- 1Universidad de Buenos Aires. Facultad de Medicina. Departamento de Microbiología, Parasitología e Inmunología, Buenos Aires, Argentina
- 2CONICET Universidad de Buenos Aires. Instituto de Investigaciones Biomédicas en Retrovirus y SIDA (INBIRS), Buenos Aires, Argentina
- 3Division of Cardiology, Hospital del Gobierno de la Ciudad de Buenos Aires "Dr. Cosme Argerich", Buenos Aires, Argentina
- 4Hospital Municipal de Rehabilitación Respiratoria María Ferrer, Buenos Aires, Argentina
- 5CONICET Universidad de Buenos Aires. Instituto de Investigaciones en Microbiología y Parasitología Médica (IMPaM), Buenos Aires, Argentina
Chronic Chagas disease cardiomyopathy (CCC) is the most important clinical manifestation of infection with Trypanosma cruzi (T. cruzi) due to its frequency and effects on morbidity and mortality. Peripheral blood mononuclear cells (PBMC) infiltrate the tissue and differentiate into inflammatory macrophages. Advances in pathophysiology show that myeloid cell subpopulations contribute to cardiac homeostasis, emerging as possible therapeutic targets. We previously demonstrated that fenofibrate, PPARα agonist, controls inflammation, prevents fibrosis and improves cardiac function in a murine infection model. In this work we investigated the spontaneous release of inflammatory cytokines and chemokines, changes in the frequencies of monocyte subsets, and fenofibrate effects on PBMC of seropositive patients with different clinical stages of Chagas disease. The results show that PBMC from Chagas disease patients display higher levels of IL-12, TGF-β, IL-6, MCP1, and CCR2 than cells from uninfected individuals (HI), irrespectively of the clinical stage, asymptomatic (Asy) or with Chagas heart disease (CHD). Fenofibrate reduces the levels of pro-inflammatory mediators and CCR2 in both Asy and CHD patients. We found that CHD patients display a significantly higher percentage of classical monocytes in comparison with Asy patients and HI. Besides, Asy patients have a significantly higher percentage of non-classical monocytes than CHD patients or HI. However, no difference in the intermediate monocyte subpopulation was found between groups. Moreover, monocytes from Asy or CHD patients exhibit different responses upon stimulation in vitro with T. cruzi lysates and fenofibrate treatment. Stimulation with T. cruzi significantly increases the percentage of classical monocytes in the Asy group whereas the percentage of intermediate monocytes decreases. Besides, there are no changes in their frequencies in CHD or HI. Notably, stimulation with T. cruzi did not modify the frequency of the non-classical monocytes subpopulation in any of the groups studied. Moreover, fenofibrate treatment of T. cruzi-stimulated cells, increased the frequency of the non-classical subpopulation in Asy patients. Interestingly, fenofibrate restores CCR2 levels but does not modify HLA-DR expression in any groups. In conclusion, our results emphasize a potential role for fenofibrate as a modulator of monocyte subpopulations towards an anti-inflammatory and healing profile in different stages of chronic Chagas disease.
Introduction
The acute phase of Trypanosoma cruzi (T. cruzi) infection is characterized by the presence of parasites in the host bloodstream that disseminate to the heart and other organs. This promotes a severe inflammatory response with recruitment of mononuclear cells, activation of resident macrophages, and release of pro-inflammatory mediators. This response is associated with parasite persistence in the heart and other tissues, due to the fact that the immune response is not efficient to wipe out the infection, leading to lifelong infection (Trachtenberg and Hare, 2017). Therefore, it goes forward to a chronic stage with a wide spectrum of manifestations, ranging from minor myocardial involvement to chronic Chagas disease cardiomyopathy (CCC) in which the tropism of the parasite for cardiac tissue constitutes one of the factors that lead to cardiac pathology (Tanowitz et al., 2015). Moreover, inflammatory processes also promote heart muscle fibrosis. Consequently, infected individuals may undergo heart chamber remodeling, congestive heart failure, and eventually death. Likewise, the persistence of activated macrophages in the tissues may create an inflammatory microenvironment that, in turn, contributes to developing tissue damage during the course of these pathological processes (Rőszer et al., 2013).
It has been described that, in response to infection with T. cruzi, cardiomyocytes and macrophages release nitric oxide (NO), cytokines and chemokines that are important to control parasite proliferation (Petray et al., 1994). However, the excess of these mediators generates harmful effects, contributing to the pathogenesis of chronic CCC (Machado et al., 2000; Gutierrez et al., 2009; Hovsepian et al., 2011; Penas et al., 2013).
Monocytes are heterogeneous, multifunctional cells that participate in cellular processes, namely, tissue repair and regeneration during heart diseases (Apostolakis et al., 2010). Advances in pathophysiology demonstrate that some subpopulations of myeloid cells contribute to cardiac homeostasis (Bajpai et al., 2018). Monocytes may differentiate into tissue-resident macrophages in specific microenvironmental conditions (Guilliams and Scott, 2017). Currently, the circulating human monocytic cells can be divided into subpopulations based on the surface expression of CD14 (a cell co-receptor for LPS) and CD16 (the low-affinity IgG receptor). They are further divided into three major subsets: a high percentage of monocytes, named classical monocytes, are CD14++ CD16− but, to a lesser extent we find two other subpopulations, such as CD14++ CD16+, intermediate monocytes, and CD14+ CD16++, which are non-classical monocytes (Wong et al., 2011). Human peripheral blood monocytes are also defined by the expression of the cell surface markers CD64 (Fcγ RI) and the chemokine receptor CD192 (also known as CCR2, a key mediator of monocyte migration) whose most prominent role is the mobilization of monocytes under physiologic and also inflammatory conditions. Besides, monocyte subpopulations can be characterized according to different levels of human leukocyte antigen D related (HLA-DR) (Shi, 2014).
CCR2 was first identified on monocytes, which constitutively express the receptor, and is dowregulated after differentiation into macrophages (Fantuzzi et al., 1999). Particularly, CCR2 plays important roles in tissue recruitment and transmigration of monocytes through the endothelial layer under inflammatory conditions. After myocardial injury, CCR2- macrophages promote the regeneration of cardiac tissue and functional recovery of the heart, through expansion of the coronary vasculature and physiological proliferation of cardiomyocytes (Lavine et al., 2014; Leid et al., 2016). The resident macrophage population was shown to expand in response to cardiac injury by participating in the immune surveillance of this tissue, which raises important questions about the fate and function of macrophages during the development of heart failure (Epelman et al., 2014; Heidt et al., 2014). On the other hand, the recruitment of monocytes, their differentiation into macrophages and their activation have a causal role in ventricular dysfunction (Hulsmans et al., 2018) and fibrosis (Sica et al., 2014; Satoh et al., 2017).
Like in many other situations, monocytes/macrophages as innate immune cells recognize T. cruzi pathogen-associated molecular patterns (PAMPs) and activate lymphocytes and the adaptive immune response during Chagas disease (Teixeira et al., 2011; Andrade and Gollob KJ, 2014). It has been shown that individuals infected with T. cruzi, with severe heart disease, display a profile of subsets of monocytes that suggests a more pronounced inflammatory environment compared with patients with heart failure unrelated to T. cruzi infection (Pérez-Mazliah et al., 2018). Besides, it was reported that the intermediate monocyte subpopulation is associated with CCC (Gómez-Olarte et al., 2019). On the other hand, it has been proposed that monocytes play a role as immunoregulators in asymptomatic Chagas disease patients by activating lymphocytes and, thus, the adaptive immunity through the expression of the co-stimulatory molecules CD80 and CD86. Indeed, the expression of the latter was associated with a higher frequency of Treg cells in asymptomatic individuals (Pinto et al., 2018).
Peroxisome proliferator-activated receptors (PPARs), members of the steroid hormone receptor superfamily, are ligand-dependent nuclear transcription factors. Fenofibrate, a PPAR-α ligand, is a third-generation fibric acid derivative currently used clinically as a hypolipidemic agent to lessen the risk of atherosclerosis (Ling and Luoma, 2013). More than two decades ago, it was shown that PPARs and their ligands can repress inflammatory genes in activated monocytes and macrophages (Ricote et al., 1998; Tontonoz et al., 1998). However, the role of the PPARα receptors and their ligands on cardiac remodeling, repair and functionality exerted by monocytes/macrophages in the context of infection with T. cruzi has not been extensively studied. The efficacy of PPARα agonists, including fenofibrate, as regulators of inflammation and remodeling of the extracellular matrix of the heart has been reported (Lockyer et al., 2010). Fenofibrate has been shown to be able to prevent cardiac inflammation and fibrosis in diabetic mice (Zhang et al., 2016). Furthermore, it has been shown to exert cardioprotective effects against various cardiac disorders, namely, in vivo models of cardiac hypertrophy produced by pressure overload (Zou et al., 2013) or experimental autoimmune myocarditis in rats (Cheng et al., 2016) and also in patients with systolic dysfunction (Yin et al., 2013) or experimental myocardial infarction (Garg et al., 2016). Due to its ability to prevent interstitial and perivascular fibrosis in kidney, liver, lung and heart in different experimental models, fenofibrate has recently been proposed as a potential antifibrotic agent (McVicker and Bennett, 2017).
In previous studies, our group developed an experimental model of Chagas disease, in which mice were sequentially infected with two T. cruzi strains, which differ in genetic background and lethality, leading to clear signs of left ventricular dysfunction. In this model, we show that fenofibrate controls inflammation, prevents fibrosis, and improves heart function (Cevey et al., 2017). Furthermore, PPAR agonists contribute to neovascularization and redirect pro-inflammatory to healing macrophages in experimental trypanosomiasis (Penas et al., 2013; Penas et al., 2015; Cevey et al., 2016; Garg et al., 2016; Penas et al., 2016; Cevey et al., 2017; McVicker and Bennett, 2017; Penas et al., 2017; Rada et al., 2020).
Consequently, the aim of this work was to characterize the monocyte populations of patients in different phases of CCC and to study the effect of fenofibrate on these cells in culture. This would allow identifying possible therapeutic targets promoting fenofibrate as a coadjuvant to anti-parasitic treatment.
Materials and Methods
Ethics Statement
Informed consent was signed by each subject. The study protocol is in line with the ethical guidelines of the Declaration of Helsinki and was approved by the Ethics Committee of the “Hospital General de Agudos Dr. Cosme Argerich” and of the “Hospital Municipal de Rehabilitación Respiratoria María Ferrer”, Buenos Aires, Argentina.
Study Cohort
Subjects were recruited at the Cardiology Department of both Hospital General de Agudos Dr. Cosme Argerich and Hospital Municipal de Rehabilitación Respiratoria MaríaFerrer (Ciudad Autónoma de Buenos Aires, Argentina).
Inclusion Criteria
Men and women between 18 and 60 years, with positive serology for Chagas disease were included. Each seropositive participant underwent a clinical and cardiological evaluation to determine the clinical stage of the disease. The classification of patients was carried out according to the Chagas Consensus (Healthy or Chagas Stage 0, I, II, and III) in accordance with the criteria of the Argentine Society of Cardiology (Mitelman, 2011). In this work and consistent with this classification, we named the different groups according to the absence of symptoms (Asy) or the presence of any cardiac damage (CHD). Control group included healthy individuals (HI), men and women between 18 and 60 years, with negative serology for Chagas disease. None of the subjects should have co-morbidities at the time of sample collection, nor have received previous treatment for Chagas disease nor with lipid-lowering agents from the group of fibrates or statins.
Peripheral Blood Mononuclear Cells (PBMC) Isolation
Whole blood (10 to 15 ml) was collected from participants by venipuncture into heparinized tubes (Vacutainer, BD Biosciences). Plasma was obtained by centrifugation and stored at −80°C. Peripheral blood mononuclear cells (PBMC) were isolated by Ficoll–Paque™ PLUS density gradient centrifugation (GE Healthcare, Amersham, Sweden). PBMC were washed twice and suspended in complete culture medium: RPMI 1640 (Invitrogen Life Technologies, Grand Island, NY, USA) supplemented with fetal bovine serum (FBS) 10% (Internegocios S.A., Argentina) and antibiotics (50 μg/ml of PenStrep®). All experiments were performed using freshly isolated PBMC.
T. cruzi Culture and Lysate
Vero cells were cultured in cell culture flasks of 175 cm2 with RPMI supplemented with 10% fetal bovine serum (FBS), 100 IU/ml Penicillin, 0.1 mg/ml Streptomycin and 2 mM L-glutamine. When culture reached an approximate 50% confluence, it was infected with parasites of the RA strain of T. cruzi. After 6 h, the cells were washed with fresh culture medium to remove non-infective parasites and incubated at 37°C for 48 h.
On day 5 post-infection (dpi), trypomastigotes were harvested from the supernatant. The culture medium was collected, two washes were performed with cold PBS and then it was centrifuged at 18,000×g at 4°C for 5 min. The parasite pellet was stored at −80°C. After one month of collection, all sediments were pooled and lysed to obtain trypomastigote proteins. Briefly, sediments were resuspended in lysis buffer (PBS, 10 µM E-64 and 3 µg/ml protease inhibitor) and subjected to 3 freeze/thaw cycles (−80°C/room temperature) of 30 min each. Then, it was incubated overnight at −80°C and centrifuged at 17,000×g at 4°C for 10 min. Supernatant was collected, and the protein concentration was quantified by the Bradford method using a commercial protein assay (Bio-Rad, USA) and bovine serum albumin (BSA) (Sigma-Aldrich Co, USA) as a standard (Kruger, 1994) as described previously by our group (Cevey et al., 2019; Penas et al., 2020; Rada et al., 2020).
In Vitro Treatments
According to the experiment, cells were pre-treated for 15 min with 100 µM Fenofibrate® (Daunlip®, Montpellier S.A, Argentina. PubChem Compound Database CID = 3339, Fen) resuspended in PBS (Cevey et al., 2017). Then, cells were stimulated or not with T. cruzi lysate (10 µg/ml) for 20 h.
Flow Cytometry
This experiment included 17 healthy individuals, 7 asymptomatic patients with positive serology for Chagas disease and 9 with Chagas heart disease. Cells from all experimental groups were cultured for 16–20 h after treatment.
PBMC were stained with LIVE/DEAD™ fixable dye (Invitrogen) at room temperature for 15 min and labeled with the following antibodies at 4°C for 30 min: CD14 (#E-AB-F1209C, Elabscience), CD16 (#E-AB-F1005M, Elabscience), HLA-DR (#E-AB-F1111H, Elabscience), and CCR2 (#357209, Elabscience). Then, cells were washed, fixed and acquired using a FACS Canto (Becton Dickinson). Post-acquisition analysis was performed using FlowJo version 10 software (FlowJo LLC, Ashland, Oregon, USA). In all cases, isotype-matched mAb were used as controls.
Gating Strategies
Peripheral blood mononuclear cells (PBMC) were gated based on forward scatter (FSC) and side scatter (SSC) parameters. After excluding doublets and debris using FSC-Width vs. FSC-Area, the strategy used to differentiate the three subsets of monocytes was based on CD14 and CD16 expression: classical (CD14high/CD16neg), intermediate (CD14high/CD16pos) and non-classical (CD14low/CD16pos) monocytes. Then, in both total monocytes and subpopulations, the percentage (%) and mean fluorescence intensity (MFI) of the membrane markers CCR2 and HLA-DR was calculated. The MFI was calculated as the geometric mean of the expression.
RNA Purification
Total RNA was obtained from PBMC using Quick-zol reagent (Kalium Technologies, Argentina), treated with RQ1 RNase-Free DNase (PromegaCo., USA). Total RNA was reverse-transcribed using M-MLV Reverse Transcriptase (Promega Co., USA), according to manufacturer’s instructions as described previously by our group (Cevey et al., 2019; Penas et al., 2020; Rada et al., 2020).
Quantitative Reverse Transcription Polymerase Chain Reaction (RT-qPCR)
mRNA expression was performed using 5× HOT FIREPOL EVAGREEN qPCR (SolisBioDyne, Estonia) in a StepOnePlus Real-Time PCR System. Parameters were: 52°C for 2 min, 95°C for 15 min, and 40 cycles at 95°C for 15 s, specific Tm °C for 30 s and 72°C for 1 min. Normalization was carried out using β-Actin mRNA. Quantification was performed using the comparative threshold cycle (Ct) method, as all the primer pairs (target gene/reference gene) were amplified using comparable efficiencies (relative quantity, 2−ΔΔCt) (Schmittgen and Livak, 2008; Bustin et al., 2009). To evaluate the expression of inflammatory mediators in the PBMC of both the asymptomatic (Asy) and cardiac (CHD) patient groups, the PBMC of healthy individuals (HI) were used as reference control (Figure 1). On the other hand, to study the effects of fenofibrate treatment in vitro, on the expression of inflammatory mediators in PBMC of Asy and CHD patients, PBMC samples from each patient not treated with fenofibrate were taken as reference controls (Figure 2). mRNA expression of IL-12, TGF-β, IL-6 and MCP-1 was measured in 20 healthy individuals, 13 asymptomatic patients and 28 cardiac patients. CCR2 mRNA expression was measured in 6 healthy individuals, 4 asymptomatic patients and 5 cardiac patients.
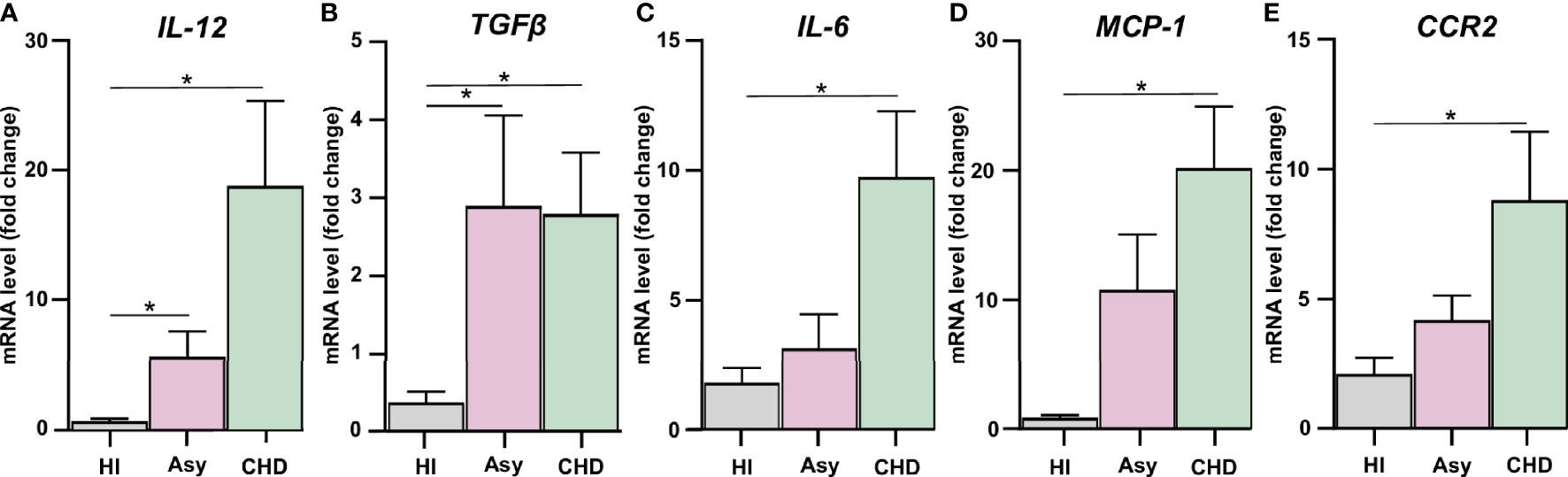
Figure 1 Assessment of the expression of pro-inflammatory mediators and CCR2. Expression of IL-12 (A), TGF-β (B), IL-6 (C), MCP-1 (D), and CCR2 (E) were determined by RT-qPCR in PBMC of healthy individuals (HI), seropositive asymptomatic (Asy) or Chagas heart disease (CHD) after 48 h of culture. mRNA expression was analyzed and normalized against β-Actin. Results are expressed as the mean of 3 independent experiments. Differences between groups were analyzed by Kruskal–Wallis test (mean ± SEM) followed by Dunn’s post hoc test. *P < 0.05. PBMC of Asy or CHD vs. PBMC of HI.
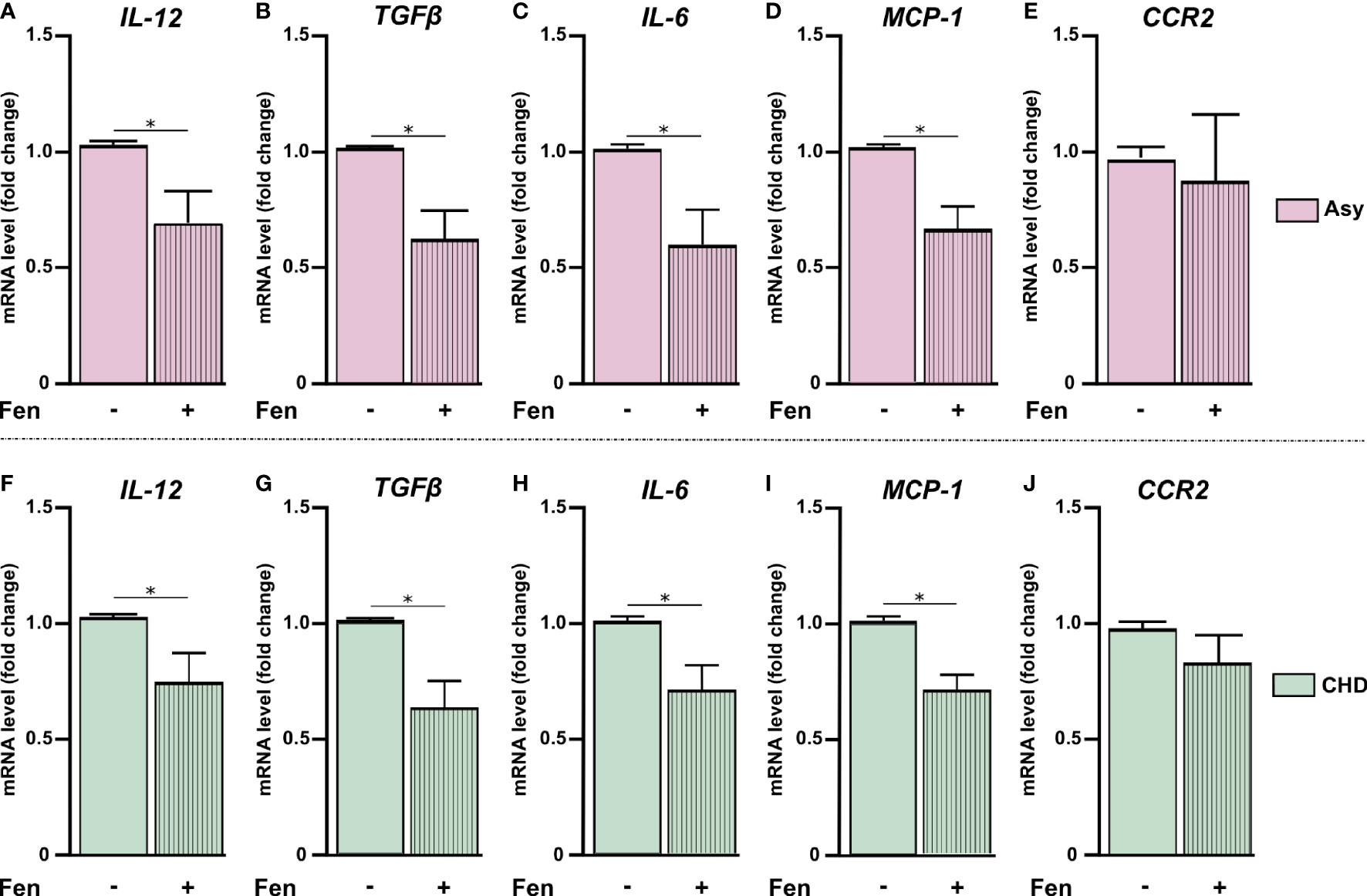
Figure 2 Fenofibrate modulates pro-inflammatory mediators’ expression. PBMC were treated in vitro or not with 100 µM of fenofibrate. After 48 h, IL-12, TGF-β, IL-6, MCP-1, and CCR2 mRNA was measured in asymptomatic (Asy) (A-E) and Chagas heart disease patients (CHD) (F-J). mRNA levels were determined by RT-qPCR and normalized against β-Actin. Results are expressed as mean of 3 independent experiments. Differences between fenofibrate-treated PBMC were analyzed using the Wilcoxon test for paired samples and are shown as the mean of the experiments ± SEM. *P < 0.05. Fen-treated PBMC vs. untreated PBMC.
Primer Sequences
Statistical Analysis
Different statistical tests were used for this work. To compare baseline cytokine mRNA expression between healthy donors, asymptomatic or Chagas heart disease patients, nonparametric Kruskal–Wallis test, and then the Dunn´s multiple comparisons test were performed. For the study of the effect of fenofibrate in mRNA expression, a non-parametric Wilcoxon test was used for paired samples. Mixed-effects model analysis was performed to analyze differences between experimental groups in flow cytometry assays. The Tukey post-hoc test was performed to compare every mean with every other mean. Differences were considered statistically significant when P <0.05. All analyses were performed using the Prism 7.0 Software.
Results
Cohort Characteristics of Chagas Disease Patients
Table 1 shows clinical and electrocardiographic findings in the cohort of Chagas disease patients under study. They were classified according to the absence of symptoms (Asy) or the presence of any cardiac damage (CHD) in stage I, II, or III according to severity. Control group included healthy individuals (HI), men and women between 18 and 60 years, with negative serology for Chagas disease. Most patients were born in disease endemic areas with vectorial transmission.
Pro-Inflammatory Mediators’ Expression in PBMC From Chagas Disease Patients
Groups of seropositive patients with different clinical forms for Chagas disease were included in this study, namely, with cardiomyopathy and without evidence of cardiac symptoms, and also healthy individuals. In order to evaluate whether spontaneous release of inflammatory cytokines and chemokines were differentially expressed, cultured PBMC from patients with Chagas disease were analyzed. mRNA levels of IL-12, IL-6, TGF-β, MCP-1, and CCR2 were determined by RT-qPCR, to assess the basal level production of pro-inflammatory mediators. We observed that PBMC from Chagas disease patients displayed a higher level of cytokines than cells from HI, irrespective of the clinical stage of the disease. The expression of IL-12 in PBMC of Asy patients with positive serology for Chagas but without heart disease was higher than HI. However, in patients with CHD its expression was even higher, as shown in Figure 1A. A similar result can be observed in the evaluation of TGF-β, since PBMC of both groups of patients show increased levels of this cytokine with respect to the values of healthy individuals (Figure 1B). However, when the expression of IL-6, MCP-1, and CCR2 was evaluated, we found that only PBMC from patients with heart disease displayed significantly increased levels of these cytokines in comparison with healthy individuals (Figures 1C–E).
Fenofibrate Reduces Inflammatory Mediator Levels in PBMC From Chagas Heart Disease Patients
We have previously reported that fenofibrate significantly reduces the extension of heart infiltrates and the expression of pro-inflammatory cytokines in a murine model of mixed-stains infection with bloodstream trypomastigotes (Cevey et al., 2017; Rada et al., 2020). In this work, we observed that pro-inflammatory mediators displayed higher levels of expression in cultured PBMC from Chagas disease patients in comparison with those of HI. In order to evaluate whether treatment with fenofibrate was able to promote a reduction of pro-inflammatory cytokines in isolated PBMC in vitro, we assessed the levels of mRNA expression of those mediators. We previously demonstrated, in a work by our group and in line with other studies, that 100 µM of fenofibrate is the optimal concentration at which inflammatory mediators are inhibited without affecting the viability of primary cardiomyocyte cultures (Penas et al., 2015; Nahrendorf, 2018). As shown in Figure 2, in vitro treatment of PBMC from Asy and CHD patients with 100 µM fenofibrate, reduces the levels of IL-12, TGF-β, IL-6, MCP1 in comparison with untreated cells (Figures 2A–D, F–I). However, CCR2 expression levels were not significantly modified in Asy or CHD patients (Figures 2E, J). Likewise, fenofibrate does not modify the expression of any of the studied cytokines in PBMC from healthy individuals (Supplementary Figure S1A).
Patients With Different Stages of Chagas Disease Display Changes in the Frequencies of Monocyte Subsets
Different subpopulations of monocytes are involved in the progression of Chagas disease cardiomyopathy. To determine whether certain monocyte subsets were particularly expressed in Chagas disease patients, according to the disease stage, the monocyte population in whole PBMC from HI, Asy and CHD patients was characterized by flow cytometry analysis (FACS), according to the expression of CD14 and CD16, as classical (CD14high CD16neg), intermediate (CD14high CD16pos) and non-classical (CD14low CD16pos) (Figure 3A). Figures 3B–D show the effects of T. cruzi stimulation and treatment of PBMC with Fen, on the percentage of classical, intermediate, and non-classical monocytes from uninfected, asymptomatic and Chagas heart disease patients. The results depicted in Figure 3B in T. cruzi unstimulated and untreated cells, show a significantly higher level of classical monocytes in CHD patients in comparison with Asy. However, under the same conditions the subpopulation of intermediate monocytes, CD14high CD16pos, does not show differences between CHD and Asy or HI (Figure 3C). Notably, T. cruzi unstimulated and untreated cells from Asy patients have a significantly higher percentage of non-classical monocytes than HI (Figure 3D).
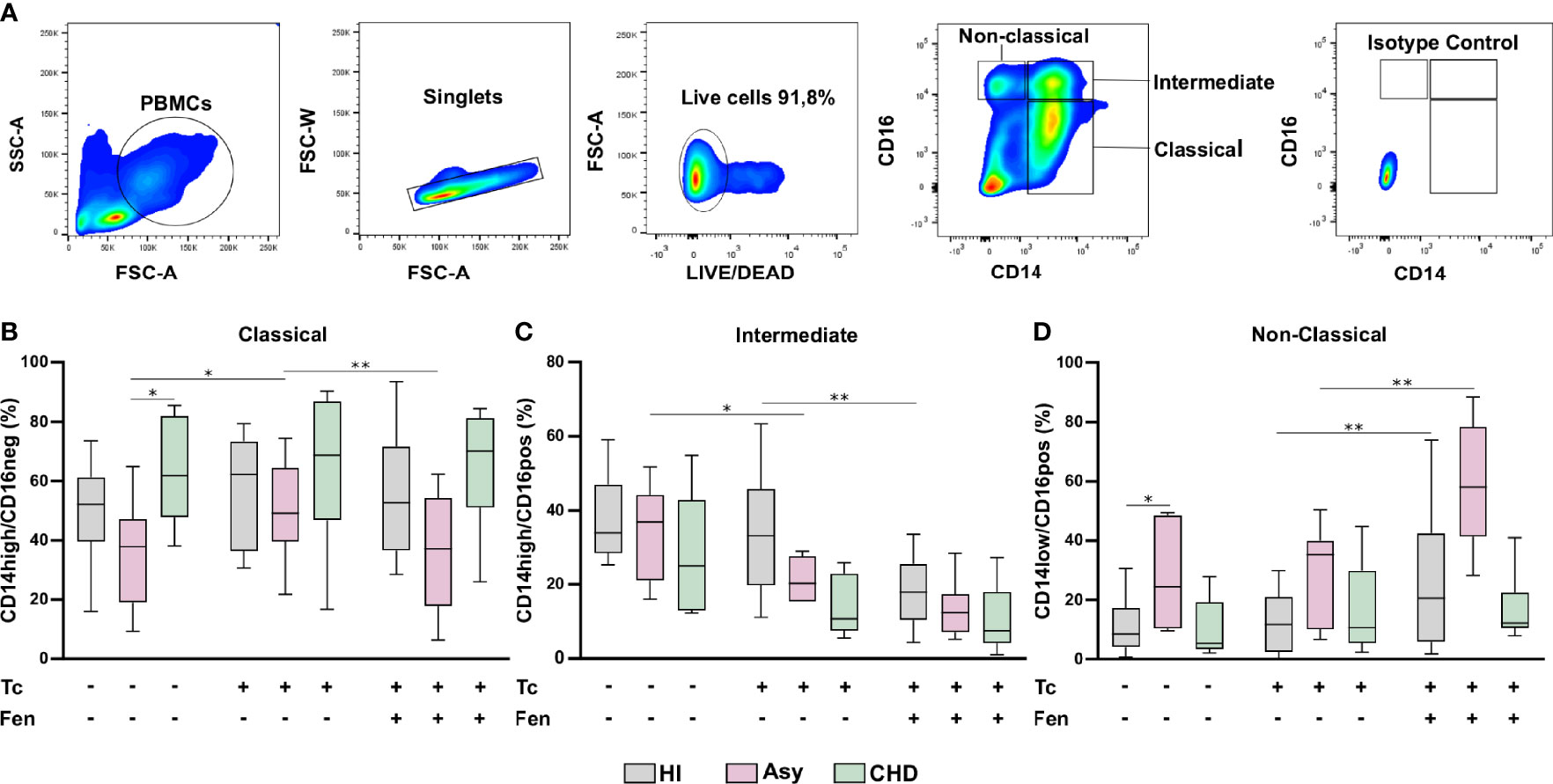
Figure 3 Percentage of monocyte subpopulations in patients with Chagas heart disease (CHD), asymptomatic (Asy) and healthy individuals (HI) with/without in vitro treatment. A representative analysis of the gating strategy used in this study to differentiate the three monocyte subpopulations is shown (A). PBMC were stimulated or not with T. cruzi lysate (Tc) and treated or not with fenofibrate (Tc + Fen). After 20 h according to CD14 and CD16 expression, they were classified as Classical (CD14high/CD16neg), Intermediate (CD14high/CD16pos) and Non-Classical (CD14low/CD16pos). Percentage of classical (B), intermediate (C) and non-classical (D) monocytes for HI, Asy and CHD unstimulated, stimulated with Tc lysate and with Tc lysate + Fen treatment. These data were analyzed by fitting a mixed model with a Tukey post-hoc test and the results are expressed as the mean of the experiments ± SEM. *P < 0.05. CHD vs. Asy; Asy vs. HI. *P < 0.05. Tc vs. untreated; **P < 0.01. Tc + Fen vs. Tc.
In Vitro Stimulation With T. cruzi and Fenofibrate Treatment Changes the Prevalence of Monocyte Subsets According to the Stage of the Disease
We sought to determine whether monocytes from Asy or CHD patients exhibit different responses against the parasite. To this aim, purified PBMC were stimulated in vitro with T. cruzi lysates (10 μg/ml) for 24 h. Figure 3B shows that stimulation with lysates significantly increases the percentage of CD14high CD16neg, classical monocytes, of the Asy group, but does not change their frequencies in CHD or HI groups. On the other hand, stimulation with T. cruzi lysates decreases the percentage of CD14high CD16pos, Intermediate monocytes, in Asy patients, and a trend to decreased frequencies is also observed in CHD patients (Figure 3C). However, no changes were observed in the percentage of this monocyte subset in HI (Figure 3C). Notably, the stimulation with T. cruzi did not modify the frequency of non-classical subpopulation, CD14lowCD16pos, monocytes in any of the groups studied (Figure 3D).
Fenofibrate pre-treatment of T. cruzi-stimulated monocytes exerted a modulatory effect, decreasing the percentage of classic monocytes in the Asy population (Figure 3B). Figure 3C shows that fenofibrate decreases the frequency of CD14highCD16pos cells in HI, but we only observed a trend to decrease this cell subset in Asy and CHD patients. Interestingly, fenofibrate significantly increased the percentage of non-classical cells in the HI and Asy groups but this was not modified in the CHD group (Figure 3D). Of note, fenofibrate alone does not modify any monocyte subpopulation in HI, Asy, and CHD (Figure S1B).
In Vitro T. cruzi Stimulation and Fenofibrate Treatment Modify CCR2 and HLA-DR Expression in Monocyte Subsets
The most prominent role of CCR2 is believed to be in the mobilization of monocytes under physiologic and also inflammatory conditions. With the purpose to establish the expression of CCR2 on total monocytes (CD14+ cells), according to clinical status, PBMC from CHD, Asy, and HI groups were stained with CD14 and CCR2 and analyzed by FACS (Figure 4A). The results show high basal levels of CCR2 in CD14+CCR2+ monocytes of all groups (Figures 4B-D). When CD14+CCR2+ cells were stimulated with T. cruzi lysates, its percentage in Asy and CHD patients decreased significantly, and also in healthy controls (Figures 4B–D). Furthermore, especially in Asy, the pretreatment with fenofibrate of monocytes stimulated with T. cruzi induces a trend to restore the basal levels of CD14+CCR2+ cells (Figure 4C). Besides, that fenofibrate does not modify CCR2+ levels in unstimulated CD14+ cells (Supplementary Figure S1C).
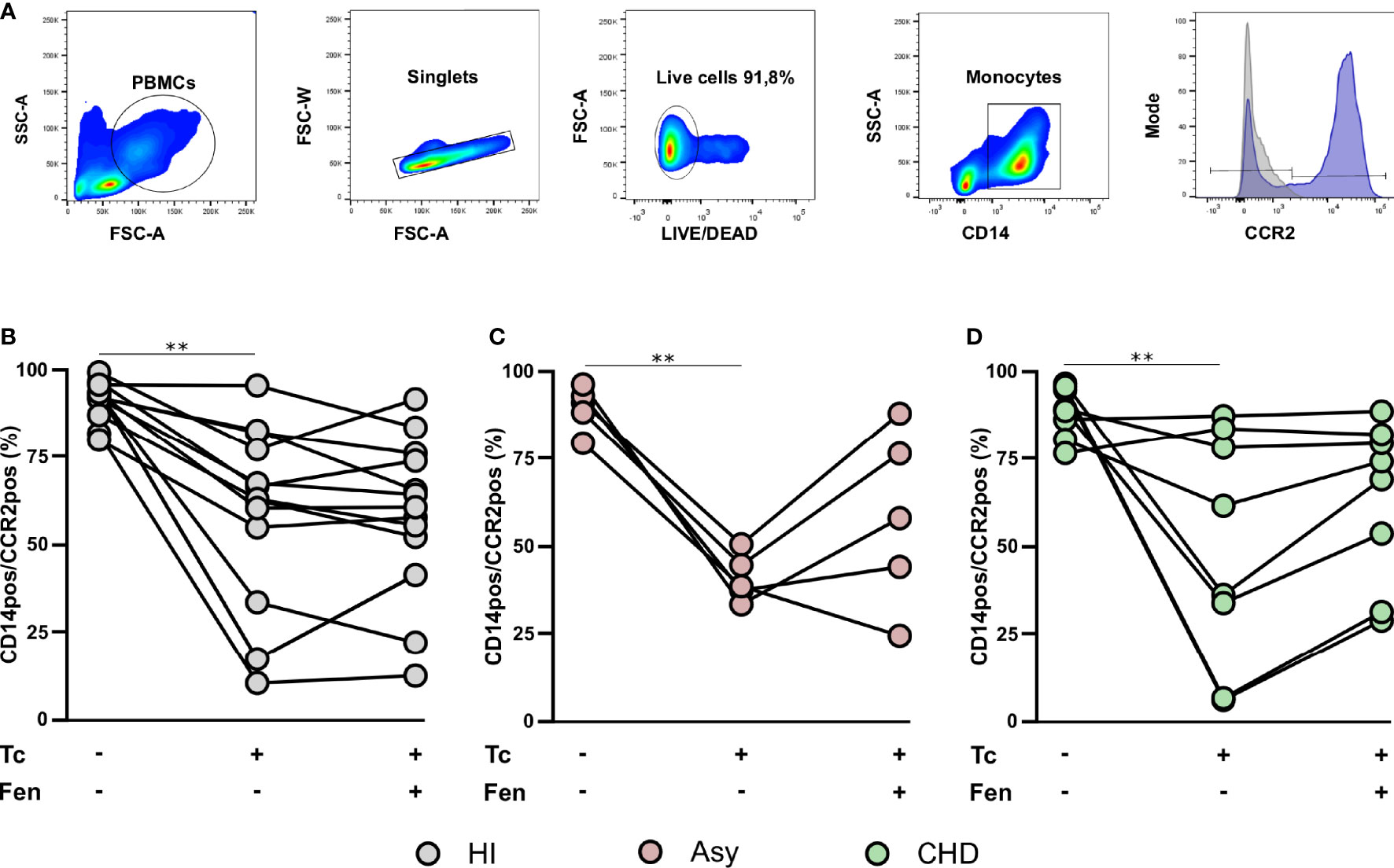
Figure 4 T. cruzi stimulation and fenofibrate treatment modify CCR2 in CD14pos cells. Percentage of CCR2 was determined in basal CD14pos cells after 20 h of T. cruzi lysate (Tc) stimulation or fenofibrate (Tc + Fen) treatment. Monocytes were selected based on FSC and SSC. After excluding doublets and debris, live cells were selected, monocytes were classified by CD14 positive staining. Representative histograms show the number of events and expression level of CCR2 (A). The percentages of CD14pos/CCR2pos monocytes are shown in healthy individuals (HI) (B), asymptomatic (Asy) (C) and patients with Chagas heart disease (CHD) (D), where each patient is represented by a circle. The results are shown as the mean of the experiments ± SEM. These data were analyzed by fitting a mixed model with a Tukey post-hoc test. **P < 0.01. Tc vs. untreated.
When CCR2 expression was analyzed in the different monocyte subpopulations, we observed that the percentage of CCR2+ cells in HI and CHD decreased in the classical monocyte subpopulation stimulated with T. cruzi lysates. Furthermore, fenofibrate treatment restored the baseline level only in monocytes from CHD patients and HI (Figure 5A). The intermediate monocyte subpopulation showed a decrease in its expression upon stimulation with T. cruzi, which was only significant in monocytes from HI. However, fenofibrate did not restore the decreased expression of CCR2 (Figure 5B). Regarding the subpopulation of non-classical monocytes, the stimulation with T. cruzi significantly decreased the percentage of cells expressing CCR2 in the CHD and HI groups, although only with a trend in Asy patients. Treatment with fenofibrate significantly increased the percentage of CCR2 in Asy and CHD as shown in Figure 5C. In order to determine the expression of HLA-DR in different disease stages, PBMC of the three experimental groups were stained with CD14 and HLA-DR (Figure 6A). Figures 6B, C show no significant differences between cells stimulated in vitro with T. cruzi lysates in comparison with unstimulated cells, in both Asy and HI. However, there is a trend to increased expression of HLA-DR with T. cruzi stimulation in CHD patients, according to its mean fluorescence intensity (MFI) (Figure 6D). As in the case of CCR2, fenofibrate alone did not modify the increased expression of HLA-DR in unstimulated and treated cells (Supplementary Figure S1C). This was also observed in cells from Asy and HI stimulated with T. cruzi. However, fenofibrate shows a clear tendency to inhibit the increased HLA-DR expression of PBMC from CHD upon stimulation with T. cruzi (Figure 6D).
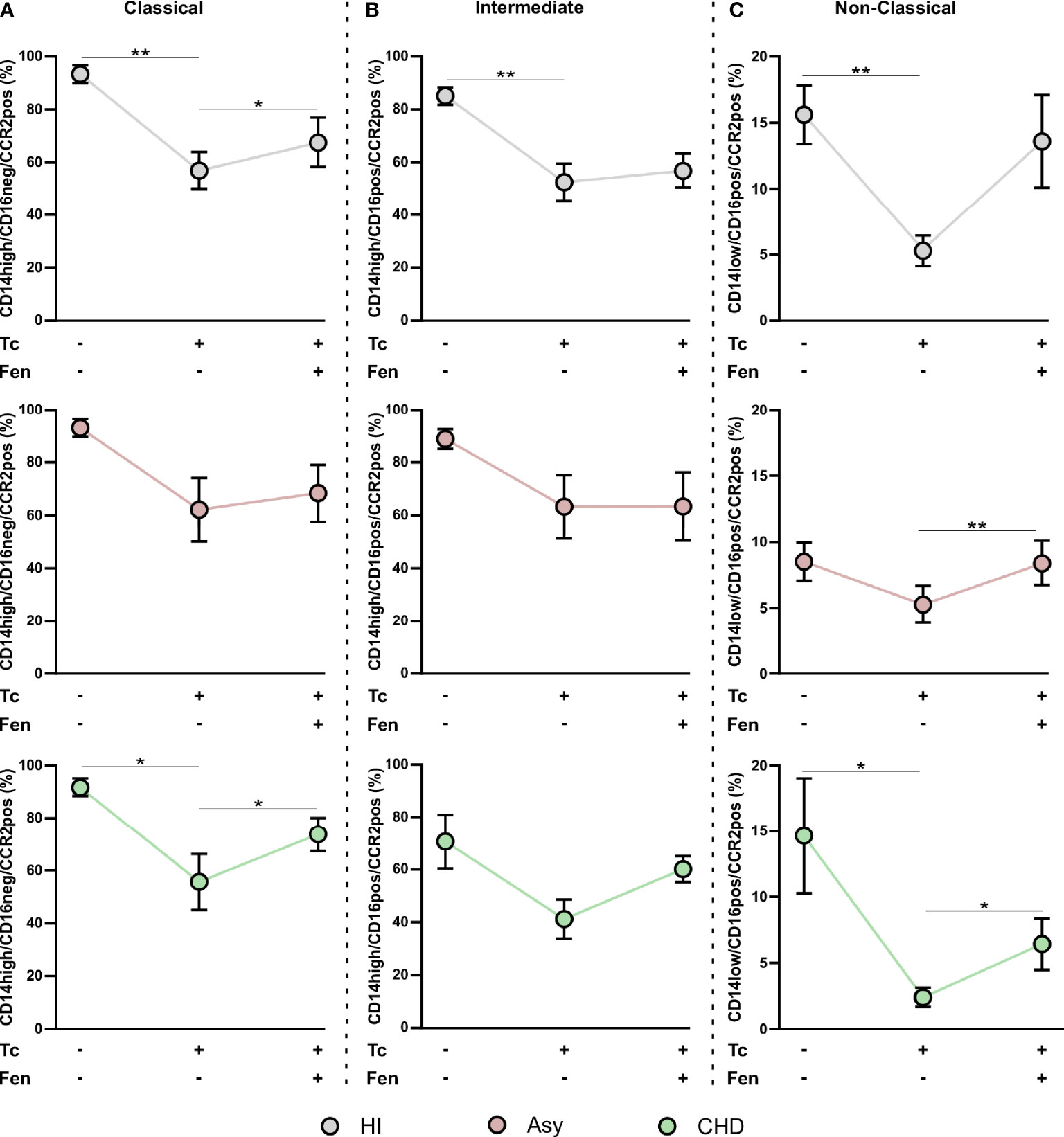
Figure 5 T. cruzi stimulation and fenofibrate treatment modify CCR2 in monocyte subpopulations. Percentage of CCR2+ cells was determined in PBMC stimulated or not with T. cruzi lysate (Tc) and treated or not with fenofibrate (Tc + Fen) after 20 h, according to CD14 and CD16 expression. It shows the percentage of classical (CD14high/CD16neg) (A), intermediate (CD14high/CD16pos) (B) and non-classical (CD14low/CD16pos) (C) monocytes with CCR2+ expression. The results are shown as the mean of the experiments ± SEM. These data were analyzed by fitting a mixed effect model with a Tukey post-hoc test. *P < 0.05; **P < 0.01.
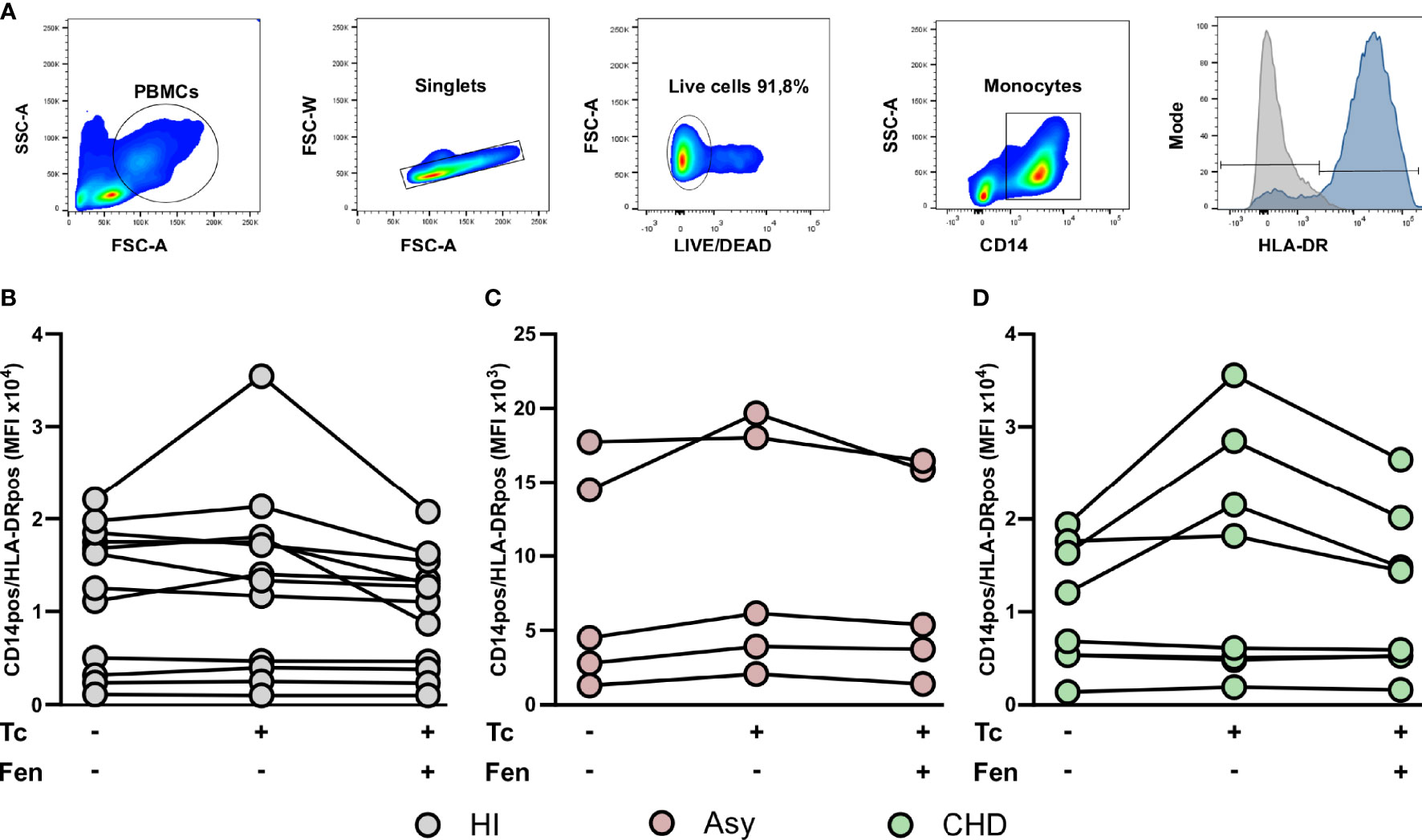
Figure 6 HLA-DR expression in monocyte. The mean fluorescence intensity (MFI) of HLA-DR was determined in basal CD14pos cells after 20 h of T. cruzi lysate (Tc) stimulation or fenofibrate (Tc + Fen) treatment. Monocytes were selected based on FSC and SSC. After excluding doublets and debris, live cells were selected, monocytes were classified by CD14 positive staining. The mean fluorescence intensity (MFI) of HLA-DR was calculated both in total monocytes (A). It shows the mean fluorescence intensity (MFI) of CD14pos/HLA-DRpos monocytes in healthy (HI) (B), asymptomatic (Asy) (C) and chronic Chagas disease (CHD) patients (D), where each patient is represented by a circle. The results are shown as the mean of the experiments ± SEM. These data were analyzed by fitting a mixed effect model with a Tukey post-hoc test.
When we studied HLA-DR expression in the classical monocyte subpopulation, we observed that stimulation with T. cruzi tends to raise the MFI of monocytes from CHD patients and treatment with fenofibrate displays a trend to restore it to basal levels (Figure 7A). We found that both stimulation with T. cruzi lysates and fenofibrate treatment did not modify HLA-DR expression in intermediate or non-classical monocyte subpopulations, as shown in Figures 7B, C. Besides, fenofibrate neither modifies CCR2+ nor HLA-DR levels in unestimulated monocytes subsets of CHD, Asy or HI (Supplementary Figure S2A).
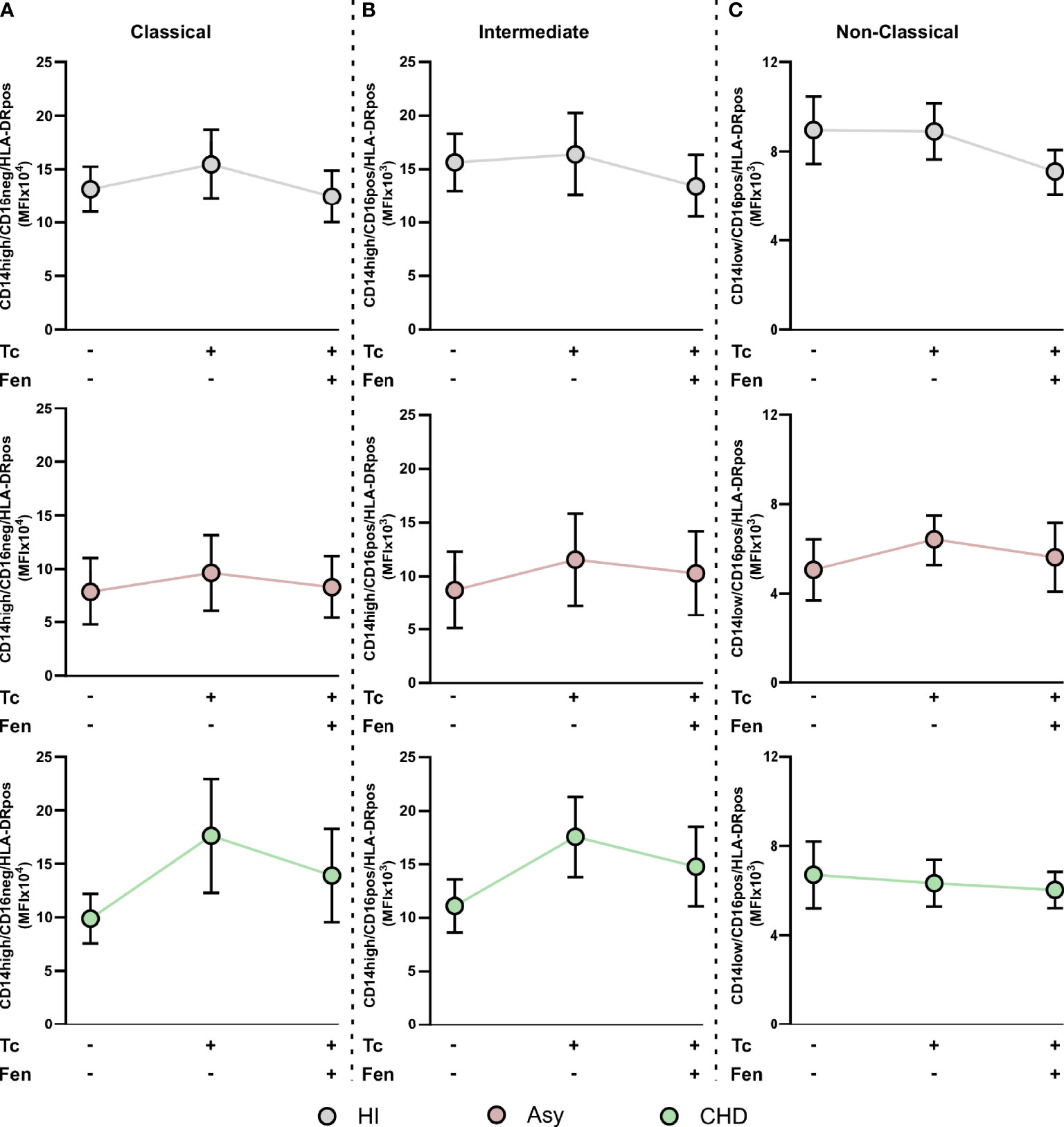
Figure 7 HLA-DR expression in T. cruzi stimulated and fenofibrate treated monocyte subpopulations. The mean fluorescence intensity percentage of HLA-DR+ cells was determined in PBMC stimulated or not with T. cruzi lysate (Tc) and treated or not with fenofibrate (Tc + Fen) after 20 h, according to CD14 and CD16 expression. It shows the mean fluorescence intensity (MFI) of classical (CD14high/CD16neg) (A), intermediate (CD14high/CD16pos) (B) and non-classical (CD14low/CD16pos) (C) monocytes with HLA-DR+ expression. The results are shown as the mean of the experiments ± SEM. These data were analyzed by fitting a mixed effect model with a Tukey post-hoc test.
Discussion
Monocytes are heterogeneous and multifunctional cells. As components of the innate immune response, they participate not only in inflammation and fibrosis, but also in tissue repair and regeneration during heart diseases (Apostolakis et al., 2010). Consequently, inflammatory cells, such as monocytes, are increasingly being considered as potential drug targets for the treatment of different heart conditions (Nahrendorf, 2018). Regarding Chagas disease, while the relevance of parasite persistence as a trigger of tissue damage is currently acknowledged in the development of CCC, the role of the different components of the inflammatory response remains unclear (Zhang and Tarleton, 1999; Lopez et al., 2018; Wesley et al., 2019).
In the present work, we first determined the spontaneous expression of pro-inflammatory mediators, such as MCP-1 and its receptor CCR2, and IL-12, TGF-β, IL-6 in cultured PBMC from seropositive patients with different clinical forms of Chagas disease. The results show that PBMC from CHD patients displayed a significantly higher expression level of these mediators than cells from uninfected individuals. Moreover, in Asy patients we observed a significantly increased expression of IL-12 and TGF-β, and a trend to increase in the other cytokines evaluated (Figure 1). These results are in consonance with those found by other authors in neutrophils and monocytes for similar groups of patients (Souza et al., 2004; Campi-Azevedo et al., 2015; Medeiros et al., 2017; Pinto et al., 2018).
Recently, Dey et al. showed that mouse CD11c+ classical dendritic cells, but not CD11b+ Ly6c+ inflammatory monocytes, are the source of IL-6 required for the expansion of protective Th17 cells against drug-resistant Leishmania donovani (Dey et al., 2020). Previous studies by Stäger et al. also reported that IL-6 deficiency was associated with the expansion of IL-10-producing Treg cells, while expansion was not observed in IL-12p40-deficient mice, stressing the role of IL-6 in the control of infection (Stäger et al., 2006). Regarding our results, it must be noted however, that the actual source of these, and also the other cytokines was not investigated. Independently of this fact, it is clear that both IL-12 and IL-6 are associated with a more severe outcome, since they were particularly elevated in symptomatic patients in comparison with asymptomatic ones or healthy controls. Since in the context of parasite persistence in the tissues, T. cruzi induces a substantial increase in pro-inflammatory mediators and reactive oxygen and nitrogen species (Cevey et al., 2017; Penas et al., 2017), this scenario would favor tissue damage and contributes to the clinical outcome observed in symptomatic patients with Chagas disease. For this reason, it is desirable to find an anti-inflammatory therapy that might be useful as a coadjuvant of the antiparasitic treatment to preclude the onset of heart damage during the course of infection.
Previous studies from our group showed that PPAR agonists, such as fenofibrate, a potent hypolipidemic drug, also bears anti-inflammatory properties in the context of experimental Chagas disease (Cevey et al., 2017; Rada et al., 2020). In this work we demonstrated that in vitro treatment with fenofibrate significantly reduces the expression of pro-inflammatory mediators, in PBMC (Figure 2). These findings are in line with those of Krysiak et al., in which fenofibrate decreased the release of TNFα, IL-1β, IL-6, and MCP-1 by human monocytes. Those effects were accompanied by a decrease in plasma C-reactive protein levels, which could be clinically relevant in the prevention of vascular complications (Krysiak et al., 2011). In this regard, we demonstrated that fenofibrate controls inflammation, prevents fibrosis, contributes to neovascularization and improves left ventricular function, in an experimental murine model of Chagas disease (Cevey et al., 2017). Moreover, in another work, we showed that this occurs through IL-10-dependent and -independent mechanisms (Rada et al., 2020). These anti-inflammatory and protective effects of fenofibrate have been also evaluated in models of autoimmune myocarditis (Cheng et al., 2016), skeletal muscle inflammation (Dai et al., 2016), and cardiac ischemia/reperfusion models (Sugga et al., 2012).
In this work, we studied the monocyte population of patients with different stages of Chagas disease. Our results show that patients with CHD have a significantly higher percentage of classical monocytes. Besides, we determined that there are no significant differences between HI, Asy, or with CHD individuals in the intermediate monocyte subpopulation. Interestingly, we found that Asy patients have a significantly higher percentage of non-classical monocyte subpopulation (Figure 3), suggesting they are in an alert and patrolling state. In this sense, the work of Cros et al. demonstrated that human non-classical monocytes exhibited endothelial crawling behavior after adoptive transfer to mice (Cros et al., 2010). Our results are in line with a work by Pérez-Mazliah et al. who showed increased levels of non-classical monocytes in T. cruzi-infected individuals with mild or no signs of cardiac disease, as well as in patients suffering from dilated cardiomyopathy unrelated to T. cruzi infection. In contrast, they also showed that the monocyte profile in T. cruzi-infected individuals with severe cardiomyopathy was slanted to the classical and intermediate subsets (Pérez-Mazliah et al., 2018). Consequently, in vitro experiments showed that CD16+ monocytes have higher mobility than their CD16- counterparts (Randolph et al., 2002). This behavior suggests that non-classical monocytes are constantly inspecting the endothelium for signs of inflammation or damage and preparing to rapidly transmigrate (Wong et al., 2012). Another study attributed the differences in the characterization of the monocyte subpopulations, partly due to the fact that individuals infected with parasites of different discrete typing units (DTU) triggers different immunological impact which could influence the progression of the disease (Passos et al., 2015).
In our work, we evaluated whether in vitro treatment with fenofibrate promotes changes in monocyte subpopulations. We determined that T. cruzi increased the percentage of classical monocytes of Asy patients, while fenofibrate treatment inhibited this effect. In contrast, there were no changes in the classical monocyte population from patients with CHD. On the other hand, T. cruzi produced a significant decrease in the intermediate population of Asy patients that was not modified by fenofibrate. Notably, fenofibrate significantly increased the percentage of non-classical monocytes in Asy patients, suggesting that this treatment promotes a repairing and patrolling behavior. Also, it should be noted that both T. cruzi and fenofibrate were not able to significantly modify the percentage of any of the monocyte subpopulations of patients with CHD (Figure 3).
CCR2 is a chemokine receptor involved in monocyte mobilization and plays a key role in extravasation and transmigration of monocytes under inflammatory conditions (Conductier et al., 2010; Chu et al., 2014). MCP-1, ligand of CCR2, is a potent monocyte activator that is abundantly expressed in various pathological conditions. It has been demonstrated that the loss of MCP-1 by targeted gene disruption is sufficient to impair monocyte trafficking in several inflammation models (Lu et al., 1998). Studies carried out with CCR2−/− mice clearly demonstrated that this receptor exhibits functions as a major mediator of macrophage recruitment and trafficking in host defense to bacterial and parasitic infections (Hardison et al., 2006). Our results clearly show that when monocytes are stimulated with T. cruzi lysates, the percentage of CCR2 decreases in all the groups (Figure 4). It has been postulated that this might occur due to the fact that during chemotaxis, CCR2+ expressed on monocytes internalizes with the bound chemoattractant, but cycles rapidly back to the plasma membrane to maintain high responsiveness (Volpe et al., 2012; Zhao et al., 2019). Notably, fenofibrate tends to restore baseline levels of CCR2 in cells stimulated with T. cruzi (Figure 4). It has been described that CCR2− and CCR2+ macrophages have distinct functions in the heart. CCR2− macrophages are involved in various forms of tissue remodeling such as coronary development, postnatal coronary growth, and cardiac regeneration (Lavine et al., 2014; Leid et al., 2016). Moreover, some studies revealed that after neonatal cardiomyocyte injury, CCR2− macrophages contribute to cardiac tissue regeneration and functional recovery of the heart, expanding the coronary vasculature, cardiomyocyte proliferation, and physiological cardiomyocyte hypertrophy. Particularly, in the pediatric mouse heart, the absence of CCR2− macrophages demonstrated poor regenerative capacity (Epelman et al., 2014; Lavine et al., 2014; Leid et al., 2016). However, analysis of heart transplant recipients in humans revealed that CCR2− macrophages are a tissue-resident population exclusively replenished through local proliferation, whereas CCR2+ macrophages do it through monocyte recruitment and proliferation. In patients with heart failure, CCR2+ macrophage abundance is associated with left ventricular remodeling and systolic function (Bajpai et al., 2018).
Although fenofibrate restored CCR2+ in classical subpopulation of CHD patients, the significant increase in the non-classical subpopulation of Asy and CHD is of great interest due to its restorative characteristics (Figure 5). This could also explain, together with the inhibition of pro-inflammatory mediators in the heart, the improvement in cardiac function that we have demonstrated in the murine model of mixed infection by T. cruzi under treatment with fenofibrate (Cevey et al., 2017; Rada et al., 2020). Further case control studies, namely, cohorts of fenofibrate vs. placebo treated patients, are needed to test the hypothesis that fenofibrate restores the percentage of CCR2+ non-classical monocytes leading to improvement of ventricular function in patients with Chagas disease.
In this work, the expression of HLA-DR in all the groups of patients was studied. We observed a trend to increase in HLA-DR expression in T. cruzi stimulated monocytes from CHD patients. Besides, fenofibrate tends to inhibit this effect (Figure 6). Furthermore, we have shown that HLA-DR tends to increase its expression in the classical monocyte subpopulation from CHD patients stimulated with T. cruzi. Fenofibrate treatment showed a tendency to restore HLA-DR expression to baseline levels (Figure 7A). However, another study shows that not only HLA-DR increased in the classical monocytes of cardiac patients but also in those of indeterminate. Likewise, in that work a lower expression of HLA-DR on intermediate monocytes of Asy patients was observed when compared to the CHD patients (Pinto et al., 2018). Deficiency in the expression of HLA-DR may reflect the severity of a disease. The low HLA-DR MFI constitutes an independent risk factor in predicting mortality, since decreased expression of HLA-DR on monocytes was associated with impaired antigen presentation and poor prognosis (Li et al., 2020). The fact that in our study fenofibrate treatment did not modify HLA-DR expression is encouraging, since it would mean that it does not inhibit antigen presentation capacity.
In conclusion, monocyte profiling through analysis of inflammatory markers may be of value to direct appropriate coadjuvant therapies, like the one envisioned in this work by means of fenofibrate treatment, with the aim to help reduce the extensive and noxious inflammatory and profibrotic response arising in chronic Chagas heart disease patients (Figure 8).
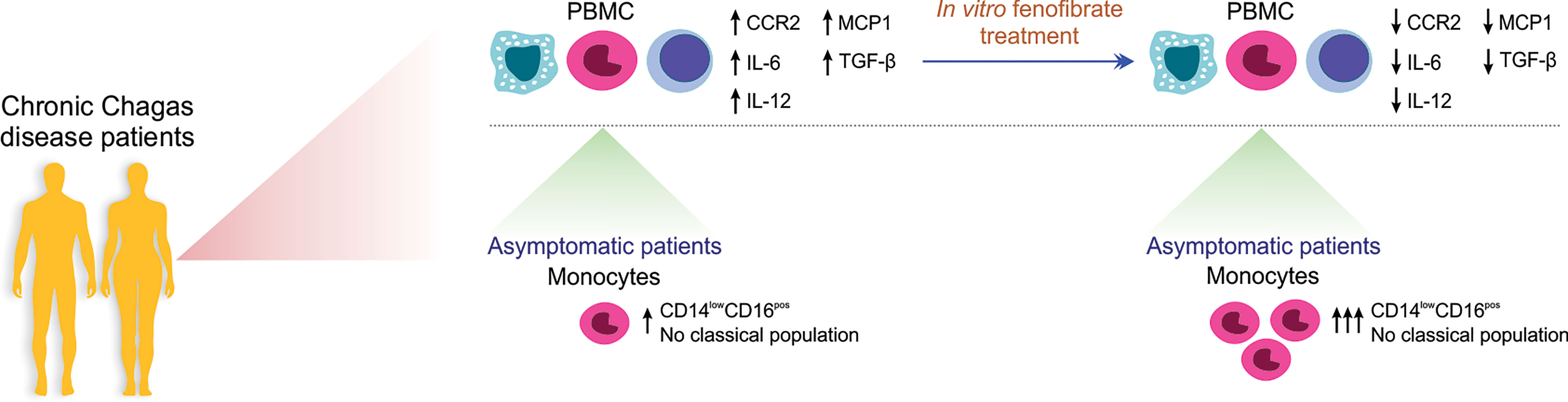
Figure 8 Schematic representation of the in vitro effect of Fenofibrate in PBMC and monocytes from patients with chronic Chagas disease. PBMC from Chagas disease patients display higher levels of IL-12, TGF-β, IL-6, MCP1, and CCR2 than cells from uninfected individuals. In vitro fenofibrate treatment exerts modulatory effect, decreasing the expression of CCR2, IL-6, IL-12, TGF-β, and MCP-1. Asymptomatic patients have a high percentage of non-classical monocytes, which increases even more after fenofibrate treatment.
Data Availability Statement
The original contributions presented in the study are included in the article/Supplementary Material. Further inquiries can be directed to the corresponding author.
Ethics Statement
The studies involving human participants were reviewed and approved by the Comité de Ética del Hospital General de Agudos Cosme Argerich, Ciudad Autónoma de Buenos Aires, Argentina. The patients/participants provided their written informed consent to participate in this study.
Author Contributions
NG and AP designed experiments and analyzed data. NG, AP, GM, AC, and FP and contributed to the writing of the manuscript. AP, AC and FP did experiments. NP, AM, JG, and MG performed the clinical studies and the collection of blood samples. NG, GM, FP, AC, and AP contributed to final approval of the version to be published. All authors contributed to the article and approved the submitted version.
Funding
This work was supported by the Universidad de Buenos Aires [Grant number 20020170100562BA] and the Agencia Nacional de Promoción Científica y Tecnológica [Grant number PICT 2016-0629].
Conflict of Interest
The authors declare that the research was conducted in the absence of any commercial or financial relationships that could be construed as a potential conflict of interest.
Publisher’s Note
All claims expressed in this article are solely those of the authors and do not necessarily represent those of their affiliated organizations, or those of the publisher, the editors and the reviewers. Any product that may be evaluated in this article, or claim that may be made by its manufacturer, is not guaranteed or endorsed by the publisher.
Acknowledgments
We would also like to thank Mr. Sergio Mazzini for his assistance in English grammar and spelling corrections. The authors thank to Dr. Lourdes Arruvito for her contributions to the analysis of cytometry results and to Virginia Gonzalez Polo for her technical assistance in flow cytometry.
Supplementary Material
The Supplementary Material for this article can be found online at: https://www.frontiersin.org/articles/10.3389/fcimb.2021.785166/full#supplementary-material
Supplementary Figures 1 | PBMC were treated in vitro or not with 100 µM of fenofibrate (Fen). After 48 h, IL-12, TGF-β, IL-6, MCP-1 and CCR2 mRNAs were measured in healthy individuals (HI) (A). PBMC were treated or not with 100 µM of Fen. After 20 h according to CD14 and CD16 expression, they were classified as Classical (CD14high/CD16neg), Intermediate (CD14high/CD16pos) and Non-Classical (CD14low/CD16pos). The percentages of Classical, Intermediate and Non-Classical and are shown for HI, asymptomatic (Asy) and patients with Chagas heart disease (CHD) (B). PBMC were treated or not with 100 µM of Fen. After 16-20 h, PBMC were classified according to CD14 and CCR2 expression. The percentages of CD14pos/CCR2pos monocytes are shown in HI, Asy and CHD, where each patient is represented by a circle (C). PBMC were treated or not with 100 µM of Fen. The mean fluorescence intensity (MFI) of HLA-DR was determined in basal CD14pos cells after 20 h of Fen treatment. It shows the MFI of CD14pos/HLA-DRpos monocytes in HI, Asy and CHD patients, where each patient is represented by a circle (D). For (A), mRNA levels were determined by RT-qPCR and normalized against β-Actin. Results are expressed as the mean of 3 independent experiments. Differences between fenofibrate-treated PBMC were analyzed using the Wilcoxon test for paired samples and are shown as the mean of the experiments ± SEM. For (B-D), the data were analyzed by fitting a mixed effect model with a Tukey post-hoc test and the results are expressed as the mean of the experiments ± SEM.
Supplementary Figures 2 | PBMC were treated or not with 100 µM of fenofibrate (Fen). After 20 h according to CD14 and CD16 expression, they were classified as Classical (CD14high/CD16neg), Intermediate (CD14high/CD16pos) and Non-Classical (CD14low/CD16pos) for healthy individuals (HI), asymptomatic (Asy) and patients with Chagas heart disease (CHD). It shows the percentage of classical, intermediate and non-classical monocytes with CCR2+ expression (A) and the mean fluorescence intensity (MFI) of classical, intermediate and non-classical monocytes with HLA-DR+ expression (B). For (A, B), the results are shown as the mean of the experiments ± SEM. These data were analyzed by fitting a mixed effect model with a Tukey post-hoc test.
References
Andrade, D. V., Gollob KJ, D. W. (2014). Acute Chagas Disease: New Global Challenges for an Old Neglected Disease. PloS Negl. Trop. Dis. 8 (7), e3010:1–10. doi: 10.1371/journal.pntd.0003010
Apostolakis, S., Lip, G. Y. H., Shantsila, E. (2010). Monocytes in Heart Failure: Relationship to a Deteriorating Immune Overreaction or a Desperate Attempt for Tissue Repair? Cardiovasc. Res. 85, 649–660. doi: 10.1093/cvr/cvp327
Bajpai, G., Schneider, C., Wong, N., Bredemeyer, A., Hulsmans, M., Nahrendorf, M., et al. (2018). The Human Heart Contains Distinct Macrophage Subsets With Divergent Origins and Functions. Nat. Med. 24, 1234–1245. doi: 10.1038/s41591-018-0059-x
Bustin, S. A., Benes, V., Garson, J. A., Hellemans, J., Huggett, J., Kubista, M., et al. (2009). The MIQE Guidelines: Minimum Information for Publication of Quantitative Real-Time PCR Experiments. Clin. Chem. 55, 611–622. doi: 10.1373/clinchem.2008.112797
Campi-Azevedo, A. C., Gomes, J. A. S., Teixeira-Carvalho, A., Silveira-Lemos, D., Vitelli-Avelar, D. M., Sathler-Avelar, R., et al. (2015). Etiological Treatment of Chagas Disease Patients With Benznidazole Lead to a Sustained Pro-Inflammatory Profile Counterbalanced by Modulatory Events. Immunobiology 220, 564–574. doi: 10.1016/j.imbio.2014.12.006
Cevey, Á. C., Mirkin, G. A., Donato, M., Rada, M. J., Penas, F. N., Gelpi, R. J., et al. (2017). Treatment With Fenofibrate Plus a Low Dose of Benznidazole Attenuates Cardiac Dysfunction in Experimental Chagas Disease. Int. J. Parasitol. Drugs Drug Resist. 7, 378–387. doi: 10.1016/j.ijpddr.2017.10.003
Cevey, Á. C., Mirkin, G. A., Penas, F. N., Goren, N. B. (2016). Low-Dose Benznidazole Treatment Results in Parasite Clearance and Attenuates Heart Inflammatory Reaction in an Experimental Model of Infection With a Highly Virulent Trypanosoma Cruzi Strain. Int. J. Parasitol. Drugs Drug Resist. 6, 12–22. doi: 10.1016/j.ijpddr.2015.12.001
Cevey, Á. C., Penas, F. N., Alba Soto, C. D., Mirkin, G. A., Goren, N. B. (2019). IL-10/STAT3/SOCS3 Axis Is Involved in the Anti-Inflammatory Effect of Benznidazole. Front. Immunol. 10, 1267. doi: 10.3389/fimmu.2019.01267
Cheng, H., Xi, Y., Chi, X., Wu, Y., Liu, G. (2016). Fenofibrate Treatment of Rats With Experimental Autoimmune Myocarditis by Alleviating Treg/Th17 Disorder. Cent. J. Immunol. 41, 64–70. doi: 10.5114/ceji.2016.58817
Chu, H. X., Arumugam, T. V., Gelderblom, M., Magnus, T., Drummond, G. R., Sobey, C. G. (2014). Role of CCR2 in Inflammatory Conditions of the Central Nervous System. J. Cereb. Blood Flow Metab. 34, 1425–1429. doi: 10.1038/jcbfm.2014.120
Conductier, G., Blondeau, N., Guyon, A., Nahon, J. L., Rovère, C. (2010). The Role of Monocyte Chemoattractant Protein MCP1/CCL2 in Neuroinflammatory Diseases. J. Neuroimmunol. 224, 93–100. doi: 10.1016/j.jneuroim.2010.05.010
Cros, J., Cagnard, N., Woollard, K., Patey, N., Zhang, S. Y., Senechal, B., et al. (2010). Human CD14dim Monocytes Patrol and Sense Nucleic Acids and Viruses via TLR7 and TLR8 Receptors. Immunity 33, 375–386. doi: 10.1016/j.immuni.2010.08.012
Dai, F., Jiang, T., Bao, Y., Chen, G., Chen, L., Zhang, Q., et al. (2016). Fenofibrate Improves High-Fat Diet-Induced and Palmitate-Induced Endoplasmic Reticulum Stress and Inflammation in Skeletal Muscle. Life Sci. 157, 158–167. doi: 10.1016/j.lfs.2016.06.008
Dey, S., Mukherjee, D., Sultana, S. S., Mallick, S., Dutta, A., Ghosh, J., et al. (2020). Combination of Mycobacterium Indicus Pranii and Heat-Induced Promastigotes Cures Drug-Resistant Leishmania Infection: Critical Role of Interleukin-6-Producing Classical Dendritic Cells. Infect. Immun. 88 (6), e00222–19. doi: 10.1128/IAI.00222-19
Epelman, S., Lavine, K. J., Beaudin, A. E., Sojka, D. K., Carrero, J. A., Calderon, B., et al. (2014). Embryonic and Adult-Derived Resident Cardiac Macrophages Are Maintained Through Distinct Mechanisms at Steady State and During Inflammation. Immunity 40, 91–104. doi: 10.1016/j.immuni.2013.11.019
Fantuzzi, L., Borghi, P., Ciolli, V., Pavlakis, G., Belardelli, F., Gessani, S. (1999). Loss of CCR2 Expression and Functional Response to Monocyte Chemotactic Protein (MCP-1) During the Differentiation of Human Monocytes: Role of Secreted MCP-1 in the Regulation of the Chemotactic Response. Blood 94, 875–883. doi: 10.1182/blood.v94.3.875.415k28_875_883
Garg, M., Khanna, D., Kalra, S., Balakumar, P. (2016). Chronic Oral Administration of Low-Dose Combination of Fenofibrate and Rosuvastatin Protects the Rat Heart Against Experimentally Induced Acute Myocardial Infarction. Fundam. Clin. Pharmacol. 30, 394–405. doi: 10.1111/fcp.12204
Gómez-Olarte, S., Bolaños, N. I., Echeverry, M., Rodríguez, A. N., Cuéllar, A., Puerta, C. J., et al. (2019). Intermediate Monocytes and Cytokine Production Associated With Severe Forms of Chagas Disease. Front. Immunol. 10, 1671. doi: 10.3389/fimmu.2019.01671
Guilliams, M., Scott, C. L. (2017). Does Niche Competition Determine the Origin of Tissue-Resident Macrophages? Nat. Rev. Immunol. 17, 451–460. doi: 10.1038/nri.2017.42
Gutierrez, F. R. S., Guedes, P. M. M., Gazzinelli, R. T., Silva, J. S. (2009). The Role of Parasite Persistence in Pathogenesis of Chagas Heart Disease. Parasite Immunol. 31, 673–685. doi: 10.1111/j.1365-3024.2009.01108.x
Hardison, J. L., Kuziel, W. A., Manning, J. E., Lane, T. E. (2006). Chemokine CC Receptor 2 Is Important for Acute Control of Cardiac Parasitism But Does Not Contribute to Cardiac Inflammation After Infection With Trypanosoma Cruzi. J. Infect. Dis. 193, 1584–1588. doi: 10.1086/503812
Heidt, T., Courties, G., Dutta, P., Sager, H. B., Sebas, M., Iwamoto, Y., et al. (2014). Differential Contribution of Monocytes to Heart Macrophages in Steady-State and After Myocardial Infarction. Circ. Res. 115, 284–295. doi: 10.1161/CIRCRESAHA.115.303567
Hovsepian, E., Mirkin, G. A., Penas, F., Manzano, A., Bartrons, R., Goren, N. B. (2011). Modulation of Inflammatory Response and Parasitism by 15-Deoxy-Δ(12,14) Prostaglandin J(2) in Trypanosoma Cruzi-Infected Cardiomyocytes. Int. J. Parasitol. 41, 553–562. doi: 10.1016/j.ijpara.2010.12.002
Hulsmans, M., Sager, H. B., Roh, J. D., Valero-Muñoz, M., Houstis, N. E., Iwamoto, Y., et al. (2018). Cardiac Macrophages Promote Diastolic Dysfunction. J. Exp. Med. 215 (2), 423–440. doi: 10.1084/jem.20171274
Kruger, N. J. (1994). The Bradford Method for Protein Quantitation. Methods Mol. Biol. 32, 9–15. doi: 10.1385/0-89603-268-X:9
Krysiak, R., Gdula-Dymek, A., Okopien, B. (2011). Effect of Simvastatin and Fenofibrate on Cytokine Release and Systemic Inflammation in Type 2 Diabetes Mellitus With Mixed Dyslipidemia. Am. J. Cardiol. 107, 1010–1018.e1. doi: 10.1016/j.amjcard.2010.11.023
Lavine, K. J., Epelman, S., Uchida, K., Weber, K. J., Nichols, C. G., Schilling, J. D., et al. (2014). Distinct Macrophage Lineages Contribute to Disparate Patterns of Cardiac Recovery and Remodeling in the Neonatal and Adult Heart. Proc. Natl. Acad. Sci. U. S. A. 111, 16029–16034. doi: 10.1073/pnas.1406508111
Leid, J., Carrelha, J., Boukarabila, H., Epelman, S., Jacobsen, S. E. W., Lavine, K. J. (2016). Primitive Embryonic Macrophages Are Required for Coronary Development and Maturation. Circ. Res. 118, 1498–1511. doi: 10.1161/CIRCRESAHA.115.308270
Ling, H., Luoma, J. T. (2013). Hilleman D. A Review of Currently Available Fenofibrate and Fenofibric Acid Formulations. Rev. Cardiol. Res. 4, 47–55. doi: 10.4021/cr270w
Li, J., Tang, Z., Xie, M., Hang, C., Yu, Y., Li, C. (2020). Association Between Elevation of Plasma Biomarkers and Monocyte Dysfunction and Their Combination in Predicting Sepsis: An Observational Single-Centre Cohort Study. Innate Immun. 26, 514–527. doi: 10.1177/1753425920926602
Lockyer, P., Schisler, J. C., Patterson, C., Willis, M. S. (2010). Minireview: Won’t Get Fooled Again: The Nonmetabolic Roles of Peroxisome Proliferator-Activated Receptors (PPARs) in the Heart. Mol. Endocrinol. 24, 1111–1119. doi: 10.1210/me.2009-0374
Lopez, M., Tanowitz, H. B., Garg, N. J. (2018). Pathogenesis of Chronic Chagas Disease: Macrophages, Mitochondria, and Oxidative Stress. Curr. Clin. Microbiol. Rep. 5, 45–54. doi: 10.1007/s40588-018-0081-2
Lu, B., Rutledge, B. J., Gu, L., Fiorillo, J., Lukacs, N. W., Kunkel, S. L., et al. (1998). Abnormalities in Monocyte Recruitment and Cytokine Expression in Monocyte Chemoattractant Protein 1-Deficient Mice. J. Exp. Med. 187, 601–608. doi: 10.1084/jem.187.4.601
Machado, F. S., Martins, G. A., Aliberti, J. C., Mestriner, F. L., Cunha, F. Q., Silva, J. S. (2000). Trypanosoma Cruzi-Infected Cardiomyocytes Produce Chemokines and Cytokines That Trigger Potent Nitric Oxide-Dependent Trypanocidal Activity. Circulation 102, 3003–3008. doi: 10.1161/01.CIR.102.24.3003
McVicker, B. L., Bennett, R. G. (2017). Novel Anti-Fibrotic Therapies. Front. Pharmacol. 8, 318. doi: 10.3389/fphar.2017.00318
Medeiros, N. I., Fares, R. C. G., Franco, E. P., Sousa, G. R., Mattos, R. T., Chaves, A. T., et al. (2017). Differential Expression of Matrix Metalloproteinases 2, 9 and Cytokines by Neutrophils and Monocytes in the Clinical Forms of Chagas Disease. PloS Negl. Trop. Dis. 11, 1–16. doi: 10.1371/journal.pntd.0005284
Mitelman, J. (2011). Argentinian Society of Cardiology. Consensus Statement on Chagas-Mazza Disease. Argentinian J. Cardiol. 79, 544–564.
Nahrendorf, M. (2018). Myeloid Cell Contributions to Cardiovascular Health and Disease. Nat. Med. 24, 711–720. doi: 10.1038/s41591-018-0064-0Myeloid
Passos, S., Carvalho, L. P., Costa, R. S., Campos, T. M., Novais, F. O., Magalhães, A., et al. (2015). Intermediate Monocytes Contribute to Pathologic Immune Response in Leishmania Braziliensis Infections. J. Infect. Dis. 211, 274–282. doi: 10.1093/infdis/jiu439
Penas, F. N., Carta, D., Cevey, Á. C., Rada, M. J., Pieralisi, A. V., Ferlin, M. G., et al. (2020). Pyridinecarboxylic Acid Derivative Stimulates Pro-Angiogenic Mediators by PI3K/AKT/mTOR and Inhibits Reactive Nitrogen and Oxygen Species and NF-κb Activation Through a Pparγ-Dependent Pathway in T. Cruzi-Infected Macrophages. Front. Immunol. 10, 2955. doi: 10.3389/fimmu.2019.02955
Penas, F. N., Carta, D., Dmytrenko, G., Mirkin, G. A., Modenutti, C. P., Cevey, Á. C., et al. (2017). Treatment With a New Peroxisome Proliferator-Activated Receptor Gamma Agonist, Pyridinecarboxylic Acid Derivative, Increases Angiogenesis and Reduces Inflammatory Mediators in the Heart of Trypanosoma Cruzi-Infected Mice. Front. Immunol. 8, 1738. doi: 10.3389/fimmu.2017.01738
Penas, F. N., Cevey, Á. C., Siffo, S., Mirkin, G. A., Goren, N. B. (2016). Hepatic Injury Associated With Trypanosoma Cruzi Infection Is Attenuated by Treatment With 15-Deoxy-Δ12,14prostaglandin J2. Exp. Parasitol. 170, 100–108. doi: 10.1016/j.exppara.2016.09.015
Penas, F., Mirkin, G. A., Hovsepian, E., Cevey, Á., Caccuri, R., Sales, M. E., et al. (2013). Pparγ Ligand Treatment Inhibits Cardiac Inflammatory Mediators Induced by Infection With Different Lethality Strains of Trypanosoma Cruzi. Biochim. Biophys. Acta - Mol. Basis Dis. 1832, 239–248. doi: 10.1016/j.bbadis.2012.08.007
Penas, F., Mirkin, G. A., Vera, M., Cevey, Á. C., González, C. D., Gómez, M. I., et al. (2015). Treatment In Vitro With Pparα and Pparγ Ligands Drives M1-To-M2 Polarization of Macrophages From T. Cruzi-Infected Mice. Biochim. Biophys. Acta 1852, 893–904. doi: 10.1016/j.bbadis.2014.12.019
Pérez-Mazliah, D. E., Castro Eiro, M. D., Álvarez, M. G., Lococo, B., Bertocchi, G., César, G., et al. (2018). Distinct Monocyte Subset Phenotypes in Patients With Different Clinical Forms of Chronic Chagas Disease and Seronegative Dilated Cardiomyopathy. PloS Negl. Trop. Dis. 12, e0006887. doi: 10.1371/journal.pntd.0006887
Petray, P. B., Rottenberg, M. E., Grinstein, S., Örn, A. (1994). Release of Nitric Oxide During the Experimental Infection With Trypanosoma Cruzi. Parasite Immunol. 16, 193–199. doi: 10.1111/j.1365-3024.1994.tb00340.x
Pinto, B. F., Medeiros, N. I., Teixeira-Carvalho, A., Eloi-Santos, S. M., Fontes-Cal, T. C. M., Rocha, D. A., et al. (2018). CD86 Expression by Monocytes Influence an Immunomodulatory Profile in Asymptomatic Patients With Chronic Chagas Disease. Front. Immunol. 9, 454. doi: 10.3389/fimmu.2018.00454
Rőszer, T., Menéndez-Gutiérrez, M. P., Cedenilla, M., Ricote, M. (2013). Retinoid X Receptors in Macrophage Biology. Trends Endocrinol. Metab. 24, 460–468. doi: 10.1016/j.tem.2013.04.004
Rada, J., Donato, M., Penas, F. N., Alba Soto, C., Cevey, Á. C., Pieralisi, A. V., et al. (2020). IL-10-Dependent and -Independent Mechanisms Are Involved in the Cardiac Pathology Modulation Mediated by Fenofibrate in an Experimental Model of Chagas Heart Disease. Front. Immunol. 11, 572178. doi: 10.3389/fimmu.2020.572178
Randolph, G. J., Sanchez-Schmitz, G., Liebman, R. M., Schäkel, K. (2002). The CD16+ (Fcγriii+) Subset of Human Monocytes Preferentially Becomes Migratory Dendritic Cells in a Model Tissue Setting. J. Exp. Med. 196, 517–527. doi: 10.1084/jem.20011608
Ricote, M., Li, A. C., Willson, T. M., Kelly, C. J., Glass, C. K. (1998). The Peroxisome Proliferator-Activated Receptor-Gamma Is a Negative Regulator of Macrophage Activation. Nature 391, 79–82. doi: 10.1038/34178
Satoh, T., Nakagawa, K., Sugihara, F., Kuwahara, R., Ashihara, M., Yamane, F., et al. (2017). Identification of an Atypical Monocyte and Committed Progenitor Involved in Fibrosis. Nature 541, 96–101. doi: 10.1038/nature20611
Schmittgen, T. D., Livak, K. J. (2008). Analyzing Real-Time PCR Data by the Comparative CTmethod. Nat. Protoc. 3, 1101–1108. doi: 10.1038/nprot.2008.73
Shi, C. (2014). Monocyte Recruitment During Infection and Inflammation. Nat. Rev. Immunol. 11, 762–774. doi: 10.1038/nri3070
Sica, A., Invernizzi, P., Mantovani, A. (2014). Macrophage Plasticity and Polarization in Liver Homeostasis and Pathology. Hepatology 59, 2034–2042. doi: 10.1002/hep.26754
Souza, P. E. A., Rocha, M. O. C., Rocha-Vieira, E., Menezes, C. A. S., Chaves, A. C. L., Gollob, K. J., et al. (2004). Monocytes From Patients With Indeterminate and Cardiac Forms of Chagas’ Disease Display Distinct Phenotypic and Functional Characteristics Associated With Morbidity. Infect. Immun. 72, 5283–5291. doi: 10.1128/IAI.72.9.5283-5291.2004
Stäger, S., Maroof, A., Zubairi, S., Sanos, S. L., Kopf, M., Kaye, P. M. (2006). Distinct Roles for IL-6 and IL-12p40 in Mediating Protection Against Leishmania Donovani and the Expansion of IL-10+ CD4+ T Cells. Eur. J. Immunol. 36, 1764–1771. doi: 10.1002/eji.200635937
Sugga, G. S., Khanam, R., Khan, M. U., Khanam, R. (2012). Protective Role of Fibrates in Cardiac Ischemia/Reperfusion. J. Adv. Pharm. Technol. Res. 3, 188. doi: 10.4103/2231-4040.101016
Tanowitz, H. B., Machado, F. S., Spray, D. C., Friedman, J. M., Weiss, O. S., Lora, J. N., et al. (2015). Developments in the Management of Chagas Cardiomyopathy. Expert Rev. Cardiovasc. Ther. 9072, 1–17. doi: 10.1586/14779072.2015.1103648
Teixeira, A. R. L., Hecht, M. M., Guimaro, M. C., Sousa AO, N. N. (2011). Pathogenesis of Chagas’ Disease: Parasite Persistence and Autoimmunity. Clin. Microbiol. Rev. 24, 592–630. doi: 10.1128/CMR.00063-10
Tontonoz, P., Nagy, L., Alvarez, J. G., Thomazy, V. A., Evans, R. M. (1998). Pparγ Promotes Monocyte/Macrophage Differentiation and Uptake of Oxidized LDL. Cell 93, 241–252. doi: 10.1016/S0092-8674(00)81575-5
Trachtenberg, B. H., Hare, J. M. (2017). Inflammatory Cardiomyopathic Syndromes. Circ. Res. 121, 803–818. doi: 10.1161/CIRCRESAHA.117.310221
Volpe, S., Cameroni, E., Moepps, B., Thelen, S., Apuzzo, T., Thelen, M. (2012). CCR2 Acts as Scavenger for CCL2 During Monocyte Chemotaxis. PloS One 7, 1–10. doi: 10.1371/journal.pone.0037208
Wesley, M., Moraes, A., Rosa A de, C., Lott Carvalho, J., Shiroma, T., Vital, T., et al. (2019). Correlation of Parasite Burden, kDNA Integration, Autoreactive Antibodies, and Cytokine Pattern in the Pathophysiology of Chagas Disease. Front. Microbiol. 10, 1856. doi: 10.3389/fmicb.2019.01856
Wong, K. L., Tai, J. J. Y., Wong, W. C., Han, H., Sem, X., Yeap, W. H., et al. (2011). Gene Expression Profiling Reveals the Defining Features of the Classical, Intermediate,and Nonclassical Human Monocyte Subsets. Blood 118(5), e16-31. doi: 10.1182/blood-2010-12-326355
Wong, K. L., Yeap, W. H., Tai, J. J. Y., Ong, S. M., Dang, T. M., Wong, S. C. (2012). The Three Human Monocyte Subsets: Implications for Health and Disease. Immunol. Res. 53, 41–57. doi: 10.1007/s12026-012-8297-3
Yin, W.-H., Chen, J.-W., Chen, Y.-H., Lin, S.-J. (2013). Fenofibrate Modulates HO-1 and Ameliorates Endothelial Expression of Cell Adhesion Molecules in Systolic Heart Failure. Acta Cardiol. Sin. 29 (3), 251–260.
Zhang, J., Cheng, Y., Gu, J., Wang, S., Zhou, S., Wang, Y., et al. (2016). Fenofibrate Increases Cardiac Autophagy via FGF21/SIRT1 and Prevents Fibrosis and Inflammation in the Hearts of Type 1 Diabetic Mice. Clin. Sci. 130 (8), 625–641. doi: 10.1042/CS20150623
Zhang, L., Tarleton, R. L. (1999). Parasite Persistence Correlates With Disease Severity and Localization in Chronic Chagas’ Disease. J. Infect. Dis. 180, 480–486. doi: 10.1086/314889
Zhao, B. N., Campbell, J. J., Salanga, C. L., Ertl, L. S., Wang, Y., Yau, S., et al. (2019). CCR2-Mediated Uptake of Constitutively Produced CCL2: A Mechanism for Regulating Chemokine Levels in the Blood. J. Immunol. 203, 3157–3165. doi: 10.4049/jimmunol.1900961
Keywords: fenofibrate, chronic Chagas disease, inflammation, monocyte subsets, cytokine
Citation: Pieralisi AV, Cevey ÁC, Penas FN, Prado N, Mori A, Gili M, Mirkin GA, Gagliardi J and Goren NB (2022) Fenofibrate Increases the Population of Non-Classical Monocytes in Asymptomatic Chagas Disease Patients and Modulates Inflammatory Cytokines in PBMC. Front. Cell. Infect. Microbiol. 11:785166. doi: 10.3389/fcimb.2021.785166
Received: 28 September 2021; Accepted: 10 December 2021;
Published: 11 March 2022.
Edited by:
Vilma G Duschak, Consejo Nacional de Investigaciones Científicas y Técnicas (CONICET), ArgentinaReviewed by:
Santiago Partida Sanchez, Nationwide Children’s Hospital, United StatesChiranjib Pal, West Bengal State University, India
Copyright © 2022 Pieralisi, Cevey, Penas, Prado, Mori, Gili, Mirkin, Gagliardi and Goren. This is an open-access article distributed under the terms of the Creative Commons Attribution License (CC BY). The use, distribution or reproduction in other forums is permitted, provided the original author(s) and the copyright owner(s) are credited and that the original publication in this journal is cited, in accordance with accepted academic practice. No use, distribution or reproduction is permitted which does not comply with these terms.
*Correspondence: Nora B. Goren, bmdvcmVuQGZtZWQudWJhLmFy
†These authors have contributed equally to this work