- 1Department of Parasitology, Harbin Medical University, Harbin, China
- 2Department of Orthopaedic Surgery, The Fourth Affiliated Hospital of Harbin Medical University, Harbin, China
- 3Clinical Laboratory, Zhuhai Maternal and Child Health Hospital, Zhuhai, China
- 4Department of Stomatology, Laixi People’s Hospital, Qingdao, China
Clonorchiasis is recognized as an important zoonotic parasitic disease worldwide. However, the roles of host long non-coding RNAs (lncRNAs) and messenger RNAs (mRNAs) in the response to Clonorchis sinensis (C. sinensis) infection remain unknown. Here we compared the expression of lncRNAs and mRNAs in the liver tissue of mice infected with C. sinensis, in order to further understand the molecular mechanisms of clonorchiasis. A total of 388 lncRNAs and 1,172 mRNAs were found to be differentially expressed with absolute value of fold change (FC) ≥ 2.0 and p < 0.05 by microarray. Compared with controls, Gm6135 and 4930581F22Rik were the most over- and under-expressed lncRNAs; flavin-containing monooxygenase 3 (Fmo3) and deleted in malignant brain tumors 1 (Dmbt1) were the most over- and under-expressed mRNAs. Moreover, functional annotation showed that the significantly different mRNAs were related with “FOXO signaling pathway”, “Wnt signaling pathway”, and “AMPK signaling pathway”. Remarkably, lncRNA Gm8801 were significantly correlated with mRNA glycerol-3-phosphate acyltransferase mitochondrial (Gpam), insulin receptor substrate 2 (Irs2), and tumor necrosis factor receptor superfamily member 19 (Tnfrsf19) in ceRNA networks. These results showed that the expression profiles of lncRNAs and mRNAs in the liver changed after C. sinensis infection. Our results provided valuable insights into the lncRNAs and mRNAs involved in clonorchiasis pathogenesis, which may be useful for future control strategies.
Introduction
Clonorchiasis, caused by Clonorchis sinensis (C. sinensis), is an emerging zoonotic parasitic disease worldwide, distributed mainly in China, Korea, and northern Vietnam (Han et al., 2012; Lai et al., 2016). C. sinensis, belonging to family Opisthorchiidae (Ovchinnikov et al., 2015), has been classified by the International Agency for Research on Cancer (IARC) as a group 1 biocarcinogen in humans in 2009 (Bouvard et al., 2009). Humans become infected by eating freshwater fish with C. sinensis metacercariae. Metacercariae exist in the small intestine and then migrate via the ampulla of Vater to the biliary ducts (Lun et al., 2005). The adult fluke inhabits the biliary passages of humans and mammals and could cause epithelial hyperplasia of the biliary mucosa and even periductal fibrosis (Chung et al., 2004). Clinical manifestations of clonorchiasis vary from asymptomatic infections to severe morbidity and mortality, including epithelial hyperplasia, cholecystitis, periductal fibrosis, hepatic fibrosis, and cholangiocarcinoma (Keiser and Utzinger, 2005). Previously, we found that hepatic apoptosis and iron overload were involved in clonorchiasis (Han et al., 2017). In addition, an aberrant expression of hepatic microRNA has been associated with clonorchiasis (Han et al., 2016). However, the mechanism of liver/biliary injury, and whether other regulatory mechanisms or other small molecules are involved, remains unclear. Therefore, exploring the molecular mechanisms underlying clonorchiasis may contribute to the development of prevention measures and targeted drugs.
The majority of long non-coding RNAs (lncRNAs) are transcribed by RNA polymerase II as the product of alternative cleavage and splicing, with sizes greater than 200 nt in length (Quinn and Chang, 2016; Yamamura et al., 2018). LncRNAs are broadly classified into those that regulate gene expression in cis and in trans (Kopp and Mendell, 2018). Recently, lncRNAs are identified to bind with proteins, RNA, DNA, or their combination to play their functions (Fasolo et al., 2019; Hu et al., 2019). Acting as miRNA sponge, decoys, scaffolds, guides, and posttranslation regulators, lncRNAs could participate in various biological processes (Rinn and Chang, 2012; Mumtaz et al., 2017; Fernandes et al., 2019). Accumulating studies showed that lncRNAs were involved in inflammation, cancer, and autoimmunity (Fitzgerald and Caffrey, 2014; Marques-Rocha et al., 2015; Renganathan and Felley-Bosco, 2017).
Recent studies found that parasite infection changed the expression profiles of lncRNAs in the host. For example, numerous lncRNAs were differentially expressed in S. japonicum-infected liver and spleen compared to control (Xia et al., 2020). Furthermore, differentially expressed lncRNAs and their predicted target mRNAs were found associated with immunology and liver pathology (Xia et al., 2020). Besides, lncRNA WNT5b and H19 were found downregulated to regulate the metabolic reprogramming and differentiation of adipocytes in the protoscoleces of Echinococcus granulosus-infected mice (Lu et al., 2020). LncRNAs were also identified as important regulatory factors in regulating immunological stress during cystic echinococcosis (Yu et al., 2018). However, knowledge of lncRNAs and their biological functions in clonorchiasis remains limited.
This study used microarray analysis to assess the expression of lncRNAs and mRNAs in the liver tissue of mice infected with C. sinensis. Next, we analyzed the possible biological processes and pathways associated with C. sinensis infections. Our findings support this powerful new approach for the elucidation of the molecular mechanisms of clonorchiasis.
Methods
Mice, Parasites, and Infection
C. sinensis metacercariae were harvested from the cyprinid Pseudorasbora parva captured in Songhuajiang River, an endemic area of C. sinensis infection in China. Metacercariae were collected as described previously (Han et al., 2017). In brief, the fish were digested with a pepsin-HCl (0.6%) artificial gastric juice at 37°C for 12 h with vigorous shaking. With mesh sizes of 1,000 μm, 300 μm, and 106 μm, the digested mixture was collected and added to the conical-shaped glass and kept standing for 30 min. After discarding the supernatant, the precipitate was transferred to a glass dish and then the metacercariae were collected using a capillary pipette under a dissection microscope.
Male BALB/c mice (aged 8 weeks, 20 g) were obtained from the Harbin Medical University Laboratory Animal Center (Harbin, China). All the animals were housed in an air-conditioned room at 24°C under a 12-h dark/light cycle with free access to standard laboratory food and water. Mice were divided into two groups randomly with three mice per group, and each mice in the infected group was orally infected with 100 metacercariae, while the control group was given the same volume of 0.9% NaCl. The detection of eggs in feces indicates the successful establishment of an animal model of C. sinensis infection (Hong et al., 2003). Thus, feces were collected every day and microscopically examined by the Kato–Katz method (Hong et al., 2003).
C. sinensis undergoes rapid development, and the adults develop matured at 4 week postinfection (wpi) after being infected by metacercariae (Lun et al., 2005). Our previous study also found hepatocyte apoptosis and iron overload in C. sinensis-infected rats at 4 wpi (Zhang et al., 2008; Han et al., 2017). Therefore, the mice in both infected and control groups were sacrificed under ether anesthesia by cervical dislocation at 4 wpi. Then, livers were removed and immediately stored at -80°C after being washed by nuclease-free PBS. All animal care and experimental procedures were conducted according to the guidelines for animal use in toxicology. The study protocol was approved by the Animal Care and Use Committee of the Harbin Medical University.
RNA Extraction
Total RNA was extracted from 100 mg of tissue using TRIzol Reagent (Life Technologies, Carlsbad, CA, USA) following the manufacturer’s instructions. RNA quantity was measured using a SmartSpec Plus spectrophotometer (Bio-Rad, Hercules, CA, USA). RNA purity was evaluated by the A260/A280 ratio. Total RNA was quantified using a NanoDrop ND-2000 (Thermo Scientific, Waltham, MA, USA), and RNA integrity was assessed using an Agilent Bioanalyzer 2100 (Agilent Technologies, Santa Clara, CA, USA).
Microarray Analysis
Three pairs of liver samples were selected for lncRNA microarray analysis using an Agilent Mouse Gene Expression Array (8*60K, design ID: 028005). The microarray contains 55,681 probes for 39,430 mouse mRNA and 16,251 mouse lncRNAs, which are derived from RefSeq Build 37, Ensemble Release 55, Unigene Build 176, GenBank (Apr 2009), and RIKEN 3. Sample preparation, microarray hybridization, and washing were performed based on the manufacturer’s standard protocols with minor modifications. After that, the arrays were scanned using an Agilent Scanner G2505C microarray scanner (Agilent Technologies). Raw data were extracted using Feature Extraction (version 10.7.1.1; Agilent Technologies). The microarray profiling was conducted in the laboratory of the OE Biotechnology Company in Shanghai, People’s Republic of China.
Bioinformatics Analysis
Firstly, quantile normalization and subsequent data processing were done through GeneSpring software (version 12.0; Agilent Technologies). Differentially expressed genes (mRNAs and lncRNAs) were identified by the absolute value of (FC) > 2 and p value < 0.05 (Student’s t test). Gene ontology (GO) analysis was used to identify the potential biological functions of the differentially expressed mRNAs (www.geneontology.org). Biological process (BP), cellular component (CC), and molecular function (MF) were involved in the GO terms. Fisher’s exact test was applied to classify the GO categories. Enrichment scores were calculated based on log10 (p-value). The lower the p-value, the more significant the GO term (p < 0.05 is required). Furthermore, the Kyoto Encyclopedia of Genes and Genomes (KEGG) pathway analysis (http://www.kegg.jp) was applied to predict the possible pathways. Hierarchical clustering was used to display the distinguishable genes’ expression pattern among six liver tissue samples (Cluster 3.0 and TreeView 2.0).
In order to further predict the potential regulatory relationship between mRNA and lncRNA, a ceRNA network was constructed by combining our previous miRNA expression profiling date (Additional File: Table S1). The processing of construction was as follows: i) Pearson’s correlation coefficients were used for analyzing lncRNAs and their associated mRNAs, based on correlation coefficients ≥0.90 and p < 0.01 considered statistically significant (Additional File: Table S4). ii) mRNA and lncRNA shared at least 4 miRNAs. iii) p ≤ 0.05. Cytoscape software 3.1.1 (US National Institute of General Medical Sciences) was used for constructing the ceRNA network.
Quantitative Reverse Transcription-Polymerase Chain Reaction
Total RNA was extracted from the liver, and the integrity was evaluated using agarose gel electrophoresis stained with ethidium bromide. Quantitative reverse transcription-polymerase chain reaction (qRT-PCR) was performed on six differentially expressed mRNAs and five lncRNAs to confirm the results of the microarray analysis. Based on the protocols provided by the manufacturers, a SYBR Green RT-PCR Kit (TransStart Top Green qPCR SuperMix, TransGen Biotech Co., Ltd., Beijing, China) on a Light Cycler 96 system (Roche, Indianapolis, Indiana) was used for qRT-PCR. Primers for the lncRNAs and mRNAs are shown in Table 1. Threshold cycle (Ct) values were used to quantify the expression levels of lncRNAs and mRNAs. Glyceraldehyde 3-phosphate dehydrogenase (GAPDH) was used as an internal control for measurement of lncRNAs and mRNAs using the 2-ΔΔCt method. Three biological replicates were conducted for each sample.
Statistical Analysis
PRISM version 8.0 software (GraphPad, San Diego, CA, USA) was used for data analysis. All data are expressed as mean ± standard deviation (SD). Student’s t-tests were employed to analyze qRT-PCR data. p < 0.05 was considered statistically significant.
Results
Differentially Expressed lncRNAs and mRNAs
In order to explore the lncRNA and mRNA expression profiles in the liver in response to C. sinensis infection, microarray analysis was employed to compare expressions in uninfected and C. sinensis-infected liver tissues. A total of 388 lncRNAs (115 up and 273 down, Additional File: Table S2) and 1,172 mRNAs (687 up and 485 down, Additional File: Table S3) were found to be differentially expressed with absolute value of FC ≥ 2.0 and p < 0.05 by microarray. The differences in lncRNA and mRNA expression profiles between the two groups are shown in Figure 1. Compared with the control group, 4930581F22Rik (absolute value of FC = 140.37) was the most downregulated lncRNA, and Gm6135 (absolute value of FC = 44.27) was the most upregulated lncRNA in the infected group. Furthermore, Dmbt1 (absolute value of FC = 294.36) was the most downregulated mRNA, encoding DMBT1 protein, whereas Fmo3 (absolute value of FC = 703.50) was the most upregulated mRNA, encoding FMO3 protein. In order to further understand the similarities at the transcriptomic level, cluster analysis was performed on all the differentially expressed genes. The infected group and the control group clustered separately in the heat map (Figure 2). These results indicated that altered expressions of lncRNAs and mRNAs may play an important role in clonorchiasis.
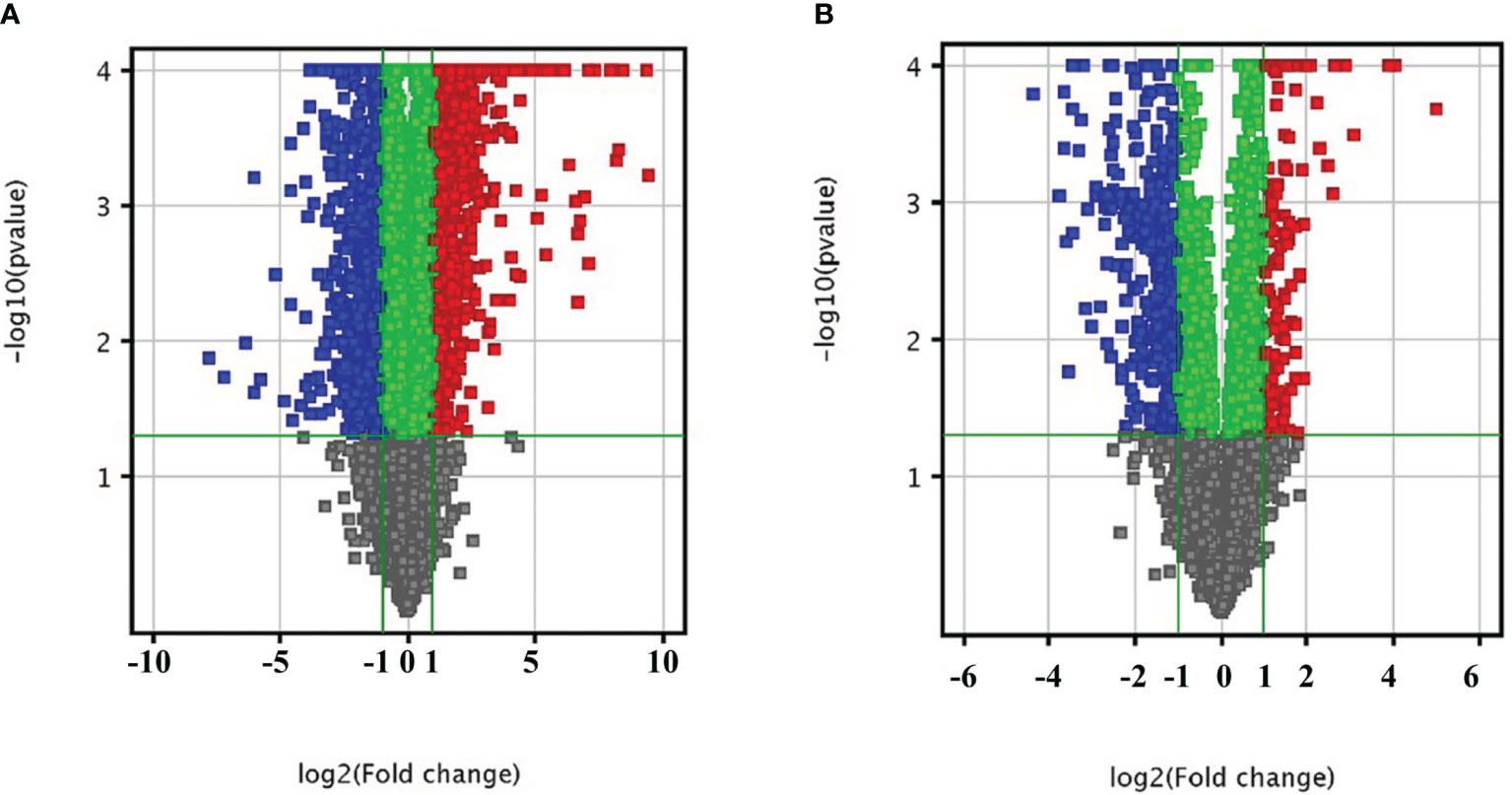
Figure 1 Bioinformatics analysis of differentially expressed mRNAs (A) and lncRNAs (B) in liver tissue infected with C. sinensis. The vertical axis corresponds to the negative logarithm of p value with base 10 (-log10(p value)), and the horizontal axis represents the logarithm of fold change with base 2 (log2(fold change)). The significantly up- and downregulated RNAs are presented as red or blue squares, respectively.
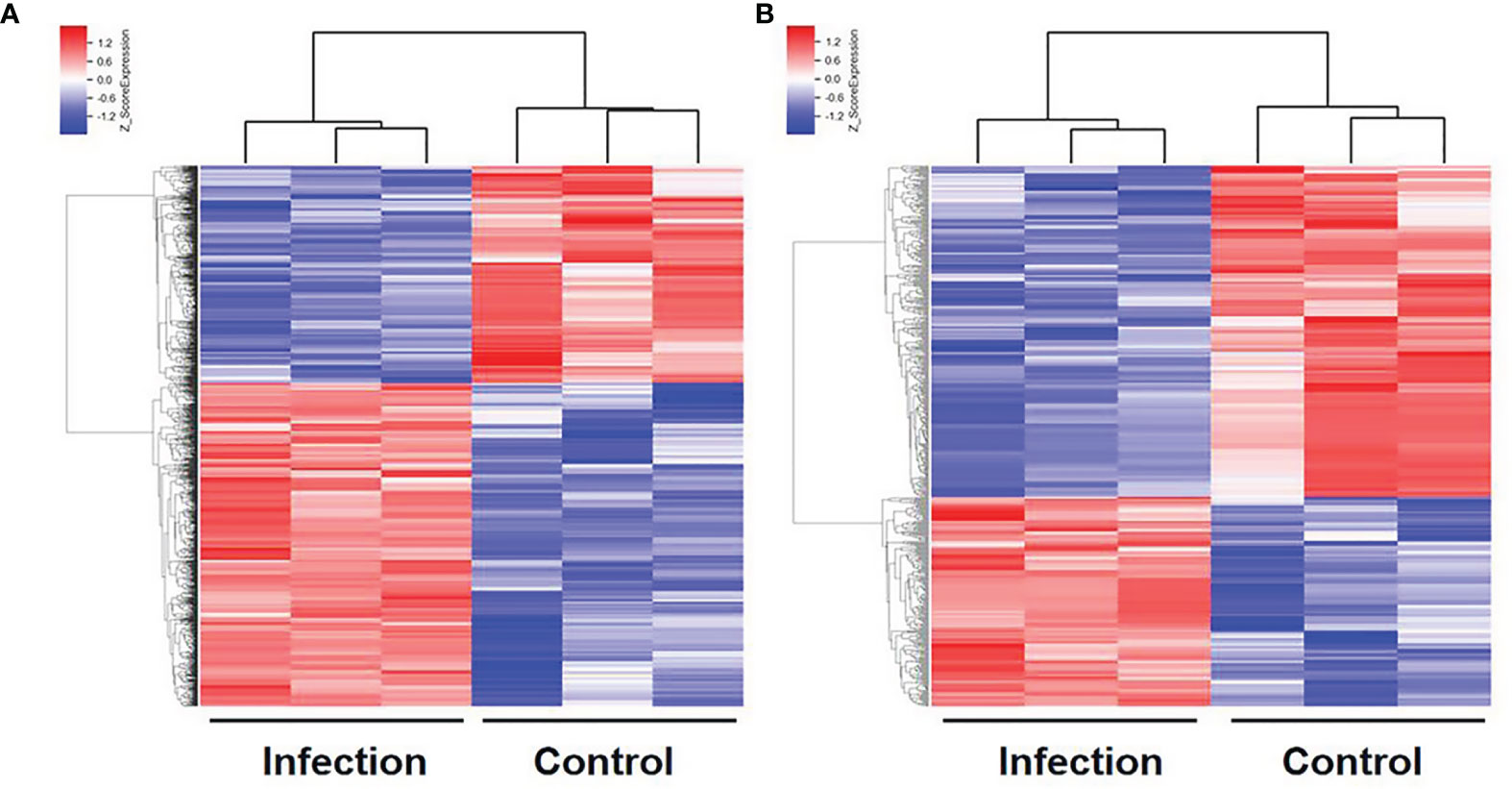
Figure 2 Hierarchical clustering of differentially expressed mRNAs (A) and lncRNAs (B) in the liver with C. sinensis infection. Blue indicates decreased relative expression, and red indicates increased relative expression.
The qRT-PCR Validation
To evaluate the reliability of microarray results, six mRNAs (Pax2, Btg2, Dmbt1, Irs2, Tnfrsf19, and Gpam) and five lncRNAs (Fate1, Meg3, Snhg3, Gm6135, and Gm8801) were selected for qRT-PCR. Dmbt1 was the most downregulated mRNA, whereas GM6135 was the most upregulated lncRNA. The lncRNA Gm8801 was chosen because it was strongly correlated with mRNA Gapm, Irs2, and Tnfrsf19 in our ceRNA network. Beside, two mRNAs (Pax2 and Btg2) and three lncRNAs (Fate1, Meg3, and Snhg3) were randomly chosen. The qRT-PCR results were consistent with the results of the microarray (Figure 3).
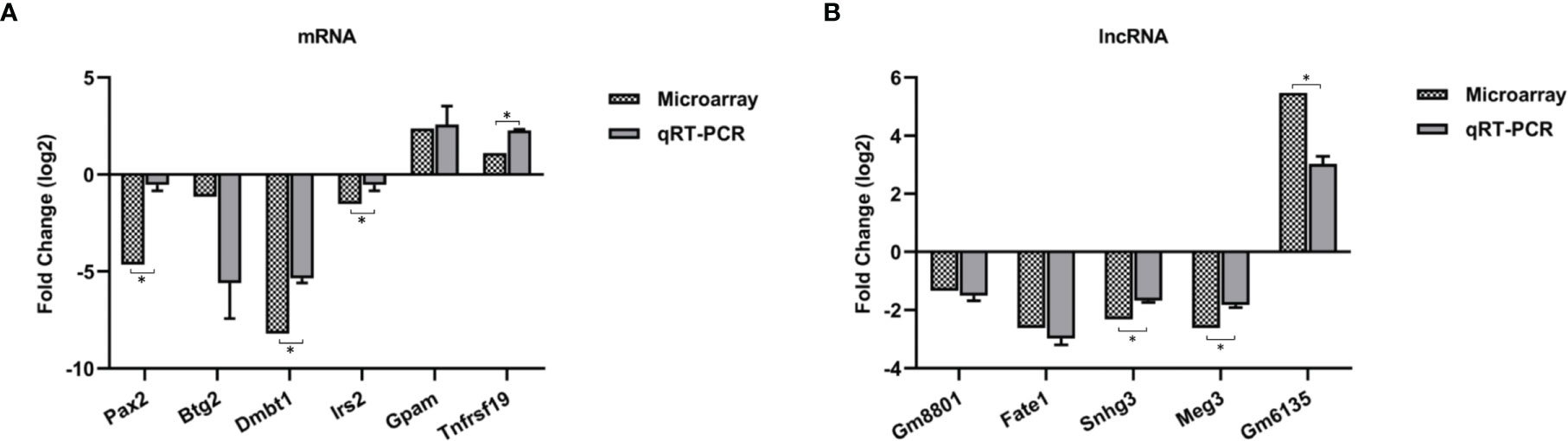
Figure 3 Validation for the expression of 6 differentially expressed mRNAs (A) and 5 lncRNAs (B) by qRT-PCR. Three biological repeats were included in each gene. *p < 0.05.
GO and KEGG Enrichment Analyses
GO analysis and KEGG enrichment analysis were applied to assess the function roles of differentially expressed mRNAs. Figure 4 shows the top 20 terms in the gene enrichment and pathway analyses of differentially expressed mRNAs induced by C. sinensis infection. For instance, differentially expressed mRNAs were mainly involved in “response to glucose”, “regulation of lipid metabolic process”, “negative regulation of fatty acid oxidation”, “negative regulation of toll-like receptor 4 (TLR4) signaling pathway”, and “positive regulation of Wnt signaling pathway, planar cell polarity pathway” (Figure 4A). The cellular components included “protein phosphatase type 2A complex” and “RNA polymerase II transcription factor complex”, while molecular functions were associated with “biotin carboxylase activity” and “ATPase activity, coupled”. KEGG pathway enrichment analysis was used to further understand the predicted biological functions of the mRNAs. The enriched pathways included “FoxO signaling pathway”, “AMPK signaling pathway”, “Wnt signaling pathway”, “Glucagon signaling pathway”, and “Insulin signaling pathway”. These results showed the disorder of lipid metabolism and glucose metabolism in clonorchiasis.
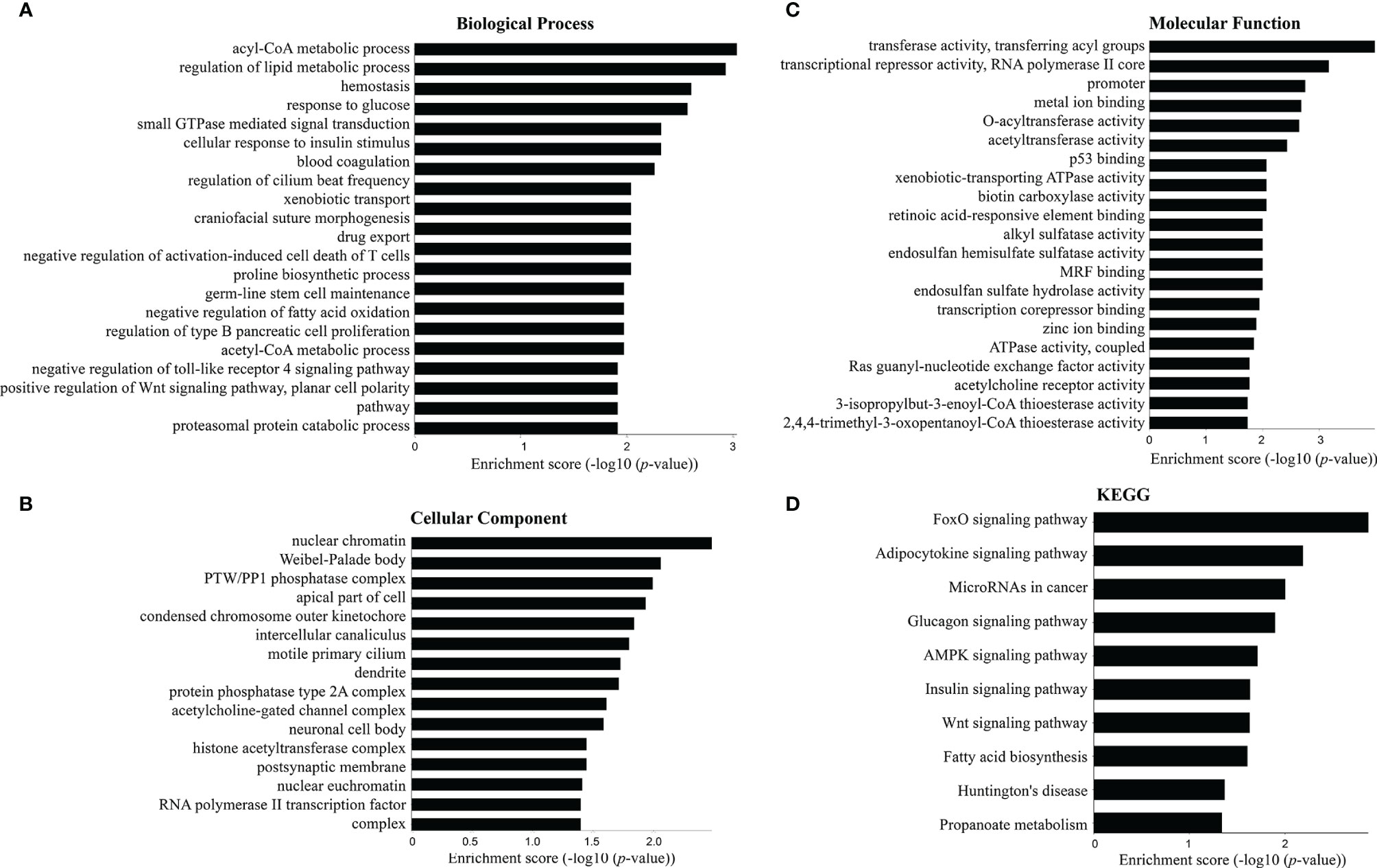
Figure 4 Top 20 terms in the gene enrichment and pathway analysis of differentially expressed mRNAs induced by C. sinensis infection. (A) Biological process. (B) Cellular component. (C) Molecular function. (D) The most significant KEGG pathway for the differentially expressed mRNAs.
Co-Expression Networks of lncRNAs and mRNAs
In order to analyze correlations among the differentially expressed lncRNAs and mRNAs and reveal their underlying mechanisms, a ceRNA was constructed (Figure 5 and Table S5). In these networks, each lncRNA can be related to one or more mRNAs, and each mRNA can also be associated with one or more lncRNAs. For example, mRNA Gpam was upregulated and associated with GM8801 (A_55_P2114498), A_30_P01020438, A_30_P01029457, and 10 other lncRNAs. Both mRNA Irs2 (downregulated) and Tnfrsf19 (upregulated) were found sharing 13 lncRNAs including Gm8801, A_30_P01020438, and A_30_P01029457. in the network. Additionally, the scatter plots with correlation of selected lncRNA Gm8801 and mRNA Gpam, Tnfrsf19, and Irs2 in the co-expression network are shown in Figure S1, respectively. LncRNA Gm8801 was found negatively correlated with mRNA Gpam (R2 = 0.9301) and Tnfrsf19 (R2 = 0.8745), while it was positively correlated with mRNA Irs2 (R2 = 0.8252), respectively. The lncRNAs and mRNAs with the highest correlation degrees are listed in Figure 6, respectively. Among the upregulated lncRNAs, A_50_P01032756 was the one with the highest mRNA correlation degree, while lncRNA Gm8801 was the top one regarding the mRNA correlation degree. As for the mRNAs, up-regulated Adamts13 was correlated with the most lncRNAs, while and down-regulated Cpne5 was the top one regarding the lncRNAs correlation degree.
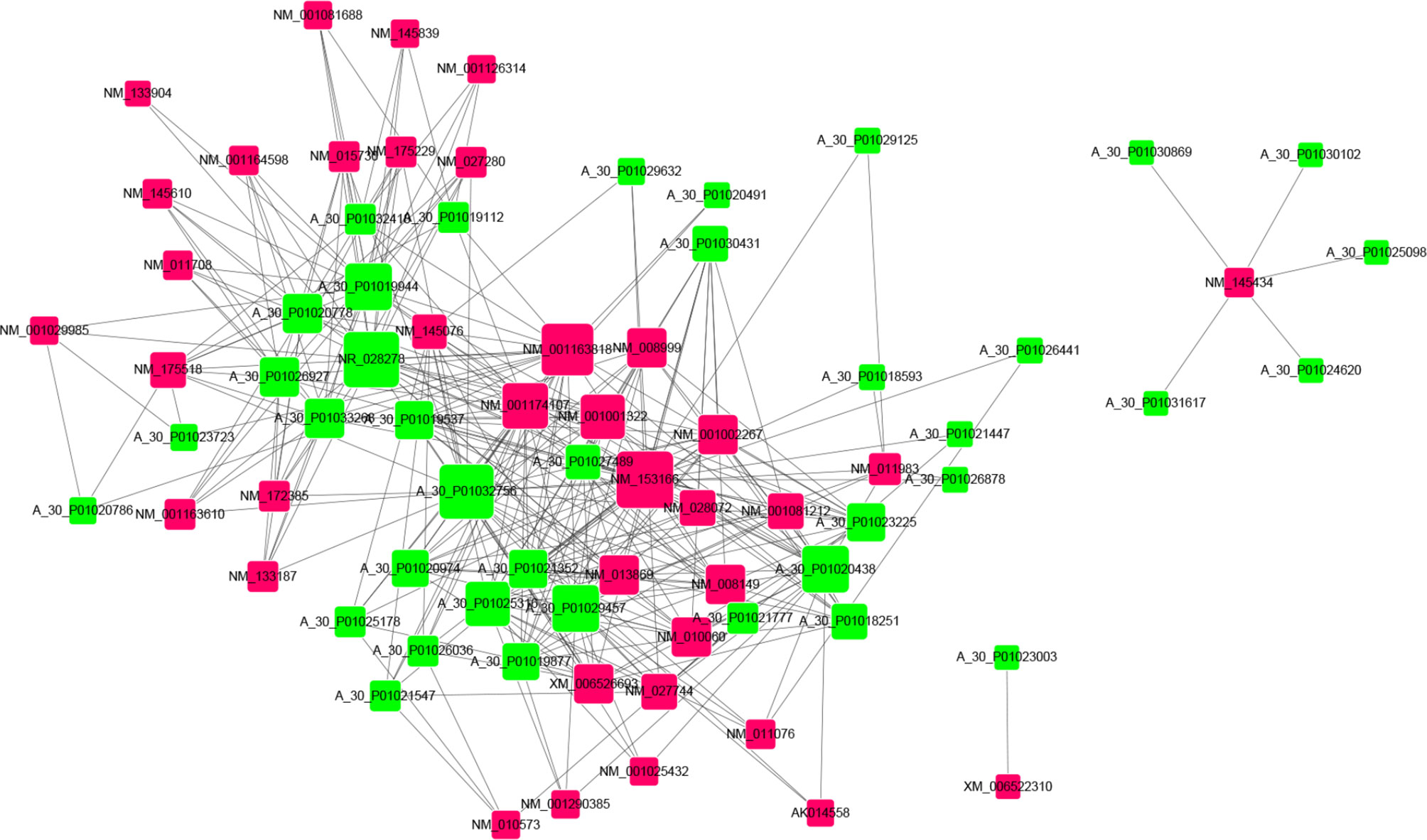
Figure 5 CeRNA network of the differentially expressed lncRNAs with their associate mRNAs. Co-expression lncRNA–mRNA pairs were identified using strict screening criteria (correlation coefficient ≥ 0.90 and p ≤ 0.01). Different colors were used to show different genes, with green for lncRNAs and red for mRNAs.
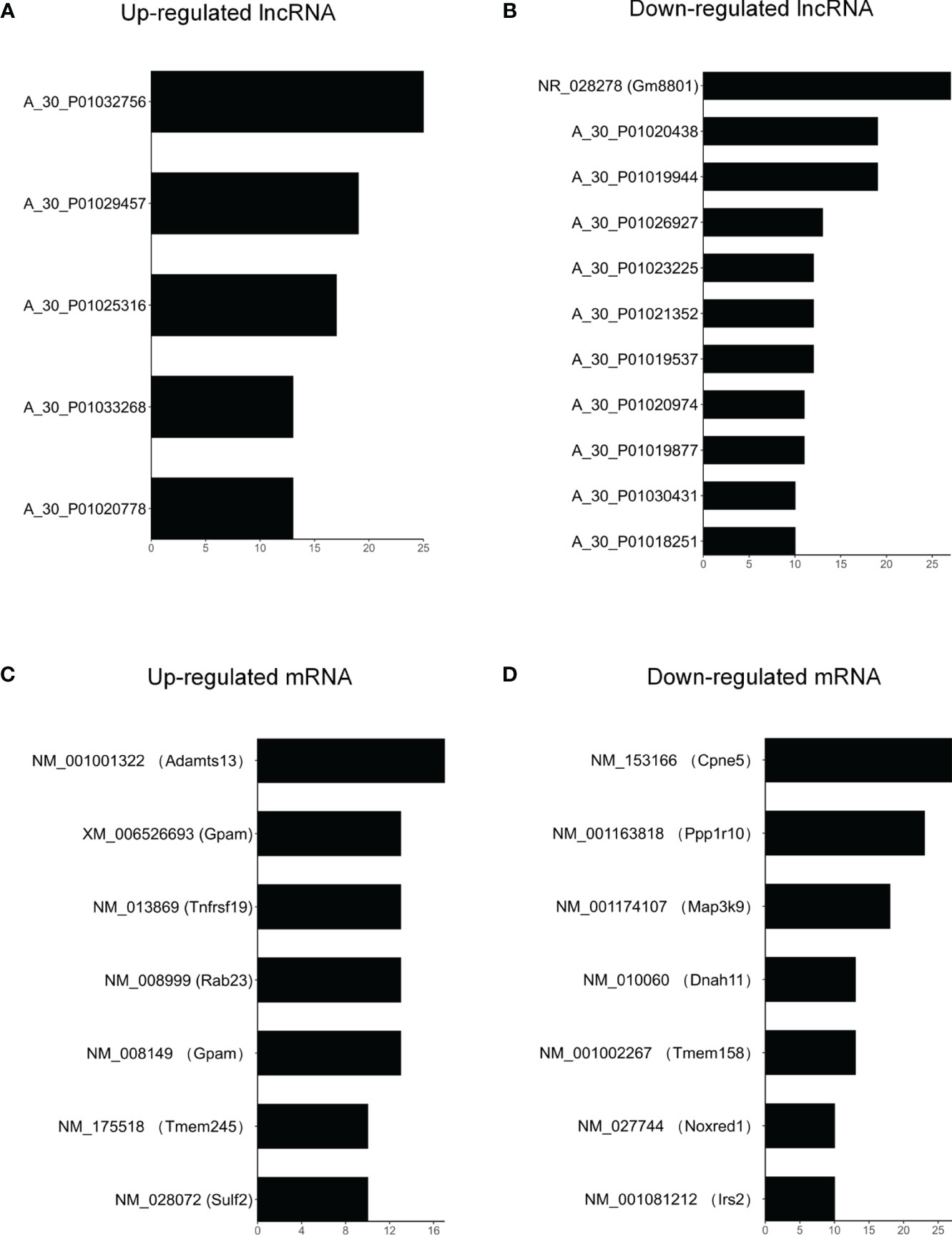
Figure 6 The top correlated lncRNA-mRNA pairs. (A) Up-regulated and (B) down-regulated lncRNAs with correlation degrees > 10. (C) Up-regulated and (D) down-regulated mRNAs with correlation degrees > 10. Correlation levels of 38 lncRNAs and 37 mRNAs were evaluated by correlation degrees in the network. The degree is equal to counts of significantly correlated lncRNA-mRNA pairs (P < 0.01) involving the corresponding lncRNA or mRNA.
Verification of the Correlation Between lncRNA Gm8801 and mRNAs
To verify the reliability of the correlation network, we selected lncRNA Gm8801 (downregulated), which was the only annotated lncRNA in the network, as the central lncRNA to build a subnetwork of the lncRNA–mRNA correlation. In this subnetwork, 13 mRNAs positively correlated with lncRNA Gm8801 expression were downregulated, while 14 mRNAs negatively correlated with Gm8801 expression were upregulated (Figure 7A). Subsequently, among the mRNAs correlated with Gm8801 lncRNA, mRNAs Gpam, Tnfrsf19, and Irs2 were selected to be preliminarily verified by qPCR. As shown in Figure 7B, mRNA Irs2 was decreased, while mRNA Gpam and Tnfrsf19 were increased at the same time point coinciding with the decrease of lncRNA Gm8801 in the C. sinensis-infected group. These results indicated that mRNA Irs2 was positively correlated with lncRNA Gm8801, while mRNA Gpam and Tnfrsf19 were negatively correlated with Gm8801, which is consistent with the prediction.
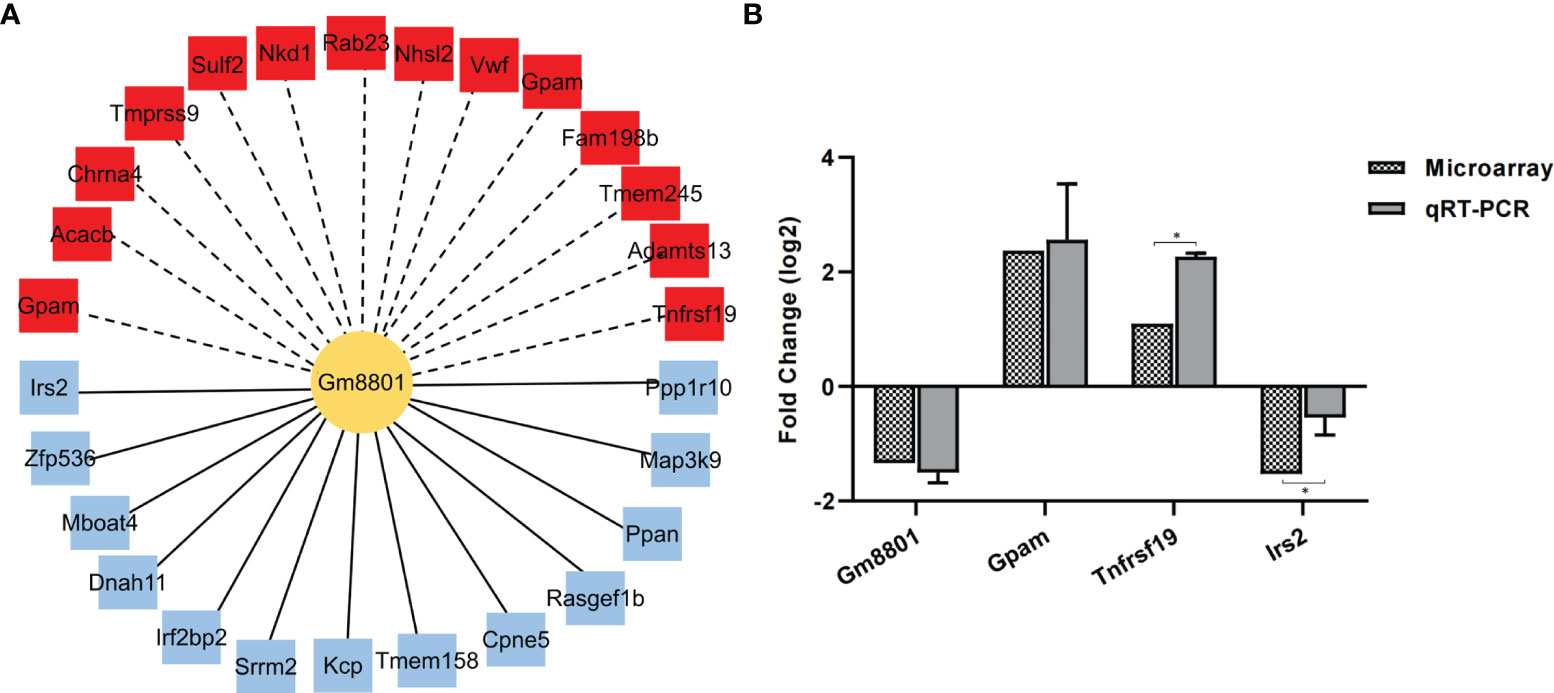
Figure 7 The correlation between mRNAs and lncRNA Gm8801. (A) The mRNAs correlated with Gm 8801 lncRNA. The red color indicates upregulation, while the blue color indicates downregulation. The solid lines mean positive correlation, and the dashed line means negative correlation. (B) The levels of mRNA Irs2, Gpam, Tnfrsf19, and lncRNA Gm8801. *p < 0.05.
Discussion
Previous studies on the interactions between parasites and their hosts have focused mainly on protein-coding genes (Melo et al., 2013; He et al., 2016). Recently, it was reported that lncRNAs play important roles in gene imprinting, cell differentiation, and tumorigenesis and are also involved in host–pathogen interactions (Jathar et al., 2017). For instance, RNA-Seq revealed that differentially expressed lncRNAs of Schistosoma mansoni were mainly involved in RNA-dependent DNA replication and G-protein coupled receptor (GPCR) signaling pathway/GPCR activity (Vasconcelos et al., 2017). However, the expression profiles of lncRNAs specific to C. sinensis infection were unclear. In this study, the expression profiles of lncRNAs and mRNAs in the liver of C. sinensis-infected mice at 4 wpi were fully explored using microarray analysis.
In this study, a total of 388 lncRNAs and 1,172 mRNAs were dysregulated in C. sinensis-infected group. As the most upregulated lncRNA, Gm6135 is primarily localized to the cytoplasm and participates in posttranslational modification (Rashid et al., 2016; Ji et al., 2019). LncRNA Gm6135 could increase the expression of toll-like receptor 4 (TLR4), by competitive binding to miR-203-3p, further activating the secretion of pro-inflammatory cytokines in mice (Ji et al., 2019; Loganathan et al., 2020). A previous study showed that TLR4 was upregulated in activated hematopoietic stem cells (HSCs) and myofibroblasts infected with C. sinensis (Yan et al., 2015). Thus, differentially expressed lncRNA Gm6135 may regulate the expression of TLR4 to induce inflammatory response in clonorchiasis. However, the role of 4930581F22Rik (the most downregulated lncRNA) has not been described clearly. The FMO3 protein encoded by Fmo3 (the most upregulated mRNA) is found associated with gallstone formation in mice (Chen et al., 2019). FMO3 protein and its metabolite (TMAO) participate in the TAF–FMO3–TMAO pathway, which could regulate lipid metabolism (Schugar and Brown, 2015; Warrier et al., 2015), while the downregulated mRNA Dmbt1 could encode the DMBT1 protein, which is related to liver injury and repair mechanisms in liver diseases (Bisgaard et al., 2002; Deng et al., 2012). Therefore, we speculate that mRNA Fmo3 and Dmbt1 might contribute to lipid metabolism and liver injury in clonorchiasis.
KEGG enrichment analysis showed that the top 20 predicted pathways mainly participated in “FOXO signaling pathway”, “Wnt signaling pathway”, and “AMPK signaling pathway”. For instance, mRNA Irs2 and Crebbp were rich in “FOXO signaling pathway”, which was involved in cellular differentiation, apoptosis, cell proliferation, DNA damage and repair, and oxidative stress (Gomes et al., 2008; Vurusaner et al., 2012). Nk1d1 and Crebbp mRNA were rich in “Wnt signaling pathway”, which played critical roles in precancerous lesions, malignant transformation of liver cells, and malignant expansion of cancer cells (Zhang et al., 2017). Nr1d1 mRNA was also involved in “negative regulation of TLR4 signaling pathway” in biological processes. TLR contributes to host defense by regulating host innate and adaptive immune responses (Iwasaki and Medzhitov, 2004). TLR4 was involved in immune responses, including increased levels of tumor necrosis factor alpha and interferon gamma during clonorchiasis (Yan et al., 2015). Interestingly, we also found that Irs2 and Gpam mRNA participated in “response to glucose” in biological processes. Moreover, “regulation of lipid metabolic process”, “negative regulation of fatty acid oxidation”, “Glucagon signaling pathway”, and “Fatty acid biosynthesis” demonstrated to be involved in C. sinensis infection. Therefore, we speculated that C. sinensis infection affected the cell function as well as lipid and glucose metabolism of the host.
Based on our previous miRNA expression profiling data (Additional File: Table S1) and differentially expressed lncRNAs and mRNAs, a ceRNA network was constructed, in order to explore the functions of lncRNAs. In ceRNA networks, mRNA Gpam and Tnfrsf19 were negatively correlated with lncRNA Gm8801 and A_30_P01020438, indicating an upregulation of Gpam and Tnfrsf19 mRNA in the C. sinensis-infected group. Gpam mRNA is correlated with T lymphocyte proliferation, lipid metabolism, defense against viruses, and IL-2 secretion (Brockmoller et al., 2012; Warrier et al., 2015). In addition, Tnfrsf19 mRNA belongs to the tumor necrosis factor receptor superfamily, which commonly transduces cytokine signals and is associated with poor prognosis in various types of cancer (Spanjaard et al., 2007; Fafilek et al., 2013; Loftus et al., 2013; Schon et al., 2014). Otherwise, Irs2 mRNA was positively correlated with Gm8801 and A_30_P01020438 lncRNA, indicating a downregulation of Irs2 in the C. sinensis-infected group. Irs2 plays requisite roles in cell proliferation, apoptosis, migration, and tumor invasion (Lea et al., 2014; Zhao et al., 2014). Moreover, Irs2 mRNA is also important in hepatic nutrient homeostasis, as it mediates the anabolic effects of insulin through the PI3K-Akt cascade (Rother et al., 1998; Andersson et al., 2004) and suppresses gluconeogenesis (Valverde et al., 2003). Therefore, we hypothesized that lncRNA Gm8801 and A_30_P01020438 might play important roles in host metabolism and liver injury in clonorchiasis by regulating its co-expressed mRNA.
In this study, a microarray approach was used to explore the differential expression profiles of lncRNAs and mRNAs in clonorchiasis for the first time. However, there were some limitations that should be addressed. We only analyzed the expression profiles of lncRNAs and mRNAs at 4 weeks after being infected with C. sinensis, which corresponds to the early stage. However, C. sinensis infection is a dynamic process; the expression profiles of lncRNAs and mRNAs are also needed for detection at the late stages of infection. Moreover, the potential interaction between LncRNA and mRNA is needed to validate in the future.
Conclusions
In this study, we investigated the differential expression profiles of lncRNAs (388) and mRNAs (1,172) in the livers of mice infected with C. sinensis. Our results provided valuable insights into the lncRNAs and mRNAs involved in clonorchiasis, which may be useful for future control strategies. In the future, the functions of the differentially expressed lncRNAs and mRNAs in the pathogenesis of C. sinensis infection need to be further verified.
Data Availability Statement
The datasets presented in this study can be found in online repositories. The names of the repository/repositories and accession number(s) can be found below: https://www.ncbi.nlm.nih.gov/geo/, accession ID: GSE188841.
Ethics Statement
The animal study was reviewed and approved by the Care and Use of Laboratory Animals of the Ministry of Science and Technology, China.
Author Contributions
SH and Xi-LZ offered advice for the article framework and conceived the conception and design of the trial. SH and Xi-LZ drafted the first manuscript and participated in trial communication and monitoring. Xu-LZ, XJ, XL, JD, JZ, SD, RC, BS, YH and NG modified the manuscript the carried out statistical calculations. All authors participated in revision of the manuscript and approved the final version.
Funding
This work was supported by the National Natural Science Foundation of China (81971958, 81601785, and 81401684), Heilongjiang Provincial Natural Science Foundation of China (LH2020H016), Post-doctoral Science Foundation (LBH-Q174), and Postgraduate Research & Practice Innovation Program of Harbin Medical University (YJSKYCX2019-67HYD). The funder had no role in the study design, data collection and analysis, decision to publish, or preparation of the manuscript.
Conflict of Interest
The authors declare that the research was conducted in the absence of any commercial or financial relationships that could be construed as a potential conflict of interest.
Publisher’s Note
All claims expressed in this article are solely those of the authors and do not necessarily represent those of their affiliated organizations, or those of the publisher, the editors and the reviewers. Any product that may be evaluated in this article, or claim that may be made by its manufacturer, is not guaranteed or endorsed by the publisher.
Acknowledgments
We thank the staff of the laboratory animal research center of Harbin Medical University for their cooperation and support.
Supplementary Material
The Supplementary Material for this article can be found online at: https://www.frontiersin.org/articles/10.3389/fcimb.2021.754224/full#supplementary-material
Supplementary Figure 1 | The scatter plots with correlation of selected lncRNA and mRNA in co-expression network. LncRNA Gm8801 relative expression levels and Gpam mRNA (A), Tnfrsf19 mRNA (B), Irs2 mRNA (C) relative expression levels.
Supplementary Table 1 | The expression profile of differentially expressed miRNAs in the liver of control and infected groups.
Supplementary Table 2 | The expression profile of differentially expressed lncRNAs in the liver of control and infected groups.
Supplementary Table 3 | The expression profile of differentially expressed mRNAs in the liver of control and infected groups.
Supplementary Table 4 | Raw data from the lncRNA-mRNA correlation analysis.
Supplementary Table 5 | Raw data from ceRNA network analysis.
Abbreviations
GO, Gene Ontology; KEGG, Kyoto Encyclopedia of Genes and Genomes; lncRNA, long non-coding RNA; mRNA, messenger RNA; qPCR, real-time quantitative PCR; TLR4, toll-like receptor; Pax2, paired box 2; Btg2, B cell translocation gene 2, anti-proliferative; Dmbt1, deleted in malignant brain tumors 1; Irs2, insulin receptor substrate 2; Tnfrsf19, tumor necrosis factor receptor superfamily, member 19; Gpam, glycerol-3-phosphate acyltransferase, mitochondrial; Fate1, fetal and adult testis expressed 1; Meg3, maternally expressed 3; Snhg3, small nucleolar RNA host gene 3; Gm6135, prediticted gene 6135; Gm8801, protein phosphatase 1, regulatory subunit 10 pseudogene; Crebbp, CREB (cyclic adenosine monophosphate (cAMP) response element-binding protein; GAPDH, glyceraldehyde 3-phosphate dehydrogenase.
References
Andersson, U., Filipsson, K., Abbott, C. R., Woods, A., Smith, K., Bloom, S. R., et al. (2004). AMP-Activated Protein Kinase Plays a Role in the Control of Food Intake. J. Biol. Chem. 279 (13), 12005–12008. doi: 10.1074/jbc.C300557200
Bisgaard, H. C., Holmskov, U., Santoni-Rugiu, E., Nagy, P., Nielsen, O., Ott, P., et al. (2002). Heterogeneity of Ductular Reactions in Adult Rat and Human Liver Revealed by Novel Expression of Deleted in Malignant Brain Tumor 1. Am. J. Pathol. 161 (4), 1187–1198. doi: 10.1016/S0002-9440(10)64395-7
Bouvard, V., Baan, R., Straif, K., Grosse, Y., Secretan, B., El Ghissassi, F., et al. (2009). A Review of Human Carcinogens–Part B: Biological Agents. Lancet Oncol. 10 (4), 321–322. doi: 10.1016/s1470-2045(09)70096-8
Brockmoller, S. F., Bucher, E., Muller, B. M., Budczies, J., Hilvo, M., Griffin, J. L., et al. (2012). Integration of Metabolomics and Expression of Glycerol-3-Phosphate Acyltransferase (GPAM) in Breast Cancer-Link to Patient Survival, Hormone Receptor Status, and Metabolic Profiling. J. Proteome Res. 11 (2), 850–860. doi: 10.1021/pr200685r
Chen, Y., Weng, Z., Liu, Q., Shao, W., Guo, W., Chen, C., et al. (2019). FMO3 and its Metabolite TMAO Contribute to the Formation of Gallstones. Biochim. Biophys. Acta Mol. Basis Dis. 1865 (10), 2576–2585. doi: 10.1016/j.bbadis.2019.06.016
Chung, B. S., Zhang, H., Choi, M. H., Jeon, D., Li, S., Lee, M., et al. (2004). Development of Resistance to Reinfection by Clonorchis Sinensis in Rats. Korean J. Parasitol 42 (1), 19–26. doi: 10.3347/kjp.2004.42.1.19
Deng, H., Gao, Y. B., Wang, H. F., Jin, X. L., Xiao, J. C. (2012). Expression of Deleted in Malignant Brain Tumours 1 (DMBT1) Relates to the Proliferation and Malignant Transformation of Hepatic Progenitor Cells in Hepatitis B Virus-Related Liver Diseases. Histopathology 60 (2), 249–260. doi: 10.1111/j.1365-2559.2011.04082.x
Fafilek, B., Krausova, M., Vojtechova, M., Pospichalova, V., Tumova, L., Sloncova, E., et al. (2013). Troy, a Tumor Necrosis Factor Receptor Family Member, Interacts With Lgr5 to Inhibit Wnt Signaling in Intestinal Stem Cells. Gastroenterology 144 (2), 381–391. doi: 10.1053/j.gastro.2012.10.048
Fasolo, F., Patrucco, L., Volpe, M., Bon, C., Peano, C., Mignone, F., et al. (2019). The RNA-Binding Protein ILF3 Binds to Transposable Element Sequences in SINEUP lncRNAs. FASEB J. 33 (12), 13572–13589. doi: 10.1096/fj.201901618RR
Fernandes, J. C. R., Acuna, S. M., Aoki, J. I., Floeter-Winter, L. M., Muxel, S. M. (2019). Long Non-Coding RNAs in the Regulation of Gene Expression: Physiology and Disease. Noncoding RNA 5 (1), 17. doi: 10.3390/ncrna5010017
Fitzgerald, K. A., Caffrey, D. R. (2014). Long Noncoding RNAs in Innate and Adaptive Immunity. Curr. Opin. Immunol. 26, 140–146. doi: 10.1016/j.coi.2013.12.001
Gomes, A. R., Brosens, J. J., Lam, E. W. (2008). Resist or Die: FOXO Transcription Factors Determine the Cellular Response to Chemotherapy. Cell Cycle 7 (20), 3133–3136. doi: 10.4161/cc.7.20.6920
Han, S., Tang, Q., Chen, R., Li, Y., Shu, J., Zhang, X. (2017). Hepatic Iron Overload is Associated With Hepatocyte Apoptosis During Clonorchis Sinensis Infection. BMC Infect. Dis. 17 (1), 531. doi: 10.1186/s12879-017-2630-3
Han, S., Tang, Q., Lu, X., Chen, R., Li, Y., Shu, J., et al. (2016). Dysregulation of Hepatic microRNA Expression Profiles With Clonorchis Sinensis Infection. BMC Infect. Dis. 16 (1), 724. doi: 10.1186/s12879-016-2058-1
Han, S., Zhang, X., Wen, J., Li, Y., Shu, J., Ling, H., et al. (2012). A Combination of the Kato-Katz Methods and ELISA to Improve the Diagnosis of Clonorchiasis in an Endemic Area, China. PloS One 7 (10), e46977. doi: 10.1371/journal.pone.0046977
He, J. J., Ma, J., Elsheikha, H. M., Song, H. Q., Zhou, D. H., Zhu, X. Q. (2016). Proteomic Profiling of Mouse Liver Following Acute Toxoplasma Gondii Infection. PloS One 11 (3), e0152022. doi: 10.1371/journal.pone.0152022
Hong, S. T., Choi, M. H., Kim, C. H., Chung, B. S., Ji, Z. (2003). The Kato-Katz Method is Reliable for Diagnosis of Clonorchis Sinensis Infection. Diagn. Microbiol. Infect. Dis. 47 (1), 345–347. doi: 10.1016/s0732-8893(03)00113-5
Hu, Y. P., Jin, Y. P., Wu, X. S., Yang, Y., Li, Y. S., Li, H. F., et al. (2019). LncRNA-HGBC Stabilized by HuR Promotes Gallbladder Cancer Progression by Regulating miR-502-3p/SET/AKT Axis. Mol. Cancer 18 (1), 167. doi: 10.1186/s12943-019-1097-9
Iwasaki, A., Medzhitov, R. (2004). Toll-Like Receptor Control of the Adaptive Immune Responses. Nat. Immunol. 5 (10), 987–995. doi: 10.1038/ni1112
Jathar, S., Kumar, V., Srivastava, J., Tripathi, V. (2017). Technological Developments in lncRNA Biology. Adv. Exp. Med. Biol. 1008, 283–323. doi: 10.1007/978-981-10-5203-3_10
Ji, T. T., Wang, Y. K., Zhu, Y. C., Gao, C. P., Li, X. Y., Li, J., et al. (2019). Long Noncoding RNA Gm6135 Functions as a Competitive Endogenous RNA to Regulate Toll-Like Receptor 4 Expression by Sponging miR-203-3p in Diabetic Nephropathy. J. Cell Physiol. 234 (5), 6633–6641. doi: 10.1002/jcp.27412
Keiser, J., Utzinger, J. (2005). Emerging Foodborne Trematodiasis. Emerg. Infect. Dis. 11 (10), 1507–1514. doi: 10.3201/eid1110.050614
Kopp, F., Mendell, J. T. (2018). Functional Classification and Experimental Dissection of Long Noncoding RNAs. Cell 172 (3), 393–407. doi: 10.1016/j.cell.2018.01.011
Lai, D. H., Hong, X. K., Su, B. X., Liang, C., Hide, G., Zhang, X., et al. (2016). Current Status of Clonorchis Sinensis and Clonorchiasis in China. Trans. R Soc. Trop. Med. Hyg 110 (1), 21–27. doi: 10.1093/trstmh/trv100
Lea, M. A., Pourat, J., Patel, R., desBordes, C. (2014). Growth Inhibition of Colon Cancer Cells by Compounds Affecting AMPK Activity. World J. Gastrointest Oncol. 6 (7), 244–252. doi: 10.4251/wjgo.v6.i7.244
Loftus, J. C., Dhruv, H., Tuncali, S., Kloss, J., Yang, Z., Schumacher, C. A., et al. (2013). TROY (TNFRSF19) Promotes Glioblastoma Survival Signaling and Therapeutic Resistance. Mol. Cancer Res. 11 (8), 865–874. doi: 10.1158/1541-7786.MCR-13-0008
Loganathan, T. S., Sulaiman, S. A., Abdul Murad, N. A., Shah, S. A., Abdul Gafor, A. H., Jamal, R., et al. (2020). Interactions Among Non-Coding RNAs in Diabetic Nephropathy. Front. Pharmacol. 11, 191. doi: 10.3389/fphar.2020.00191
Lu, Y., Liu, H., Yang, X. Y., Liu, J. X., Dai, M. Y., Wu, J. C., et al. (2020). Microarray Analysis of lncRNA and mRNA Reveals Enhanced Lipolysis Along With Metabolic Remodeling in Mice Infected With Larval Echinococcus Granulosus. Front. Physiol. 11:1078. doi: 10.3389/fphys.2020.01078
Lun, Z. R., Gasser, R. B., Lai, D. H., Li, A. X., Zhu, X. Q., Yu, X. B., et al. (2005). Clonorchiasis: A Key Foodborne Zoonosis in China. Lancet Infect. Dis. 5 (1), 31–41. doi: 10.1016/S1473-3099(04)01252-6
Marques-Rocha, J. L., Samblas, M., Milagro, F. I., Bressan, J., Martinez, J. A., Marti, A. (2015). Noncoding RNAs, Cytokines, and Inflammation-Related Diseases. FASEB J. 29 (9), 3595–3611. doi: 10.1096/fj.14-260323
Melo, M. B., Nguyen, Q. P., Cordeiro, C., Hassan, M. A., Yang, N., McKell, R., et al. (2013). Transcriptional Analysis of Murine Macrophages Infected With Different Toxoplasma Strains Identifies Novel Regulation of Host Signaling Pathways. PloS Pathog. 9 (12), e1003779. doi: 10.1371/journal.ppat.1003779
Mumtaz, P. T., Bhat, S. A., Ahmad, S. M., Dar, M. A., Ahmed, R., Urwat, U., et al. (2017). LncRNAs and Immunity: Watchdogs for Host Pathogen Interactions. Biol. Proced Online 19, 3. doi: 10.1186/s12575-017-0052-7
Ovchinnikov, V. Y., Afonnikov, D. A., Vasiliev, G. V., Kashina, E. V., Sripa, B., Mordvinov, V. A., et al. (2015). Identification of microRNA Genes in Three Opisthorchiids. PloS Negl. Trop. Dis. 9 (4), e0003680. doi: 10.1371/journal.pntd.0003680
Quinn, J. J., Chang, H. Y. (2016). Unique Features of Long non-Coding RNA Biogenesis and Function. Nat. Rev. Genet. 17 (1), 47–62. doi: 10.1038/nrg.2015.10
Rashid, F., Shah, A., Shan, G. (2016). Long Non-Coding RNAs in the Cytoplasm. Genomics Proteomics Bioinf. 14 (2), 73–80. doi: 10.1016/j.gpb.2016.03.005
Renganathan, A., Felley-Bosco, E. (2017). Long Noncoding RNAs in Cancer and Therapeutic Potential. Adv. Exp. Med. Biol. 1008, 199–222. doi: 10.1007/978-981-10-5203-3_7
Rinn, J. L., Chang, H. Y. (2012). Genome Regulation by Long Noncoding RNAs. Annu. Rev. Biochem. 81, 145–166. doi: 10.1146/annurev-biochem-051410-092902
Rother, K. I., Imai, Y., Caruso, M., Beguinot, F., Formisano, P., Accili, D. (1998). Evidence That IRS-2 Phosphorylation is Required for Insulin Action in Hepatocytes. J. Biol. Chem. 273 (28), 17491–17497. doi: 10.1074/jbc.273.28.17491
Schon, S., Flierman, I., Ofner, A., Stahringer, A., Holdt, L. M., Kolligs, F. T., et al. (2014). Beta-Catenin Regulates NF-kappaB Activity via TNFRSF19 in Colorectal Cancer Cells. Int. J. Cancer 135 (8), 1800–1811. doi: 10.1002/ijc.28839
Schugar, R. C., Brown, J. M. (2015). Emerging Roles of Flavin Monooxygenase 3 in Cholesterol Metabolism and Atherosclerosis. Curr. Opin. Lipidol. 26 (5), 426–431. doi: 10.1097/MOL.0000000000000215
Spanjaard, R. A., Whren, K. M., Graves, C., Bhawan, J. (2007). Tumor Necrosis Factor Receptor Superfamily Member TROY is a Novel Melanoma Biomarker and Potential Therapeutic Target. Int. J. Cancer 120 (6), 1304–1310. doi: 10.1002/ijc.22367
Valverde, A. M., Burks, D. J., Fabregat, I., Fisher, T. L., Carretero, J., White, M. F., et al. (2003). Molecular Mechanisms of Insulin Resistance in IRS-2-Deficient Hepatocytes. Diabetes 52 (9), 2239–2248. doi: 10.2337/diabetes.52.9.2239
Vasconcelos, E. J. R., daSilva, L. F., Pires, D. S., Lavezzo, G. M., Pereira, A. S. A., Amaral, M. S., et al. (2017). The Schistosoma Mansoni Genome Encodes Thousands of Long non-Coding RNAs Predicted to be Functional at Different Parasite Life-Cycle Stages. Sci. Rep. 7 (1), 10508. doi: 10.1038/s41598-017-10853-6
Vurusaner, B., Poli, G., Basaga, H. (2012). Tumor Suppressor Genes and ROS: Complex Networks of Interactions. Free Radic. Biol. Med. 52 (1), 7–18. doi: 10.1016/j.freeradbiomed.2011.09.035
Warrier, M., Shih, D. M., Burrows, A. C., Ferguson, D., Gromovsky, A. D., Brown, A. L., et al. (2015). The TMAO-Generating Enzyme Flavin Monooxygenase 3 Is a Central Regulator of Cholesterol Balance. Cell Rep. 10 (3), 326–338. doi: 10.1016/j.celrep.2014.12.036
Xia, T., Giri, B. R., Liu, J., Du, P., Li, X., Li, X., et al. (2020). RNA Sequencing Analysis of Altered Expression of Long Noncoding RNAs Associated With Schistosoma Japonicum Infection in the Murine Liver and Spleen. Parasit Vectors 13 (1), 601. doi: 10.1186/s13071-020-04457-9
Yamamura, S., Imai-Sumida, M., Tanaka, Y., Dahiya, R. (2018). Interaction and Cross-Talk Between non-Coding RNAs. Cell Mol. Life Sci. 75 (3), 467–484. doi: 10.1007/s00018-017-2626-6
Yan, C., Li, X. Y., Li, B., Zhang, B. B., Xu, J. T., Hua, H., et al. (2015). Expression of Toll-Like Receptor (TLR) 2 and TLR4 in the Livers of Mice Infected by Clonorchis Sinensis. J. Infect. Dev. Ctries 9 (10), 1147–1155. doi: 10.3855/jidc.6698
Yu, A., Wang, Y., Yin, J., Zhang, J., Cao, S., Cao, J., et al. (2018). Microarray Analysis of Long non-Coding RNA Expression Profiles in Monocytic Myeloid-Derived Suppressor Cells in Echinococcus Granulosus-Infected Mice. Parasit Vectors 11 (1), 327. doi: 10.1186/s13071-018-2905-6
Zhang, W., Duan, N., Song, T., Li, Z., Zhang, C., Chen, X. (2017). The Emerging Roles of Forkhead Box (FOX) Proteins in Osteosarcoma. J. Cancer 8 (9), 1619–1628. doi: 10.7150/jca.18778
Zhang, X., Jin, Z., Da, R., Dong, Y., Song, W., Chen, X., et al. (2008). Fas/FasL-Dependent Apoptosis of Hepatocytes Induced in Rat and Patients With Clonorchis Sinensis Infection. Parasitol. Res. 103 (2), 393–399. doi: 10.1007/s00436-008-0985-5
Keywords: clonorchiasis, liver, long non-coding RNAs, mRNA, microarray analysis
Citation: Han S, Zhang X-L, Jiang X, Li X, Ding J, Zuo L-J, Duan S-S, Chen R, Sun B-B, Hu X-Y, Gao Y-N and Zhang X-L (2022) Long Non-Coding RNA and mRNA Expression Analysis in Liver of Mice With Clonorchis sinensis Infection. Front. Cell. Infect. Microbiol. 11:754224. doi: 10.3389/fcimb.2021.754224
Received: 06 August 2021; Accepted: 07 December 2021;
Published: 19 January 2022.
Edited by:
Guofeng Cheng, Tongji University, ChinaReviewed by:
Laurence A. Marchat, Instituto Politécnico Nacional, MexicoJingyi Liu, Shanghai Veterinary Research Institute (CAAS), China
Copyright © 2022 Han, Zhang, Jiang, Li, Ding, Zuo, Duan, Chen, Sun, Hu, Gao and Zhang. This is an open-access article distributed under the terms of the Creative Commons Attribution License (CC BY). The use, distribution or reproduction in other forums is permitted, provided the original author(s) and the copyright owner(s) are credited and that the original publication in this journal is cited, in accordance with accepted academic practice. No use, distribution or reproduction is permitted which does not comply with these terms.
*Correspondence: Xiao-Li Zhang, emhhbmd4aWFvbGkxMTE5QDE2My5jb20=