- 1Division II of In Vitro Diagnostics for Infectious Diseases, Institute for In Vitro Diagnostics Control, National Institutes for Food and Drug Control, Beijing, China
- 2Institute of Preventive Veterinary Sciences & Department of Veterinary Medicine, Zhejiang University College of Animal Sciences, Hangzhou, China
- 3Zhejiang Provincial Key Laboratory of Preventive Veterinary Medicine, Hangzhou, China
- 4State Key Laboratory for Diagnosis and Treatment of Infectious Diseases, National Clinical Research Center for Infectious Diseases, National Medical Center for Infectious Diseases, The First Affiliated Hospital, College of Medicine, Zhejiang University, Hangzhou, China
- 5Hainan Institute of Zhejiang University, Sanya, China
Listeria monocytogenes remains a significant public health threat, causing invasive listeriosis manifested as septicemia, meningitis, and abortion, with up to 30% of cases having a fatal outcome. Tracking the spread of invasive listeriosis requires an updated knowledge for virulence factors (VFs) and antimicrobial resistance features, which is an essential step toward its clinical diagnosis and treatment. Taking advantage of high-throughput genomic sequencing, we proposed that the differential genes based on the pathogenomic composition could be used to evaluate clinical observations and therapeutic options for listeriosis. Here, we performed the comparative genomic analysis of 60 strains from five continents with a diverse range of sources, representing serotypes 1/2a, 1/2b, 1/2c, and 4b, comprising lineage I and lineage II and including 13 newly contributed Chinese isolates from clinical cases. These strains were associated with globally distributed clonal groups linked with confirmed foodborne listeriosis outbreak and sporadic cases. We found that L. monocytogenes strains from clonal complex (CC) CC8, CC7, CC9, and CC415 carried most of the adherence and invasive genes. Conversely, CC1, CC2, CC4, and CC6 have the least number of adherence and invasive genes. Additionally, Listeria pathogenicity island-1 (LIPI-1), LIPI-2, intracellular survival, surface anchoring, and bile salt resistance genes were detected in all isolates. Importantly, LIPI-3 genes were harbored in CC3, CC224, and ST619 of the Chinese isolates and in CC1, CC4, and CC6 of other worldwide isolates. Notably, Chinese isolates belonging to CC14 carried antibiotic resistance genes (ARGs) against β-lactams (blaTEM-101, blaTEM-105) and macrolide (ermC-15), whereas CC7 and CC8 isolates harbored ARGs against aminoglycoside (aadA10_2, aadA6_1), which may pose a threat to therapeutic efficacy. Phylogenomic analysis showed that CC8, CC7, and CC5 of Chinese isolates, CC8 (Swiss and Italian isolates), and CC5 and CC7 (Canadian isolates) are closely clustered together and belonged to the same CC. Additionally, CC381 and CC29 of Chinese isolates shared the same genomic pattern as CC26 of Swiss isolate and CC37 of Canadian isolate, respectively, indicating strong phylogenomic relation between these isolates. Collectively, this study highlights considerable clonal diversity with well-recognized virulence and antimicrobial-resistant determinants among Chinese and worldwide isolates that stress to design improved strategies for clinical therapies.
Introduction
Listeria monocytogenes is the genetically heterogeneous species (Kathariou, 2002) implicated in numerous outbreaks of invasive listeriosis reported globally, characterized by septicemia, meningoencephalitis, and maternal–fetal infection leading to abortion (Shoai-Tehrani et al., 2019; Pilmis et al., 2020). The acquisition of this disease is mainly due to the consumption of contaminated food (Lomonaco et al., 2015). Clinically, listeriosis is among the obligatory notifiable diseases in several countries. Although listeriosis is associated with a low incidence rate in humans (Lomonaco et al., 2015), this disease is of great concern due to recurrent outbreaks linked with high mortality and morbidity rates. In China, the incidence of invasive listeriosis is associated with a high case fatality rate (Fan et al., 2019) and high mortality worldwide (EFSA, 2012; Self et al., 2019). Currently, L. monocytogenes is composed of four phylogenetic lineages and 13 classified serotypes. In the clinics, serotypes 1/2a, 1/2b, 1/2c, and 4b cause the majority of human listeriosis, which is associated with lineage I and lineage II (Den Bakker et al., 2013).
The ability of L. monocytogenes to invade and proliferate within host cells depends on a collection of virulence factors (VFs) at each step of the invasive process during the host–pathogen interaction (Vasquez-Boland et al., 2001; Bergman et al., 2013). The presence of various adhesive and invasive genes promotes adhesion or binding and invasion during infection (Burkholder and Bhunia, 2010; Ghosh and Higgins, 2018). In listeriosis, Listeria pathogenicity island-1 (LIPI-1) genes are essential for intracellular growth, multiplication, and further spread to adjacent cells during the infectious cycle (Kocks et al., 1992; Vasquez-Boland et al., 1992; Gouin et al., 1994). Invasion-associated surface protein internalin facilitates adherence and internalization of a host cell. The internalin family representing LIPI-2 particularly internalin A and internalin B (InlA and InlB) plays essential roles in overcoming host barriers (Dussurget et al., 2004). Additionally, listeriolysin S (LLS toxin) representing LIPI-3, which promotes posttranslational modifications, is crucial for its biological and bactericidal activity and host microbiota-related activity (Cotter et al., 2008).
During the infectious cycle, various stress proteins play a crucial role in the early stages of L. monocytogenes intracellular growth (Rouquette et al., 1998) by preventing the accumulation of altered proteins, which might be toxic for bacteria under stress conditions (Gaillot et al., 2000). On the other hand, intracellular modulator proteins modulate the interferon response by chromatin remodeling (Lebreton et al., 2011), autophagy evasion (Dortet et al., 2011), and dampening the normal immune response (Gouin et al., 2010), which is crucial for the bacterium survival within the host.
Intracellular survival proteins are necessary for intracellular proliferation (O’Riordan et al., 2003) and contribute to the integrity of L. monocytogenes cell wall, swimming motility, and resistance to osmotic stress (Alonzo and Freitag, 2010). Immune evasion proteins such as peptidoglycan modification are critical for bacterial survival (Rae et al., 2011) and conferred the resistance to lysozyme (Boneca et al., 2007). Surface-anchoring proteins are responsible for the maturation of lipoproteins (Reglier-Poupet et al., 2003), and bile salt promotes resisting the acute toxicity of bile and for intestinal persistence (Dusserget et al., 2002).
Recently, whole-genome sequencing (WGS) has proven to be a promising and predictive approach for potential virulence and functional characterization of VFs of L. monocytogenes strain (Fox et al., 2016), which would not only help to understand virulence mechanisms or strategies but also potentially help to monitor the risk of causing listeriosis (Reddy and Lawrence, 2014). In the past, despite the spectacular gains in knowledge, the virulence contents or factors of globally distributed clonal groups of L. monocytogenes remain poorly addressed. In listeriosis, a number of key VFs played a crucial role in the pathogenesis and survival of L. monocytogenes within the host during the infectious process (Dussurget et al., 2014). These VFs need to be investigated in order to better understand and interpret the clinical syndrome of invasive listeriosis for disease treatment and public health surveillance. To identify the virulence contents, resistance profiles, and pathogenic potential and establish the phylogenomic relationship, we have selected all available Chinese invasive isolates as well as global contextual isolates that have a clear link with foodborne listeriosis outbreaks, associated with mortality, and high case fatality rate. Moreover, the comparative investigations on phylogenetic relationships between Chinese and global isolates in relation to VFs and antimicrobial resistance features provide an improved understanding of the global epidemiology of invasive listeriosis caused by L. monocytogenes.
Materials and Methods
Collection of L. monocytogenes Isolates
From the year 2012 until now, 13 confirmed clinical isolates were recovered in China for this investigation. All the bacteria were confirmed as L. monocytogenes by a commercial biochemical test (API Listeria, BioMérieux, France). These bacterial isolates were further subjected to serotyping based on a serum agglutination test (NISSEIKEN Co. Ltd., Japan).
A total of 60 global L. monocytogenes clinical isolates were selected and analyzed in this study to represent diverse geographical locations: China (n = 24) including 13 new strains in this study as described previously, Canada (n = 25), Switzerland (n = 5), USA (n = 4), and Italy (n = 2). These strains are from blood (n = 37), cerebrospinal fluid (CSF) (n = 7), abortion (n = 7), and stool samples (n = 9) (Table 1). The reason for selecting these isolates is that all of them were largely globally distributed epidemic clones (ECs) with virulence features and have been confirmed with the foodborne outbreaks of listeriosis and sporadic cases with high case fatality rates and mortality rates. The information about these clinical isolates can be found in Table 1 (Farber et al., 2000; Pagotto et al., 2006; Baldry, 2010; Knabel et al., 2012; Gaulin et al., 2014; Reimer et al., 2019; Ragon et al., 2008; Lomonaco et al., 2011; Tasara et al., 2014; Centorame et al., 2015; Tasara et al., 2015; Thomas et al., 2015; Tasara et al., 2016; Zhang et al., 2016; Orsini et al., 2019).
Antibiotic Susceptibility Testing
Antibiotic susceptibility of 13 Chinese isolates was assayed using the broth microdilution minimum inhibitory concentrations (MICs) method according to the Clinical and Laboratory Standards Institute (CLSI) breakpoint guidelines (CLSI, 2015). The multiple classes of antimicrobials along with MIC range (µg/ml) used in the assay are as follows: β-lactams [ampicillin (AMP), 0.06–32]; penems [imipenem (IPM), 0.032–16], trimethoprim-sulfamethoxazole [cotri-moxazole (COT), 0.032–16], aminoglycosides [gentamicin (GEN), 0.032–16], tetracyclines [tetracycline (TET), 0.032–16], quinolones [ciprofloxacin (CIP), 0.032–16], phenicols [chloramphenicol (CHL), 0.125–64], and ammonium compounds [benzalkonium chloride (BB), 0.25–128]. Breakpoints for ampicillin and trimethoprim-sulfamethoxazole are as per the CLSI guidelines M45-A3 (CLSI, 2015). Since there are no relevant criteria for tetracycline, chloramphenicol, ciprofloxacin, gentamycin, and ciprofloxacin, the susceptibility results of these antibiotics were interpreted based on breakpoints of Staphylococcus spp (CLSI, 2017) as reported previously (Tahoun et al., 2017). Staphylococcus aureus ATCC29213 and Escherichia coli ATCC 25922 were used as quality control strains.
Whole-Genome Sequencing and Bioinformatic Analysis
The bacteria were cultured in Brain Heart Infusion (BHI) broth, and genomic DNA was extracted from the L. monocytogenes isolates using a commercial kit (Tiangen Biotech Beijing, Co., Ltd.) according to the manufacturer’s protocol. DNA quality and concentrations were analyzed by fluorometer using Qubit dsDNA HS Assay (Thermo Fisher Scientific, United States). For each isolate, paired-end genomic libraries were prepared using Nextera DNA Flex library preparation kit (Tiangen Biotech Beijing, Co., Ltd.). Sequencing was performed employing MiSeq Reagent Kit v2 (2x150bp) on the MiSeq platform (Illumina, United States), the paired and raw reads were trimmed using Trimmomatic (Galaxy version 0.36.6) (Bolger et al., 2014), and then draft genomes were assembled by SPAdes 3.12.0 genome assembler (Bankevich et al., 2012).
Furthermore, genomic sequencing data were retrieved from Genbank, Sequence Read Archive (SRA), and NCBI database. For the downstream bioinformatic analysis, SPAdes v3.12.0 genome assembler was used for genomic assembly, Package Snippy v4.4.4 was used to obtain single-nucleotide polymorphisms (SNPs) alignment, and a phylogenetic tree was constructed by IQ-TREE v1.6.12 with the TVM+F+R3 model. Assembled genomes were submitted to multi-locus sequencing typing (MLST) tool (version 2.3.2) (Carroll et al., 2017), which performs in silico analysis to determine the MLST profile. Furthermore, with the aim of identifying the antibiotic resistance genes (ARGs), plasmids, and VFs, the draft genomes of strains were investigated using the software ABRicate (Galaxy version.8) by applying the different types of databases, such as NCBI AMR finder Plus (Feldgarden et al., 2019), [ARG-ANNOT] (Gupta et al., 2014), CARD (Jia et al., 2017), ResFinder (Elbediwi et al., 2020a; Xu et al., 2020), and Plasmidfinder (Elbediwi et al., 2020b; Elbediwi et al., 2020c).
Statistical and Data Projection Analysis
Data were interpreted using the GraphPad Prism 7 software (GraphPad Software, Inc., USA) and PHYLOViZ software 2.0 using the goeBURST algorithm (Feil et al., 2004) for providing the scalable data integration and visualization for multiple phylogenetic inference methods.
Results
Serotypes, Lineages, Sequence Types, and Clonal Complex Analysis
Among the 60 isolates, 61% belonged to blood followed by CSF (12%), abortion (11%), and stool (16%) with predominant serogroup 1/2a (61%) followed by 1/2b (18%), 4b (20%), and 1/2c (1%) that were composed of lineage II (63%) and lineage I (37%) (Figures 1A, B). Serum agglutination test showed that among 13 Chinese isolates, seven (54%) belonged to 1/2a, five (39%) belonged to 1/2b, and one belonged to 1/2c. The results of the serum agglutination assay were confirmed by the in silico genomic analysis for these 13 Chinese clinical isolates. Overall, these isolates were grouped into 26 different sequence type (STs), 25 were assigned to the clonal complexes (CCs) and one singleton based on querying the MLST database. Among different STs, ST120 (13.33%), ST292 (6.66%), ST8 (6.66%), ST7 (5%), ST87 (5%), ST4 (3.33%), ST91 (3.33%), and ST3 (3.33%) were dominant from blood; followed by ST120 (3.33%), ST1 (3.33%), and ST2 (3.33%) from CSF; and ST8 (3.33%), ST9 (3.33%), ST121 (3.33%), ST1 (3.33%), ST6 (3.33%), and ST9 (3.33%) from stool (Figure 2A). Similarly, CC8 (26.65%), CC7 (6.66%), CC87 (5%), CC4 (3.33%), CC3 (3.33%), and CC14 (3.33%) were dominant from blood; followed by CC8 (3.33%), CC1 (3.33%), and CC2 (3.33%) from CSF; and CC8 (3.33%), CC9 (3.33%), CC121 (3.33%), CC1 (3.33%), CC6 (3.33%), and CC9 (3.33%) from stool (Figure 2B).
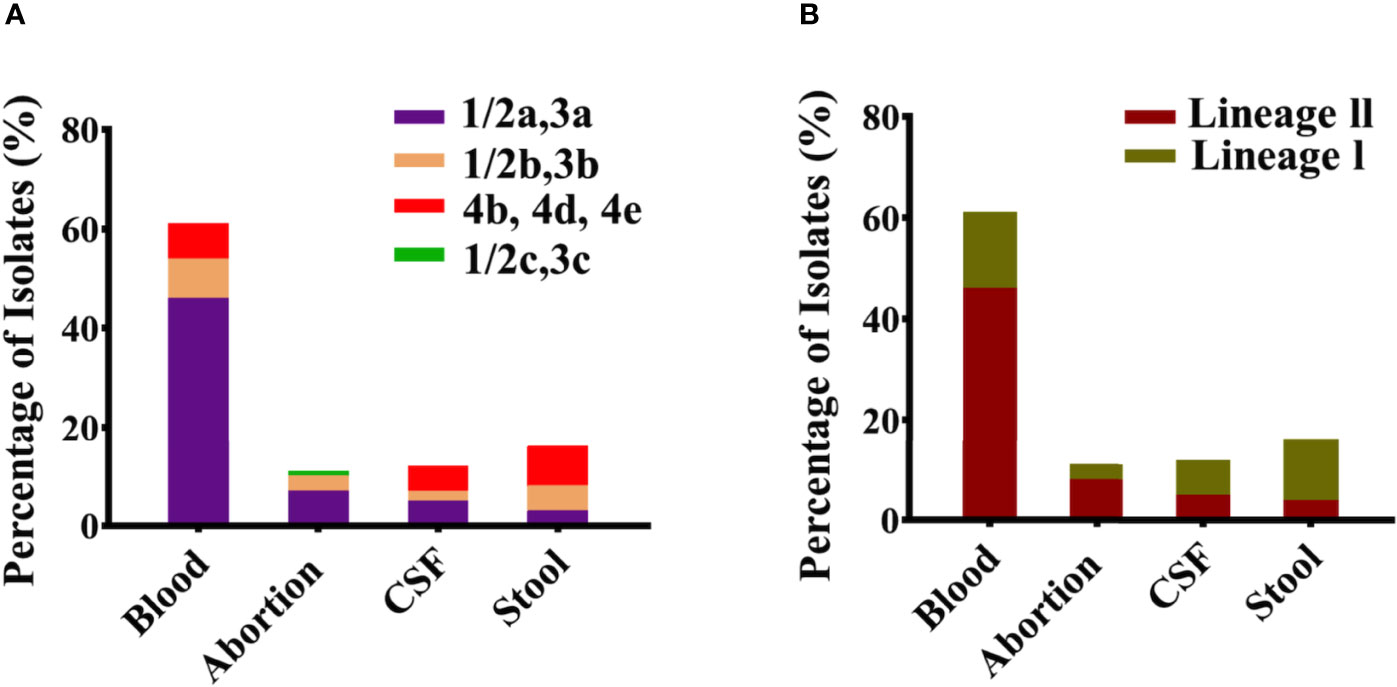
Figure 1 Classification of serotypes and lineages for all clinical Listeria monocytogenes isolates. (A) Serotypes 1/2a, 1/2b, 1/2c, and 4b representing individually each group/category of blood, abortion, cerebrospinal fluid (CSF), and stool isolates (n = 60). (B) Lineage I and Lineage II representing individually each group/category of blood, abortion, CSF, and stool isolates (n = 60).
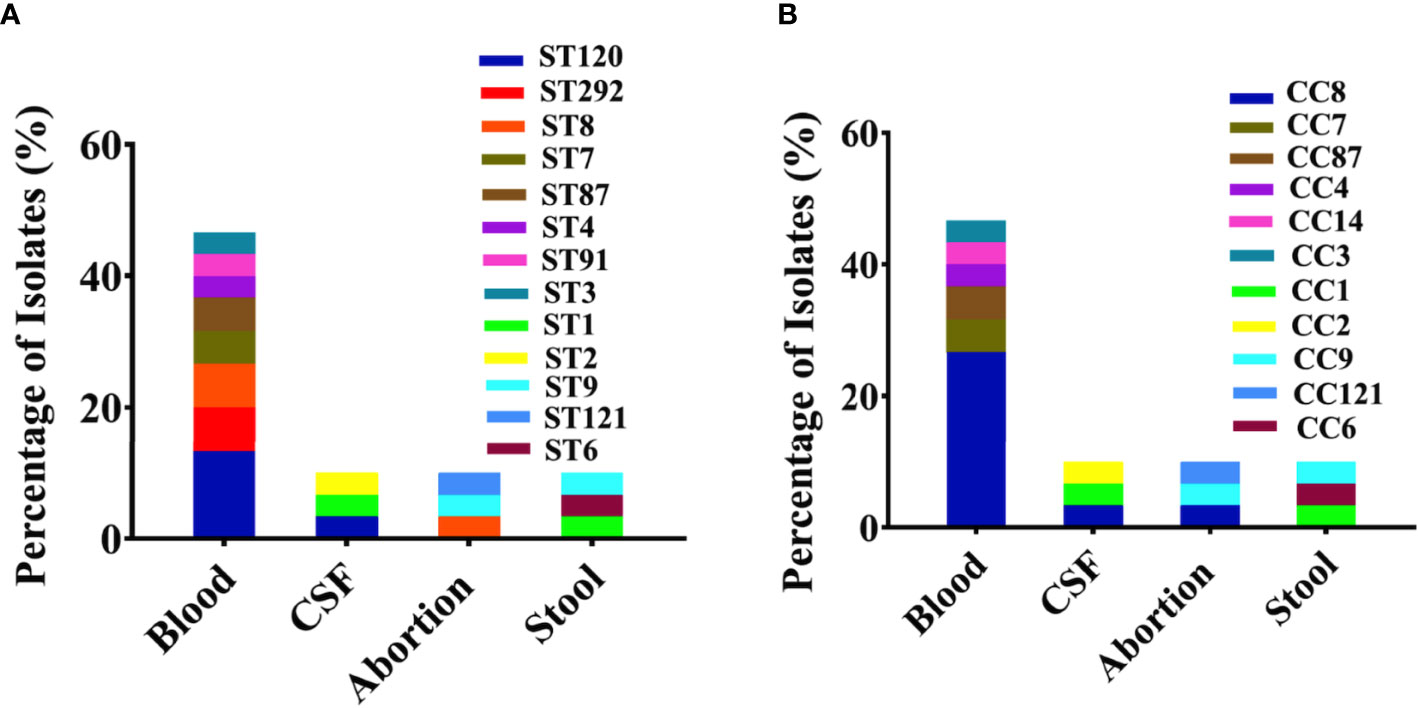
Figure 2 Genetic background with sequence types (STs) and clonal complexes (CCs) for all clinical Listeria monocytogenes isolates. (A) Major sequence types (STs) representing individually each group/category of blood, abortion, cerebrospinal fluid (CSF), and stool isolates (n = 60) are shown with colors. (B) Major CCs representing individually each group/category of blood, abortion, CSF, and stool isolates (n = 60) are shown with colors.
Genetic Relationship and Phylogenomic Analysis Among Chinese and Worldwide Isolates
The minimum spanning tree (MST) showed the relationship among various STs of isolates from different countries. Figure 3 illustrated that CC8 of Chinese isolates showed the divergence to CC8 of Canadian isolates but closely clustered together with the European CC8 isolates (Italian and Switzerland), while CC5 and CC7 of Chinese and Canadian isolates also showed a close resemblance.
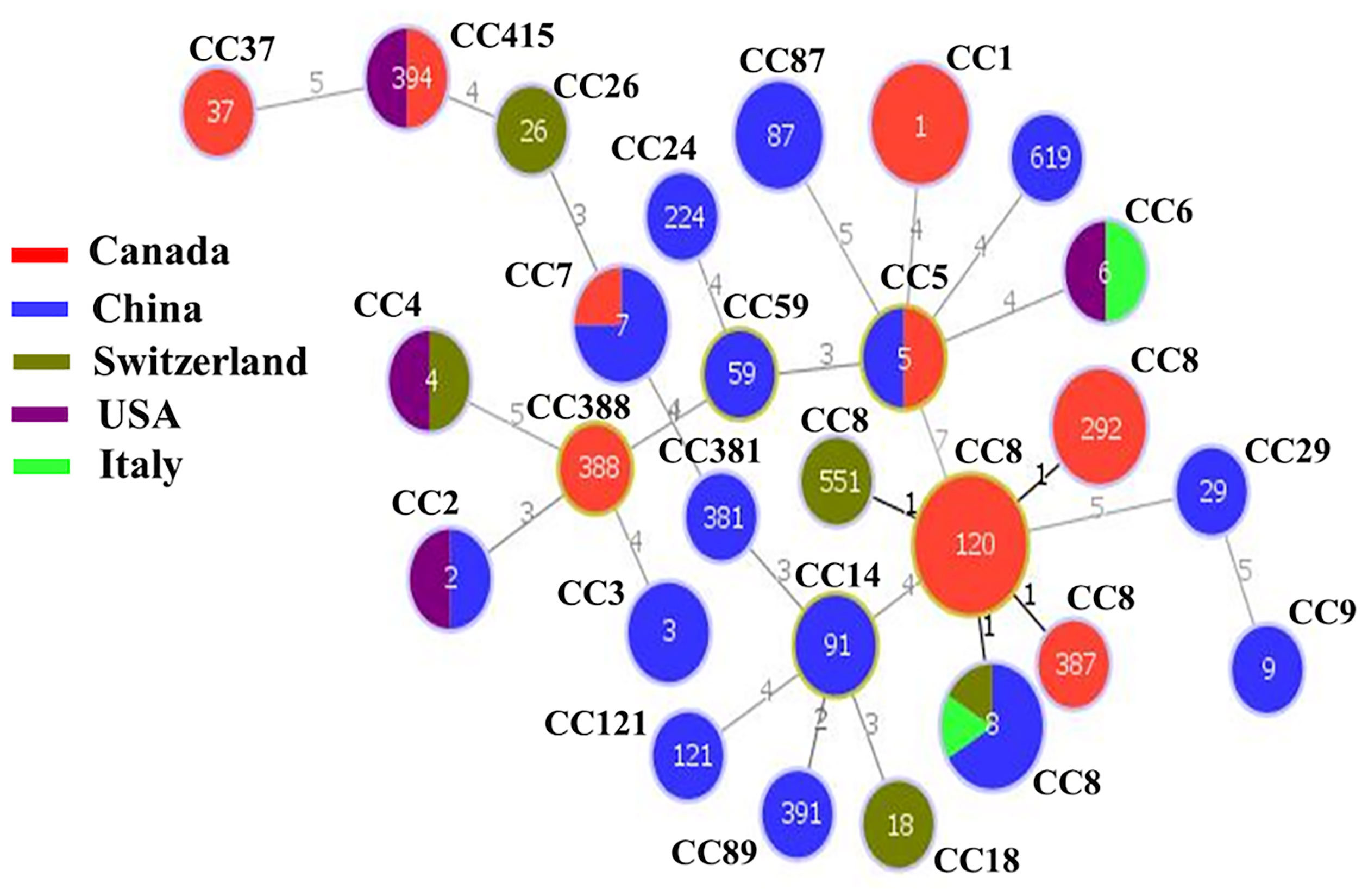
Figure 3 The minimum spanning tree (MST) illustrating the phylogenetic relationship based on sequence types (STs) allelic profiles of clinical strains of worldwide Listeria monocytogenes isolates. There are 60 isolates from various countries including China, Canada, Switzerland, USA, and Italy. Each circle represents one ST. The size of the circle is proportional to the number of isolates, and the color within the circle represents country of isolates. Links between the circles are represented according to the number of allelic mismatch between STs.
A maximum likelihood (ML) phylogenetic tree was constructed with the best model TVM+F+R3 by IQ-tree v1.6.12 using 139,505 SNPs, which were identified from core genome alignments (Figure 4) with Snippy v3.1. The phylogenetic tree showed that a high number of isolates with serotype 1/2a (brown color) in the upper clade including L0369 (Chinese isolate belonging to 1/2c, gray color), middle clade (dark brownish red) 1/2b, and lower clade (blue color) 4b (Figure 4). Phylogenomic analysis showed that CC8 of Chinese isolates (L0386, L087, SHL004, L0457) has shown a close resemblance to two Swiss isolates (Lm60 and LmN1546) and one Italian isolate 17439 that belonged to CC8. Furthermore, three Chinese isolates (L0458, L0381, and SHL005) from CC7 have shown the same conformity as CC7 of the Canadian isolate (10-0813). Conversely, in one clade, the genome of CC29 of the Chinese isolate showed the same pattern of genes to CC37 of the Canadian isolate (10-4754). Interestingly, these isolates were of serotype 1/2a and composed of lineage II and showed a similar pattern of genomic diversity with the absence of vip, pIA, and LIPI-3 genes (Figure 4).
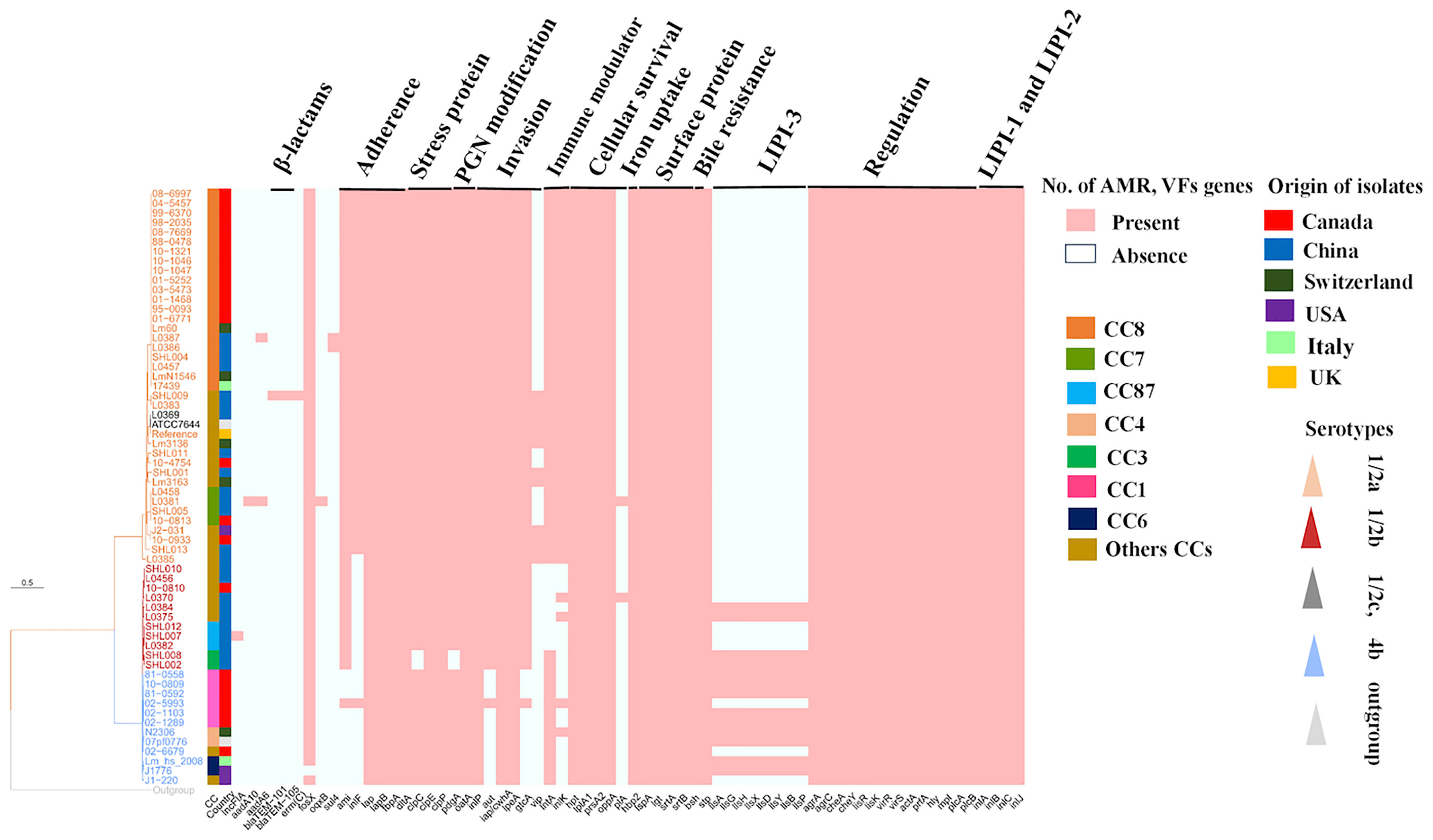
Figure 4 Phylogenomic analysis and heat map composition of antibiotic resistance genes (ARGs) and virulence factors (VFs) of worldwide Listeria monocytogenes clinical isolates. There are 60 isolates from different countries including China, Canada, Switzerland, USA, and Italy. Here, 139,505 single-nucleotide polymorphisms (SNPs) were used to construct the maximum likelihood phylogenomic tree for all compared genomes. EGD-e (reference strain), ATCC7644 (control strain), Listeria inoccua-CFSAN044836 (out-group strain). Tree scale represents the genetic distance between the isolates used to construct the tree. On the right, there is a heat map about pathogenic composition with clonal complexes (CCs); Plasmid: (incFIA_1); ARGs: aminoglycosides: aadA10_2, aadA6_1; β-lactams: blaTEM-101, blaTEM-105; macrolide: ermC-15; fosfomycin: fosX_2; quinolones: oqx_1; sulfonamide: sul4_1; Adherence genes: dltA, ami, inlF, iap, iapB, fbpA; stress-related genes: clpC, clpE, clpP; peptidoglycan modification genes: pdgA, oatA; invasion genes: inlP, aut, iap/cwhA, iapB, ipeA, gtc, vip; immune modulator genes: lntA, inlK; intracellular survival genes: hpt, ipIA, prsA2, oppA, pIA; iron uptake genes: hbp2; surface protein-anchoring genes: IspA, Igt, srtA, srtB; bile resistance genes: bsh; enzymatic genes: stp; listeriolysin S (LLS) toxin (LIPI-3): llsA, llsG, llsH, llsX, llsD, llsY, llSB, llsP; regulation genes, agrA, agrC, cheA, cheY, lisR, lisK, virR, virS; Listeria pathogenicity islands-1 (LIPI-1) genes: actA, prfA, hly, mpl, plcA, plcB; Listeria pathogenicity islands-2 (LIPI-2) genes: inlA, inlB, inlC, inlJ.
It has to be mentioned that one Chinese isolate (SHL001) CC381 has shown phylogenetic association with the Swiss isolate (Lm 3163) CC26 that were of serotype 1/2a and lineage II. Both shared similar gene patterns by the absence of pIA and LIPI-3 genes. Moreover, CC2 and CC5 of the Chinese isolates (SHL010, L0456) were closely linked to CC5 of the Canadian isolate (10-0810) composed of lineage I with the absence of vip, intA, inlK, pIA, and LIPI-3 genes (Figure 4).
Adherence, Invasion Genes, Along With LIPI-1, LIPI-2, And LIPI-3 Genes
The adhesive and invasive genes such as inlF, ami, and aut were harbored in CC8 (n = 22), CC7 (n = 3), CC415 (n = 2), CC9 (n = 1), and CC14 (n = 2). These strains were serotype 1/2a and comprised lineage II from Canada, USA, and China (Figure 4). A similar pattern for the presence of genes was followed by CC26, CC18, CC14, and CC381 from the same serotype 1/2a and lineage ll, while inlF and aut genes were missing from CC1 Canadian isolates (n = 5) of serotype 4b that was comprised lineage l. Notably, one isolate (02-993) from CC1 showed aberrant features by the presence of these virulence genes (Figure 4). In our study, inlF was also missing from 33% of the isolates, which belonged to CC2, CC3, CC4, CC5, CC6, CC59, CC87, CC121, CC224, and CC388 that comprised lineage I and lineage II from China, Canada, USA, Switzerland, and Italy. On the other hand, aut seems to be missing from 12% of isolates belonging to CC2, CC4, CC6, CC121, and CC388, comprising lineage I and II from China, USA, Switzerland, Italy, and Canada. Moreover, ami seems to be absent from 17% of isolates including CC1, CC2, CC6, CC4, and CC388 of serotype 4b that comprised lineage I from Canada, China, Italy, USA, and Switzerland.
LIPI-1 (plcB, mpl, plcA, hyl, actA, prfA) and LIPI-2 (inlA, inlB, inlC, inlJ) genes were detected in all genomes. Chinese isolates, CC3/ST3 (n = 3), CC224/ST224 (n = 1), and ST619 (n = 1) from serotype 1/2b and worldwide isolates including CC4/ST4 (n = 2), CC6/ST6 (n = 2), and CC1/ST1 (n = 5) of serotype 4b harbored LIPI-3 (llsA, llsG, llsH, llsX, llsD, llsY, llsB, llsP) genes (Figure 4).
Stress, Immune Modulator, Peptidoglycan Modification, Intracellular Survival, Hexose Phosphate Transporter, Surface Anchoring, and Bile Salt Resistance Genes
Only two Chinese isolates lacked clpC gene, while all other isolates harbored the stress-related genes clpC, clpE, and clpP. The presence of immune modulator genes such as lntA and inlK were harbored in CC8, CC7, CC415, CC9, and CC14 that comprised lineage II. A similar pattern for the presence of genes was followed by CC26, CC18, CC14, CC89, CC415, CC121, and CC381 that comprised lineage II from Switzerland, China, Canada, and USA, whereas these genes were missing from CC2 and CC87 isolates. Additionally, lntA gene was missing from 8% of isolates including CC5, CC59, CC224, and CC388 that comprised lineage I from China and Canada, while the inlK gene was missing from 16% of isolates including CC6, CC4, CC5, and CC3 that comprised lineage I from the USA, Italy, Switzerland, China, and Canada (Figure 4). All the isolates harbored intracellular survival genes lpIA1, oppA, and prsA2, hexose phosphate transporter hbpt2 gene, surface-anchoring lspA, and bile salt hydrolase bsh genes (Figure 4).
Antibiotic Resistance Genes and Plasmid Genes
The antimicrobial susceptibility results showed that all the Chinese isolates were 100% sensitive to eight different antibiotics (shown in Antibiotic Susceptibility Testing section). The prediction of ARGs was performed based on in-house galaxy format. We have found the ARGs in three Chinese isolates (Figure 4). Specifically, one genome of Chinese isolate (CC14/ST91) harbored ARGs to β-lactams (blaTEM-101, blaTEM-105) and macrolide (ermC_15). Furthermore, an isolate (ST7/CC7) also harbored ARGs to aminoglycosides (aadA10_2 and aadA6_1) and quinolones (oqxB_1), while another isolate CC8/ST8 carried aadA6_2 and sul4_1 against aminoglycosides and sulfonamides, respectively. Our results showed that only one Chinese isolate (CC87/ST87) harbored incFIA_1 plasmid gene (Figure 4).
Discussion
L. monocytogenes has the potential to cause invasive listeriosis in humans including septicemia, meningoencephalitis, and maternal–fetal infection leading to abortion. The clinical strains analyzed in this study were recovered from systemic investigation and distributed among different regions and associated with clinical outbreaks; therefore, investigating the VFs provided a unique opportunity for understanding the complete genetic makeup of a particular strain.
Overall, these clinical isolates were grouped into 1/2a, 1/2b, 4b, and 1/2c serotypes (Figure 1A). Serotype 1/2a was prevalent in the Chinese, Canadian, and Swiss isolates, while 1/2c (L0369 isolate) was identified only in the Chinese isolates, and 4b were mostly associated with the Canadian, USA, and Italian isolates. Serotypes 1/2a and 1/2b were likely to possess greater invasion ability than 1/2c (rarely involved) in listeriosis and may cross the placental barrier (Holch et al., 2013). A study reported that 95% of isolates in listeriosis were of serotypes 1/2a, 1/2b, and 4b (Althaus et al., 2014). Most of the isolates were composed of lineage II, followed by lineage I (Figure 1B), which is in accordance with other studies (Chen et al., 2009; Meloni et al., 2009). In this study, most of the clinical isolates belonged to serotype 1/2a that was associated with lineage ll. Additionally, previous studies also witnessed the overrepresentation of lineage ll among clinical isolates. For example, 504 clinical isolates of L. monocytogenes recovered for genomic study during 1958–2010 from Sweden belong to lineage ll (Lopez-Valladares et al., 2017), while 52 epidemiological L. monocytogenes isolates collected between 1981 and 2011 and 41 isolates from lineage ll were reported as the causative agents for the invasive listeriosis outbreak in Canada (Knabel et al., 2012). Furthermore, a study aiming at the evolutionary relationship of invasive listeriosis of 20 outbreak-associated isolates from the USA showed that the majority of L. monocytogenes isolates were from lineage ll (Bergholz et al., 2016). While another investigation showed that 46 invasive listeriosis case outbreaks belong to lineage ll in Austria (Fretz et al., 2010). In China, among 46 clinical strains, 24 strains were found from lineage ll from invasive listeriosis during the study period of 2014–2016 (Zhang et al., 2019).
We have found considerable diversity in STs from clinical sources, which were linked with clinical outbreaks, high case fatality rates, and deaths. For example, the major STs including ST120 (13.33%), ST8 (6.66%), and ST1 (3.33%) (Figure 2A) had been associated mainly with Canadian isolates, while ST87 (5%), ST7 (5%), and ST9 (3.33%) were mainly from China; higher prevalence of these STs was also reported by other studies (Maury et al., 2016; Wang et al., 2018; Zhang et al., 2019). Nevertheless, the application of MLST to subtype L. monocytogenes has provided updated information regarding the population structure within world regions for clinical isolates (Ragon et al., 2008; Chenal-Francisque et al., 2011; Wang et al., 2012).
The MST of STs of different isolates (Figure 3) illustrated that CC8 of Chinese isolates exhibited divergence from Canadian isolates but showed close proximity to European CC8 isolates (Italian and Switzerland). Similarly, CC5 and CC7 of Chinese and Canadian isolates showed a close resemblance. This shows that different strains from a variety of countries might show a similar pattern of genes. The close proximity pattern in relation to distributed genes from different regions also suggests that bacteria may frequently be transported between places of food production, possibly alongside imported raw materials. On the contrary, it is particularly interesting that other genes were conserved among specific CCs, serotypes, and countries.
The presence of adhesive and invasive genes such as inlF, ami, and aut harbored in CC8, CC7, CC415, CC9, and CC14 composed of lineage II (Figure 4). A similar pattern for the presence of genes was followed by CC26, CC18, CC14, CC415, and CC381, which might indicate that these strains have the capacity to cross the blood–brain barrier and fetoplacental barrier. Importantly, the inlF gene encodes for a protein that mediates invasion of the brain of the host by binding with vimentin (Ghosh and Higgins, 2018). Additionally, the virulence gene aut, which encodes for the aut protein, is crucial for the entry of L. monocytogenes to the host cell, unaffected by the regulation of prfA gene (Cabanes et al., 2004). Similarly, ami gene encodes an autolytic amidase with an N-terminal catalytic domain and a C-terminal cell wall-anchoring domain, which has been reported to be involved in the adhesion to eukaryotic cells via its cell wall-binding domain (Milohanic et al., 2001).
Conversely, inlF and aut genes were missing from all Canadian CC1 (n = 5) isolates. Notably, one isolate (02-993) from CC1 showed aberrant features by the presence of these virulence genes. This difference might be due to variation in other genes, as ST relations were based on only seven genes. In our study, inlF was also missing from 33% of isolates belonging to CC2, CC3, CC4, CC5, CC6, CC59, CC87, CC121, CC224, and CC388 composed of lineage I and lineage II (Figure 4). On the other hand, aut seems to be missing from 12% of isolates that belonged to CC2, CC4, CC6, CC121, and CC388 comprising lineage I and II. Moreover, ami seems to be absent from 17% of isolates including CC1, CC2, CC6, CC4, and CC388 that comprised lineage I (Figure 4). Collectively, these findings may suggest that ST1/CC1, ST2/CC2, ST4/CC4, and ST6/CC6 isolates have lost the crucial genes relating to adherence in the host based on the lack of inlF and aut genes. The previous investigation reported that CC1 and CC2 have been verified to be strongly associated with clinical origin particularly human central nervous system (CNS) and maternal–neonatal (MN) listeriosis (Maury et al., 2016).
All the isolates harbored adherence and invasive genes of LIPI-1 (actA, prfA, hlyA, mpl, plcA, plcB) and LIPI-2 (inlA, inlB, inlC, inlJ) (Figure 4). A previous study reported that InlA&InlB helps in binding and invasion (Dramsi et al., 1995) and promotes the entry into the host cell by the process of phagocytosis (Cossart and Toledo‐Arana, 2008). Laterally, the bacteria may further cross the blood–brain barrier and the placental barrier through the hematogenous route. One study (Gouin et al., 2010) reported that InlC dampens the host innate response induced by a pathogen. Furthermore, LLO, a pore-forming toxin that is encoded by HlyA, acts with exo- enzymes (PlcA, PlcB) to facilitate phagosome escape and cell-to-cell spread within the host (Marquis et al., 1995). The master virulence regulator PrfA controls the expression of hlyA and actA genes (Travier et al., 2013). Intracellular motility is dependent upon the ActA protein, which is essential for the polymerization of host F-actin (Kocks et al., 1992).
In the present study, Chinese isolates of CC224, CC3, and ST619 of serotype 1/2b and worldwide isolates belonged to CC1, CC4, and CC6 of serotype 4b that harbored the LIPI-3 genes (Figure 4). These LIPI-3 genes are strongly associated with serotype 4b because of their higher virulence potential by surviving in polymorphonuclear neutrophils (PMNs) (Cotter et al., 2008). These findings further showed the presence of LIPI-3 genes in different CCs belonging to Chinese and worldwide isolates.
Notably, all the isolates harbored stress-related genes, such as clpP, clpE, and clpC. Chinese CC3 isolates (blood, n = 2) lacked crucial virulence gene clpC (Figure 4). It has to be mentioned here that clpC acts synergistically with clpE (another stress gene) that is involved in the expression of virulence (Nair et al., 1999), while Gaillot et al. (2001) witnessed that the clpP gene is essential for intracellular survival in macrophages and modulates LLO-dependent anti-Listeria protection. This showed that CC3 of the Chinese isolates may have lost the crucial genes related to stress.
The presence of immune modulator genes, such as lntA and inlK, were detected in CC8, CC7, CC415, CC9, and CC14, which were composed of lineage II. The lntA gene modulates the interferon responses by playing with chromatin-related mechanism (Lebreton et al., 2011), while the inlK gene (Dortet et al., 2011) plays an important role in the escape of autophagy recognition. A similar pattern for the presence of genes was followed by CC26, CC18, CC14, CC89, CC415, CC121, and CC381, while these genes were missing from CC2 and CC87 isolates (Figure 4). Additionally, lntA genes were missing from 8% of isolates including CC5, CC59, CC224, and CC388 that comprised lineage I, while the inlK gene was missing from 16% isolates including CC6, CC4, CC5, and CC3 that comprised lineage I (Figure 4).
It is worthy to be mentioned that the peptidoglycan modification genes pgdA and oatA were harbored in all isolates except CC3 isolates, where the pgdA gene was absent; this gene protects L. monocytogenes from killing by phagocytes possibly by acetylating the muramic acid residues of peptidoglycan and deacetylates N-acetyl-glucosamine residues (Boneca et al., 2007; Rae et al., 2011), conferring different levels of resistance to antimicrobial peptides and lysozymes. This shows that CC3 Chinese isolates may have lost the crucial genes related to lysozyme, and antimicrobials, which might be trapped by the host defense mechanism during the infection process.
Notably, all the isolates harbored the intracellular survival genes hpt, lpIA1, and prsA2 (Figure 4). Previous findings reported that the lpIA1 gene is necessary for intracellular replication, and LpIA1-dependent utilization of host lipoyl peptides enables L. monocytogenes cytosolic growth and virulence (Keeney et al., 2007). Additionally, PrsA2-like chaperones were reported to assist in the folding of proteins translocated across the bacterial membrane, whereas these proteins were found to be essential for bacterial viability and relevant to host infection (Alonzo and Freitag, 2010). L. monocytogenes possesses hexose phosphate (HP) transporter as a source of carbon and energy that mediates rapid intracellular replication (Chico-Calero et al., 2002).
Moreover, all the isolates carried genes for surface-anchoring lspA and bile salt hydrolase bsh (Figure 4), which help the bacteria to survive under stress encountered within the gastrointestinal tract, including bile. Previous studies reported that LspA is responsible for the maturation of lipoproteins in pathogenesis (Reglier-Poupet et al., 2003), and BSH activity increases under anaerobic conditions, suggesting that anaerobic conditions influence stress responses (Dussurget et al., 2002).
The antimicrobial susceptibility results demonstrated that all the tested isolates (100%) were sensitive to various antibiotics, while the prediction of ARGs showed that three Chinese isolates were resistant (Figure 4). Among the Chinese isolates, those of CC14 harbored the resistance gene (blaTEM-101, blaTEM-105) to β-lactams and (ermC-15) erythromycin. The most common and important mechanism through which bacteria can become resistant against β-lactams is by expressing β-lactamases, for example, extended-spectrum β-lactamases (ESBLs) (Poirel et al., 2007; Queenan and Bush, 2007; Jacoby, 2009). On the other hand, erythromycin resistance is due to the presence of ribosomal RNA (rRNA) methylases, encoded by the erm genes (Roberts et al., 1999; Roberts, 2008). CC7 isolate harbored resistance gene against aminoglycoside (aadA10_2, aadA6_1) and quinolone (oqxB_1). The major encountered aminoglycoside resistance mechanism is due to the modification of enzymes, such as acetyltransferases, acetyltransferases, and phosphotransferases (Wright and Thompson, 1999; Ramirez and Tolmansky, 2010). It has to be mentioned that quinolone resistance may result in either a decreased outer-membrane permeability or mutations of the molecular targets of topoisomerase IV or DNA gyrase enzyme (Hooper, 2000; Ruiz, 2003; Jacoby, 2005; Jiang et al., 2021). The CC8 isolate harbored ARGs against aminoglycoside (aadA6_1) and sulfonamide (sul4_1). Sulfonamide resistance is raised by mutations in the folP gene encoding dihydropteroate synthase (DHPS) enzyme (Skold, 2001; Grape, 2006). These collective findings indicated that Chinese isolates conferred the resistance to important clinical drugs such as β-lactams, aminoglycosides, and erythromycin class; β-lactam class is the primary therapeutic option for human listeriosis together with aminoglycosides (Leong et al., 2016).
Parallelly, L. monocytogenes is known to carry several plasmids that often confer resistance to antimicrobial agents or even increased stress resistance (Kuenne et al., 2013). The analysis of the plasmids identified that none of the isolates in this study carried a plasmid except only CC87 Chinese isolate harbored plasmid incFI_A gene (Figure 4). Taken together, these findings suggest that Chinese clones are somewhat more resistant due to the presence of resistance genes as described previously (Elbediwi et al., 2019; Wang et al., 2019; Elbediwi et al., 2020c; Paudyal et al., 2020) as compared to other worldwide isolates that may pose a significant threat to the public. Therefore, these findings call for prompt action and stress for adapting a one-health approach (Zhou et al., 2020) for formulating the policies on microbiological safety (Paudyal et al., 2018).
We have found through phylogenetic analysis that Chinese and Swiss isolates are closely related and clustered together in the same clade and belonged to the same CC (Figure 4). For example, three CC8 Chinese isolates showed a close resemblance to two Swiss isolates and one Italian isolate (CC8), CC7 of three Chinese isolates phylogenetically related to CC7 of one Canadian isolate, and one CC5 showed a similar pattern of genes as CC5 of the Canadian isolate. On the other hand, CC381 of the Chinese isolates shared a similar pattern with CC26 (Swiss isolate), and CC29 was closely linked with CC37 of the Canadian isolate. This might suggest the possible”antigen switching” from one region to another with the opportunity to adapt and acquire resistance, persistence, and virulence under diverse geographical locations.
Conclusion
These findings demonstrated that CC8, CC7, CC9, and CC415 contained virulence and invasive genes inlF, ami, and aut, while CC1, CC2, CC4, and CC6 lacked these genes. Chinese isolates that belonged to CC8, CC7, CC5, CC81, and CC29 shared similar genomic patterns with other worldwide clones. Furthermore, LIPI-3 genes were harbored in CC3, CC224, and ST619 genomes of the Chinese isolates and CC1, CC4, and CC6 of other worldwide isolates. LIPI-1 and LIPI-2 as well as genes involved in intracellular survival, surface anchoring, and bile salt hydrolase were harbored in all examined isolates. Additionally, the presence of various ARGs and plasmid genes in Chinese clones may pose a serious risk to public health. In a nutshell, uncovering the diversity of VFs and features of antimicrobial resistance of L. monocytogenes for better clinical therapies for invasive listeriosis could improve the patient outcome. The considerable clonal and genomic diversity along with well-recognized virulence-associated genotypes and antimicrobial-resistant determinants was shared among Chinese and worldwide isolates that stress to design and improve the strategies of clinical therapies. Apart from these, factors other than enhanced virulence such as survival, growth characteristics in food-associated environment, and host immune status should also be considered in further investigation.
Data Availability Statement
The datasets generated for this study can be found in NCBI Bioproject number PRJNA688596.
Ethics Statement
Written informed consent was obtained from the individual(s) for the publication of any potentially identifiable images or data included in this article.
Author Contributions
Conceptualization: MY and SX. Investigation: DS, TA, and WC. Validation: DS and WC. Data analysis: TA and HP. Writing-original draft preparation: TA. writing-review and editing: MY and TA. Project administration and funding acquisition: MY and SX. All authors contributed to the article and approved the submitted version.
Funding
This work was supported by the National Program on Key Research Project of China (2019YFE0103900) as well as the European Union’s Horizon 2020 Research and Innovation Programme under Grant Agreement No. 861917–SAFFI, Zhejiang Provincial Natural Science Foundation of China (LR19C180001), Zhejiang Provincial Key R&D Program of China (2021C02008 and 2020C02032), and Opening Fund of Key Laboratory of Microorganism Technology and Bioinformatics Research of Zhejiang Province (2017E10010).
Conflict of Interest
The authors declare that the research was conducted in the absence of any commercial or financial relationships that could be construed as a potential conflict of interest.
Publisher’s Note
All claims expressed in this article are solely those of the authors and do not necessarily represent those of their affiliated organizations, or those of the publisher, the editors and the reviewers. Any product that may be evaluated in this article, or claim that may be made by its manufacturer, is not guaranteed or endorsed by the publisher.
Supplementary Material
The Supplementary Material for this article can be found online at: https://www.frontiersin.org/articles/10.3389/fcimb.2021.718840/full#supplementary-material
Supplementary Table 1 | The name of repositories along with accession number of clinical isolates.
References
Alonzo, F., 3rd, Freitag, N. E. (2010). Listeria Monocytogenes PrsA2 is Required for Virulence Factor Secretion and Bacterial Viability Within the Host Cell Cytosol. Infect. Immun. 78 (11), 4944–4957. doi: 10.1128/IAI.00532-10
Althaus, D., Lehner, A., Brisse, S., Maury, M., Tasara, T., Stephan, R. (2014). Characterization of Listeria Monocytogenes Strains Isolated During 2011-2013 From Human Infections in Switzerland. Foodborne Pathog. Dis. 11 (10), 753–775. doi: 10.1089/fpd.2014.1747
Baldry, S. (2010). Attack of the Clones. Nat. Rev. Microbiol. 8 (6), 390–390. doi: 10.1038/nrmicro2369
Bankevich, A., Nurk, S., Antipov, D., Gurevich, A. A., Dvorkin, M., Kulikov, A. S., et al. (2012). SPAdes: A New Genome Assembly Algorithm and its Applications to Single- Cell Sequencing. J. Comput. Biol. 19 (5), 455–477. doi: 10.1089/cmb.2012.0021
Bergholz, T. M., Den Bakker, H. C., Katz, L. S., Silk, B. J., Jackson, K. A., Kucerova, Z., et al. (2016). Determination of Evolutionary Relationships of Outbreakassociated Listeria Monocytogenes Strains of Serotypes 1/2a and 1/2b by Wholegenome Sequencing. Appl. Environ. Microbiol. 82, 928–938. doi: 10.1128/AEM.02440-15
Bergmann, S., Beard, P. M., Pasche, B., Lienenklaus, S., Weiss, S., Gahan, C. G., et al. (2013). Influence of Internalin A Murinisation on Host Resistance to Orally Acquired Listeriosis in Mice. BMC Microbiol. 13, 90. doi: 10.1186/1471-2180-13-90
Bolger, A. M., Lohse, M., Usadel, B. (2014). Trimmomatic: A Flexible Trimmer for Illumina Sequence Data. Bioinformatics 30, 2114–2120. doi: 10.1093/bioinformatics/btu170
Boneca, I. G., Dussurget, O., Cabanes, D., Nahori, M. A., Sousa, S., Lecuit, M., et al. (2007). A Critical Role for Peptidoglycan N-Deacetylation in Listeria Evasion From the Host Innate Immune System. Proc. Natl. Acad. Sci. U.S.A. 104997- (3), 1002. doi: 10.1073/pnas.0609672104
Burkholder, K. M., Bhunia, A. K. (2010). Listeria Monocytogenes Uses Listeria Adhesion Protein (LAP) to Promote Bacterial Transepithelial Translocation and Induces Expression of LAP Receptor Hsp60. Infect. Immun. 78 (12), 5062–5073. doi: 10.1128/IAI.00516-10
Cabanes, D., Dussurget, O., Dehoux, P., Cossart, P. (2004). Auto, a Surface Associated Autolysin of Listeria Monocytogenes Required for Entry Into Eukaryotic Cells and Virulence. Mol. Microbiol. 51 (6), 1601–1614. doi: 10.1111/j.1365-2958.2003.03945.x
Carroll, L. M., Kovac, J., Miller, R. A., Wiedmann, M. (2017). Rapid, High- Throughput Identification of Anthrax-Causing and Emetic Bacillus Cereus Group Genome Assemblies via BTyper, a Computational Tool for Virulence-Based Classification of Bacillus Cereus Group Isolates by Using Nucleotide-Sequencing Data. Appl. Environ. Microbiol. 83, e1096–e1017. doi: 10.1128/AEM.01096-17
Centorame, P., Acciari, V. A., Orsini, M., Torresi, M., Iannetti, L., Angius, A., et al. (2015). Whole-Genome Sequence of Listeria Monocytogenes Serovar 4b Strain IZSAM_Lm_hs2008, Isolated From a Human Infection in Italy. Genome Announc 3 (2), e00053–15. doi: 10.1128/genomeA.00053-15
Chenal-Francisque, V., Lopez, J., Cantinelli, T., Caro, V., Tran, C., Leclercq, A. (2011). Worldwide Distribution of Major Clones of Listeria Monocytogenes. Emerg. Infect. Dis. 17, 1110–1112. doi: 10.3201/eid/1706.101778
Chen, J., Zhang, X., Mei, L., Jiang, L., Fang, W. (2009). Prevalence of Listeria in Chinese Food Products From 13 Provinces Between 2000 and 2007 and Virulence Characterization of Listeria Monocytogenes Isolates. Foodborne Pathog. Dis. 6 (1), 7–14. doi: 10.1089/fpd.2008.0139
Chico-Calero, I., Suarez, M., Gonzalez-Zorn, B., Scortti, M., Slaghuis, J., Goebel, W., et al. (2002). European Listeria Genome, Consortium Hpt, a Bacterial Homolog of the Microsomal Glucose- 6-Phosphate Translocase, Mediates Rapid Intracellular Proliferation in Listeria. Proc. Natl. Acad. Sci. U.S.A. 99 (1), 431–436. doi: 10.1073/pnas.012363899
Clinical, and Laboratory Standards Institute [CLSI]. (2015). Performance Standards for Antimicrobial Susceptibility Testing: 24th Informational Supplement (M100- S24) (Wayne, PA: Clinical and Laboratory Standards Institute).
Clinical, and Laboratory Standards Institute [CLSI]. (2017). M100 Performance Standards for Antimicrobial Susceptibility Testing (M100) (Wayne, PA: Clinical and Laboratory Standards Institute).
Cossart, P., Toledo-Arana, A. (2008). Listeria Monocytogenes, a Unique Model in Infection Biology: An Overview. Microbes Infect. 10, 1041–1050. doi: 10.1016/j.micinf.2008.07.043
Cotter, P. D., Draper, L. A., Lawton, E. M., Daly, K. M., Groeger, D. S., Casey, P. G., et al. (2008). Listeriolysin S, a Novel Peptide Haemolysin Associated With a Subset of Lineage I Listeria Monocytogenes. PloS Pathog. 4 (9), e1000144. doi: 10.1371/journal.ppat.1000144
Den Bakker, H. C., Desjardins, C. A., Griggs, A. D., Peters, J. E., Zeng, Q., Young, S. K., et al. (2013). Evolutionary Dynamics of the Accessory Genome of Listeria Monocytogenes. PloS One 8 (6), e67511. doi: 10.1371/journal.pone.0067511
Dortet, L., Mostowy, S., Samba-Louaka, A., Gouin, E., Nahori, M. A., Wiemer, E. A., et al. (2011). Recruitment of the Major Vault Protein by Inlk: A Listeria Monocytogenes Strategy to Avoid Autophagy. PloS Pathog. 7 (8), e1002168. doi: 10.1371/annotation/a70544fc-6d8b-4549-921a-9e86557b0ffc
Dramsi, S., Biswas, I., Maguin, E., Braun, L., Mastroeni, P., Cossart, P. (1995). Entry of Listeria Monocytogenes Into Hepatocytes Requires Expression of inIB, a Surface Protein of the Internalin Multigene Family. Mol. Microbiol. 16 (2), 251–261. doi: 10.1111/j.1365-2958.1995.tb02297.x
Dussurget, O., Bierne, H., Cossart, P. (2014). The Bacterial Pathogen Listeria Monocytogenes and the Interferon Family: Type I, Type II and Type III Interferons. Front. Cell. Infect. Microbiol. 4, 50. doi: 10.3389/fcimb.2014.00050
Dussurget, O., Cabanes, D., Dehoux, P., Lecuit, M., Buchrieser, C., Glaser, P., et al. (2002). Listeria Monocytogenes Bile Salt Hydrolase is a PrfA-Regulated Virulence Factor Involved in the Intestinal and Hepatic Phases of Listeriosis. Mol. Microbiology 45, 1095–1106. doi: 10.1046/j.1365-2958.2002.03080.x
Dussurget, O., Pizarro-Cerda, J., Cossart, P. (2004). Molecular Determinants of Listeria Monocytogenes Virulence. Annu. Rev. Microbiol. 58, 587– 610. doi: 10.1146/annurev.micro.57.030502.090934
Elbediwi, M., Li, Y., Paudyal, N., Pan, H., Li, X., Xie, S., et al. (2019). Global Burden of Colistin Resistant Bacteria: Mobilized Colistin Resistant Genes Study. Microorganisms 7 (10), 1980–2018. doi: 10.2139/ssrn.3304294
Elbediwi, A., Pan, H., Biswas, S., Li, Y., Yue, M. (2020a). Emerging Colistin Resistance in Salmonella Enterica Serovar Newport Isolates From Human Infections. Emerg. Microbes Infect. 9 (1), 535–538. doi: 10.1080/22221751.2020.1733439
Elbediwi, C., Pan, H., Zhou, X., Rankin, S. C., Schifferli, D. M., Yue, M. (2020b). Detection of Mcr-9-Harbouring ESBL-Producing Salmonella Newport Isolated From an Outbreak in a Large-Animal Teaching Hospital in the USA. J. Antimicrob. Chemother. 76 (4), 1107–1109. doi: 10.1093/jac/dkaa544
Elbediwi, M., Wu, B., Pan, H., Jiang, Z., Biswas, S., Li, Y., et al. (2020c). Genomic Characterization of Mcr-1-Carrying Salmonella Enterica Serovar 4,[5],12:I:- ST 34 Clone Isolated From Pigs in China. Front. Bioeng. Biotechnol. 8, 663. doi: 10.3389/fbioe.2020.00842
European Centre for Disease Prevention and Control. (2017) Annual Epidemiological Report 2016-Listeriosis. Available at: https://ecdc.europa.eu/en/publications-data/listeriosis-annual-epidemiological-report-2016-2014-data.
European Food Safety Authority, European Center for Disease Prevention and Control. (2012). The European Union Summary Report on Trends and Sources of Zoonoses, Zoonotic Agents and Food-Borne Outbreaks in 2010. EFSA J. 150, 1–44. doi: 10.2903/j.efsa.2012.2597
Fan, Z., Xie, J., Li, Y., Wang, H. (2019). Listeriosis in Mainland China: A Systematic Review. Int. J. Infect. Dis. 81, 17–24. doi: 10.1016/j.ijid.2019.01.007
Farber, J. M., Daley, E. M., MacKie, M. T., Limerick, B. (2000). A Small Outbreak of Listeriosis Potentially Linked to the Consumption of Imitation Crab Meat. Lett. Appl. Microbiol. 31 (2), 100–104. doi: 10.1046/j.1365-2672.2000.00775.x
Feil, E. J., Li, B. C., Aanensen, D. M., Hanage, W. P., Spratt, B. G. (2004). eBURST Inferring Patterns of Evolutionary Descent Among Clusters of Related Bacterial Genotypes From Multilocus Sequence Typing Data. J. Bacteriol. 186 (5), 1518–1530. doi: 10.1128/JB.186.5.1518-1530.2004
Feldgarden, M., Brover, V., Haft, D. H., Prasad, A. B., Slotta, D. J., Tolstoy, I., et al. (2019). Validating the AMRFinder Tool and Resistance Gene Database by Using Antimicrobial Resistance Genotype-Phenotype Correlations in a Collection of Isolates. Antimicrob. Agents Chemother. 63, e483–e419. doi: 10.1128/AAC.00483-19
Fox, E. M., Allnutt, T., Bradbury, M. I., Fanning, S., Chandry, P. S. (2016). Comparative Genomics of the Listeria Monocytogenes ST204 Subgroup. Front. Microbiol. 2057, 1–12. doi: 10.3389/fmicb.2016.02057
Fretz, R., Sagel, U., Ruppitsch, W., Pietzka, A. T., Stöger, A., Huhulescu, S., et al. (2010). Listeriosis Outbreak Caused by Acid Curd Cheese “Quargel”, Austria and Germany 2009. Eurosurveillance 15, 1–2. doi: 10.2807/ese.15.05.19477-en
Gaillot, O., Bregenholt, S., Jaubert, F., Di Santo, J. P., Berche, P. (2001). Stress-Induced ClpP Serine Protease of Listeria Monocytogenes is Essential for Induction of Listeriolysin O-Dependent Protective Immunity. Infect. Immun. 69 (8), 4938–4943. doi: 10.1128/IAI.69.8.4938-4943.2001
Gaillot, O., Pellegrini, E., Bregenholt, S., Nair, S., Berche, P. (2000). The ClpP Serine Protease is Essential for the Intracellular Parasitism and Virulence of Listeria Monocytogenes. Mol. Microbiol. 35 (6), 1286–1294. doi: 10.1046/j.1365-2958.2000.01773.x
Gaulin, C., Currie, A., Gravel, G., Hamel, M., Leblanc, M. A., Ramsay, D., et al. (2014). Summary of 11 Years of Enteric Outbreak Investigations and Criteria to Initiate an Investigation, Province of Quebec Through 2012. J. Food Prot. 77 (9), 1563–1570. doi: 10.4315/0362-028X.JFP-13-530
Ghosh, P., Higgins, D. E. (2018). Listeria Monocytogenes Infection of the Brain. J. Vis. Exp. 140, e58723. doi: 10.3791/58723
Gouin, E., Adib-Conquy, M., Balestrino, D., Nahori, M. A., Villiers, V., Colland, F., et al. (2010). The Listeria Monocytogenes inlC Protein Interferes With Innate Immune Responses by Targeting the IkB Kinase Subunit Ikkα. Proc. Natl. Acad. Sci. U.S.A. 107 (40), 17333–17338. doi: 10.1073/pnas.1007765107
Gouin, E., Mengaud, J., Cossart, P. (1994). The Virulence Gene Cluster of Listeria Monocytogenes is Also Present in Listeria Ivanovii, an Animal Pathogen, and Listeria Seeligeri, a non Pathogenic Species. Infect. Immun. 62 (8), 3550–3553. doi: 10.1128/iai.62.8.3550-3553.1994
Grape, M. (2006). Molecular Basis for Trimethoprim and Sulfonamide Resistance in Gram-Negative Pathogenes. PhD Thesis. Karolinska institute Stockholm, Sweden
Gupta, S. K., Padmanabhan, B. R., Diene, S. M., Lopez-Rojas, R., Kempf, M., Landraud, L., et al. (2014). A New Bioinformatic Tool to Discover Antibiotic Resistance Genes in Bacterial Genomes. Antimicrob. Agents. Chemother. 58 (1), 212–220. doi: 10.1128/AAC.01310-13
Holch, A., Webb, K., Lukjancenko, O., Ussery, D., Rosenthal, B. M., Gram, L. (2013). Genome Sequencing Identifies Two Nearly Unchanged Strains of Persistent Listeria Monocytogenes Isolated at Two Different Fish Processing Plants Sampled 6 Years Apart. Appl. Environ. Microbiol. 79 (9), 2944–2295. doi: 10.1128/AEM.03715-12
Hooper, D. C. (2000). Mechanisms of Action and Resistance of Older and Newer Fluoroquinolones. Clin. Infect. Dis. 31 Suppl 2, S24–S28. doi: 10.1086/314056
Jacoby, G. A. (2005). Mechanisms of Resistance to Quinolones. Clin. Infect. Dis. 41 (Supplement_2), S120–S126. doi: 10.1086/428052
Jacoby, G. A. (2009). AmpC Beta-Lactamases. Clin. Microbiol. Rev. 22 (1), 161–182. doi: 10.1128/CMR.00036-08
Jiang, Z., Anwar, T. M., Peng, X., Biswas, S., Elbediwi, M., Li, Y., et al. (2021). Prevalence and Antimicrobial Resistance of Salmonella Recovered From Pig-Borne Food Products in Henan, China. Food Control 121, 107535. doi: 10.1016/j.foodcont.2020.107535
Jia, B., Raphenya, A. R., Alcock, B., Waglechner, N., Guo, P., Tsang, K. K., et al. (2017). Expansion and Model-Centric Curation of the Comprehensive Antibiotic Resistance Database. Nucleic. Acids Res. 45, D566–D573 doi: 10.1093/nar/gkw1004
Kathariou, S. (2002). Virulence and Pathogenicity, a Food Safety Perspective. J. Food Prot. 65, 1811–1829. doi: 10.4315/0362-028X-65.11.1811
Keeney, K. M., Stuckey, J. A., O’Riordan, M. X. (2007). LplA1-Dependent Utilization of Host Lipoyl Peptides Enables Listeria Cytosolic Growth and Virulence. Mol. Microbiol. 66 (3), 758–770. doi: 10.1111/j.1365-2958.2007.05956.x
Knabel, S. J., Reimer, A., Verghese, B., Lok, M., Ziegler, J., Farber, J., et al. (2012). Sequence Typing Confirms That a Predominant Listeria Monocytogenes Clone Caused Human Listeriosis Cases and Outbreak in Canada From 1988 to 2010. J. Clin. Microbiol. 50 (5), 1748–1751. doi: 10.1128/JCM.06185-11
Kocks, C., Gouin, E., Tabouret, M., Berche, P., Ohayon, H., Cossart, P. (1992). L. Monocytogenes-Induced Actin Assembly Requires the actA Gene Product, a Surface Protein. Cell 68 (3), 521–531. doi: 10.1016/0092-8674(92)90188-I
Kuenne, C., Billion, A., Mraheil, M. A., Strittmatter, A., Daniel, R., Goesmann, A., et al. (2013). Reassessment of the Listeria Monocytogenes Pan-Genome Reveals Dynamic Integration Hotspots and Mobile Genetic Elements as Major Components of the Accessory Genome. BMC Genomics 14 (1), 447. doi: 10.1186/1471-2164-14-47
Lebreton, A., Lakisic, G., Job, V., Fritsch, L., Tham, T. N., Camejo, A., et al. (2011). Bacterial Protein Targets the BAHD1 Chromatin Complex to Stimulate Type III Interferon Response. Science 331 (6022), 1319–1321. doi: 10.1126/science.1200120
Leong, D., Alvarez-Ordóñez, A., Jooste, P., Jordan, K. (2016). Listeria Monocytogenes in Food: Control by Monitoring the Food-Processing Environment. Afr. J. Microbiol. Res. 10, 1–14. doi: 10.5897/AJMR2015.7832
Lomonaco, S., Knabel, S. J., Dalmasso, A., Civera, T., Bottero, M. T. (2011). Novel Multiplex Single Nucleotide Polymorphism-Based Method for Identifying Epidemic Clones of Listeria Monocytogenes. Appl. Environ. Microbiol. 77 (17), 6290–6294. doi: 10.1128/AEM.00429-11
Lomonaco, S., Nucera, D., Filipello, V. (2015). The Evolution and Epidemiology of Listeria Monocytogenes in Europe and the United States. Infect. Genet. Evol. 35, 172–183. doi: 10.1016/j.meegid.2015.08.008
Lopez-Valladares, G., Danielsson-Tham, M. L., Goering, R. V., Tham, W. (2017). Lineage II (Serovar 1/2a and 1/2c) Human Listeria Monocytogenes Pulsed-Field Gel Electrophoresis Types Divided Into PFGE Groups Using the Band Patterns Below 145.5 Kb. Foodborne Pathog. Dis. 14, 8–16. doi: 10.1089/fpd.2016.2173
Marquis, H., Doshi, V., Portnoy, D. A. (1995). The Broad-Range Phospholipase C and a Metalloprotease Mediate Listeriolysin O-Independent Escape of Listeria Monocytogenes From a Primary Vacuole in Human Epithelial Cells. Infect. Immun. 63 (11), 4531–4534. doi: 10.1128/iai.63.11.4531-4534.1995
Maury, M. M., Tsai, Y. H., Charlier, C., Touchon, M., Chenal-Francisque, V., Leclercq, A. (2016). Uncovering Listeria Monocytogenes Hypervirulence by Harnessing its Biodiversity. Nat. Genet. 48 (3), 308–313. doi: 10.1038/ng.3501
McMullen, P., David, G., Gillaspy Allison, F., Gipson, J. B., Linda, D., et al. (2012). Genome Sequence of Listeria monocytogenes 07PF0776, A Cardiotrophic Serovar 4b Strain. J. Bacteriol. 194 (13), 3552–3552. doi: 10.1128/JB.00616-12
Meloni, D., Galluzzo, P., Mureddu, A., Piras, F., Griffiths, M., Mazzette, R. (2009). Listeria Monocytogenes in RTE Foods Marketed in Italy: Prevalence and Automated EcoRI Ribotyping of the Isolates. Int. J. Food Microbiol. 129 (2), 166–173. doi: 10.1016/j.ijfoodmicro.2008.11.014
Milohanic, E., Jonquieres, R., Cossart, P., Berche, P., Gaillard, J. L. (2001). The Autolysin Ami Contributes to the Adhesion of Listeria Monocytogenes to Eukaryotic Cells via its Cell Wall Anchor. Mol. Microbiol. 200139, (5) 1212–24. doi: 10.1111/j.1365-2958.2001.02208.x
Nair, S., Frehel, C., Nguyen, L., Escuyer, V., Berche, P. (1999). ClpE, a Novel Member of the HSP100 Family, is Involved in Cell Division and Virulence of Listeria Monocytogenes. Mol. Microbiol. 31, 185–196. doi: 10.1046/j.1365-2958.1999.01159.x
O’Riordan, M., Moors, M. A., Portnoy, D. A. (2003). Listeria Intracellular Growth and Virulence Require Host-Derived Lipoic Acid. Science 302 (5644), 462–464. doi: 10.1126/science.1088170
Orsini, M., Ordinelli, A., Cornacchia, A., Acciari, V., Centorame, P., Torresi, M., et al. (2019). Whole-Genome Sequence of Listeria Monocytogenes Serovar 1/2a Strain IZSAM_Lm_15_17439_A144, Representative of a Human Outbreak in 2008. Microbiol. Resource Announcements 8 (36), e00074–e00019. doi: 10.1128/MRA.00074-19
Pagotto, F., Ng, L. K., Clark, C., Farber, J. (2006). Canadian Listeriosis Reference Service. Foodborne Pathog. Dis. 3 (1), 132–137. doi: 10.1089/fpd.2006.3.132
Paudyal, N., Pan, H., Liao, X., Li, X., Fang, W., Yue, M. (2018). A Meta-Analysis of Major Foodborne Pathogens in Chinese Food Commodities Between 2006 and 2016. mSphere 15 (4), 187–197. doi: 10.1089/fpd.2017.2417
Paudyal, N., Pan, H., Wu, B., Zhou, X., Zhou, X., Chai, W., et al. (2020). Persistent Asymptomatic Human Infections by Salmonella Enterica Serovar Newport in China. mSphere 5, 3, e00163–e00120. doi: 10.1128/mSphere.00163-20
Pilmis, B., Leclercq, A., Maury, M. M., Moura, A., Bracq-Dieye, H., Thouvenot, P., et al. (2020). Cutaneous Listeriosis, a Case Series of 16 Consecutive Patients Over 25 Years. J. Infect. 80 (2), 232–254. doi: 10.1016/j.jinf.2019.10.004
Poirel, L., Pitout, J. D., Nordmann, P. (2007). Carbapenemases: Molecular Diversity and Clinical Consequences. Future Microbiol. 2 (5), 501–512. doi: 10.2217/17460913.2.5.501
Queenan, A. M., Bush, K. (2007). Carbapenemases: The Versatile Beta- Lactamases. Clin. Microbiol. Rev. 20 (3), 440–458. doi: 10.1128/CMR.00001-07
Rae, C. S., Geissler, A., Adamson, P. C., Portnoy, D. A. (2011). Mutations of the Listeria Monocytogenes Peptidoglycan N-Deacetylase and O-Acetylase Result in Enhanced Lysozyme Sensitivity, Bacteriolysis, and Hyperinduction of Innate Immune Pathways. Infect. Immun. 79 (9), 3596–3606. doi: 10.1128/IAI.00077-11
Ragon, M., Wirth, T., Hollandt, F., Lavenir, R., Lecuit, M., Le Monnier, A., et al. (2008). A New Perspective on Listeria Monocytogenes Evolution. PloS Pathog. 4 (9), e1000146. doi: 10.1371/journal.ppat.1000146
Ramirez, M. S., Tolmasky, M. E. (2010). Aminoglycoside Modifying Enzymes. Drug Resist. Updates: Rev. Commentaries Antimicrobial Anticancer Chemother. 13 (6), 151–171. doi: 10.1016/j.drup.2010.08.003
Reddy, S., Lawrence, M. L. (2014). Virulence Characterization of Listeria Monocytogenes. Methods Mol. Biol. 1157, 157–165. doi: 10.1007/978-1-4939-0703-8_13
Reglier-Poupet, H., Frehel, C., Dubail, I., Beretti, J. L., Berche, P., Charbit, A., et al. (2003). Maturation of Lipoproteins by Type II Signal Peptidase is Required for Phagosomal Escape of Listeria Monocytogenes. J. Biol. Chem. 278 (49), 49469– 49477. doi: 10.1074/jbc.M307953200
Reimer, A., Weedmark, K., Petkau, A., Peterson, C. L., Walker, M., Knox, N., et al. (2019). Shared Genome Analyses of Notable Listeriosis Outbreaks, Highlighting the Critical Importance of Epidemiological Evidence, Input Datasets and Interpretation Criteria. Microb. Genom. 5 (1), e000237. doi: 10.1099/mgen.0.000237
Roberts, M. C. (2008). Update on Macrolide-Lincosamide-Streptogramin, Ketolide, and Oxazolidinone Resistance Genes. FEMS Microbiol. Lett. 282 (2), 147–159. doi: 10.1111/j.1574-6968.2008.01145.x
Roberts, M. C., Sutcliffe, J., Courvalin, P., Jensen, L. B., Rood, J., Seppala, H. (1999). Nomenclature for Macrolide and Macrolide-Lincosamide- Streptogramin B Resistance Determinants. Antimicrob. Agents Chemother. 43 (12), 2823–2830. doi: 10.1128/AAC.43.12.2823
Rouquette, C., de Chastellier, C., Nair, S., Berche, P. (1998). The ClpC ATPase of Listeria Monocytogenes is a General Stress Protein Required for Virulence and Promoting Early Bacterial Escape From the Phagosome of Macrophages. Mol. Microbiol. 27 (6), 1235–1245. doi: 10.1046/j.1365-2958.1998.00775.x
Ruiz, J. (2003). Mechanisms of Resistance to Quinolones: Target Alterations, Decreased Accumulation and DNA Gyrase Protection. J. Antimicrob. Chemother. 51 (5), 1109–1117. doi: 10.1093/jac/dkg222
Self, J. L., Conrad, A., Stroika, S., Jackson, A., Whitlock, L., Jackson, K. A., et al. (2019). Multistate Outbreak of Listeriosis Associated With Packaged Leafy Green Salads, United States and Canad-2016. Emerg. Infect. Dis. 25 (8), 1461–1468. doi: 10.3201/eid2508.180761
Shoai-Tehrani, M., Pilmis, B., Maury, M. M., Robineau, O., Disson, O., Jouvion, G., et al. (2019). Listeria Endovascular Infections Study Group. Listeria Monocytogenes-Associated Endovascular Infections: A Study of 71 Consecutive Cases. The J. Infect. 79 (4), 322–333. doi: 10.1016/j.jinf.2019.07.013
Skold, O. (2001). Resistance to Trimethoprim and Sulfonamides. Vet. Res. 32 (3-4), 261–273. doi: 10.1051/vetres:2001123
Tahoun, A., Abou Elez, R. M. M., Abdelfatah, E. N., Elsohaby, I., El-Gedawy, A. A., Elmoslemany, A. M. (2017). Listeria Monocytogenes in Raw Milk, Milking Equipment and Dairy Workers: Molecular Characterization and Antimicrobial Resistance Patterns. J. Glob Antimicrob. Resist. 10, 264–270. doi: 10.1016/j.jgar.2017.07.008
Tasara, T., Ebner, R., Klumpp, J., Stephan, R. (2015). Complete Genome Sequence of Listeria Monocytogenes N2306, a Strain Associated With the 2013- 2014 Listeriosis Outbreak in Switzerland. Genome Announc 3 (3), e00553–15. doi: 10.1128/genomeA.00553-15
Tasara, T., Klumpp, J., Bille, J., Stephan, R. (2016). Genome Sequences of Listeria Monocytogenes Strains Responsible for Cheese- and Cooked Ham Product-Associated Swiss Listeriosis Outbreaks in 2005 and 2011. Genome Announc. 4 (2), e00106–e00116. doi: 10.1128/genomeA.00106-16
Tasara, T., Weinmaier, T., Klumpp, J., Rattei, T., Stephan, R. (2014). Complete Genome Sequence of Listeria Monocytogene Lm60, a Strain With an Enhanced Cold Adaptation Capacity. Genome Announc. 2 (6), e01248–e01214. doi: 10.1128/genomeA.01248-14
Thomas, M. K., Murray, R., Flockhart, L., Pintar, K., Fazil, A., Nesbitt, A. (2015). Estimates of Foodborne Illness-Related Hospitalizations and Deaths in Canada for 30 Specified Pathogens and Unspecified Agents. Foodborne Pathog. Dis. 12 (10), 820–827. doi: 10.1089/fpd.2015.1966
Travier, L., Guadagnini, S., Gouin, E., Dufour, A., Chenal-Francisque, V., Cossart, P. (2013). ActA Promotes Listeria Monocytogenes Aggregation, Intestinal Colonization and Carriage. PloS Pathog. 9 (1), e1003131. doi: 10.1371/journal.ppat.1003131
Vazquez-Boland, J. A., Kocks, C., Dramsi, S., Ohayon, H., Geoffroy, C., Mengaud, J. (1992). Nucleotide Sequence of the Lecithinase Operon of Listeria Monocytogenes and Possible Role of Lecithinase in Cell-to-Cell Spread. Infect. Immun. 60 (1), 219–230. doi: 10.1128/iai.60.1.219-230.1992
Vázquez-Boland, J. A., Kuhn, M., Berche, P., Chakraborty, T., Domı́nguez-Bernal, G., Goebel, W., et al. (2001). Listeria Pathogenesis and Molecular Virulence Determinants. Clin. Microbiol. Rev. 14, 584–640. doi: 10.1128/CMR.14.3.584-640.2001
Wang, X., Biswas, S., Paudyal, N., Pan, H., Li, X., Fang, W., et al. (2019). Antibiotic Resistance in Salmonella Typhimurium Isolates Recovered From the Food Chain Through National Antimicrobial Resistance Monitoring System Between 1996 and 2016. Front. Microbiol. 10, 985. doi: 10.3389/fmicb.2019.00985
Wang, H., Luo, L., Zhang, Z., Deng, J., Wang, Y., Miao, Y., et al. (2018). Prevalence and Molecular Characteristics of Listeria Monocytogenes in Cooked Products and its Comparison With Isolates From Listeriosis Cases. Front. Med. 12 (1), 104–112. doi: 10.1007/s11684-017-0593-9
Wang, Y., Zhao, A., Zhu, R., Lan, R., Jin, D., Cui, Z. (2012). Genetic Diversity and Molecular Typing of Listeria Monocytogenes in China. BMC Microbiol. 12, 119. doi: 10.1186/1471-2180-12-119
Wright, G. D., Thompson, P. R. (1999). Aminoglycoside Phosphotransferases: Proteins, Structure, and Mechanism. Front. Bio Sci. 4, D9–21. doi: 10.2741/wright
Xu, X., Chen, Y., Pan, H., Pang, Z., Li, F., Peng, X., et al. (2020). Genomic Characterization of Salmonella Uzaramo for Human Invasive Infection. Microb. Genom. 6 (7), mgen000401. doi: 10.1099/mgen.0.000401
Zhang, J., Cao, G., Xu, X., Allard, M., Li, P., Brown, E., et al. (2016). Evolution and Diversity of Listeria Monocytogenes From Clinical and Food Samples in Shanghai, China. Front. Microbiol. 7, 1138. doi: 10.3389/fmicb.2016.01138
Zhang, X., Niu, Y., Liu, Y., Lu, Z., Wang, D., Cui, X., et al. (2019). Isolation and Characterization of Clinical Listeria Monocytogenes in Beijing, China 2014-2016. Front. Microbiol. 10, 981. doi: 10.3389/fmicb.2019.00981
Keywords: Listeria monocytogenes, listeriosis, genetic diversity, virulence factors, antimicrobial resistance
Citation: Shi D, Anwar TM, Pan H, Chai W, Xu S and Yue M (2021) Genomic Determinants of Pathogenicity and Antimicrobial Resistance for 60 Global Listeria monocytogenes Isolates Responsible for Invasive Infections. Front. Cell. Infect. Microbiol. 11:718840. doi: 10.3389/fcimb.2021.718840
Received: 01 June 2021; Accepted: 15 July 2021;
Published: 27 October 2021.
Edited by:
Changyong Cheng, Zhejiang Agriculture and Forestry University, ChinaCopyright © 2021 Shi, Anwar, Pan, Chai, Xu and Yue. This is an open-access article distributed under the terms of the Creative Commons Attribution License (CC BY). The use, distribution or reproduction in other forums is permitted, provided the original author(s) and the copyright owner(s) are credited and that the original publication in this journal is cited, in accordance with accepted academic practice. No use, distribution or reproduction is permitted which does not comply with these terms.
*Correspondence: Min Yue, bXl1ZUB6anUuZWR1LmNu
†These authors have contributed equally to this work