- 1School of Public Health, Shantou University, Shantou, China
- 2College of Animal Science and Veterinary Medicine, Heilongjiang Bayi Agricultural University, Daqing, China
- 3Key Laboratory of Zoonosis Research, Ministry of Education, Institute of Zoonosis, College of Veterinary Medicine, Jilin University, Changchun, China
- 4The Seventh Affiliated Hospital, Sun Yat-sen University, Shenzhen, China
- 5Institute of NBC Defence, PLA Army, Beijing, China
Clonorchiasis, which is caused by Clonorchis sinensis, is an important foodborne disease worldwide. The excretory-secretory products (ESPs) of C. sinensis play important roles in host-parasite interactions by acting as causative agents. In the present study, the ESPs and sera positive for C. sinensis were collected to identify proteins specific to the sera of C. sinensis (i.e., proteins that do not cross-react with Fasciola hepatica and Schistosoma japonicum) at different infection periods. Briefly, white Japanese rabbits were artificially infected with C. sinensis, and their sera were collected at 7 days post-infection (dpi), 14 dpi, 35 dpi, and 77 dpi. To identify the specific proteins in C. sinensis, a co-immunoprecipitation (Co-IP) assay was conducted using shotgun liquid chromatography tandem-mass spectrometry (LC-MS/MS) to pull down the sera roots of C. sinensis, F. hepatica, and S. japonicum. For the annotated proteins, 32, 18, 39, and 35 proteins specific to C. sinensis were pulled down by the infected sera at 7, 14, 35, and 77 dpi, respectively. Three proteins, Dynein light chain-1, Dynein light chain-2 and Myoferlin were detected in all infection periods. Of these proteins, myoferlin is known to be overexpressed in several human cancers and could be a promising biomarker and therapeutic target for cancer cases. Accordingly, this protein was selected for further studies. To achieve a better expression, myoferlin was truncated into two parts, Myof1 and Myof2 (1,500 bp and 810 bp), based on the antigenic epitopes provided by bioinformatics. The estimated molecular weight of the recombinant proteins was 57.3 ku (Myof1) and 31.3 ku (Myof2). Further, both Myof1 and Myof2 could be probed by the sera from rabbits infected with C. sinensis. No cross-reaction occurred with the positive sera of S. japonica, F. hepatica, and negative controls. Such findings indicate that myoferlin may be an important diagnostic antigen present in the ESPs. Overall, the present study provides new insights into proteomic changes between ESPs and hosts in different infection periods by LC-MS/MS. Moreover, myoferlin, as a biomarker, may be used to develop an objective method for future diagnosis of clonorchiasis.
Introduction
Clonorchis sinensis is an important foodborne pathogen that causes clonorchiasis as well as liver and biliary diseases when raw fish with C. sinensis metacercariae is consumed (Ju et al., 2009). Juvenile fluke, excysting in the duodenum of the host, migrate to the intrahepatic bile ducts, where they develop into adults and survive for more than one decade. It primarily affects mammals, such as dogs, cats, and humans, and its typical clinical symptoms include jaundice, cholangitis, and biliary obstruction (Qian et al., 2016). Clonorchiasis is also closely related to liver fibrosis, other human hepatobiliary diseases, and cholangiocarcinoma (CCA) (Pak et al., 2017). In 2009, C. sinensis was classified as a class I biological carcinogen (Véronique et al., 2009). It is estimated that 15 million people suffer from clonorchiasis, and approximately 200 million people, primarily in East and Southeast Asia, such as China, South Korea, and Vietnam, are at risk of infection (Qian et al., 2016; Tang et al., 2016).
Excretory-secretory products (ESPs), which are released by excretory organs during parasitism, can stimulate the host immune response, play important roles in host-parasite interactions, and provide attractive materials for identifying antigenic candidates and new drug targets (Li et al., 2020). Different sources of worms produce various antigen substances and distinct immune response procedures (Pino et al., 1986). Thus, identifying the proteins in ESPs is crucial for understanding the mechanisms inherent to parasite-induced pathogenesis. The ESPs of C. sinensis are highly sensitive and specific antigens for the diagnosis of clonorchiasis (Cho et al., 2020). During the development of C. sinensis, complex antigens that can affect the host immune system are secreted. Although the antigenic and pathogenic functions of C. sinensis have been investigated for several decades, the components and roles of C. sinensis ESPs remain limited (Li et al., 2004). The components of C. sinensis ESPs are complex; however, they mainly include 7-8, 26-28, and 34-37 ku proteins, with the range of 26-45 ku playing major roles in the production of antibodies in infected rabbits (Hong et al., 2001; Hong et al., 2002). These antigenic candidates, to a great extent, are yet to be characterized. Therefore, discovering reliable and prognostic markers for clonorchiasis diagnosis is of great importance. The protein components of ESPs in several species, such as F. gigantica, S. japonicum, S. mansoni, and Paragonimus westermani, have already been characterized using proteomics approaches based on mass spectrometry (Lee et al., 2006; Guillou et al., 2007; Liu et al., 2009; Huang et al., 2019).
Exploiting proteomic tools can enable the identification of more sensitive and specific serodiagnostic antigens that do not cross-react with other parasites. Hence, in this study, the co-immunoprecipitation (Co-IP) assay was used to pull down three types of serum. The sera of rabbits infected with C. sinensis were collected at 7 days post infection (dpi), 14 dpi, 35 dpi, and 77 dpi, and the sera positive for F. hepatica and S. japonicum were also collected. Immunoprecipitation was assessed and characterized using liquid chromatography-tandem mass spectrometry (LC-MS/MS). The objective of in this study was to identify more sensitive and specific antigenic targets in ESPs, and provide more potential diagnostic antigens for clonorchiasis.
Materials and Methods
Parasites and Sera
C. sinensis metacercariae were collected from naturally infected Pseudorasbora parva in the endemic area of Qiqihar, Heilongjiang province, China. Muscular tissue was digested with artificial digestive juice (1% pepsin-hydrochloric acid, Aladdin, China). White Japanese rabbits were purchased from Yisi Experimental Animal Technology Corporation (Changchun City, Jilin Province, China). Fecal examination was conducted before selection to exclude any prior infection with C. sinensis. This study was approved by the Animal Health, Animal Care, and Use Committee of the Heilongjiang Bayi Agricultural University. Twenty rabbits (8-9-month-old) negative for C. sinensis were selected and randomly divided into two groups: control group and C. sinensis-infected group (n=10 each). Rabbits in the experimental group were infected orally with 500 viable metacercariae, while rabbits in the control group were mock-inoculated with 0.85% w/v NaCl solution without metacercariae. After 18 days of infection, the feces of rabbits were collected for fecal examination. Blood samples from each animal were collected aseptically into tubes without anticoagulant, and at 7, 14, 35, and 77 dpi, sera were separated by centrifugation and preserved at -80°C for further use. Positive sera for F. hepatica and S. japonicum were both obtained from artificially infected rabbits. The rabbits were orally infected with 40 viable F. hepatica metacercariae, and the sera were obtained at 90 dpi, stored at the College of Animal Science and Veterinary Medicine, Heilongjiang Bayi Agricultural University. After 42 dpi, positive sera of S. japonicum were acquired from rabbits, which infected with 1000 ± 10 S. japonicum cercariae, the sera were provided by the Laboratory Animal Center of Shanghai Veterinary Research Institute, Chinese Academy of Agriculture Sciences.
Collection and Preparation of C. sinensis ESPs
CSESPs were prepared according to standard procedures (Pak et al., 2017). Briefly, C. sinensis adults were separated from the bile ducts of infected rabbits, washed three times with PBS (stored at 37°C), and preincubated in Locke’s medium (NaCl 8.9 g, KCl 0.42 g, NaHCO3 0.2 g, and CaCl2 0.24 g (wt/vol) at 37°C and 5% CO2 for 1 h. Thereafter, the parasites were transferred to fresh medium and incubated for 48 h; the medium was changed at 6-h intervals. Finally, the collected cultures were centrifuged at 10 000 g for 30 min at 4°C, to obtain the supernatant, filtered (0.22 μm), and stored at -80°C.
Co-IP
The protein A/G plus-agarose immunoprecipitation kit (Santa Cruz Biotechnology, USA) was used for Co-IP according to the manufacturer’s instructions. Briefly, 200 μL of serum positive and negative (7, 14, 35, and 77 dpi) for C. sinensis was added into tubes; rabbit sera positive for S. japonicum and F. hepatica were handled in the same manner. Protein A/G plus-agarose beads (30 μL) were added to each tube and incubated overnight at 4°C. The beads were pelleted by centrifugation at 1,000 g for 30 s at 4°C. Five hundred micrograms C. sinensis ESPs (500 µg) was precleared by incubation with protein A/G plus-agarose beads, and incubated for 1.5 h at 4°C. The pellet, collected by centrifugation at 1,000 × g for 5 min at 4°C, was washed three times with PBS buffer, and resuspended in 50 μL SDS loading buffer. The samples were then boiled at 100°C for 10 min. Ten microliters of the sample was analyzed by SDS-PAGE, and the remaining 40 μL was stored for mass spectrometry identification.
Trypsin Digestion
The stained protein bands were cut into 1 mm3 pieces and washed twice with 200 µL of mass spectrometry (MS) water for 10 min. The gels were detained with 50% acetonitrile (ACN) in 50 mM ammonium bicarbonate (ABC), dehydrated by washing with 100% ACN until the gel turned white, and then washed twice with 200 µL of MS water for 10 min/wash. ACN was added to induce dehydration, which occurred until the colloidal particles turned white. Thereafter, they were vacuum dried for 10 min. Proteins on the gels were treated with 200 µL of 10 mM DTT for 1 h at 37°C and subsequently alkylated with 200 µL of 55 mM iodoacetamide (IAM) for 30 min in the dark. The gels were then washed with digested buffer and treated with ACN, as described above. The suspension was washed with the following solutions: MS water (once), ACN (once), MS water (once), and ACN (once) for 10 min/wash, each experiment group was performed three times.
Liquid Chromatography-Tandem Mass Analysis
Peptides were separated by liquid chromatography using an Acclaim Pep Map 100 column and an EASY-Spray column on an EASY-NLC 1,000 system. The flow rate was set to 0.600 μL/min, and gradient elution was performed for 88 min. The gradient was generated using mobile phase A, which comprised of 100% ddH2O containing 0.1% formic acid, and mobile phase B, which consisted of 100% ACN containing 0.1% formic acid. Label-free mass spectrometry was performed using a QE HF-X mass spectrometer. The scan events were composed of one single full MS scan and a 3 s MS/MS scan dependent on the previous scan data. The spray voltages were set at 3.8 kV, and the heated capillary temperature was 320°C. The parameters of the MS/MS scan were as follows: resolution, 15,000; auto gain control target, under 2 × 104; maximum isolation time, 30 ms; and normalized collision energy, 27%, each experiment group was performed three times.
Data Analysis
Specific proteins were analyzed using Proteome Discoverer 2.4.1.15. Trypsin was employed as the enzyme, which cleaved after all lysine and arginine residues, with up to two missed cleavages allowed. Carbamidomethylating of cysteine was specified as fixed modification, and protein N-terminal acetylation, oxidation of methionine, and pyro-glutamate formation from glutamine were considered as variable modifications for all groups. The raw file of the mass spectrum was identified and analyzed using the commercial software, Max Quant (Thermo Fisher Scientific, Waltham, MA, USA). The precursor ion mass tolerance was set to 15 ppm and the fragment ion mass tolerance was set to 0.02 Da. All data were searched as a single batch with PSM and protein FDR set to 1% using a target decoy approach. The search parameters were as follows: species, C. sinensis; dynamic modification, oxidation; mass tolerance of the precursor ion, ± 15 ppm; fragment ion mass tolerance, ± 0.5 Da; and protein false discovery rate (FDR), 0.01. The maximum number of missed cleavages was two. Kyoto Encyclopedia of Genes and Genomes (KEGG) analysis of the proteins of C. sinensis was performed to select the most significant pathway and to analyze the relationship between the abundance of specific proteins of different pathways and the different periods of infection.
C. sinensis Myoferlin cDNA Protein Expression
Cloning of cDNAs encoding myoferlin from C. sinensis was achieved using the Uniprot database (protein ID H2KUF2). Further, the bioinformatics, including domains, antigen epitopes, and associated interacting proteins, was analyzed. Ultimately, to achieve a better expression of the protein, it was truncated into two parts: Myof1 (aa 160-aa 660) and Myof2 (aa 600-aa 870). The two coding regions of myoferlin were amplified by polymerase chain reaction (PCR) using the following primers: 5’-GGA TCC ACC ATA AAG GAT GTC CGT CA-3’ and 5’-CTC GAG CAG ACA ATG ACT CGT AGC TCA T-3’ (Cs-Myof1), or 5’-GGA TCC CTA CCA CTA GTA AAA GAG CAC G-3’ and 5’-CTC GAG CAC AAC CAA AAG AAC GAT GTC TC-3’ (Cs-Myof2), the underline represents restriction enzyme cutting sites (BamHI, GGA TCC and XhoI, CTC GAG). The resulting DNA was digested, purified, and ligated into the BamHI and XhoI cloning sites of the pET32a plasmid designed for expression. Recombinant plasmids (pET32a-CsMyof1 and pET32a-CsMyof2) were transformed into E. coli BL21 (DE3) cells, and positive clones were selected. Following induction with 1 mM IPTG, bacterial cells were harvested and lysed by sonication in PBS buffer. The lysate was centrifuged at 12,000 g for 20 min at 4°C, and the supernatant was collected. The fusion protein was purified by gel cutting, frozen, and melted five times using liquid nitrogen repeatedly. After centrifugation at 12,000 g for 20 min at 4°C, the supernatant was collected for further use.
Western Blot
The purified recombinant CsMyof1 and CsMyof2 were subjected to SDS-PAGE (12% gel) and transferred to a Hybond-C pure NC membranes (Immobilon-P, 0.45 μm; Millipore). The membranes were subsequently blocked with blocking buffer (5% w/v skim milk in PBS-T buffer) overnight at 4°C. The sera of rabbits infected with C. sinensis, S. japonicum, F. hepatica, and the negative sera were used as primary antibodies at a dilution of 1:200; the sera were incubated with membranes for 2 h at 37°C. Thereafter, the membranes were washed three times with PBS-T buffer for 30 min. Further incubation was performed using goat anti-rabbit IgG antibody conjugated with HRP at a dilution of 1:5,000 in blocking buffer for 1 h. After washing five times with PBS-T buffer, the membranes were treated with a diaminobenzidine substrate solution for 10 min.
Results
Collection of Sera and ESPs
The metacercariae of C. sinensis were collected from Pseudorasbora parva (Figure 1A). Fecal examination and morphological examination of eggs were carried out (Figure 1B). The first identification was performed at 19 dpi, and all infections were confirmed at 25 dpi. After the infected rabbits were sacrificed, the worms were detected in their hepatobiliary tract (Figure 1C); however, no worms were observed in control rabbits. C. sinensis was collected and made into films (Figure 1D), which complies with its typical characteristics, such as the branched testicles and S-shaped excretory sac. The model of rabbits infected with C. sinensis was successfully established, and sera at different periods of infection (7, 14, 35, and 77 dpi) were collected. Adult C. sinensis worms were collected and cultured in vitro for 48 h to prepare the ESPs. The majority of proteins among the ESPs ranged in molecular weight from 10 to 170 ku (Figure S1A). A total of 334 proteins were obtained, among which 254 annotated proteins were obtained from the C. sinensis protein library by BLAST homology comparison and Uniport identification (Figure S1B).
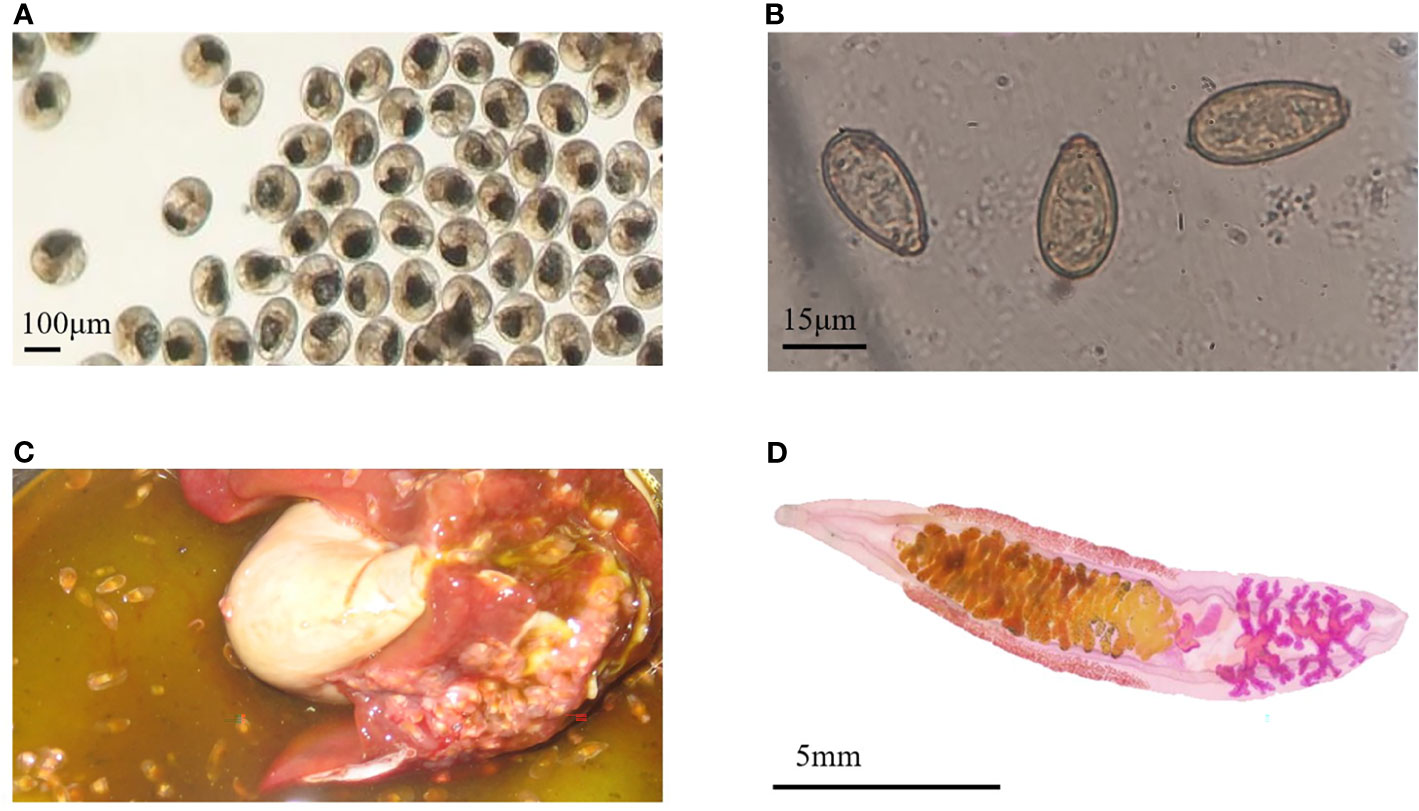
Figure 1 Infection characteristics. (A) The Metacercariae of Clonorchis sinensis used to infect rabbits. (B) Clonorchis sinensis eggs observed under an optical microscope. (C) Adult worms in the hepatobiliary tract. (D) The adult worm collected from the liver of infected rabbit.
LC-MS/MS Analysis and the Identification of Sera Proteins at Different Periods
An immuno-proteomic approach was used to identify the proteins secreted by C. sinensis, specifically using infection sera to pull down the C. sinensis ESPs that might be involved in host-parasite interactions. The results of the Co-IP assay revealed that the antibodies from serum at the periods of 7, 14, 35, and 77 dpi, could recognize and pull down the specific proteins from C. sinensis ESPs, with most proteins ranging in molecular weight from 15 to 170 ku (Figure 2). There were 32, 18, 39, and 35 proteins in the first round of differential screening between positive and negative serum samples (Figure 3A). The second round of screening was carried out by comparing and analyzing different types of positive sera, including F. hepatica, S. japonicum, and C. sinensis, based on the first screening (Figure 3B). According to the LC-MS/MS analysis, the obtained data was compared with the C. sinensis protein library to screen the differential proteins in each period. There were 13, 9, 16, and 15 types of proteins specific to the periods of 7, 14, 35, and 77 dpi with C. sinensis, respectively (Tables 1–4). Five proteins could only be detected in the early stage (7 dpi), which may explain their induction of an early response in the host, and three proteins were found to be specifically co-purified in all four periods of C. sinensis (Table 5), including dynein light chain-1, dynein light chain-2, and myoferlin, which were characterized by label-free quantification and functional analysis. These three proteins are involved in body movement and energy metabolism, and were found to display high expression at different stages, thereby providing energy for C. sinensis. Myoferlin, an important protein related to tumors, is a member of the ferlin family and is a type II transmembrane protein with a single transmembrane domain at the C terminus.
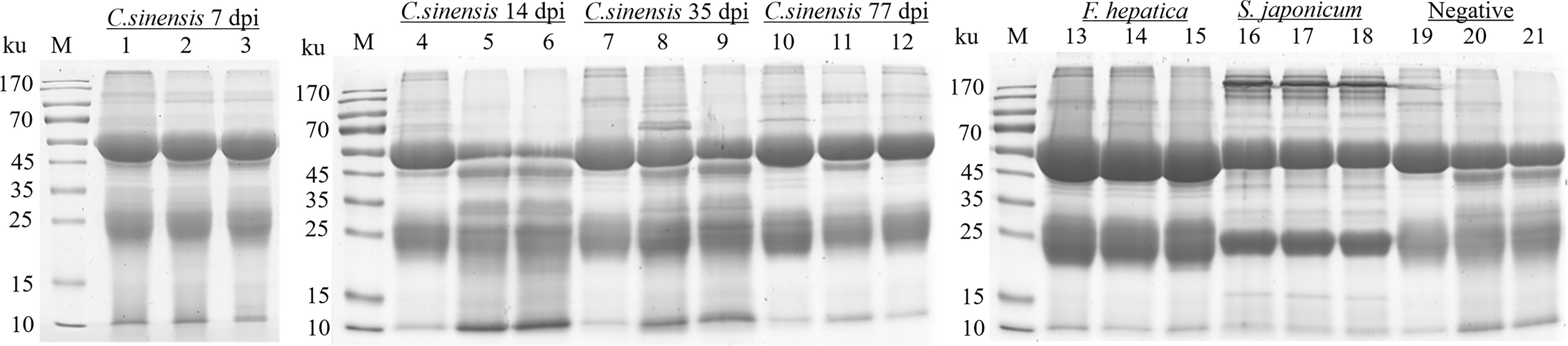
Figure 2 SDS-PAGE analysis of rabbit sera samples of C. sinensis at different infection period; F. hepatica and S. japonicum were co-cultured with the C. sinensis ESPs. Lines 1-3: proteins pulled down by rabbit serum at 7 dpi with C. sinensis. Lines 4-6: proteins pulled down by rabbit serum at 14 dpi with C. sinensis. Lines 7-9: proteins pulled down by rabbit serum at 35 dpi with C. sinensis. Lines 10-12: proteins pulled down by rabbit serum at 77 dpi with C. sinensis. Lines 13-15: proteins pulled down by F. hepatica serum. Lines 16-18: proteins pulled down by S. japonicum serum. Lines 19-21: proteins pulled down by rabbit negative serum.
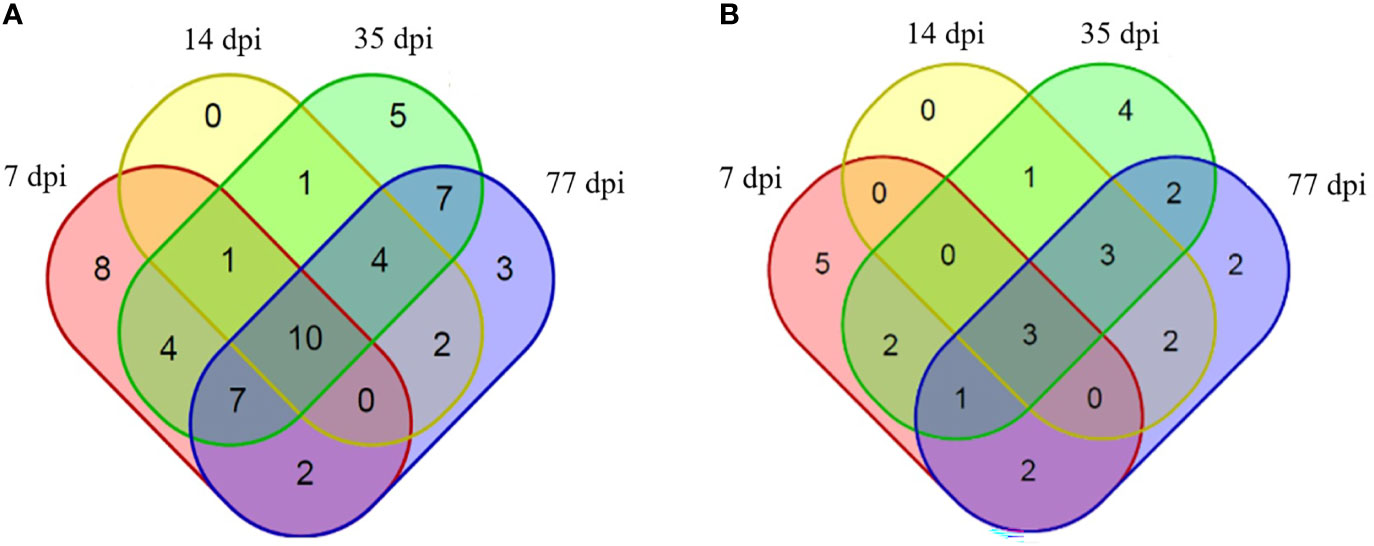
Figure 3 Proteins identified to be binding to rabbit sera at different infection time points. (A) Proteins specifically identified to bind to rabbit serum at 7 dpi with C. sinensis (pink), 14 dpi (yellow), 35 dpi (green) and 77 dpi (blue), compared with negative serum. (B) Proteins specifically identified to bind to rabbit serum at 7 dpi with C. sinensis (pink), 14 dpi (yellow), 35 dpi (green), and 77 dpi (blue), compared with S. japonicum serum, F. hepatica serum, and negative serum.
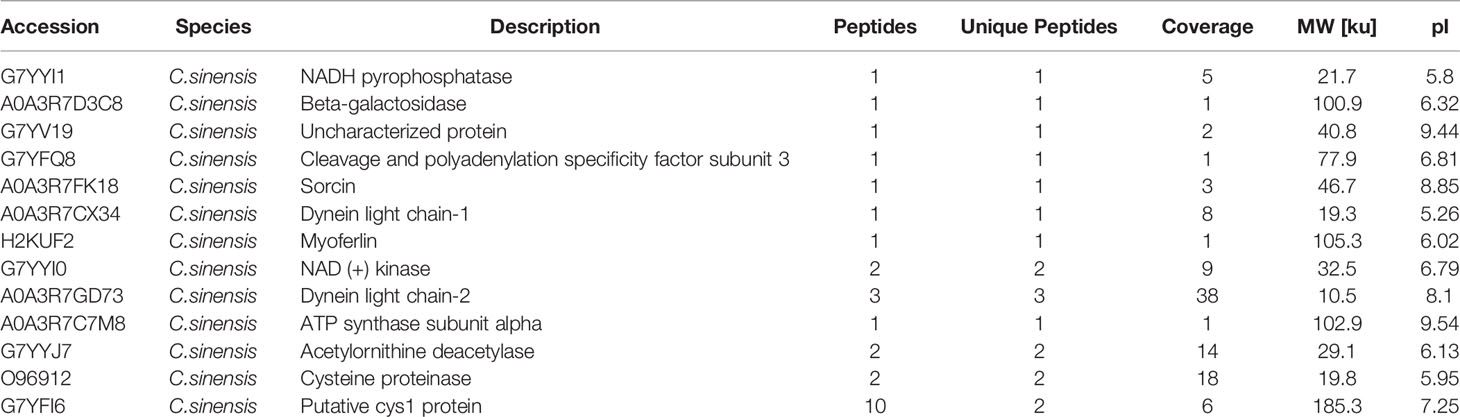
Table 1 Clonorchis sinensis excretory and secretory products which were detected by sera of rabbits in 7 dpi.
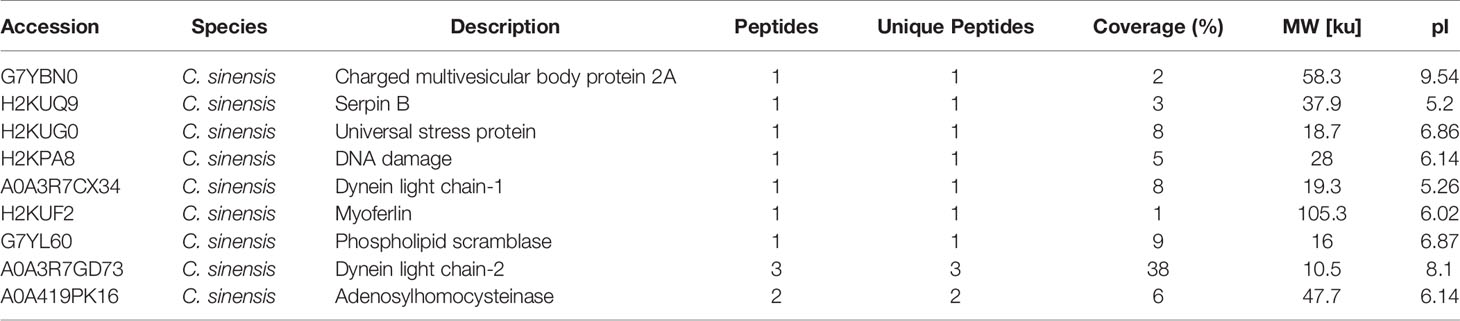
Table 2 Clonorchis sinensis excretory and secretory products which were detected by sera of rabbits in 14 dpi.
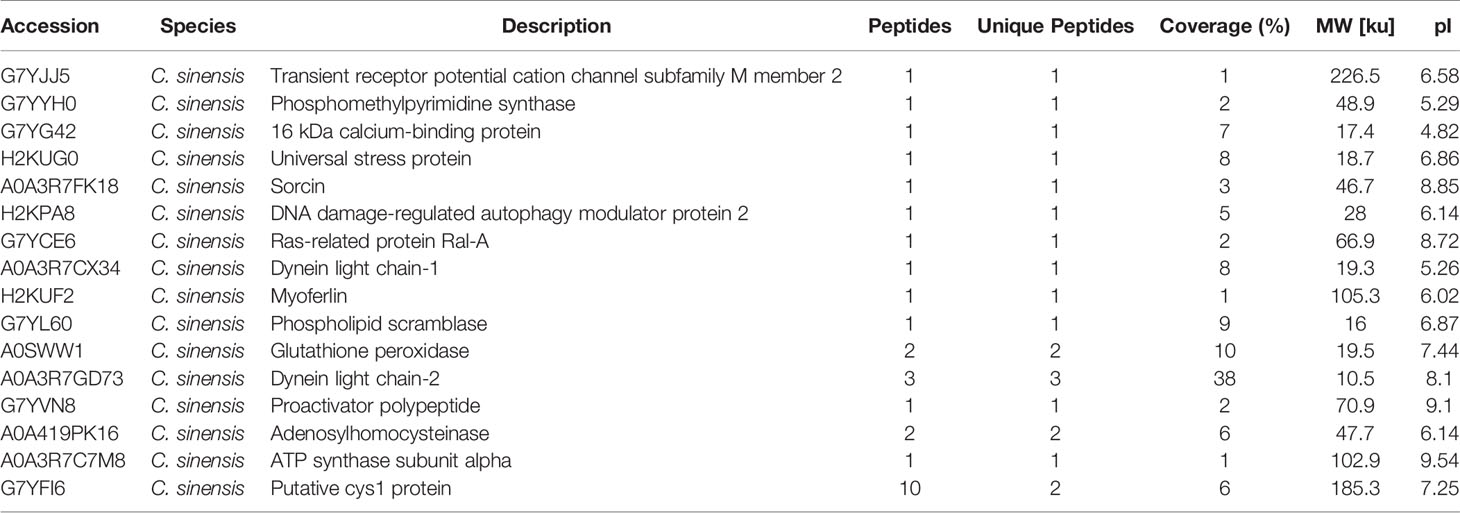
Table 3 Clonorchis sinensis excretory and secretory products which were detected by sera of rabbits in 35 dpi.
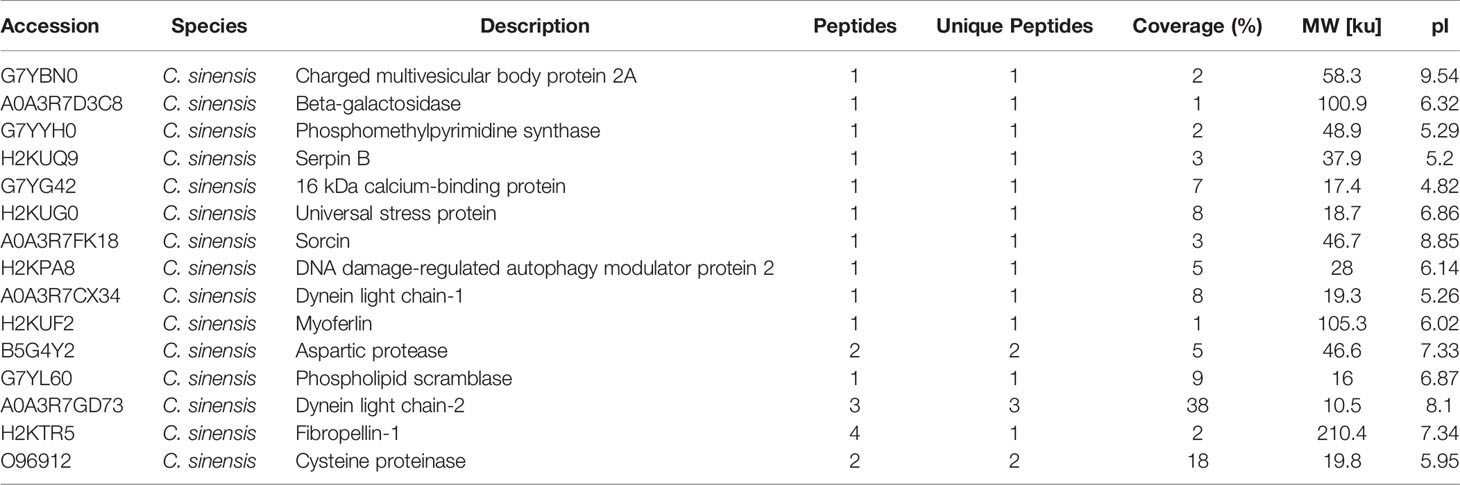
Table 4 Clonorchis sinensis excretory and secretory products which were detected by sera of rabbits in 77 dpi.

Table 5 Clonorchis sinensis excretory and secretory products which were detected by rabbit post-infection in all four periods.
The Selected Signal Path Based on KEGG
Twenty-seven proteins were detected in C. sinensis, but not in F. hepatica and S. japonicum, where they participate in host-parasite interactions. KEGG analysis revealed that thease proteins were mainly involved in oxidative phosphorylation, membrane transport, signal transduction, and metabolism. The abundance of proteins was different in each period of infection; cystoskeleton, oxidative phosphorylation, and mRNA surveillance pathway-related proteins were highly expressed at the early infection stage (7 dpi), and may be involved in early immune evasion (Figure 4A). In the middle stage of parasite infection (14 dpi), membrane trafficking related proteins were evidently increased, and may play important roles in providing energy for parasites (Figure 4B). NOD-like receptor signaling pathway, glutathione metabolism, and lysosome-related proteins were highly expressed at the late infection stage (35 dpi and 77 dpi); lysosomes secreted by C. sinensis can effectively inactivate the proteins released by hosts to protect themselves (Figures 4C, D).
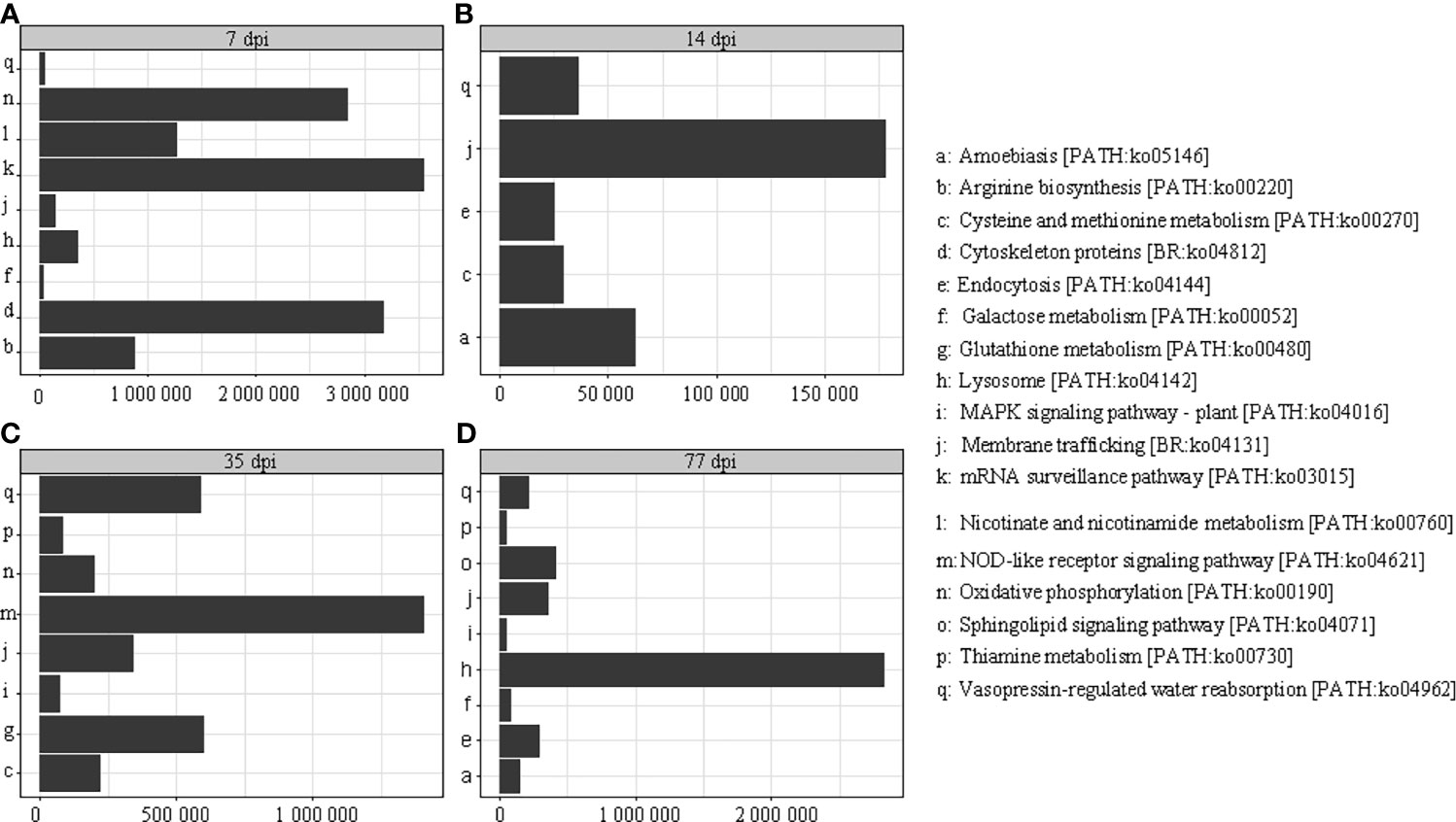
Figure 4 Kyoto Encyclopedia of Genes and Genomes (KEGG) analysis of the different periods after infection; the different periods are displayed on the horizontal axis and the abundance expressed by specific proteins is displayed on the longitudinal axis. (A) KEGG analysis of proteins at 7 dpi with C. sinensis. (B) KEGG analysis of proteins at 14 dpi with C. sinensis. (C) KEGG analysis of proteins at 35 dpi with C. sinensis. (D) KEGG analysis of proteins at 77 dpi with C. sinensis.
Analysis of the Amino Acid Sequence of Myoferlin
Myoferlin has two same domains: protein kinase C conserved region 2 (aa415-aa514, aa655-aa783). Regions with significant homology to the C2 domain have been identified in many proteins. The C2 domain is thought to be involved in calcium-dependent phospholipid binding and membrane-targeting processes, such as subcellular localization. Myoferlin also has two low-complexity domains (domain I, aa254-aa265 and II aa388-aa399) and a transmembrane region (aa887-aa909) (Figure S2A). The B cell epitopes of Myoferlin were predicted via IEDB (http://tools.iedb.org/), which resulted in ten linear epitopes (Table S1) and five discontinuous epitopes (Table S2). The predicted scores were all greater than 0.5, which indicated that the protein has a potential for binding antigens. The protein network interactions of myoferlin were also predicted (https://string-db.org/), which resulted in a total of 11 associated proteins (Figure S2B). Among these relative proteins, ATPase (ASAN1) is required for the post-translational delivery of tail-anchored proteins to the endoplasmic reticulum. ATPase also recognizes and selectively binds to the transmembrane domain of TA proteins in the cytosol. Vesicle-associated membrane protein 2 (VAMP2), a member of the SNARE family, is the first synaptobrevin studied in synaptic vesicles, and is regarded as a molecule that plays a key role in the process of cell growth, neurotransmission, hormone secretion, and insulin-dependent glucose uptake.
Gene Cloning and Antigenicity Analysis of Recombinant Proteins
To achieve a better expression, myoferlin was truncated into two parts (Myof1 and Myof2) according to the antigenic epitopes provided by bioinformatics. The Myof1 and Myof2 (1,500 bp and 810 bp) genes were amplified using specific oligonucleotide primers (Figure 5A) and sub-cloned into the E. coli pET32a expression vector. After sub-cloning, the size of the inserted DNA was confirmed by restriction digestion with BamHI and XhoI (Figure 5B). Subsequently, the cloned Myof1 and Myof2 were successfully expressed in E. coli BL21 (DE3) cells. The recombinant proteins were purified by gel cutting and analyzed by SDS-PAGE (Figure 6A). The molecular weights of the recombinant proteins were determined to be approximately 60 ku (Myof1) and 31 ku (Myof2). The sera from rabbits infected with C. sinensis could be probed at different levels, and the sera positive for S. japonica, F. hepatica, and from naive rabbit could hardly be probed (Figure 6B), which indicates that myoferlin may be a potential antigen for the detection of clonorchiasis.
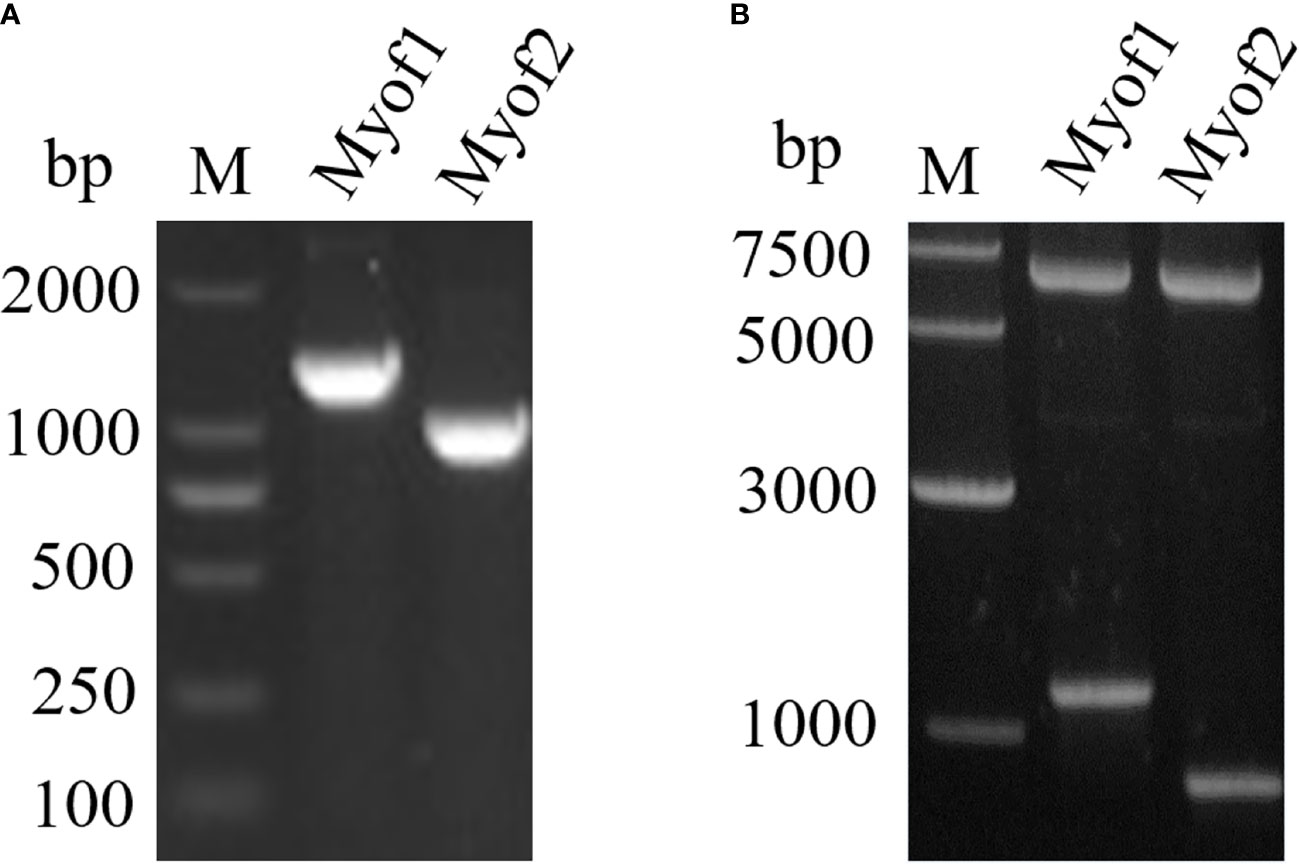
Figure 5 Amplification and cloning of Myof1 and Myof2. (A) The amplified genes of Myof1 and Myof2 (1500 bp and 810 bp) using specific oligonucleotide primers. (B) Cloning of the coding sequence for Myof1 and Myof2 into the pET32a vector; the inserted DNA was digested with BamHI and XhoI.
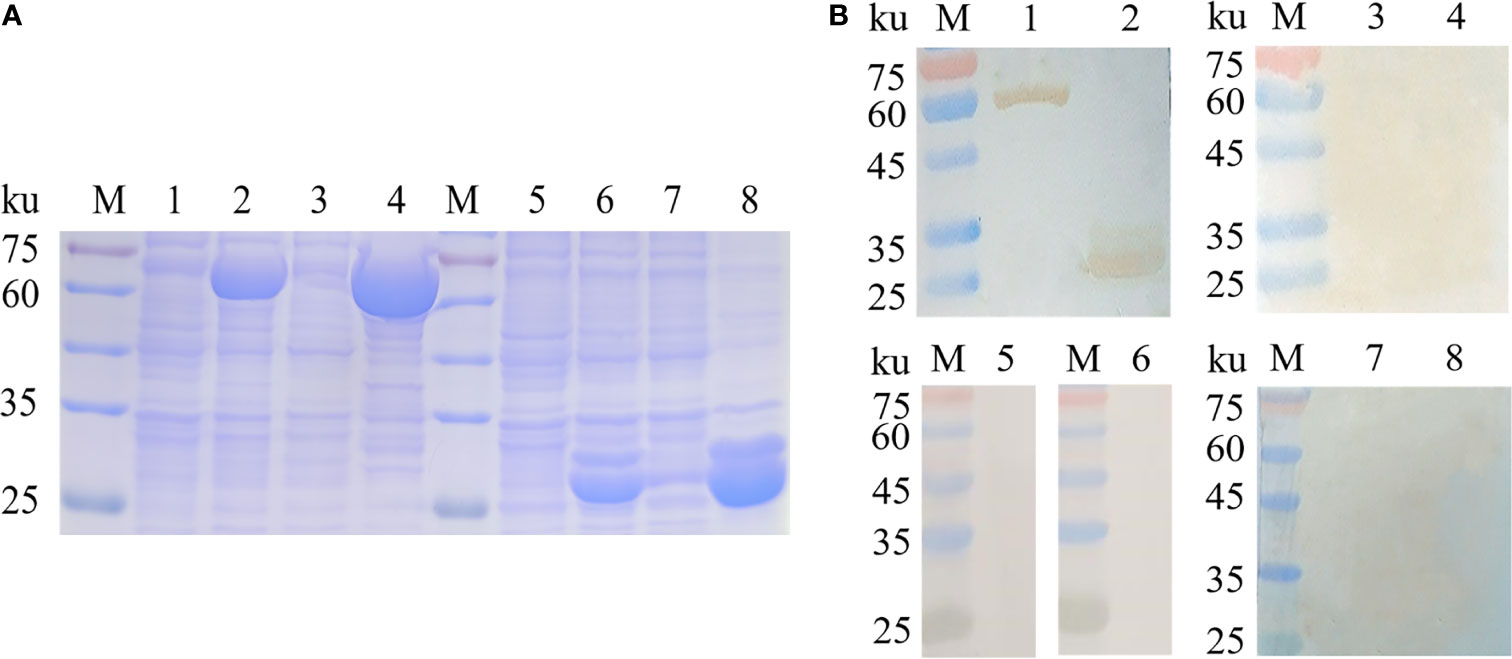
Figure 6 Expression and antigenicity analysis of Myof1 and Myof2. (A) The recombinant proteins, Myof1 and Myof2, were subjected to SDS-PAGE (12% gel). M: standard molecular size; Line 1: Un-induced recombinant Myof1-C/BL21; Line 2: Induced recombinant pET-32a-Myof1-C/BL21 with IPTG; Lines 4-5: Supernatant and precipitate of the expression product from the recombinant pET-32a-Myof1 with IPTG induction, respectively. Line 5: Un-induced recombinant Myof2-C/BL21; Line 6: Induced recombinant pET-32a-Myof2-C/BL21 with IPTG; Lines 7-8: Supernatant and precipitate of the expression product from the recombinant pET-32a-Myof2 with IPTG induction, respectively. (B) The recombinant proteins reacted with different sera. M: standard molecular size; Lines 1-2: The recombinant proteins, Myof1 and Myof2, incubated with positive sera of the rabbit infected with C sinensis; Lines 3-4: Negative serum of rabbit as a control. Lines 5-6: The recombinant proteins, Myof1 and Myof2, incubated with positive sera of the rabbit infected with S. japonicum. Lines 7-8: The recombinant proteins, Myof1 and Myof2, incubated with positive sera of the rabbit infected with F. hepatica.
Discussion
Clonorchiasis, a foodborne trematodiasis, is an emerging public health problem in China, Korea, and Vietnam (Na et al., 2020). Direct parasite irritation in the bile duct epithelium can cause not only mechanical irritation, but also chemical impairment. Epidemiological and experimental studies have reported that C. sinensis infections can induce biliary epithelial hyperplasia, periductal fibrosis, and cystic changes in the ducts, and may also facilitate the development of cholangiocarcinoma (Qian et al., 2016). ESPs, consisting of a complex mixture of proteins, carbohydrates, and lipids, are generally considered to play important roles in host-parasite interactions, including invasion, digestion, detoxification, and immune evasion (Kim et al., 2021). C. sinensis continuously releases ESPs (complex mixture of proteins, carbohydrates, lipids, etc.) from the excretory orifice to the outside. Cells exposed to ESPs exert a variety of pathophysiological reactions, including proliferation, apoptosis, destruction of redox homeostasis, and inflammation (Serradell et al., 2007; Kim et al., 2008a). The transcriptomic and proteomic profiles of human cholangiocarcinoma cells treated with ESPs demonstrated that host mRNA was upregulated or downregulated to varying degrees; upregulated genes were related to tumorigenesis, cell proliferation, and differentiation, while downregulated genes were related to apoptosis (Pak et al., 2014). Identification of proteins in ESPs is thus crucial to our understanding of the mechanisms underlying parasite-induced pathogenesis. Proteomic analysis of C. sinensis ESPs revealed that the identified proteins have high immunogenicity and certain specificity, such as detoxifying enzymes, myoglobin proteases, and grain-like growth factors (Mulvenna et al., 2010). Cathepsin F is the major protein in ESPs at 0-3 h, and the main protein of enzymatic proteolytic activity (Kang et al., 2010). ESPs at 0-5 h regulate the proliferation and apoptosis of cholangiocarcinoma cells (Kim et al., 2008b). Cysteine, ESPs at 0-12 h, induce cytotoxicity (Pak et al., 2009). ESPs from C. sinensis are directly exposed to the host immune system and are widely used as antigens in serological assays. Further, the antigens of ESPs tend to be superior to those of crude extracts in C. sinensis for serodiagnosis of clonorchiasis. Methionine aminopeptidase 2 acid phosphatase, and fructose-1,6-bisphosphatase were found to display high specificity and sensitivity, indicating that they are potential diagnostic antigens (Zheng et al., 2011; Zheng et al., 2013).
Although many studies have revealed the components of ESPs at different periods of C. sinensis, few studies have examined the relationship between ESPs and sera at different infection periods. Such finding can not only help us identify the key proteins that interact with sera, but also establish an early diagnosis method for clonorchiasis. Five proteins were found in this study, which were only present in the7 dpi interacting with ESPs. Among them, the cleavage and polyadenylation specificity factor proteins exist widely in many organisms (Shi and Manley, 2015). Most mRNA precursors (pre-mRNAs) are cleaved and polyadenylated at the 3’ end prior to their export from the nucleus (Sun et al., 2020). This sequence of events is carefully orchestrated, providing both a tight regulation of cleavage/polyadenylation and the opportunity to select from multiple cleavage sites with various affinities (Gruber and Zavolan, 2019). Switching between these sites can lead to changes in the length and sequence of the 3’ UTR of mRNAs, which has many effects on protein expression, mRNA stability, and localization (El Mouali and Balsalobre, 2019). Acetylornithine deacetylase is mainly involved in the ornithine cycle, and the formation of N-acetyl-glutamate from glutamate and acetyl-CoA in a reaction catalyzed by N-acetyl-glutamate synthase (Javid-Majd and Blanchard, 2000). NOD-like receptors are a large family of 22 intracellular proteins in humans that perform a diverse array of cellular functions and play key roles in the regulation of innate immune responses (Lupfer et al., 2020).
To date, ESPs have been known to contain sensitive antigens for the diagnosis of clonorchiasis, and popular antigenic candidates are yet to be characterized in detail. In this study, proteomic and Co-IP assays were used to pull down three types of serum: C. sinensis, F. hepatica, and S. japonicum interacting with the ESPs of C. sinensis. Proteins specific to C. sinensis were identified by cross-screening using shotgun LC-MS/MS. Due to the limitations of the existing database, only 254 of the 334 proteins were annotated in the total mass spectrum of ESPs. We identified multiple proteins from positive serum samples of C. sinensis at different infection periods, and the functions of the identified proteins were classified based on various biological processes. Protein relative pathway analysis revealed that these proteins were mainly involved in oxidative phosphorylation, signal transduction, and metabolism, thereby playing important roles in the interaction between parasites and hosts. Among these proteins, we focused on Myoferlin, one of the three proteins detected in the serum collected at the four infection periods. Myoferlin, which belongs to the ferlin family, is an evolutionarily conserved family of vesicle fusion proteins that is reported to be involved in myoblast fusion, vesicle trafficking, and plasma membrane integrity (Turtoi et al., 2013). Recent studies have shown that myoferlin is overexpressed in several human cancers and enhances tumor progression by regulating migration, invasion, and tumorigenesis (Zhang et al., 2018). Myoferlin is highly involved in oxaliplatin resistance and tumor progression in gastric cancer. The protein could be a promising biomarker and a therapeutic target for cases of oxaliplatin-resistant gastric cancer (Gupta et al., 2021). Studies have shown that myoferlin is also involved in pivotal physiological functions related to numerous cell membranes, such as extracellular secretion, endocytosis, vesicle trafficking, membrane repair, membrane receptor recycling, and secreted protein efflux (Gu et al., 2020).
However, the function of myoferlin in clonorchiasis, especially its effects on detection sensitivity, has received little attention. Thus, we cloned and expressed both Myof1 and Myof2 of the protein to determine their reactogenicity. According to the cross-reactivity analysis results, both Myof1 and Myof2 did not show any cross-reactivity with the sera from F. hepatica, S. japonicum, and uninfected rabbits. Further, both parts of myoferlin demonstrated a higher degree of specificity to the sera of infected rabbits. Our findings indicate that the immuno-proteomic approaches used in this study could have a significant effect on the identification of serodiagnostic antigens against clonorchiasis. Additionally, myoferlin may be a potential protein for the immunological diagnosis of clonorchiasis.
Data Availability Statement
The datasets presented in this study can be found in online repositories. The names of the repository/repositories and accession number(s) can be found below: http://www.proteomexchange.org/, PXD028236.
Ethics Statement
The animal study was reviewed and approved by Animal Health, Animal Care and Use Committee of Heilongjiang Bayi Agricultural University.
Author Contributions
C-RW and Q-CC designed the project and experiments. X-XM and Y-YQ conducted the experiments. Z-GC and R-RJ analyzed the data. C-LL and J-FG made the images. X-XM, Y-YQ and Q-CC prepared the manuscript. All authors contributed to the article and approved the submitted version.
Funding
This work was supported by the National Natural Science Foundation of China (Grant Nos. 31672399, 81802869 and 32172886), Heilongjiang Postdoctoral Science Foundation (Grant No. LBH-Z19191), and the Heilongjiang Provincial Postdoctoral Natural Science Foundation of China (Grant No. LH2021C071).
Conflict of Interest
The authors declare that the research was conducted in the absence of any commercial or financial relationships that could be construed as a potential conflict of interest.
Publisher’s Note
All claims expressed in this article are solely those of the authors and do not necessarily represent those of their affiliated organizations, or those of the publisher, the editors and the reviewers. Any product that may be evaluated in this article, or claim that may be made by its manufacturer, is not guaranteed or endorsed by the publisher.
Acknowledgments
We thank Qinglian Biotech Co., Ltd. (Beijing, China) for providing the experimental equipment and commercial database, and the Laboratory Animal Center of Shanghai Veterinary Research Institute, Chinese Academy of Agriculture Sciences for providing the sera positive for S. japonicum.
Supplementary Material
The Supplementary Material for this article can be found online at: https://www.frontiersin.org/articles/10.3389/fcimb.2021.779259/full#supplementary-material
Supplementary Figure 1 | Mass spectrometry analysis of the ESP components. (A) SDS-PAGE analysis of the total ESPs of C. sinensis. (B) Comparative analysis between the identified proteins and C. sinensis protein library.
Supplementary Figure 2 | Domains and interacting proteins prediction of myoferlin. (A) The domains predetermination of myoferlin. (B) The protein network interaction predetermination of myoferlin.
References
Cho, P. Y., Lee, J. Y., Kim, T. I., Song, J. H., Hong, S. J., Yoo, W. G., et al. (2020). Serodiagnostic Antigens of Clonorchis Sinensis Identified and Evaluated by High-Throughput Proteogenomics. PLoS Negl. Trop. Dis. 14, e0008998. doi: 10.1371/journal.pntd.0008998
El Mouali, Y., Balsalobre, C. (2019). 3′Untranslated Regions: Regulation at the End of the Road. Curr. Genet. 65, 127–131. doi: 10.1007/s00294-018-0877-x
Gruber, A. J., Zavolan, M. (2019). Alternative Cleavage and Polyadenylation in Health and Disease. Nat. Rev. Genet. 20, 599–614. doi: 10.1038/s41576-019-0145-z
Guillou, F., Roger, E., Moné, Y., Rognon, A., Grunau, C., Théron, A., et al. (2007). Excretory-Secretory Proteome of Larval Schistosoma Mansoni and Echinostoma Caproni, Two Parasites of Biomphalaria Glabrata. Mol. Biochem. Parasitol. 155, 45–56. doi: 10.1016/j.molbiopara.2007.05.009
Gu, H. J., Peng, Y. R., Chen, Y. H. (2020). An Emerging Therapeutic Approach by Targeting Myoferlin (MYOF) for Malignant Tumors. Curr. Top. Med. Chem. 20, 1509–1515. doi: 10.2174/1568026620666200618123436
Gupta, S., Yano, J., Mercier, V., Htwe, H. H., Shin, H. R., Rademaker, G., et al. (2021). Lysosomal Retargeting of Myoferlin Mitigates Membrane Stress to Enable Pancreatic Cancer Growth. Nat. Cell Biol. 23, 232–242. doi: 10.1038/s41556-021-00644-7
Hong, S. J., Kim, T. Y., Song, K. Y., Sohn, W. M., Kang, S. Y. (2001). Antigenic Profile and Localization of Clonorchis Sinensis Proteins in the Course of Infection. Korean J. Parasitol. 39, 307–312. doi: 10.3347/kjp.2001.39.4.307
Hong, S. J., Yun Kim, T., Gan, X. X., Shen, L. Y., Sukontason, K., Sukontason, K., et al. (2002). Clonorchis Sinensis: Glutathione s-Transferase as a Serodiagnostic Antigen for Detecting Igg and Ige Antibodies. Exp. Parasitol. 101, 231–233. doi: 10.1016/s0014-4894(02)00112-1
Huang, S. Y., Yue, D. M., Hou, J. L., Zhang, X. X., Zhang, F. K., Wang, C. R., et al. (2019). Proteomic Analysis of Fasciola Gigantica Excretory and Secretory Products (Fgesps) Interacting With Buffalo Serum of Different Infection Periods by Shotgun LC-MS/MS. Parasitol Res. 118, 453–460. doi: 10.1007/s00436-018-6169-z
Javid-Majd, F., Blanchard, J. S. (2000). Mechanistic Analysis of the ARGE-Encoded N-Acetylornithine Deacetylase. Biochemistry 39, 1285–1293. doi: 10.1021/bi992177f
Ju, J. W., Joo, H. N., Lee, M. R., Cho, S. H., Cheun, H. I., Kim, J. Y., et al. (2009). Identification of a Serodiagnostic Antigen, Legumain, by Immunoproteomic Analysis of Excretory-Secretory Products of Clonorchis Sinensis Adult Worms. Proteomics 9, 3066–3078. doi: 10.1002/pmic.200700613
Kang, J. M., Bahk, Y. Y., Cho, P. Y., Hong, S. J., Kim, T. S., Sohn, W. M., et al. (2010). A Family of Cathepsin F Cysteine Proteases of Clonorchis Sinensis is the Major Secreted Proteins That are Expressed in the Intestine of the Parasite. Mol. Biochem. Parasitol. 170, 7–16. doi: 10.1016/j.molbiopara.2009.11.006
Kim, Y. J., Choi, M. H., Hong, S. T., Bae, Y. M. (2008a). Proliferative Effects of Excretory/Secretory Products From Clonorchis Sinensis on the Human Epithelial Cell Line HEK293 via Regulation of the Transcription Factor E2F1. Parasitol Res. 102, 411–417. doi: 10.1007/s00436-007-0778-2
Kim, E. M., Kim, J. S., Choi, M. H., Hong, S. T., Bae, Y. M. (2008b). Effects of Excretory/Secretory Products From Clonorchis Sinensis and the Carcinogen Dimethylnitrosamine on the Proliferation and Cell Cycle Modulation of Human Epithelial HEK293T Cells. Korean J. Parasitol. 46, 127–132. doi: 10.3347/kjp.2008.46.3.127
Kim, J. W., Yi, J., Park, J., Jeong, J. H., Kim, J., Won, J., et al. (2021). Transcriptomic Profiling of Three-Dimensional Cholangiocyte Spheroids Long Term Exposed to Repetitive Clonorchis Sinensis Excretory-Secretory Products. Parasit Vectors. 14, 213. doi: 10.1186/s13071-021-04717-2
Lee, E. G., Na, B. K., Bae, Y. A., Kim, S. H., Je, E. Y., Ju, J. W., et al. (2006). Identification of Immunodominant Excretory-Secretory Cysteine Proteases of Adult Paragonimus Westermani by Proteome Analysis. Proteomics 6, 1290–1300. doi: 10.1002/pmic.200500399
Li, S., Chen, X., Zhou, J., Xie, Z., Shang, M., He, L., et al. (2020). Amino Acids Serve as an Important Energy Source for Adult Flukes of Clonorchis Sinensis. PLoS Negl. Trop. Dis. 14, e0008287. doi: 10.1371/journal.pntd.0008287
Li, S. Y., Chung, B. S., Choi, M. H., Hong, S. T. (2004). Organ-Specific Antigens of Clonorchis Sinensis. Korean J. Parasitol. 42, 169–174. doi: 10.3347/kjp.2004.42.4.169
Liu, F., Cui, S. J., Hu, W., Feng, Z., Wang, Z. Q., Han, Z. G. (2009). Excretory/Secretory Proteome of the Adult Developmental Stage of Human Blood Fluke, Schistosoma Japonicum. Mol. Cell Proteomics. 8, 1236–1251. doi: 10.1074/mcp.M800538-MCP200
Lupfer, C. R., Anand, P. K., Qi, X. P., Zaki, H. (2020). Editorial: Role of NOD-Like Receptors in Infectious and Immunological Diseases. Front. Immunol. 11, 923. doi: 10.3389/fimmu.2020.00923
Mulvenna, J., Sripa, B., Brindley, P. J., Gorman, J., Jones, M. K., Colgrave, M. L., et al. (2010). The Secreted and Surface Proteomes of the Adult Stage of the Carcinogenic Human Liver Fluke Opisthorchis Viverrini. Proteomics 10, 1063–1078. doi: 10.1002/pmic.200900393
Na, B. K., Pak, J. H., Hong, S. J. (2020). Clonorchis Sinensis and Clonorchiasis. Acta Trop. 203, 105309. doi: 10.1016/j.actatropica.2019.105309
Pak, J. H., Bashir, Q., Kim, I. K., Hong, S. J., Maeng, S., Bahk, Y. Y., et al. (2017). Clonorchis Sinensis Excretory-Secretory Products Promote the Migration and Invasion of Cholangiocarcinoma Cells by Activating the Integrin β4-FAK/Src Signaling Pathway. Mol. Biochem. Parasitol. 06, 214. doi: 10.1016/j.molbiopara.2017.03.002
Pak, J. H., Kim, I. K., Kim, S. M., Maeng, S., Song, K. J., Na, B. K., et al. (2014). Induction of Cancer-Related MicroRNA Expression Profiling Using Excretory-Secretory Products of Clonorchis Sinensis. Parasitol Res. 113, 4447–4455. doi: 10.1007/s00436-014-4127-y
Pak, J. H., Moon, J. H., Hwang, S. J., Cho, S. H., Seo, S. B., Kim, T. S. (2009). Proteomic Analysis of Differentially Expressed Proteins in Human Cholangiocarcinoma Cells Treated With Clonorchis Sinensis Excretory-Secretory Products. J. Cell Biochem. 108, 1376–1388. doi: 10.1002/jcb.22368
Pino, H. S., Petitt, M., Beckstead, J. H., McKerrow, J. H. (1986). Preparation of Mouse Monoclonal Antibodies and Evidence for a Host Immune Response to the Preacetabular Gland Proteinase of Schistosoma Mansoni Cercariae. Am. J. Trop. Med. Hyg. 35, 536–543. doi: 10.4269/ajtmh.1986.35.536
Qian, M. B., Utzinger, J., Keiser, J., Zhou, X. N. (2016). Clonorchiasis. Lancet 387, 800–810. doi: 10.1016/S0140-6736(15)60313-0
Serradell, M. C., Guasconi, L., Cervi, L., Chiapello, L., Masih, D. T. (2007). Excretory-Secretory Products From Fasciola Hepatica Induce Eosinophil Apoptosis by a Caspase-Dependent Mechanism. Vet. Immunol. Immunopathol. 117, 197–208. doi: 10.1016/j.vetimm.2007.03.007
Shi, Y., Manley, J. L. (2015). The End of the Message: Multiple Protein-RNA Interactions Define the mRNA Polyadenylation Site. Genes Dev. 29, 889–897. doi: 10.1101/gad.261974.115
Sun, Y., Hamilton, K., Tong, L. (2020). Recent Molecular Insights Into Canonical Pre-mRNA 3′-End Processing. Transcription 11, 83–96. doi: 10.1080/21541264.2020.1777047
Tang, Z. L., Huang, Y., Yu, X. B. (2016). Current Status and Perspectives of Clonorchis Sinensis and Clonorchiasis: Epidemiology, Pathogenesis, Omics, Prevention and Control. Infect. Dis. Poverty. 5, 71. doi: 10.1186/s40249-016-0166-1
Turtoi, A., Blomme, A., Bellahcène, A., Gilles, C., Hennequière, V., Peixoto, P., et al. (2013). Myoferlin is a Key Regulator of EGFR Activity in Breast Cancer. Cancer Res. 73, 5438–5448. doi: 10.1158/0008-5472.CAN-13-1142
Véronique, B., Robert, B., Kurt, S., Yann, G., Béatrice, S., Fatiha, E. G., et al. (2009). A Review of Human Carcinogens-Part B: Biological Agents. Lancet Oncol. 10, 321–322. doi: 10.1016/s1470-2045(09)70096-8
Zhang, T., Li, J., He, Y., Yang, F., Hao, Y., Jin, W., et al. (2018). A Small Molecule Targeting Myoferlin Exerts Promising Anti-Tumor Effects on Breast Cancer. Nat. Commun. 9, 3726. doi: 10.1038/s41467-018-06179-0
Zheng, M., Hu, K., Liu, W., Hu, X., Hu, F., Huang, L., et al. (2011). Proteomic Analysis of Excretory Secretory Products From Clonorchis Sinensis Adult Worms: Molecular Characterization and Serological Reactivity of a Excretory-Secretory Antigen-Fructose-1,6-Bisphosphatase. Parasitol Res. 109, 737–744. doi: 10.1007/s00436-011-2316-5
Zheng, M., Hu, K., Liu, W., Li, H., Chen, J., Yu, X. (2013). Proteomic Analysis of Different Period Excretory Secretory Products From Clonorchis Sinensis Adult Worms: Molecular Characterization, Immunolocalization, and Serological Reactivity of Two Excretory Secretory Antigens-Methionine Aminopeptidase 2 and Acid Phosphatase. Parasitol Res. 112, 1287–1297. doi: 10.1007/s00436-012-3264-4
Keywords: Clonorchis sinensis, ESPs, Co-IP, myoferlin, diagnosis
Citation: Ma X-X, Qiu Y-Y, Chang Z-G, Gao J-F, Jiang R-R, Li C-L, Wang C-R and Chang Q-C (2021) Identification of Myoferlin, a Potential Serodiagnostic Antigen of Clonorchiasis, via Immunoproteomic Analysis of Sera From Different Infection Periods and Excretory-Secretory Products of Clonorchis sinensis. Front. Cell. Infect. Microbiol. 11:779259. doi: 10.3389/fcimb.2021.779259
Received: 18 September 2021; Accepted: 04 October 2021;
Published: 18 October 2021.
Edited by:
Nian-Zhang Zhang, Lanzhou Veterinary Research Institute (CAAS), ChinaReviewed by:
Xi Zhang, Zhengzhou University, ChinaWen-Bin Zheng, Shanxi Agricultural University, China
Copyright © 2021 Ma, Qiu, Chang, Gao, Jiang, Li, Wang and Chang. This is an open-access article distributed under the terms of the Creative Commons Attribution License (CC BY). The use, distribution or reproduction in other forums is permitted, provided the original author(s) and the copyright owner(s) are credited and that the original publication in this journal is cited, in accordance with accepted academic practice. No use, distribution or reproduction is permitted which does not comply with these terms.
*Correspondence: Qiao-Cheng Chang, changqiaocheng2001@163.com
†These authors have contributed equally to this work and share first authorship