- 1Section for Clinical Oral Microbiology, Department of Odontology, Faculty of Health and Medical Sciences, University of Copenhagen, Copenhagen, Denmark
- 2Laboratory of Food Biotechnology, Department of Health Sciences and Technology, ETH Zürich, Zürich, Switzerland
- 3Singapore Centre for Environmental Life Sciences Engineering (SCELSE) Nanyang Technological University, Singapore, Singapore
- 4Lundbeck Foundation GeoGenetics Centre, Globe Institute, University of Copenhagen, Copenhagen, Denmark
- 5Costerton Biofilm Center, Department of Immunology and Microbiology, Faculty of Health and Medical Sciences, University of Copenhagen, Copenhagen, Denmark
Background: Streptococcus species are predominant members of the oral microbiota in both health and diseased conditions. The purpose of the present study was to explore if different ecological characteristics, such as oxygen availability and presence of periodontitis, associates with transcriptional activity of predominant members of genus Streptococcus. We tested the hypothesis that genetically closely related Streptococcus species express different transcriptional activities in samples collected from environments with critically different ecological conditions determined by site and inflammatory status.
Methods: Metagenomic and metatranscriptomic data was retrieved from 66 oral samples, subgingival plaque (n=22), tongue scrapings (n=22) and stimulated saliva (n=22) collected from patients with periodontitis (n=11) and orally healthy individuals (n=11). Species-specific transcriptional activity was computed as Log2(RNA/DNA), and transcriptional activity of predominant Streptococcus species was compared between multiple samples collected from different sites in the same individual, and between individuals with different oral health status.
Results: The predominant Streptococcus species were identified with a site-specific colonization pattern of the tongue and the subgingival plaque. A total of 11, 4 and 2 pathways expressed by S. parasanguinis, S. infantis and S. salivarius, respectively, were recorded with significantly higher transcriptional activity in saliva than in tongue biofilm in healthy individuals. In addition, 18 pathways, including pathways involved in synthesis of peptidoglycan, amino acid biosynthesis, glycolysis and purine nucleotide biosynthesis expressed by S. parasanguinis and 3 pathways expressed by S. salivarius were identified with significantly less transcriptional activity in patients with periodontitis.
Conclusion: Data from the present study significantly demonstrates the association of site-specific ecological conditions and presence of periodontitis with transcriptional activity of the predominant Streptococcus species of the oral microbiota. In particular, pathways expressed by S. parasanguinis being involved in peptidoglycan, amino acid biosynthesis, glycolysis, and purine nucleotide biosynthesis were identified to be significantly associated with oral site and/or inflammation status.
Introduction
The oral microbiota is comprised by more than 700 different species, which makes it the second most diverse found in the human body (Dewhirst et al., 2010). The composition of the permanent oral microbiota is shaped by thousands of years of co-evolution together with the host (Fellows Yates et al., 2021), which is why the oral microbiota through symbiotic interactions with human natural defense systems is essential for maintenance of oral health (Marsh and Zaura, 2017).
While the oral microbiota is highly individualized and diverse, genus Streptococcus is the predominant genus of the oral microbiota across populations and during both healthy and diseased conditions (Chattopadhyay et al., 2019). A likely explanation to these findings is the versatility of Streptococcus species, including being facultative anaerobic, which means that they thrive in areas with plenty or lack of oxygen (Abranches et al., 2018). In addition, Streptococcus species express specific surface characteristics, which enables them to colonize both teeth and the oral mucosa, thereby protecting them from transfer to the gastrointestinal channel during swallowing (Jenkinson, 1994). Finally, Streptococcus species are proficient in carbohydrate metabolism, which is most often the prime nutrient offered by the oral cavity (Takahashi, 2015).
Carbohydrates from food intake and salivary glycoproteins are the main nutrients of Streptococcus species, which in addition to providing energy can be stored as intracellular reservoirs (Busuioc et al., 2009). Furthermore, some Streptococcus species can deposit carbohydrates extracellularly as part of the oral biofilm matrix, which is why Streptococcus species are critically involved in biofilm pathogenicity (Koo et al., 2013). While carbohydrate metabolism of some Streptococcus species is limited to a small number of different carbohydrates, other Streptococcus species, such as Streptococcus mutans, are capable of degrading almost all types of carbohydrates (Moye et al., 2014). Indeed, carbohydrate metabolism performed by Streptococcus species is a double-edged sword. On the more negative side, these bacteria degrade carbohydrates anaerobically via fermentation processes that locally lead to acid production and a decrease in biofilm fluid pH, which links Streptococcus species with dental caries. On the positive side, the acid production resulting from streptococcal carbohydrate fermentation may function as a regulatory mechanism of the biofilm in situations characterized by a transient increase in local pH, for example during inflammatory conditions such as periodontitis (Nyvad and Takahashi, 2020).
In the oral cavity, Streptococcus species live closely together in polymicrobial biofilms, shaped by synergistic and antagonistic interactions with other members of the biofilm community (Lamont et al., 2018). Therefore, the interaction of Streptococcus species and their phenotypic profile in situations with different ecological conditions should ideally be studied using in-vivo clinical samples (Belstrøm, 2020). Indeed, Streptococcus species are closely related genetically, which is why it is often not possible to distinguish them at species level, using 16S rDNA methods (Lal et al., 2011). Thus, most information on metabolic characteristics such as carbohydrate metabolism of Streptococcus species comes from in-vitro culture microbiology, based on analysis of gene expression by Streptococcus species grown in either mono- or polymicrobial biofilms (Abranches et al., 2018). Therefore, much is to be learned on how different Streptococcus species adapt to changes in ecological conditions, such as presence of chronic periodontal inflammation, in complex biofilms in-vivo.
The oral cavity is unique compared to other body sites, since it is composed of different locations, which despite being anatomically close, are determined by critically different ecological conditions (Kilian et al., 2016). For example, the tongue surface is characterized by a slow epithelial turn-over and deep anaerobic crypts, which favors growth of a mature biofilm (Asakawa et al., 2018). On the other hand, in individuals who perform regular oral hygiene, subgingival dental plaque is a more immature biofilm, formed in predominantly aerobic conditions (Berger et al., 2018). Therefore, the tongue and the subgingival biofilm constitute microbial communities shaped by critically different ecological conditions. Saliva is sterile when entering the oral cavity (Schrøder et al., 2017). Accordingly, saliva is a conglomerate of bacteria shed from different oral surfaces, with subgingival dental and tongue biofilm as some of the prime donor sites (Segata et al., 2012). As compared to tongue and subgingival biofilms, the salivary microbiota is primarily planktonic (Belstrøm, 2020). Indeed, Streptococcus species are highly abundant in both tongue and subgingival dental biofilms as well as in saliva (Segata et al., 2012), which is why concomitant characterization of Streptococcus species in these samples offer the possibility to study how Streptococcus species adapt to different ecological conditions.
The purpose the present study was to determine if different ecological characteristics such as oxygen availability and conditions of chronic periodontal inflammation associate with differential transcriptional activities of predominant members of genus Streptococcus. We applied paired metagenomics and metatranscriptomics to characterize and compare transcriptional activity of the predominant Streptococcus species identified in clinical samples, i.e. tongue biofilm, subgingival dental biofilm and saliva collected from orally healthy individuals and patients with periodontitis. We tested the hypothesis that transcriptional activities of predominant Streptococcus species associate with periodontal status.
Methods
Study Population and Sample Collection
The present study is part of a series of studies based on characterization of the oral microbiota in samples collected from the same cohort. Thus, the study population and sample collection has been described in detail (Belstrøm et al., 2021). In brief, a total of 66 microbial samples were collected from 11 patients with chronic periodontitis, and 11 orally healthy controls at the Department of Odontology, University of Copenhagen in January 2018 The study was approved by the regional ethical committee (H-16016368) and reported to the local data authorization of University of Copenhagen (SUND-2018-8).
The periodontitis group (M/F: 5/6), out of which four were current smokers, had a mean age of 64 yrs. (55-77 yrs.), The control group (M/F: 4/7) had a mean age of 60 yrs. (50-71 yrs.), and included three daily smokers. Exclusion criteria in both groups: age< 50 yrs., systemic diseases, use of any kind of medication including usage of antibiotics within the last three months.
Microbial samples, subgingival dental plaque (n=22), tongue scrapings (n=22) and stimulated saliva (n=22), were collected between 8:00 AM and 11:00 AM, according to standardized protocols as previously described (Belstrøm et al., 2021). Subgingival plaque and tongue coatings were suspended in 2 mL Sodium Chloride (9mg/mL). Immediately, after collection each sample was divided in two aliquots of 1 mL each, one each for metagenomics and metatranscriptomics analysis. RNAlater (Life Technologies, Denmark) was added to the aliquot allocated for metatranscriptomics and all aliquots were immediately stored at -80°C until further processing.
Metagenomic and Metatranscriptomic Library Preparation and Sequencing
Raw data were downloaded from NCBI SRA PRJNA678453. The laboratory procedures used for DNA and RNA extraction and sequencing have been described in detail (Belstrøm et al., 2021).
Read Preprocessing, Taxonomic, and Functional Profiling
Raw sequencing reads pre-processing was performed as described previously (Belstrøm et al., 2021). Briefly, non-biological sequences, low quality RNA reads identified as ribosomal RNA as well as DNA and RNA reads mapping to the human genomes were discarded from the dataset.
Taxonomic composition of metagenomic reads was determined using MetaPhlan (Beghini et al., 2021) (version 3.0.1, 25 Jun 2020), –min_ab 0.000001 using the latest available database i.e., mpa_v30_CHOCOPhlAn_201901). MetaPhlan relies on read mapping against a built-in collection of clade-specific marker genes database, which allows an unambiguous estimation of species relative abundance across samples with a low species resolution false positives rate as compared to read classifiers.
Sample-specific taxonomic profiles generated using MetaPhlAn on metagenomes were used to filter the HUMAnN (Franzosa et al., 2018) built-in pangenomes database to the organism present in the sample. Metagenomic and metatranscriptomic reads were then mapped using Bowtie2 to sample-specific pangenomes. Metagenomic and metatranscriptomic reads failing to align to the pangenome databases were then blasted against UniRef90 using DIAMOND (Buchfink et al., 2015). Hits were counted per gene family and normalized for length and alignment quality. Gene family abundances were then combined into structured pathways using MetaCyc (Caspi et al., 2014) and sum-normalized to relative abundances. Functional gene family tables were regrouped to KEGG’s orthologs and Enzyme (Enzyme commission number, i.e., EC numbers) with the provided humann2 UniRef90_to_KO and UniRef90_to_EC numbers mapping files, respectively (Franzosa et al., 2018).
Quantification of Species-Specific Transcriptional Activity
We determined species transcriptional activity as described in (Schirmer et al., 2018). Briefly, the pathway copies per million (cpm) proportions is computed for each species in the metagenomes (i.e., DNA samples) and metatranscriptomes (i.e., RNA samples) within all the subjects. Only pathways detected in both metagenomes and metatranscriptomes were considered. Differences in overall species-level transcriptional activity between sites and health status were tested using two-sided nonparametric Kruskal-Wallis tests and the generated p.values were FDR-corrected to adjust for multiple comparisons. Significant differences between groups were considered for p.values lower or equal to 0.05.
Results
Site-Specific Distribution of Predominant Streptococcus Species in Oral Health
Figure 1 displays relative abundance (DNA) of the 16 predominant Streptococcus species across all samples. As can be seen, the proportion of taxa of predominant Streptococcus species was significantly influenced by the oral site. Accordingly, Streptococcus parasanguinis, Streptococcus salivarius and Streptococcus infantis were the most abundant species identified in tongue biofilm, but almost completely absent in subgingival plaque. On the other hand, Streptococcus oralis, Streptococcus sanguinis and Streptococcus gordonii were all highly abundant in subgingival plaque, and only sporadically present in tongue biofilm. All Streptococcus species identified with high abundance in either tongue biofilm or subgingival plaque were concomitantly abundant in saliva samples collected from the same individual.
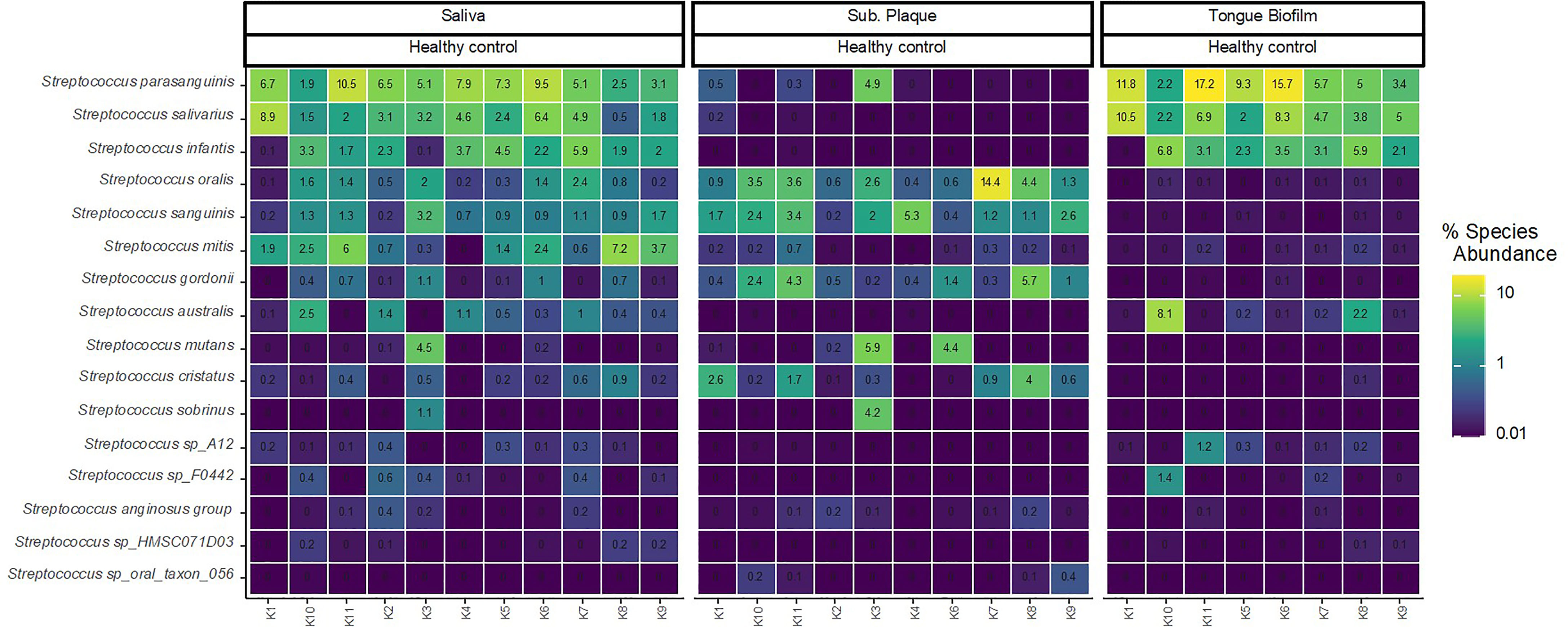
Figure 1 Site-specific distribution of predominant Streptococcus species. Relative abundance of the predominant Streptococcus species in 29 samples (saliva n = 11, subgingival plaque n = 10, tongue biofilm n = 8) in healthy conditions. Color code denotes the percentage read abundance, where light colors indicates increasing abundance and dark colors decreasing abundance.
Oral Site Associates With Overall Activity of Predominant Streptococcus Species in Oral Health
Bacterial activity, as evaluated by log2(RNA/DNA) of each pathway expressed by the selected predominant Streptococcus species, was compared between sites in orally healthy conditions. Figure 2A shows the distribution of log2(RNA/DNA) pathway level expression of S. parasanguinis, S. salivarius and S. infantis in tongue biofilm versus saliva samples. As can be seen, S. parasanguinis and S. infantis were significantly more transcriptionally active in saliva than in corresponding tongue biofilm. On the other hand, S. sanguinis, S. oralis and S. gordonii appeared significantly less active in saliva than in subgingival plaque samples collected from the same individual (Figure 2B).
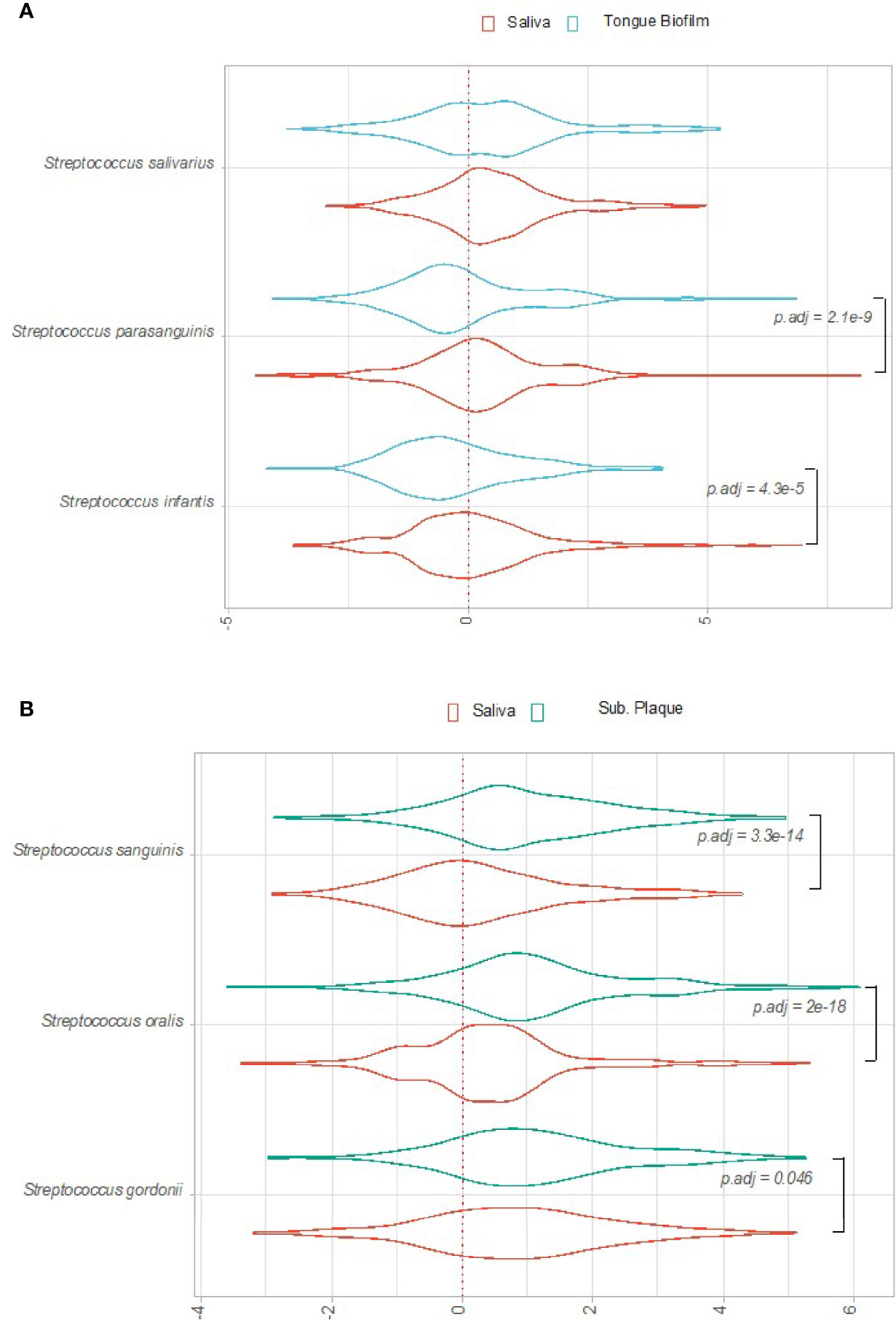
Figure 2 Overall transcriptional activity of predominant Streptococcus species in oral health. Transcriptional activity of predominant Streptococcus species, as evaluated by log2(RNA/DNA) with each dot representing a specific pathway. (A) Saliva versus tongue biofilm. (B) Saliva versus subgingival plaque. Color coding: red: saliva, blue: tongue biofilm, green: subgingival plaque.
Species-Specific Transcriptional Activity of Specific Pathways Differentiates Between Oral Sites in Health
Figures 3A–C show pathways of S. parasanguinis, S. infantis and S. salivarius, which were identified with significantly different Log2(RNA/DNA) expression in saliva versus tongue biofilm in healthy individuals. A total of 11, 4 and 2 pathways expressed by S. parasanguinis, S. infantis and S. salivarius, respectively, were recorded with significantly higher activity in saliva than in tongue biofilm. On the contrary, no pathways were identified with significantly higher expression by S. parasanguinis, S. infantis and S. salivarius found in tongue biofilm.
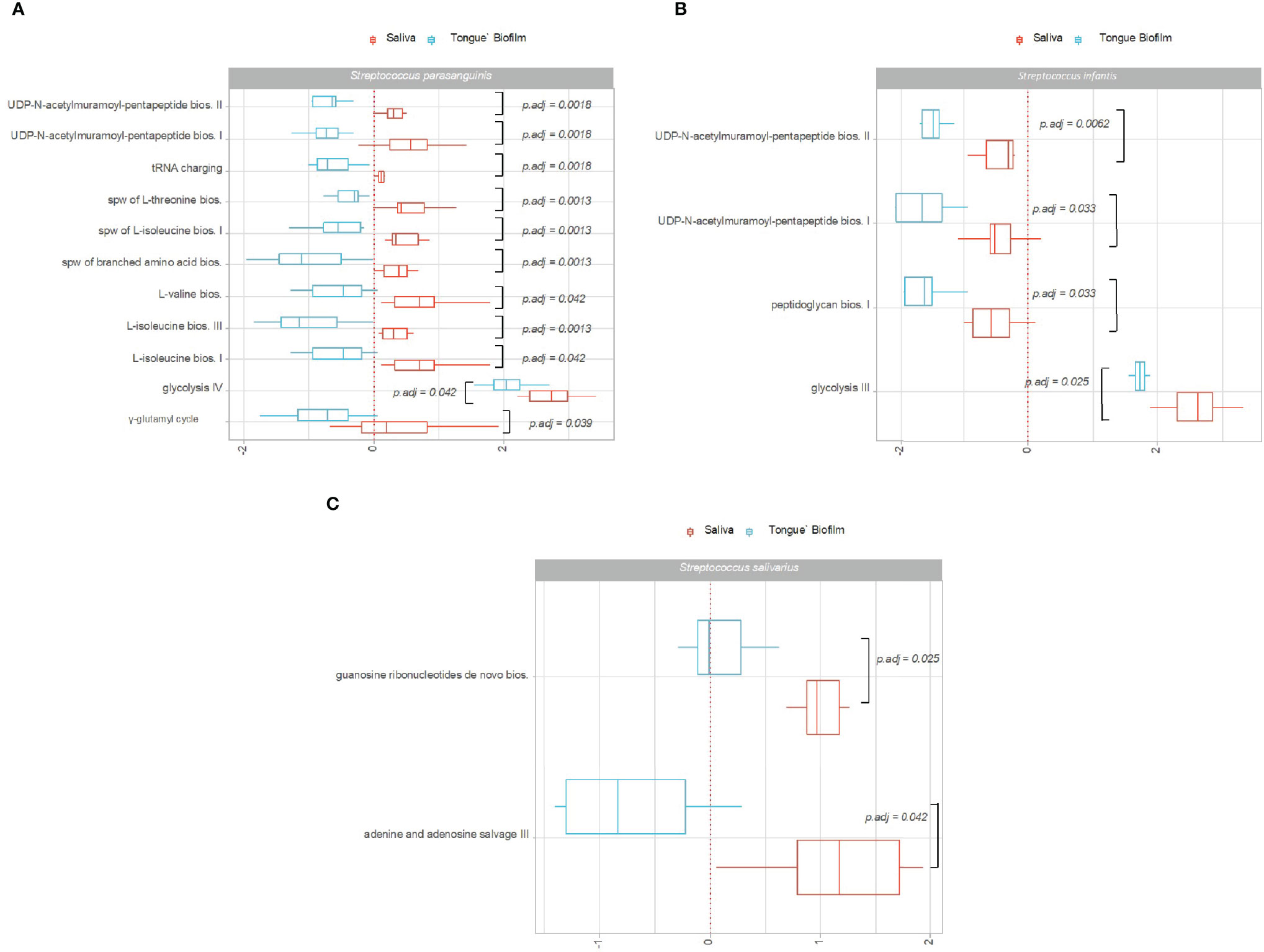
Figure 3 Pathway expression of predominant Streptococcus species in saliva versus tongue biofilm. Pathways identified with significantly different log2(RNA/DNA) in saliva versus tongue biofilm in oral health with each dot representing a specific sample. (A) Streptococcus parasanguinis, (B) Streptococcus infantis, (C) Streptococcus salivarius. Boxplots display first and third quartiles, median. Whiskers display 1.5 * IQR. Color coding: red: saliva, blue: tongue biofilm.
Significant transcriptional activity of pathways expressed by S. sanguinis and S. oralis in subgingival plaque versus saliva is visualized as Log2(RNA/DNA) in Figures 4A, B. As can be seen, 3 pathways expressed by S. oralis and one pathway expressed by S. sanguinis were observed with significantly higher transcriptional activity in subgingival plaque as compared to saliva in healthy individuals. On the other hand, no pathways were recorded with significantly higher transcriptional activity in saliva. Finally, no pathways were expressed significantly differently by S. gordonii in subgingival plaque versus saliva.
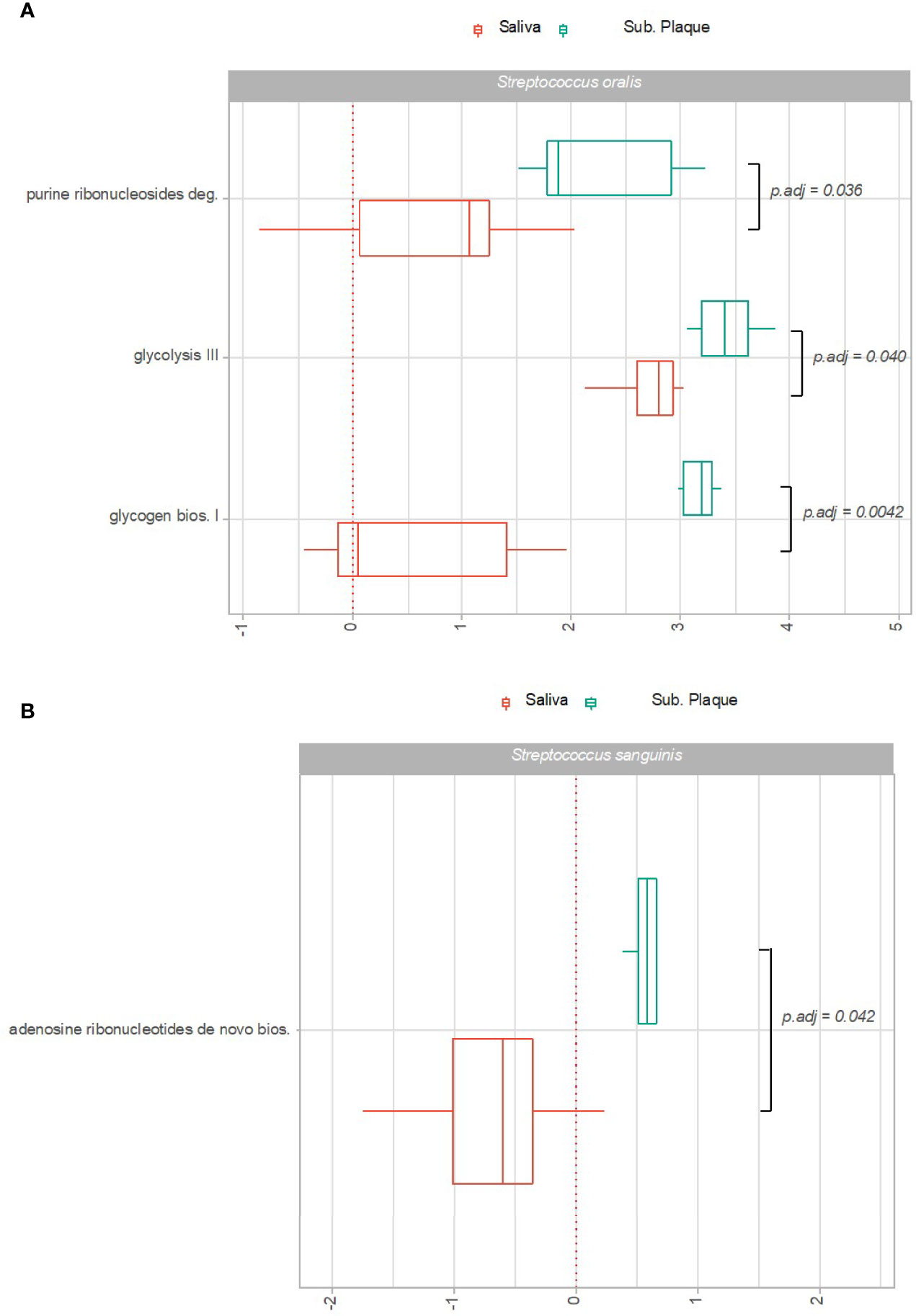
Figure 4 Pathway expression of predominant Streptococcus species in saliva versus subgingival plaque. Pathways identified with significantly different log2(RNA/DNA) in saliva versus subgingival plaque in oral health with each dot representing a specific sample. (A) Streptococcus oralis, (B) Streptococcus sanguinis. Boxplots display first and third quartiles, median. Whiskers display 1.5 * IQR. Color coding: red: saliva, green: subgingival plaque.
Periodontal Status Associates With Overall Bacterial Activity of Predominant Streptococcus Species
In general, higher transcriptional activity of Streptococcus species was observed in samples from orally healthy individuals than in patients with periodontitis. Accordingly, a significantly higher transcriptional activity of S. salivarius, S. parasanguinis, S. oralis, S. infantis and S. gordonii was noted in saliva from healthy controls as compared to patients with periodontitis. Likewise, significantly higher transcriptional activities of S. salivarius, S. parasanguinis, and S. oralis was seen in tongue biofilm from orally healthy individuals versus patients with periodontitis. Finally, a significantly higher activity of S. oralis was observed in subgingival plaque from healthy persons (Figure 5).
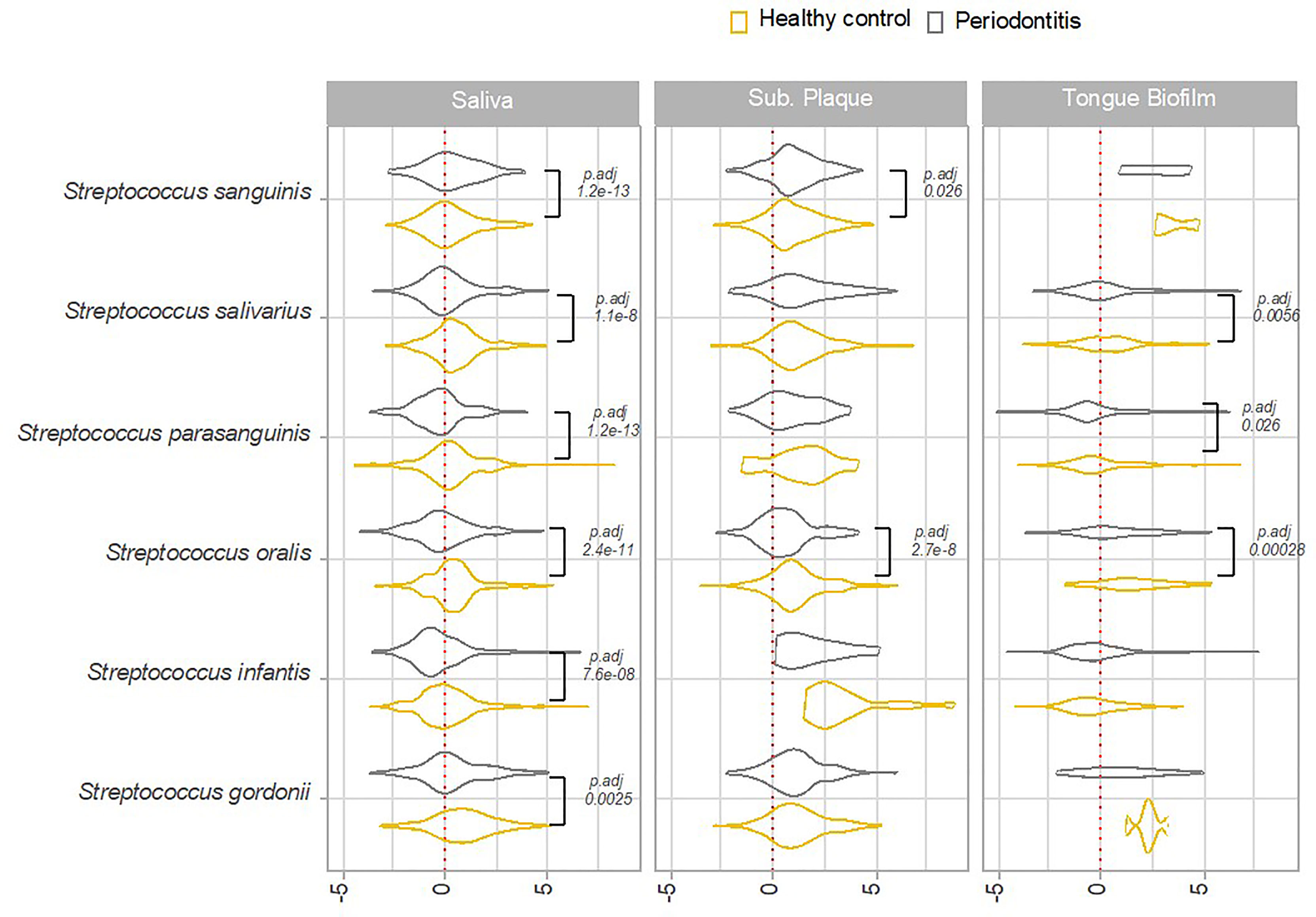
Figure 5 Overall transcriptional activity of Streptococcus species in oral health versus periodontitis. Transcriptional activity of predominant Streptococcus species in periodontitis versus oral health, as evaluated by log2(RNA/DNA), with each dot representing a specific pathway. Color coding: yellow: healthy control, grey: periodontitis.
Species-Specific Transcriptional Activity of Specific Pathways Differentiates Periodontitis From Oral Health
Transcriptional activity of specific pathways in saliva by S. parasanguinis and S. salivarius was significantly associated with oral health status. Specifically, transcriptional activity of 18 pathways expressed by S. parasanguinis and 3 pathways expressed by S. salivarius was significantly less transcribed in saliva from patients with periodontitis versus healthy controls (Figures 6A, B). In addition, three pathways expressed by S. parasanguinis were identified with significantly less transcriptional activity in tongue biofilm collected from periodontitis patients (Figure 7). Finally, no specific pathways were identified with significantly different transcriptional activity in subgingival plaque from periodontitis patients versus healthy controls.
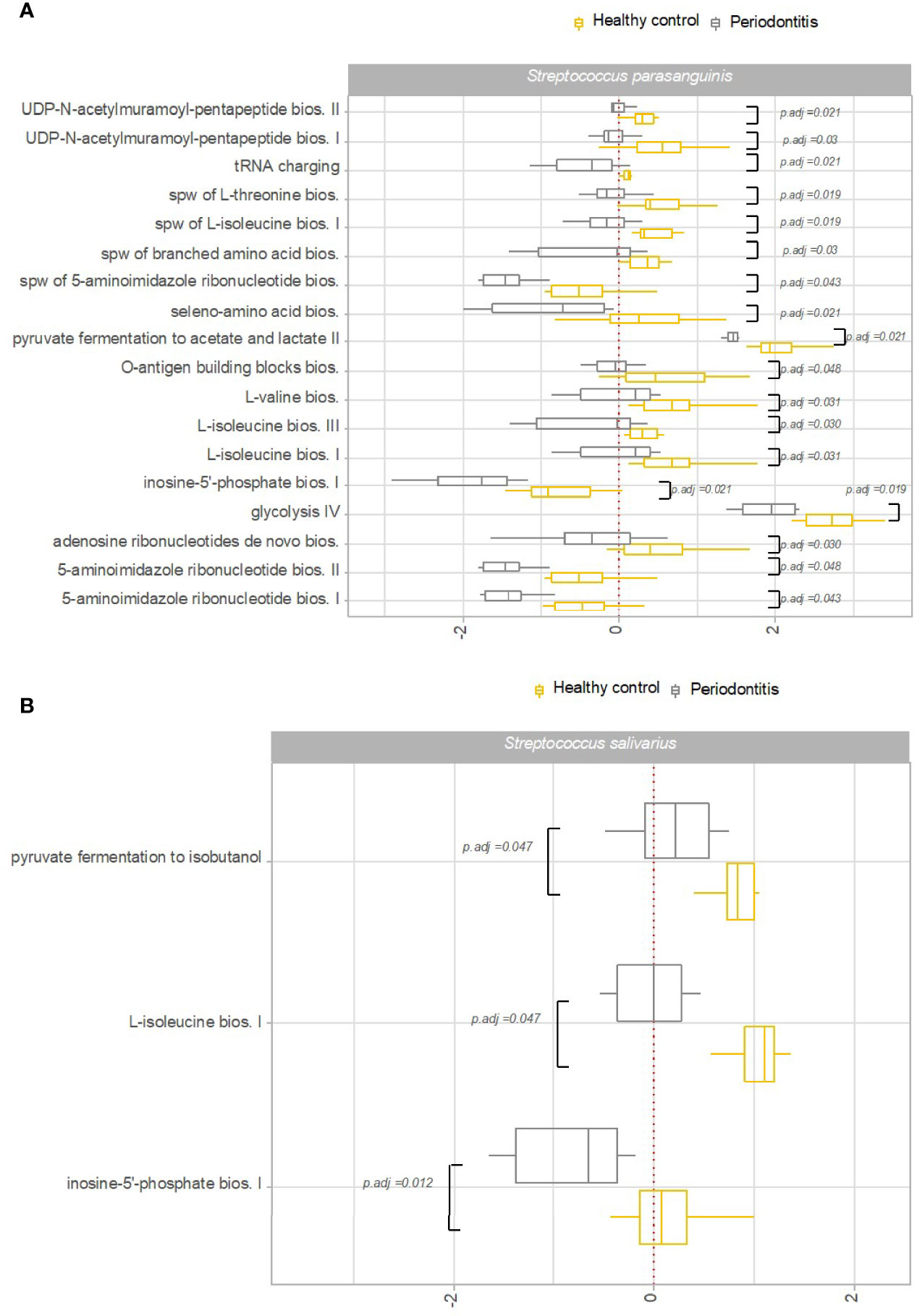
Figure 6 Pathway expression of Streptococcus species in saliva in periodontitis versus oral health. Pathways identified with significantly different log2(RNA/DNA) in saliva from patients with periodontitis versus oral health, with each dot representing a specific sample. (A) Streptococcus parasanguinis, (B) Streptococcus salivarius. Boxplots display first and third quartiles, median. Whiskers display 1.5 * IQR. Color coding: yellow: healthy control, grey: periodontitis.
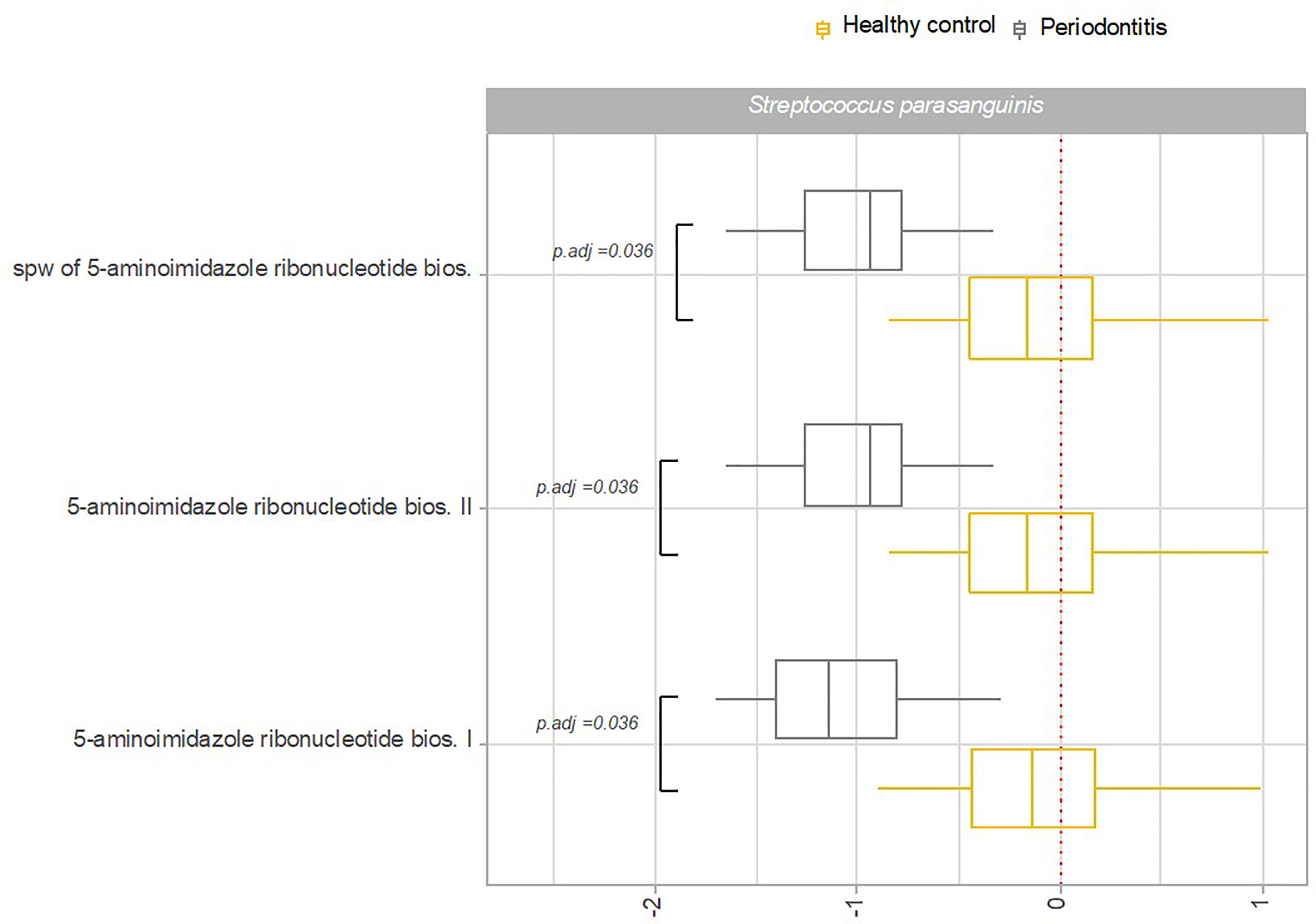
Figure 7 Pathway expression of Streptococcus species in tongue biofilm in periodontitis versus oral health. Pathways identified with significantly different log2(RNA/DNA) in tongue biofilm from patients with periodontitis versus oral health with each dot representing a specific sample. Streptococcus parasanguinis. Boxplots display first and third quartiles, median. Whiskers display 1.5 * IQR. Color coding: yellow: healthy control, grey: periodontitis.
Discussion
The purpose of the present study was to unravel if transcriptional activity of predominant Streptococcus species associates with ecological conditions determined by oral site and periodontitis. The main finding was that transcriptional activity of specific Streptococcus species differed significantly at various oral sites and in healthy oral conditions versus periodontitis. To the best of our knowledge, this is the first study to perform paired metagenomics and metatranscriptomics to characterize and compare transcriptional activity of predominant Streptococcus species in clinical samples collected from multiple oral compartments in healthy conditions and periodontitis.
The first important finding was that in health, the predominant Streptococcus species expressed a site-specific colonization pattern of the tongue and tooth surface. Accordingly, S. parasanguinis, S. salivarius and S. infantis were identified with high relative abundance in tongue biofilm, but almost completely absent in subgingival biofilm in the same individual. In contrast, S. oralis, S. sanguinis and S. gordonii expressed a complete opposite pattern with high abundance in subgingival biofilm in combination with near absence in tongue biofilm (Figure 1). Whole genome sequencing of most Streptococcus species, including parasanguinis and salivarius has been performed, and comparative genomics of genus Streptococcus has revealed that the average Streptococcus genome is comprised of approx. 1991 genes, out of which the core genome that is shared by all strains is approx. 369 genes (Gao et al., 2014). In addition, phylogenomic analyses of 138 Streptococcus genomes revealed branching of Streptococcus strains in two distinct groups, with one population comprised by Streptococcus pyogenes, bovis, mutans and salivarius, and the other population composed of Streptococcus mitis, anginosus and unknown groups (Gao et al., 2014). Interestingly, this branching was confirmed in the present study by site-specific preferences of S. salivarius versus S. mitis and mutans (Figure 1). Thus, the antagonistic colonization patterns most likely represent a combination of species-specific ecological preferences and different virulence factors, which facilitate interaction with surface adhesion molecules differing between the teeth and the tongue.
Species-specific transcriptional activity was associated with oral site in healthy conditions. In general, higher transcriptional activities were observed in saliva versus tongue biofilm, whereas higher activities were seen in subgingival plaque as compared to saliva (Figures 2A, B). In addition, major differences were noted in transcriptional activity of S. parasanguinis, S. infantis and S. salivarius in saliva versus tongue, but the association of site-specific ecological conditions and transcriptional activity in health differed significantly between these Streptococcus species (Figure 2A). Accordingly, 11, 4 and 2 pathways expressed by S. parasanguinis, S. infantis and S. salivarius, respectively, were identified with significantly higher transcriptional activity in saliva versus tongue biofilm (Figures 3A–C). Besides being identified with the most significant pathways, S. parasanguinis was also recorded with a characteristic activity pattern, with 10 out of 11 pathways identified being more active (log2(RNA/DNA)>0) in saliva and less active (log2(RNA/DNA)<0) in tongue biofilm (Figure 3A). Notably, most pathways identified as transcriptionally active were involved in either synthesis of peptidoglycan (UDP-N-acetylmuramoyl-pentapeptide biosynthesis), amino acid biosynthesis, or carbohydrate metabolism (glycolysis), which may be involved in supply of energy and molecules for biosynthetic processes and bacterial growth. Importantly, the main difference between saliva and tongue biofilm is oxygen availability and biofilm maturity stage, with the tongue being characterized by a mature biofilm thriving in anaerobic conditions (Segata et al., 2012).
Therefore, data which shows significantly higher transcriptional activity of S. parasanguinis of relevant metabolic pathways involved in bacterial growth and carbohydrate degradation, in aerobic versus anaerobic conditions, highlights the important role of the facultative anaerobe S. parasanguinis in initial biofilm formation performed in anaerobic environment. Indeed, this is in line with the current view of S. parasanguinis as one of the primary colonizers of the dental biofilm (Kolenbrander et al., 2000).
In general, minor differences were observed in species-specific transcriptional activity in saliva versus subgingival plaque in healthy conditions. Accordingly, only 3 pathways expressed by S. oralis and 1 pathways expressed by S. sanguinis were seen with significantly higher activity in subgingival plaque than in saliva (Figures 4A, B), whereas no significant pathways expressed by S. gordonii were identified. Importantly, the ecological conditions found in the subgingival environment in healthy conditions are in general more comparable with saliva, with aerobic surroundings on the tooth surface versus anaerobic conditions at the tongue surface (Wake et al., 2016; Hall et al., 2017). Likewise, as long as regular oral hygiene is maintained, the maturity stage of the biofilm found in the subgingival environment is immature as compared to that of the tongue (Belstrøm et al., 2018). Therefore, the finding of more comparable bacterial activity profiles in subgingival plaque and saliva versus saliva and tongue demonstrates the resilience of the permanent microbiota, in healthy conditions, to adapt to the ecological surroundings (Rosier et al., 2018).
An important finding, however, was that the 2 out of 3 pathways identified with different expression by S. oralis in subgingival plaque and saliva were involved in carbohydrate metabolism (glycolysis and glycogen biosynthesis). Therefore, data suggest that S. oralis is more efficient in carbohydrate degradation and storage, when being part of a polymicrobial biofilm, than in the planktonic environment offered by saliva. Indeed, this finding is of particular interest, since S. oralis has been reported to have an important role in homeostasis of oral biofilms (Thurnheer and Belibasakis, 2018). The capacity to synthesize intra- or extracellular polysaccharides or both, can promote survival of the bacteria and enables acid generation during starvation, in the absence of carbohydrate intake. Importantly, excessive carbohydrate metabolism of Streptococcus species, which leads to frequent critical decrease in pH of the biofilm, is the central act in the development of dental caries (Pitts et al., 2017). It is therefore interesting that we have recently demonstrated that a coordinated perturbation of short-term sugar stress induces a significant increase in relative abundance of Streptococcus species in the oral cavity even though regular oral hygiene is maintained (Lundtorp-Olsen et al., 2021). Indeed, carbohydrate metabolism of Streptococcus species may also be beneficial to maintenance of biofilm homeostasis, in conditions with transient increase in pH caused by local inflammation of the tooth supporting tissue – periodontitis (Nyvad and Takahashi, 2020). Thus, these findings underline the need of biofilm control through regular oral hygiene in combination with limited carbohydrate availability to control the doubled edged nature of carbohydrate metabolism performed by the predominant Streptococcus species (Philip et al., 2018).
Transcriptional activity in saliva was significantly associated with periodontitis, where significantly lower activities of S. parasanguinis, S. salivarius, S. infantis, S. gordonii and S. oralis were recorded. Periodontitis was also moderately associated with transcriptional activity of the tongue biofilm, as expressed by significantly lower activity of S. parasanguinis, S. salivarius and S. oralis. Finally, periodontitis was less associated with transcriptional activity of subgingival plaque, with S. sanguinis and S. oralis as the only species being significantly associated with oral health status (Figure 5). The finding of a significantly lower transcriptional activity of Streptococcus species in saliva is in concert with a previous study, where we identified lower bacterial activity of firmicutes in saliva from patients with periodontitis, versus healthy controls (Belstrøm et al., 2017). However, in that previous report we did not have the sequencing depth to reach species-level characterization of transcriptional activity. Thus, this study adds significantly to this finding by identification of transcriptional activity of specific members of genus Streptococcus as the part of the salivary microbiota to associate with periodontal status. It is however surprising that periodontitis was only minimally associated with transcriptional activity of Streptococcus species in subgingival plaque, when considering that this biofilm is the one found in close proximity to the local inflammation of the periodontal tissues (Kumar et al., 2021). Indeed, several studies have used metagenomics to identify a pathogenic signature of the subgingival microbiota in periodontitis (Wang et al., 2013; Shi et al., 2015). In that respect, one explanation might be that the ecological conditions, such as a decrease in oxygen availability and an increase in pH, which periodontitis exerts locally are more associated with increased activity of proposed periodontal pathogens (Duran-Pinedo et al., 2014), rather than a decrease in activity of Streptococcus species.
Another important finding was that periodontitis was differentially associated with transcriptional activity of predominant Streptococcus species in saliva. Accordingly, 18 pathways expressed by S. parasanguinis and 3 pathways expressed by S. salivarius were recorded with significantly less activity in patients with periodontitis, whereas the activity of specific pathways of the remaining predominant Streptococcus species was not associated with periodontitis (Figures 6A, B). Interestingly, transcriptional activity of both S. parasanguinis and S. salivarius of the same 3 pathways involved in 5-aminoimidazole ribonucleotide biosynthesis were associated with periodontitis (Figures 6A, B). In addition, S. parasanguinis was the only Streptococcus species identified in tongue biofilm, with activity that was associated with periodontitis (Figure 7). Notably, the majority of pathways expressed by S. parasanguinis, which were recorded with less activity in saliva from patients with periodontitis, were also involved in peptidoglycan and amino acid biosynthesis, and 10 out of 11 pathways that were identified with higher transcriptional activity in saliva versus tongue biofilm in health, were also associated with periodontitis. In addition, a significantly lower transcriptional activity of glycolysis expressed by S. parasanguinis was noted in saliva from patients with periodontitis versus healthy controls (Figure 6A).
Interestingly, data therefore suggest that transcriptional activity of S. parasanguinis, in contrast to the remaining predominant Streptococcus species characterized in the present study, is heavily associated with ecological characteristics offered by both oral site as and presence of chronic oral inflammation. Indeed, S. parasanguinis in saliva from orally healthy individuals were identified with significantly higher transcriptional activity of pathways involved in peptidoglycan and amino acid biosynthesis as well as glycolysis, than S. parasanguinis in concomitant tongue samples. On the contrary, conditions of periodontitis was associated with significantly less expression of the same pathways by S. parasanguinis in saliva. When considering that S. parasanguinis was highly abundant in both saliva and tongue biofilm (Figure 1), changes in transcriptional activity of this particular species would most likely affect the phenotypic profile of the total biofilm community with potential to affect oral homeostasis. Importantly, the two major oral diseases, i.e. dental caries and periodontitis are multifactorial diseases, where compositional changes of the oral microbiota play a critical role in pathogenesis. However, the specific species involved in the two diseases are critically different and possess almost antagonistic virulence factors (Marsh and Zaura, 2017). It is therefore noteworthy that S. parasanguinis is one of the only oral bacterial species that has been reported to associate with both caries (Aas et al., 2008), and has been given a role in periodontitis as accessory pathogen to the periodontal pathogen Aggregatibacter actinomycemcomitans (Fine et al., 2013). Thus, data from the present study illuminates the versatility of S. parasanguinis to thrive in areas with critically different ecological conditions, which could be an explanation as to why, this particular Streptococcus species associates with both dental caries and periodontitis.
The present study has several limitations, including the relative low sample size. However, the sample size was comparable with other metagenomics studies on the oral microbiota (Kumar et al., 2021). Studies with much larger sample sizes have been performed on the oral microbiota using more cost effective molecular methods such as microarray and 16S sequencing (Willis and Gabaldón, 2020), but these methods do not provide any information on bacterial activity, which was the purpose to address in the present study. Indeed, the sequencing depth of the current dataset in combination with concomitant sampling was sufficient to discriminate transcriptional activity of selected Streptococcus species. However, this was only possible as the selected species were predominant and identified with relative abundance ≥ 2% in all samples (Figure 1). Accordingly, a larger number of samples or an increased sequencing depth would be needed, to obtain species-level resolution of transcriptional activity of more peripheral members of the oral microbiota, including proposed periodontal pathogens such as Porphyromonas gingivalis, which is usually identified with relative abundance ≤ 1% (Damgaard et al., 2019). Also, current smokers were included in both the healthy and the periodontitis group. However, a comparable number of current smokers were present in the two groups studied. Smoking has been demonstrated to associate with characteristics of the subgingival microbiota in both healthy individuals and patients with periodontitis (Bizzarro et al., 2013; Mason et al., 2015), whereas the effect on the salivary microbiota is probably minimal (Belstrøm et al., 2014). Importantly, smoking has not been shown to associate with relative abundance or activity of predominant Streptococcus species, which is why smokers were not excluded in the present study. The main strength of the present study was concomitant sampling from multiple oral sites from both healthy individuals and patients with periodontitis, which facilitated the possibility to study the association of both site-specific ecological conditions and oral inflammation with transcriptional activity of the predominant members of the oral microbiota – the oral streptococci.
In conclusion, data from the present study clearly demonstrates the association of site-specific ecological conditions and presence of chronic oral inflammation with transcriptional activity of the predominant Streptococcus species of the oral microbiota. In particular, pathways expressed by S. parasanguinis being involved in peptidoglycan and amino acid biosynthesis and glycolysis were identified to be significantly associated with oral site and inflammation status. Further studies are needed to reveal if different activity patterns of S. parasanguinis link this particular bacterial species with dental caries and periodontitis.
Data Availability Statement
The datasets presented in this study can be found in online repositories. The names of the repository/repositories and accession number(s) can be found below: https://www.ncbi.nlm.nih.gov/, PRJNA678453.
Ethics Statement
The study was approved by the regional ethical committee (H-16016368) and reported to the local data authorization of University of Copenhagen (SUND-2018-8). The patients/participants provided their written informed consent to participate in this study.
Author Contributions
DB, FC, and MG defined the bioinformatic and biostatistical analyses, which were performed by FC. DB, FG, and MG wrote the first draft of the paper, which was critically revised by MM, MS, and CS. All authors contributed to the article and approved the submitted version.
Funding
Financial support was provided solely by SCELSE.
Conflict of Interest
The authors declare that the research was conducted in the absence of any commercial or financial relationships that could be construed as a potential conflict of interest.
Publisher’s Note
All claims expressed in this article are solely those of the authors and do not necessarily represent those of their affiliated organizations, or those of the publisher, the editors and the reviewers. Any product that may be evaluated in this article, or claim that may be made by its manufacturer, is not guaranteed or endorsed by the publisher.
Acknowledgments
We thank the volunteers, who with their samples contributed to this work. We are also grateful to SCELSE for funding and supporting the present study.
References
Aas, J. A., Griffen, A. L., Dardis, S. R., Lee, A. M., Olsen, I., Dewhirst, F. E., et al. (2008). Bacteria of Dental Caries in Primary and Permanent Teeth in Children and Young Adults. J. Clin. Microbiol. 46 (4), 1407–1417. doi: 10.1128/JCM.01410-07
Abranches, J., Zeng, L., Kajfasz, J. K., Palmer, S. R., Chakraborty, B., Wen, Z. T., et al. (2018). Biology of Oral Streptococci. Microbiol. Spectr. 6 (5). doi: 10.1128/microbiolspec.GPP3-0042-2018
Asakawa, M., Takeshita, T., Furuta, M., Kageyama, S., Takeuchi, K., Hata, J., et al. (2018). Tongue Microbiota and Oral Health Status in Community-Dwelling Elderly Adults. mSphere 3 (4), e00332–e00318. doi: 10.1128/mSphere.00332-18
Beghini, F., McIver, L. J., Blanco-Míguez, A., Dubois, L., Asnicar, F., Maharjan, S., et al. (2021). Integrating Taxonomic, Functional, and Strain-Level Profiling of Diverse Microbial Communities With Biobakery 3. Elife 10, e65088. doi: 10.7554/eLife.65088
Belstrøm, D. (2020). The Salivary Microbiota in Health and Disease. J. Oral. Microbiol. 12 (1), 1723975. doi: 10.1080/20002297.2020.1723975
Belstrøm, D., Constancias, F., Drautz-Moses, D. I., Schuster, S. C., Veleba, M., Mahé, F., et al. (2021). Species-Specific Gene Expression of the Oral Microbiota Reflects Ecological Conditions. NPJ Biofilms Microbiomes. Accepted for publication. doi: 10.1038/s41522-021-00247-y
Belstrøm, D., Constancias, F., Liu, Y., Yang, L., Drautz-Moses, D. I., Schuster, S. C., et al. (2017). Metagenomic and Metatranscriptomic Analysis of Saliva Reveals Disease-Associated Microbiota in Patients With Periodontitis and Dental Caries. NPJ Biofilms Microbiomes. 3, 23. doi: 10.1038/s41522-017-0031-4
Belstrøm, D., Holmstrup, P., Nielsen, C. H., Kirkby, N., Twetman, S., Heitmann, B. L., et al. (2014). Bacterial Profiles of Saliva in Relation to Diet, Lifestyle Factors, and Socioeconomic Status. J. Oral. Microbiol. 6. doi: 10.3402/jom.v6.23609
Belstrøm, D., Sembler-Møller, M. L., Grande, M. A., Kirkby, N., Cotton, S. L., Paster, B. J., et al. (2018). Impact of Oral Hygiene Discontinuation on Supragingival and Salivary Microbiomes. JDR Clin. Trans. Res. 3 (1), 57–64 doi: 10.1177/2380084417723625
Berger, D., Rakhamimova, A., Pollack, A., Loewy, Z. (2018). Oral Biofilms: Development, Control, and Analysis. High Throughput. 7 (3), 24. doi: 10.20944/preprints201808.0174.v1
Bizzarro, S., Loos, B. G., Laine, M. L., Crielaard, W., Zaura, E. (2013). Subgingival Microbiome in Smokers and non-Smokers in Periodontitis: An Exploratory Study Using Traditional Targeted Techniques and a Next-Generation Sequencing. J. Clin. Periodontol. 40 (5), 483–492. doi: 10.1111/jcpe.12087
Buchfink, B., Xie, C., Huson, D. H. (2015). Fast and Sensitive Protein Alignment Using DIAMOND. Nat. Methods 12 (1), 59–60. doi: 10.1038/nmeth.3176
Busuioc, M., Mackiewicz, K., Buttaro, B. A., Piggot, P. J. (2009). Role of Intracellular Polysaccharide in Persistence of Streptococcus Mutans. J. Bacteriol. 191 (23), 7315–7322. doi: 10.1128/JB.00425-09
Caspi, R., Altman, T., Billington, R., Dreher, K., Foerster, H., Fulcher, C. A., et al. (2014). The MetaCyc Database of Metabolic Pathways and Enzymes and the BioCyc Collection of Pathway/Genome Databases. Nucleic Acids Res. 42 (Database issue), D459–D471. doi: 10.1093/nar/gkt1103
Chattopadhyay, I., Verma, M., Panda, M. (2019). Role of Oral Microbiome Signatures in Diagnosis and Prognosis of Oral Cancer. Technol. Cancer Res. Treat. 18, 1533033819867354. doi: 10.1177/1533033819867354
Damgaard, C., Danielsen, A. K., Enevold, C., Massarenti, L., Nielsen, C. H., Holmstrup, P., et al. (2019). Porphyromonas Gingivalis in Saliva Associates With Chronic and Aggressive Periodontitis. J. Oral. Microbiol. 11 (1), 1653123. doi: 10.1080/20002297.2019.1653123
Dewhirst, F. E., Chen, T., Izard, J., Paster, B. J., Tanner, A. C., Yu, W. H., et al. (2010). The Human Oral Microbiome. J. Bacteriol. 192 (19), 5002–5017. doi: 10.1128/JB.00542-10
Duran-Pinedo, A. E., Chen, T., Teles, R., Starr, J. R., Wang, X., Krishnan, K., et al. (2014). Community-Wide Transcriptome of the Oral Microbiome in Subjects With and Without Periodontitis. ISME J. 8 (8), 1659–1672. doi: 10.1038/ismej.2014.23
Fellows Yates, J. A., Velsko, I. M., Aron, F., Posth, C., Hofman, C. A., Austin, R. M., et al. (2021). The Evolution and Changing Ecology of the African Hominid Oral Microbiome. Proc. Natl. Acad. Sci. U. S. A. 118 (20), e2021655118. doi: 10.1073/pnas.2021655118
Fine, D. H., Markowitz, K., Fairlie, K., Tischio-Bereski, D., Ferrendiz, J., Furgang, D., et al. (2013). A Consortium of Aggregatibacter Actinomycetemcomitans, Streptococcus Parasanguinis, and Filifactor Alocis Is Present in Sites Prior to Bone Loss in a Longitudinal Study of Localized Aggressive Periodontitis. J. Clin. Microbiol. 51 (9), 2850–2861. doi: 10.1128/JCM.00729-13
Franzosa, E. A., McIver, L. J., Rahnavard, G., Thompson, L. R., Schirmer, M., Weingart, G., et al. (2018). Species-Level Functional Profiling of Metagenomes and Metatranscriptomes. Nat. Methods 15 (11), 962–968. doi: 10.1038/s41592-018-0176-y
Gao, X. Y., Zhi, X. Y., Li, H. W., Klenk, H. P., Li, W. J. (2014). Comparative Genomics of the Bacterial Genus Streptococcus Illuminates Evolutionary Implications of Species Groups. PloS One 9 (6), e101229. doi: 10.1371/journal.pone.0101229
Hall, M. W., Singh, N., Ng, K. F., Lam, D. K., Goldberg, M. B., Tenenbaum, H. C., et al. (2017). Inter-Personal Diversity and Temporal Dynamics of Dental, Tongue, and Salivary Microbiota in the Healthy Oral Cavity. NPJ Biofilms Microbiomes. 3, 2. doi: 10.1038/s41522-016-0011-0
Jenkinson, H. F. (1994). Cell Surface Protein Receptors in Oral Streptococci. FEMS Microbiol. Lett. 121 (2), 133–140. doi: 10.1111/j.1574-6968.1994.tb07089.x
Kilian, M., Chapple, I. L., Hannig, M., Marsh, P. D., Meuric, V., Pedersen, A. M., et al. (2016). The Oral Microbiome - An Update for Oral Healthcare Professionals. Br. Dent. J. 221 (10), 657–666. doi: 10.1038/sj.bdj.2016.865
Kolenbrander, P. E., Palmer, R. J., Jr, Rickard, A. H., Jakubovics, N. S., Chalmers, N. I., Diaz, P. I. (2000). Bacterial Interactions and Successions During Plaque Development. Periodontol 2006 (42), 47–79. doi: 10.1111/j.1600-0757.2006.00187.x
Koo, H., Falsetta, M. L., Klein, M. I. (2013). The Exopolysaccharide Matrix: A Virulence Determinant of Cariogenic Biofilm. J. Dent. Res. 92 (12), 1065–1073. doi: 10.1177/0022034513504218
Kumar, P. S., Dabdoub, S. M., Ganesan, S. M. (2021). Probing Periodontal Microbial Dark Matter Using Metataxonomics and Metagenomics. Periodontol 2000. 85 (1), 12–27. doi: 10.1111/prd.12349
Lal, D., Verma, M., Lal, R. (2011). Exploring Internal Features of 16S rRNA Gene for Identification of Clinically Relevant Species of the Genus Streptococcus. Ann. Clin. Microbiol. Antimicrob. 10, 28. doi: 10.1186/1476-0711-10-28
Lamont, R. J., Koo, H., Hajishengallis, G. (2018). The Oral Microbiota: Dynamic Communities and Host Interactions. Nat. Rev. Microbiol. 16 (12), 745–759. doi: 10.1038/s41579-018-0089-x
Lundtorp-Olsen, C., Enevold, C., Juel Jensen, C. A., Stofberg, S. N., Twetman, S., Belstrøm, D. (2021). Impact of Probiotics on the Salivary Microbiota and Salivary Levels of Inflammation-Related Proteins During Short-Term Sugar Stress: A Randomized Controlled Trial. Pathogens 10 (4), 392. doi: 10.3390/pathogens10040392
Marsh, P. D., Zaura, E. (2017). Dental Biofilm: Ecological Interactions in Health and Disease. J. Clin. Periodontol. 44 (Suppl 18), S12–S22. doi: 10.1111/jcpe.12679
Mason, M. R., Preshaw, P. M., Nagaraja, H. N., Dabdoub, S. M., Rahman, A., Kumar, P. S. (2015). The Subgingival Microbiome of Clinically Healthy Current and Never Smokers. ISME J. 9 (1), 268–272. doi: 10.1038/ismej.2014.114
Moye, Z. D., Zeng, L., Burne, R. A. (2014). Fueling the Caries Process: Carbohydrate Metabolism and Gene Regulation by Streptococcus Mutans. J. Oral. Microbiol., 6. doi: 10.3402/jom.v6.24878
Nyvad, B., Takahashi, N. (2020). Integrated Hypothesis of Dental Caries and Periodontal Diseases. J. Oral. Microbiol. 12 (1), 1710953. doi: 10.1080/20002297.2019.1710953
Philip, N., Suneja, B., Walsh, L. J. (2018). Ecological Approaches to Dental Caries Prevention: Paradigm Shift or Shibboleth? Caries Res. 52 (1-2), 153–165. doi: 10.1159/000484985
Pitts, N. B., Zero, D. T., Marsh, P. D., Ekstrand, K., Weintraub, J. A., Ramos-Gomez, F., et al. (2017). Dental Caries. Nat. Rev. Dis. Primers. 3, 17030. doi: 10.1038/nrdp.2017.30
Rosier, B. T., Marsh, P. D., Mira, A. (2018). Resilience of the Oral Microbiota in Health: Mechanisms That Prevent Dysbiosis. J. Dent. Res. 97 (4), 371–380. doi: 10.1177/0022034517742139
Schirmer, M., Franzosa, E. A., Lloyd-Price, J., McIver, L. J., Schwager, R., Poon, T. W., et al. (2018). Dynamics of Metatranscription in the Inflammatory Bowel Disease Gut Microbiome. Nat. Microbiol. 3 (3), 337–346. doi: 10.1038/s41564-017-0089-z
Schrøder, S. A., Bardow, A., Eickhardt-Dalbøge, S., Johansen, H. K., Homøe, P. (2017). Is Parotid Saliva Sterile on Entry to the Oral Cavity? Acta Otolaryngol. 137 (7), 762–764. doi: 10.1080/00016489.2016.1272002
Segata, N., Haake, S. K., Mannon, P., Lemon, K. P., Waldron, L., Gevers, D., et al. (2012). Composition of the Adult Digestive Tract Bacterial Microbiome Based on Seven Mouth Surfaces, Tonsils, Throat and Stool Samples. Genome Biol. 13 (6), R42. doi: 10.1186/gb-2012-13-6-r42
Shi, B., Chang, M., Martin, J., Mitreva, M., Lux, R., Klokkevold, P., et al. (2015). Dynamic Changes in the Subgingival Microbiome and Their Potential for Diagnosis and Prognosis of Periodontitis. mBio 6 (1), e01926–e01914. doi: 10.1128/mBio.01926-14
Takahashi, N. (2015). Oral Microbiome Metabolism: From "Who Are They?" to "What Are They Doing?". J. Dent. Res. 94 (12), 1628–1637. doi: 10.1177/0022034515606045
Thurnheer, T., Belibasakis, G. N. (2018). Streptococcus Oralis Maintains Homeostasis in Oral Biofilms by Antagonizing the Cariogenic Pathogen Streptococcus Mutans. Mol. Oral. Microbiol. 33 (3), 234–239. doi: 10.1111/omi.12216
Wake, N., Asahi, Y., Noiri, Y., Hayashi, M., Motooka, D., Nakamura, S., et al. (2016). Temporal Dynamics of Bacterial Microbiota in the Human Oral Cavity Determined Using an In Situ Model of Dental Biofilms. NPJ Biofilms Microbiomes. 2, 16018. doi: 10.1038/npjbiofilms.2016.18
Wang, J., Qi, J., Zhao, H., He, S., Zhang, Y., Wei, S., et al. (2013). Metagenomic Sequencing Reveals Microbiota and Its Functional Potential Associated With Periodontal Disease. Sci. Rep. 3, 1843. doi: 10.1038/srep01843
Keywords: periodontitis, Streptococcus, metagenomics, metatranscriptomics, oral cavity
Citation: Belstrøm D, Constancias F, Markvart M, Sikora M, Sørensen CE and Givskov M (2021) Transcriptional Activity of Predominant Streptococcus Species at Multiple Oral Sites Associate With Periodontal Status. Front. Cell. Infect. Microbiol. 11:752664. doi: 10.3389/fcimb.2021.752664
Received: 03 August 2021; Accepted: 07 September 2021;
Published: 21 September 2021.
Edited by:
Marcia Pinto Alves Mayer, University of São Paulo, BrazilReviewed by:
Ashu Sharma, University at Buffalo, United StatesJ Christopher Fenno, University of Michigan, United States
Copyright © 2021 Belstrøm, Constancias, Markvart, Sikora, Sørensen and Givskov. This is an open-access article distributed under the terms of the Creative Commons Attribution License (CC BY). The use, distribution or reproduction in other forums is permitted, provided the original author(s) and the copyright owner(s) are credited and that the original publication in this journal is cited, in accordance with accepted academic practice. No use, distribution or reproduction is permitted which does not comply with these terms.
*Correspondence: Daniel Belstrøm, dbel@sund.ku.dk; Florentin Constancias, florentinconstancias@gmail.com
†These authors have contributed equally to this work