- 1Department of Laboratory Medicine, The First Affiliated Hospital of Guangzhou Medical University, Guangzhou, China
- 2Department of Infectious Diseases and Public Health, Jockey Club College of Veterinary Medicine and Life Sciences, City University of Hong Kong, Hong Kong, Hong Kong, SAR, China
- 3State Key Lab of Chemical Science and Drug Discovery, Department of Applied Biology and Chemical Technology, The Hong Kong Polytechnic University, Hong Kong, Hong Kong, SAR, China
Acinetobacter sp. is among the ESKAPE organisms which represent the major nosocomial pathogens that exhibited a high resistance rate. A. pittii, frequently associated with antimicrobial resistance particularly to carbapenems, is one of the most common Acinetobacter species causing invasive infection. Pandrug resistant A. pittii has rarely been reported. Here, we report the case of a patient with acute exacerbations of chronic obstructive pulmonary disease three years after double lung transplantation and developed severe pneumonia associated with pandrug resistant A. pittii infection. Phenotypic and genomic characteristics of this pandrug resistant isolate (17-84) was identified, and the mechanisms underlying its resistance phenotypes were analyzed. Isolate 17-84 belonged to ST63, carried a non-typable and non-transferable plasmid encoding multiple acquired resistance genes including carbapenemase gene blaOXA-58. Point mutations and acquired resistance genes were identified which were associated with different drug resistance phenotypes. To our knowledge, this is the first detailed phenotypic and genomic characterization of PDR A. pittii causing severe infections in clinical settings. Findings from us and others indicate that A. pittii could serve as a reservoir for carbapenem determinants. The emergence of such a superbug could pose a serious threat to public health. Further surveillance of PDR A. pittii strains and implementation of stricter control measures are needed to prevent this emerging pathogen from further disseminating in hospital settings and the community.
Introduction
Acinetobacter species are among the high-priority nosocomial pathogens which could cause severe infections among immunocompromised patients (Howard et al., 2012). Infections caused by Acinetobacter spp. could be untreatable as they exhibit the potential to develop resistance to a wide range of antibiotics, particularly carbapenems (Wong et al., 2019). Carbapenem resistance in Acinetobacter spp. is mainly caused by the production of carbapenem-hydrolyzing class D β-lactamases (CHDLs) including OXA-23-like, OXA-58-like, and OXA-24-like enzymes (Ayibieke et al., 2020). Acinetobacter pittii, formerly termed genomospecies 3 of the A. calcoaceticus-A. baumannii complex, is a close relative of A. baumanii which is increasingly recognized as a significant cause of hospital-acquired infections (Chusri et al., 2014). A recent multicenter investigation in Japan suggested A. pittii was the most common species causing invasive Acinetobacter infection (Kiyasu et al., 2020). The terms multidrug resistance (MDR), extensive drug resistance (XDR) and pandrug resistance (PDR) were used worldwide to define the non-susceptibility level of isolates, which are resistant to at least one agent in three or more antimicrobial categories, to at least one agent in all but two or fewer categories and to all agents in all categories, respectively (Magiorakos et al., 2012). MDR A. pittii including those resistant to antibiotics quinolones, carbapenems, etc., was reported across continents, yet PDR A. pittii was rarely reported (Gu et al., 2015; Brasiliense et al., 2019; Chen et al., 2019a; Cosgaya et al., 2019; Chopjitt et al., 2021). Here, we report the case of a patient with acute exacerbations of chronic obstructive pulmonary disease (AECOPD) three years after double lung transplantation (DLT) and developed severe pneumonia associated with PDR A. pittii infection. Currently available therapeutic options for such isolates remained limited and relied largely on antibiotics recently approved by the Food and Drug Administration (FDA) and European Medicines Agency (EMA) such as eravacycline, rifabutin and cefiderocol (Livermore et al., 2016; Trecarichi et al., 2019). This severe situation called for urgent action to prevent the healthcare-associated transmission of such PDR A. pittii.
Materials and Methods
Patient Information and Strain Isolation
In 2017, a 59-year-old male patient was admitted to hospital (Day 0) with a diagnosis of AECOPD. The patient underwent DLT three years before hospitalization and regular medical examinations revealed no abnormalities after DLT. On Day 2, the patient was referred to an intensive care unit (ICU) due to the development of severe symptoms complicated with type 2 respiratory failure. A CT scan showed inflammations and fibrosis, reduced transparency and patchy shadows in both lungs, inferring occurrence of bilaterally pleural effusion. He received mechanical ventilation and other supporting treatments. On Day 20, Stenotrophomonas maltophilia, Acinetobacter sp. and Aspergillus sp. strains were recoverable from the sputum sample. On Day 25, a sputum culture became positive for carbapenem-resistant Acinetobacter sp. Amphotericin B, imipenem, meropenem and polymyxin B were introduced. A tracheal aspiration culture on Day 34 was positive for carbapenem- and colistin-resistant Acinetobacter sp. From Day 35, lung infection worsened with giant pulmonary bulla and gradual decline in leukocytes is continued. The patient discharged following critical illness and was transferred to another medical center on Day 41. A sputum culture performed on Day 35 flagged positive for carbapenem- and colistin-resistant Acinetobacter sp. (isolate 17-84).
Species Identification, Antimicrobial Susceptibility Testing, and Conjugation Assays
The species of the isolate was identified using the matrix-assisted laser desorption ionization/time of flight mass spectrometry (MALDI-TOF MS) (Bruker Daltonik GmbH, Bremen, Germany) and verified with the whole genome sequencing results. Antimicrobial susceptibility of 15 commonly used antibiotics (amikacin, gentamicin, kanamycin, meropenem, imipenem, ertapenem, cefotaxime, ampicillin, aztreonam, ciprofloxacin, colistin, tetracycline, tigecycline, trimethoprim-sulfamethoxazole, azithromycin) was tested using broth dilution method and interpreted according to the Clinical Laboratory Standards Institute (CLSI) guidelines, with the exception of tigecycline and colistin (CLSI, 2020). The breakpoint of colistin was interpreted according to the European Committee on Antimicrobial Susceptibility Testing (EUCAST) recommendations (EUCAST, 2020). No interpretive criteria are available for tigecycline from CLSI, EUCAST or FDA. Plasmid transferability was tested by conjugation using a rifampicin-resistant mutant of A. baumannii ATCC 17978 as the recipient strain. Presumptive transconjugants were selected using Mueller-Hinton II agar plates supplemented with meropenem (0.5 mg/L) and rifampicin (600 mg/L).
Whole Genome Sequencing and Bioinformatics Analysis
Genomic DNA was extracted from overnight cultures by using the PureLink Genomic DNA Mini Kit (Invitrogen, Carlsbad, CA, USA). Whole genome sequencing was performed using both the Illumina HiSeq (Illumina, San Diego, CA) and the Oxford nanopore MinION (Oxford, UK) platforms. Hybrid assembly of both sequencing reads was conducted using Unicycler (v 0.4.4) (Wick et al., 2017). Complete genome sequence was annotated by the RAST tool and edited manually (Overbeek et al., 2013). Multilocus sequence typing (MLST) was conducted using MLST v2.1 (Seemann, 2021). Acquired antibiotic resistance genes were identified by ResFinder 4.1 (Bortolaia et al., 2020). Plasmid replicons were analyzed using the A. baumannii PCR-based replicon typing (AB-PBRT) scheme (Bertini et al., 2010). Insertion sequences (ISs) are identified using ISfinder (Siguier et al., 2006). Plasmid map was plotted using DNAPlotter v1.11 (Carver et al., 2009). Mutations in the pmrCAB and lpxACD operons which were associated with polymyxin resistance were determined by aligning their amino acid sequences of strain 17-84 with that of a polymyxin-susceptible strain A. pittii ST220 (NZ_CP029610) (Zhang et al., 2018). Point mutations conferring resistance to quinolones (gyrA/B and parC/E) were detected by comparing the coding sequences in the 17-84 genome with the corresponding sequences in the genome of an ST63 quinolone-susceptible A. pittii strain in our collection (data not shown).
Results and Discussion
Isolate 17-84 was identified as A. pittii, displaying a PDR profile to aminoglycoside (amikacin, gentamicin, kanamycin), β-lactam (meropenem, imipenem, ertapenem, cefotaxime, ampicillin, aztreonam), quinolone (ciprofloxacin), polymyxin (colistin), tetracycline (tetracycline), sulfonamide (trimethoprim-sulfamethoxazole) and macrolide (azithromycin). MIC values and the associated mechanism of resistance are shown on Table 1.
Isolate 17-84 contained a 3,914,011 bp chromosome and a 108,715 bp plasmid designated as p17-84_OXA. It belonged to ST63 A. pittii (Pasteur Scheme). Resistance genes including blaADC-25 and blaOXA-51 both not associated with insertion sequences were identified on the chromosome of isolate 17-84. blaADC-25 and blaOXA-51-like genes were both naturally occurring β-lactamase-encoding genes in Acinetobacter spp. which does not contribute to intrinsic β-lactam resistance unless an insertion sequence such as ISAba1 is located upstream (Zhou et al., 2020). Previous studies have reported the mutations on different chromosome-encoded genes associated with quinolone, polymyxin and tetracycline resistance among Acinetobacter spp. In isolate 17-84, quinolone resistance-associated mutations in topoisomerase parC (S84L), DNA gyrase gyrA (S81L) and gyrB (A414T) genes were detected. Among these, gyrA (S81L) and parC (S84L) target site mutations have been demonstrated to lower the affinity for quinolone, while the function of A414T mutation in gyrB remained to be investigated (Hujer et al., 2009). The major mechanisms of resistance to colistin in Acinetobacter sp. included complete loss of lipopolysaccharide (LPS) resulting from mutations in lpxACD genes, and phosphoethanolamine addition to LPS mediated through mutations in the pmrCAB operon (Beceiro et al., 2014). Mutations in the pmrC gene including Q143L, L146F, Q148K, I171V, and L258S were detected in strain 17-84 and whether these mutations contributed to the colistin resistance phenotype remined to be studied. No acquired tetracycline resistance determinant was observed in strain 17-84, and its resistance to tetracycline could be associated with the presence of diverse efflux pump genes (adeDE, adeN-adeIJK, adeL-adeFGH, adeRS-adeAB) belonging to the resistance-nodulation-division (RND) family that were reported to confer multidrug resistance (Pagdepanichkit et al., 2016). The function of these efflux pump genes warrant further verification.
Plasmid p17-84_OXA contained 132 ORFs with a GC content of 39.6%. It was 100% identical to the 105,591 bp plasmid pOXA58_100004 (CP027249) from an A. pittii strain isolated from Sichuan province in China at 90% coverage. Compared with pOXA58_100004, plasmid p17-84_OXA has lost the floR resistance gene and gained a ~9 Kb fragment encoding hypothetical proteins. p17-84_OXA and pOXA58_100004 both carried multiple antimicrobial resistance genes, including aph(3’)-VIb, aac(3’)-IId, blaOXA-58, blaPER-1, sul2, msr(E), mph(E) and strA (2 copies) and strB genes (Figure 1). aph(3’)-VIb and aac(3)-IId confer aminoglycoside resistance by encoding O-phosphotransferase-type and N-acetyltransferase-type aminoglycoside-modifying enzymes, respectively. blaOXA-58 and blaPER-1 confer β-lactam resistance, with the former encoding carbapenem-hydrolysing class D β-lactamase oxacillinase OXA-58. blaOXA-58 was bracketed by insertion sequences ISAba3 with the genetic structure of ISAba3-blaOXA-58-ISAba3. ISAba3 drove the overexpression of blaOXA-58, which is associated with imipenem resistance (Nguyen et al., 2020). sul2 confers resistance to sulfonamide. msr(E) and mph(E) are associated with macrolide resistance. strA and strB genes confer resistance to streptomycin. p17-84_OXA was non-typeable by AB-PBRT and non-transferable by conjugation under the experimental conditions.
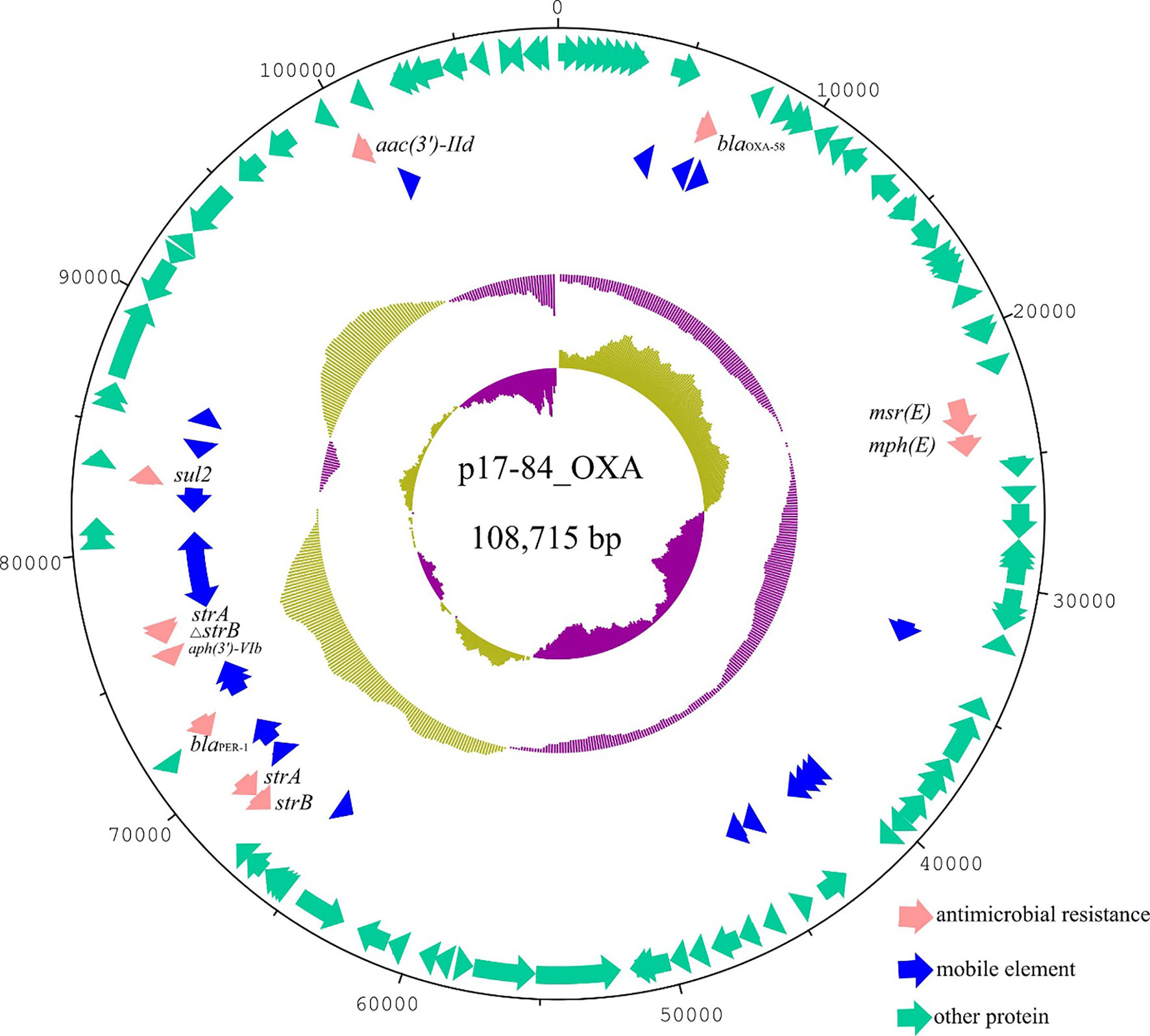
Figure 1 Circular plasmid map of p17-84_OXA. Pink, blue, and green arrows indicated the antimicrobial resistance genes, mobile elements and other ORFs, respectively. Antimicrobial resistance genes carried by the plasmid were labelled.
Infections caused by MDR or PDR A. baumannii have been widely acknowledged, whereas non-baumannii Acinetobacter spp. infections are rarely reported in China (Yang et al., 2012; Zarrilli et al., 2013; Al Atrouni et al., 2016). The phenotypical species identification methods available to most diagnostic laboratories were not able to accurately differentiate the species belonging to the A. baumannii complex (Vijayakumar et al., 2019). The technological limitations in species discrimination have led to underestimation of non-baumannii Acinetobacter species in human infections (Pailhories et al., 2018). This could have led to the rare report of infections caused by PDR A. pitti previously, even though the pandrug-resistance phenotype in A. baumannii complex is common in some clinical settings (Karakonstantis et al., 2020a; Karakonstantis et al., 2020b). According to a recent study from a French hospital, A. pittii was isolated more frequently associated with bloodstream infections than A. baumannii, highlighting the importance of A. pittii in clinical settings (Pailhories et al., 2018). Chen et al. reported the co-occurrence of two carbapenemase genes, blaOXA-58 and blaNDM-1, on a single plasmid in A. pittii (Chen et al., 2019b). Yang et al. (Yang et al., 2012) reported an outbreak of ST63 carbapenem-resistant A. pittii which carried a 45-kb novel blaNDM-1-bearing plasmid in a Chinese ICU. This evidence suggested ST63 A. pittii was readily transmissible and has the capacity to acquire antimicrobial determinants and become competent, which could pose serious threat on public health. In vivo emergence of resistance to last resort antimicrobials including tigecycline and colistin was reported to be quite common in Acinetobacter spp (Karakonstantis, 2020). The fitness cost associated with acquired resistance and the virulence of PDR Acinetobacter sp. remained a major issue for medical treatment (Karakonstantis, 2020).Besides, differentiating A. baumannii complex infection from colonization remains difficult and further complicated particularly in polymicrobial infections such as the case in the current study (Karakonstantis and Kritsotakis, 2021). We acknowledge the limitation that the antimicrobial resistance profiles of strain 17-84 to recently approved antibiotics including eravacycline, cefiderocol and rifabutin, which are potential last resort treatment options, were not tested (Karakonstantis et al., 2020c; Luna et al., 2020). A further study on the treatment of infections caused by such PDR Acinetobacter sp. is underway to provide more insights into the therapeutic scheme.
In conclusion, we reported a case of severe pneumonia due to A. pittii infection in a patient with AECOPD three years after DLT. Phenotypic and genomic characterization indicated the A. pittii strain belonged to ST63, harbored a blaOXA-58-bearing MDR plasmid, carried resistance-associated point mutations and efflux pump genes, and exhibited a PDR phenotype. To our knowledge, this is the first detailed characterization of PDR A. pittii causing severe infections in clinical settings. Attentions should be paid to this emerging pathogen to prevent it from further disseminating in hospital settings and the community.
Data Availability Statement
The datasets presented in this study can be found in online repositories. The names of the repository/repositories and accession number(s) can be found in the article/supplementary material. The complete genome sequence of Acinetobacter pittii strain 17-84 was deposited in GenBank with accession numbers CP059479 (chromosome) and CP059478 (plasmid p17-84_OXA) under BioProject accession PRJNA648287.
Ethics Statement
The studies involving human participants were reviewed and approved by the Ethics Committee of the First Affiliated Hospital of Guangzhou Medical University. The patients/participants provided their written informed consent to participate in this study.
Author Contributions
LYang and ND collected and characterized the strain and participated in manuscript writing. ND, CX, and LYe performed the whole genome sequencing, bioinformatics analysis and the conjugation assay. SC designed and supervised the study, interpreted the data, and wrote the manuscript. All authors contributed to the article and approved the submitted version.
Funding
This study was supported by the Natural Science Foundation of Guangdong Province (2018A030310170) and the Collaborative Research Fund from the Research Grant Council of the Government of Hong Kong SAR (C5026‐16G).
Conflict of Interest
The authors declare that the research was conducted in the absence of any commercial or financial relationships that could be construed as a potential conflict of interest.
Publisher’s Note
All claims expressed in this article are solely those of the authors and do not necessarily represent those of their affiliated organizations, or those of the publisher, the editors and the reviewers. Any product that may be evaluated in this article, or claim that may be made by its manufacturer, is not guaranteed or endorsed by the publisher.
References
Al Atrouni, A., Joly-Guillou, M.-L., Hamze, M., Kempf, M. (2016). Reservoirs of non-Baumannii Acinetobacter Species. Front. Microbiol. 7, 49. doi: 10.3389/fmicb.2016.00049
Ayibieke, A., Kobayashi, A., Suzuki, M., Sato, W., Mahazu, S., Prah, I., et al. (2020). Prevalence and Characterization of Carbapenem-Hydrolyzing Class D β-Lactamase-Producing Acinetobacter Isolates From Ghana. Front. Microbiol. 11. doi: 10.3389/fmicb.2020.587398
Beceiro, A., Moreno, A., Fernández, N., Vallejo, J. A., Aranda, J., Adler, B., et al. (2014). Biological Cost of Different Mechanisms of Colistin Resistance and Their Impact on Virulence in Acinetobacter Baumannii. Antimicrobial Agents chemotherapy 58, 518–526. doi: 10.1128/AAC.01597-13
Bertini, A., Poirel, L., Mugnier, P. D., Villa, L., Nordmann, P., Carattoli, A. (2010). Characterization and PCR-Based Replicon Typing of Resistance Plasmids in Acinetobacter Baumannii. Antimicrobial Agents Chemother. 54, 4168–4177. doi: 10.1128/AAC.00542-10
Bortolaia, V., Kaas, R. S., Ruppe, E., Roberts, M. C., Schwarz, S., Cattoir, V., et al. (2020). ResFinder 4.0 for Predictions of Phenotypes From Genotypes. J. Antimicrobial Chemother. 75, 3491–3500. doi: 10.1093/jac/dkaa345
Brasiliense, D. M., Lima, K. V. B., Pérez-Chaparro, P. J., Mamizuka, E. M., De Oliveira Souza, C., Dutra, L. M. G., et al. (2019). Emergence of Carbapenem-Resistant Acinetobacter Pittii Carrying the blaOXA-72 Gene in the Amazon Region, Brazil. Diagn. Microbiol. Infect. Dis. 93, 82–84. doi: 10.1016/j.diagmicrobio.2018.07.017
Carver, T., Thomson, N., Bleasby, A., Berriman, M., Parkhill, J. (2009). DNAPlotter: Circular and Linear Interactive Genome Visualization. Bioinformatics 25, 119–120. doi: 10.1093/bioinformatics/btn578
Chen, Y., Guo, P., Huang, H., Huang, Y., Wu, Z., Liao, K. (2019b). Detection of Co-Harboring OXA-58 and NDM-1 Carbapenemase Producing Genes Resided on a Same Plasmid From an Acinetobacter Pittii Clinical Isolate in China. Iranian J. Basic Med. Sci. 22, 106. doi: 10.22038/ijbms.2018.28934.6994
Chen, F.-J., Huang, W.-C., Liao, Y.-C., Wang, H.-Y., Lai, J.-F., Kuo, S.-C., et al. (2019a). Molecular Epidemiology of Emerging Carbapenem Resistance in Acinetobacter Nosocomialis and Acinetobacter Pittii in Taiwato 2014. Antimicrobial Agents Chemother. 63, e02007–e02018. doi: 10.1128/AAC.02007-18
Chopjitt, P., Putthanachote, N., Ungcharoen, R., Hatrongjit, R., Boueroy, P., Akeda, Y., et al. (2021). Genomic Characterization of Clinical Extensively Drug-Resistant Acinetobacter Pittii Isolates. Microorganisms 9, 242. doi: 10.3390/microorganisms9020242
Chusri, S., Chongsuvivatwong, V., Rivera, J. I., Silpapojakul, K., Singkhamanan, K., Mcneil, E., et al. (2014). Clinical Outcomes of Hospital-Acquired Infection With Acinetobacter Nosocomialis and Acinetobacter Pittii. Antimicrobial Agents Chemother. 58, 4172–4179. doi: 10.1128/AAC.02992-14
CLSI (2020). Performance Standards for Antimicrobial Susceptibility Testing. Approved Standard. CLSI Document M100 (PA: CLSI Wayne).
Cosgaya, C., Ratia, C., Marí-Almirall, M., Rubio, L., Higgins, P. G., Seifert, H., et al. (2019). In Vitro and In Vivo Virulence Potential of the Emergent Species of the Acinetobacter Baumannii (Ab) Group. Front. Microbiol. 10, 2429. doi: 10.3389/fmicb.2019.02429
Gu, D.-X., Hu, Y.-J., Zhou, H.-W., Zhang, R., Chen, G.-X. (2015). Substitutions of Ser83Leu in GyrA and Ser80Leu in ParC Associated With Quinolone Resistance in Acinetobacter Pittii. Microbial Drug Resistance 21, 345–351. doi: 10.1089/mdr.2014.0057
Howard, A., O’donoghue, M., Feeney, A., Sleator, R. D. (2012). Acinetobacter Baumannii: An Emerging Opportunistic Pathogen. Virulence 3, 243–250. doi: 10.4161/viru.19700
Hujer, K. M., Hujer, A. M., Endimiani, A., Thomson, J. M., Adams, M. D., Goglin, K., et al. (2009). Rapid Determination of Quinolone Resistance in Acinetobacter Spp. J. Clin. Microbiol. 47, 1436–1442. doi: 10.1128/JCM.02380-08
Karakonstantis, S. (2020). A Systematic Review of Implications, Mechanisms, and Stability of In Vivo Emergent Resistance to Colistin and Tigecycline in Acinetobacter Baumannii. J. Chemother. 33, 1–11. doi: 10.1080/1120009X.2020.1794393
Karakonstantis, S., Gikas, A., Astrinaki, E., Kritsotakis, E. I. (2020a). Excess Mortality Due to Pandrug-Resistant Acinetobacter Baumannii Infections in Hospitalized Patients. J. Hosp. Infect. 106, 447–453. doi: 10.1016/j.jhin.2020.09.009
Karakonstantis, S., Kritsotakis, E. I. (2021). Systematic Review and Meta-Analysis of the Proportion and Associated Mortality of Polymicrobial (vs Monomicrobial) Pulmonary and Bloodstream Infections by Acinetobacter Baumannii Complex. Infection 1–13. doi: 10.1007/s15010-021-01663-0
Karakonstantis, S., Kritsotakis, E. I., Gikas, A. (2020b). Pandrug-Resistant Gram-Negative Bacteria: A Systematic Review of Current Epidemiology, Prognosis and Treatment Options. J. Antimicrobial Chemother. 75, 271–282. doi: 10.1093/jac/dkz401
Karakonstantis, S., Kritsotakis, E. I., Gikas, A. (2020c). Treatment Options for K. Pneumoniae, P. Aeruginosa and A. Baumannii Co-Resistant to Carbapenems, Aminoglycosides, Polymyxins and Tigecycline: An Approach Based on the Mechanisms of Resistance to Carbapenems. Infection 48, 835–851. doi: 10.20944/preprints202006.0173.v1
Kiyasu, Y., Hitomi, S., Funayama, Y., Saito, K., Ishikawa (2020). Characteristics of Invasive Acinetobacter Infection: A Multicenter Investigation With Molecular Identification of Causative Organisms. J. Infection Chemother. 26, 475–482. doi: 10.1016/j.jiac.2019.12.010
Livermore, D. M., Mushtaq, S., Warner, M., Woodford, N. (2016). In Vitro Activity of Eravacycline Against Carbapenem-Resistant Enterobacteriaceae and Acinetobacter Baumannii. Antimicrobial Agents Chemother. 60, 3840–3844. doi: 10.1128/AAC.00436-16
Luna, B., Trebosc, V., Lee, B., Bakowski, M., Ulhaq, A., Yan, J., et al. (2020). A Nutrient-Limited Screen Unmasks Rifabutin Hyperactivity for Extensively Drug-Resistant Acinetobacter Baumannii. Nat. Microbiol. 5, 1134–1143. doi: 10.1038/s41564-020-0737-6
Magiorakos, A. P., Srinivasan, A., Carey, R., Carmeli, Y., Falagas, M., Giske, C., et al. (2012). Multidrug-Resistant, Extensively Drug-Resistant and Pandrug-Resistant Bacteria: An International Expert Proposal for Interim Standard Definitions for Acquired Resistance. Clin. Microbiol. Infect. 18, 268–281. doi: 10.1111/j.1469-0691.2011.03570.x
Nguyen, T. A., Pham, S. C., Ly, A. K., Nguyen, C. V. V., Nguyen, T. T., Ha, T. M. (2020). Overexpression of blaOXA-58 Gene Driven by ISAba3 is Associated With Imipenem Resistance in a Clinical Acinetobacter Baumannii Isolate From Vietnam. bioRxiv. 2020, e7213429 doi: 10.1155/2020/7213429
Overbeek, R., Olson, R., Pusch, G. D., Olsen, G. J., Davis, J. J., Disz, T., et al. (2013). The SEED and the Rapid Annotation of Microbial Genomes Using Subsystems Technology (RAST). Nucleic Acids Res. 42, D206–D214. doi: 10.1093/nar/gkt1226
Pagdepanichkit, S., Tribuddharat, C., Chuanchuen, R. (2016). Distribution and Expression of the Ade Multidrug Efflux Systems in Acinetobacter Baumannii Clinical Isolates. Can. J. Microbiol. 62, 794–801. doi: 10.1139/cjm-2015-0730
Pailhories, H., Tiry, C., Eveillard, M., Kempf, M. (2018). Acinetobacter Pittii Isolated More Frequently Than Acinetobacter Baumannii in Blood Cultures: The Experience of a French Hospital. J. Hosp. Infect 99, 360–363. doi: 10.1016/j.jhin.2018.03.019
Seemann, T. (2021) Mlst. Available at: https://github.com/tseemann/mlst (Accessed March 1 2021).
Siguier, P., Pérochon, J., Lestrade, L., Mahillon, J., Chandler, M. (2006). ISfinder: The Reference Centre for Bacterial Insertion Sequences. Nucleic Acids Res. 34, D32–D36. doi: 10.1093/nar/gkj014
Trecarichi, E. M., Quirino, A., Scaglione, V., Longhini, F., Garofalo, E., Bruni, A., et al. (2019). Successful Treatment With Cefiderocol for Compassionate Use in a Critically Ill Patient With XDR Acinetobacter Baumannii and KPC-Producing Klebsiella Pneumoniae: A Case Report. J. Antimicrobial Chemother. 74, 3399–3401. doi: 10.1093/jac/dkz318
Vijayakumar, S., Biswas, I., Veeraraghavan, B. (2019). Accurate Identification of Clinically Important Acinetobacter Spp.: An Update. Future Sci. OA 5, FSO395. doi: 10.2144/fsoa-2018-0127
Wick, R. R., Judd, L. M., Gorrie, C. L., Holt, K. E. (2017). Unicycler: Resolving Bacterial Genome Assemblies From Short and Long Sequencing Reads. PloS Comput. Biol. 13, e1005595. doi: 10.1371/journal.pcbi.1005595
Wong, M. H.-Y., Chan, B., Chan, E. W.-C., Chen, S. (2019). Over-Expression of ISAba1-Linked Intrinsic and Exogenously Acquired OXA Type Carbapenem-Hydrolysing-Class D-ß-Lactamase-Encoding Genes is Key Mechanism Underlying Carbapenem Resistance in Acinetobacter Baumannii. Front. Microbiol. 10, 2809. doi: 10.3389/fmicb.2019.02809
Yang, J., Chen, Y., Jia, X., Luo, Y., Song, Q., Zhao, W., et al. (2012). Dissemination and Characterization of NDM-1-Producing Acinetobacter Pittii in an Intensive Care Unit in China. Clin. Microbiol. Infect. 18, E506–E513. doi: 10.1111/1469-0691.12035
Zarrilli, R., Pournaras, S., Giannouli, M., Tsakris, A. (2013). Global Evolution of Multidrug-Resistant Acinetobacter Baumannii Clonal Lineages. Int. J. Antimicrobial Agents 41, 11–19. doi: 10.1016/j.ijantimicag.2012.09.008
Zhang, Y., Wang, Q., Yin, Y., Chen, H., Jin, L., Gu, B., et al. (2018). Epidemiology of Carbapenem-Resistant Enterobacteriaceae Infections: Report From the China CRE Network. Antimicrob. Agents Chemother. 62, e01882-17. doi: 10.1128/AAC.01882-17
Keywords: pandrug-resistance, Acinetobacter pittii, AECOPD, whole genome sequencing, blaOXA-58, point mutations
Citation: Yang L, Dong N, Xu C, Ye L and Chen S (2021) Emergence of ST63 Pandrug-Resistant Acinetobacter pittii Isolated From an AECOPD Patient in China. Front. Cell. Infect. Microbiol. 11:739211. doi: 10.3389/fcimb.2021.739211
Received: 10 July 2021; Accepted: 28 September 2021;
Published: 14 October 2021.
Edited by:
Milena Dropa, University of São Paulo, BrazilReviewed by:
Stamatis Karakonstantis, University Hospital of Heraklion, GreeceMaria Fernanda Mojica, Case Western Reserve University, United States
Copyright © 2021 Yang, Dong, Xu, Ye and Chen. This is an open-access article distributed under the terms of the Creative Commons Attribution License (CC BY). The use, distribution or reproduction in other forums is permitted, provided the original author(s) and the copyright owner(s) are credited and that the original publication in this journal is cited, in accordance with accepted academic practice. No use, distribution or reproduction is permitted which does not comply with these terms.
*Correspondence: Sheng Chen, c2hlY2hlbkBjaXR5dS5lZHUuaGs=
†These authors have contributed equally to this work