- 1Centre for Children’s Health Research, School of Biomedical Sciences, Faculty of Health, The Queensland University of Technology, Brisbane, QLD, Australia
- 2Nindooinbah Pastoral Company, Nindooinbah, QLD, Australia
Heavy tick burden on beef cattle account for huge economic losses globally, with an estimated value of US$22-30 billion per annum. In Australia, ticks cost the northern beef industry approximately A$170-200 million. Methods to evaluate and predict tick resistance would therefore be of great value to the global cattle trade. Exosomes (EX) are small extracellular vesicles (EVs) of ~30-150nm diameter and have gained popularity for their diagnostic and prognostic potential. EX contain, among other biomolecules, various types of RNA including micro-RNA (miRNA) and long noncoding RNA (lncRNA). MiRNA specifically have been validated as therapeutic biomarkers as they perform regulatory functions at the post-transcriptional level and are differentially expressed between divergent groups. The objective of the present study was to evaluate the miRNA profiles of EV and fractionated exosomal samples of high and low tick-resistant beef cattle to highlight potential miRNA biomarkers of tick resistance. Cows (n = 3/group) were classified into high or low tick resistant groups according to a novel scoring system. EVs and EX were isolated and fractionated from the blood plasma of high and low tick resistant cattle using established isolation and enrichment protocols. The resultant EX and non-EX samples were processed for next generation miRNA sequencing. Offspring of the cows in each high and low tick resistant group underwent the same processing for blood plasma EX, non-EX and miRNA analysis to evaluate the heritability of miRNA associated with tick resistance. A total of 2631 miRNAs were identified in EX and non-EX fractionated samples from high and low tick-resistant beef cattle. MiR-449a was highly expressed in maternal high tick-resistant EX samples. Of these, 174 were novel miRNAs, and 10 were differentially expressed (DE) (FDR < 0.05). These 10 DE miRNAs were also present in EVs, and three miRNAs were highly expressed: miR-2419-3p, miR-7861-3p and miR-2372-5p. Although 196 novel miRNAs were identified in fractionated samples of offspring, no miRNA were differentially expressed in these animals.
1 Introduction
Ticks pose a considerable threat to livestock globally, specifically in beef cattle farming. It has been estimated that 80% of the world’s cattle are at risk, with US$20 to US$30 billion economic losses per annum link to tick and tick-borne diseases (Lew-Tabor and Rodriguez Valle, 2016; Burrow et al., 2019). In Australia, the tick burden costs the northern beef industry around A$170-200 million (Playford et al., 2005). Tick infestation causes stress and weakens the immune system, which affects the performance of the beef cow (Mapholi et al., 2014). Each engorging female tick is responsible for an average 1.37 ± 0.25 g bodyweight loss in Bos taurus cattle (Jonsson, 2006). Tick-borne diseases increase cattle mortality, chronic morbidity, and treatment costs (Jonsson et al., 2008; Hurtado and Giraldo-Ríos, 2018). Farm management systems use conventional options such as acaricides to control tick infestation, however this is not a sustainable strategy in the long term (Mapholi et al., 2014). Intensive usage of acaricides causes pressure on pasture systems and leads to selection for acaricide-resistant tick populations (Kasaija et al., 2021). Lack of understanding on the whole genome of parasites and antigenic variation challenge sustainable use of parasite vaccines against ticks (Barré et al., 2011; Lew-Tabor and Rodriguez Valle, 2016). Farmers utilize different grazing management techniques such as pasture rotation and pasture burning to reduce exposure and control tick populations (Kasaija et al., 2021). Unfortunately, climactic conditions desirable for cattle herds are also ideal for tick propagation, and grazing herds are more susceptible to heavy tick burden (Young et al., 1988; Kasaija et al., 2021). Cattle-ticks represent a top priority endemic disease for the red meat industry in Australia (Lane et al., 2015).
Tick burden affects not only cattle but is also linked to human diseases. For instance, a glycoprotein from tick saliva, α-Gal, causes an allergic condition termed α-Gal syndrome in humans, resulting in delayed hypersensitivity to consumed red meat products (Cabezas-Cruz et al., 2019). Interestingly, some cattle exhibit a natural resistance to ticks and carry a low tick burden (Burrow et al., 2019). The study of the physiological or genetic mechanisms that confer this natural resistance offers an opportunity to identify alternative and more effective tick control methodologies (Marima et al., 2020). The level of tick resistance varies among different cow breeds (Marufu et al., 2011; Marima et al., 2020). The cattle tick resistance is considered as a polygenic trait which includes morphological, physiological and behavioural traits, and, therefore heritability plays a main role (Mapholi et al., 2014). These factors suggest genetic selection is a considerable option towards the development of a sustainable cattle tick control methodology.
Extracellular vesicles (EV) are a heterogenous group of nanoparticles that originate from the endosomal sorting complex required for transport (ESCRT) pathway, or shed directly from the plasma membrane (van Niel et al., 2018). EVs are classified into subpopulations by their origin, size, morphology, and protein markers specific to each subtype. Exosomes (EX) are an EV subtype of diameter ~30 – 150 nm and carry unique molecular cargo that has been used in biomarker development and targeted therapeutics (Zhang et al., 2019; Abeysinghe et al., 2020; Dai et al., 2020; Kugeratski et al., 2021). Exosomal cargo contains complex functional molecules ranging from proteins (Crookenden et al., 2016), lipids (Record et al., 2014), mRNAs (Valadi et al., 2007) and miRNAs (Valadi et al., 2007). Differential expression of exosomal miRNA have been the focus of numerous studies involving divergent groups (Colitti et al., 2019; Ma et al., 2019; Wang et al., 2019). EX are intercellular communicators (Zhang et al., 2019) and are associated with major cellular processes like signal transduction (Gangoda et al., 2015), immune responses (Greening et al., 2015) and antigen presentation (Mittelbrunn et al., 2011). EX can be transferred from mother to fetus via the placenta, which supports the idea that EX are important for maternal-fetal communication (Sheller-Miller et al., 2016).
While a previous study identified single nucleotide polymorphisms and chromosome segments associated with tick burden, to date there have been no biomarkers or genetic variants identified to account for tick resistance (Porto Neto et al., 2011).
In this study, high and low tick resistant beef cattle were classified according to a novel tick scoring system. Next-generation miRNA sequencing was carried out on plasma-derived EX and non-EX particles from high and low tick cattle to evaluate their miRNA profiles and assess differential expression of miRNA.
2 Materials and Methods
2.1 Animals, Management and Blood Collection
The animals, management, and sample collections were approved by the Animal Welfare Unit, UQ Research and Innovation, the University of Queensland (UQCCR/459/16). A total of 199 animals were selected randomly and tick scores were given accordingly (Table 1).
The animals were carefully examined for evidence of tick infestation as part of a thorough physical exam. Animals were hand checked for the presence and absence of ticks on their hind regions and belly over a three-month period. A scoring system was developed (1-5, A or B), (1) no identifiable tick burden, (2) < 10 ticks, (3) 20 to 100 ticks, (4) 100 to 200 ticks, (5) > 200 ticks with (A) representing crusting and (B) no crusting (Figure 1). Animals with a score of >3 were treated as part of the commercial program and those with <3 untreated.
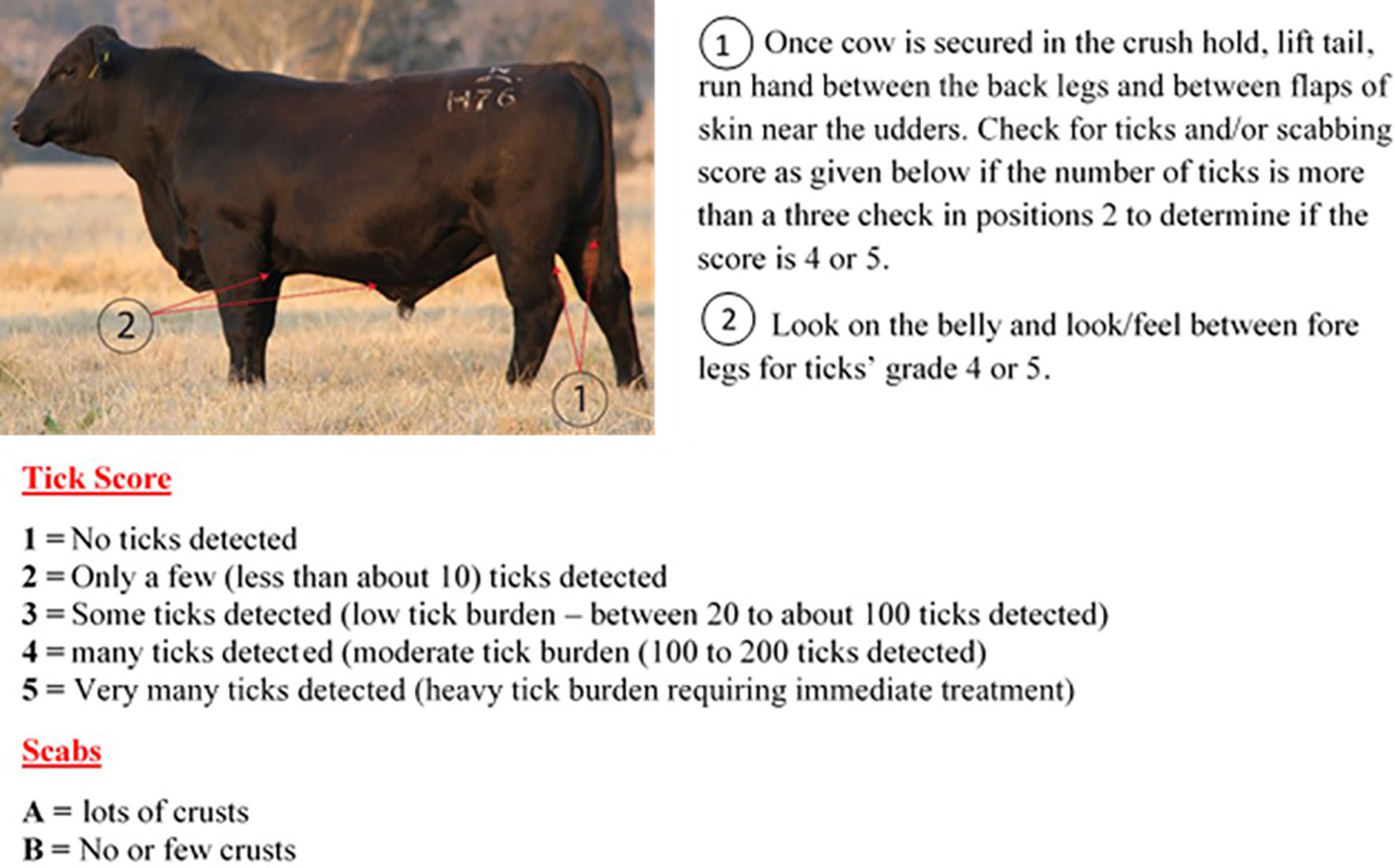
Figure 1 Cow tick scoring methodology based on the number of ticks and scabs detected in the body of the cow.
Blood was collected from mother/sire and offspring in EDTA vacutainer tubes and plasma separated by centrifugation at 3000 x rcf for 10 min at 4°C. Plasma was stored at -80°C until required for EV/EX isolation. Detailed information of sire and dam histories and other relevant information (e.g. last tick treatment, weight, pasture location) was recorded as part of the commercial program.
From this larger animal group, 3 high tick-resistant (1B) and 3 low tick-resistant (5A) mother/sire and offspring (highest and lowest tick burden animals) blood plasma were used for EV and EX isolation.
2.2 Extracellular Vesicle (EV) Isolation
EVs were isolated from the blood plasma of animals using as established sequential centrifugation protocol (Koh et al., 2018). Briefly, plasma was centrifuged at 2,000 x rcf for 30 min at 4°C and 12,000 x rcf for 30 min at 4°C to remove cellular debris and apoptotic bodies. It was then filtered through a 0.22-μm polyether sulfone membrane filter (Corning Inc., Corning, NY) and then ultracentrifuged at 100,000 x rcf for 2 hr at 4°C. Finally, the pellets containing the EVs were resuspended in 500 μl Dulbecco’s Phosphate Buffered Saline (DPBS, pH 7.0 – 7.2; Gibco, Life Technologies Australia Pty Ltd) and stored at -80°C for further analysis.
2.3 EX Isolation and Characterization
2.3.1 Size Exclusion Chromatography (SEC)
EX were isolated from plasma by ultracentrifugation and size exclusion chromatography (SEC) as previously described (Koh et al., 2018). The high and low tick resistant samples from mother/sire and offspring were pooled separately. Briefly, 500 µL EV suspensions resulting from sequential centrifugation were fractionated using qEV original size exclusion columns (Izon Science, New Zealand). Individual 500 µL fractions were eluted from the column and collected in separate 1.5 mL microcentrifuge tubes (a total of 16 fractions), as per manufacturer’s instructions. The fractions were collected as follow s; 1 – 6 as void volume and particles >200 nm, 7 – 10 as exosomal (EX) fractions (particles <200 nm), and 11 – 16 as soluble proteins (non-EX) fractions. One column was used per animal groups to maintain group heterogeneity. In between uses, the columns were flushed with 0.5 mL 1M NaOH solution, followed by 15-20 mL filtered DPBS.
Three EX and non-EX samples from each of extreme high (1b) and low (5a) tick resistant groups were pooled for SEC miRNA analysis.
Quantification of protein concentration of SEC fractions was evaluated using Bicinchoninic Acid (BCA) assay (Sigma-Aldrich, St Louis, MO, USA) and bovine serum albumin (Sigma-Aldrich, St Louis, MO, USA) dilutions were used as standards. The size distribution by Nanoparticle tracking analysis was conducted as previously described (Koh et al., 2018).
2.4 Exosomal miRNA Isolation
EX fraction 7-10 (EX) and 11-16 (Non-EX) pooled and samples were incubated with TRIzol at room temperature for 5 minutes (2.5 volume of sample: 7.5 volume of TRIzol). Chloroform was added (2.5 volume of sample: 1.5 volume of Chloroform) and centrifuged for 15 min at 4°C, at 12,000 rcf. The upper aqueous layer was carefully transferred to a new microcentrifuge tube and 1.5 volumes of 100% ethanol was added. This mixture was passed through a miRNeasy mini column (miRNeasy mini kit, 217004, QIAGEN) and miRNA was isolated according to the manufacturer’s protocol.
2.5 MiRNA
2.5.1 Sequencing and Data Analysis
Isolated miRNA samples were sent to the Australian Genome Research Facility (AGRF) for next generation sequencing. NEBNext® Multiplex Small RNA Library Prep Set for Illumina® was used for library preparation and Novaseq S1 platform was used for single end 100bp sequencing. A quality control was measured for each sample and samples greater than 78.19% bases above Q30 were selected. The reads were also screened for the presence of any Illumina adapter/overrepresented sequences (adapter sequence as per the library preparation kit: AGATCGGAAGAGCACACGTCTGAACTCCAGTCAC) and cross-species contamination. Reads were trimmed and length filtered using Trim Galore! to be between 14 and 38 base pairs long. The cleaned sequence reads were then aligned against the Bos taurus genome (Build version UMD3.1). The STAR aligner (v2.5.3a) was used to map reads to the genomic sequences (https://github.com/alexdobin/STAR/blob/master/doc/STARmanual.pdf) and alignment files were in BAM format. The counts of reads mapping to each known miRNA were identified using unitas (https://sourceforge.net/projects/unitas/). The differential gene expression was performed using edgeR (version 3.30.3) of R package 4.0.3 (https://bioconductor.org/packages/release/bioc/html/edgeR.html). False discovery rate (FDR) analysis was performed to correct for multiple hypothesis testing and set to 0.05 (FDR<0.05). Only miRNA meeting the FDR cut-off were considered statistically significant. Further, miRNAs meeting the FDR cut-off were filtered with log fold change (logFC), in which the upregulated DE miRNAs were considered if the logFC ≥ 1.5 and logFC ≤ −1.5 for downregulated DE miRNAs.
For volcano plots, cut offs were -log10(FDR) ≤ 1.3 and fold change threshold values were −1.5 ≤logFC ≥ 1.5.
MiRDeep2 was used to identify novel miRNAs in each sample https://www.mdc-berlin.de/n-rajewsky#t-data,software&resources (Friedländer et al., 2012). (Reference genome: UMD3.1; Hairpin: Bos taurus (Btau_5.0.1) hairpin obtained miRbase; Mature: Bos taurus (Btau_5.0.1) mature miRNA obtained from miRbase; Other mature: mature miRNA from related organisms, i.e. Capra hircus (CHIR_2.0) and Ovis aries (Oar_v4.0); UCSC browser species: Cow).
2.5.2 Pathway Analysis
Four different miRNA target prediction tools (1. miRanda from miRNet version 2.0 (https://www.mirnet.ca/) (Fan et al., 2016); 2. miRmap (http://mirmap.ezlab.org.) (Vejnar et al., 2013); 3. TargetScanHuman 8.0 (http://www.targetscan.org/vert_80/) (Agarwal et al., 2015); 4. miRWalk (http://mirwalk.umm.uni-heidelberg.de/search_mirnas/) were used to identify multiple target binding sites of the 10 differentially expressed Mother SEC (EX and non-EX) miRNAs.
In miRNet target prediction, the Bos taurus was used as organism, miRBase ID as ID type and target type was miRanda genes. For Degree filter “All network nodes” were selected with a cut off value of greater than 3, greater than 100 cutoff was used for betweenness, and all networks were to connect with the shortest path (Herrnreiter et al., 2021).
Bos taurus was used as the organism for miRmap and 10 DE miRNAs were checked for targets individually. Targets which were above 95% of power exact was chosen as confident miRNA targets. All the individual miRNA targets were pooled together to make a total list of miRmap targets of 10 DE miRNAs (Vejnar et al., 2013).
In TargetScanHuman 8.0, individual miRNAs were used as inputs and only broadly conserved sites were identified as confident miRNAs (poorly conserved sites and other miRBased annotations were not included due to potential false positive targets). Next the gene target results were lifted from Human GRCh38/hg38 to ARS-UCD 1.2 bosTau9 genome using the UCSC table browser – Lift Genome Annotation feature (https://genome.ucsc.edu/cgi-bin/hgLiftOver). For this > 0.1 ratio of conserved bases used as the minimum ratio (Scheel et al., 2017). Next, the individual miRNA targets were pooled together to make a total list of miRNA targets.
MiRWalk, putative target genes of 10 DE miRNAs were predicted with a cutoff binding probability > 0.98 (Li et al., 2021).
Intersection of target genes from four miRNA target prediction tools were used as the confident target sites of differentially expressed miRNAs from Mother SEC samples (EX and non-EX samples). Genes shared with at least 2 target prediction tools were selected as the confident target genes.
The search tool for retrieval of interacting genes (STRING) (https://string-db.org) database was utilized for generation of protein-protein interaction (PPI) network for the intersected target genes (Szklarczyk et al., 2019). Using the STRING database, genes with a score ≥ 0.4 (Karimizadeh et al., 2019; Li et al., 2020) and species “Bos taurus” were chosen to build a network model visualized by Cytoscape (v3.9.0) (Shannon et al., 2003). Protein subcellular localization was visualized using compartments feature in StringDB (Binder et al., 2014).
3 Results
3.1 Nanoparticle Tracking Analysis (NTA)
Individual EX and non-EX fractions were assessed for their particle concentration (Figure 2). EX fractions 7 – 10 show the highest particle concentrations in fractions 8 – 10 (~4 x 1010 – 8 x 1010 particles/mL). Non-EX fractions 11 – 16 generally had a particle concentration < 2 x 1010 particles/mL.
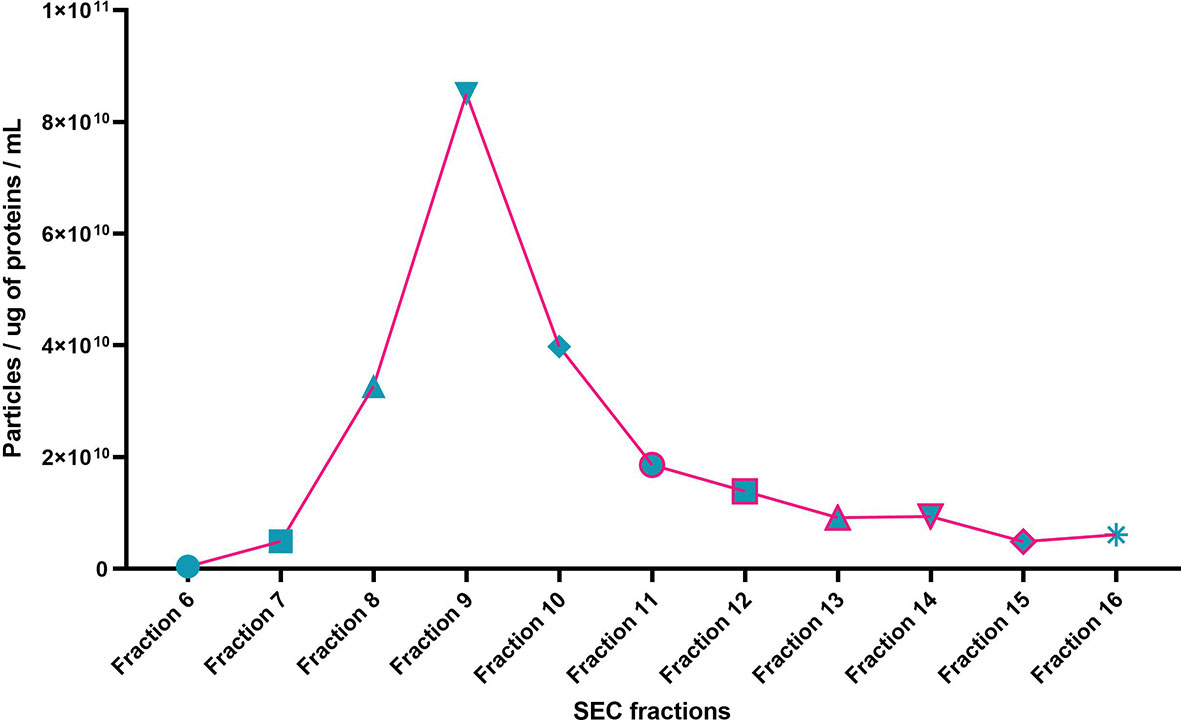
Figure 2 Particle size distribution per total protein for SEC fractions 6 – 16 of a representative sample (NTA particles per mL / total protein (µg/mL). Particle per ug of protein per mL.
3.2 Quality Assessment for Exosomal miRNA Samples From High and Low Tick Resistant Beef Cow Blood Plasma
All the samples yielded more than 100pg (0.1 ng) of miRNA according to quality control testing (Table 2).
3.3 miRNA Expression Profiles
3.3.1 SEC (EX and Non-EX) miRNA Profiles- Mother/Sire – High vs. Low Tick Resistant
2632 miRNAs were identified in high and low tick resistant cattle. 2458 miRNAs were identified in miRbase database, and 174 novel miRNAs were identified by miRDeep2. The total list of miRNAs including the novel miRNAs are attached in Supplementary file 1 (Abeysinghe, 2021). Figure 3A shows DE miRNA between high and low tick-resistant cattle, of which there were 10 DE miRNA in total. Specifically, mir-449a-5p, miR-2285-4-3p, miR-12000-5p were more highly expressed in high tick-resistant cattle, and miR-3578-5p, miR-2323-3p were more highly expressed in low tick resistant cattle (EX and non-EX fraction). In non-EX samples, low tick-resistant show a higher expression level of miR-188-3p and miR-3578-5p compared to high tick resistant. Most of the novel miRNAs were common to both groups, however 9 and 7 novel miRNAs were unique to high-tick resistant and low-tick resistant EX samples, respectively (Figure 3B). The sequence fragment with the highest read counts from the precursor miRNA loop was considered as the novel mature miRNA (Figure 3C). The total list of gene targets predicted by miRWalk, miRanda, TargetScan and miRmap are shown in Supplementary file 2 (Abeysinghe, 2021). There were 37 shared genes which are shared with at least 2 target prediction [Supplementary file 3 (Abeysinghe, 2021)]. Out of those 37 target genes, 16 genes were appeared in protein subcellular localization visualized by compartment analysis of stringDB (Table 3) and the protein-protein interaction (PPI) networks are shown in Figure 3D.
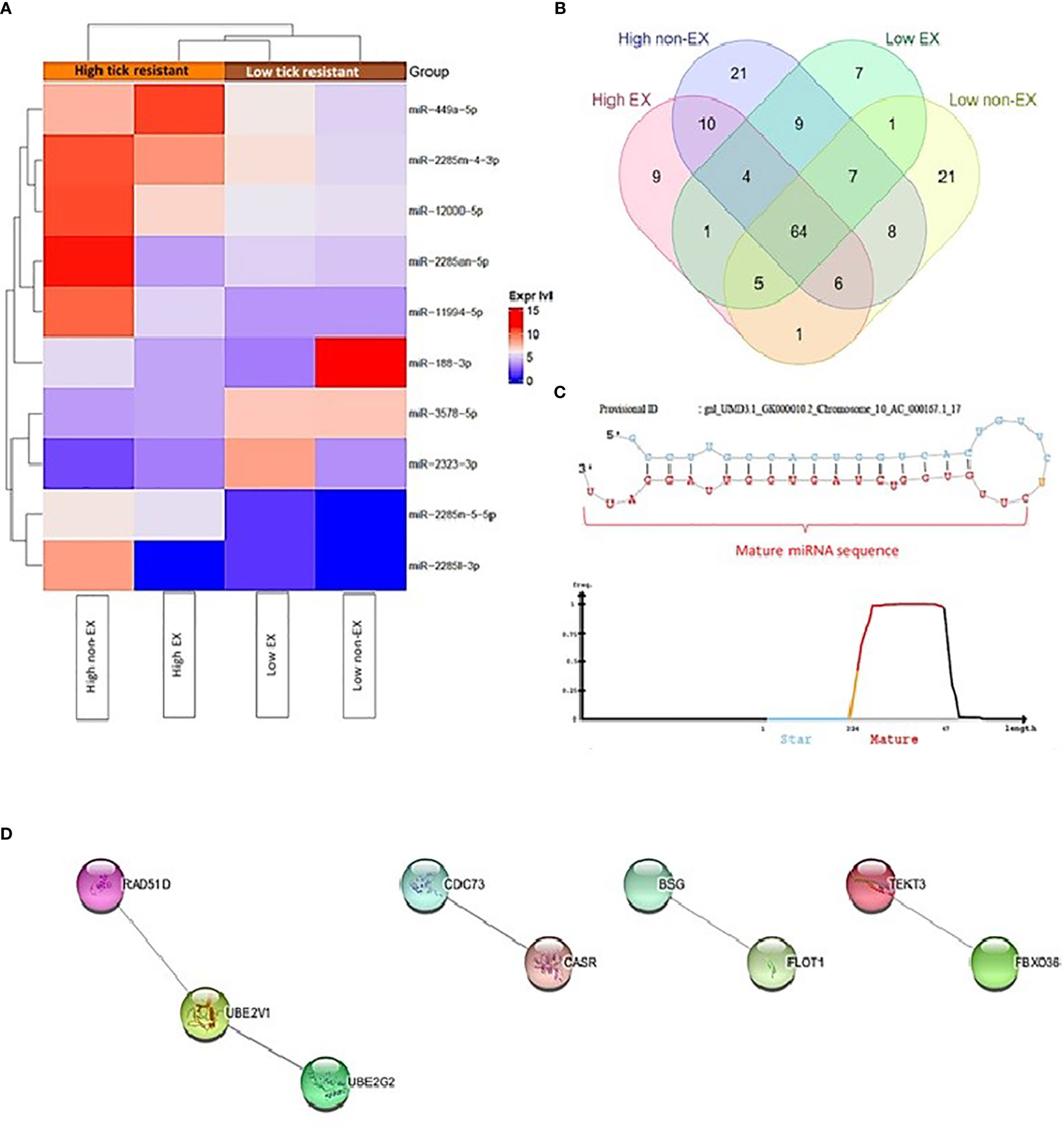
Figure 3 (A) Heatmap of differential expression of miRNAs between high and low tick resistant mother plasma SEC samples (EX and non-EX) (B) Novel miRNAs distributed among high and low tick resistant mother plasma SEC samples (EX and non-EX) (C) An exemplary novel miRNA loop which shows the predicted mature miRNA sequence (D) Protein-Protein interaction (PPI) networks generated by StringDB for the 37 gene targets predicted by miRmapper, TargetScan, miRmapper & miRWalk (Singletons are not included on the figure).
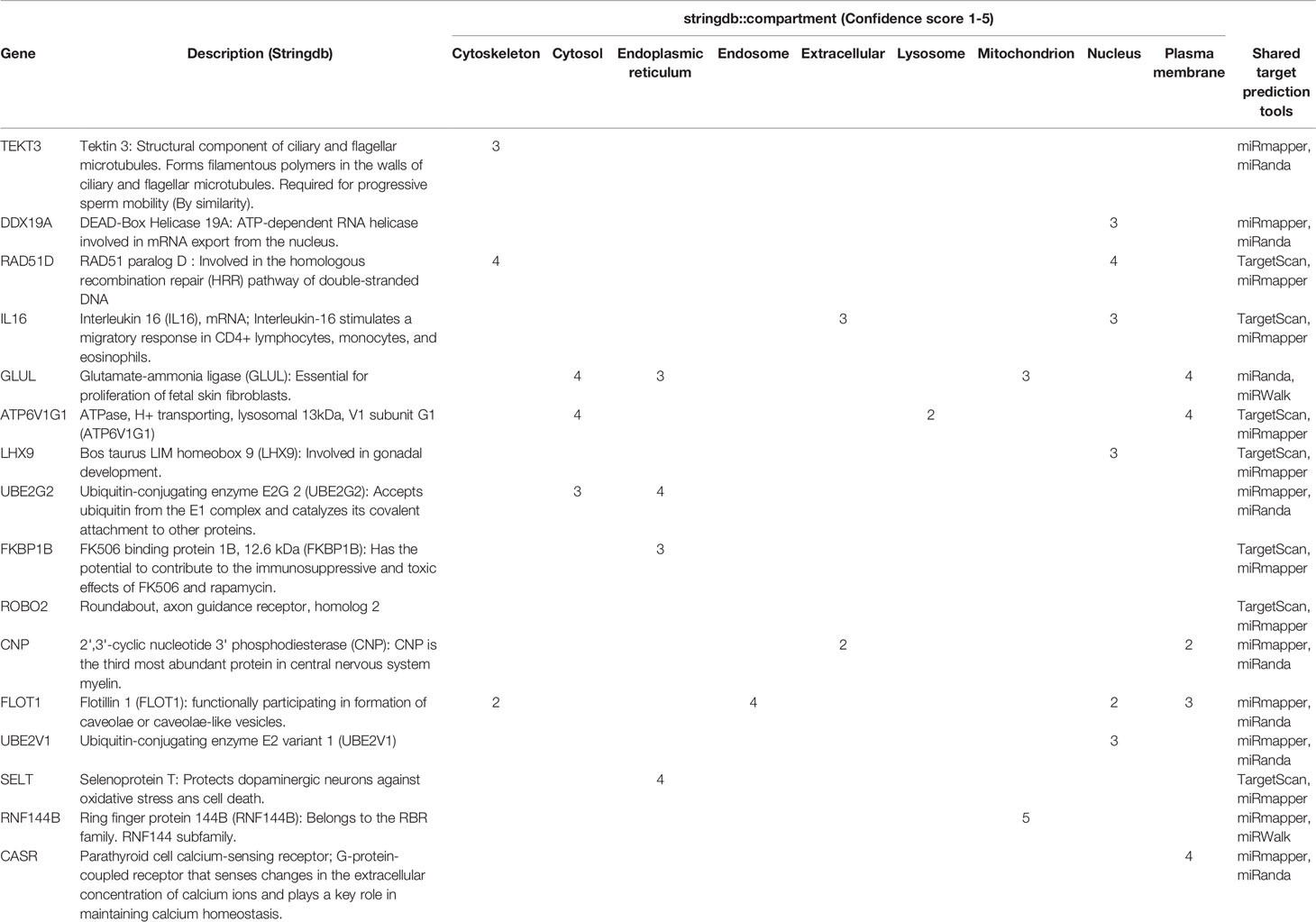
Table 3 Target genes of Mother SEC DE miRNAs intersected by four target prediction tools and the 16 target gene interactions generated by compartment analysis in StringDB.
3.4 EV miRNA Profiles: High vs. Low Tick-Resistant (Mother/Sire)
EV samples from high and low tick-resistant mother plasma samples generated 2808 miRNAs. The miRDeep2 identified 350 novel miRNAs from the sequence data, in which more than 70% were shared novel miRNAs between high and low tick-resistant plasma EV samples. Only 44 novel miRNAs expressed in high tick resistant mother plasma EV samples compared to 59 novel miRNAs identified in low tick-resistant mother plasma EV population. The total expressed miRNA list is in Supplementary file 4 (Abeysinghe, 2021).
The 10 differentially expressed miRNAs from the mother exosomes were included in the list as shown inTable 4. The top DE miRNAs from mother exosomes, miR-449a-5p, miR-2285-4-3p and miR-12000-5p are highly expressed in high tick resistant mother EVs than low tick-resistant mother EV population in which the pattern is similar as in Figure 3A.
The top three miRNAs which were above the threshold (FDR < 0.05) from EdgeR DGE analysis are shown in Figure 4 below. The average miRNA count of miR-2419-3p, miR-7861-3p and miR-2372-5p were higher in high tick resistant compared to low tick resistant mother EV population.
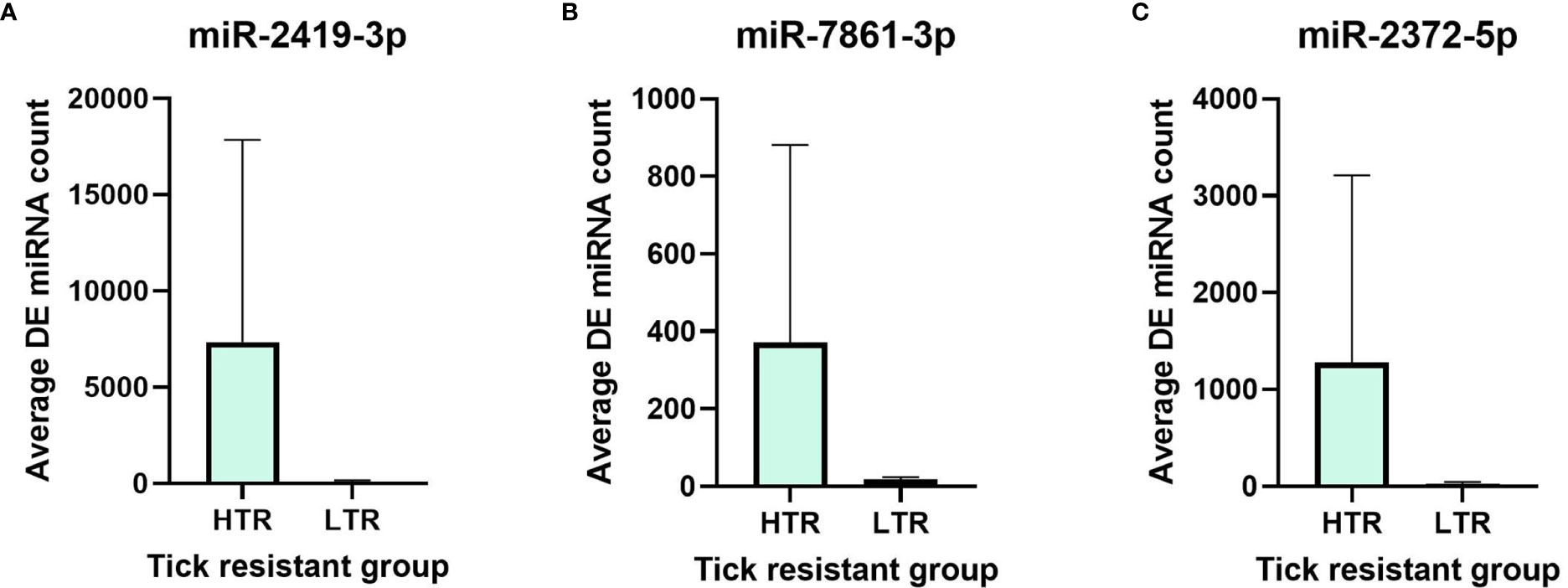
Figure 4 Differential expression of top 3 DE miRNAs (A) miR-2419-3p, (B) miR-7861-3p and (C) miR-2372-5p between high tick resistance (HTR) and low tick resistance (LTR) mother EV samples.
3.5 EX and Non-EX miRNA Profiles: Offspring of High and Low Tick-Resistant Mother/Sire
A total of 196 novel miRNAs were identified in the offspring of high and low-tick resistant mothers/sires (Figure 5A). Most miRNAs were common to both groups (Figure 3A). Out of 2254 DE miRNAs between offspring of high and low tick-resistant mothers/sires, none were below the FDR cut-off in both EX and non-EX samples (Figure 5B). However, the 10 DE miRNAs from mother exosome samples were present in the DE offspring miRNA list. The total list of miRNAs is provided in Supplementary file 5 (Abeysinghe, 2021).
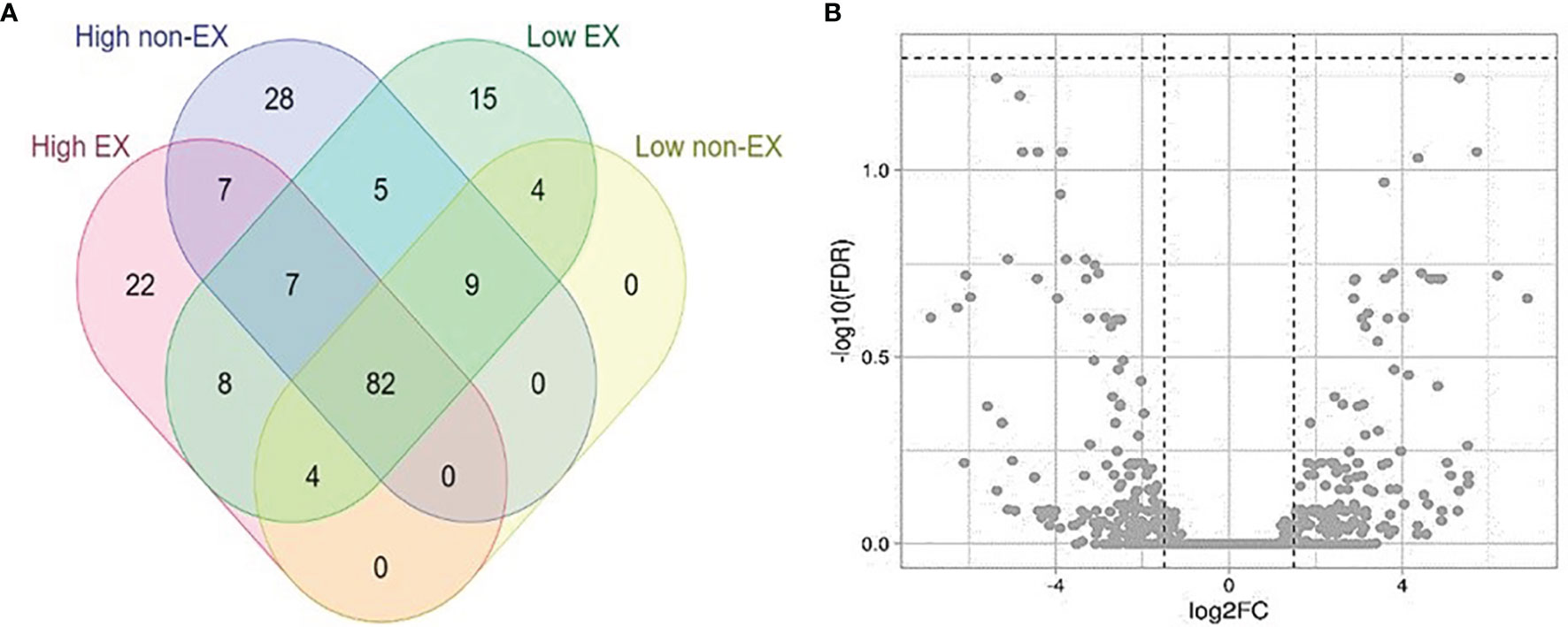
Figure 5 (A) Novel miRNAs distributed among high and low tick resistant offspring plasma SEC samples (EX and non-EX). (B) Volcano plot of differentially expressed miRNAs in high and low tick resistant offspring SEC samples. The cut off values : [Y axis -log10(FDR) cut off is 1.3 (FDR < 0.05); X axis −1.5 ≤logFC ≥ 1.5].
4 Discussion
This is the first study to evaluate miRNA profiles of high and low tick-resistant cattle. Inflammation due to tick infestation may disrupt bovine immune system function and thus facilitate differential expression of miRNAs in low tick-resistant cows. It is interesting to note that differential expression of miRNA is observed at the end stage after tick burden has been established, while DE miRNAs are conserved in the offspring their expression levels are not significantly altered. Further studies may sample cows at an earlier timepoint prior to tick exposure in order to assess any biological vulnerabilities which may point to a predisposition to low tick-resistance.
4.1 Differential Expression of miRNA in High and Low Tick-Resistant Cattle
4.1.1 Mir-449a
Mir-449a was highly expressed in maternal high tick-resistant EX. It is commonly associated with cell death, cell-cycle arrest and differentiation, but has also been implicated in many other biological pathways (Lizé et al., 2011). For example, mir-449a acts as a tumor suppressor by inhibiting inflammation and tumor metastasis (Vejnar et al., 2013; Fan et al., 2016). A recent study established that miR-449a is DE in beef cattle with divergent feed efficiency phenotypes (Mukiibi et al., 2020). Finally, in a bovine endometrial receptivity study involving both in vivo (IVV) and in vitro (IVT) produced embryos, miR-449a was found to be DE based on whether embryos were IVV or IVT derived (Ponsuksili et al., 2014). As such, mir-449a may be considered as a kind of master regulator of a diverse array of biological processes.
4.1.2 Altered Signaling Pathways Are Associated With Inflammation and the Cell Cycle
The 10 DE miRNAs in the current study regulate inflammatory-related pathways and suggest that perturbations to these pathways are related to tick-resistance. For example, the NFķβ signaling pathway and chemokine signaling pathway are both inflammatory pathways that are affected by novel and differentially expressed miRNA in this study. Additionally, the Ras, PI3KT-Akt, and pathways related to cancer are interconnected pathways and known to be related to cell proliferation and differentiation (Downward, 2003; Hemmings and Restuccia, 2012). Therefore, it is possible that low tick resistance is linked to dysregulation of the immune system and pathways related to normal cell cycle function, leading to the inability of the host cattle to reject tick infiltration.
4.1.3 Plasma EV and EX Exhibit Unique Differentially Expressed miRNA Profiles
The 10 DE miRNAs determined in maternal EX samples were also found in maternal EV samples. However, these 10 DE miRNAs were not among the top DE miRNAs in the maternal EV samples, which supports the idea that EX represent a subpopulation of EVs. Larger vesicles, for example microvesicles (diameter >200 nm) may indeed carry more genetic material, including miRNA. Additionally, the 100,000 rcf UC pellet may contain circulating miRNA and other RNA fragments. A recent study illustrates the presence of higher number of EX proteins using the UC+SEC isolation and enrichment strategy for blood plasma EX (Turner et al., 2021). UC followed by SEC of plasma samples may therefore contain uniquely sorted exosomal cargo and provides a better cross-section of genetic materials including miRNA. Differential miRNA expression in EX and non-EX samples suggest the involvement of miRNA in the regulation of many different biological functions at both the cellular and systemic level (Zhang et al., 2019; Abeysinghe et al., 2020).
Highly expressed miRNAs from maternal EV populations relates to key bovine traits of interests. An Irish study has revealed polymorphisms in bovine miR-2419 modify its binding properties to target genes related to milk production (Braud, 2017). A recent multi-omics analysis shows that miR-2419-3p is associated with muscle fatty acid traits of Nelore cattle, which may exhibit regulatory function at mRNA or protein level (Cardoso et al., 2021). In a previous study, miR-7861 was differentially expressed as a unique miRNA in Bovine serum compared to EX (Zhao et al., 2016).
4.1.4 Differential Expression of EX miRNA Is Not Heritable
The lack of miRNA differential expression in offspring plasma EX could be related to environmental factors, fewer exposure to ticks or calf immune system immaturity rather than being a genetic trait (Yu et al., 2018). A low correlation of circulating plasma miRNA transcript level between human mother-child duos has been observed in a recent study (Dypås et al., 2020). Future studies may perform sequential sample of the same cattle throughout their lifetime, to provide a time course of the changes occurring at a physiological level that leads to high or low tick-resistance.
This is the first exploratory study on EV and EX vs non-EX miRNA expression profiles of cows and their offspring based on a novel tick scoring system. As a step further, proteomic studies are currently ongoing to ascertain the plasma EX protein expression profiles between the high and low-tick resistant cattle using the same animals used in this study (unpublished data). Further studies expanding the animal number may validate and follow on from the basis of this work to identify the potential role of miRNA as biomarkers or regulators of tick resistance in cattle.
Data Availability Statement
The datasets presented in this study can be found in online repositories. The names of the repository/repositories and accession number(s) can be found in the article/Supplementary Material.
Ethics Statement
The animal study was reviewed and approved by Animal Welfare Unit, UQ Research and Innovation, the University of Queensland (UQCCR/459/16). Written informed consent was obtained from the owners for the participation of their animals in this study.
Author Contributions
Conceptualization, PA, NT, HP, KV, NC, NM, MM, and JL. Investigation, PA. Writing—original draft preparation, PA. Writing—review and editing, NT, KV, MM, and JL. Visualization, PA. Supervision, MM and JL. All authors have read and agreed to the published version of the manuscript.
Funding
This project is funded by Advance Queensland Innovative Partnership (AQIP01115-16RD1) program. Nindooinbah Pastoral Company partnered in this project. PA and NT are supported by a student scholarship from the Australian Research Council (Grant No: ARC LP160101854) and QUT HDR Tuition fee sponsorship. Our laboratory experiments were funded, in part, by funding from a partnership fund (DRCX1302) between the New Zealand Ministry of Business, Innovation and Employment and New Zealand dairy farmers through DairyNZ Inc.
Conflict of Interest
Authors NC and MM were employed by company Nindooinbah Pastoral Company.
The remaining authors declare that the research was conducted in the absence of any commercial or financial relationships that could be construed as a potential conflict of interest.
Publisher’s Note
All claims expressed in this article are solely those of the authors and do not necessarily represent those of their affiliated organizations, or those of the publisher, the editors and the reviewers. Any product that may be evaluated in this article, or claim that may be made by its manufacturer, is not guaranteed or endorsed by the publisher.
Supplementary Material
The Supplementary Material for this article can be found online at: https://www.frontiersin.org/articles/10.3389/fcimb.2021.780424/full#supplementary-material
References
Abeysinghe, P. (2021). Differentially Expressed Extracellular Vesicle, Exosome and Non-Exosome miRNA Profile in High and Low Tick-Resistant Beef Cattle. DRAFT VERSION. Harvard Dataverse. doi: 10.7910/DVN/R1SISD
Abeysinghe, P., Turner, N., Morean Garcia, I., Mosaad, E., Peiris, H. N., Mitchell, M. D. (2020). The Role of Exosomal Epigenetic Modifiers in Cell Communication and Fertility of Dairy Cows. Int. J. Mol. Sci. 21, 9106. doi: 10.3390/ijms21239106
Agarwal, V., Bell, G. W., Nam, J. W., Bartel, D. P. (2015). Predicting Effective microRNA Target Sites in Mammalian mRNAs. Elife 4, e05005. doi: 10.7554/eLife.05005
Barré, N., Happold, J., Delathière, J.-M., Desoutter, D., Salery, M., de Vos, A., et al. (2011). A Campaign to Eradicate Bovine Babesiosis From New Caledonia. Ticks Tick-borne Dis. 2, 55–61. doi: 10.1016/j.ttbdis.2010.11.001
Binder, J. X., Pletscher-Frankild, S., Tsafou, K., Stolte, C., O’Donoghue, S. I., Schneider, R., et al. (2014). COMPARTMENTS: Unification and Visualization of Protein Subcellular Localization Evidence. Database 2014. doi: 10.1093/database/bau012
Braud, M. (2017). Identification and Analysis of Polymorphisms in miRNA Genes and Associated Targets in Bos Taurus and Bos Primigenius for Genomic Selection in Cattle. Ph.D. Tesis, National University of Ireland, Galway, Ireland. Available at: https://scholar.google.com/scholar_lookup?title=Identification+and+Analysis+of+Polymorphisms+in+miRNA+Genes+and+Associated+Targets+in+Bos+Taurus+and+Bos+Primigenius+for+Genomic+Selection+in+Cattle&author=Braud,+M.&publication_year=2017.
Burrow, H. M., Mans, B. J., Cardoso, F. F., Birkett, M. A., Kotze, A. C., Hayes, B. J., et al. (2019). Towards a New Phenotype for Tick Resistance in Beef and Dairy Cattle: A Review. Anim. Production Sci. 59, 1401–1427. doi: 10.1071/AN18487
Cabezas-Cruz, A., Hodžić, A., Román-Carrasco, P., Mateos-Hernández, L., Duscher, G. G., Sinha, D. K., et al. (2019). Environmental and Molecular Drivers of the α-Gal Syndrome. Front. Immunol. 10, 1210. doi: 10.3389/fimmu.2019.01210
Cardoso, T. F., Coutinho, L. L., Bruscadin, J. J., da Silva Diniz, W. J., Petrini, J., Andrade, B. G. N., et al. (2021). Multi-Omics A Pp Roach Reveals miR-SNPs Affecting Muscle Fatty Acids Profile in Nelore Cattle. Genes 12, 67. doi: 10.3390/genes12010067
Colitti, M., Sgorlon, S., Licastro, D., Stefanon, B. (2019). Differential Expression of miRNAs in Milk Exosomes of Cows Subjected to Group Relocation. Res. Vet. Sci. 122, 148–155. doi: 10.1016/j.rvsc.2018.11.024
Crookenden, M. A., Walker, C. G., Peiris, H., Koh, Y., Heiser, A., Loor, J. J., et al. (2016). Short Communication: Proteins From Circulating Exosomes Represent Metabolic State in Transition Dairy Cows. J. Dairy Sci. 99, 7661–7668. doi: 10.3168/jds.2015-10786
Dai, J., Su, Y., Zhong, S., Cong, L., Liu, B., Yang, J., et al. (2020). Exosomes: Key Players in Cancer and Potential Therapeutic Strategy. Signal Transduct. Target. Ther. 5, 145. doi: 10.1038/s41392-020-00261-0
Downward, J. (2003). Targeting RAS Signalling Pathways in Cancer Therapy. Nat. Rev. Cancer 3, 11–22. doi: 10.1038/nrc969
Dypås, L. B., Gützkow, K. B., Olsen, A.-K., Duale, N. (2020). MiRNA Profiles in Blood Plasma From Mother-Child Duos in Human Biobanks and the Implication of Sample Quality: Circulating miRNAs as Potential Early Markers of Child Health. PloS One 15, e0231040. doi: 10.1371/journal.pone.0231040
Fan, Y., Siklenka, K., Arora, S. K., Ribeiro, P., Kimmins, S., Xia, J. (2016). Mirnet - Dissecting miRNA-Target Interactions and Functional Associations Through Network-Based Visual Analysis. Nucleic Acids Res. 44, W135–W141. doi: 10.1093/nar/gkw288
Friedländer, M. R., Mackowiak, S. D., Li, N., Chen, W., Rajewsky, N. (2012). Mirdeep2 Accurately Identifies Known and Hundreds of Novel microRNA Genes in Seven Animal Clades. Nucleic Acids Res. 40, 37–52. doi: 10.1093/nar/gkr688
Gangoda, L., Boukouris, S., Liem, M., Kalra, H., Mathivanan, S. (2015). Extracellular Vesicles Including Exosomes are Mediators of Signal Transduction: Are They Protective or Pathogenic? Proteomics 15, 260–271. doi: 10.1002/pmic.201400234
Greening, D. W., Gopal, S. K., Xu, R., Simpson, R. J., Chen, W. (2015). Exosomes and Their Roles in Immune Regulation and Cancer. Semin. Cell Dev. Biol. 40, 72–81. doi: 10.1016/j.semcdb.2015.02.009
Hemmings, B. A., Restuccia, D. F. (2012). PI3K-PKB/Akt Pathway. Cold Spring Harb. Perspect. Biol. 4, a011189–a011189. doi: 10.1101/cshperspect.a011189
Herrnreiter, C. J., Li, X., Luck, M. E., Zilliox, M. J., Choudhry, M. A. (2021). Integrated Analysis of Dysregulated microRNA and mRNA Expression in Intestinal Epithelial Cells Following Ethanol Intoxication and Burn Injury. Sci. Rep. 11, 20213. doi: 10.1038/s41598-021-99281-1
Jonsson, N. N. (2006). The Productivity Effects of Cattle Tick (Boophilus Microplus) Infestation on Cattle, With Particular Reference to Bos Indicus Cattle and Their Crosses. Vet. Parasitol. 137, 1–10. doi: 10.1016/j.vetpar.2006.01.010
Jonsson, N. N., Bock, R. E., Jorgensen, W. K. (2008). Productivity and Health Effects of Anaplasmosis and Babesiosis on Bos Indicus Cattle and Their Crosses, and the Effects of Differing Intensity of Tick Control in Australia. Vet. Parasitol. 155, 1–9. doi: 10.1016/j.vetpar.2008.03.022
Karimizadeh, E., Sharifi-Zarchi, A., Nikaein, H., Salehi, S., Salamatian, B., Elmi, N., et al. (2019). Analysis of Gene Expression Profiles and Protein-Protein Interaction Networks in Multiple Tissues of Systemic Sclerosis. BMC Med. Genomics 12, 199. doi: 10.1186/s12920-019-0632-2
Kasaija, P. D., Estrada-Peña, A., Contreras, M., Kirunda, H., de la Fuente, J. (2021). Cattle Ticks and Tick-Borne Diseases: A Review of Uganda’s Situation. Ticks Tick Borne Dis. 12, 101756. doi: 10.1016/j.ttbdis.2021.101756
Koh, Y. Q., Almughlliq, F. B., Vaswani, K., Peiris, H. N., Mitchell, M. D. (2018). Exosome Enrichment by Ultracentrifugation and Size Exclusion Chromatography. Front. Biosci. (Landmark Ed) 23, 865–874. doi: 10.2741/4621
Kugeratski, F. G., Hodge, K., Lilla, S., McAndrews, K. M., Zhou, X., Hwang, R. F., et al. (2021). Quantitative Proteomics Identifies the Core Proteome of Exosomes With Syntenin-1 as the Highest Abundant Protein and a Putative Universal Biomarker. Nat. Cell Biol. 23, 631–641. doi: 10.1038/s41556-021-00693-y
Lane, J., Jubb, T., Shephard, R., Webb-Ware, J., Fordyce, G. (2015). “Priority List of Endemic Diseases for the Red Meat Industries. Final Report B.AHE.0010. Meat and Livestock Australia,” in GHD Pty Ltd2015. Meat and Livestock Australia Limited. Available at: http://era.daf.qld.gov.au/id/eprint/5030/.
Lew-Tabor, A. E., Rodriguez Valle, M. (2016). A Review of Reverse Vaccinology Approaches for the Development of Vaccines Against Ticks and Tick Borne Diseases. Ticks Tick-borne Dis. 7, 573–585. doi: 10.1016/j.ttbdis.2015.12.012
Li, C. Y., Cai, J.-H., Tsai, J. J. P., Wang, C. C. N. (2020). Identification of Hub Genes Associated With Development of Head and Neck Squamous Cell Carcinoma by Integrated Bioinformatics Analysis. Front. Oncol. 10. doi: 10.3389/fonc.2020.00681
Li, D., Knox, B., Gong, B., Chen, S., Guo, L., Liu, Z., et al. (2021). Identification of Translational microRNA Biomarker Candidates for Ketoconazole-Induced Liver Injury Using Next-Generation Sequencing. Toxicol. Sci. 179, 31–43. doi: 10.1093/toxsci/kfaa162
Lizé, M., Klimke, A., Dobbelstein, M. (2011). MicroRNA-449 in Cell Fate Determination. Cell Cycle 10, 2874–2882. doi: 10.4161/cc.10.17.17181
Mapholi, N. O., Marufu, M. C., Maiwashe, A., Banga, C. B., Muchenje, V., MacNeil, M. D., et al. (2014). Towards a Genomics Approach to Tick (Acari: Ixodidae) Control in Cattle: A Review. Ticks Tick-borne Dis. 5, 475–483. doi: 10.1016/j.ttbdis.2014.04.006
Marima, J. K., Nel, C. L., Marufu, M. C., Jonsson, N. N., Dube, B., Dzama, K. (2020). A Genetic and Immunological Comparison of Tick-Resistance in Beef Cattle Following Artificial Infestation With Rhipicephalus Ticks. Exp. Appl. Acarol. 80, 569–590. doi: 10.1007/s10493-020-00480-8
Marufu, M. C., Qokweni, L., Chimonyo, M., Dzama, K. (2011). Relationships Between Tick Counts and Coat Characteristics in Nguni and Bonsmara Cattle Reared on Semiarid Rangelands in South Africa. Ticks Tick-borne Dis. 2, 172–177. doi: 10.1016/j.ttbdis.2011.07.001
Ma, S., Tong, C., Ibeagha-Awemu, E. M., Zhao, X. (2019). Identification and Characterization of Differentially Expressed Exosomal microRNAs in Bovine Milk Infected With Staphylococcus Aureus. BMC Genomics 20, 934. doi: 10.1186/s12864-019-6338-1
Mittelbrunn, M., Gutierrez-Vazquez, C., Villarroya-Beltri, C., Gonzalez, S., Sanchez-Cabo, F., Gonzalez, M. A., et al. (2011). Unidirectional Transfer of microRNA-Loaded Exosomes From T Cells to Antigen-Presenting Cells. Nat. Commun. 2, 282. doi: 10.1038/ncomms1285
Mukiibi, R., Johnston, D., Vinsky, M., Fitzsimmons, C., Stothard, P., Waters, S. M., et al. (2020). Bovine Hepatic Mirnaome Profiling and Differential miRNA Expression Analyses Between Beef Steers With Divergent Feed Efficiency Phenotypes. Sci. Rep. 10, 19309. doi: 10.1038/s41598-020-73885-5
Hurtado, 0. J. B., Giraldo-Ríos, C. (2018). “Economic and Health Impact of the Ticks in Production Animals,” in Ticks and Tick-Borne Pathogens. Eds. M, A. a., Perera, P. K.
Playford, M., Rabiee, A., Lean, I., Ritchie, M (2005). Review of Research Needs for Cattle Tick Control, Phases I and II. Locked Bag991, North Sydney NSW: Meat & Livestock Australia Ltd. pp. 2059. Available at: https://scholar.google.com.au/scholar?cites=12231663165070136875&as_sdt=2005&sciodt=0,5&hl=en.
Ponsuksili, S., Tesfaye, D., Schellander, K., Hoelker, M., Hadlich, F., Schwerin, M., et al. (2014). Differential Expression of miRNAs and Their Target mRNAs in Endometria Prior to Maternal Recognition of Pregnancy Associates With Endometrial Receptivity for In Vivo- and In Vitro-Produced Bovine Embryos1. Biol. Reprod. 91, 1–12. doi: 10.1095/biolreprod.114.121392
Porto Neto, L. R., Jonsson, N. N., D’Occhio, M. J., Barendse, W. (2011). Molecular Genetic a Pp Roaches for Identifying the Basis of Variation in Resistance to Tick Infestation in Cattle. Vet. Parasitol. 180, 165–172. doi: 10.1016/j.vetpar.2011.05.048
Record, M., Carayon, K., Poirot, M., Silvente-Poirot, S. (2014). Exosomes as New Vesicular Lipid Transporters Involved in Cell-Cell Communication and Various Pathophysiologies. Biochim. Biophys. Acta 1841, 108–120. doi: 10.1016/j.bbalip.2013.10.004
Scheel, T. K. H., Moore, M. J., Luna, J. M., Nishiuchi, E., Fak, J., Darnell, R. B., et al. (2017). Global Ma Pp Ing of miRNA-Target Interactions in Cattle (Bos Taurus). Sci. Rep. 7, 8190–8190. doi: 10.1038/s41598-017-07880-8
Shannon, P., Markiel, A., Ozier, O., Baliga, N. S., Wang, J. T., Ramage, D., et al. (2003). Cytoscape: A Software Environment for Integrated Models of Biomolecular Interaction Networks. Genome Res. 13, 2498–2504. doi: 10.1101/gr.1239303
Sheller-Miller, S., Lei, J., Saade, G., Salomon, C., Burd, I., Menon, R. (2016). Feto-Maternal Trafficking of Exosomes in Murine Pregnancy Models. Front. Pharmacol. 7, 432. doi: 10.3389/fphar.2016.00432
Szklarczyk, D., Gable, A. L., Lyon, D., Junge, A., Wyder, S., Huerta-Cepas, J., et al. (2019). STRING V11: Protein–Protein Association Networks With Increased Coverage, Su Pp Orting Functional Discovery in Genome-Wide Experimental Datasets. Nucleic Acids Res. 47, D607–D613. doi: 10.1093/nar/gky1131
Turner, N. P., Abeysinghe, P., Kwan Cheung, K. A., Vaswani, K., Logan, J., Mitchell, M. D. (2021). A Comparison of Blood Plasma Exosome Enrichment Strategies for Proteomic Analysis, 02 September 2021, PREPRINT (Version 1) available at Research Square. doi: 10.21203/rs.3.rs-819042/v1
Valadi, H., Ekström, K., Bossios, A., Sjöstrand, M., Lee, J. J., Lötvall, J. O. (2007). Exosome-Mediated Transfer of mRNAs and MicroRNAs Is a Novel Mechanism of Genetic Exchange Between Cells. Nat Cell Biol. 9 (6), 654–9.
van Niel, G., D’Angelo, G., Raposo, G. (2018). Shedding Light on the Cell Biology of Extracellular Vesicles. Nat. Rev. Mol. Cell Biol. 19, 213–228. doi: 10.1038/nrm.2017.125
Vejnar, C. E., Blum, M., Zdobnov, E. M. (2013). Mirmap Web: Comprehensive microRNA Target Prediction Online. Nucleic Acids Res. 41, W165–W168. doi: 10.1093/nar/gkt430
Wang, X., Tian, F., Chen, C., Feng, Y., Sheng, X., Guo, Y., et al. (2019). Exosome-Derived Uterine microRNAs Isolated From Cows With Endometritis Impede Blastocyst Development. Reprod. Biol. 19, 204–209. doi: 10.1016/j.repbio.2019.06.003
Young, A. S., Groocock, C. M., Kariuki, D. P. (1988). Integrated Control of Ticks and Tick-Borne Diseases of Cattle in Africa. Parasitology 96, 403–432. doi: 10.1017/S0031182000058388
Yu, H.-R., Huang, L.-H., Li, S.-C. (2018). Roles of microRNA in the Immature Immune System of Neonates. Cancer Lett. 433, 99–106. doi: 10.1016/j.canlet.2018.06.014
Zhang, Y., Liu, Y., Liu, H., Tang, W. H. (2019). Exosomes: Biogenesis, Biologic Function and Clinical Potential. Cell Biosci. 9, 19. doi: 10.1186/s13578-019-0282-2
Keywords: extracellular vesicles, exosome, tick, beef cattle, miRNA
Citation: Abeysinghe P, Turner N, Peiris H, Vaswani K, Cameron N, McGhee N, Logan J and Mitchell MD (2021) Differentially Expressed Extracellular Vesicle, Exosome and Non-Exosome miRNA Profile in High and Low Tick-Resistant Beef Cattle. Front. Cell. Infect. Microbiol. 11:780424. doi: 10.3389/fcimb.2021.780424
Received: 21 September 2021; Accepted: 01 December 2021;
Published: 17 December 2021.
Edited by:
Jesús Valdés, Instituto Politécnico Nacional de México (CINVESTAV), MexicoReviewed by:
Shahid Karim, University of Southern Mississippi, United StatesMilton Y Nishiyama-Jr, Butantan Institute, Brazil
Copyright © 2021 Abeysinghe, Turner, Peiris, Vaswani, Cameron, McGhee, Logan and Mitchell. This is an open-access article distributed under the terms of the Creative Commons Attribution License (CC BY). The use, distribution or reproduction in other forums is permitted, provided the original author(s) and the copyright owner(s) are credited and that the original publication in this journal is cited, in accordance with accepted academic practice. No use, distribution or reproduction is permitted which does not comply with these terms.
*Correspondence: Pevindu Abeysinghe, abeysinghe.abeysingh@hdr.qut.edu.au