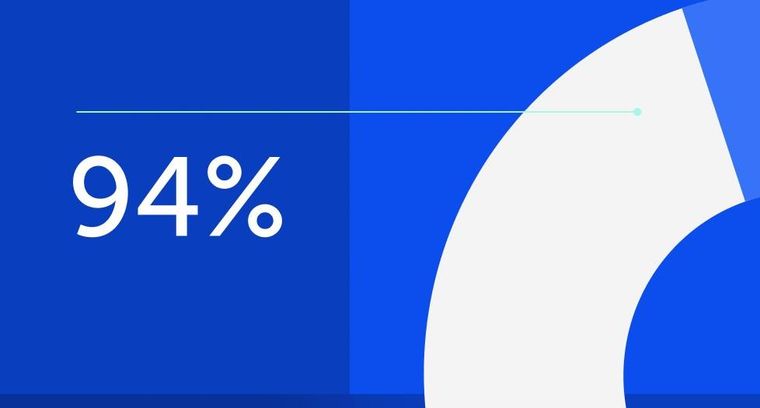
94% of researchers rate our articles as excellent or good
Learn more about the work of our research integrity team to safeguard the quality of each article we publish.
Find out more
ORIGINAL RESEARCH article
Front. Cell. Infect. Microbiol., 24 January 2022
Sec. Bacteria and Host
Volume 11 - 2021 | https://doi.org/10.3389/fcimb.2021.789672
This article is part of the Research TopicResistance Mechanisms of AcinetobacterView all 4 articles
Objectives: The spread of antibiotic resistant bacteria is an important threat for human health. Acinetobacter baumannii bacteria impose such a major issue, as multidrug- to pandrug-resistant strains have been isolated, rendering some infections untreatable. In this context, carbapenem-resistant A. baumannii bacteria were ranked as top priority by both WHO and CDC. In addition, A. baumannii bacteria survive in harsh environments, being capable of resisting to disinfectants and to persist prolonged periods of desiccation. Due to the high degree of variability found in A. baumannii isolates, the search for new antibacterials is very challenging because of the requirement of drug target conservation amongst the different strains. Here, we screened a chemical library to identify compounds active against several reference strains and carbapenem-resistant A. baumannii bacteria.
Methods: A repurposing drug screen was undertaken to identify A. baumannii growth inhibitors. One hit was further characterized by determining the IC50 and testing the activity on 43 modern clinical A. baumannii isolates, amongst which 40 are carbapenem-resistant.
Results: The repurposing screen led to the identification of a harmine-derived compound, called HDC1, which proves to have bactericidal activity on the multidrug-resistant AB5075-VUB reference strain with an IC50 of 48.23 µM. In addition, HDC1 impairs growth of 43 clinical A. baumannii isolates.
Conclusions: We identified a compound with inhibitory activity on all tested strains, including carbapenem-resistant clinical A. baumannii isolates.
The rise of antibiotic resistant bacteria is a global threat for healthcare, making it possible to succumb to diseases that were previously treatable (Wong et al., 2017). This has been acknowledged by both the World Health Organization (WHO) and the Centers for Disease Control and Prevention (CDC), which generated a list of drug-resistant pathogens for which new antibiotics are urgently needed (World Health Organization, 2017; CDC, 2019). The top priorities of these lists are antibiotic-resistant Acinetobacter baumannii bacteria (Whiteway et al., 2021).
A. baumannii is a Gram-negative, opportunistic bacterium, belonging to the ESKAPE group (Enterococcus faecium, Staphylococcus aureus, Klebsiella pneumoniae, Acinetobacter baumannii, Pseudomonas aeruginosa and Enterobacter spp.) of the most problematic nosocomial pathogens (Rice, 2008; Santajit and Indrawattana, 2016; Whiteway et al., 2021). While A. baumannii is a ubiquist (i.e. it can be found in soil, on human skin and in water sources), its presence especially imposes a threat in clinical settings (Vallenet et al., 2008; Wieland et al., 2018). This is due to a remarkable combination of resistance capabilities of A. baumannii, which is able to persist prolonged periods of desiccation, resist to disinfectants and to acquire drug resistance at a high rate (Da Silva and Domingues, 2016; Zeidler and Müller, 2019). Infections caused by A. baumannii commonly occur in immunocompromised patients and manifest as ventilator-assisted pneumonia, bacteremia and to a lesser extent skin or urinary tract infections (Weiner et al., 2016; Whiteway et al., 2021). Treatment of these infections becomes increasingly difficult, as multidrug-resistant, extensively drug-resistant or even pandrug-resistant strains have been reported, with the latter being resistant to all available antibiotics, including carbapenems (Magiorakos et al., 2012; Gallagher et al., 2015). An important hurdle in the development of new antimicrobials against A. baumannii is the high diversity found between isolates, leading to a still open pan-genome (Adams et al., 2008).
In this context, the discovery of new inhibitory molecules, active against multidrug-resistant A. baumannii strains, is therefore crucial (World Health Organization, 2017; CDC, 2019). Harmine and harmine derivatives are β-carbolines that present pharmacological effects including anti-inflammatory, neuroprotective, antidiabetic, and antitumor activities (Zhang et al., 2020). β-carbolines also show anti-infective potential (Faheem et al., 2021). For natural and synthetic β-carboline analogs, antibacterial, antifungal, antiviral, antimalarial, antileishmanial and antitrypanosomal properties have been reported. Most studies deal with biological evaluation of the molecules and, only in some rare cases, potential mechanisms of action have been proposed. Mechanistic studies demonstrated that harmine effectively repressed the HSV-2 induced upregulation of Interleukin-1β (IL-1β), Tumor Necrosis Factor-α (TNF-α), Interleukin-6 (IL-6), and Interleukin-8 (IL-8) (Chen et al., 2015). Mechanistically, substituted β-carbolines exhibit their anti-infective properties via several targets, including Topoisomerase II, DNA gyrase, MAPK, PfHsp90 or trypanothione reductase (Faheem et al., 2021).
For example, it has been shown that harmine exerts antileishmanial activity both in vitro and in vivo and causes necrosis by a nonspecific membrane damage in Leishmania donovani promastigotes (Lala et al., 2004). Harmine inhibits P. falciparum heat shock protein 90 by specific competition with its ATP-binding domain (Shahinas et al., 2012). Harmine analogs were further studied as binders to P. falciparum heat shock protein 90 (PfHsp90) and two compounds inhibited the parasite in vitro at micromolar concentrations, reducing parasitemia, and prolonging the survival of P. berghei-infected mice (Bayih et al., 2016). Adequate substitution of the β-carboline scaffold led to enhanced nematicidal effect (Xia et al., 2019). Manzamine alkaloids, which are complex natural compounds consisting of a β-carboline nucleus, have been isolated and found to exhibit potent anti-infective activities by inhibiting target kinases like GSK-3β and MtSK (Ashoka et al., 2021).
9-substituted harmine derivatives show an inhibitory effect on dengue virus and impair the maturation and release of virus particles to the extracellular medium affecting the spreading of the infection (Quintana et al., 2016). Their mode of action still needs to be clarified. 9N-methylharmine neither affects viral adsorption-internalization events nor viral RNA synthesis. The antiviral activity is not related to the ability of the compound to downregulate p38 MAPK phosphorylation.
Harmine derivatives with 7,9- or 2,7,9-substituted 7-oxy-1-methyl-β-carboline scaffolds inhibit activity of SerB2, an essential metabolic enzyme and suspected virulence factor of Mycobacterium tuberculosis (Frédérick et al., 2012; Carvalho et al., 2017; Carvalho et al., 2020; Pierson et al., 2020).
In this paper, we aimed at the discovery of a compound active against most clinical isolates. We performed a repurposing screen on a compound library, which led to the identification of a harmine-derived compound, called HDC1, with inhibitory activity on the growth of all the tested clinical isolates, amongst which 40 are carbapenem-resistant.
A compound library of the Namur Medicine & Drug Innovation Center (NAMEDIC) was provided for a growth inhibition screen against A. baumannii. All compounds were dissolved in 100% DMSO and used for the initial screen at 100 µM. The active compound HDC1 (1-methyl-2-benzyl-7-benzyloxy-9-benzyl-β-carbolin-2-ium bromide) was synthesized as previously described (Frédérick et al., 2012) with the following optimizations: 1-methyl-7-hydroxy-β-carboline was synthesized by adding 1-methyl-7-methoxy-β-carboline (0.600 g, 2.83 mmol, 1 equiv.), hydrobromic acid (12 ml, 48% in H2O) and acetic acid (12 ml) into a round-bottom flask, equipped with reflux condenser. The mixture was refluxed overnight under argon atmosphere and subsequently added to distilled water (100 ml). The precipitate was isolated via filtration, washed with cold water and dried under vacuum yielding 1-methyl-7-hydroxy-β-carboline with 81% (0.454 g) yield. 1H-NMR (500 MHz, DMSO-d6) δ (ppm): 12.59 (s, 1H), 10.62 (s, 1H), 8.39-8.29 (m, 2H), 8.21 (d, J = 8.7 Hz, 1H), 7.03 (d, J = 1.7 Hz, 1H), 6.89 (dd, J = 8.7, 1.7 Hz, 1H), 2.94 (s, 3H). Next, 1-methyl-2-benzyl-7-benzyloxy-9-benzyl-β-carbolin-2-ium bromide was synthesized. First 1-methyl-7-hydroxy-β-carboline (0.500 g, 2.52 mmol, 1 equiv.) was dissolved in anhydrous N,N,-dimethylformamide (20 ml) into a flame-dried microwave vial under argon atmosphere. Then KOtBu (0.849 g, 7.57 mmol, 3 equiv.) was added and the mixture was stirred for 30 minutes at room temperature. Subsequently benzyl bromide (3.00 ml, 25.2 mmol, 10 equiv.) was added and the mixture was heated overnight at 75°C. Afterwards the crude mixture was filtered, and the precipitate was washed with CH2Cl2. The volatiles in the filtrate were removed under reduced pressure and the crude product was subjected to column chromatography (cyclohexane/ethyl acetate) yielding the desired product with 46% (0.638 g) yield. 1H-NMR (500 MHz, DMSO-d6) δ (ppm): 8.86 (d, J = 6.6 Hz, 1H), 8.72 (d, J = 6.6 Hz, 1H), 8.49 (d, J = 8.8 Hz, 1H), 7.57 (d, J = 2.1 Hz, 1H), 7.50-7.46 (m, 2H), 7.40-7.25 (m, 9H), 7.23 (dd, J = 8.8, 2.1 Hz, 1H), 7.12 (d, J = 7.2 Hz, 2H), 6.99 (d, J = 7.2 Hz, 2H), 6.02 (s, 2H), 5.99 (s, 2H), 5.27 (s, 2H), 2.85 (s, 3H). 13C-NMR (126 MHz, DMSO-d6) δ (ppm): 162.8 (Cq), 148.0 (Cq), 139.6 (Cq), 137.5 (Cq), 136.2 (Cq), 135.4 (Cq), 134.7 (Cq), 133.5 (Cq), 129.1 (CH), 129.0 (CH), 128.5 (CH), 128.3 (CH), 127.5 (CH), 126.6 (CH), 125.4 (CH), 124.9 (CH), 114.7 (CH), 113.7 (CH), 112.8 (Cq), 95.0 (CH), 70.1 (CH2), 59.8 (CH2), 48.3 (CH2), 16.0 (CH3). The spectroscopic data were in accordance with those reported by Frédérick et al. (2012).
Taking into account the high genomic dynamics observed for A. baumannii bacteria, we used the proposed modern nomenclature in the field (Gallagher et al., 2015) by renaming the sub-cultured isolates by adding “-VUB” to the strain name, although these strains are a priori identical or very similar to the ones provided by the National Reference Center (NRC) Laboratory for Antibiotic-Resistant Gram-Negative Bacilli (CHU UCL Namur, Yvoir, Belgium). All A. baumannii reference strains and clinical isolates were cultured at 37°C in LB broth media under agitation (355 cpm) conditions for 16h before subsequent experiments. For IC50 determination, bacterial cells corresponding to OD600nm=0.1 were transferred to a 96 well flat bottom plate (Greiner, Austria) containing varying concentrations of HDC1 (0.1; 1; 10; 25; 50; 75; 100 and 1000 µM) in LB broth media with 1% DMSO. For determination of the activity of HDC1 on the 43 clinical A. baumannii isolates, bacterial cells corresponding to OD600nm=0.1 were transferred to a 96 well flat bottom plate (Greiner, Austria) containing 100 µM of HDC1 in LB broth media with 1% DMSO. For both experiments, the positive control included bacterial cells corresponding to OD600nm=0.1 of the used strains in LB broth media with 1% DMSO. The negative control included both LB broth media and LB broth media supplemented with 1% DMSO. Collection of data was done using the Cytation 1 (BioTek, United States). The OD600nm absorbance of all bacterial cultures was measured every 30 min for 24h at a temperature of 37°C and agitation of 355 cpm (cycles per minute). In all analysis, growth inhibition is defined as decreasing absorbance values over time compared to the positive control. The classification of strains into inhibition levels is based on the ratio of the final absorbance value of the HDC1-treated bacteria (OD600nm; HDC1) compared to the final absorbance value of the non-treated control (OD600nm; control) as followed: (i) low inhibition (OD600nm; HDC1 > 50% of OD600nm; control); (ii) intermediate inhibition (10% of OD600nm; control < OD600nm; HDC1 < or = 50% of OD600nm; control) and (iii) complete inhibition (OD600nm; HDC1 < or = 10% OD600nm; control). The data were measured in biological triplicate.
After absorbance measurements for IC50 determination, the positive control (AB5075-VUB grown in LB with 1% DMSO) and AB5075-VUB grown in presence of 100 µM HDC1, were resuspended in PBS, brought to the same OD600nm and plated on LB agar plates in appropriate dilutions. After 16 h incubation of the LB agar plates at 37°C, CFUs were counted to assess any bacteriostatic or bactericidal effect. All data was measured in biological triplicate. To estimate the initial bacterial load, the relationship between the absorbance at OD600nm and CFUs was determined for the strain AB5075-VUB. This was done by plating serial dilutions of bacterial cultures with a known OD600nm on LB agar and counting the corresponding CFUs (see Supplementary Table 1).
For the determination of the IC50, GraphPad Prism 9 (GraphPad Software, LLC) was used. After 20 hours, the OD600nm absorbance kinetic data were obtained and normalized using the positive control absorbance value as 100% viability and the absorbance value of 1 mM HDC1 as 0% viability. The analysis was then done on the normalized data by nonlinear regression curve fitting. The IC50 value is shown with a 95% confidence interval (CI).
All data shown are represented as mean ± standard deviation of three biological replicates, except otherwise stated. CFU were statistically analyzed by an unpaired t-test. All growth curves were statistically analyzed by a Mann Whitney test. The p values < 0.05 were considered significant.
The initial screen from a chemical library of the Namur Medicine & Drug Innovation Center from the University of Namur (UNamur) aimed at the identification of growth inhibitors for problematic multidrug-resistant A. baumannii strains. The strain initially used for this screen is a multidrug-resistant A. baumannii reference strain, AB5075-VUB. This strain is a derivative from the parental strain AB5075 that was clonally isolated in our laboratory at the VUB (Vrije Universiteit Brussel).
The screen showed complete growth inhibition by one compound called HDC1, for it is a harmine-derived compound (Figure 1). HDC1 was originally synthesized for an anticancer drug screen. Interestingly, compounds with anticancer activity have lately been explored as potential antimicrobials (Cheng et al., 2019). In line with this tendency, HDC1 was further characterized for other activities.
Figure 1 (A) Structure of HDC1. (B) Growth curve of AB5075-VUB in presence (grey triangles) and absence (black spheres) of 100 µM of HDC1. (C) Non-linear regression curve of normalized absorbance reads in function of compound concentration. (D) Number of viable bacteria after 24 h incubation without and with 100 µM of HDC1. The initial bacterial load is represented by the dashed line on the plot. All data points in this figure are shown as mean ± standard deviation of three independent biological replicates. ***p < 0.001.
To determine the potency of the compound, the minimum concentration required for 50% growth inhibition, IC50, was determined for the AB5075-VUB strain. The analysis showed that HDC1 has an IC50 of 48.23 µM (95% CI 44.76-51.83) (Figure 1C). To determine the potential antimicrobial effect of HDC1 on AB5075-VUB viability, the strain was grown in presence of 100 µM of HDC1. After 24 h incubation, the bacteria were resuspended in fresh media without the compound and plated on LB agar plates for CFUs enumeration. After 24h incubation, the number of recovered bacteria is significantly different when bacteria are incubated with the compound compared to the control group (Figure 1D). While a 40-fold increase in CFUs is observed for the control group, a 1000-fold decrease of CFUs is observed in the presence of HDC1, compared to the initial bacterial inoculum. This shows a significant bactericidal activity of HDC1 on the multidrug-resistant AB5075-VUB reference strain.
A. baumannii has a highly dynamic genome (Wright et al., 2016). The presence of mobile genetic elements and the efficient acquisition of genes through horizontal gene transfer are not only responsible for the pathogen’s success in obtaining drug resistance and environmental persistence, but they are also the reason isolates have become more and more diverse (Adams et al., 2008; Imperi et al., 2011; Wright et al., 2016). The core genome of the pathogen’s strains is reported to be relatively small and the accessory genome of strains can be up to 25-46% unique (Adams et al., 2008; Imperi et al., 2011). This heterogeneity found in isolates renders the search for antimicrobial compounds increasingly difficult. It is therefore important for a new potential antimicrobial to exert its activity not only on a few A. baumannii strains, but on a multitude of diverse and clinically relevant isolates.
To determine the activity of HDC1 on recent isolates, we used 43 recent A. baumannii clinical isolates, amongst which 40 are carbapenem-resistant strains (Valcek et al., 2021). In addition to these recent clinical strains, clonal isolates of three frequently used reference strains were also included. Two of these strains, ATCC19606 and ATCC17978, are older type strains, compared to the more recent and multidrug-resistant AB5075 reference strain (Gallagher et al., 2015; Harding et al., 2018). The third reference strain, DSM30011-VUB, is an environmental isolate (Repizo et al., 2017).
The growth of all tested strains was impaired by the presence of HDC1 (Figure 2), with low, intermediate, or complete inhibition levels. An overview of the different resistance profiles against HDC1 can be found in Table 1. Complete inhibition of growth is observed for all three reference strains and 13 recent isolates, while most of the clinical isolates show an intermediate inhibition profile. The AB193-VUB isolate showed the least sensitivity to HDC1. No correlation could be established between the sensitivity of the tested strains to the HDC1 compound, and the presence of the antibiotic-resistance genes found in the modern clinical isolates tested.
Figure 2 (A) Growth curves of 43 clinical A. baumannii isolates. (B) Growth curves of three A. baumannii reference strains (ATCC19606-VUB, ATCC17978-VUB and DSM30011-VUB). All strains were incubated with 100 µM of HDC1 (grey triangles) or without HDC1 (black spheres) for 24 h. All data points are shown as mean ± standard deviation of three independent biological replicates. X axis and y axis correspond to respectively time (h) and absorbance values measured at 600 nm.
Table 1 Growth inhibition levels on three reference strains and 43 clinical isolates of A. baumannii.
In this study, a repurposing drug screen led to the discovery of a compound with inhibitory activity on the growth of a multidrug-resistant Acinetobacter baumannii reference strain, AB5075-VUB. This compound, named HDC1, is a harmine-derivative previously designed to have anticancer properties. The anticancer screen showed that the compound acts as a protein synthesis inhibitor at a concentration of 0.7 µM (Frédérick et al., 2012). In our study, HDC1 was shown to have bactericidal activity on AB5075-VUB with an IC50 of 48.23 µM. As the compound was previously found to be cytotoxic in HEPG2 cells at 50 µM, this limits the potential of HDC1 as a new antimicrobial without further modification of the molecule (Marx et al., 2019). Differences in active concentrations of HDC1 between cancer and bacterial cells could be attributed to cell size and target(s) presence and/or abundance, altered metabolism states and the presence or absence of post-translational modifications. However, as multidrug-, extensively drug- and pandrug-resistant A. baumannii strains are emerging and spreading, every option deems to be explored.
Due to the high diversity between A. baumannii isolates, one of the main hurdles in the discovery of new compounds is to find compounds capable of targeting the majority of the isolates. Here, we report a compound to have inhibitory activity on all the tested and recent carbapenem-resistant A. baumannii isolates. Our test shows various degrees of growth inhibition: from complete to intermediate to only slight inhibition. In addition, the 4 reference strains used in our study all show a high degree of sensitivity to HDC1. Taken together, this raises the following questions (i) what contributes to this difference in growth inhibition levels, (ii) what could be the target(s) of HDC1 and (iii) why is the whole AB5075-VUB bacterial population not killed by HDC1 in the tested conditions, since a significant bactericidal effect is observed? The phase variation observed in AB5075 might be the answer to the last question. Phase variation is a morphological feature of A. baumannii colonies where 2 different opacity phenotypes are observed: opaque and translucent (Tipton et al., 2015). Opaque and translucent colonies exhibit multiple phenotypic differences, including cell morphology, surface motility, biofilm formation and virulence, but of particular interest also a difference in resistance to antibiotics and thus potentially to HDC1 (Tipton et al., 2015; Chin et al., 2018). Interestingly, a recent study showed that HDC1 inhibits the phosphoserine phosphatase of Mycobacterium tuberculosis, MtSerB2 (Pierson et al., 2020). MtSerB2 catalyzes the last step in the L-serine biosynthetic pathway and is involved in immune evasion mechanisms of M. tuberculosis (Pierson et al., 2020). In AB5075-VUB, a homolog of MtSerB2 is present: AbSerB. This indicates a putative target of HDC1 in A. baumannii. A possible explanation for the different growth inhibition profiles of the clinical isolates could be the presence of mutations in the serB gene. Although serB is highly conserved in all our tested A. baumannii strains (sequence identity between 98.45-100%), no correlation could be found between mutations in serB and the growth inhibition profiles of the A. baumannii isolates (Supplementary Table 2). The target(s) of HDC1 in A. baumannii remain(s) to be determined. Interestingly, it has been shown for M. tuberculosis that certain compounds are more efficient at inhibiting growth of the bacterium itself, than inhibiting the enzyme only, suggesting different mechanisms of action or intracellular accumulation of the compounds (Haufroid and Wouters, 2019). Additional resistance mechanisms, potentially countering such effects, could explain the higher resistance to HDC1 of some clinical isolates. Nevertheless, HDC1 has broad activity on all the tested recent clinical A. baumannii isolates of our study, which prompts the further exploration of this compound and/or cognate putative target in A. baumannii for drug discovery.
In conclusion, HDC1 is a potent harmine-derived compound with antibacterial activity identified using a multidrug-resistant A. baumannii strain, that also significantly inhibits the growth of diverse, recent, and carbapenem-resistant clinical A. baumannii isolates.
The original contributions presented in the study are included in the article/Supplementary Material. Further inquiries can be directed to the corresponding author.
Drafting of the manuscript, AB and CV. Corrections of the manuscript, AB, MV, ME, CP, JW, SB, and CV. HDC1 production, ME and SB. Preliminary drug screen, TQ, CP, and CV. Bioinformatics, AB and CH. Experiments, AB, CW, and CV. Data analyses, AB, MV, JW, and CV. All authors contributed to the article and approved the submitted version.
AB is recipient of a PhD fellowship Strategic Basic Research of the Research Foundation – Flanders (FWO, File number: 77258). ME and SB acknowledge financial support of the Research Council at the Vrije Universiteit Brussel (VUB) through the IRP funding scheme. CV acknowledges the financial support from the Flanders Institute for Biotechnology (VIB). This project has received funding from the European Union’s Horizon 2020 research and innovation program under the Marie Sklodowska-Curie grant agreement No 748032.
The authors declare that the research was conducted in the absence of any commercial or financial relationships that could be construed as a potential conflict of interest.
All claims expressed in this article are solely those of the authors and do not necessarily represent those of their affiliated organizations, or those of the publisher, the editors and the reviewers. Any product that may be evaluated in this article, or claim that may be made by its manufacturer, is not guaranteed or endorsed by the publisher.
We are grateful to Pr. Tom Coenye for providing us the strain AB5075 and Dr. Suzana Salcedo for the strains ATCC19606, ATCC17978 and DSM30011, as well as for fruitful discussions. We would like to thank Pr. Pierre Bogaerts, Pr. Te-din Huang and Pr. Olivier Denis, from the National Reference Center (NRC) Laboratory for Antibiotic-Resistant Gram-Negative Bacilli (CHU UCL Namur, Yvoir, Belgium) for providing the recent clinical isolates of A. baumannii. We also thank the members of Namedic (NARILIS-UNamur), in particular Pr. B. Masereel and L. Pochet for their research that allowed setting up the chemical library used in the present screening.
The Supplementary Material for this article can be found online at: https://www.frontiersin.org/articles/10.3389/fcimb.2021.789672/full#supplementary-material
Supplementary Table 1 | Relationship between the absorbance (OD600nm) and the colony forming units (CFUs) per ml for the strain AB5075-VUB. The values are shown for overnight cultures that were diluted for accurate absorbance measurements. Rep = Biological replicate. At an OD600nm=1, the CFU/ml corresponds to 3.2 ± 0.3 108 CFU/ml.
Supplementary Table 2 | Percentage of nucleotide identity of the serB gene in 43 clinical isolates and four reference strains compared to the reference AB5075-UW (NZ_CP008706.1) with AA substitution where applicable.
Adams, M. D., Goglin, K., Molyneaux, N., Hujer, K. M., Lavender, H., Jamison, J. J., et al. (2008). Comparative Genome Sequence Analysis of Multidrug-Resistant Acinetobacter Baumannii. J. Bacteriol. 190, 8053–8064. doi: 10.1128/JB.00834-08
Ashoka, P., Faheem, Kumar, B., Chander, S., Chandra Sekhar, K., Sankaranarayanan, M. (2021). Anti-Infective Potential of Manzamine Alkaloids - A Review. Med. Chem. doi: 10.2174/1573406417666210803101740
Bayih, A. G., Folefoc, A., Mohon, A. N., Eagon, S., Anderson, M., Pillai, D. R. (2016). In Vitro and In Vivo Anti-Malarial Activity of Novel Harmine-Analog Heat Shock Protein 90 Inhibitors: A Possible Partner for Artemisinin. Malar. J. 15, 1–11. doi: 10.1186/s12936-016-1625-7
Carvalho, A., Chu, J., Meinguet, C., Kiss, R., Vandenbussche, G., Masereel, B., et al. (2017). A Harmine-Derived Beta-Carboline Displays Anti-Cancer Effects In Vitro by Targeting Protein Synthesis. Eur. J. Pharmacol. 805, 25–35. doi: 10.1016/j.ejphar.2017.03.034
Carvalho, A., Viaene, J., Vandenbussche, G., De Braekeleer, K., Masereel, B., Wouters, J., et al. (2020). A New Potential Anti-Cancer Beta-Carboline Derivative Decreases the Expression Levels of Key Proteins Involved in Glioma Aggressiveness: A Proteomic Investigation. Drug Dev. Res. 81, 32–42. doi: 10.1002/ddr.21600
CDC. (2019). Antibiotic Resistance Threats in the United States. Atlanta, GA: U.S. Department of Health and Human Services, CDC. doi: 10.15620/cdc:82532
Cheng, Y. S., Sun, W., Xu, M., Shen, M., Khraiwesh, M., Sciotti, R. J., et al. (2019). Repurposing Screen Identifies Unconventional Drugs With Activity Against Multidrug Resistant Acinetobacter Baumannii. Front. Cell Infect. Microbiol. 9:438. doi: 10.3389/fcimb.2018.00438
Chen, D., Su, A., Fu, Y., Wang, X., Lv, X., Xu, W., et al. (2015). Harmine Blocks Herpes Simplex Virus Infection Through Downregulating Cellular NF-κb and MAPK Pathways Induced by Oxidative Stress. Antiviral Res. 123, 27–38. doi: 10.1016/j.antiviral.2015.09.003
Chin, C. Y., Tipton, K. A., Farokhyfar, M., Burd, E. M., Weiss, D. S., Rather, P. N. (2018). A High-Frequency Phenotypic Switch Links Bacterial Virulence and Environmental Survival in Acinetobacter Baumannii. Nat. Microbiol. 3, 563–569. doi: 10.1038/s41564-018-0151-5
Da Silva, G., Domingues, S. (2016). Insights on the Horizontal Gene Transfer of Carbapenemase Determinants in the Opportunistic Pathogen Acinetobacter baumannii. Microorganisms 4:29. doi: 10.3390/microorganisms4030029
Faheem, Kumar, B. K., Sekhar, K. V. G. C., Kunjiappan, S., Jamalis, J., Balaña-Fouce, R., et al. (2021). Recent Update on the Anti-Infective Potential of B-Carboline Analogs. Mini Rev. Med. Chem. 21. doi: 10.2174/1389557520666201001130114
Frédérick, R., Bruyére, C., Vancraeynest, C., Reniers, J., Meinguet, C., Pochet, L., et al. (2012). Novel Trisubstituted Harmine Derivatives With Original In Vitro Anticancer Activity. J. Med. Chem. 55, 6489–6501. doi: 10.1021/jm300542e
Gallagher, L. A., Ramage, E., Weiss, E. J., Radey, M., Hayden, H. S., Held, K. G., et al. (2015). Resources for Genetic and Genomic Analysis of Emerging Pathogen Acinetobacter Baumannii. J. Bacteriol. 197, 2027–2035. doi: 10.1128/JB.00131-15
Harding, C. M., Hennon, S. W., Feldman, M. F. (2018). Uncovering the Mechanisms of Acinetobacter Baumannii Virulence. Nat. Rev. Microbiol. 176, 139–148. doi: 10.1038/nrmicro.2017.148
Haufroid, M., Wouters, J. (2019). Targeting the Serine Pathway: A Promising Approach Against Tuberculosis? Pharmaceuticals 12, 1–20. doi: 10.3390/ph12020066
Imperi, F., Antunes, L. C. S., Blom, J., Villa, L., Iacono, M., Visca, P., et al. (2011). The Genomics of Acinetobacter Baumannii: Insights Into Genome Plasticity, Antimicrobial Resistance and Pathogenicity. IUBMB Life 63, 1068–1074. doi: 10.1002/iub.531
Lala, S., Pramanick, S., Mukhopadhyay, S., Bandyopadhyay, S., Basu, M. K. (2004). Harmine: Evaluation of its Antileishmanial Properties in Various Vesicular Delivery Systems. J. Drug Target. 12, 165–175. doi: 10.1080/10611860410001712696
Magiorakos, A. P., Srinivasan, A., Carey, R. B., Carmeli, Y., Falagas, M. E., Giske, C. G., et al. (2012). Multidrug-Resistant, Extensively Drug-Resistant and Pandrug-Resistant Bacteria: An International Expert Proposal for Interim Standard Definitions for Acquired Resistance. Clin. Microbiol. Infect. 18, 268–281. doi: 10.1111/j.1469-0691.2011.03570.x
Marx, S., Van Gysel, M., Breuer, A., Dal Maso, T., Michiels, C., Wouters, J., et al. (2019). Potentialization of Anticancer Agents by Identification of New Chemosensitizers Active Under Hypoxia. Biochem. Pharmacol. 162, 224–236. doi: 10.1016/j.bcp.2019.01.004
Pierson, E., Haufroid, M., Gosain, T. P., Chopra, P., Singh, R., Wouters, J. (2020). Identification and Repurposing of Trisubstituted Harmine Derivatives as Novel Inhibitors of Mycobacterium Tuberculosis Phosphoserine Phosphatase. Molecules 25, 1–17. doi: 10.3390/molecules25020415
Quintana, V. M., Piccini, L. E., Panozzo Zénere, J. D., Damonte, E. B., Ponce, M. A., Castilla, V. (2016). Antiviral Activity of Natural and Synthetic β-Carbolines Against Dengue Virus. Antiviral Res. 134, 26–33. doi: 10.1016/j.antiviral.2016.08.018
Repizo, G. D., Viale, A. M., Borges, V., Cameranesi, M. M., Taib, N., Espariz, M., et al. (2017). The Environmental Acinetobacter Baumannii Isolate DSM30011 Reveals Clues Into the Preantibiotic Era Genome Diversity, Virulence Potential, and Niche Range of a Predominant Nosocomial Pathogen. Genome Biol. Evol. 9, 2292–2307. doi: 10.1093/gbe/evx162
Rice, L. B. (2008). Federal Funding for the Study of Antimicrobial Resistance in Nosocomial Pathogens: No ESKAPE. J. Infect. Dis. 197, 1079–1081. doi: 10.1086/533452
Santajit, S., Indrawattana, N. (2016). Mechanisms of Antimicrobial Resistance in ESKAPE Pathogens. BioMed. Res. Int. 2016. doi: 10.1155/2016/2475067
Shahinas, D., MacMullin, G., Benedict, C., Crandall, I., Pillaid, D. R. (2012). Harmine is a Potent Antimalarial Targeting Hsp90 and Synergizes With Chloroquine and Artemisinin. Antimicrob. Agents Chemother. 56, 4207–4213. doi: 10.1128/AAC.00328-12
Tipton, K. A., Dimitrova, D., Rather, P. N. (2015). Phase-Variable Control of Multiple Phenotypes in Acinetobacter Baumannii Strain AB5075. J. Bacteriol. 197, 2593–2599. doi: 10.1128/JB.00188-15
Valcek, A., Bogaerts, P., Denis, O., Huang, T.-D., van der Henst, C. (2021). Molecular Epidemiology of Carbapenem-Resistant Acinetobacter Baumannii Strains in Belgian Acute-Care Hospitals. medRrxiv. doi: 10.1101/2021.07.06.21259728
Vallenet, D., Nordmann, P., Barbe, V., Poirel, L., Mangenot, S., Bataille, E., et al. (2008). Cruveiller S.Comparative Analysis of Acinetobacters: Three Genomes for Three Lifestyles. PloS One 3, e1805. doi: 10.1371/journal.pone.0001805
Weiner, L. M., Webb, A. K., Limbago, B., Dudeck, M. A., Patel, J., Kallen, A. J., et al. (2016). Antimicrobial-Resistant Pathogens Associated With Healthcare-Associated Infections: Summary of Data Reported to the National Healthcare Safety Network at the Centers for Disease Control and Prevention, 2011–2014. Infect. Control Hosp. Epidemiol. 37, 1288–1301. doi: 10.1017/ice.2016.174
Whiteway, C., Breine, A., Philippe, C., van der Henst, C. (2021). Acinetobacter Baumannii. Trends Microbiol. doi: 10.1016/j.tim.2021.11.008
Wieland, K., Chhatwal, P., Vonberg, R. P. (2018). Nosocomial Outbreaks Caused by Acinetobacter Baumannii and Pseudomonas Aeruginosa: Results of a Systematic Review. Am. J. Infect. Control 46, 643–648. doi: 10.1016/j.ajic.2017.12.014
Wong, D., Nielsen, T. B., Bonomo, R. A., Pantapalangkoor, P., Luna, B., Spellberg, B. (2017). Clinical and Pathophysiological Overview of Acinetobacter Infections: A Century of Challenges. Clin. Microbiol. Rev. 30, 409–447. doi: 10.1128/CMR.00058-16
World Health Organization (2017). Global Priority List of Antibiotic-Resistant Bacteria to Guide Research, Discovery, and Development of New Antibiotics Genève, Switzerland: WHO.
Wright, M. S., Iovleva, A., Jacobs, M. R., Bonomo, R. A., Adams, M. D. (2016). Genome Dynamics of Multidrug-Resistant Acinetobacter Baumannii During Infection and Treatment. Genome Med. 8, 1–12. doi: 10.1186/s13073-016-0279-y
Xia, Y., Qi, Y. M., Yu, X. H., Wang, B. F., Cao, R. H., Jiang, D. X. (2019). Nematicidal Effect Against Bursaphelenchus Xylophilus of Harmine Quaternary Ammonium Derivatives, Inhibitory Activity and Molecular Docking Studies on Acetylcholinesterase. Eur. J. Plant Pathol. 153, 111–122. doi: 10.1007/s10658-018-1559-8
Zeidler, S., Müller, V. (2019). Coping With Low Water Activities and Osmotic Stress in Acinetobacter Baumannii: Significance, Current Status and Perspectives. Environ. Microbiol. 21, 2212–2230. doi: 10.1111/1462-2920.14565
Keywords: Acinetobacter baumannii, carbapenem-resistant, Gram-negative, pathogenic bacteria, repurposed compound, drug screening
Citation: Breine A, Van Gysel M, Elsocht M, Whiteway C, Philippe C, Quinet T, Valcek A, Wouters J, Ballet S and Van der Henst C (2022) Antimicrobial Activity of a Repurposed Harmine-Derived Compound on Carbapenem-Resistant Acinetobacter baumannii Clinical Isolates. Front. Cell. Infect. Microbiol. 11:789672. doi: 10.3389/fcimb.2021.789672
Received: 05 October 2021; Accepted: 15 December 2021;
Published: 24 January 2022.
Edited by:
Brock Aaron Arivett, Middle Tennessee State University, United StatesReviewed by:
Rafael Franco-Cendejas, National Institute of Rehabilitation Luis Guillermo Ibarra Ibarra, MexicoCopyright © 2022 Breine, Van Gysel, Elsocht, Whiteway, Philippe, Quinet, Valcek, Wouters, Ballet and Van der Henst. This is an open-access article distributed under the terms of the Creative Commons Attribution License (CC BY). The use, distribution or reproduction in other forums is permitted, provided the original author(s) and the copyright owner(s) are credited and that the original publication in this journal is cited, in accordance with accepted academic practice. No use, distribution or reproduction is permitted which does not comply with these terms.
*Correspondence: Charles Van der Henst, Y2hhcmxlcy52YW5kZXJoZW5zdEB2dWIudmliLmJl
Disclaimer: All claims expressed in this article are solely those of the authors and do not necessarily represent those of their affiliated organizations, or those of the publisher, the editors and the reviewers. Any product that may be evaluated in this article or claim that may be made by its manufacturer is not guaranteed or endorsed by the publisher.
Research integrity at Frontiers
Learn more about the work of our research integrity team to safeguard the quality of each article we publish.