- 1Center for Global Infectious Disease Research, Seattle Children’s Research Institute, Seattle, WA, United States
- 2Department of Global Health, University of Washington, Seattle, WA, United States
- 3Department of Obstetrics & Gynecology, University of Washington, Seattle, WA, United States
- 4Department of Pediatrics, University of Washington, Seattle, WA, United States
- 5Department of Obstetrics and Gynecology, University of Washington and Sahlgrenska Academy, University of Gothenburg, Gothenburg, Sweden
Group B streptococcus (GBS) is a gram-positive bacteria that asymptomatically colonizes the vaginal tract. However, during pregnancy maternal GBS colonization greatly predisposes the mother and baby to a wide range of adverse outcomes, including preterm birth (PTB), stillbirth, and neonatal infection. Although many mechanisms involved in GBS pathogenesis are partially elucidated, there is currently no approved GBS vaccine. The development of a safe and effective vaccine that can be administered during or prior to pregnancy remains a principal objective in the field, because current antibiotic-based therapeutic strategies do not eliminate all cases of invasive GBS infections. Herein, we review our understanding of GBS disease pathogenesis at the maternal-fetal interface with a focus on the bacterial virulence factors and host defenses that modulate the outcome of infection. We follow GBS along its path from an asymptomatic colonizer of the vagina to an invasive pathogen at the maternal-fetal interface, noting factors critical for vaginal colonization, ascending infection, and vertical transmission to the fetus. Finally, at each stage of infection we emphasize important host-pathogen interactions, which, if targeted therapeutically, may help to reduce the global burden of GBS.
Introduction
Group B Streptococcus (GBS) (also called Streptococcus agalactiae) is a gram-positive, β-hemolytic, and chain-forming bacterium that can asymptomatically colonize the human vaginal and gastrointestinal tracts. However, during pregnancy, GBS can become highly invasive and pathogenic to the fetus and mother, leading to adverse outcomes. Invasive GBS infections during pregnancy result in preterm birth (PTB), stillbirth, and fetal death. The fetus and neonate are highly susceptible to GBS, causing sepsis, pneumonia, and meningitis which, in severe cases, leads to long-lasting sequelae that can affect the child’s central nervous system and lung function (Bianchi-Jassir et al., 2017; Madrid et al., 2017; Seale et al., 2017b). In the mother, GBS infections can lead to maternal sepsis and postpartum endometritis (Hall et al., 2017). The complex pathogenesis of maternal and infant GBS infection arises due to the large arsenal of the bacterium’s virulence factors, which can vary widely across strains and undergo altered expression depending on the host niche. Some virulence factors are implicated in a single disease outcome, such as the role of HvgA in neonatal meningitis (Tazi et al., 2010). Other virulence factors are more broadly associated with GBS pathogenesis, including the hemolysin (also called β-hemolysin/cytolysin) (Pritzlaff et al., 2001; Liu et al., 2004; Hensler et al., 2005; Lembo et al., 2010); the molecular basis of which is the GBS pigment, or granadaene and has direct cytotoxic effects against many types of host cells (Whidbey et al., 2013; Gendrin et al., 2015; Whidbey et al., 2015; Boldenow et al., 2016; Armistead et al., 2020a). Host-pathogen interactions that alter the balance between vaginal tract colonization and GBS invasive disease ascending into the uterus are beginning to be elucidated (Vornhagen et al., 2018a). Further investigation of the GBS bacterial factors enabling colonization of the vagina and invasive disease will facilitate the design of novel GBS prophylactics and treatments that directly target pathogenesis. In this review, we summarize the seminal studies and recent advances that define our understanding of GBS disease pathogenesis at the maternal-fetal interface, focusing on the interplay between bacterial virulence factors and host defenses that ultimately leads to GBS clearance or invasion.
Methods
Search Strategy and Selection Criteria
To identify recent and seminal GBS research relating to maternal colonization, ascending infection, and fetal injury, literature searches were performed using Google Scholar and PubMed Central. Variants of the terms “pregnancy,” “preterm labor,” “premature,” “vaginal colonization,” “colonization,” “uterus,” “ascending infection,” “placenta,” “fetus,” “vaccine” were used in combination with “Group B Streptococcus” or “Streptococcus agalactiae.” The literature search was limited to articles published between 2014-2021. However, we used snowball searches of article reference list to identify studies that were seminal for recent work in the field, including work published prior to 2014.
GBS Epidemiology and Prophylactic Measures
Global Epidemiology of GBS Disease Outcomes
GBS colonizes the rectovaginal space in approximately 20-25% of women worldwide, with rates varying widely in different parts of the world (Seale et al., 2017a). In a meta-analysis that pooled estimates of GBS colonization from 85 countries, the prevalence of colonization varied between 7-14% in Central America and Asia to 35% in the Caribbean. Europe, North America, and Australia had similar prevalence rates of 15-20%. Interestingly, estimates in Africa varied widely with West and South Africa at 14 and 25%, respectively (Russell et al., 2017). Due to the asymptomatic nature of rectovaginal colonization, it is difficult to determine if the prevalence of GBS colonization is changing over time; however, there is evidence that invasive GBS infection of nonpregnant adults is increasing. Between 2008 to 2018 within the Active Bacterial Core surveillance network, the incidence of invasive GBS infection in nonpregnant individuals increased from 8.1 to 10.8 cases per 100,000 adults, representing 11.5% of the US population (Francois Watkins et al., 2019). These studies highlight that GBS contributes significantly to the global burden of disease, and in addition, emphasize that GBS rectovaginal colonization is common worldwide.
Although GBS vaginal colonization is asymptomatic in most women, it is of particular concern during pregnancy due to the significant risk for adverse fetal and neonatal outcomes (Figure 1). Maternal GBS infection is a leading cause of infection-induced PTB and stillbirth (Seale et al., 2017a). Further, in 2015 more than 20 million neonates were exposed to maternal GBS out of 140 million live births worldwide, and half of neonates born to pregnant GBS-colonized women are estimated to themselves become colonized (Seale et al., 2017a). In a conservative analysis of international data from a single year, at least 90,000 infant deaths (<3 months of age), 10,000 cases of disability in children, 57,000 fetal infections or stillbirths, and 3.5 million cases of PTB were attributed to GBS. A disproportionate burden of these GBS cases occurred in Africa, which accounts for 54% of annual GBS cases and 64% of all fetal and infant deaths (Seale et al., 2017a). In addition to its fetal and neonatal disease burden, GBS can cause serious maternal disease. Recently, it was estimated that there are at least 33,000 annual cases of maternal invasive GBS disease worldwide (Seale et al., 2017a). Another systematic literature review found that 0.38 women per 1,000 pregnancies experience invasive GBS disease with symptoms ranging from endocarditis or pneumonia to maternal death in severe cases (Hall et al., 2017). GBS invasive disease in pregnancy poses significant risks to both the fetus and mother.
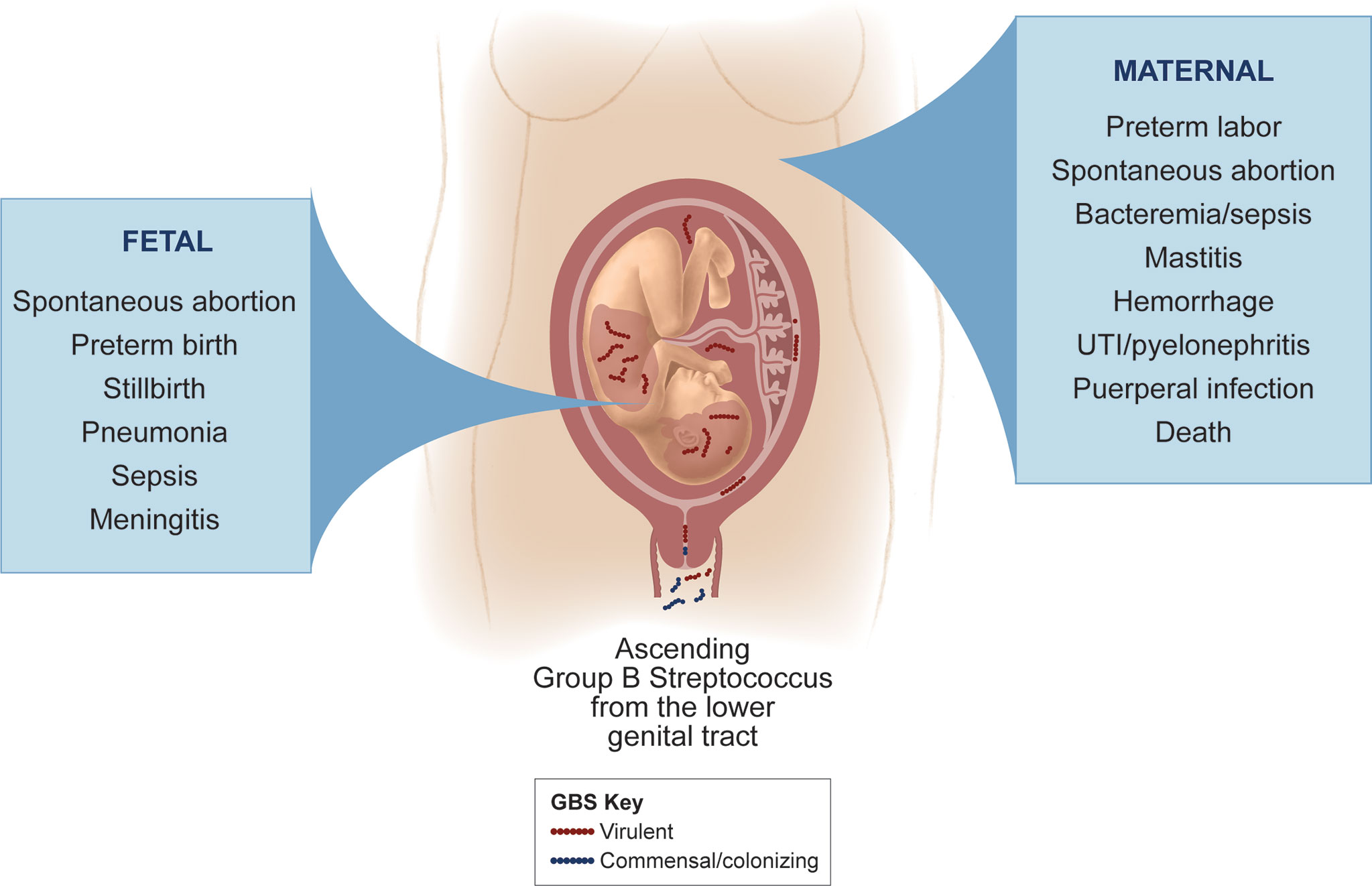
Figure 1 GBS disease manifestations for mothers and fetuses. GBS is considered a commensal colonizer of the maternal vaginal tract, but ascension of GBS into the uterus can lead to adverse perinatal outcomes. GBS can invade the amniotic cavity and fetus to cause a variety of disease manifestations in the fetus (left panel), some of which can occur even with a limited infection of the placental chorioamniotic membranes. Maternal GBS invasive disease is more infrequent and can vary in severity (right panel); more severe outcomes tend to occur in the setting of chorioamniotic membrane rupture and/or with maternal co-morbidities that compromise the immune response.
Importantly, the prevalence of maternal, fetal, and neonatal disease are likely underestimated. In low-income countries that have the highest rates of invasive GBS cases, the microbiological cause of sepsis, PTB, stillbirth, or death may not be recognized if cultures are not obtained or if formalized pre- or antenatal visits are inaccessible. In addition, policies for GBS screening are highly variable both geographically and across high, middle, and low income countries. Policies regarding screening generally fall into two categories: microbiological or risk-factor based screening. Microbiological screening, sometimes known as universal screening, tests all mothers at a specific gestational age. In contrast, risk-factor based screening is more cautious in that mothers are tested only if they present with symptoms of GBS invasive disease (such as preterm labor) or if they have a history of adverse pregnancy outcomes. Screening methods, however, are not necessarily consistent among nations with similar economic status; for example the United States performs universal microbiological screening by rectovaginal swab at 35-37 weeks gestation, while Sweden and the United Kingdom utilize risk-based screening (Le Doare et al., 2017). Mothers who test positive for GBS are generally administered intravenous antibiotics during labor, called intrapartum antibiotic prophylaxis (IAP). Unfortunately IAP policies also vary due to cost or rising concerns of antibiotic resistance. Discrepancies in both screening and antibiotic use can have drastic effects on GBS case counts and birth outcomes. When symptoms present only after delivery, cases may not be attributed to GBS until late in the clinical course. Subclinical cases that can still lead to lifelong complications may remain undetected and untreated, and may never be linked to GBS infection. Additionally, by the time symptoms present in the clinic it may be too late to intervene. Rapid and low-cost testing strategies that can be implemented in low-resource settings would likely allow for more accurate estimates of the GBS burden of disease, and might provide opportunities to reduce this burden through IAP intervention.
GBS Serotypes and Capsule Polysaccharide-Based Vaccines
Many GBS virulence factors influence a strain’s ability to colonize or cause severe disease. One key virulence factor is the sialylated GBS capsular polysaccharide (CPS), first identified by Dr. Rebecca Lancefield in the 1930s (Lancefield, 1934). To date, there are ten known capsular serotypes (Ia, Ib, II-IX), although six account for 98% of GBS colonization worldwide (Ia, Ib, II-V) (Russell et al., 2017). These same serotypes cover over 99% of overall cases, including EOD and LOD (Nanduri et al., 2019). Not unlike colonization rates and disease burden, GBS serotype frequencies vary geographically. For example, 25% of colonizing strains are serotype III worldwide, but this serotype only accounts for up to 10% of cases in Asian and African countries. Other serotypes such as V-IX are more prevalent in Africa and Southern, South-Eastern, and Eastern Asia (Russell et al., 2017). In a meta-analysis, serotype Ia and III were linked to 31 and 27% of GBS maternal invasive cases, respectively (Hall et al., 2017), while fetal and neonatal disease was dominated by serotype III which was responsible for 62% of cases. Serotypes Ia, Ib, and V are also commonly associated with EOD and LOD, but their contribution is largely dwarfed by serotype III (Madrid et al., 2017).
Although the GBS capsule is involved in a variety of immune evasion mechanisms (Marques et al., 1992; Takahashi et al., 1999; Weiman et al., 2010; Uchiyama et al., 2019), the GBS CPS conjugated to an immunogenic protein carrier is a well-studied vaccine strategy. Vaccination of pregnant rats with CPS III conjugate reduced vaginal colonization, chorioamnionitis, and vertical transmission (Chiarot et al., 2018), spurring the examination of these vaccines in human clinical trials. In nonpregnant women, this vaccine induced heightened antibody responses and delayed vaginal and rectal acquisition of GBS (Hillier et al., 2019). A major limitation of CPS-based vaccines is the need to confer protection against multiple serotypes, and thus multivalent CPS vaccine strategies are of high interest. A Novartis/GSK trivalent vaccine (Novartis/GSK GBS3) comprised of CPS Ia/Ib/III conjugate is considered safe in pregnant women, elicited high maternal antibody titers and the antibodies were placentally transferred to infants (Swamy et al., 2020). Unfortunately, a trivalent vaccine still does not include all clinically-relevant serotypes and could lead to selection of non-vaccine strains through capsule switching, a phenomenon that has been observed in many high-risk populations and among ST-17 strains (Bellais et al., 2012; Meehan et al., 2014; Neemuchwala et al., 2016; Nagano et al., 2019). Further, a recent study identified patients that were co-infected by multiple GBS serotypes at once (Jisuvei et al., 2020), which may enable capsule switching. Thus, a hexavalent CPS conjugate vaccine derived from serotypes Ia, Ib, II, III, IV, and V would cover more clinically-relevant strains (Bianchi-Jassir et al., 2020). A hexavalent CPS conjugate vaccine (Pfizer GBS6) induced the production of opsonophagocytic antibodies in vaccinated rhesus macques and protected murine infant pups from lethal GBS challenge upon maternal vaccination (Buurman et al., 2019). This Pfizer GBS6 vaccine was also well-tolerated in healthy adults and induced high antibody titers (Absalon et al., 2021), but its immunogenicity and efficacy has not been examined in clinical trials in pregnant women. Compared to whole CPS-based vaccines that are difficult to produce, an alternative strategy might utilize a more specific CPS epitope that can be chemically synthesized. One group has utilized X-ray crystallography and epitope mapping to design a synthetic CPS III glycan-conjugate vaccine (Michon et al., 2006; Carboni et al., 2017; Carboni et al., 2020), which appears to confer similar levels of protection to whole CPS conjugate (Oldrini et al., 2020). However, to address the challenge of capsule switching, highly technical and intensive X-ray crystallography studies would be needed to identify relevant epitopes for the other CPS types. A more recent development that might simplify this problem is the production of GBS strains that express chimeric CPS. In a mouse model, maternal vaccination with a chimeric CPS V/IX conjugate resulted in the protection of pups from infection by GBS serotypes V or IX (Campisi et al., 2021). Owing to the limited immunogenicity of CPS, the difficulty of attaining serotype coverage, and challenges related to CPS manufacturing, other virulence factors may serve as better or alternate vaccine targets.
GBS Sequence Types and Virulence Factor Epidemiology
Aside from its serotype distinction, GBS can also be clustered genetically through a technique known as multi-locus sequence typing. This is performed by sequencing seven housekeeping genes for any GBS strain-of-interest, and the combination of these seven genomic loci generates a sequence type (ST) (sometimes called clonal complex or CC) (Jones et al., 2003). Among a global and ecologically diverse sample of strains, four major sequence types were found, ST-1, ST-17, ST19, and ST23 (Jones et al., 2003). Of these, ST-1 and ST-19 were largely associated with asymptomatic carriage, ST-23 was common among both asymptomatic carriers and invasive disease cases, and ST-17 serotype III strains were attributed to neonatal invasive infections (Jones et al., 2003). In contrast, a study of 225 invasive isolates from Taiwan implicated both ST-1 and ST-17 as invasive isolates causing maternal and neonatal disease (Tsai et al., 2019). Importantly, ST-17 strains are emerging internationally and are associated with an increased propensity for neonatal meningitis and antibiotic resistance (Tazi et al., 2010; Teatero et al., 2016; Kao et al., 2019). However, antibiotic resistance is also emerging among non-ST-17 strains (Hays et al., 2016). This increase in antibiotic resistance poses a significant threat, as IAP is currently the only FDA-approved GBS treatment for pregnant women and the only preventative measure against vertical transmission of GBS. Hypervirulent strains, including ST-17, have also recently been isolated from tilapia (Evans et al., 2009; Chen, 2019), and there are rising concerns that tilapia-adapted GBS might serve as a zoonotic route for human transmission (Sun et al., 2016). These challenges emphasize important gaps in screening for antibiotic resistant isolates, which could inform intrapartum antibiotic prophylaxis. In addition, hypervirulent ST-17 GBS may have emerged in humans following zoonotic transmission from cows (Bisharat et al., 2004), and thus improved GBS surveillance among animal reservoirs might provide insight into the emergence of clinically significant isolates and consequent challenges.
GBS Vaginal Colonization and Persistence
Maternal GBS Colonization Is a Risk Factor for Subsequent Disease
During infection of the maternal-fetal interface, GBS first encounters the maternal rectovaginal tract. In fact, one of the best-defined risk factors for fetal or neonatal adverse outcomes is maternal colonization (Bianchi-Jassir et al., 2017). Although only about 25% of women are colonized (Russell et al., 2017), GBS is often considered a member of the host microbiota because carriage is usually asymptomatic. However, temporal carriage of vaginal GBS can be incredibly variable; wherein one patient may be persistently colonized whereas another may experience recurring or intermittent colonization (Brzychczy-Wloch et al., 2014; Sroka-Oleksiak et al., 2020). Additional studies are needed to understand how colonization varies temporally and across populations, particularly among pregnant and nonpregnant women. Current findings suggest that vaginal colonization is regulated by many overlapping bacterial and host factors. Gastrointestinal and rectal GBS colonization are likely to modulate maternal colonization by directly seeding GBS into the vaginal tract (Meyn et al., 2009). For this review, we will focus on vaginal colonization as this location is the primary source of GBS that can lead to maternal-fetal disease. Unless otherwise specified, GBS colonization or carriage refers to that within the vaginal tract.
Interactions Between the Vaginal Microbiome and GBS Are Complex
The vaginal microbiota is composed of a wide array of taxa that varies geographically, by racial or ethnic group, throughout the menstrual cycle, and during pregnancy (Sroka-Oleksiak et al., 2020). Many taxa, including Prevotella bivia, Veillonella spp., Eubacterium siraeum, and Staphylococcus spp. have been associated with GBS vaginal colonization in nonpregnant women. In contrast, bacterial vaginosis (BV)-associated bacterium 1 and 2, Prevotella genogroups 3 and 4, Dialister species type 2, and Megasphaera species type 1 are associated with a lack of GBS (Rosen et al., 2017). Many of these latter taxa are associated with bacterial vaginosis (BV), a heterogeneous vaginal microbial dysbiosis (Onderdonk et al., 2016). However, a recent small study of nonpregnant Chinese women observed increased Prevotella and Megasphaera spp. abundance among women who were GBS-colonized (Mu et al., 2019). Other studies using rats and mice have uncovered relationships between GBS colonization and the presence of BV-associated bacteria such as P. bivia (Cools et al., 2016) and Gardnerella vaginalis (Gilbert et al., 2021). Clearly, the relationships between GBS and other vaginal taxa are complicated. Conflicting findings across studies may be due in part to variation in vaginal microbiome composition, which is common across human cohorts and when comparing laboratory animals in different facilities; discrepancies in culturing and sequencing methods further complicates the comparison of such studies. Although majority of findings regarding GBS’ interaction with other microbes are associative, GBS co-colonization with Candida albicans observed clinically (Cools et al., 2016) is supported mechanistically by observations of interaction between the GBS BspA adhesin and the C. albicans hyphae-specific surface protein Als3 (Rego et al., 2016). Whether the presence of any of these taxa alter GBS colonization rates in humans is unknown, as well as the mechanisms by which colonization may be altered. Future studies should interrogate changes in niche remodeling, nutrient availability and the immune environment that may occur alongside shifts in microbiome composition. It is possible that a combination of these factors contributes to the variable and fluctuating rates of GBS carriage.
Vaginal Lactobacilli: Key Species That Limit GBS Colonization and Persistence
The healthy vaginal tract maintains an acidic pH due to the Lactobacillus species that metabolize glycogen and produce lactic acid (Boskey et al., 2001). In reproductive-age women, the microbiome can be categorized into 5 community state types (CSTs I-V) based on the dominating Lactobacillus species. Mechanisms by which Lactobacilli restrain GBS colonization at the microbiome-level remain unclear, but multiple studies have partially defined the interactions between different Lactobacillus species and GBS. Lactobacilli can have antagonistic effects on the growth of GBS and host cell interactions in vitro (Bodaszewska et al., 2010; Ortiz et al., 2014; Marziali et al., 2019; He et al., 2020). While in human observational vaginal microbiome studies, the relationship between Lactobacillus-replete microbiomes and GBS colonization is less clear (Kubota et al., 2002; Altoparlak et al., 2004; Daniel Johannes Rönnqvist et al., 2006; Brzychczy-Wloch et al., 2014; Rick et al., 2017), there is evidence that reduced taxonomic diversity and Lactobacillus dominance are possible mechanisms of microbiome-based protection against GBS during pregnancy (Sroka-Oleksiak et al., 2020). An exception is CST IV, which encompasses a more diverse and dysbiotic assortment of non-Lactobacillus anaerobes accompanied by increased vaginal pH (Ravel et al., 2011). This CST can be further differentiated into IV-A and IV-B (Gajer et al., 2012), and CST IV-A has been linked to increased GBS risk and appears to be Streptococcus and Prevotella-dominant (Rosen et al., 2017; Brotman et al., 2018). These data suggest that Lactobacilli alone do not explain differences in GBS carriage status, although this genus likely affects GBS colonization in concert with other vaginal taxa. A better understanding of what promotes Lactobacillus-replete vaginal microbiomes during pregnancy is needed, and how these changes alter the vaginal environment including immune responses remains to be determined. Interestingly, a subset of specific pathogen-free mice appears to possess Lactobacillus-dominant vaginal flora that might more closely mimic that of the healthy human vagina. Preliminary evidence suggests that this murine CST may impair GBS persistence (Vrbanac et al., 2018). A mechanistic understanding of the environmental or genetic cues that culminate in the development of this human-like murine vaginal microbiome could allow for more relevant studies of GBS vaginal colonization and persistence in mice.
GBS Adaptation in the Vaginal Tract Favor Virulence
GBS is frequently described as an opportunist or pathobiont due to its ability to transition from an asymptomatic colonizer to a potent pathogen (Armistead et al., 2019). During this transition, GBS must adapt to its new niche in order to successfully colonize and persist in the vaginal tract. Luckily, GBS encodes a plethora of two-component systems (TCS), each comprised of a sensor histidine kinase and DNA-binding response regulator (denoted by S and R, respectively), that grant the ability to respond to diverse environmental stimuli.
One well-studied GBS TCS, CovR/S (also known as CsrR/S), responds to low pH (Park et al., 2012), which is likely encountered in the healthy human vaginal tract. CovR/S is a master regulator of GBS virulence, and more than a hundred genes undergo transcriptional changes in response to CovR. Interestingly, one phenotype of GBS lacking this TCS (denoted as ΔcovRS or ΔcovR) is hyper-adhesion, which occurs due to increased expression of cell surface adhesins (Lamy et al., 2004; Park et al., 2012). CovR/S also regulates expression of the cyl operon (Jiang et al., 2005), which encodes factors required for the biosynthesis of GBS’ characteristic hemolysin (Whidbey et al., 2013; Whidbey et al., 2015; Armistead et al., 2019; Armistead et al., 2020a). This virulence factor is a pro-inflammatory cytotoxin that is essential for many facets of GBS disease including pneumonia, sepsis, meningitis, PTB, and invasive infection of adults (Nizet et al., 1996; Nizet et al., 1997; Gibson et al., 1999; Doran et al., 2002; Doran et al., 2003; Hensler et al., 2005; Whidbey et al., 2013; Whidbey et al., 2015; Boldenow et al., 2016; Armistead et al., 2020a; Armistead et al., 2020b), and the pro-inflammatory nature of this factor is likely the reason hyperhemolytic GBS strains have reduced vaginal persistence (Gendrin et al., 2015; Patras et al., 2015). Another more recently characterized TCS that is important for GBS vaginal carriage is SaeR/S, which was identified by a transcriptomic screen using mice that were vaginally colonized with GBS. The SaeR/S system regulates the transcription of surface adhesin PbsP during murine vaginal colonization, and in fact pbsP is upregulated more than 250-fold during murine vaginal colonization and GBS lacking PbsP exhibit reduced vaginal persistence (Cook et al., 2018). While the extracellular signal recognized by SaeR/S is unknown, it is hypothesized to be a small peptide, potentially an antimicrobial peptide (AMP) present in the vaginal tract. Future studies should aim to establish the relevance of SaeR/S in human vaginal colonization and persistence, and define the roles of other SaeR-regulated factors.
In contrast to CovR/S and SaeR/S, other TCSs confer protection against host defenses encountered in the vaginal tract, prolonging GBS survival. DltR/S is involved in cell wall lipoteichoic acid (LTA) maintenance, and importantly, loss of this system alters LTA expression and increases GBS susceptibility to AMPs common in the reproductive tract (Poyart et al., 2001; Poyart et al., 2003). LiaR/S also allows evasion of antimicrobial defenses by regulating cell wall synthesis and cell membrane modification, permitting GBS to evade AMPs (Madanchi et al., 2020). Although these TCS may contribute to vaginal persistence, their roles have yet to be interrogated in vivo.
GBS Factors That Facilitate Vaginal Adhesion and Persistence
GBS encodes numerous surface adhesins and invasins that interact with host epithelia and promote infection, although the data for some of these surface proteins is more convincing than for others. BspA, a member of the antigen I/II protein family, mediates binding to vaginal epithelial cells through interactions between its own V domain and the host gp340 surface receptor (Rego et al., 2016). Further, GBS surface β protein has recently been shown to interact with epithelial CEACAM1 and 5 receptors (van Sorge et al., 2021), which are common in the vagina and uterus, respectively (Islam et al., 2018). As previously discussed, PbsP promotes GBS vaginal persistence in mice (Cook et al., 2018). Apart from direct binding to host epithelial cell receptors, GBS can also interact with host extracellular matrix (ECM) components. The serine-rich repeat glycoprotein Srr1 binds to keratin 4 or fibrinogen (Samen et al., 2007; Mistou et al., 2009; Sheen et al., 2011; Seo et al., 2013); in murine models, this interaction contributes to cervicovaginal persistence in a fibrinogen- and Srr1-latch domain-dependent manner (Wang et al., 2014). FbsC (also called BsaB) similarly binds the host ECM via laminin and fibrinogen to allow cervicovaginal adhesion of GBS (Park et al., 2012; Buscetta et al., 2014). Other surface proteins, such as pili, are also important during GBS colonization. For example, bacterial competition studies show that pilus-null GBS are outcompeted by an isogenic wild-type strain during vaginal colonization of mice (Sheen et al., 2011). Although some GBS surface proteins have not been studied extensively in vivo, the above factors appear have been directly linked to the vaginal tract.
The Vaginal Immune Response Can Restrict GBS
GBS must also overcome the vaginal immune response in order to achieve persistent colonization. Vaginal tract colonization induces the recruitment of numerous immune cells, which can facilitate bacterial clearance through diverse mechanisms (Figure 2, vaginal tract). The production of IL-1β, IL-6, IL-8, IL-23, IL-17, IFN-γ, and TNF-α are associated with reduced GBS colonization (Patras et al., 2013; Carey et al., 2014; Patras et al., 2015; Sweeney et al., 2020). These proinflammatory mediators are primarily produced by neutrophils recruited through IL-8, CXCL1, and CXCL2 (Patras et al., 2013; Carey et al., 2014; Patras et al., 2015), macrophages (Carey et al., 2014), and vaginal epithelial cells (Patras et al., 2013). In addition, vagina-resident mast cells contribute to GBS clearance through the hemolysin-induced release of pre-formed inflammatory mediators, including histamine (Gendrin et al., 2015). However, the roles of natural killer and dendritic cells (DCs) in vaginal immune responses are incompletely understood. In mice, vaginal clearance is associated with Th1, Th2, and Th17 responses (Carey et al., 2014; Patras et al., 2015). Studies using B cell-deficient or neonatal Fc receptor-null mice suggest mucosal B cells are involved in the clearance of vaginal GBS (Baker et al., 2017). Further, increases in serotype-specific anti-CSP antibody titers and opsonophagocytic activity (OPA) correlate with reduced colonization rates (Kwatra et al., 2015). Importantly, multiple studies have identified strain-level differences in immune responses that may partially explain differences in strain and serotype colonization of the vagina (Patras et al., 2015; Sweeney et al., 2020).
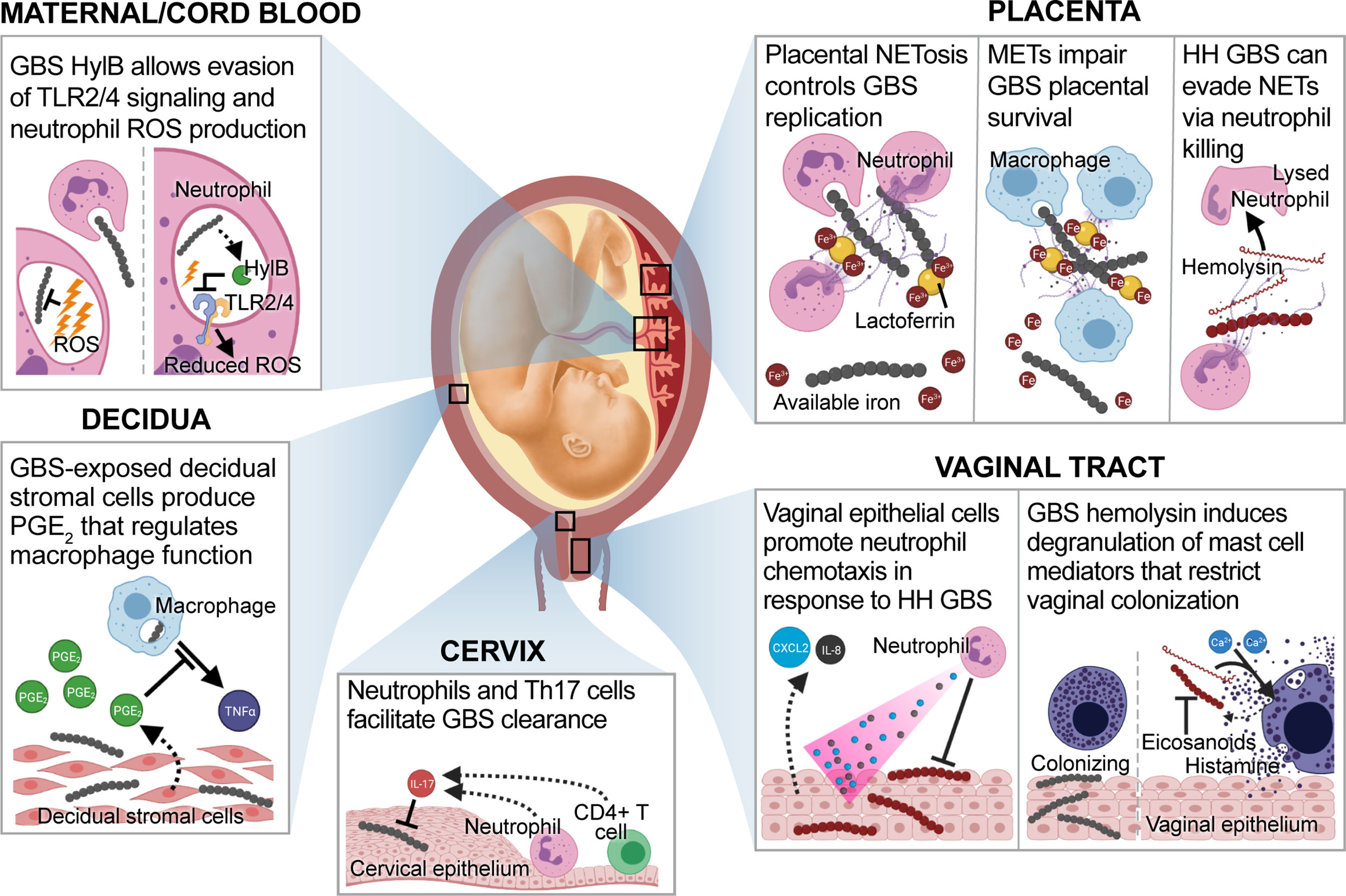
Figure 2 GBS-directed host immune responses at the maternal-fetal interface. During GBS infection at the maternal-fetal interface, a variety of immune cell interactions can occur. In the vaginal tract, epithelial cells exposed to hyperhemolytic (HH) GBS secrete C-X-C motif chemokine ligand 2 (CXCL2) and interleukin (IL)-8 which mediate GBS elimination through the recruitment of neutrophils (Patras et al., 2013). Whereas wild-type GBS colonize the lower genital tract in the presence of mast cells, HH GBS induce mast cell degranulation in a hemolysin and calcium-dependent manner. This results in the release of pre-formed mast cell mediators, such as eicosanoids and histamine that mediate GBS clearance (Gendrin et al., 2015). During ascending infection, cervical Th17 cells that produce IL-17 are associated with GBS clearance (Patras et al., 2015). Decidual stromal cells exposed to GBS produce prostaglandin E2 (PGE2), which suppresses macrophage tumor necrosis factor alpha (TNF-α) production (Rogers et al., 2018). Placental neutrophils produce neutrophil extracellular traps (NETs) that immobilize GBS and facilitate clearance via phagocytosis and nutritional immunity. NET-contained lactoferrin sequesters ferric iron (Fe3+), resulting in GBS growth arrest (Kothary et al., 2017). HH GBS evade placental NETs by hemolysin-mediated killing of neutrophils (Boldenow et al., 2016). Macrophages control placental GBS replication through a similar mechanism, and macrophage extracellular traps (METs) contain similar products (Doster et al., 2018). Finally, neutrophils in maternal and cord blood phagocytose GBS and produce reactive oxygen species (ROS) to mediate bacterial killing. However, GBS expressing hyaluronidase (HylB) inhibit this mechanism by interfering with toll-like receptor 2 and 4 signaling. Downstream effects of this immune dampening suppress ROS production and facilitate GBS survival (Coleman et al., 2021).
Novel Prophylactics to Prevent Vaginal Colonization
The ability to prevent maternal vaginal colonization would eliminate a significant proportion of GBS morbidity and mortality. GBS interactions in the vaginal tract highlight important bottlenecks and are defined by specific host-pathogen interactions that could be targeted by therapeutics. Although CPS conjugate vaccination is correlated with reductions in GBS carriage, serotype coverage and capsule switching are still major concerns. Thus, the development of other novel strategies remains a priority.
The vaginal microbiome is malleable, and therefore therapies capable of inducing changes in composition might successfully restrict or eliminate GBS. Although it remains unclear whether Lactobacilli inhibit GBS colonization in vivo, probiotic Lactobacillus species have potential as a novel therapeutic. In mice, serial vaginal inoculation with probiotic L. reuteri CRL1324 confers partial protection against GBS, and this effect is mediated at least in part by mucosal immunity (De Gregorio et al., 2015; De Gregorio et al., 2016). Human clinical trials for oral probiotic Lactobacilli are also encouraging. A small randomized controlled trial found that administration of L. rhamnosus GR-1 and L. reuteri RC-14 during the third trimester reduced GBS rectovaginal colonization rates at delivery (NCT01577108) (Ho et al., 2016). In another study, the same probiotics reduced the abundance of vaginal GBS and BV-associated taxa (Liu et al., 2020). In addition, the probiotic L. salivarius CECT9145 also reduced GBS rectovaginal colonization during pregnancy (Martín et al., 2019). Though these studies sound promising, others have observed no protective effect (Farr – NCT03008421, Sharpe – SCRD42020216531) (Olsen et al., 2018; Farr et al., 2020; Sharpe et al., 2021), and thus larger studies are required to confirm efficacy. It is also worth noting that due to differences in vaginal CST and microbiome composition, probiotics may not serve as a universal prophylactic against GBS. Furthermore, it is unknown how stable or long-lasting probiotic-induced changes to the microbiome are. As such, it is essential that follow-up studies are performed across international cohorts and in combination with substantial characterization of the vaginal microbiome to better inform the effects of oral probiotic use.
GBS utilizes many surface adhesins and invasins during the vaginal colonization stage, which have been targeted by vaccines (Table 1). Maternal vaccination with a chimeric protein derived from six variants of the pilus type 2a backbone subunit (6xD3) resulted in protection of neonates against lethal challenge with GBS that carry type 2a pili (Nuccitelli et al., 2011); however, vaginal colonization was not interrogated. A similar mouse model determined that maternal vaccination with a C5a peptidase-containing vaccine protected neonates against lethal challenge and, furthermore, prevented subsequent vaginal colonization of the dam (Santillan et al., 2008; Santillan et al., 2011). BibA also holds promise as a vaccine, as mucosal vaccination with recombinant BibA resulted in protection of mice against both systemic and vaginal challenge (dos Santos et al., 2020). Moreover, this vaccine also conferred protection to neonatal mice in a maternal vaccination model (Santi et al., 2009). These studies highlight that adhesin-based vaccines are encouraging, although these strategies are more nascent than CPS conjugate vaccines and thus many remain in preclinical stages. Even so, these vaccines are not without limitations. A major challenge in targeting adhesins is their functional redundancy, and thus future studies need to test which adhesins are dispensable in vivo and which are not. In addition, while the surface adhesins are better conserved than CPS, GBS adhesins may vary by strain or across ST lineages. Consequently, adhesin-based strategies may still need to target multiple GBS factors in order to prevent colonization by all relevant strains. Despite this limitation, novel vaccines utilizing GBS adhesins and invasins antigens warrant future investigation in clinical trials, and should be considered as an attractive alternative to CPS-based vaccines.
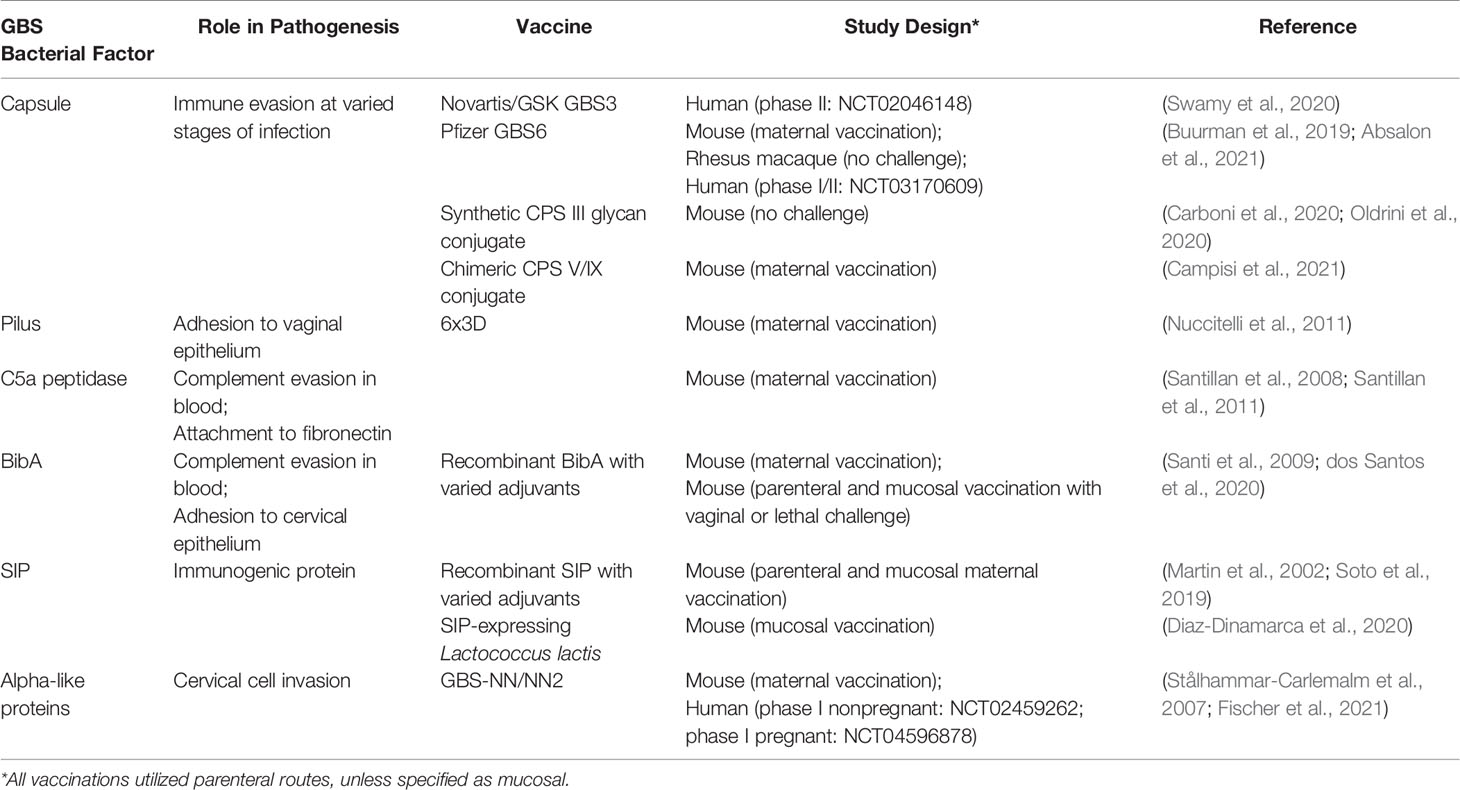
Table 1 Novel in-pipeline vaccines that target GBS determinants of vaginal colonization and invasive disease.
Finally, vaccine strategies that target GBS factors important for vaginal colonization should consider the route of administration as mucosal immunizations may better direct immune response to relevant tissue sites. Intranasal immunization of ethanol-killed whole GBS generated both robust systemic and mucosal responses and enhanced GBS clearance from the vaginal tract. In contrast, intramuscular immunization promoted systemic and mucosal antibodies response, but there was no difference in GBS vaginal colonization between vaccinated and control mice (Baker et al., 2017). Additionally, studies on the exceptionally well-conserved surface immunogenic protein (SIP) highlight important lessons for GBS vaccination. First, parenteral or mucosal vaccination of dams with recombinant SIP led to neonatal protection from GBS invasive disease (Martin et al., 2002). Second, a subcutaneous vaccination of SIP with AbISCO-100 adjuvant resulted in both humoral and cellular responses that protected against GBS vaginal colonization, whereas the vaccine adjuvanted with aluminum hydroxide resulted in only a weak humoral response. Serum or T cell transfer from vaccinated mice significantly reduced vaginal GBS burden in naïve mice, with T cells showing slightly higher efficacy than serum (Soto et al., 2019). This study emphasizes the importance of novel adjuvants in the formulation of a successful vaccine. Finally, mucosal vaccination may be more effective in stimulating immune cells in the vaginal tract; however, mucosal delivery is challenging. A third study by this group demonstrated a novel use for Lactococcus lactis as a delivery system for recombinant SIP, which again resulted in a strong humoral and cellular response that aided in vaginal clearance (Diaz-Dinamarca et al., 2020). GBS vaccines targeting SIP with an optimized adjuvant represent a promising platform to reduce GBS vaginal colonization.
GBS Ascending Infection
Ascension Of GBS From the Vaginal Tract May Require Transient Interaction With the Cervix
Most pathogens that cause intra-amniotic infections are vaginal tract commensals, highlighting the importance of ascending infection as a mechanism of invasive disease during pregnancy (Vornhagen et al., 2016; Vornhagen et al., 2018a; Romero et al., 2019). As a nonmotile bacterium, it is puzzling that GBS is able to ascend from the colonized vagina to the uterus. However, multiple mechanisms have been implicated in this stage of infection. GBS can promote the loss of vaginal epithelial barrier function by stimulating vaginal exfoliation (Vornhagen et al., 2018a). Additionally, GBS can interact with epithelial cells of the cervix using its diverse adhesins and invasins. Thus, cervical cell adhesion and invasion may promote GBS ascending infection into the uterus. Some adhesins, such as FbsC and Srr1, exhibit redundant function in the vagina and cervix (Buscetta et al., 2014; Jiang and Wessels, 2014; Wang et al., 2014). In contrast, BibA mediates complement evasion in the blood but also appears to mediate cervical cell adhesion (Santi et al., 2007), although this was not explicitly demonstrated in their murine model of ascending infection. GBS Alps also permit interaction with the cervical epithelium, as their binding to host α1β1 integrins or glycosaminoglycans allows cervical cell invasion in vitro (Bolduc et al., 2002; Baron et al., 2004; Bolduc and Madoff, 2007). The ability to invade cervical cells would confer protection from the extracellular host immune response. Although little is known regarding cervical immunity during GBS infection, IL-17-producing neutrophils or CD4+ T helper cells (Th17) appear to mediate GBS clearance within the murine cervix (Patras et al., 2015) (Figure 2, cervix). The roles of other immune cells at this site, including CD8+ T cells that may mediate clearance of intracellular GBS, remain undefined. As the murine cervix is small, in vivo studies of cervical immunity and infection are challenging and may be better suited for human studies or large animal models.
The Cervical Mucus Plug: A Barrier to Ascension
Whether direct interaction with the cervical epithelium is required for ascending infection or not, GBS must pass through the cervix to gain entry to the uterus. During pregnancy, a cervical mucus plug (CMP) is formed in the cervix to separate the lower genital tract from the uterus. The CMP is unique to human pregnancy and is made of viscoelastic material that serves as a physical barrier. However, varied permeability, size, and mucosal adhesion is thought to correlate with risk of uterine infections that may induce PTB (Becher et al., 2009; Smith-Dupont et al., 2017; Lacroix et al., 2020). Such changes may be induced by the dysbiotic vaginal microbiota through the secretion of bacterial vaginosis-associated mucinases (Olmsted et al., 2003; Hoang et al., 2020). In addition to serving as a physical barrier, CMPs contain a variety of antimicrobial compounds including cytokines, lactoferrin, lysozyme, and AMPs (Hein et al., 2002; Lee et al., 2011; Arko et al., 2012). Thus, the CMP may be intrinsically antimicrobial and serve as a checkpoint in preventing vaginal pathogens from ascending through the cervix to invade the uterus (Hein et al., 2001; Becher et al., 2009). One study interrogating mechanisms of protection using CMPs isolated from pregnant Danish women quantified AMPs and immune mediators present therein and found that native concentrations of AMPs (including cathelicidin, elafin, and lysozyme) in the CMP were insufficient to kill GBS in vitro (Vornhagen et al., 2018b). Despite this inadequacy, cytokines and chemokines from the CMPs could activate immune cells in the blood, thereby enabling the CMP to enhance complement-mediated pathogen killing as observed. Interestingly, CMPs collected from women who received antibiotics exhibited bactericidal effects that were lost upon treatment with penicillinases (Vornhagen et al., 2018b), suggesting that another protective mechanism of CMPs may be through antibiotic retention. While ex vivo studies of CMPs collected from women receiving antibiotics may not accurately reflect the degree of their antimicrobial nature, a combination of antimicrobial products and host factors contained in the CMPs may work in concert to synergistically inhibit bacterial ascension in vivo.
GBS Hijacks Host Epithelial-Mesenchymal Transition to Facilitate Ascension of Bacteria Into the Uterus
One host mechanism that can protect against an ascending infection into the uterus is vaginal epithelial exfoliation. This process is facilitated by epithelial-mesenchymal transition (EMT), a highly coordinated loss of epithelial cell tight junctions that leads to the detachment of apical cells, resulting in exfoliation of the epithelium. This phenomenon typically results in efflux of cells harboring pathogenic vaginal bacteria (Schleimer et al., 2007); however, GBS appears to exploit this response to facilitate ascending infection. During vaginal epithelial cell infection, GBS binds and activates α1β1 integrins, leading to a signaling cascade that displaces β-catenin upon the breakdown of adherens junctions. Once displaced, β-catenin can translocate to the nucleus and activate transcription of multiple genes associated with EMT, including SNAIL1, MYC, and AXIN2 (Vornhagen et al., 2018a). In vivo, the expression of these transcripts results in vaginal exfoliation, which typically eradicates other vaginal pathogens. Interestingly, GBS colonization of the murine vaginal tract was unaltered despite EMT-induced vaginal exfoliation, and instead this phenomenon was associated with increased ascending infection (Figure 3). Administration of recombinant α1β1 integrin to GBS-infected pregnant mice rescued this phenotype, and nearly eliminated subsequent in utero transmission to pups and placentas (Vornhagen et al., 2018a). This strategy appears, thus far, to be unique to GBS and many aspects of this mechanism remain undefined, such as the GBS factor that activates host α1β1 integrin to promote EMT. In addition, it is unclear how EMT impacts vaginal colonization of GBS in humans.
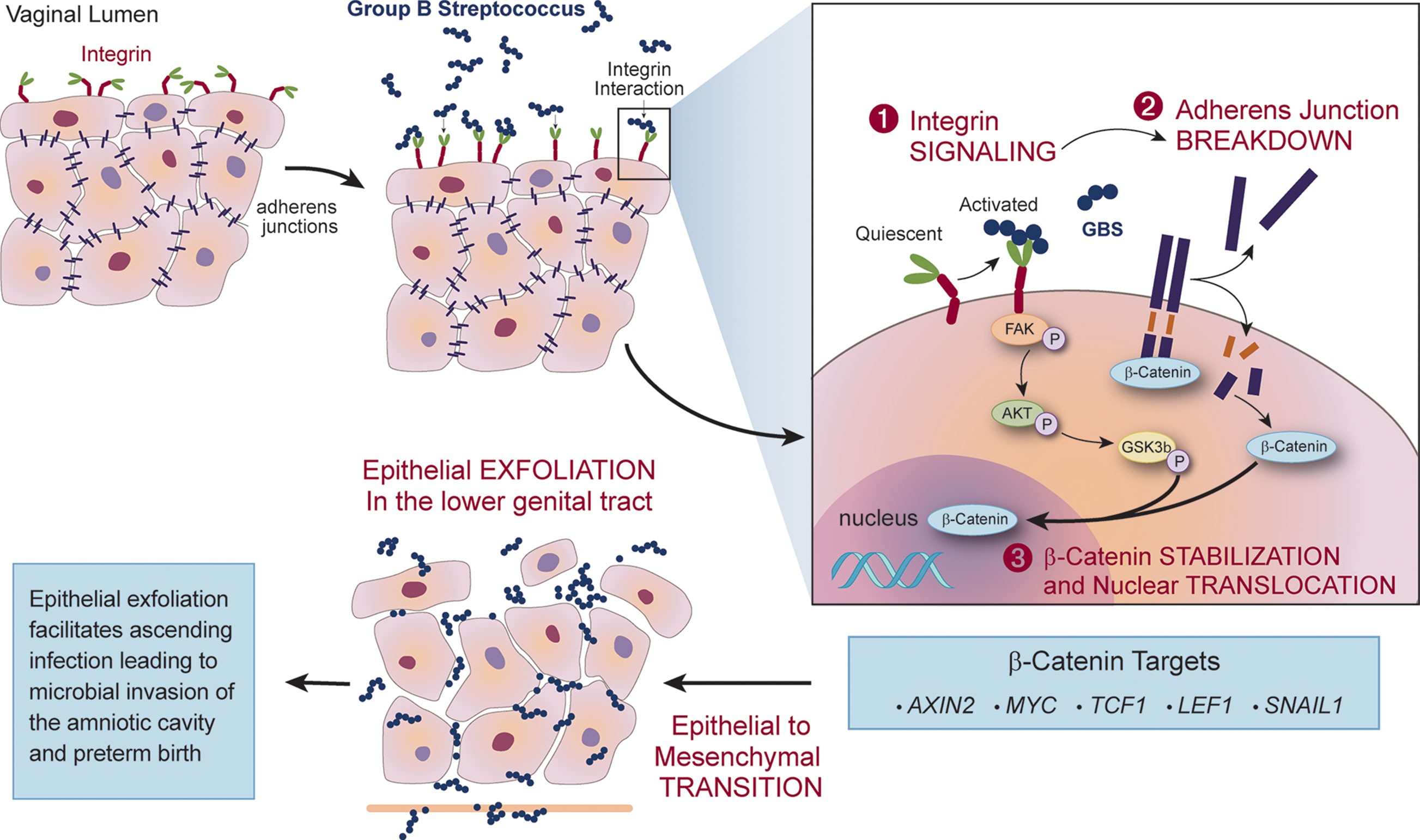
Figure 3 GBS exploits epithelial-mesenchymal transition (EMT) and vaginal epithelial exfoliation to permit ascending infection. During vaginal infection, GBS binds integrins on the epithelial surface and activates integrin signaling that results in the breakdown of adherens junctions. Displaced β-catenin translocates to the nucleus, activating transcriptional changes of β-catenin targets associated with EMT. Expression of these transcripts in vivo leads to vaginal epithelial exfoliation, which facilitates GBS ascension to the uterus and is associated with increased risk for microbial invasion of the amniotic cavity and preterm birth.
GBS Hyaluronidase Impedes Immune Detection and Aids in Ascension to the Uterus
Hyaluronan (HA) (also called hyaluronic acid) levels increase in the cervix throughout pregnancy, reaching their height during parturition. This glycosaminoglycan plays a structural role in the ECM, and plays additional roles in cell migration, adhesion, and inflammation through its digestion into fragments that interact with a diverse array of host receptors, including toll-like receptor (TLR) 2 and 4 (Dusio et al., 2011; Dicker et al., 2014; Mahendroo, 2019). HA is crucial for maintaining epithelial polarization and barrier function, and loss of HA is associated with ascending infection and subsequent infection-induced PTB (Akgul et al., 2014). Additionally, low molecular weight HA serves as a damage-associated molecular pattern (DAMP) that initiates TLR2 and 4-mediated inflammation during tissue injury (Termeer et al., 2002; Taylor et al., 2004; Scheibner et al., 2006). GBS exploits this axis through the secretion of hyaluronidase (HylB), which cleaves the pro-inflammatory HA DAMPs into disaccharides, which bind to TLR2/4 and block recognition of GBS ligands. This ultimately results in a dampened immune response (Kolar et al., 2015). Interestingly, GBS clinical strains isolated from cases of PTB or invasive neonatal infection had high HylB activity, and HylB activity was associated with ascending infection, reduced uterine inflammation, and increased rates of PTB in mice (Vornhagen et al., 2016). Although these studies partially define interactions in this immune evasion mechanism, the precise timing, immune mediators, and cell types involved remain unidentified.
Novel Prophylactics During Ascending Infection
One prophylactic strategy that might prevent ascending infection is through the inhibition of Alps-mediated cervical cell invasion. This invasion phenotype is mediated by Alps N-termini, which are partially conserved across this protein family. Alps harbor tandem repeats that are immunodominant during natural infection, but the N-terminus is highly immunogenic in the absence of these repeats (Bolduc et al., 2002; Bolduc and Madoff, 2007). A peptide derived from the fusion of αC (also called AlpC) and Rib N-termini (hereby called GBS-NN) confers mice with protection against systemic lethal challenge with αC or Rib-expressing strains (Table 1). Antibodies against GBS-NN also inhibit epithelial cell invasion (Stålhammar-Carlemalm et al., 2007). A vaccine composed of alum-adjuvanted GBS-NN in combination with GBS-NN2, a second fusion peptide derived from N-terminal domains from the other Alps, has been designed (Minervax ApS, Denmark). This vaccine is expected to protect against nearly all GBS clinical isolates since 99% of strains express at least one Alp (Gabrielsen et al., 2017). The results from a phase I randomized double-blind, placebo-controlled study of healthy, non-pregnant women are promising (NCT02459262). Nearly all participants vaccinated with GBS-NN experienced elevated titers, especially when administered as two doses adjuvanted with alum (Fischer et al., 2021). A follow-up trial similarly tested the dual vaccine, GBS-NN/NN2, in healthy non-pregnant women (NCT03807245). This vaccine will also undergo safety and immunogenicity trials in HIV-positive and -negative pregnant women, including up to a six-month follow-up period for the women and their babies following delivery (NCT04596878). This vaccine represents a novel strategy through which ascension and cervical persistence may be targeted to ultimately prevent GBS invasive disease.
Inhibiting the trafficking of GBS from the vagina into the uterus would represent a major accomplishment in preventing fetal infection and the ensuing early-onset neonatal GBS invasive disease. However, in order to inhibit ascension, many large knowledge gaps must be filled. For example, the host factors facilitating GBS trafficking into the uterus are unknown, but may include the host vaginal microbiota, nutritional status, genetics and cervical anatomy. Further, it is exceedingly difficult to identify when GBS enters the uterus in patients, particularly because GBS invasion begins asymptomatically and symptoms only manifest at the time of a fulminant infection. Although murine models for ascending infection exist, their gestational length and fetal development are vastly different compared to humans and, thus, such models are limited in their ability to identify temporal relationships with relevance to human GBS infection. A nonhuman primate with many close similarities to human pregnancy including gestation, fetal development and sensitivity to pathogens is a better suited model of studying ascending infection and for evaluation of therapeutic strategies.
GBS Vertical Transmission
Role of the Immune Cells at the Maternal-Fetal Interface in Preventing Vertical Transmission
The placenta and maternal decidua serves as a multi-layered barrier rich in immune cells that separates maternal and fetal tissues (Figure 4). The placental disc is composed of a chorionic villous tree that is immersed in maternal blood, where gas and nutrient exchange takes place. Hematogenous pathogens can invade the placenta and gain access to the fetal circulation through the placental chorionic villi. Alternatively, maternal vaginal pathogens can ascend into the uterus through the cervix where they may either encounter the maternal decidua and chorioamniotic membranes or the placenta disc, depending on placental location (which can vary). It is unusual for the placenta to cover the cervix, a condition called placenta previa, so we will consider the most typical scenario where a vaginal pathogen would encounter the maternal decidua and subsequently, the chorioamniotic membranes on its path into the amniotic fluid. Thus, the immune cell rich decidua and chorioamniotic membranes comprise a unique immune environment that can contribute to pathogen clearance or facilitate invasion depending on pathogen virulence and host response.
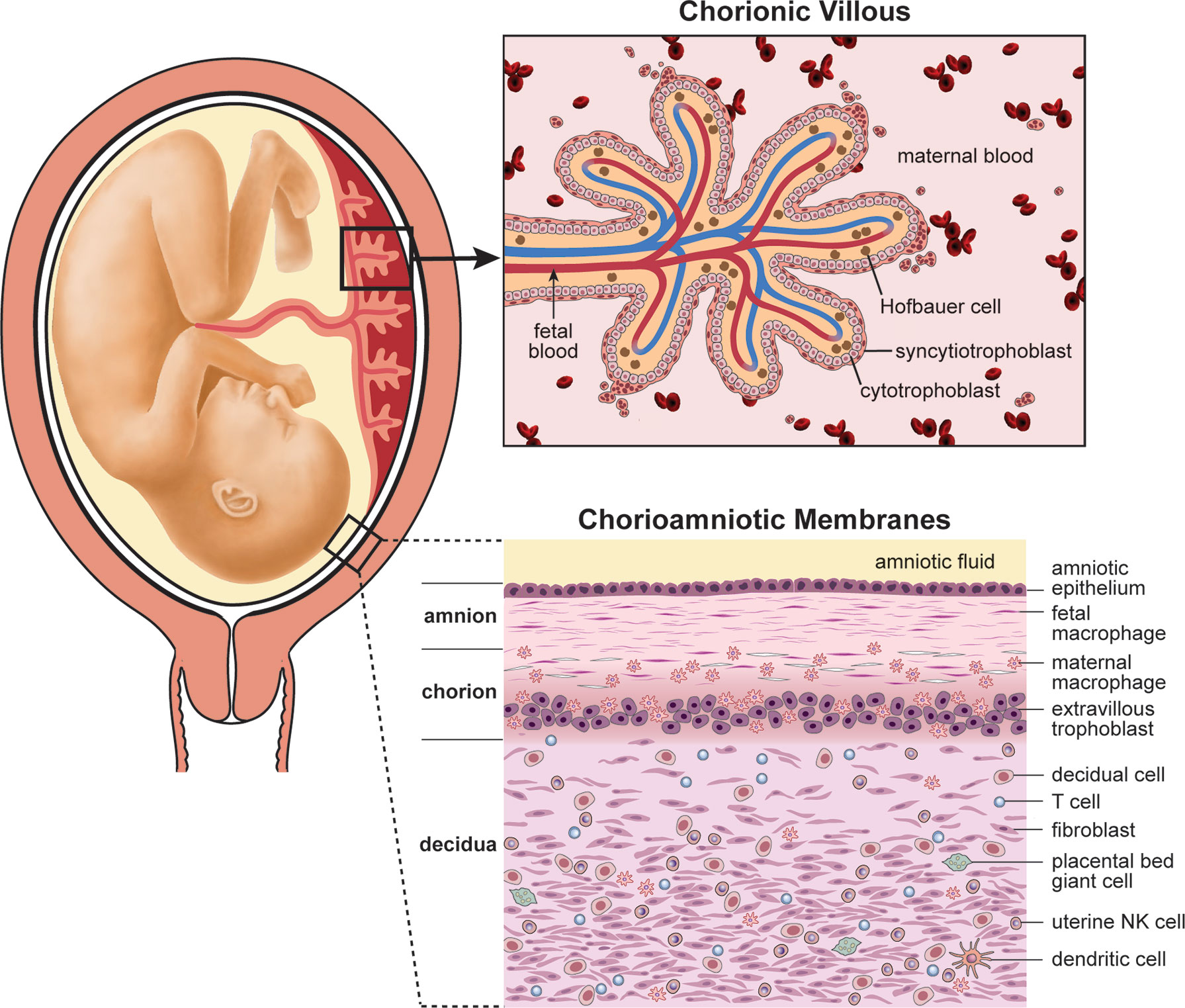
Figure 4 Human placental anatomy. This illustration depicts the components of the human placenta (chorionic villous tree), placental chorioamniotic membranes and the associated maternal decidua. GBS must traffic across either the decidua and chorioamniotic membranes or the chorionic villi to infect the fetus. GBS chorioamnionitis and culture-positive amniotic fluid is common in cases of fetal or neonatal invasive disease; thus, it is thought that GBS invasive disease occurs most commonly due to trafficking across the chorioamniotic membranes.
A variety of immune cells are present in the maternal-fetal interface, and many aspects of their function and protective mechanisms have been studied in the context of GBS infection and chorioamnionitis (Figure 2, placenta/decidua). To study immune responses in the decidua, an in vitro approach is to use decidualized immortalized endometrial stromal cells, which mimic this cell type albeit with limitations due to immortalization. Studies using these immortalized decidual cells have determined that GBS stimulation of the mitogen-activated protein kinase (MAPK) pathway leads to NF-κB activation and programmed necroptosis through c-Jun N-terminal kinase (JNK) and p38, respectively (Flaherty et al., 2019). Using the same decidualized cell line in a co-culture study with the macrophage-like cell line THP-1, another study observed that in response to GBS, decidual stromal cells produce prostaglandin E2 (PGE2) that alters the THP-1-mediated immune response through paracrine signaling. Specifically, PGE2 enhanced inflammation, but inhibited THP-1 production of TNF-α and reduced phagocytosis of GBS. Conditioned medium from ex vivo cultured human placental membranes induced a similar effect on GBS-stimulated THP-1 cells. Mice with GBS chorioamnionitis exhibited macrophage infiltration and increased PGE2 (Rogers et al., 2018). Further support for paracrine signaling as a mechanism of placental immune regulation, IL-1 derived from the choriodecidua is needed for amniotic release of human beta defensin-1 during extraplacental membrane infection by GBS (Boldenow et al., 2015). Thus, host-directed therapies may be effective through direct targeting of cells that contribute to GBS infection, as well as through paracrine signaling that affects bystander cells that modulate immune responses.
Primary placental cells are perhaps an excellent model to study GBS host-pathogen interactions in the placenta. A study using primary human fetal macrophages isolated from the chorionic villous tree observed that protein kinase D signaling was critical for the GBS-induced production of inflammatory cytokines, NLRP3 inflammasome activation, and activation of NF-κB (Sutton et al., 2019). This pathway represents another host response that might be targeted therapeutically, although it is important to note that these downstream phenotypes likely contribute to both GBS clearance and immunopathology. A limitation of using primary placental immune cells is that they are unlikely to model conditions that are relevant in vivo. For example, when grown in isolation primary macrophages do not receive the immunologic cross-talk from stromal cells to exert paracrine effects. Thus, ex vivo analyses of placental immunity should seek to incorporate other cell types and signals encountered in vivo. One interesting approach, albeit engineered, is the novel “fetal membrane on a chip,” a system that can be easily modified to incorporate stimuli such as GBS, cytokines, hormones, prostaglandins, nutrients, or inhibitors; effluent samples can be collected for downstream analyses including Luminex or mass spectrometry (Aronoff, 2020). Overall, primary placental cells serve as an interesting model of host-pathogen interactions, but benefit from the addition of paracrine effects and novel approaches to model the anatomical complexity of the placenta.
Although in vitro technologies are improving, the best way to study immune responses is in a host. Thus, animal models of pregnancy-associated GBS infection and GBS chorioamnionitis are essential to inform placental immunity. In a murine model of GBS vaginal inoculation during pregnancy, neutrophil infiltrates were observed in the choriodecidua and placenta; further, these neutrophils produced neutrophil extracellular traps (NETs) that immobilized GBS and repressed GBS growth via lactoferrin-mediated iron sequestration (Kothary et al., 2017). Another group noted NET production in non-pregnant mice inoculated vaginally with GBS, which was dependent on GBS hemolysin (Carey et al., 2014), suggesting that GBS virulence factors may mediate this neutrophil response. However, hyperhemolytic GBS can evade NETs via direct neutrophil killing (Boldenow et al., 2016). Interestingly, production of extracellular traps is not a mechanism unique to neutrophils; primary placental macrophages release similar macrophage extracellular traps (METs) during GBS infection ex vivo. METs were produced in an ROS-dependent manner, contained a variety of matrix metalloproteases and were observed in the fetal chorioamniotic membranes (Doster et al., 2018). The production of these extracellular traps may adversely affect pregnancy outcome, as the production of matrix metalloproteases and neutrophil elastase can stimulate uterine contractions and are associated with chorioamnionitis and PTB (Sundrani et al., 2012; Tambor et al., 2013; Walsh et al., 2018). Animal models of GBS infection in pregnancy are key for elucidating complex host-pathogen responses that begin with vaginal inoculation and result in invasion of the amniotic cavity and fetus.
GBS Trafficking Into the Amniotic Cavity
After crossing the maternal decidua and chorioamniotic membranes, GBS must traffic into the amniotic fluid and adapt to a new niche in order to subsequently infect the fetus. When GBS was grown in amniotic fluid, transcriptional changes altering metabolism of amino acids and carbohydrates was observed, which is likely related to the nutritional requirements of GBS in amniotic fluid. Further, growth in amniotic fluid also resulted in differentially expression in virulence-associated genes. Adhesins were downregulated, whereas, capsule, hemolysin, and IL-8 proteinase were upregulated, suggesting that GBS modulates its virulence factors in response to amniotic fluid (Sitkiewicz et al., 2009). Expression of the fru2 metabolic operon which encodes factors involved in fructose metabolism (Patron et al., 2017) was induced, suggesting that this carbon source might be important for GBS survival in this niche. Although these studies highlight adaptations that GBS might undergo following microbial invasion of the amniotic cavity, the relevance of these findings in vivo are unclear. Recently, a transposon screen identified a novel GBS transcription factor, MrvR, that was important for survival in amniotic fluid. MrvR; deletion of mrvR reduced GBS survival in amniotic fluid and impaired biofilm formation. Pregnant mice vaginally-infected by MrvR-null GBS exhibited normal vaginal persistence and consistent ascending infection, but these mice did not experience PTB (Dammann et al., 2021), suggesting that MrvR may mainly promote bacterial survival in vivo.
Once GBS has invaded the amniotic cavity, immune recognition and inflammatory mediators contribute to the development of adverse pregnancy outcomes. In a nonhuman primate model, inoculation of GBS into the amniotic fluid increased amniotic fluid IL-1β, TNF-α, IL-6, and IL-8 cytokine/chemokine levels (Gravett et al., 1994). When these individual cytokines were inoculated into the amniotic fluid of a rhesus macaque model to interrogate the effects of each individual cytokine, only the nonhuman primates inoculated with IL-1β or TNF-α developed preterm labor (Sadowsky et al., 2006). These observations suggest that certain inflammatory cytokines are sufficient to induce preterm labor and PTB. Prostaglandins, which increase cervical softening, are also observed to increase in the amniotic fluid during GBS invasion of the chorioamniotic membranes and amniotic cavity (Boldenow et al., 2016; Coleman et al., 2021). A recent study found that higher amniotic fluid IL-6 and IL-8 concentrations are also useful predictors of fetal lung injury in a nonhuman primate model of GBS chorioamnionitis (McCartney et al., 2021). Thus, amniotic fluid cytokines and chemokines play a role in the development of adverse maternal and fetal outcomes during GBS infection.
Finally, recent studies have begun to evaluate the roles of immune cells in the amniotic fluid on bacterial clearance. Human amniotic fluid neutrophils actively phagocytose GBS ex vivo in a manner similar to what has been observed by neutrophils in the blood (Gomez-Lopez et al., 2017). This represents one mechanism that may contribute to reduction of GBS burden in the amniotic cavity, although this has not been established in vivo. Other phagocytes such as macrophages may also mediate amniotic fluid clearance, although this has not been examined. Further, immune cell subsets that contribute to pro-inflammatory cytokine responses associated with adverse outcomes remain undefined.
GBS Virulence Factors Modulate Crucial Balance Between Chorioamnionitis and Microbial Invasion of the Amniotic Cavity
GBS can modulate host immune responses at the maternal-fetal interface to promote chorioamnionitis and microbial invasion of the amniotic cavity. An important virulence factor GBS employs to gain access to the amniotic cavity through invasion of the chorioamniotic membranes is hemolysin. In fact, GBS clinical strains isolated from the chorioamniotic membranes or amniotic fluid of women in preterm labor from a small case series exhibited a hyperhemolytic phenotype. GBS hemolysin induces the release of proinflammatory cytokines, such as IL-6, IL-8, IL-1β, and TNF-α in chorioamniotic membranes, culminating in barrier disruption (Whidbey et al., 2013). The cytotoxic activity of hemolysin is driven through intercalation of the toxin within the plasma membrane, leading to bilayer disruption followed by rapid efflux of potassium ions. In human macrophages, hemolysin-mediated potassium ion efflux triggers NLRP3 inflammasome formation and activates caspase 1 signaling, ultimately causing cell death by pyroptosis (Whidbey et al., 2015). Although caspase 1 activation can promote GBS clearance (Costa et al., 2012), the induction of robust inflammatory responses is likely detrimental during pregnancy, as the host must balance inflammation during infection to protect the mother and immunoregulatory responses to prevent preterm labor (Whidbey et al., 2015). Notably, in a pregnant murine model, hyperhemolytic GBS promoted preterm labor and significantly increased the rate of fetal death compared to an isogenic nonhemolytic strain. Intriguingly, NLRP3 inflammasome activation exacerbated GBS-mediated fetal injury. However, other host factors may also contribute to these pregnancy complications, as fetal death was still observed in NLRP3-deficient mice, albeit at lower levels than wildtype mice (Whidbey et al., 2015). In pregnant nonhuman primates that were choriodecidually infected with hyperhemolytic COH1ΔcovR, GBS trafficked across the chorioamniotic membranes to invade the amniotic fluid and fetus more frequently than with a non-hemolytic GBS strain (COH1ΔcovRΔcylE) (Boldenow et al., 2016). Microbial invasion of the amniotic cavity was associated with robust neutrophilic infiltration of the chorioamniotic membranes and production of neutrophil extracellular traps and proinflammatory cytokines. The hyperhemolytic GBS strain subverted all of these host innate immune responses to infect the amniotic fluid and ultimately lead to adverse outcomes including fetal sepsis and PTB (Boldenow et al., 2016).
In contrast to the robust proinflammatory response to hemolysin, GBS can also dampen immune responses at the maternal-fetal interface through the action of HylB, which interferes with TLR2/4-mediated immune signaling (Kolar et al., 2015). Similar to COH1ΔcovR-infected animals, pregnant nonhuman primates choriodecidually infected with GB37, a GBS strain with high HylB activity, consistently experienced preterm labor, microbial invasion of the amniotic cavity and fetal sepsis (Coleman et al., 2021). In these experiments, neutrophils also infiltrated the chorioamniotic membranes, but were not successful in containing the infection and preventing microbial invasion of the amniotic cavity. Ex vivo experiments using paired maternal and neonatal cord blood indicate that HylB-mediated TLR2/4 dampening resulting in reduced production of antimicrobial reactive oxygen species (Figure 2, maternal/cord blood) (Coleman et al., 2021). Finally, digital spatial profiling of GBS-infected choriomaniotic membranes highlighted HylB-specific dampening of inflammatory responses (Coleman et al., 2021). This blunting of innate immune responses observed in GB37-infected animals is directly in contrast to the rapid proinflammatory response observed with the hyperhemolytic GBS (Boldenow et al., 2016). These studies highlight key virulence factor-mediated differences between two GBS strains that induce similar adverse outcomes in pregnant nonhuman primates, and emphasizes the diverse nature of GBS virulence factors.
Placental Chorioamniotic Weakening After GBS Infection
GBS infection and inflammation of the amniotic fluid can contribute to preterm premature rupture of membranes (pPROM) through weakening of the placental chorioamniotic membranes, ultimately inducing PTB. Multiple factors contribute to membrane weakening, including matrix metalloproteinases, apoptosis, oxidative stress, and other neutrophil proteases (Lannon et al., 2014; Romero et al., 2014). An important mechanism contributing to pPROM was demonstrated using a nonhuman primate model of GBS is EMT (Weed et al., 2020). EMT is a coordinated process whereby epithelial cells change morphology, retract their cytoskeleton, lose cell-cell adhesions and become motile; overall, this process reduces tissue integrity. In a nonhuman primate model, a choriodecidual inoculation of GBS was associated with transcriptional responses in the chorioamniotic membranes characterized by downregulation of cytostructural genes and microRNA gene expression (i.e. miR-200b, miR-203-5p) consistent with EMT (Vanderhoeven et al., 2014). Immunohistochemistry of the chorioamniotic membranes also demonstrated partial loss of surface E-cadherin, increased N-cadherin expression, and vimentin staining near areas of neutrophil influx, all signs of reduced amniotic epithelial integrity (Weed et al., 2020). Thus, in addition to the role of EMT in vaginal exfoliation, EMT may also impair chorioamniotic membrane tissue integrity and predispose GBS-infected pregnant women to pPROM.
Conclusion
In summary, GBS inflicts an annual public health burden on pregnant women and neonates, which is disproportionately concentrated in low-income countries. National and region-specific discrepancies between screening guidelines and the use of IAP confounds the accurate detection of invasive GBS disease cases, limiting the ability to control the associated adverse outcomes through intervention. Although a combination of a global universal screening approach and IAP use would result in the greatest reduction in maternal, fetal, and neonatal adverse outcomes, this is not realistic for many low-income countries that lack resources to implement these approaches; further, some higher income countries have a low prevalence of invasive GBS disease, leading them to instead adopt a more cost-effective risk-based approach to treating GBS in pregnancy. The solution to reducing invasive GBS disease in pregnant women and neonates is not simple, but the development of a GBS vaccine would be a major step forward in preventing disease.
This review highlights recent discoveries that elucidate the interplay between GBS and the host, defining the mechanisms that GBS employs to successfully disseminate to vulnerable host niches during pregnancy. In recent years, major strides have been made to improve our understanding of GBS vaginal colonization, ascending infection, and congenital infection, ultimately facilitating the strategic development of several candidate vaccines with demonstrated efficacy in animal models or human clinical trials. Although it is too early to forecast whether these vaccines will someday receive FDA-approval, their success symbolizes a major step towards reducing the global burden of GBS invasive disease.
Author Contributions
All authors contributed to the article and approved the submitted version.
Funding
This work was supported by funding from the National Institutes of Health grants R01AI133976, R01AI145890, R01HD098713 to LR and KW, R01AI152268 to LR., T32AI055396 to AF (PI: Fang), T32AI007509 to AB (PI: Campbell), and seed funds from Seattle Children’s Research Institute to L.R. This work was also supported by the P51OD010425, which is the core grant of the Washington National Primate Research Center. Additional support came from a U42OD011123, which funds the breeding colony at the Washington National Primate Research Center.
Conflict of Interest
The authors declare that the research was conducted in the absence of any commercial or financial relationships that could be construed as a potential conflict of interest.
Publisher’s Note
All claims expressed in this article are solely those of the authors and do not necessarily represent those of their affiliated organizations, or those of the publisher, the editors and the reviewers. Any product that may be evaluated in this article, or claim that may be made by its manufacturer, is not guaranteed or endorsed by the publisher.
Acknowledgments
The authors acknowledge Jessie Brown for graphic design. BioRender.com was used to create most elements of Figure 2.
References
Absalon, J., Segall, N., Block, S. L., Center, K. J., Scully, I. L., Giardina, P. C., et al. (2021). Safety and Immunogenicity of a Novel Hexavalent Group B Streptococcus Conjugate Vaccine in Healthy, Non-Pregnant Adults: A Phase 1/2, Randomised, Placebo-Controlled, Observer-Blinded, Dose-Escalation Trial. Lancet Infect. Dis. 21, 263–274. doi: 10.1016/S1473-3099(20)30478-3
Akgul, Y., Word, R. A., Ensign, L. M., Yamaguchi, Y., Lydon, J., Hanes, J., et al. (2014). Hyaluronan in Cervical Epithelia Protects Against Infection-Mediated Preterm Birth. J. Clin. Invest. 124, 5481–5489. doi: 10.1172/JCI78765
Altoparlak, U., Kadanali, A., Kadanali, S. (2004). Genital Flora in Pregnancy and Its Association With Group B Streptococcal Colonization. Int. J. Gynecol. Obstet. 87, 245–246. doi: 10.1016/j.ijgo.2004.08.006
Arko, D., Dovnik, A., Fokter, N., Takač, I. (2012). The Role of Genital Pathogens in Morbidity Following Diathermy Loop Excision of the Transformation Zone of the Uterine Cervix. Int. J. Gynecol. Obstet. 117, 27–29. doi: 10.1016/j.ijgo.2011.11.015
Armistead, B., Herrero-Foncubierta, P., Coleman, M., Quach, P., Whidbey, C., Justicia, J., et al. (2020a). Lipid Analogs Reveal Features Critical for Hemolysis and Diminish Granadaene Mediated Group B Streptococcus Infection. Nat. Commun. 11 (1), 502. doi: 10.1038/s41467-020-15282-0
Armistead, B., Quach, P., Snyder, J. M., Santana-Ufret, V., Furuta, A., Brokaw, A., et al. (2020b). Hemolytic Membrane Vesicles of Group B Streptococcus Promote Infection. J. Infect. Dis. 223 (8), 1488–1496. doi: 10.1093/infdis/jiaa548
Armistead, B., Whidbey, C., Iyer, L. M., Herrero-Foncubierta, P., Quach, P., Haidour, A., et al. (2019). The Cyl Genes Reveal the Biosynthetic and Evolutionary Origins of the Group B Streptococcus Hemolytic Lipid, Granadaene. Front. Microbiol. 10, 3123. doi: 10.3389/fmicb.2019.03123
Aronoff, D. M. (2020). Deconstructing Extraplacental Membranes to Understand Bacterial Chorioamnionitis. Trans. Am. Clin. Climatol. Assoc. 131, 72–79.
Baker, J. A., Lewis, E. L., Byland, L. M., Bonakdar, M., Randis, T. M., Ratner, A. J. (2017). Mucosal Vaccination Promotes Clearance of Streptococcus Agalactiae Vaginal Colonization. Vaccine 35, 1273–1280. doi: 10.1016/j.vaccine.2017.01.029
Baron, M. J., Bolduc, G. R., Goldberg, M. B., Aupérin, T. C., Madoff, L. C. (2004). Alpha C Protein of Group B Streptococcus Binds Host Cell Surface Glycosaminoglycan and Enters Cells by an Actin-Dependent Mechanism. J. Biol. Chem. 279, 24714–24723. doi: 10.1074/jbc.M402164200
Becher, N., Waldorf, K. A., Hein, M., Uldbjerg, N. (2009). The Cervical Mucus Plug: Structured Review of the Literature. Acta Obstet. Gynecol. Scand. 88, 502–513. doi: 10.1080/00016340902852898
Bellais, S., Six, A., Fouet, A., Longo, M., Dmytruk, N., Glaser, P., et al. (2012). Capsular Switching in Group B Streptococcus CC17 Hypervirulent Clone: A Future Challenge for Polysaccharide Vaccine Development. J. Infect. Dis. 206, 1745–1752. doi: 10.1093/infdis/jis605
Bianchi-Jassir, F., Paul, P., To, K. N., Carreras-Abad, C., Seale, A. C., Jauneikaite, E., et al. (2020). Systematic Review of Group B Streptococcal Capsular Types, Sequence Types and Surface Proteins as Potential Vaccine Candidates. Vaccine 38, 6682–6694. doi: 10.1016/j.vaccine.2020.08.052
Bianchi-Jassir, F., Seale, A. C., Kohli-Lynch, M., Lawn, J. E., Baker, C. J., Bartlett, L., et al. (2017). Preterm Birth Associated With Group B Streptococcus Maternal Colonization Worldwide: Systematic Review and Meta-Analyses. Clin. Infect. Dis. 65, S133–S142. doi: 10.1093/cid/cix661
Bisharat, N., Crook, D. W., Leigh, J., Harding, R. M., Ward, P. N., Coffey, T. J., et al. (2004). Hyperinvasive Neonatal Group B Streptococcus Has Arisen From a Bovine Ancestor. J. Clin. Microbiol. 42, 2161–2167. doi: 10.1128/JCM.42.5.2161-2167.2004
Bodaszewska, M., Brzychczy-Włoch, M., Gosiewski, T., Adamski, P., Strus, M., Heczko, P. B. (2010). Evaluation of Group B Streptococcus Susceptibility to Lactic Acid Bacteria Strains. Med. Dosw. Mikrobiol. 62, 153–161.
Boldenow, E., Hogan, K. A., Chames, M. C., Aronoff, D. M., Xi, C., Loch-Caruso, R. (2015). Role of Cytokine Signaling in Group B Streptococcus-Stimulated Expression of Human Beta Defensin-2 in Human Extraplacental Membranes. Am. J. Reprod. Immunol. 73, 263–272. doi: 10.1111/aji.12325
Boldenow, E., Gendrin, C., Ngo, L., Bierle, C., Vornhagen, J., Coleman, M., et al. (2016). Group B Streptococcus Circumvents Neutrophils and Neutrophil Extracellular Traps During Amniotic Cavity Invasion and Preterm Labor. Sci. Immunol. 1 (4), eaah4576. doi: 10.1126/sciimmunol.aah4576
Bolduc, G. R., Baron, M. J., Gravekamp, C., Lachenauer, C. S., Madoff, L. C. (2002). The Alpha C Protein Mediates Internalization of Group B Streptococcus Within Human Cervical Epithelial Cells. Cell. Microbiol. 4, 751–758. doi: 10.1046/j.1462-5822.2002.00227.x
Bolduc, G. R., Madoff, L. C. (2007). The Group B Streptococcal Alpha C Protein Binds α1β1-Integrin Through a Novel KTD Motif That Promotes Internalization of GBS Within Human Epithelial Cells. Microbiology 153, 4039–4049. doi: 10.1099/mic.0.2007/009134-0
Boskey, E. R., Cone, R. A., Whaley, K. J., Moench, T. R. (2001). Origins of Vaginal Acidity: High D/L Lactate Ratio Is Consistent With Bacteria Being the Primary Source. Hum. Reprod. 16, 1809–1813. doi: 10.1093/humrep/16.9.1809
Brotman, R. M., Shardell, M. D., Gajer, P., Fadrosh, D., Chang, K., Silver, M. I., et al. (2018). Association Between the Vaginal Microbiota, Menopause Status, and Signs of Vulvovaginal Atrophy. Menopause 25, 1321–1330. doi: 10.1097/gme.0b013e3182a4690b
Brzychczy-Wloch, M., Pabian, W., Majewska, E., Zuk, M., Kielbik, J., Gosiewski, T., et al. (2014). Dynamics of Colonization With Group B Streptococci in Relation to Normal Flora in Women During Subsequent Trimesters of Pregnancy. New Microbiol. 37, 307–319.
Buscetta, M., Papasergi, S., Firon, A., Pietrocola, G., Biondo, C., Mancuso, G., et al. (2014). FbsC, A Novel Fibrinogen-Binding Protein, Promotes Streptococcus Agalactiae-Host Cell Interactions. J. Biol. Chem. 289, 21003–21015. doi: 10.1074/jbc.M114.553073
Buurman, E. T., Timofeyeva, Y., Gu, J., Kim, J. H., Kodali, S., Liu, Y., et al. (2019). A Novel Hexavalent Capsular Polysaccharide Conjugate Vaccine (GBS6) for the Prevention of Neonatal Group B Streptococcal Infections by Maternal Immunization. J. Infect. Dis. 220, 105–115. doi: 10.1093/infdis/jiz062
Campisi, E., Rosini, R., Romano, M. R., Balducci, E., Pinto, V., Brogioni, B., et al. (2021). Group B Streptococcus Chimeric Capsular Polysaccharides as Novel Multivalent Vaccine Candidates. Glycoconj. J. 38 (4), 447–457. doi: 10.1007/s10719-021-10000-4
Carboni, F., Adamo, R., Fabbrini, M., De Ricco, R., Cattaneo, V., Brogioni, B., et al. (2017). Structure of a Protective Epitope of Group B Streptococcus Type III Capsular Polysaccharide. Proc. Natl. Acad. Sci. U. S. A. 114, 5017–5022. doi: 10.1073/pnas.1701885114
Carboni, F., Angiolini, F., Fabbrini, M., Brogioni, B., Corrado, A., Berti, F., et al. (2020). Evaluation of Immune Responses to Group B Streptococcus Type III Oligosaccharides Containing a Minimal Protective Epitope. J. Infect. Dis. 221, 943–947. doi: 10.1093/infdis/jiz551
Carey, A. J., Tan, C. K., Mirza, S., Irving-Rodgers, H., Webb, R. I., Lam, A., et al. (2014). Infection and Cellular Defense Dynamics in a Novel 17β-Estradiol Murine Model of Chronic Human Group B Streptococcus Genital Tract Colonization Reveal a Role for Hemolysin in Persistence and Neutrophil Accumulation. J. Immunol. 192, 1718–1731. doi: 10.4049/jimmunol.1202811
Chen, S. L. (2019). Genomic Insights Into the Distribution and Evolution of Group B Streptococcus. Front. Microbiol. 10, 1447. doi: 10.3389/fmicb.2019.01447
Chiarot, E., Spagnuolo, A., Maccari, S., Naimo, E., Acquaviva, A., Cecchi, R., et al. (2018). Protective Effect of Group B Streptococcus Type-III Polysaccharide Conjugates Against Maternal Colonization, Ascending Infection and Neonatal Transmission in Rodent Models. Sci. Rep. 8 (1), 2593. doi: 10.1038/s41598-018-20609-5
Coleman, M., Armistead, B., Orvis, A., Quach, P., Brokaw, A., Gendrin, C., et al. (2021). Hyaluronidase Impairs Neutrophil Function and Promotes Group B Streptococcus Invasion and Preterm Labor in Nonhuman Primates. MBio 12, 1–18. doi: 10.1128/mBio.03115-20
Cook, L. C. C., Hu, H., Maienschein-Cline, M., Federle, M. J. (2018). A Vaginal Tract Signal Detected by the Group B Streptococcus Saers System Elicits Transcriptomic Changes and Enhances Murine Colonization. Infect. Immun. 86 (4), e00762–17. doi: 10.1128/IAI.00762-17
Cools, P., Jespers, V., Hardy, L., Crucitti, T., Delany-Moretlwe, S., Mwaura, M., et al. (2016). A Multi-Country Cross-Sectional Study of Vaginal Carriage of Group B Streptococci (GBS) and Escherichia Coli in Resource-Poor Settings: Prevalences and Risk Factors. PloS One 11 (1), e0148052. doi: 10.1371/journal.pone.0148052
Costa, A., Gupta, R., Signorino, G., Malara, A., Cardile, F., Biondo, C., et al. (2012). Activation of the NLRP3 Inflammasome by Group B Streptococci. J. Immunol. 188, 1953–1960. doi: 10.4049/jimmunol.1102543
Dammann, A. N., Chamby, A. B., Catomeris, A. J., Davidson, K. M., Tettelin, H., Pijkeren, J. P. V., et al. (2021). Genome-Wide Fitness Analysis of Group B Streptococcus in Human Amniotic Fluid Reveals a Transcription Factor That Controls Multiple Virulence Traits. PloS Pathog. 17 (3), e1009116. doi: 10.1371/journal.ppat.1009116
Daniel Johannes Rönnqvist, P., Birgitta Forsgren-Brusk, U., Elisabeth Grahn-Håkansson, E. (2006). Lactobacilli in the Female Genital Tract in Relation to Other Genital Microbes and Vaginal pH. Acta Obstet. Gynecol. Scand. 85, 726–735. doi: 10.1080/00016340600578357
De Gregorio, P. R., Juárez Tomás, M. S., Leccese Terraf, M. C., Nader-Macías, M. E. F. (2015). Preventive Effect of Lactobacillus Reuteri CRL1324 on Group B Streptococcus Vaginal Colonization in an Experimental Mouse Model. J. Appl. Microbiol. 118, 1034–1047. doi: 10.1111/jam.12739
De Gregorio, P. R., Juárez Tomás, M. S., Nader-Macías, M. E. F. (2016). Immunomodulation of Lactobacillus Reuteri CRL1324 on Group B Streptococcus Vaginal Colonization in a Murine Experimental Model. Am. J. Reprod. Immunol. 75, 23–35. doi: 10.1111/aji.12445
Diaz-Dinamarca, D. A., Hernandez, C., Escobar, D. F., Soto, D. A., Muñoz, G. A., Badilla, J. F., et al. (2020). Mucosal Vaccination With Lactococcus Lactis-Secreting Surface Immunological Protein Induces Humoral and Cellular Immune Protection Against Group B Streptococcus in a Murine Model. Vaccines 8, 146. doi: 10.3390/vaccines8020146
Dicker, K. T., Gurski, L. A., Pradhan-Bhatt, S., Witt, R. L., Farach-Carson, M. C., Jia, X. (2014). Hyaluronan: A Simple Polysaccharide With Diverse Biological Functions. Acta Biomater. 10, 1558–1570. doi: 10.1016/j.actbio.2013.12.019
Doran, K. S., Chang, J. C. W., Benoit, V. M., Eckmann, L., Nizet, V. (2002). Group B Streptococcal β-Hemolysin/Cytolysin Promotes Invasion of Human Lung Epithelial Cells and the Release of Interleukin-8. J. Infect. Dis. 185, 196–203. doi: 10.1086/338475
Doran, K. S., Liu, G. Y., Nizet, V. (2003). Group B Streptococcal β-Hemolysin/Cytolysin Activates Neutrophil Signaling Pathways in Brain Endothelium and Contributes to Development of Meningitis. J. Clin. Invest. 112, 736–744. doi: 10.1172/jci17335
dos Santos, N. F. B., da Silva, L. R., Costa, F. J. M. D., de Mattos, D. M., de Carvalho, E., Ferreira, L. C., et al. (2020). Immunization With a Recombinant BibA Surface Protein Confers Immunity and Protects Mice Against Group B Streptococcus (GBS) Vaginal Colonization. Vaccine 38, 5286–5296. doi: 10.1016/j.vaccine.2020.05.076
Doster, R. S., Sutton, J. A., Rogers, L. M., Aronoff, D. M., Gaddy, J. A. (2018). Streptococcus Agalactiae Induces Placental Macrophages to Release Extracellular Traps Loaded With Tissue Remodeling Enzymes via an Oxidative Burst-Dependent Mechanism. MBio 9 (6), e02084–18. doi: 10.1128/mBio.02084-18
Dusio, G. F., Cardani, D., Zanobbio, L., Mantovani, M., Luchini, P., Battini, L., et al. (2011). Stimulation of TLRs by LMW-HA Induces Self-Defense Mechanisms in Vaginal Epithelium. Immunol. Cell Biol. 89, 630–639. doi: 10.1038/icb.2010.140
Evans, J. J., Klesius, P. H., Pasnik, D. J., Bohnsack, J. F. (2009). Human Streptococcus Agalactiae Isolate in Nile Tilapia (Oreochromis Niloticus). Emerg. Infect. Dis. 15, 774–776. doi: 10.3201/eid1505.080222
Farr, A., Sustr, V., Kiss, H., Rosicky, I., Graf, A., Makristathis, A., et al. (2020). Oral Probiotics to Reduce Vaginal Group B Streptococcal Colonization in Late Pregnancy. Sci. Rep. 10 (1), 19745. doi: 10.1038/s41598-020-76896-4
Flaherty, R. A., Magel, M., Aronoff, D. M., Gaddy, J. A., Petroff, M. G., Manning, S. D. (2019). Modulation of Death and Inflammatory Signaling in Decidual Stromal Cells Following Exposure to Group B Streptococcus. Infect. Immun. 87 (12), e00729–19. doi: 10.1128/IAI.00729-19
Fischer, P., Pawlowsk, i. A., Cao, D., Bell, D., Kitson, G., Darsley, M., et al. (2021). Safety and Immunogenicity of a Prototype Recombinant Alpha-Like Protein Subunit Vaccine (GBS-NN) Against Group B Streptococcus in a Randomised Placebo-Controlled Double-Blind Phase 1 Trial in Healthy Adult Women. Vaccine 39, 4489–4499. doi: 10.1016/J.VACCINE.2021.06.046
Francois Watkins, L. K., McGee, L., Schrag, S. J., Beall, B., Jain, J. H., Pondo, T., et al. (2019). Epidemiology of Invasive Group B Streptococcal Infections Among Nonpregnant Adults in the United State-2016. JAMA Intern. Med. 179, 479–488. doi: 10.1001/jamainternmed.2018.7269
Gabrielsen, C., Mæland, J. A., Lyng, R. V., Radtke, A., Afset, J. E. (2017). Molecular Characteristics of Streptococcus Agalactiae Strains Deficient in Alpha-Like Protein Encoding Genes. J. Med. Microbiol. 66, 26–33. doi: 10.1099/JMM.0.000412
Gajer, P., Brotman, R. M., Bai, G., Sakamoto, J., Schütte, U. M. E., Zhong, X., et al. (2012). Temporal Dynamics of the Human Vaginal Microbiota. Sci. Transl. Med. 4 (132), 132ra52. doi: 10.1126/scitranslmed.3003605
Gendrin, C., Vornhagen, J., Ngo, L., Whidbey, C., Boldenow, E., Santana-Ufret, V., et al. (2015). Mast Cell Degranulation by a Hemolytic Lipid Toxin Decreases GBS Colonization and Infection. Sci. Adv. 1 (6), e1400225. doi: 10.1126/sciadv.1400225
Gibson, R. L., Nizet, V., Rubens, C. E., McMenamin, P. (1999). Group B Streptococcal β-Hemolysin Promotes Injury of Lung Microvascular Endothelial Cells. Pediatr. Res. 45, 626–634. doi: 10.1203/00006450-199905010-00003
Gilbert, N. M., Foster, L. R., Cao, B., Yin, Y., Mysorekar, I. U., Lewis, A. L. (2021). Gardnerella Vaginalis Promotes Group B Streptococcus Vaginal Colonization, Enabling Ascending Uteroplacental Infection in Pregnant Mice. Am. J. Obstetrics Gynecol. (Mosby Inc.) 224 (5), 530.e1–530.e17. doi: 10.1016/j.ajog.2020.11.032
Gomez-Lopez, N., Romero, R., Garcia-Flores, V., Xu, Y., Leng, Y., Alhousseini, A., et al. (2017). Amniotic Fluid Neutrophils can Phagocytize Bacteria: A Mechanism for Microbial Killing in the Amniotic Cavity. Am. J. Reprod. Immunol. 78 (4), 10.1111/aji.12723. doi: 10.1111/aji.12723
Gravett, M. G., Witkin, S. S., Haluska, G. J., Edwards, J. E. L., Cook, M. J., Novy, M. J. (1994). An Experimental Model for Intraamniotic Infection and Preterm Labor in Rhesus Monkeys. Am. J. Obstet. Gynecol. 171, 1660–1667. doi: 10.1016/0002-9378(94)90418-9
Hall, J., Adams, N. H., Bartlett, L., Seale, A. C., Lamagni, T., Bianchi-Jassir, F., et al. (2017). Maternal Disease With Group B Streptococcus and Serotype Distribution Worldwide: Systematic Review and Meta-Analyses. Clin. Infect. Dis. 65, S112–S124. doi: 10.1093/cid/cix660
Hays, C., Louis, M., Plainvert, C., Dmytruk, N., Touak, G., Trieu-Cuot, P., et al. (2016). Changing Epidemiology of Group B Streptococcus Susceptibility to Fluoroquinolones and Aminoglycosides in France. Antimicrob. Agents Chemother. 60, 7424–7430. doi: 10.1128/AAC.01374-16
Hein, M., Helmig, R. B., Schønheyder, H. C., Ganz, T., Uldbjerg, N. (2001). An In Vitro Study of Antibacterial Properties of the Cervical Mucus Plug in Pregnancy. Am. J. Obstet. Gynecol. 185, 586–592. doi: 10.1067/mob.2001.116685
Hein, M., Valore, E. V., Helmig, R. B., Uldbjerg, N., Ganz, T. (2002). Antimicrobial Factors in the Cervical Mucus Plug. Am. J. Obstet. Gynecol. 187, 137–144. doi: 10.1067/mob.2002.123034
He, Y., Niu, X., Wang, B., Na, R., Xiao, B., Yang, H. (2020). Evaluation of the Inhibitory Effects of Lactobacillus Gasseri and Lactobacillus Crispatus on the Adhesion of Seven Common Lower Genital Tract Infection-Causing Pathogens to Vaginal Epithelial Cells. Front. Med. 7, 284. doi: 10.3389/fmed.2020.00284
Hensler, M. E., Liu, G. Y., Sobczak, S., Benirschke, K., Nizet, V., Heldt, G. P. (2005). Virulence Role of Group B Streptococcus β-Hemolysin/Cytolysin in a Neonatal Rabbit Model of Early-Onset Pulmonary Infection. J. Infect. Dis. 191, 1287–1291. doi: 10.1086/428946
Hillier, S. L., Ferrieri, P., Edwards, M. S., Ewell, M., Ferris, D., Fine, P., et al. (2019). A Phase 2, Randomized, Control Trial of Group B Streptococcus (GBS) Type III Capsular Polysaccharide-Tetanus Toxoid (GBS III-TT) Vaccine to Prevent Vaginal Colonization With GBS III. Clin. Infect. Dis. 68, 2079–2086. doi: 10.1093/cid/ciy838
Hoang, T., Toler, E., DeLong, K., Mafunda, N. A., Bloom, S. M., Zierden, H. C., et al. (2020). The Cervicovaginal Mucus Barrier to HIV-1 Is Diminished in Bacterial Vaginosis. PloS Pathog. 16 (1), e1008236. doi: 10.1371/journal.ppat.1008236
Ho, M., Chang, Y. Y., Chang, W. C., Lin, H. C., Wang, M. H., Lin, W. C., et al. (2016). Oral Lactobacillus Rhamnosus GR-1 and Lactobacillus Reuteri RC-14 to Reduce Group B Streptococcus Colonization in Pregnant Women: A Randomized Controlled Trial. Taiwan J. Obstet. Gynecol. 55, 515–518. doi: 10.1016/j.tjog.2016.06.003
Islam, E. A., Anipindi, V. C., Francis, I., Shaik-Dasthagirisaheb, Y., Xu, S., Leung, N., et al. (2018). Specific Binding to Differentially Expressed Human Carcinoembryonic Antigenrelated Cell Adhesion Molecules Determines the Outcome of Neisseria Gonorrhoeae Infections Along the Female Reproductive Tract. Infect. Immun. 86 (8), e00092–18. doi: 10.1128/IAI.00092-18
Jiang, S. M., Cieslewicz, M. J., Kasper, D. L., Wessels, M. R. (2005). Regulation of Virulence by a Two-Component System in Group B Streptococcus. J. Bacteriol. 187, 1105–1113. doi: 10.1128/JB.187.3.1105-1113.2005
Jiang, S., Wessels, M. R. (2014). BsaB, A Novel Adherence Factor of Group B Streptococcus. Infect. Immun. 82, 1007–1016. doi: 10.1128/IAI.01014-13
Jisuvei, S. C., Osoti, A., Njeri, M. A. (2020). Prevalence, Antimicrobial Susceptibility Patterns, Serotypes and Risk Factors for Group B Streptococcus Rectovaginal Isolates Among Pregnant Women at Kenyatta National Hospital, Kenya; A Cross-Sectional Study. BMC Infect. Dis. 20 (1), 302. doi: 10.1186/s12879-020-05035-1
Jones, N., Bohnsack, J. F., Takahashi, S., Oliver, K. A., Chan, M. S., Kunst, F., et al. (2003). Multilocus Sequence Typing System for Group B Streptococcus. J. Clin. Microbiol. 41, 2530–2536. doi: 10.1128/JCM.41.6.2530-2536.2003
Kao, Y., Tsai, M. H., Lai, M. Y., Chu, S. M., Huang, H. R., Chiang, M. C., et al. (2019). Emerging Serotype III Sequence Type 17 Group B Streptococcus Invasive Infection in Infants: The Clinical Characteristics and Impacts on Outcomes. BMC Infect. Dis. 19 (1), 538. doi: 10.1186/s12879-019-4177-y
Kolar, S. L., Kyme, P., Tseng, C. W., Soliman, A., Kaplan, A., Liang, J., et al. (2015). Group B Streptococcus Evades Host Immunity by Degrading Hyaluronan. Cell Host Microbe 18, 694–704. doi: 10.1016/j.chom.2015.11.001
Kothary, V., Doster, R. S., Rogers, L. M., Kirk, L. A., Boyd, K. L., Romano-Keeler, J., et al. (2017). Group B Streptococcus Induces Neutrophil Recruitment to Gestational Tissues and Elaboration of Extracellular Traps and Nutritional Immunity. Front. Cell. Infect. Microbiol. 7, 19. doi: 10.3389/fcimb.2017.00019
Kubota, T., Nojima, M., Itoh, S. (2002). Vaginal Bacterial Flora of Pregnant Women Colonized With Group B Streptococcus. J. Infect. Chemother. 8, 326–330. doi: 10.1007/s10156-002-0190-x
Kwatra, G., Adrian, P. V., Shiri, T., Buchmann, E. J., Cutland, C. L., Madhi, S. A. (2015). Natural Acquired Humoral Immunity Against Serotype-Specific Group B Streptococcus Rectovaginal Colonization Acquisition in Pregnant Women. Clin. Microbiol. Infect. 21, 568.e13–568.e21. doi: 10.1016/j.cmi.2015.01.030
Lacroix, G., Gouyer, V., Gottrand, F., Desseyn, J. L. (2020). The Cervicovaginal Mucus Barrier. Int. J. Mol. Sci. 21, 1–23. doi: 10.3390/ijms21218266
Lamy, M. C., Zouine, M., Fert, J., Vergassola, M., Couve, E., Pellegrini, E., et al. (2004). CovS/CovR of Group B Streptococcus: A Two-Component Global Regulatory System Involved in Virulence. Mol. Microbiol. 54, 1250–1268. doi: 10.1111/j.1365-2958.2004.04365.x
Lancefield, R. C. (1934). A Serological Differentiation of Specific Types of Bovine Hemolytic Streptococci (Group B). J. Exp. Med. 59, 441–458. doi: 10.1084/jem.59.4.441
Lannon, S. M. R., Vanderhoeven, J. P., Eschenbach, D. A., Gravett, M. G., Waldorf, K. M. A. (2014). Synergy and Interactions Among Biological Pathways Leading to Preterm Premature Rupture of Membranes. Reprod. Sci. 21, 1215–1227. doi: 10.1177/1933719114534535
Le Doare, K., O’Driscoll, M., Turner, K., Seedat, F., Russell, N. J., Seale, A. C., et al. (2017). Intrapartum Antibiotic Chemoprophylaxis Policies for the Prevention of Group B Streptococcal Disease Worldwide: Systematic Review. Clin. Infect. Dis. 65, S143–S151. doi: 10.1093/cid/cix654
Lee, D. C., Hassan, S. S., Romero, R., Tarca, A. L., Bhatti, G., Gervasi, M. T., et al. (2011). Protein Profiling Underscores Immunological Functions of Uterine Cervical Mucus Plug in Human Pregnancy. J. Proteomics 74, 817–828. doi: 10.1016/j.jprot.2011.02.025
Lembo, A., Gurney, M. A., Burnside, K., Banerjee, A., De Los Reyes, M., Connelly, J. E., et al. (2010). Regulation of CovR Expression in Group B Streptococcus Impacts Blood-Brain Barrier Penetration. Mol. Microbiol. 77, 431–443. doi: 10.1111/j.1365-2958.2010.07215.x
Liu, G. Y., Doran, K. S., Lawrence, T., Turkson, N., Puliti, M., Tissi, L., et al. (2004). Sword and Shield: Linked Group B Streptococcal β-Hemolysin/Cytolysin and Carotenoid Pigment Function to Subvert Host Phagocyte Defense. Proc. Natl. Acad. Sci. U. S. A. 101, 14491–14496. doi: 10.1073/pnas.0406143101
Liu, Y., Huang, Y., Cai, W., Li, D., Zheng, W., Xiao, Y., et al. (2020). Effect of Oral Lactobacillusrhamnosus GR-1 and Lactobacillusreuteri RC-14 on Vaginal Group B Streptococcus Colonization and Vaginal Microbiome in Late Pregnancy. Nan Fang Yi Ke Da Xue Xue Bao 40, 1753–1759. doi: 10.12122/j.issn.1673-4254.2020.12.09
Madanchi, H., Shoushtari, M., Kashani, H. H., Sardari, S. (2020). Antimicrobial Peptides of the Vaginal Innate Immunity and Their Role in the Fight Against Sexually Transmitted Diseases. New Microbes New Infect. 34, 100627. doi: 10.1016/j.nmni.2019.100627
Madrid, L., Seale, A. C., Kohli-Lynch, M., Edmond, K. M., Lawn, J. E., Heath, P. T., et al. (2017). Infant Group B Streptococcal Disease Incidence and Serotypes Worldwide: Systematic Review and Meta-Analyses. Clin. Infect. Dis. 65, S160–S172. doi: 10.1093/cid/cix656
Mahendroo, M. (2019). Cervical Hyaluronan Biology in Pregnancy, Parturition and Preterm Birth. Matrix Biol. 78–79, 24–31. doi: 10.1016/j.matbio.2018.03.002
Marques, M. B., Kasper, D. L., Pangburn, M. K., Wessels, M. R. (1992). Prevention of C3 Deposition by Capsular Polysaccharide Is a Virulence Mechanism of Type III Group B Streptococci. Infect. Immun. 60, 3986–3993. doi: 10.1128/iai.60.10.3986-3993.1992
Martín, V., Cárdenas, N., Ocaña, S., Marín, M., Arroyo, R., Beltrán, D., et al. (2019). Rectal and Vaginal Eradication of Streptococcus Agalactiae (Gbs) in Pregnant Women by Using Lactobacillus Salivarius Cect 9145, A Target-Specific Probiotic Strain. Nutrients 11 (4), 810. doi: 10.3390/nu11040810
Martin, D., Rioux, S., Gagnon, E., Boyer, M., Hamel, J., Charland, N., et al. (2002). Protection From Group B Streptococcal Infection in Neonatal Mice by Maternal Immunization With Recombinant Sip Protein. Infect. Immun. 70, 4897–4901. doi: 10.1128/IAI.70.9.4897-4901.2002
Marziali, G., Foschi, C., Parolin, C., Vitali, B., Marangoni, A. (2019). In-Vitro Effect of Vaginal Lactobacilli Against Group B Streptococcus. Microb. Pathog. 136, 103692. doi: 10.1016/j.micpath.2019.103692
McCartney, S. A., Kapur, R., Liggitt, H. D., Baldessari, A., Coleman, M., Orvis, A., et al. (2021). Amniotic Fluid Interleukin 6 and Interleukin 8 Are Superior Predictors of Fetal Lung Injury Compared With Maternal or Fetal Plasma Cytokines or Placental Histopathology in a Nonhuman Primate Model. Am. J. Obstet. Gynecol. 225 (1), 89.e1–89.e16. doi: 10.1016/j.ajog.2020.12.1214
Meehan, M., Cunney, R., Cafferkey, M. (2014). Molecular Epidemiology of Group B Streptococci in Ireland Reveals a Diverse Population With Evidence of Capsular Switching. Eur. J. Clin. Microbiol. Infect. Dis. 33, 1155–1162. doi: 10.1007/s10096-014-2055-5
Meyn, L. A., Krohn, M. A., Hillier, S. L. (2009). Rectal Colonization by Group B Streptococcus as a Predictor of Vaginal Colonization. Am. J. Obstet. Gynecol. 201, 76.e1–76.e7. doi: 10.1016/j.ajog.2009.02.011
Michon, F., Uitz, C., Sarkar, A., D’Ambra, A. J., Laude-Sharp, M., Moore, S., et al. (2006). Group B Streptococcal Type II and III Conjugate Vaccines: Physicochemical Properties That Influence Immunogenicity. Clin. Vaccine Immunol. 13, 936–943. doi: 10.1128/CVI.00122-06
Mistou, M. Y., Dramsi, S., Brega, S., Poyart, C., Trieu-Cuot, P. (2009). Molecular Dissection of the Seca2 Locus of Group B Streptococcus Reveals That Glycosylation of the Srr1 LPXTG Protein Is Required for Full Virulence. J. Bacteriol. 191, 4195–4206. doi: 10.1128/JB.01673-08
Mu, X., Zhao, C., Yang, J., Wei, X., Zhang, J., Liang, C., et al. (2019). Group B Streptococcus Colonization Induces Prevotella and Megasphaera Abundance-Featured Vaginal Microbiome Compositional Change in Non-Pregnant Women. PeerJ 7, e7474. doi: 10.7717/peerj.7474
Nagano, N., Koide, S., Hayashi, W., Taniguchi, Y., Tanaka, H., Maeyama, Y., et al. (2019). Population-Level Transition of Capsular Polysaccharide Types Among Sequence Type 1 Group B Streptococcus Isolates With Reduced Penicillin Susceptibility During a Long-Term Hospital Epidemic. Int. J. Antimicrob. Agents 53, 203–210. doi: 10.1016/j.ijantimicag.2018.11.003
Nanduri, S. A., Petit, S., Smelser, C., Apostol, M., Alden, N. B., Harrison, L. H., et al. (2019). Epidemiology of Invasive Early-Onset and Late-Onset Group B Streptococcal Disease in the United Stateto 2015: Multistate Laboratory and Population-Based Surveillance. JAMA Pediatr. 173, 224–233. doi: 10.1001/jamapediatrics.2018.4826
Neemuchwala, A., Teatero, S., Athey, T. B. T., McGeer, A., Fittipaldi, N. (2016). Capsular Switching and Other Large-Scale Recombination Events in Invasive Sequence Type 1 Group B Streptococcus. Emerg. Infect. Dis. 22, 1941–1944. doi: 10.3201//eid2211.152064
Nizet, V., Gibson, R. L., Chi, E. Y., Framson, P. E., Hulse, M., Rubens, C. E. (1996). Group B Streptococcal Beta-Hemolysin Expression Is Associated With Injury of Lung Epithelial Cells. Infect. Immun. 64, 3818–3826. doi: 10.1128/iai.64.9.3818-3826.1996
Nizet, V., Gibson, R. L., Rubens, C. E. (1997). The Role of Group B Streptococci β-Hemolysin Expression in Newborn Lung Injury. Adv. Exp. Med. Biol. (Springer New York LLC), 627–630. doi: 10.1007/978-1-4899-1825-3_146
Nuccitelli, A., Cozzi, R., Gourlay, L. J., Donnarumma, D., Necchi, F., Norais, N., et al. (2011). Structure-Based Approach to Rationally Design a Chimeric Protein for an Effective Vaccine Against Group B Streptococcus Infections. Proc. Natl. Acad. Sci. U. S. A. 108, 10278–10283. doi: 10.1073/pnas.1106590108
Oldrini, D., del Bino, L., Arda, A., Carboni, F., Henriques, P., Angiolini, F., et al. (2020). Structure-Guided Design of a Group B Streptococcus Type III Synthetic Glycan–Conjugate Vaccine. Chem. - A Eur. J. 26, 7018–7025. doi: 10.1002/chem.202000284
Olmsted, S. S., Meyn, L. A., Rohan, L. C., Hillier, S. L. (2003). Glycosidase and Proteinase Activity of Anaerobic Gram-Negative Bacteria Isolated From Women With Bacterial Vaginosis. Sex Transm. Dis. 30, 257–261. doi: 10.1097/00007435-200303000-00016
Olsen, P., Williamson, M., Traynor, V., Georgiou, C. (2018). The Impact of Oral Probiotics on Vaginal Group B Streptococcal Colonisation Rates in Pregnant Women: A Pilot Randomised Control Study. Women Birth 31, 31–37. doi: 10.1016/j.wombi.2017.06.012
Onderdonk, A. B., Delaney, M. L., Fichorova, R. N. (2016). The Human Microbiome During Bacterial Vaginosis. Clin. Microbiol. Rev. 29, 223–238. doi: 10.1128/CMR.00075-15
Ortiz, L., Ruiz, F., Pascual, L., Barberis, L. (2014). Effect of Two Probiotic Strains of Lactobacillus on In Vitro Adherence of Listeria Monocytogenes, Streptococcus Agalactiae, and Staphylococcus Aureus to Vaginal Epithelial Cells. Curr. Microbiol. 68, 679–684. doi: 10.1007/s00284-014-0524-9
Park, S. E., Jiang, S., Wessels, M. R. (2012). CsrRS and Environmental pH Regulate Group B Streptococcus Adherence to Human Epithelial Cells and Extracellular Matrix. Infect. Immun. 80, 3975–3984. doi: 10.1128/IAI.00699-12
Patras, K. A., Rösler, B., Thoman, M. L., Doran, K. S. (2015). Characterization of Host Immunity During Persistent Vaginal Colonization by Group B Streptococcus. Mucosal Immunol. 8, 1339–1348. doi: 10.1038/mi.2015.23
Patras, K. A., Wang, N. Y., Fletcher, E. M., Cavaco, C. K., Jimenez, A., Garg, M., et al. (2013). Group BStreptococcusCovR Regulation Modulates Host Immune Signalling Pathways to Promote Vaginal Colonization. Cell. Microbiol. 15, 1154–1167. doi: 10.1111/cmi.12105
Patron, K., Gilot, P., Rong, V., Hiron, A., Mereghetti, L., Camiade, E. (2017). Inductors and Regulatory Properties of the Genomic Island-Associated Fru2 Metabolic Operon of Streptococcus Agalactiae. Mol. Microbiol. 103, 678–697. doi: 10.1111/mmi.13581
Poyart, C., Lamy, M. C., Boumaila, C., Fiedler, F., Trieu-Cuot, P. (2001). Regulation of D-Alanyl-Lipoteichoic Acid Biosynthesis in Streptococcus Agalactiae Involves a Novel Two-Component Regulatory System. J. Bacteriol. 183, 6324–6334. doi: 10.1128/JB.183.21.6324-6334.2001
Poyart, C., Pellegrini, E., Marceau, M., Baptista, M., Jaubert, F., Lamy, M. C., et al. (2003). Attenuated Virulence of Streptococcus Agalactiae Deficient in D-Alanyl-Lipoteichoic Acid Is Due to an Increased Susceptibility to Defensins and Phagocytic Cells. Mol. Microbiol. 49, 1615–1625. doi: 10.1046/j.1365-2958.2003.03655.x
Pritzlaff, C. A., Chang, J. C. W., Kuo, S. P., Tamura, G. S., Rubens, C. E., Nizet, V. (2001). Genetic Basis for the β-Haemolytic/Cytolytic Activity of Group B Streptococcus. Mol. Microbiol. 39, 236–248. doi: 10.1046/j.1365-2958.2001.02211.x
Ravel, J., Gajer, P., Abdo, Z., Schneider, G. M., Koenig, S. S. K., McCulle, S. L., et al. (2011). Vaginal Microbiome of Reproductive-Age Women. Proc. Natl. Acad. Sci. U. S. A. 108, 4680–4687. doi: 10.1073/pnas.1002611107
Rego, S., Heal, T. J., Pidwill, G. R., Till, M., Robson, A., Lamont, R. J., et al. (2016). Structural and Functional Analysis of Cell Wall-Anchored Polypeptide Adhesin BspA in Streptococcus Agalactiae. J. Biol. Chem. 291, 15985–16000. doi: 10.1074/jbc.M116.726562
Rick, A. M., Aguilar, A., Cortes, R., Gordillo, R., Melgar, M., Samayoa-Reyes, G., et al. (2017). Group B Streptococci Colonization in Pregnant Guatemalan Women: Prevalence, Risk Factors, and Vaginal Microbiome. Open Forum Infect. Dis. 4 (1), ofx020. doi: 10.1093/ofid/ofx020
Rogers, L. M., Anders, A. P., Doster, R. S., Gill, E. A., Gnecco, J. S., Holley, J. M., et al. (2018). Decidual Stromal Cell-Derived PGE2 Regulates Macrophage Responses to Microbial Threat. Am. J. Reprod. Immunol. 80 (4), e13032. doi: 10.1111/aji.13032
Romero, R., Dey, S. K., Fisher, S. J. (2014). Preterm Labor: One Syndrome, Many Causes. Sci. (80-. ). 345, 760–765. doi: 10.1126/science.1251816
Romero, R., Gomez-Lopez, N., Winters, A. D., Jung, E., Shaman, M., Bieda, J., et al. (2019). Evidence That Intra-Amniotic Infections Are Often the Result of an Ascending Invasion - A Molecular Microbiological Study. J. Perinat. Med. 47, 915–931. doi: 10.1515/jpm-2019-0297
Rosen, G. H., Randis, T. M., Desai, P. V., Sapra, K. J., Ma, B., Gajer, P., et al. (2017). Group B Streptococcus and the Vaginal Microbiota. J. Infect. Dis. (Oxford Univ. Press), 744–751. doi: 10.1093/infdis/jix395
Russell, N. J., Seale, A. C., O’Driscoll, M., O’Sullivan, C., Bianchi-Jassir, F., Gonzalez-Guarin, J., et al. (2017). Maternal Colonization With Group B Streptococcus and Serotype Distribution Worldwide: Systematic Review and Meta-Analyses. Clin. Infect. Dis. 65, S100–S111. doi: 10.1093/cid/cix658
Sadowsky, D. W., Adams, K. M., Gravett, M. G., Witkin, S. S., Novy, M. J. (2006). Preterm Labor Is Induced by Intraamniotic Infusions of Interleukin-1β and Tumor Necrosis Factor-α But Not by Interleukin-6 or Interleukin-8 in a Nonhuman Primate Model. Am. J. Obstet. Gynecol. 195, 1578–1589. doi: 10.1016/j.ajog.2006.06.072
Samen, U., Eikmanns, B. J., Reinscheid, D. J., Borges, F. (2007). The Surface Protein Srr-1 of Streptococcus Agalactiae Binds Human Keratin 4 and Promotes Adherence to Epithelial HEp-2 Cells. Infect. Immun. 75, 5405–5414. doi: 10.1128/IAI.00717-07
Santi, I., D, M., CL, G., G, G., JL, T., M, S. (2009). BibA Induces Opsonizing Antibodies Conferring In Vivo Protection Against Group B Streptococcus. J. Infect. Dis. 200, 564–570. doi: 10.1086/603540
Santillan, D. A., Andracki, M. E., Hunter, S. K. (2008). Protective Immunization in Mice Against Group B Streptococci Using Encapsulated C5a Peptidase. Am. J. Obstet. Gynecol. 198, 114.e1–114.e6. doi: 10.1016/j.ajog.2007.06.003
Santillan, D. A., Rai, K. K., Santillan, M. K., Krishnamachari, Y., Salem, A. K., Hunter, S. K. (2011). Efficacy of Polymeric Encapsulated C5a Peptidasebased Group B Streptococcus Vaccines in a Murine Model. Am. J. Obstetrics Gynecol. (Mosby Inc.), 249.e1–249.e8. doi: 10.1016/j.ajog.2011.06.024
Santi, I., Scarselli, M., Mariani, M., Pezzicoli, A., Masignani, V., Taddei, A., et al. (2007). BibA: A Novel Immunogenic Bacterial Adhesin Contributing to Group B Streptococcus Survival in Human Blood. Mol. Microbiol. 63, 754–767. doi: 10.1111/j.1365-2958.2006.05555.x
Scheibner, K. A., Lutz, M. A., Boodoo, S., Fenton, M. J., Powell, J. D., Horton, M. R. (2006). Hyaluronan Fragments Act as an Endogenous Danger Signal by Engaging Tlr2. J. Immunol. 177, 1272–1281. doi: 10.4049/jimmunol.177.2.1272
Schleimer, R. P., Kato, A., Kern, R., Kuperman, D., Avila, P. C. (2007). Epithelium: At the Interface of Innate and Adaptive Immune Responses. J. Allergy Clin. Immunol. 120, 1279–1284. doi: 10.1016/j.jaci.2007.08.046
Seale, A. C., Bianchi-Jassir, F., Russell, N. J., Kohli-Lynch, M., Tann, C. J., Hall, J., et al. (2017a). Estimates of the Burden of Group B Streptococcal Disease Worldwide for Pregnant Women, Stillbirths, and Children. Clin. Infect. Dis. 65, S200–S219. doi: 10.1093/cid/cix664
Seale, A. C., Blencowe, H., Bianchi-Jassir, F., Embleton, N., Bassat, Q., Ordi, J., et al. (2017b). Stillbirth With Group B Streptococcus Disease Worldwide: Systematic Review and Meta-Analyses. Clin. Infect. Dis. 65, S125–S132. doi: 10.1093/cid/cix585
Seo, H. S., Minasov, G., Seepersaud, R., Doran, K. S., Dubrovska, I., Shuvalova, L., et al. (2013). Characterization of Fibrinogen Binding by Glycoproteins Srr1 and Srr2 of Streptococcus Agalactiae. J. Biol. Chem. 288, 35982–35996. doi: 10.1074/jbc.M113.513358
Sharpe, M., Shah, V., Freire-Lizama, T., Cates, E. C., McGrath, K., David, I., et al. (2021). Effectiveness of Oral Intake of Lactobacillus Rhamnosus GR-1 and Lactobacillus Reuteri RC-14 on Group B Streptococcus Colonization During Pregnancy: A Midwifery-Led Double-Blind Randomized Controlled Pilot Trial. J. Matern. Neonatal Med. 34, 1814–1821. doi: 10.1080/14767058.2019.1650907
Sheen, T. R., Jimenez, A., Wang, N. Y., Banerjee, A., van Sorge, N. M., Doran, K. S. (2011). Serine-Rich Repeat Proteins and Pili Promote Streptococcus Agalactiae Colonization of the Vaginal Tract. J. Bacteriol. 193, 6834–6842. doi: 10.1128/JB.00094-11
Sitkiewicz, I., Green, N. M., Guo, N., Bongiovanni, A. M., Witkin, S. S., Musser, J. M. (2009). Transcriptome Adaptation of Group B Streptococcus to Growth in Human Amniotic Fluid. PloS One 4 (7), e6114. doi: 10.1371/journal.pone.0006114
Smith-Dupont, K. B., Wagner, C. E., Witten, J., Conroy, K., Rudoltz, H., Pagidas, K., et al. (2017). Probing the Potential of Mucus Permeability to Signify Preterm Birth Risk. Sci. Rep. 7 (1), 10302. doi: 10.1038/s41598-017-08057-z
Soto, J. A., Diaz-Dinamarca, D. A., Soto, D. A., Barrientos, M. J., Carrión, F., Kalergis, A. M., et al. (2019). Cellular Immune Response Induced by Surface Immunogenic Protein With AbISCO-100 Adjuvant Vaccination Decreases Group B Streptococcus Vaginal Colonization. Mol. Immunol. 111, 198–204. doi: 10.1016/j.molimm.2019.04.025
Sroka-Oleksiak, A., Gosiewski, T., Pabian, W., Gurgul, A., Kapusta, P., Ludwig-Słomczyńska, A. H., et al. (2020). Next-Generation Sequencing as a Tool to Detect Vaginal Microbiota Disturbances During Pregnancy. Microorganisms 8, 1–15. doi: 10.3390/microorganisms8111813
Stålhammar-Carlemalm, M., Waldemarsson, J., Johnsson, E., Areschoug, T., Lindahl, G. (2007). Nonimmunodominant Regions Are Effective as Building Blocks in a Streptococcal Fusion Protein Vaccine. Cell Host Microbe 2, 427–434. doi: 10.1016/j.chom.2007.10.003
Sundrani, D. P., Chavan-Gautam, P. M., Pisal, H. R., Mehendale, S. S., Joshi, S. R. (2012). Matrix Metalloproteinase-1 and -9 in Human Placenta During Spontaneous Vaginal Delivery and Caesarean Sectioning in Preterm Pregnancy. PloS One 7 (1), e29855. doi: 10.1371/journal.pone.0029855
Sun, J., Fang, W., Ke, B., He, D., Liang, Y., Ning, D., et al. (2016). Inapparent Streptococcus Agalactiae Infection in Adult/Commercial Tilapia. Sci. Rep. 6, 26319. doi: 10.1038/srep26319
Sutton, J. A., Rogers, L. M., Dixon, B. R. E. A., Kirk, L., Doster, R., Algood, H. M., et al. (2019). Protein Kinase D Mediates Inflammatory Responses of Human Placental Macrophages to Group B Streptococcus. Am. J. Reprod. Immunol. 81. doi: 10.1111/aji.13075
Swamy, G. K., Metz, T. D., Edwards, K. M., Soper, D. E., Beigi, R. H., Campbell, J. D., et al. (2020). Safety and Immunogenicity of an Investigational Maternal Trivalent Group B Streptococcus Vaccine in Pregnant Women and Their Infants: Results From a Randomized Placebo-Controlled Phase II Trial. Vaccine 38, 6930–6940. doi: 10.1016/j.vaccine.2020.08.056
Sweeney, E. L., Gardiner, S., Tickner, J., Trim, L., Beagley, K. W., Carey, A. J. (2020). Group B Streptococcus Serotypes Ia and V Induce Differential Vaginal Immune Responses That may Contribute to Long Term Colonization of the Female Reproductive Tract. Am. J. Reprod. Immunol. 83 (1), e13199. doi: 10.1111/aji.13199
Takahashi, S., Aoyagi, Y., Adderson, E. E., Okuwaki, Y., Bohnsack, J. F. (1999). Capsular Sialic Acid Limits C5a Production on Type III Group B Streptococci. Infect. Immun. 67, 1866–1870. doi: 10.1128/iai.67.4.1866-1870.1999
Tambor, V., Kacerovsky, M., Lenco, J., Bhat, G., Menon, R. (2013). Proteomics and Bioinformatics Analysis Reveal Underlying Pathways of Infection Associated Histologic Chorioamnionitis in pPROM. Placenta 34, 155–161. doi: 10.1016/j.placenta.2012.11.028
Taylor, K. R., Trowbridge, J. M., Rudisill, J. A., Termeer, C. C., Simon, J. C., Gallo, R. L. (2004). Hyaluronan Fragments Stimulate Endothelial Recognition of Injury Through TLR4. J. Biol. Chem. 279, 17079–17084. doi: 10.1074/jbc.M310859200
Tazi, A., Disson, O., Bellais, S., Bouaboud, A., Dmytruk, N., Dramsi, S., et al. (2010). The Surface Protein HvgA Mediates Group B Streptococcus Hypervirulence and Meningeal Tropism in Neonates. J. Exp. Med. 207, 2313–2322. doi: 10.1084/jem.20092594
Teatero, S., Ramoutar, E., McGeer, A., Li, A., Melano, R. G., Wasserscheid, J., et al. (2016). Clonal Complex 17 Group B Streptococcus Strains Causing Invasive Disease in Neonates and Adults Originate From the Same Genetic Pool. Sci. Rep. 6, 20047. doi: 10.1038/srep20047
Termeer, C., Benedix, F., Sleeman, J., Fieber, C., Voith, U., Ahrens, T., et al. (2002). Oligosaccharides of Hyaluronan Activate Dendritic Cells via Toll-Like Receptor 4. J. Exp. Med. 195, 99–111. doi: 10.1084/jem.20001858
Tsai, M. H., Hsu, J. F., Lai, M. Y., Lin, L. C., Chu, S. M., Huang, H. R., et al. (2019). Molecular Characteristics and Antimicrobial Resistance of Group B Streptococcus Strains Causing Invasive Disease in Neonates and Adults. Front. Microbiol. 10, 264. doi: 10.3389/fmicb.2019.00264
Uchiyama, S., Sun, J., Fukahori, K., Ando, N., Wu, M., Schwarz, F., et al. (2019). Dual Actions of Group B Streptococcus Capsular Sialic Acid Provide Resistance to Platelet-Mediated Antimicrobial Killing. Proc. Natl. Acad. Sci. U. S. A. 116, 7465–7470. doi: 10.1073/pnas.1815572116
Vanderhoeven, J. P., Bierle, C. J., Kapur, R. P., McAdams, R. M., Beyer, R. P., Bammler, T. K., et al. (2014). Group B Streptococcal Infection of the Choriodecidua Induces Dysfunction of the Cytokeratin Network in Amniotic Epithelium: A Pathway to Membrane Weakening. PloS Pathog. 10 (3), e1003920. doi: 10.1371/journal.ppat.1003920
van Sorge, N. M., Bonsor, D. A., Deng, L., Lindahl, E., Schmitt, V., Lyndin, M., et al. (2021). Bacterial Protein Domains With a Novel Ig-Like Fold Target Human CEACAM Receptors. EMBO J. 40 (7), e106103. doi: 10.15252/embj.2020106103
Vornhagen, J., Armistead, B., Santana-Ufret, V., Gendrin, C., Merillat, S., Coleman, M., et al. (2018a). Group B Streptococcus Exploits Vaginal Epithelial Exfoliation for Ascending Infection. J. Clin. Invest. 128, 1985–1999. doi: 10.1172/JCI97043
Vornhagen, J., Quach, P., Boldenow, E., Merillat, S., Whidbey, C., Ngo, L. Y., et al. (2016). Bacterial Hyaluronidase Promotes Ascending GBS Infection and Preterm Birth. MBio. 7 (3):e00781–16. doi: 10.1128/mBio.00781-16
Vornhagen, J., Quach, P., Santana-Ufret, V., Alishetti, V., Brokaw, A., Armistead, B., et al. (2018b). Human Cervical Mucus Plugs Exhibit Insufficiencies in Antimicrobial Activity Towards Group B Streptococcus. J. Infect. Dis. 217, 1626–1636. doi: 10.1093/infdis/jiy076
Vrbanac, A., Riestra, A. M., Coady, A., Knight, R., Nizet, V., Patras, K. A. (2018). The Murine Vaginal Microbiota and Its Perturbation by the Human Pathogen Group B Streptococcus. BMC Microbiol. 18 (1), 197. doi: 10.1186/s12866-018-1341-2
Walsh, S. W., Nugent, W. H., Solotskaya, A. V., Anderson, C. D., Grider, J. R., Strauss, J. F. (2018). Matrix Metalloprotease-1 and Elastase Are Novel Uterotonic Agents Acting Through Protease-Activated Receptor 1. Reprod. Sci. 25, 1058–1066. doi: 10.1177/1933719117732162
Wang, N. Y., Patras, K. A., Seo, H. S., Cavaco, C. K., Rösler, B., Neely, M. N., et al. (2014). Group B Streptococcal Serine-Rich Repeat Proteins Promote Interaction With Fibrinogen and Vaginal Colonization. J. Infect. Dis. (Oxford Univ. Press) 210 (6), 982–991. doi: 10.1093/infdis/jiu151
Weed, S., Armistead, B., Coleman, M., Denny Liggit, H., Johnson, B., Tsai, J., et al. (2020). MicroRNA Signature of Epithelial-Mesenchymal Transition in Group B Streptococcal Infection of the Placental Chorioamniotic Membranes. J. Infect. Dis. 222, 1713–1722. doi: 10.1093/infdis/jiaa280
Weiman, S., Uchiyama, S., Lin, F. Y. C., Chaffin, D., Varki, A., Nizet, V., et al. (2010). O-Acetylation of Sialic Acid on Group B Streptococcus Inhibits Neutrophil Suppression and Virulence. Biochem. J. 428, 163–168. doi: 10.1042/BJ20100232
Whidbey, C., Harrell, M. I., Burnside, K., Ngo, L., Becraft, A. K., Iyer, L. M., et al. (2013). A Hemolytic Pigment of Group B Streptococcus Allows Bacterial Penetration of Human Placenta. J. Exp. Med. 210, 1265–1281. doi: 10.1084/jem.20122753
Keywords: group B streptococcus, bacteria, pregnancy, colonization, vagina, placenta, fetus, preterm birth
Citation: Brokaw A, Furuta A, Dacanay M, Rajagopal L and Adams Waldorf KM (2021) Bacterial and Host Determinants of Group B Streptococcal Vaginal Colonization and Ascending Infection in Pregnancy. Front. Cell. Infect. Microbiol. 11:720789. doi: 10.3389/fcimb.2021.720789
Received: 05 June 2021; Accepted: 09 August 2021;
Published: 03 September 2021.
Edited by:
Irina Burd, Johns Hopkins University, United StatesReviewed by:
Raja Veerapandian, Texas Tech University Health Sciences Center El Paso, United StatesAnbarasu Kumarasamy, Bharathidasan University, India
Copyright © 2021 Brokaw, Furuta, Dacanay, Rajagopal and Adams Waldorf. This is an open-access article distributed under the terms of the Creative Commons Attribution License (CC BY). The use, distribution or reproduction in other forums is permitted, provided the original author(s) and the copyright owner(s) are credited and that the original publication in this journal is cited, in accordance with accepted academic practice. No use, distribution or reproduction is permitted which does not comply with these terms.
*Correspondence: Kristina M. Adams Waldorf, YWRhbXNrQHV3LmVkdQ==; Lakshmi Rajagopal, bGFrc2htaS5yYWphZ29wYWxAc2VhdHRsZWNoaWxkcmVucy5vcmc=
†These authors have contributed equally to this work and share first authorship