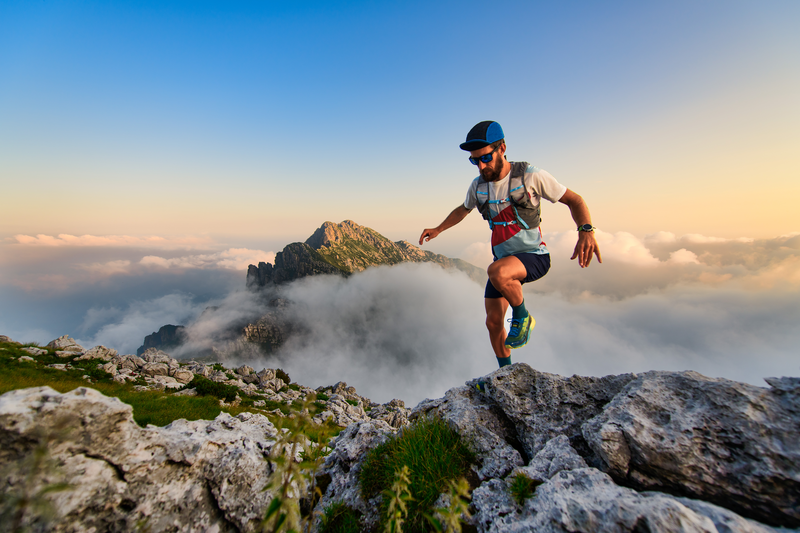
95% of researchers rate our articles as excellent or good
Learn more about the work of our research integrity team to safeguard the quality of each article we publish.
Find out more
MINI REVIEW article
Front. Cell. Infect. Microbiol. , 08 October 2021
Sec. Biofilms
Volume 11 - 2021 | https://doi.org/10.3389/fcimb.2021.736186
Introduction: After insertion into the bone, implants osseointegrate, which is required for their long-term success. However, inflammation and infection around the implants may lead to implant failure leading to peri-implantitis and loss of supporting bone, which may eventually lead to failure of implant. Surface chemistry of the implant and lack of cleanliness on the part of the patient are related to peri-implantitis. The only way to get rid of this infection is decontamination of dental implants.
Objective: This systematic review intended to study decontamination of microbial biofilm methods on titanium implant surfaces used in dentistry.
Methods: The electronic databases Springer Link, Science Direct, and PubMed were explored from their inception until December 2020 to identify relevant studies. Studies included had to evaluate the efficiency of new strategies either to prevent formation of biofilm or to treat matured biofilm on dental implant surfaces.
Results and Discussion: In this systematic review, 17 different groups of decontamination methods were summarized from 116 studies. The decontamination methods included coating materials, mechanical cleaning, laser treatment, photodynamic therapy, air polishing, anodizing treatment, radiation, sonication, thermal treatment, ultrasound treatment, chemical treatment, electrochemical treatment, antimicrobial drugs, argon treatment, and probiotics.
Conclusion: The findings suggest that most of the decontamination methods were effective in preventing the formation of biofilm and in decontaminating established biofilm on dental implants. This narrative review provides a summary of methods for future research in the development of new dental implants and decontamination techniques.
Dental implants are number one choice for replacement of teeth. It is a valuable option in several treatment scenarios, e.g., (i) when teeth are lost to non-periodontal diseases, infection due to caries, traumatic injuries; (ii) loss of teeth due to periodontal diseases but the remaining teeth can be maintained; and (iii) combined scenarios requiring replacement of missing teeth (Greenwell et al., 2019). An implant is typically composed of titanium or titanium alloys because of its biocompatibility and mechanical properties. Recent studies show that titanium dental implants survived for more than 20 years, regardless of their long-term exposure to the oral environment (Chen et al., 2017; Albrektsson et al., 2019). After insertion into the bone, implant osseointegration is expected to occur and is fundamental for its long-term success (Alghamdi, 2018). However, dental implants cannot be used for treatment of periodontal or dental disease (Greenwell et al., 2019). In addition, inflammatory reaction and infection around the implants may occur and can lead to implant failure, a phenomenon called peri-implantitis (Berglundh et al., 2019). Peri-implantitis is caused by plaque bacteria, leading to inflammation of mucosa and subsequent bone loss surrounding the implant, which may ultimately result in total implant failure (Berglundh et al., 2019). Surface chemistry and lack of cleanliness are intimately associated with peri-implantitis (Rupp et al., 2018).
Implant loss often triggers a devastating psychological impact on patients’ lives, with significantly high financial losses to families and healthcare systems (Alzahrani and Gibson, 2018; Frazadmoghadam et al., 2018; Hoeksema et al., 2018). Peri-implantitis is reported to occur at different levels of severity, with about 10% of them being lost within a 5-year period (Koldsland et al., 2010; Caton J. et al., 2018). It has been reported that peri‐implantitis takes place in 3–47% of dental implants (Koldsland et al., 2010; Matarazzo et al., 2018; French et al., 2019). Peri-implantitis is frequently related to periodontal pathogens and usually treated with antibiotics (Ting et al., 2018; Hussain et al., 2018). Bacterial biofilm has been advocated to be the leading etiological factor in the causation of peri-implantitis. Biofilm is a highly structured, matrix-enclosed bacterial community in a sessile state (Magana et al., 2018). Biofilm formation is a typical feature of bacterial growth, causing evasion from host cells and avoiding competition with other microbial communities (Guilhen et al., 2017). Several steps are needed to form biofilms, which include (i) planktonic bacteria adhesion on the surface, (ii) adhesion on the surface to establish a firm platform, (iii) co-aggregation with other bacteria, which further stabilizes their buildup architecture, (iv) growth by absorbing nutrition from the environment until maturation phase, and (v) detachment of a portion of biofilms to invade another vulnerable site (Guilhen et al., 2017). It is more challenging to eradicate established biofilm colonization than to eradicate the circulating contamination itself (i.e., planktonic microbiota). This significantly increases resistance to antibiotic treatment (Arciola et al., 2018). Resident microbiota and matrix in biofilm may hinder access of antimicrobials locally, preventing their penetration into deeper layers of biofilm (Kuang et al., 2018). Therefore, it is deemed imperative that studies in the future focus on the prevention or reduction of biofilm formation.
Several techniques for decontamination of implants have been used over the years. However, none of them have successfully produced optimal results. Mechanisms underpinning these processes are still not understood fully and decontamination of implants remains challenging. A previous systematic review was performed a few years ago (Grischke et al., 2016). Thus, an updated review is necessary. Additionally, other systematic reviews have only evaluated the ability of a selected type of method for decontamination of the implant surface (Louropoulou et al., 2015; Chouirfa et al., 2018; Dutra et al., 2018; Mishra and Chowdhary, 2018). The primary objective of this systematic review was to study and report evidence on all currently investigated methods for decontaminating pathogenic microbiota on dental implant surfaces.
A systematic search was performed in Science Direct, PubMed, and Springer Link from inception up until December 2020. The search was limited to studies published in the English language due to the authors’ inability to translate other published languages in the literature. The following search terms were used: “dental” AND “implant” AND “titanium” AND “bacteria” AND “biofilm” AND (“treatment” OR “decontamination” OR “eradication” OR “cleaning” OR “remove”). Wildcards, e.g., asterisk (*), a question mark ()?, or other symbols that designate the selected database, were applied. Original papers comprising of experimental studies that used titanium materials for the studies were included in the present review. The studies included should have reported the presence of microbial biofilm both by bacteria and fungi. After date was extracted, it was entered into Microsoft Excel in a data extraction form for enabling summarization of data and final report writing. Data analysis was completed using SPSS Software Version 23 and PRISM Software Version 7 (GraphPad Inc.).
The electronic exploration yielded six hundred and forty-three results (n = 643). Duplicates were removed, and on additional screening, one hundred and sixteen studies comprised this review (n =116) (Schwarz et al., 2005; Duarte et al., 2009; Ewald and Ihde, 2009; Größner-Schreiber et al., 2009; Sennhenn-Kirchner et al., 2009; Tamai et al., 2009; Gonçalves et al., 2010; Baffone et al., 2011; Ercan et al., 2011; Fröjd et al., 2011; Giordano et al., 2011; Mohn et al., 2011; Ntrouka et al., 2011; Bürgers et al., 2012; Cortizo et al., 2012; Lilja et al., 2012; Rehman et al., 2012; Subramanian et al., 2012; Trujillo et al., 2012; Alcheikh et al., 2013; Cochis et al., 2013; De Giglio et al., 2013; Diab Al-Radha et al., 2013; Eick et al., 2013; Holmberg et al., 2013; Idlibi et al., 2013; Roberts et al., 2013; Chen et al., 2014; Ciandrini et al., 2014; Drago et al., 2014; Godoy-Gallardo et al., 2014; Hauser-Gerspach et al., 2014; Kaliaraj et al., 2014; Kang et al., 2014; Lv et al., 2014; Massa et al., 2014; Sahrmann et al., 2014; Schmage et al., 2014; Schmidt et al., 2014; Yamada et al., 2014; Abdulkareem et al., 2015; Charalampakis et al., 2015; Cruz et al., 2015; de Avila et al., 2015; Duske et al., 2015; Janković et al., 2015; Jennings et al., 2015; Lewandowska et al., 2015; Narendrakumar et al., 2015; Park et al., 2015; Yucesoy et al., 2015; Wood et al., 2015; Zhang et al., 2015; Ayre et al., 2016; Chen et al., 2016; Ciandrini et al., 2016; Cochis et al., 2016; Cotolan et al., 2016; Cunha et al., 2016; Giannelli et al., 2016; Godoy-Gallardo et al., 2016; Gopal et al., 2016; Guan et al., 2016; John et al., 2016; Kuehl et al., 2016; Kotsakis et al., 2016; Mang et al., 2016; Preissner et al., 2016; Rodríguez-Contreras et al., 2016; Shi et al., 2016; Verardi et al., 2016; Al-Hashedi et al., 2017; Batsukh et al., 2017; Canullo et al., 2017; Ciandrini et al., 2017; Cometa et al., 2017; Dostie et al., 2017; Eick et al., 2017; Ferraris et al., 2017; Giannelli et al., 2017; Granick et al., 2017; Hirschfeld et al., 2017; Kim et al., 2017; Kulkarni Aranya et al., 2017; Li et al., 2017; Macpherson et al., 2017; Matthes et al., 2017; Prieto-Borja et al., 2017; Schmidt et al., 2017; Strever et al., 2017; Ramesh et al., 2017; Wang et al., 2017; Wiedmer et al., 2017; Ye et al., 2017; Zhang et al., 2017; Al-Hashedi et al., 2018; Akhavan et al., 2018; Atefyekta et al., 2018; Azizi et al., 2018; Ferraris et al., 2018; Fukushima et al., 2018; Hidalgo-Robatto et al., 2018; Hoyos-Nogués et al., 2018; Lee et al., 2018; Montelongo-Jauregui et al., 2018; Pantaroto et al., 2018; Pissinis et al., 2018; Santos-Coquillat et al., 2018; Schneider et al., 2018; Souza et al., 2018; Trobos et al., 2018; Vilarrasa et al., 2018; Wang et al., 2018; Zhang et al., 2018; Huang et al., 2019; Schmidt et al., 2019).
A flowchart (Supplementary Information 1) demonstrates the procedure of inclusion and exclusion of pertinent articles. We excluded review papers, non-English language studies, and those not relevant to the focus of this review. Our analysis revealed that the topic of our systematic review has been deeply researched for the previous 10 years, especially in the years 2017–2018. The interest on this topic is mainly because of titanium being an inert material with the properties of encouraging tissue healing and bacterial colonization resistance, as compared with other implant materials (Chen et al., 2017).
Numerous studies have attempted to evaluate different methods to decontaminate dental biofilms. The decontamination methods included in this systematic review were categorized into 19 groups, namely, the coating of titanium materials (47.4%), mechanical cleaning (10.3%), laser treatment (9.5%), photodynamic therapy (4.3%), air polishing (3.4%), anodizing treatment (3.4%), radiation (3.4%), sonication (0.9%), thermal treatment (0.9%), ultrasound (0.9%), chemical treatment (13.8%), electrochemical treatment (1.7%), antimicrobial drugs (0.9%), argon treatment (0.9%), and probiotics (0.9%). Table 1 presented the decontamination methods using specialized instruments and the findings of the respective studies. These decontamination methods were then divided into two types based on whether the biofilm was already established (biofilm-treatment) or not (biofilm-prevention). Supplementary Information 2 shows the coating of the titanium materials, method of decontamination, and type of decontamination method.
Table 1 Decontamination methods using specialized instruments and the findings of the respective studies.
In this systematic review, 63 studies focused on biofilm-prevention methods (n = 63, 54.3%) (Duarte et al., 2009; Ewald and Ihde, 2009; Größner-Schreiber et al., 2009; Sennhenn-Kirchner et al., 2009; Tamai et al., 2009; Baffone et al., 2011; Ercan et al., 2011; Fröjd et al., 2011; Giordano et al., 2011; Cortizo et al., 2012; Rehman et al., 2012; Subramanian et al., 2012; Trujillo et al., 2012; Alcheikh et al., 2013; De Giglio et al., 2013; Holmberg et al., 2013; Roberts et al., 2013; Godoy-Gallardo et al., 2014; Lv et al., 2014; Kaliaraj et al., 2014; Kang et al., 2014; Massa et al., 2014; Yamada et al., 2014; Abdulkareem et al., 2015; de Avila et al., 2015; Janković et al., 2015; Jennings et al., 2015; Lewandowska et al., 2015; Narendrakumar et al., 2015; Wood et al., 2015; Yucesoy et al., 2015; Zhang et al., 2015; Ayre et al., 2016; Cochis et al., 2016; Cotolan et al., 2016; Cunha et al., 2016; Godoy-Gallardo et al., 2016; Gopal et al., 2016; Guan et al., 2016; Kuehl et al., 2016; Rodríguez-Contreras et al., 2016; Shi et al., 2016; Ciandrini et al., 2017; Cometa et al., 2017; Ferraris et al., 2017; Giannelli et al., 2017; Hirschfeld et al., 2017; Kulkarni Aranya et al., 2017; Macpherson et al., 2017; Schmidt et al., 2017; Ye et al., 2017; Akhavan et al., 2018; Atefyekta et al., 2018; Ferraris et al., 2018; Hidalgo-Robatto et al., 2018; Hoyos-Nogués et al., 2018; Pantaroto et al., 2018; Pissinis et al., 2018; Santos-Coquillat et al., 2018; Trobos et al., 2018; Vilarrasa et al., 2018; Wang et al., 2018; Zhang et al., 2018), whereas 51 studies focused on biofilm-treatment methods (n = 51, 44.0%) (Schwarz et al., 2005; Gonçalves et al., 2010; Mohn et al., 2011; Ntrouka et al., 2011; Bürgers et al., 2012; Cochis et al., 2013; Diab Al-Radha et al., 2013; Eick et al., 2013; Idlibi et al., 2013; Chen et al., 2014; Drago et al., 2014; Hauser-Gerspach et al., 2014; Sahrmann et al., 2014; Schmage et al., 2014; Schmidt et al., 2014; Charalampakis et al., 2015; Cruz et al., 2015; Duske et al., 2015; Park et al., 2015; Chen et al., 2016; Ciandrini et al., 2016; Mang et al., 2016; Giannelli et al., 2016; John et al., 2016; Kotsakis et al., 2016; Preissner et al., 2016; Verardi et al., 2016; Al-Hashedi et al., 2017; Batsukh et al., 2017; Canullo et al., 2017; Dostie et al., 2017; Eick et al., 2017; Granick et al., 2017; Kim et al., 2017; Li et al., 2017; Matthes et al., 2017; Prieto-Borja et al., 2017; Ramesh et al., 2017; Strever et al., 2017; Wang et al., 2017; Wiedmer et al., 2017; Zhang et al., 2017; Al-Hashedi et al., 2018; Azizi et al., 2018; Fukushima et al., 2018; Lee et al., 2018; Montelongo-Jauregui et al., 2018; Schneider et al., 2018; Souza et al., 2018; Huang et al., 2019; Schmidt et al., 2019), while two studies offered their novel method to work for both biofilm-prevention and biofilm-treatment measures (n = 2, 1.7%) (Lilja et al., 2012; Ciandrini et al., 2014).
Twenty-two studies applied oral normal flora in human participants who were either healthy or diagnosed with peri-implantitis or periodontitis (n = 22, 19.0%) (Schwarz et al., 2005; Größner-Schreiber et al., 2009; Rehman et al., 2012; Diab Al-Radha et al., 2013; Cochis et al., 2013; Idlibi et al., 2013; Abdulkareem et al., 2015; Charalampakis et al., 2015; de Avila et al., 2015; Duske et al., 2015; Park et al., 2015; John et al., 2016; Kotsakis et al., 2016; Verardi et al., 2016; Al-Hashedi et al., 2017; Dostie et al., 2017; Li et al., 2017; Matthes et al., 2017; Al-Hashedi et al., 2018; Wang et al., 2018; Schmidt et al., 2019). One hundred and five studies performed in-vitro experiments (n = 105, 90.5%) (Duarte et al., 2009; Ewald and Ihde, 2009; Sennhenn-Kirchner et al., 2009; Tamai et al., 2009; Gonçalves et al., 2010; Baffone et al., 2011; Ercan et al., 2011; Fröjd et al., 2011; Mohn et al., 2011; Ntrouka et al., 2011; Bürgers et al., 2012; Cortizo et al., 2012; Lilja et al., 2012; Subramanian et al., 2012; Trujillo et al., 2012; Alcheikh et al., 2013; De Giglio et al., 2013; Diab Al-Radha et al., 2013; Eick et al., 2013; Holmberg et al., 2013; Roberts et al., 2013; Chen et al., 2014; Ciandrini et al., 2014; Drago et al., 2014; Godoy-Gallardo et al., 2014; Hauser-Gerspach et al., 2014; Kaliaraj et al., 2014; Kang et al., 2014; Lv et al., 2014; Massa et al., 2014; Sahrmann et al., 2014; Schmidt et al., 2014; Schmage et al., 2014; Yamada et al., 2014; Abdulkareem et al., 2015; Cruz et al., 2015; de Avila et al., 2015; Duske et al., 2015; Janković et al., 2015; Jennings et al., 2015; Lewandowska et al., 2015; Narendrakumar et al., 2015; Park et al., 2015; Wood et al., 2015; Yucesoy et al., 2015; Zhang et al., 2015; Ayre et al., 2016; Chen et al., 2016; Ciandrini et al., 2016; Cochis et al., 2016; Cotolan et al., 2016; Cunha et al., 2016; Giannelli et al., 2016; Godoy-Gallardo et al., 2016; Gopal et al., 2016; Guan et al., 2016; Kotsakis et al., 2016; Mang et al., 2016; Preissner et al., 2016; Rodríguez-Contreras et al., 2016; Shi et al., 2016; Verardi et al., 2016; Batsukh et al., 2017; Canullo et al., 2017; Ciandrini et al., 2017; Cometa et al., 2017; Dostie et al., 2017; Eick et al., 2017; Ferraris et al., 2017; Giannelli et al., 2017; Granick et al., 2017; Hirschfeld et al., 2017; Kim et al., 2017; Kulkarni Aranya et al., 2017; Li et al., 2017; Macpherson et al., 2017; Matthes et al., 2017; Prieto-Borja et al., 2017; Ramesh et al., 2017; Schmidt et al., 2017; Strever et al., 2017; Wang et al., 2017; Wiedmer et al., 2017; Ye et al., 2017; Zhang et al., 2017; Akhavan et al., 2018; Atefyekta et al., 2018; Azizi et al., 2018; Ferraris et al., 2018; Fukushima et al., 2018; Hidalgo-Robatto et al., 2018; Hoyos-Nogués et al., 2018; Lee et al., 2018; Montelongo-Jauregui et al., 2018; Pantaroto et al., 2018; Pissinis et al., 2018; Santos-Coquillat et al., 2018; Schneider et al., 2018; Souza et al., 2018; Trobos et al., 2018; Vilarrasa et al., 2018; Wang et al., 2018; Zhang et al., 2018; Huang et al., 2019), 11 studies performed in-vivo experiments on humans (n = 11, 9.5%) (Schwarz et al., 2005; Größner-Schreiber et al., 2009; Sennhenn-Kirchner et al., 2009; Baffone et al., 2011; Fröjd et al., 2011; Rehman et al., 2012; Cochis et al., 2013; Idlibi et al., 2013; Charalampakis et al., 2015; Cruz et al., 2015; John et al., 2016; Al-Hashedi et al., 2017; Al-Hashedi et al., 2018; Schmidt et al., 2019), while two studies performed both in-vitro and in-vivo experiments (n = 2, 1.7%) (Giordano et al., 2011; Kuehl et al., 2016). Amongst the in-vivo experiments which recruited human volunteers, 11 studies used titanium discs (n = 11, 9.5%) (Schwarz et al., 2005; Größner-Schreiber et al., 2009; Giordano et al., 2011; Rehman et al., 2012; Cochis et al., 2013; Idlibi et al., 2013; Charalampakis et al., 2015; Kuehl et al., 2016; Al-Hashedi et al., 2017; Al-Hashedi et al., 2018), while the other two studies used titanium implants (n = 2, 1.7%) (John et al., 2016; Schmidt et al., 2019).
Out of 116 studies, some studies did not use discs (n = 13, 11.2%) (Gonçalves et al., 2010; Mohn et al., 2011; Subramanian et al., 2012; Kaliaraj et al., 2014; Lewandowska et al., 2015; Narendrakumar et al., 2015; Park et al., 2015; John et al., 2016; Preissner et al., 2016; Cometa et al., 2017; Schmidt et al., 2017; Azizi et al., 2018; Schmidt et al., 2019). One study performed on discs and other form of titanium (n = 1, 0.9%) (Kulkarni et al., 2017), four studies did not state whether they used discs (n = 4, 3.4%) (Cunha et al., 2016; Macpherson et al., 2017; Ramesh et al., 2017; Akhavan et al., 2018), while the others used discs (n = 98, 84.5%) (Schwarz et al., 2005; Duarte et al., 2009; Ewald and Ihde, 2009; Größner-Schreiber et al., 2009; Sennhenn-Kirchner et al., 2009; Tamai et al., 2009; Baffone et al., 2011; Ercan et al., 2011; Fröjd et al., 2011; Ntrouka et al., 2011; Bürgers et al., 2012; Cortizo et al., 2012; Lilja et al., 2012; Rehman et al., 2012; Trujillo et al., 2012; Alcheikh et al., 2013; Cochis et al., 2013; De Giglio et al., 2013; Diab Al-Radha et al., 2013; Eick et al., 2013; Holmberg et al., 2013; Idlibi et al., 2013; Roberts et al., 2013; Chen et al., 2014; Ciandrini et al., 2014; Drago et al., 2014; Godoy-Gallardo et al., 2014; Hauser-Gerspach et al., 2014; Kang et al., 2014; Lv et al., 2014; Massa et al., 2014; Sahrmann et al., 2014; Schmidt et al., 2014; Schmage et al., 2014; Yamada et al., 2014; Abdulkareem et al., 2015; Charalampakis et al., 2015; Cruz et al., 2015; de Avila et al., 2015; Duske et al., 2015; Janković et al., 2015; Jennings et al., 2015; Wood et al., 2015; Yucesoy et al., 2015; Zhang et al., 2015; Ayre et al., 2016; Chen et al., 2016; Cochis et al., 2016; Ciandrini et al., 2016; Cotolan et al., 2016; Giannelli et al., 2016; Godoy-Gallardo et al., 2016; Gopal et al., 2016; Guan et al., 2016; Kotsakis et al., 2016; Kuehl et al., 2016; Mang et al., 2016; Rodríguez-Contreras et al., 2016; Shi et al., 2016; Verardi et al., 2016; Al-Hashedi et al., 2017; Batsukh et al., 2017; Canullo et al., 2017; Ciandrini et al., 2017; Dostie et al., 2017; Eick et al., 2017; Ferraris et al., 2017; Granick et al., 2017; Giannelli et al., 2017; Hirschfeld et al., 2017; Kim et al., 2017; Kulkarni Aranya et al., 2017; Li et al., 2017; Matthes et al., 2017; Prieto-Borja et al., 2017; Strever et al., 2017; Wang et al., 2017; Wiedmer et al., 2017; Ye et al., 2017; Zhang et al., 2017; Al-Hashedi et al., 2018; Atefyekta et al., 2018; Ferraris et al., 2018; Fukushima et al., 2018; Hidalgo-Robatto et al., 2018; Hoyos-Nogués et al., 2018; Huang et al., 2019; Lee et al., 2018; Montelongo-Jauregui et al., 2018; Pantaroto et al., 2018; Pissinis et al., 2018; Santos-Coquillat et al., 2018; Schneider et al., 2018; Souza et al., 2018; Trobos et al., 2018; Vilarrasa et al., 2018; Wang et al., 2018; Zhang et al., 2018).
Forty-nine different types of microorganisms have been used for the development of dental biofilm in the included studies for this systematic review (n = 49, Table 2). The biofilm matrix was either in single species or mixed species. Seventy-eight studies performed on single-species biofilm (n = 78, 67.2%) (Duarte et al., 2009; Ewald and Ihde, 2009; Sennhenn-Kirchner et al., 2009; Tamai et al., 2009; Gonçalves et al., 2010; Ercan et al., 2011; Giordano et al., 2011; Trujillo et al., 2012; Bürgers et al., 2012; Lilja et al., 2012; Roberts et al., 2013; Holmberg et al., 2013; De Giglio et al., 2013; Alcheikh et al., 2013; Chen et al., 2014; Drago et al., 2014; Godoy-Gallardo et al., 2014; Hauser-Gerspach et al., 2014; Kaliaraj et al., 2014; Kang et al., 2014; Lv et al., 2014; Massa et al., 2014; Schmage et al., 2014; Yamada et al., 2014; Zhang et al., 2015; Jennings et al., 2015; Narendrakumar et al., 2015; Yucesoy et al., 2015; Wood et al., 2015; Janković et al., 2015; Lewandowska et al., 2015; Ayre et al., 2016; Chen et al., 2016; Ciandrini et al., 2016; Cotolan et al., 2016; Cunha et al., 2016; Giannelli et al., 2016; Godoy-Gallardo et al., 2016; Gopal et al., 2016; Guan et al., 2016; Kuehl et al., 2016; Mang et al., 2016; Preissner et al., 2016; Rodríguez-Contreras et al., 2016; Shi et al., 2016; Batsukh et al., 2017; Canullo et al., 2017; Ciandrini et al., 2017; Cometa et al., 2017; Eick et al., 2017; Ferraris et al., 2017; Giannelli et al., 2017; Granick et al., 2017; Hirschfeld et al., 2017; Kim et al., 2017; Kulkarni Aranya et al., 2017; Macpherson et al., 2017; Prieto-Borja et al., 2017; Strever et al., 2017; Zhang et al., 2017; Wang et al., 2017; Wiedmer et al., 2017; Ye et al., 2017; Akhavan et al., 2018; Atefyekta et al., 2018; Azizi et al., 2018; Ferraris et al., 2018; Fukushima et al., 2018; Hidalgo-Robatto et al., 2018; Hoyos-Nogués et al., 2018; Lee et al., 2018; Pissinis et al., 2018; Santos-Coquillat et al., 2018; Souza et al., 2018; Trobos et al., 2018; Zhang et al., 2018; Huang et al., 2019). Ten studies performed on mixed-species biofilm (n = 10, 8.6%) (Fröjd et al., 2011; Cortizo et al., 2012; Subramanian et al., 2012; Sahrmann et al., 2014; Schmidt et al., 2014; Cruz et al., 2015; Cochis et al., 2016; Ramesh et al., 2017; Schneider et al., 2018; Vilarrasa et al., 2018), while eight studies compared single-species and mixed species biofilm (n = 8, 6.9%) (Baffone et al., 2011; Ntrouka et al., 2011; Eick et al., 2013; Ciandrini et al., 2014; Annunziata et al., 2017; Schmidt et al., 2017; Montelongo-Jauregui et al., 2018; Pantaroto et al., 2018). Decontamination methods using antimicrobial drugs, chemical treatment, electrochemical treatment, probiotic, and the findings of the respective studies are presented in Supplementary Information 3.
In experiments that used bacteria or fungi either as single species or mixed species (without the exposure to normal flora), 143 experiments applied biofilm aged less or equal to 24 h (n = 143, 64.7%), 72 experiments applied biofilm aged more than 24 h or equal to 48 h (n = 72, 32.6%), 38 experiments applied biofilm aged more than 48 h or equal to 72 h (n = 38, 17.2%), while 23 experiments applied biofilm aged more than 72 h (n = 23, 10.4%) (Table 2). Table 2 shows the list of oral microbiota used for the development of biofilm on the titanium materials.
The included studies in this systematic review applied several methods after decontamination of the titanium material either to quantify the microbial biofilm or to observe the morphological changes. Fifty-three studies used the number of colony forming unit (CFU) (n = 53, 45.7%) (Duarte et al., 2009; Ewald and Ihde, 2009; Tamai et al., 2009; Gonçalves et al., 2010; Giordano et al., 2011; Baffone et al., 2011; Mohn et al., 2011; Ntrouka et al., 2011; Cochis et al., 2013; Diab Al-Radha et al., 2013; Eick et al., 2013; Holmberg et al., 2013; Roberts et al., 2013; Chen et al., 2014; Ciandrini et al., 2014; Drago et al., 2014; Godoy-Gallardo et al., 2014; Hauser-Gerspach et al., 2014; Massa et al., 2014; Sahrmann et al., 2014; Abdulkareem et al., 2015; Charalampakis et al., 2015; Janković et al., 2015; Narendrakumar et al., 2015; Wood et al., 2015; Chen et al., 2016; Cochis et al., 2016; Giannelli et al., 2016; Guan et al., 2016; Kuehl et al., 2016; Mang et al., 2016; Preissner et al., 2016; Rodríguez-Contreras et al., 2016; Verardi et al., 2016; Ciandrini et al., 2017; Cometa et al., 2017; Dostie et al., 2017; Eick et al., 2017; Ferraris et al., 2017; Giannelli et al., 2017; Granick et al., 2017; Hirschfeld et al., 2017; Kim et al., 2017; Prieto-Borja et al., 2017; Wiedmer et al., 2017; Akhavan et al., 2018; Azizi et al., 2018; Ferraris et al., 2018; Pantaroto et al., 2018; Pissinis et al., 2018; Souza et al., 2018; Trobos et al., 2018; Vilarrasa et al., 2018). Fifty-eight studies used scanning electron microscopy (SEM) (n = 58, 50%) (Duarte et al., 2009; Sennhenn-Kirchner et al., 2009; Gonçalves et al., 2010; Ercan et al., 2011; Giordano et al., 2011; Trujillo et al., 2012; Cochis et al., 2013; Eick et al., 2013; Holmberg et al., 2013; Yamada et al., 2014; Duske et al., 2015; Godoy-Gallardo et al., 2014; Kaliaraj et al., 2014; Abdulkareem et al., 2015; Charalampakis et al., 2015; Cruz et al., 2015; de Avila et al., 2015; Lewandowska et al., 2015; Park et al., 2015; Zhang et al., 2015 ; Preissner et al., 2016; Chen et al., 2016; Cochis et al., 2016; Cotolan et al., 2016; Cunha et al., 2016; Giannelli et al., 2016; John et al., 2016; Kotsakis et al., 2016; Mang et al., 2016; Shi et al., 2016; Al-Hashedi et al., 2017; Batsukh et al., 2017; Canullo et al., 2017; Dostie et al., 2017; Giannelli et al., 2017; Kim et al., 2017; Kulkarni Aranya et al., 2017; Li et al., 2017; Matthes et al., 2017; Ramesh et al., 2017; Schmidt et al., 2017; Strever et al., 2017; Wang et al., 2017; Wiedmer et al., 2017; Zhang et al., 2017; Al-Hashedi et al., 2018; Atefyekta et al., 2018; Azizi et al., 2018; Hidalgo-Robatto et al., 2018; Lee et al., 2018; Montelongo-Jauregui et al., 2018; Pantaroto et al., 2018; Souza et al., 2018; Trobos et al., 2018; Vilarrasa et al., 2018; Wang et al., 2018; Zhang et al., 2018; Huang et al., 2019). Thirty-two studies used confocal laser scanning microscopy (CLSM) (n = 32, 27.6%) (Fröjd et al., 2011; Eick et al., 2013; Drago et al., 2014; Lv et al., 2014; Sahrmann et al., 2014; Schmidt et al., 2014; Abdulkareem et al., 2015; de Avila et al., 2015; Zhang et al., 2015; Guan et al., 2016; Kotsakis et al., 2016; Preissner et al., 2016; Dostie et al., 2017; Giannelli et al., 2017; Kim et al., 2017; Kulkarni Aranya et al., 2017; Li et al., 2017; Matthes et al., 2017; Strever et al., 2017; Wiedmer et al., 2017; Ye et al., 2017; Zhang et al., 2017; Al-Hashedi et al., 2018; Akhavan et al., 2018; Hoyos-Nogués et al., 2018; Montelongo-Jauregui et al., 2018; Souza et al., 2018; Trobos et al., 2018; Wang et al., 2018; Vilarrasa et al., 2018; Zhang et al., 2018). A few of the studies that reported the use of SEM disclosed that their SEM protocol was operated between 1 and 30 kV accelerating voltage (46.7%) (Sennhenn-Kirchner et al., 2009; Ercan et al., 2011; Trujillo et al., 2012; Cochis et al., 2013; ; Eick et al., 2013; Godoy-Gallardo et al., 2014; Cruz et al., 2015; Duske et al., 2015; Lewandowska et al., 2015; Park et al., 2015; Cotolan et al., 2016; Preissner et al., 2016; Al-Hashedi et al., 2017; Batsukh et al., 2017; Dostie et al., 2017; Kulkarni Aranya et al., 2017; Matthes et al., 2017; Ramesh et al., 2017; Al-Hashedi et al., 2018; Atefyekta et al., 2018; Lee et al., 2018; Pantaroto et al., 2018; Trobos et al., 2018; Wang et al., 2018).
In this review, the most commonly employed oral microbiota for the study of dental biofilms were Staphylococcus aureus (15.9%) (Ewald and Ihde, 2009; Tamai et al., 2009; Baffone et al., 2011; Ercan et al., 2011; Giordano et al., 2011; Alcheikh et al., 2013; De Giglio et al., 2013; Drago et al., 2014; Lv et al., 2014; Yamada et al., 2014; Janković et al., 2015; Jennings et al., 2015; Lewandowska et al., 2015; Ayre et al., 2016; Cunha et al., 2016; Giannelli et al., 2016; Gopal et al., 2016; Kuehl et al., 2016; Shi et al., 2016; Cometa et al., 2017; Ferraris et al., 2017; Giannelli et al., 2017; Prieto-Borja et al., 2017; Wang et al., 2017; Ye et al., 2017; Akhavan et al., 2018; Atefyekta et al., 2018; Ferraris et al., 2018; Hidalgo-Robatto et al., 2018; Pissinis et al., 2018; Santos-Coquillat et al., 2018; Zhang et al., 2018), Porphyromonas gingivalis (9.5%) (Gonçalves et al., 2010; Giordano et al., 2011; Holmberg et al., 2013; Eick et al., 2013; Roberts et al., 2013; Ciandrini et al., 2014; Hauser-Gerspach et al., 2014; Kang et al., 2014; Sahrmann et al., 2014; Schmidt et al., 2014; Jennings et al., 2015; Zhang et al., 2015; Chen et al., 2016; Batsukh et al., 2017; Kulkarni Aranya et al., 2017; Schmidt et al., 2017; Strever et al., 2017; Azizi et al., 2018; Lee et al., 2018; Vilarrasa et al., 2018; Huang et al., 2019), Streptococcus sanguinis (7.3%) (Duarte et al., 2009; Fröjd et al., 2011; Bürgers et al., 2012; Godoy-Gallardo et al., 2014; Hauser-Gerspach et al., 2014; Schmidt et al., 2014; Narendrakumar et al., 2015; Chen et al., 2016; Godoy-Gallardo et al., 2016; Guan et al., 2016; Kim et al., 2017; Ramesh et al., 2017; Hoyos-Nogués et al., 2018; Pantaroto et al., 2018; Souza et al., 2018), and Streptococcus mutans (7.3%) (Baffone et al., 2011; Giordano et al., 2011; Ntrouka et al., 2011; Roberts et al., 2013; Schmage et al., 2014; Ciandrini et al., 2014; Cruz et al., 2015; Narendrakumar et al., 2015; Yucesoy et al., 2015; Zhang et al., 2015; Ciandrini et al., 2016; Ye et al., 2017; Ciandrini et al., 2017; Ramesh et al., 2017; Fukushima et al., 2018; Huang et al., 2019).
In addition, 37 of the included studies (n = 37, 31.9%) also performed cell culture in their methodology to study re-osseointegration (Schwarz et al., 2005; Ewald and Ihde, 2009; Giordano et al., 2011; Cortizo et al., 2012; Alcheikh et al., 2013; De Giglio et al., 2013; Eick et al., 2013; Holmberg et al., 2013; Godoy-Gallardo et al., 2014; Hauser-Gerspach et al., 2014; Duske et al., 2015; Janković et al., 2015; Lewandowska et al., 2015; Ayre et al., 2016; Cochis et al., 2016; Cotolan et al., 2016; Giannelli et al., 2016; Guan et al., 2016; Godoy-Gallardo et al., 2016; John et al., 2016; Kotsakis et al., 2016; Shi et al., 2016; Canullo et al., 2017; Cometa et al., 2017; Eick et al., 2017; Ferraris et al., 2017; Giannelli et al., 2017; Matthes et al., 2017; Ramesh et al., 2017; Wang et al., 2017; Ye et al., 2017; Zhang et al., 2017; Atefyekta et al., 2018; Hidalgo-Robatto et al., 2018; Hoyos-Nogués et al., 2018; Lee et al., 2018; Santos-Coquillat et al., 2018). The investigated cell types in this systematic review were osteogenic sarcoma (57.4%), epithelial (2.1%), fibroblast (17.0%), macrophages (4.3%), acute monocytic leukemia (2.1%), mesenchymal stem cells (4.3%), and stromal cells (2.1%).
This review has grouped the decontamination methods into two types: (i) biofilm-prevention method and (ii) biofilm-treatment method.
The studies focused on the modification of titanium implant surfaces. For example, one of the included studies focused on the type of instrument used to modify the surfaces, either to smooth or to roughen them (Schmidt et al., 2017). This biofilm-preventing step occurs before the development of assumed biofilm. In addition to the surface roughness, materials and surface treatment, too, have an impact on the early attachment of bacteria (Batsukh et al., 2017). Therefore, it is essential to assess the effect of various implant surfaces available on the attachment of initial and late colonizing bacteria. In biofilm-treatment method, biofilm development has already taken place. Most of the included studies were for biofilm-treatment.
The most common preventive method of decontamination used in the included studies was the application of a coating on the titanium implant or discs. The mechanism of decontamination did not completely rely on the physical separation of the titanium alloy and oral microbiota.
In a study using calcifying coating solutions (containing different ionic compositions of calcium, phosphate, and zinc), the degree of crystallinity was inversely proportional to antibacterial activity against Porphyromonas gingivalis in coated discs (Kulkarni Aranya et al., 2017). Lower the dissolution rate, lesser the antibacterial effect (Kulkarni Aranya et al., 2017). The addition of titanium dioxide nanoparticles boosted the antimicrobial efficacy of hydrogen peroxide solutions and delayed the redevelopment on surfaces which were previously contaminated by bacteria (Wiedmer et al., 2017).
The second most common biofilm-treatment method of decontamination in this review is by chemical treatment. However, similar to the previous findings, their results showed that not all of the agents used for disinfection in the clinic are effective in the removal of the biofilm. Some of the agents left behind live bacteria on the surface (Dostie et al., 2017). With regards to mechanical cleaning, a complete decontamination of a surface will not be possible as certain bacteria will continue to reside in “valleys and undercuts” (Schmage et al., 2014). In general, removal of greater than 96% biofilm was reported as satisfactory for clinical health, and this was achieved by the oscillating instruments and air polishing (Schmage et al., 2014). One study focused on the application of a direct contact ultrasound at low frequency (Granick et al., 2017). They described that the ultrasound biocidal activity occurred due to acoustic microstreaming and bubble cavitation, releasing considerable energy and damaging bacterial cell walls. In another study, implants were used as cathodes to generate an alkaline environment and reactive oxygen species, and showed a twofold reduction of bacteria compared with the untreated controls (Mohn et al., 2011). Mechanisms are similar to chemical treatments. For example, the ability of hydrogen peroxide to cause bacterial biofilm disruption is associated with oxidation of a number of cellular components and production of gas (Wiedmer et al., 2017).
Staphylococcus aureus, Porphyromonas gingivalis, Streptococcus sanguinis, and Streptococcus mutans are the commonly used oral microbiota for the study of dental biofilms reported in the included studies of this review. These bacteria are all responsible for peri-implantitis (Lavere et al., 2018). They can also exist in the oral saliva of healthy adults and later transform into opportunistic pathogens to become the main cause of the host diseases (Gao et al., 2018). S. aureus is a Gram-positive, aerobic cocci and may cause infections ranging from minor skin infections to pneumonia, bacteremia, and infective endocarditis (Oliveira et al., 2018). P. gingivalis is a Gram-negative anaerobic bacteria and has a strong positive correlation with periodontal diseases (Rafiei et al., 2017). S. sanguinis, previously known as S. sanguis, is a Gram-positive, non-spore-forming, facultative anaerobe and is typically found in a healthy plaque (Zhu et al., [[NoYear]]). S. mutans is a Gram-positive cocci, a facultative anaerobe responsible for the initiation of dental caries, which may lead to the formation of dental plaque and endocarditis (Daboor et al., 2015).
An important question for surface decontamination studies is whether the different patterns of biofilm play a clinical role (Trobos et al., 2018). Biofilm‐associated antimicrobial resistance is related to a number of factors. Established biofilms are resistant to antimicrobial agents as compared with planktonic bacteria which are attributed to biofilm properties, including nature and structure, poor antibiotic penetration, bacterial cells metabolic state, nutrient and oxygen availability, and antimicrobial resistance acquired by gene transfer and mutation (Arciola et al., 2018).
There are many other factors to consider if the decontamination method is successful or not. The first is the age of the biofilm. Half of the included studies in this review evaluated on biofilm aged less or equal to 24 h. However, this may also depend on the type of resident microbiota, maturity of the biofilm, and the number of bacterial cell clusters and micro colonies (Cao et al., 2018). This is a crucial factor especially for those treatment methods aimed at established biofilms, such as in clinically diagnosed peri-implantitis. Additionally, in-vitro developed biofilm is easily affected by surface configuration than biofilm that forms in the mouth (Bevilacqua et al., 2018). Nutrients and mixed bacterial species present in more favorable environment will encourage biofilm formation (Bevilacqua et al., 2018). Quantitative differences detected in-vitro amongst surfaces with dissimilar features might not predict the rate of in-vivo colonization. Various studies analyzed the efficacy of their decontamination methods using a scanning electron microscopy (SEM). However, the energy beam by SEM on its own can be a potential decontamination strategy as the high energy (in kV) can cause bacterial cell wall destruction and internal reversible changes to genetic materials (Ghomi et al., 2005). Therefore, when a high-energy SEM captured the images of bacterial matrix for quantification, their image analysis may introduce inaccuracies.
Re-osseointegration is also an important issue taken into account when decontamination methods are performed. About one-third of the included studies in this review investigated the cell adherence on the titanium implants with biofilm contamination. This phenomenon is described as “the race for the surface,” wherein bacterial and host cells compete in colonizing implant surface (Hoyos-Nogués et al., 2018). Previous systematic review on re-osseointegration has reported that the application of single decontamination measure was not sufficient to get a desirable treatment outcome for peri-implantitis (Madi et al., 2018). Therefore, further studies must be conducted to focus on the dual mechanism (or more) to encourage re-osseointegration after biofilm decontamination. So far, there is no “gold standard” that has been specified in the decontamination of dental implants. A decontamination treatment will need to be combined with mechanical therapy for a successful outcome (Prathapachandran and Suresh, 2012).
In conclusion, this review found that the commonly used decontamination methods of microbial biofilm on dental implant surfaces focused on the addition of surface coating and chemical treatment. Further efforts should be aimed at finding the optimal implant surface property that features antimicrobial treatment that is effective and does not compromise osseointegration.
Conceptualization: JD, JK, RA. Methodology: JD, LM, SD, JK, RA. Software: JD, NR, LM, SD, JK, RA. Validation: JD, NR, JK, RA. Formal analysis: JD, NR, LM, SD, JK, RA. Investigation: JD, NR, LM, SD, JK, RA. Resources: JD, NR, LM, SD, JK, RA. Data curation: JD, NR, LM, SD, JK, RA. Writing—original draft preparation: JD, NR, SD, JK. Writing—review and editing: JD, NR, LM, SD, JK, RA. Supervision: JD, JK. Project administration: JD, NR, LM, SD, JK, RA. Funding acquisition: JD, JK, RA. All authors contributed to the article and approved the submitted version.
The work was supported by the University Research Council grant under Universiti Brunei Darussalam (grant number: UBD/RSCH/URC/RG (b)/2018/004). Allied Grant-Universiti Brunei Darusslam for Publication Cost.
The authors declare that the research was conducted in the absence of any commercial or financial relationships that could be construed as a potential conflict of interest.
All claims expressed in this article are solely those of the authors and do not necessarily represent those of their affiliated organizations, or those of the publisher, the editors and the reviewers. Any product that may be evaluated in this article, or claim that may be made by its manufacturer, is not guaranteed or endorsed by the publisher.
The Supplementary Material for this article can be found online at: https://www.frontiersin.org/articles/10.3389/fcimb.2021.736186/full#supplementary-material
Abdulkareem, E. H., Memarzadeh, K., Allaker, R. P., Huang, J., Pratten, J., Spratt, D. (2015). Anti-Biofilm Activity of Zinc Oxide and Hydroxyapatite Nanoparticles as Dental Implant Coating Materials. J. Dent. 43, 1462–1469. doi: 10.1016/j.jdent.2015.10.010
Akhavan, B., Michl, T. D., Giles, C., Ho, K., Martin, L., Sharifahmadian, O., et al. (2018). Plasma Activated Coatings With Dual Action Against Fungi and Bacteria. Appl. Mater. Today 12, 72–84. doi: 10.1016/j.apmt.2018.04.003
Albrektsson, T., Jemt, T., Mölne, J., Tengvall, P., Wennerberg, A. (2019). On Inflammation-Immunological Balance Theory-A Critical Apprehension of Disease Concepts Around Implants: Mucositis and Marginal Bone Loss may Represent Normal Conditions and Not Necessarily a State of Disease. Clin. Implant Dent. Relat. Res. 21 (1), 183–189. doi: 10.1111/cid.12711
Alcheikh, A., Pavon-Djavid, G., Helary, G., Petite, H., Migonney, V., Anagnostou, F. (2013). PolyNaSS Grafting on Titanium Surfaces Enhances Osteoblast Differentiation and Inhibits Staphylococcus Aureus Adhesion. J. Mater. Sci. Mater. Med. 24, 1745–1754. doi: 10.1007/s10856-013-4932-3
Alghamdi, H. S. (2018). Methods to Improve Osseointegration of Dental Implants in Low Quality (Type-IV) Bone: An Overview. J. Funct. Biomater. 9 (1), 7. doi: 10.3390/jfb9010007
Al-Hashedi, A. A., Laurenti, M., Amine Mezour, M., Basiri, T., Touazine, H., Jahazi, M., et al. (2018). Advanced Inorganic Nanocomposite for Decontaminating Titanium Dental Implants. J. BioMed. Mater. Res. Part B Appl. Biomater. 107 (3), 761–772. doi: 10.1002/jbm.b.34170
Al-Hashedi, A. A., Laurenti, M., Benhamou, V., Tamimi, F. (2017). Decontamination of Titanium Implants Using Physical Methods. Clin. Oral. Implants Res. 28, 1013–1021. doi: 10.1111/clr.12914
Alzahrani, A. A. H., Gibson, B. J. (2018). Scoping Review of the Role of Shared Decision Making in Dental Implant Consultations. JDR Clin. Transl. Res. 3, 130–140. doi: 10.1177/2380084418761340
Annunziata, M., Rizzo, A., Leone, C., Mangano, C., Mazzola, N., Nastri, L., et al. (2017). Bacterial Adhesion to Direct Laser Metal Formed and Mildly Acid Etched Implant Surfaces. Surf. Coat. Technol. 328, 390–397. doi: 10.1016/j.surfcoat.2017.09.011
Arciola, C. R., Campoccia, D., Montanaro, L. (2018). Implant Infections: Adhesion, Biofilm Formation and Immune Evasion. Nat. Rev. Microbiol. 16, 397–409. doi: 10.1038/s41579-018-0019-y
Atefyekta, S., Pihl, M., Lindsay, C., Heilshorn, S. C., Andersson, M. (2018). Antibiofilm Elastin-Like Polypeptide Coatings: Functionality, Stability, and Selectivity. Acta Biomater. 83, 245–256. doi: 10.1016/j.actbio.2018.10.039
Ayre, W. N., Scott, T., Hallam, K., Blom, A. W., Denyer, S., Bone, H. K., et al. (2016). Fluorophosphonate-Functionalised Titanium via a Pre-Adsorbed Alkane Phosphonic Acid: A Novel Dual Action Surface Finish for Bone Regenerative Applications. J. Mater. Sci. Mater. Med. 27, 1–12. doi: 10.1007/s10856-015-5644-7
Azizi, B., Budimir, A., Mehmeti, B., Jakovljević, S., Bago, I., Gjorgievska, E., et al. (2018). Antimicrobial Efficacy of Photodynamic Therapy and Light-Activated Disinfection Against Bacterial Species on Titanium Dental Implants. Int. J. Oral. Maxillofac. Implants 33, 831–837. doi: 10.11607/jomi.6423
Baffone, W., Sorgente, G., Campana, R., Patrone, V., Sisti, D., Falcioni, T. (2011). Comparative Effect of Chlorhexidine and Some Mouthrinses on Bacterial Biofilm Formation on Titanium Surface. Curr. Microbiol. 62, 445–451. doi: 10.1007/s00284-010-9727-x
Batsukh, N., Feng, S. W., Lee, W. F., Leu, S. J., Tsai, P. Y., Ho, K. N., et al. (2017). Effects of Porphyromonas Gingivalis on Titanium Surface by Different Clinical Treatment. J. Med. Biol. Eng. 37, 35–44. doi: 10.1007/s40846-016-0194-0
Berglundh, T., Jepsen, S., Stadlinger, B., Terheyden, H. (2019). Peri-Implantitis and its Prevention. Clin. Oral. Implants Res. 30 (2), 150–155. doi: 10.1111/clr.13401
Bevilacqua, L., Milan, A., Del Lupo, V., Maglione, M., Dolzani, L. (2018). Biofilms Developed on Dental Implant Titanium Surfaces With Different Roughness: Comparison Between In Vitro and In Vivo Studies. Curr. Microbiol. 75, 766–772. doi: 10.1007/s00284-018-1446-8
Bürgers, R., Witecy, C., Hahnel, S., Gosau, M. (2012). The Effect of Various Topical Peri-Implantitis Antiseptics on Staphylococcus Epidermidis, Candida Albicans, and Streptococcus Sanguinis. Arch. Oral. Biol. 57, 940–947. doi: 10.1016/j.archoralbio.2012.01.015
Canullo, L., Genova, T., Wang, H.-L., Carossa, S., Mussano, F. (2017). Plasma of Argon Increases Cell Attachment and Bacterial Decontamination on Different Implant Surfaces. Int. J. Oral. Maxillofac. Implants 32, 1315–1323. doi: 10.11607/jomi.5777
Cao, Y., Su, B., Chinnaraj, S., Jana, S., Bowen, L., Charlton, S., et al. (2018). Nanostructured Titanium Surfaces Exhibit Recalcitrance Towards Staphylococcus Epidermidis Biofilm Formation. Sci. Rep. 8, 1–13. doi: 10.1038/s41598-018-19484-x
Caton J, G., Armitage, G., Berglundh, T., Chapple, I. L. C., Jepsen, S., Kornman, S. K., et al. (2018). A New Classification Scheme for Periodontal and Peri-Implant Diseases and Conditions – Introduction and Key Changes From the 1999 Classification. J. Clin. Periodontol. 45, S1–S8. doi: 10.1111/jcpe.12935
Charalampakis, G., Ramberg, P., Dahlén, G., Berglundh, T., Abrahamsson, I. (2015). Effect of Cleansing of Biofilm Formed on Titanium Discs. Clin. Oral. Implants Res. 26, 931–936. doi: 10.1111/clr.12397
Chen, C. J., Chen, C. C., Ding, S. J. (2016). Effectiveness of Hypochlorous Acid to Reduce the Biofilms on Titanium Alloy Surfaces in Vitro. Int. J. Mol. Sci. 17 (7), 1161. doi: 10.3390/ijms17071161
Chen, X., Hirt, H., Li, Y., Gorr, S. U., Aparicio, C. (2014). Antimicrobial GL13K Peptide Coatings Killed and Ruptured the Wall of Streptococcus Gordonii and Prevented Formation and Growth of Biofilms. PloS One 9 (11), e111579. doi: 10.1371/journal.pone.0111579
Chen, X., Zhou, X. C., Liu, S., Wu, R. F., Aparicio, C., Wu, J. Y. (2017). In Vivo Osseointegration of Dental Implants With an Antimicrobial Peptide Coating. J. Mater. Sci. Mater. Med. 28 (5), 76. doi: 10.1007/s10856-017-5885-8
Chouirfa, H., Bouloussa, H., Migonney, V., Falentin-Daudré, C. (2018). Review of Titanium Surface Modification Techniques and Coatings for Antibacterial Applications. Acta Biomater. 83, 37–54. doi: 10.1016/j.actbio.2018.10.036
Ciandrini, E., Campana, R., Baffone, W. (2017). Live and Heat-Killed Lactobacillus Spp. Interfere With Streptococcus Mutans and Streptococcus Oralis During Biofilm Development on Titanium Surface. Arch. Oral. Biol. 78, 48–57. doi: 10.1016/j.archoralbio.2017.02.004
Ciandrini, E., Campana, R., Casettari, L., Perinelli, D. R., Fagioli, L., Manti, A., et al. (2016). Characterization of Biosurfactants Produced by Lactobacillus Spp. And Their Activity Against Oral Streptococci Biofilm. Appl. Microbiol. Biotechnol. 100, 6767–6777. doi: 10.1007/s00253-016-7531-7
Ciandrini, E., Campana, R., Federici, S., Manti, A., Battistelli, M., Falcieri, E., et al. (2014). In Vitro Activity of Carvacrol Against Titanium-Adherent Oral Biofilms and Planktonic Cultures. Clin. Oral. Investig. 18, 2001–2013. doi: 10.1007/s00784-013-1179-9
Cochis, A., Azzimonti, B., Della Valle, C., De Giglio, E., Bloise, N., Visai, L., et al. (2016). The Effect of Silver or Gallium Doped Titanium Against the Multidrug Resistant Acinetobacter Baumannii. Biomaterials 80, 80–95. doi: 10.1016/j.biomaterials.2015.11.042
Cochis, A., Fini, M., Carrassi, A., Migliario, M., Visai, L., Rimondini, L. (2013). Effect of Air Polishing With Glycine Powder on Titanium Abutment Surfaces. Clin. Oral. Implants Res. 24, 904–909. doi: 10.1111/j.1600-0501.2012.02490.x
Cometa, S., Bonifacio, M. A., Baruzzi, F., de Candia, S., Giangregorio, M. M., Giannossa, L. C., et al. (2017). Silver-Loaded Chitosan Coating as an Integrated Approach to Face Titanium Implant-Associated Infections: Analytical Characterization and Biological Activity. Anal. Bioanal. Chem. 409, 7211–7221. doi: 10.1007/s00216-017-0685-z
Cortizo, M. C., Oberti, T. G., Cortizo, M. S., Cortizo, A. M., Fernández Lorenzo De Mele, M. A. (2012). Chlorhexidine Delivery System From Titanium/Polybenzyl Acrylate Coating: Evaluation of Cytotoxicity and Early Bacterial Adhesion. J. Dent. 40, 329–337. doi: 10.1016/j.jdent.2012.01.008
Cotolan, N., Rak, M., Bele, M., Cör, A., Muresan, L. M., Milošev, I. (2016). Sol-Gel Synthesis, Characterization and Properties of TiO2and Ag-TiO2coatings on Titanium Substrate. Surf. Coat. Technol. 307, 790–799. doi: 10.1016/j.surfcoat.2016.09.082
Cruz, H. V., Henriques, M., Teughels, W., Celis, J. P., Rocha, L. A. (2015). Combined Influence of Fluoride and Biofilms on the Biotribocorrosion Behavior of Titanium Used for Dental Applications. J. Bio- Tribo-Corros. 1, 21. doi: 10.1007/s40735-015-0021-0
Cunha, A., Elie, A. M., Plawinski, L., Serro, A. P., Botelho Do Rego, A. M., Almeida, A., et al. (2016). Femtosecond Laser Surface Texturing of Titanium as a Method to Reduce the Adhesion of Staphylococcus Aureus and Biofilm Formation. Appl. Surf. Sci. 360, 485–493. doi: 10.1016/j.apsusc.2015.10.102
Daboor, S. M., Syed, F., Masood, S., Al-Azab, M. S., Nori, E. (2015). A Review on Streptococcus Mutans With Its Diseases Dental Caries, Dental Plaque and Endocarditis. Indian J. Microbiol. Res. 2 (2), 76–82.
de Avila, E. D., Lima, B. P., Sekiya, T., Torii, Y., Ogawa, T., Shi, W., et al. (2015). Effect of UV-Photofunctionalization on Oral Bacterial Attachment and Biofilm Formation to Titanium Implant Material. Biomaterials 67, 84–92. doi: 10.1016/j.biomaterials.2015.07.030
De Giglio, E., Cafagna, D., Cometa, S., Allegretta, A., Pedico, A., Giannossa, L. C., et al. (2013). An Innovative, Easily Fabricated, Silver Nanoparticle-Based Titanium Implant Coating: Development and Analytical Characterization. Anal. Bioanal. Chem. 405, 805–816. doi: 10.1007/s00216-012-6293-z
Diab Al-Radha, A. S., Younes, C., Diab, B. S., Jenkinson, H. F. (2013). Essential Oils and Zirconia Dental Implant Materials. Int. J. Oral. Maxillofac. Implants 28, 1497–1505. doi: 10.11607/jomi.3142
Dorkhan, M., Yücel-Lindberg, T., Hall, J., Svensäter, G., Davies, J. R. (2014). Adherence of Human Oral Keratinocytes and Gingival Fibroblasts to Nano-Structured Titanium Surfaces. BMC Oral. Health 14, 75. doi: 10.1186/1472-6831-14-75
Dostie, S., Alkadi, L. T., Owen, G., Bi, J., Shen, Y., Haapasalo, M., et al. (2017). Chemotherapeutic Decontamination of Dental Implants Colonized by Mature Multispecies Oral Biofilm. J. Clin. Periodontol. 44, 403–409. doi: 10.1111/jcpe.12699
Drago, L., Del Fabbro, M., Bortolin, M., Vassena, C., De Vecchi, E. (2014). Biofilm Removal and Antimicrobial Activity of Two Different Air-Polishing Powders: An In Vitro Study. J. Periodontol. 85, e363–e369. doi: 10.1902/jop.2014.140134
Duarte, P. M., Reis, A. F., de Freitas, P. M., Ota-Tsuzuki, C. (2009). Bacterial Adhesion on Smooth and Rough Titanium Surfaces After Treatment With Different Instruments. J. Periodontol. 80, 1824–1832. doi: 10.1902/jop.2009.090273
Duske, K., Jablonowski, L., Koban, I., Matthes, R., Holtfreter, B., Sckell, A., et al. (2015). Cold Atmospheric Plasma in Combination With Mechanical Treatment Improves Osteoblast Growth on Biofilm Covered Titanium Discs. Biomaterials 52, 327–334. doi: 10.1016/j.biomaterials.2015.02.035
Dutra, D., Pereira, G., Kantorski, K., Valandro, L., Zanatta, F. (2018). Does Finishing and Polishing of Restorative Materials Affect Bacterial Adhesion and Biofilm Formation? A Systematic Review. Oper. Dent. 43, E37–E52. doi: 10.2341/17-073-L
Eick, S., Markauskaite, G., Nietzsche, S., Laugisch, O., Salvi, G. E., Sculean, A. (2013). Effect of Photoactivated Disinfection With a Light-Emitting Diode on Bacterial Species and Biofilms Associated With Periodontitis and Peri-Implantitis. Photodiagnosis Photodyn. Ther. 10, 156–167. doi: 10.1016/j.pdpdt.2012.12.001
Eick, S., Meier, I., Spoerlé, F., Bender, P., Aoki, A., Izumi, Y., et al. (2017). In Vitro-Activity of Er : YAG Laser in Comparison With Other Treatment Modalities on Biofilm Ablation From Implant and Tooth Surfaces. PloS One 12, 1–15. doi: 10.1371/journal.pone.0171086
Ercan, B., Kummer, K. M., Tarquinio, K. M., Webster, T. J. (2011). Decreased Staphylococcus Aureus Biofilm Growth on Anodized Nanotubular Titanium and the Effect of Electrical Stimulation. Acta Biomater. 7, 3003–3012. doi: 10.1016/j.actbio.2011.04.002
Ewald, A., Ihde, S. (2009). Salt Impregnation of Implant Materials. Oral. Surg. Oral. Med. Oral. Pathol. Oral. Radiol. Endod. 107, 790–795. doi: 10.1016/j.tripleo.2008.12.028
Ferraris, S., Spriano, S., Miola, M., Bertone, E., Allizond, V., Cuffini, A. M., et al. (2018). Surface Modification of Titanium Surfaces Through a Modified Oxide Layer and Embedded Silver Nanoparticles: Effect of Reducing/Stabilizing Agents on Precipitation and Properties of the Nanoparticles. Surf. Coat. Technol. 344, 177–189. doi: 10.1016/j.surfcoat.2018.03.020
Ferraris, S., Truffa Giachet, F., Miola, M., Bertone, E., Varesano, A., Vineis, C., et al. (2017). Nanogrooves and Keratin Nanofibers on Titanium Surfaces Aimed at Driving Gingival Fibroblasts Alignment and Proliferation Without Increasing Bacterial Adhesion. Mater. Sci. Eng. C 76, 1–12. doi: 10.1016/j.msec.2017.02.152
Frazadmoghadam, M., Mohammadi, T. M., Mohammadi, M., Goudarzi, R. (2018). Oral Health Related Quality of Life in Patients Undertaking Implant Treatments: A Review of Literature. World Fam. Med. 16, 346–351. doi: 10.5742/MEWFM.2018.93283
French, D., Grandin, H. M., Ofec, R. (2019). Retrospective Cohort Study of 4,591 Dental Implants: Analysis of Risk Indicators for Bone Loss and Prevalence of Peri-Implant Mucositis and Peri-Implantitis. J. Periodontol. 90 (7), 691–700. doi: 10.1002/JPER.18-0236
Fröjd, V., Linderbäck, P., Wennerberg, A., Chávez de Paz, L., Svensäter, G., Davies, J. R. (2011). Effect of Nanoporous TiO2 Coating and Anodized Ca2+ Modification of Titanium Surfaces on Early Microbial Biofilm Formation. BMC Oral. Health 11, 8. doi: 10.1186/1472-6831-11-8
Fukushima, A., Mayanagi, G., Sasaki, K., Takahashi, N. (2018). Corrosive Effects of Fluoride on Titanium Under Artificial Biofilm. J. Prosthodont. Res. 62, 104–109. doi: 10.1016/j.jpor.2017.08.004
Gao, X., Zhou, J., Sun, X., Li, X., Zhou, Y. (2018). Diversity Analysis of Subgingival Microbial Bacteria in Peri-Implantitis in Uygur Population. Medicine (United States) 97 (5), e9774. doi: 10.1097/MD.0000000000009774
Ghomi, H., Rahman, M. S., Chalise, P. R., Hayashi, Y., Watanabe, M., Okino, A., et al. (2005). Experimental Investigation of Effect of Low-Energy Pulsed Atmospheric Electron Beam on Bacterial Cells. Jpn. J. Appl. Phys. Part 1 Regul. Pap. Short Notes Rev. Pap. 44, 8698–8701. doi: 10.1143/JJAP.44.8698
Giannelli, M., Landini, G., Materassi, F., Chellini, F., Antonelli, A., Tani, A., et al. (2016). The Effects of Diode Laser on Staphylococcus Aureus Biofilm and Escherichia Coli Lipopolysaccharide Adherent to Titanium Oxide Surface of Dental Implants. An in vitro study. Lasers Med. Sci. 31, 1613–1619. doi: 10.1007/s10103-016-2025-5
Giannelli, M., Landini, G., Materassi, F., Chellini, F., Antonelli, A., Tani, A., et al. (2017). Effects of Photodynamic Laser and Violet-Blue Led Irradiation on Staphylococcus Aureus Biofilm and Escherichia Coli Lipopolysaccharide Attached to Moderately Rough Titanium Surface: In Vitro Study. Lasers Med. Sci. 32, 857–864. doi: 10.1007/s10103-017-2185-y
Giordano, C., Saino, E., Rimondini, L., Pedeferri, M. P., Visai, L., Cigada, A., et al. (2011). Electrochemically Induced Anatase Inhibits Bacterial Colonization on Titanium Grade 2 and Ti6Al4V Alloy for Dental and Orthopedic Devices. Colloids Surf. B Biointerfaces 88, 648–655. doi: 10.1016/j.colsurfb.2011.07.054
Godoy-Gallardo, M., Guillem-Marti, J., Sevilla, P., Manero, J. M., Gil, F. J., Rodriguez, D., et al. (2016). Anhydride-Functional Silane Immobilized Onto Titanium Surfaces Induces Osteoblast Cell Differentiation and Reduces Bacterial Adhesion and Biofilm Formation. Mater. Sci. Eng. C 59, 524–532. doi: 10.1016/j.msec.2015.10.051
Godoy-Gallardo, M., Mas-Moruno, C., Fernández-Calderón, M. C., Pérez-Giraldo, C., Manero, J. M., Albericio, F., et al. (2014). Covalent Immobilization of Hlf1-11 Peptide on a Titanium Surface Reduces Bacterial Adhesion and Biofilm Formation. Acta Biomater. 10, 3522–3534. doi: 10.1016/j.actbio.2014.03.026
Gonçalves, F., Zanetti, A. L., Zanetti, R. V., Martelli, F. S., Avila-Campos, M. J., Tomazinho, L. F., et al. (2010). Effectiveness of 980-Mm Diode and 1064-Nm Extra-Long-Pulse Neodymium-Doped Yttrium Aluminum Garnet Lasers in Implant Disinfection. Photomed. Laser Surg. 28, 273–280. doi: 10.1089/pho.2009.2496
Gopal, J., Chun, S., Doble, M. (2016). Attenuated Total Reflection Fourier Transform Infrared Spectroscopy Towards Disclosing Mechanism of Bacterial Adhesion on Thermally Stabilized Titanium Nano-Interfaces. J. Mater. Sci. Mater. Med. 27 (8), 135. doi: 10.1007/s10856-016-5739-9
Granick, M. S., Paribathan, C., Shanmugam, M., Ramasubbu, N. (2017). Direct-Contact Low-Frequency Ultrasound Clearance of Biofilm From Metallic Implant Materials. Eplasty 17, e13.
Greenwell, H., Wang, H. L., Kornman, K. S., Tonetti, M. S. (2019). Biologically Guided Implant Therapy: A Diagnostic and Therapeutic Strategy of Conservation and Preservation Based on Periodontal Staging and Grading. J. Periodontol. 90 (5), 441–444. doi: 10.1002/JPER.18-0495
Grischke, J., Eberhard, J., Stiesch, M. (2016). Antimicrobial Dental Implant Functionalization Strategies -A Systematic Review. Dent. Mater. J. 35, 545–558. doi: 10.4012/dmj.2015-314
Größner-Schreiber, B., Teichmann, J., Hannig, M., Dörfer, C., Wenderoth, D. F., Ott, S. J. (2009). Modified Implant Surfaces Show Different Biofilm Compositions Under In Vivo Conditions. Clin. Oral. Implants Res. 20, 817–826. doi: 10.1111/j.1600-0501.2009.01729.x
Guan, B., Wang, H., Xu, R., Zheng, G., Yang, J., Liu, Z., et al. (2016). Establishing Antibacterial Multilayer Films on the Surface of Direct Metal Laser Sintered Titanium Primed With Phase-Transited Lysozyme. Sci. Rep. 6, 1–12. doi: 10.1038/srep36408
Guilhen, C., Forestier, C., Balestrino, D. (2017). Biofilm Dispersal: Multiple Elaborate Strategies for Dissemination of Bacteria With Unique Properties. Mol. Microbiol. 105, 188–210. doi: 10.1111/mmi.13698
Hauser-Gerspach, I., Mauth, C., Waltimo, T., Meyer, J., Stübinger, S. (2014). Effects of Er : YAG Laser on Bacteria Associated With Titanium Surfaces and Cellular Response In Vitro. Lasers Med. Sci. 29, 1329–1337. doi: 10.1007/s10103-013-1303-8
Hidalgo-Robatto, B. M., Aguilera-Correa, J. J., López-Álvarez, M., Romera, D., Esteban, J., González, P., et al. (2018). Fluor−Carbonated Hydroxyapatite Coatings by Pulsed Laser Deposition to Promote Cell Viability and Antibacterial Properties. Surf. Coat. Technol. 349, 736–744. doi: 10.1016/j.surfcoat.2018.06.047
Hirschfeld, J., Akinoglu, E. M., Wirtz, D. C., Hoerauf, A., Bekeredjian-Ding, I., Jepsen, S., et al. (2017). Long-Term Release of Antibiotics by Carbon Nanotube-Coated Titanium Alloy Surfaces Diminish Biofilm Formation by Staphylococcus Epidermidis. Nanomedicine 13, 1587–1593. doi: 10.1016/j.nano.2017.01.002
Hoeksema, A. R., Peters, L. L., Raghoebar, G. M., Meijer, H. J. A., Vissink, A., Visser, A. (2018). Health and Quality of Life Differ Between Community Living Older People With and Without Remaining Teeth Who Recently Received Formal Home Care: A Cross Sectional Study. Clin. Oral. Investig. 22, 2615–2622. doi: 10.1007/s00784-018-2360-y
Holmberg, K. V., Abdolhosseini, M., Li, Y., Chen, X., Gorr, S. U., Aparicio, C. (2013). Bio-Inspired Stable Antimicrobial Peptide Coatings for Dental Applications. Acta Biomater. 9, 8224–8231. doi: 10.1016/j.actbio.2013.06.017
Hoyos-Nogués, M., Buxadera-Palomero, J., Ginebra, M. P., Manero, J. M., Gil, F. J., Mas-Moruno, C. (2018). All-In-One Trifunctional Strategy: A Cell Adhesive, Bacteriostatic and Bactericidal Coating for Titanium Implants. Colloids Surf. B Biointerfaces 169, 30–40. doi: 10.1016/j.colsurfb.2018.04.050
Huang, T. C., Chen, C. J., Ding, S. J., Chen, C. C. (2019). Antimicrobial Efficacy of Methylene Blue-Mediated Photodynamic Therapy on Titanium Alloy Surfaces In Vitro. Photodiagnosis Photodyn. Ther. 25, 7–16. doi: 10.1016/j.pdpdt.2018.11.008
Hussain, M. W., Abullais, S. S., Naqash, T. A., Bhat, M. Y. S. (2018). Microbial Etiology and Antimicrobial Therapy of Peri-Implantitis: A Comprehensive Review. Open Dent. J. 12, 1113–1122. doi: 10.2174/1874210601812011113
Idlibi, A. N., Al-Marrawi, F., Hannig, M., Lehmann, A., Rueppell, A., Schindler, A., et al. (2013). Destruction of Oral Biofilms Formed in Situ on Machined Titanium (Ti) Surfaces by Cold Atmospheric Plasma. Biofouling 29, 369–379. doi: 10.1080/08927014.2013.775255
Janković, A., Eraković, S., Ristoscu, C., Mihailescu (Serban), N., Duta, L., Visan, A., et al. (2015). Structural and Biological Evaluation of Lignin Addition to Simple and Silver-Doped Hydroxyapatite Thin Films Synthesized by Matrix-Assisted Pulsed Laser Evaporation. J. Mater. Sci. Mater. Med. 26 (1), 5333. doi: 10.1007/s10856-014-5333-y
Jennings, J. A., Velasquez Pulgarin, D. A., Kunwar, D. L., Babu, J., Mishra, S. (2015). Bacterial Inhibition by Chitosan Coatings Loaded With Silver-Decorated Calcium Phosphate Microspheres. Thin Solid Films 596, 83–86. doi: 10.1016/j.tsf.2015.08.060
John, G., Becker, J., Schwarz, F. (2016). Effectivity of Air-Abrasive Powder Based on Glycine and Tricalcium Phosphate in Removal of Initial Biofilm on Titanium and Zirconium Oxide Surfaces in an Ex Vivo Model. Clin. Oral. Investig. 20, 711–719. doi: 10.1007/s00784-015-1571-8
Kaliaraj, G. S., Ramadoss, A., Sundaram, M., Balasubramanian, S., Muthirulandi, J. (2014). Studies of Calcium-Precipitating Oral Bacterial Adhesion on TiN, TiO2single Layer, and TiN/TiO2multilayer-Coated 316L SS. J. Mater. Sci. 49, 7172–7180. doi: 10.1007/s10853-014-8425-7
Kang, S. N., Jeong, C. M., Jeon, Y. C., Byon, E. S., Jeong, Y. S., Cho, L. R. (2014). Effects of Mg-Ion and Ca-Ion Implantations on P. Gingivalis and F. Nucleatum Adhesion. Tissue Eng. Regener. Med. 11, 39–46. doi: 10.1007/s13770-013-1104-y
Kim, S., Park, S. H., Chang, B., Lee, S. Y., Lee, J., Um, H., et al. (2017). Antimicrobial Effect of Photodynamic Therapy Using Methylene Blue and Red Color Diode Laser on Biofilm Attached to Sandblasted and Acid-Etched Surface of Titanium. Laser Dent. Sci. 1, 83–90. doi: 10.1007/s41547-017-0009-9
Koldsland, O. C., Scheie, A. A., Aass, A. M. (2010). Prevalence of Peri-Implantitis Related to Severity of the Disease With Different Degrees of Bone Loss. J. Periodontol. 81, 231–238. doi: 10.1902/jop.2009.090269
Kotsakis, G. A., Lan, C., Barbosa, J., Lill, K., Chen, R., Rudney, J., et al. (2016). Antimicrobial Agents Used in the Treatment of Peri-Implantitis Alter the Physicochemistry and Cytocompatibility of Titanium Surfaces. J. Periodontol. 87, 809–819. doi: 10.1902/jop.2016.150684
Kuang, X., Chen, V., Xu, X. (2018). Novel Approaches to the Control of Oral Microbial Biofilms. BioMed. Res. Int. 2018, 6498932. doi: 10.1155/2018/6498932
Kuehl, R., Brunetto, P. S., Woischnig, A.-K., Varisco, M., Rajacic, Z., Vosbeck, J., et al. (2016). Preventing Implant-Associated Infections by Silver Coating. Antimicrob. Agents Chemother. 60, 2467–2475. doi: 10.1128/AAC.02934-15
Kulkarni Aranya, A., Pushalkar, S., Zhao, M., LeGeros, R. Z., Zhang, Y., Saxena, D. (2017). Antibacterial and Bioactive Coatings on Titanium Implant Surfaces. J. BioMed. Mater. Res. Part A 105, 2218–2227. doi: 10.1002/jbm.a.36081
Kulkarni, M., Junkar, I., Humpolíček, P., Capáková, Z., Radaszkiewicz, K. A., Mikušová, N., et al. (2017). Interaction of Nanostructured TiO2 Biointerfaces With Stem Cells and Biofilm-Forming Bacteria. Mater. Sci. Eng. C 77, 500–507. doi: 10.1016/j.msec.2017.03.174
Lavere, E., Valente, N. A., Andreana, S. (2018). What Grows on Your Implants? N. Y. State Dent. J. 84, 28–32.
Lee, B. S., Shih, K. S., Lai, C. H., Takeuchi, Y., Chen, Y. W. (2018). Surface Property Alterations and Osteoblast Attachment to Contaminated Titanium Surfaces After Different Surface Treatments: An In Vitro Study. Clin. Implant Dent. Relat. Res. 20, 583–591. doi: 10.1111/cid.12624
Lewandowska, Ż., Piszczek, P., Radtke, A., Jędrzejewski, T., Kozak, W., Sadowska, B. (2015). The Evaluation of the Impact of Titania Nanotube Covers Morphology and Crystal Phase on Their Biological Properties. J. Mater. Sci. Mater. Med. 26, 1–12. doi: 10.1007/s10856-015-5495-2
Li, B., Ge, Y., Wu, Y., Chen, J., Xu, H. H. K., Yang, M., et al. (2017). Anti-Bacterial and Microecosystem-Regulating Effects of Dental Implant Coated With Dimethylaminododecyl Methacrylate. Molecules 22, 1–11. doi: 10.3390/molecules22112013
Lilja, M., Forsgren, J., Welch, K. (2012). Photocatalytic and Antimicrobial Properties of Surgical Implant Coatings of Titanium Dioxide Deposited Though Cathodic Arc Evaporation. Biotechnol. Lett. 34, 2299–2305. doi: 10.1007/s10529-012-1040-2
Louropoulou, A., Slot, D. E., van der Weijden, F. (2015). Influence of Mechanical Instruments on the Biocompatibility of Titanium Dental Implants Surfaces: A Systematic Review. Clin. Oral. Implants Res. 26, 841–850. doi: 10.1111/clr.12365
Lv, H., Chen, Z., Yang, X., Cen, L., Zhang, X., Gao, P. (2014). Layer-By-Layer Self-Assembly of Minocycline-Loaded Chitosan/Alginate Multilayer on Titanium Substrates to Inhibit Biofilm Formation. J. Dent. 42, 1464–1472. doi: 10.1016/j.jdent.2014.06.003
Macpherson, A., Li, X., McCormick, P., Ren, L., Yang, K., Sercombe, T. B. (2017). Antibacterial Titanium Produced Using Selective Laser Melting. Jom 69, 2719–2724. doi: 10.1007/s11837-017-2589-y
Madi, M., Htet, M., Zakaria, O., Alagl, A., Kasugai, S. (2018). Re-Osseointegration of Dental Implants After Periimplantitis Treatments: A Systematic Review. Implant Dent. 27, 101–110. doi: 10.1097/ID.0000000000000712
Magana, M., Sereti, C., Ioannidis, A., Mitchell, C. A., Ball, A. R., Magiorkinis, E., et al. (2018). Options and Limitations in Clinical Investigation of Bacterial Biofilms. Clin. Microbiol. Rev. 31, 1–49. doi: 10.1128/CMR.00084-16
Mang, T., Rogers, S., Keinan, D., Honma, K., Baier, R. (2016). Antimicrobial Photodynamic Therapy (aPDT) Induction of Biofilm Matrix Architectural and Bioadhesive Modifications. Photodiagnosis Photodyn. Ther. 13, 22–28. doi: 10.1016/j.pdpdt.2015.11.007
Massa, M. A., Covarrubias, C., Bittner, M., Fuentevilla, I. A., Capetillo, P., Von Marttens, A., et al. (2014). Synthesis of New Antibacterial Composite Coating for Titanium Based on Highly Ordered Nanoporous Silica and Silver Nanoparticles. Mater. Sci. Eng. C 45, 146–153. doi: 10.1016/j.msec.2014.08.057
Matarazzo, F., Sabóia-Gomes, R., Alves, B. E. S., de Oliveira, R. P., Araújo, M. G. (2018). Prevalence, Extent and Severity of Peri-Implant Diseases. A Cross-Sectional Study Based on a University Setting in Brazil. J. Periodontal Res. 53, 910–915. doi: 10.1111/jre.12582
Matthes, R., Duske, K., Kebede, T. G., Pink, C., Schlüter, R., von Woedtke, T., et al. (2017). Osteoblast Growth, After Cleaning of Biofilm-Covered Titanium Discs With Air-Polishing and Cold Plasma. J. Clin. Periodontol. 44, 672–680. doi: 10.1111/jcpe.12720
Mishra, S., Chowdhary, R. (2018). PEEK Materials as an Alternative to Titanium in Dental Implants: A Systematic Review. Clin. Implant Dent. Relat. Res. 21 (1), 208–222. doi: 10.1111/cid.12706
Mohn, D., Zehnder, M., Stark, W. J., Imfeld, T. (2011). Electrochemical Disinfection of Dental Implants – a Proof of Concept. PloS One 6 (1), e16157. doi: 10.1371/journal.pone.0016157
Montelongo-Jauregui, D., Srinivasan, A., Ramasubramanian, A., Lopez-Ribot, J. (2018). An In Vitro Model for Candida Albicans–Streptococcus Gordonii Biofilms on Titanium Surfaces. J. Fungi 4, 66. doi: 10.3390/jof4020066
Narendrakumar, K., Kulkarni, M., Addison, O. (2015). Adherence of Oral Streptococci to Nanostructured. Dent. Mat. 1, 1460–1468. doi: 10.1016/j.dental.2015.09.011
Ntrouka, V., Hoogenkamp, M., Zaura, E., van der Weijden, F. (2011). The Effect of Chemotherapeutic Agents on Titanium-Adherent Biofilms. Clin. Oral. Implants Res. 22, 1227–1234. doi: cid.12706/j.1600-0501.2010.02085.x
Oliveira, D., Borges, A., Simões, M. (2018). Staphylococcus Aureus Toxins and Their Molecular Activity in Infectious Diseases. Toxins (Basel) 10 (6), 252. doi: 10.3390/toxins10060252
Pantaroto, H. N., Ricomini-Filho, A. P., Bertolini, M. M., Dias da Silva, J. H., Azevedo Neto, N. F., Sukotjo, C., et al. (2018). Antibacterial Photocatalytic Activity of Different Crystalline TiO2phases in Oral Multispecies Biofilm. Dent. Mater. 34, e182–e195. doi: 10.1016/j.dental.2018.03.011
Park, J.-B., Lee, S.-H., Kim, N., Park, S., Jin, S.-H., Choi, B.-K., et al. (2015). Instrumentation With Ultrasonic Scalers Facilitates Cleaning of the Sandblasted and Acid-Etched Titanium Implants. J. Oral. Implantol. 41, 419–428. doi: 10.1563/AAID-JOI-D-13-00078
Pissinis, D. E., Benítez, G. A., Schilardi, P. L. (2018). Two-Step Biocompatible Surface Functionalization for Two-Pathway Antimicrobial Action Against Gram-Positive Bacteria. Colloids Surf. B Biointerfaces 164, 262–271. doi: 10.1016/j.colsurfb.2018.01.057
Prathapachandran, J., Suresh, N. (2012). Management of Peri-Implantitis. Dent. Res. J. (Isfahan) 5, 516–521. doi: 10.4103/1735-3327.104867
Preissner, S., Wirtz, H. C., Tietz, A. K., Abu-Sirhan, S., Herbst, S. R., Hartwig, S., et al. (2016). Bactericidal Efficacy of Tissue Tolerable Plasma on Microrough Titanium Dental Implants: An in-Vitro-Study. J. Biophotonics 9, 637–644. doi: 10.1002/jbio.201500189
Prieto-Borja, L., Conde, A., Arenas, M. A., de Damborenea, J. J., Esteban, J. (2017). Influence of Exposure Time on the Release of Bacteria From a Biofilm on Ti6Al4V Discs Using Sonication: An In Vitro Model. Diagn. Microbiol. Infect. Dis. 89, 258–261. doi: 10.1016/j.diagmicrobio.2017.08.018
Rafiei, M., Kiani, F., Sayehmiri, F., Sayehmiri, K., Sheikhi, A., Zamanian Azodi, M. (2017). Study of Porphyromonas Gingivalis in Periodontal Diseases: A Systematic Review and Meta-Analysis. Med. J. Islam Repub. Iran 31, 62. doi: 10.18869/mjiri.31.62
Ramesh, D., Sridhar, S., Siddiqui, D. A., Valderrama, P., Rodrigues, D. C. (2017). Detoxification of Titanium Implant Surfaces: Evaluation of Surface Morphology and Bone-Forming Cell Compatibility. J. Bio- Tribo-Corros. 3, 1–13. doi: 10.1007/s40735-017-0111-2
Rehman, A., Hu, J., Ott, S. J., Grössner-Schreiber, B. (2012). Microbial Community Composition on Modified Dental Implant Surfaces: An In Vivo Study. Int. J. Oral. Maxillofac. Implants 27, 811–819.
Roberts, J. L., Khan, S., Emanuel, C., Powell, L. C., Pritchard, M. F., Onsøyen, E., et al. (2013). An In Vitro Study of Alginate Oligomer Therapies on Oral Biofilms. J. Dent. 41, 892–899. doi: 10.1016/j.jdent.2013.07.011
Rodríguez-Contreras, A., Marqués-Calvo, M. S., Gil, F. J., Manero, J. M. (2016). Modification of Titanium Surfaces by Adding Antibiotic-Loaded PHB Spheres and PEG for Biomedical Applications. J. Mater. Sci. Mater. Med. 27 (8), 124. doi: 10.1007/s10856-016-5723-4
Rupp, F., Liang, L., Geis-Gerstorfer, J., Scheideler, L., Hüttig, F. (2018). Surface Characteristics of Dental Implants: A Review. Dent. Mater. 34, 40–57. doi: 10.1016/j.dental.2017.09.007
Sahrmann, P., Zehnder, M., Mohn, D., Meier, A., Imfeld, T., Thurnheer, T. (2014). Effect of Low Direct Current on Anaerobic Multispecies Biofilm Adhering to a Titanium Implant Surface. Clin. Implant Dent. Relat. Res. 16, 552–556. doi: 10.1111/cid.12018
Santos-Coquillat, A., Gonzalez Tenorio, R., Mohedano, M., Martinez-Campos, E., Arrabal, R., Matykina, E. (2018). Tailoring of Antibacterial and Osteogenic Properties of Ti6Al4V by Plasma Electrolytic Oxidation. Appl. Surf. Sci. 454, 157–172. doi: 10.1016/j.apsusc.2018.04.267
Schmage, P., Kahili, F., Nergiz, I., Scorziello, T. M., Platzer, U., Pfeiffer, P. (2014). Cleaning Effectiveness of Implant Prophylaxis Instruments. Int. J. Oral. Maxillofac. Implants 29, 331–337. doi: 10.11607/jomi.2524
Schmidt, J. C., Astasov-Frauenhoffer, M., Hauser-Gerspach, I., Schmidt, J. P., Waltimo, T., Weiger, R., et al. (2014). Efficacy of Various Side-to-Side Toothbrushes for Noncontact Biofilm Removal. Clin. Oral. Investig. 18, 793–800. doi: 10.1007/s00784-013-1047-7
Schmidt, K. E., Auschill, T. M., Heumann, C., Frankenberger, R., Eick, S., Sculean, A., et al. (2017). Influence of Different Instrumentation Modalities on the Surface Characteristics and Biofilm Formation on Dental Implant Neck, In Vitro. Clin. Oral. Implants Res. 28, 483–490. doi: 10.1111/clr.12823
Schmidt, K. E., Auschill, T. M., Sculean, A., Arweiler, N. B. (2019). Clinical Evaluation of Non-Surgical Cleaning Modalities on Titanium Dental Implants During Maintenance Care: A 1-Year Follow-Up on Prosthodontic Superstructures. Clin. Oral. Investig. 23 (4), 1921–1930. doi: 10.1007/s00784-018-2640-6
Schneider, S., Rudolph, M., Bause, V., Terfort, A. (2018). Electrochemical Removal of Biofilms From Titanium Dental Implant Surfaces. Bioelectrochemistry 121, 84–94. doi: 10.1016/j.bioelechem.2018.01.008
Schwarz, F., Sculean, A., Romanos, G., Herten, M., Horn, N., Scherbaum, W., et al. (2005). Influence of Different Treatment Approaches on the Removal of Early Plaque Biofilms and the Viability of SAOS2 Osteoblasts Grown on Titanium Implants. Clin. Oral. Investig. 9, 111–117. doi: 10.1007/s00784-005-0305-8
Sennhenn-Kirchner, S., Schwarz, P., Schliephake, H., Konietschke, F., Brunner, E., Borg-Von Zepelin, M. (2009). Decontamination Efficacy of Erbium:Yttrium-Aluminium-Garnet and Diode Laser Light on Oral Candida Albicans Isolates of a 5-Day In Vitro Biofilm Model. Lasers Med. Sci. 24, 313–320. doi: 10.1007/s10103-008-0561-3
Shi, Y. Y., Li, M., Liu, Q., Jia, Z. J., Xu, X. C., Cheng, Y., et al. (2016). Electrophoretic Deposition of Graphene Oxide Reinforced Chitosan–Hydroxyapatite Nanocomposite Coatings on Ti Substrate. J. Mater. Sci. Mater. Med. 27, 1–13. doi: 10.1007/s10856-015-5634-9
Souza, J. G. S., Cordeiro, J. M., Lima, C. V., Barão, V. A. R. (2018). Citric Acid Reduces Oral Biofilm and Influences the Electrochemical Behavior of Titanium: An in Situ and In Vitro Study. J. Periodontol. 90 (2), 149–158. doi: 10.1002/JPER.18-0178
Strever, J. M., Lee, J., Ealick, W., Peacock, M., Shelby, D., Susin, C., et al. (2017). Erbium, Chromium: Yttrium-Scandium-Gallium-Garnet Laser Effectively Ablates Single-Species Biofilms on Titanium Disks Without Detectable Surface Damage. J. Periodontol. 88, 484–492. doi: 10.1902/jop.2016.160529
Subramanian, B., Ananthakumar, R., Kobayashi, A., Jayachandran, M. (2012). Surface Modification of 316L Stainless Steel With Magnetron Sputtered TiN/VN Nanoscale Multilayers for Bio Implant Applications. J. Mater. Sci. Mater. Med. 23, 329–338. doi: 10.1007/s10856-011-4500-7
Tamai, K., Kawate, K., Kawahara, I., Takakura, Y., Sakaki, K. (2009). Inorganic Antimicrobial Coating for Titanium Alloy and its Effect on Bacteria. J. Orthop. Sci. 14, 204–209. doi: 10.1007/s00776-008-1306-7
Ting, M., Craig, J., Balkin, B. E., Suzuki, J. B. (2018). Peri-Implantitis: A Comprehensive Overview of Systematic Reviews. J. Oral. Implantol. 44, 225–247. doi: 10.1563/aaid-joi-D-16-00122
Trobos, M., Juhlin, A., Shah, F. A., Hoffman, M., Sahlin, H., Dahlin, C. (2018). In Vitro Evaluation of Barrier Function Against Oral Bacteria of Dense and Expanded Polytetrafluoroethylene (PTFE) Membranes for Guided Bone Regeneration. Clin. Implant Dent. Relat. Res. 20, 738–748. doi: 10.1111/cid.12629
Trujillo, N. A., Oldinski, R. A., Ma, H., Bryers, J. D., Williams, J. D., Popat, K. C. (2012). Antibacterial Effects of Silver-Doped Hydroxyapatite Thin Films Sputter Deposited on Titanium. Mater. Sci. Eng. C 32, 2135–2144. doi: 10.1016/j.msec.2012.05.012
Verardi, G., Cenci, M. S., Maske, T. T., Webber, B., dos Santos, L. R. (2016). Antiseptics and Microcosm Biofilm Formation on Titanium Surfaces. Braz. Oral. Res. 30, 1–6. doi: 10.1590/1807-3107BOR-2016.vol30.0030
Vilarrasa, J., Delgado, L. M., Galofré, M., Àlvarez, G., Violant, D., Manero, J. M., et al. (2018). In Vitro Evaluation of a Multispecies Oral Biofilm Over Antibacterial Coated Titanium Surfaces. J. Mater. Sci. Mater. Med. 29 (11), 164. doi: 10.1007/s10856-018-6168-8
Wang, D., Haapasalo, M., Gao, Y., Ma, J., Shen, Y. (2018). Antibiofilm Peptides Against Biofilms on Titanium and Hydroxyapatite Surfaces. Bioact. Mater. 3, 418–425. doi: 10.1016/j.bioactmat.2018.06.002
Wang, J., Wu, G., Liu, X., Sun, G., Li, D., Wei, H. (2017). A Decomposable Silica-Based Antibacterial Coating for Percutaneous Titanium Implant. Int. J. Nanomedicine 12, 371–379. doi: 10.2147/IJN.S123622
Wiedmer, D., Petersen, F. C., Lönn-Stensrud, J., Tiainen, H. (2017). Antibacterial Effect of Hydrogen Peroxide-Titanium Dioxide Suspensions in the Decontamination of Rough Titanium Surfaces. Biofouling 33, 451–459. doi: 10.1080/08927014.2017.1322585
Wood, N. J., Jenkinson, H. F., Davis, S. A., Mann, S., O’Sullivan, D. J., Barbour, M. E. (2015). Chlorhexidine Hexametaphosphate Nanoparticles as a Novel Antimicrobial Coating for Dental Implants. J. Mater. Sci. Mater. Med. 26, 1–10. doi: 10.1007/s10856-015-5532-1
Yamada, Y., Yamada, M., Ueda, T., Sakurai, K. (2014). Reduction of Biofilm Formation on Titanium Surface With Ultraviolet-C Pre-Irradiation. J. Biomater. Appl. 29, 161–171. doi: 10.1177/0885328213518085
Ye, J., Shao, C., Zhang, X., Guo, X., Gao, P., Cen, Y., et al. (2017). Effects of DNase I Coating of Titanium on Bacteria Adhesion and Biofilm Formation. Mater. Sci. Eng. C 78, 738–747. doi: 10.1016/j.msec.2017.04.078
Yucesoy, D. T., Hnilova, M., Boone, K., Arnold, P. M., Snead, M. L., Tamerler, C. (2015). Chimeric Peptides as Implant Functionalization Agents for Titanium Alloy Implants With Antimicrobial Properties. JOM 67, 754–766. doi: 10.1007/s11837-015-1350-7
Zhang, H., Komasa, S., Mashimo, C., Sekino, T., Okazaki, J. (2017). Effect of Ultraviolet Treatment on Bacterial Attachment and Osteogenic Activity to Alkali-Treated Titanium With Nanonetwork Structures. Int. J. Nanomedicine 12, 4633–4646. doi: 10.2147/IJN.S136273
Zhang, Y., Zheng, Y., Li, Y., Wang, L., Bai, Y., Zhao, Q., et al. (2015). Tantalum Nitride-Decorated Titanium With Enhanced Resistance to Microbiologically Induced Corrosion and Mechanical Property for Dental Application. PloS One 10, 1–22. doi: 10.1371/journal.pone.0130774
Zhang, T., Zhou, W., Jia, Z., Wei, Q., Fan, D., Yan, J., et al. (2018). Polydopamine-Assisted Functionalization of Heparin and Vancomycin Onto Microarc-Oxidized 3D Printed Porous Ti6Al4V for Improved Hemocompatibility, Osteogenic and Anti-Infection Potencies. Sci. China Mater. 61, 579–592. doi: 10.1007/s40843-017-9208-x
Keywords: dental implant, decontamination, titanium, bacteria, biofilm
Citation: Dhaliwal JS, Abd Rahman NA, Ming LC, Dhaliwal SKS, Knights J and Albuquerque Junior RF (2021) Microbial Biofilm Decontamination on Dental Implant Surfaces: A Mini Review. Front. Cell. Infect. Microbiol. 11:736186. doi: 10.3389/fcimb.2021.736186
Received: 04 July 2021; Accepted: 09 September 2021;
Published: 08 October 2021.
Edited by:
Jean-Paul Motta, U1220 Institut de Recherche en Santé Digestive (INSERM), FranceReviewed by:
Ferdinand Xiankeng Choong, Karolinska Institutet (KI), SwedenCopyright © 2021 Dhaliwal, Abd Rahman, Ming, Dhaliwal, Knights and Albuquerque Junior. This is an open-access article distributed under the terms of the Creative Commons Attribution License (CC BY). The use, distribution or reproduction in other forums is permitted, provided the original author(s) and the copyright owner(s) are credited and that the original publication in this journal is cited, in accordance with accepted academic practice. No use, distribution or reproduction is permitted which does not comply with these terms.
*Correspondence: Jagjit Singh Dhaliwal, amFnaml0LmRoYWxpd2FsQHViZC5lZHUuYm4=
Disclaimer: All claims expressed in this article are solely those of the authors and do not necessarily represent those of their affiliated organizations, or those of the publisher, the editors and the reviewers. Any product that may be evaluated in this article or claim that may be made by its manufacturer is not guaranteed or endorsed by the publisher.
Research integrity at Frontiers
Learn more about the work of our research integrity team to safeguard the quality of each article we publish.