- 1NovaBiotics Ltd, Aberdeen, United Kingdom
- 2Department of Biochemistry, University of Cambridge, Cambridge, United Kingdom
- 3SNIPR Biome, University of Copenhagen, Copenhagen, Denmark
- 4School of Pharmacy and Life Sciences, Robert Gordon University, Aberdeen, United Kingdom
- 5College of Medical, Veterinary & Life Sciences, University of Glasgow, Glasgow, United Kingdom
- 6Proteomics Facility Services, Moredun Research Institute, Penicuik, United Kingdom
Pseudomonas aeruginosa is a major opportunistic human pathogen which employs a myriad of virulence factors. In people with cystic fibrosis (CF) P. aeruginosa frequently colonises the lungs and becomes a chronic infection that evolves to become less virulent over time, but often adapts to favour persistence in the host with alginate-producing mucoid, slow-growing, and antibiotic resistant phenotypes emerging. Cysteamine is an endogenous aminothiol which has been shown to prevent biofilm formation, reduce phenazine production, and potentiate antibiotic activity against P. aeruginosa, and has been investigated in clinical trials as an adjunct therapy for pulmonary exacerbations of CF. Here we demonstrate (for the first time in a prokaryote) that cysteamine prevents glycine utilisation by P. aeruginosa in common with previously reported activity blocking the glycine cleavage system in human cells. Despite the clear inhibition of glycine metabolism, cysteamine also inhibits hydrogen cyanide (HCN) production by P. aeruginosa, suggesting a direct interference in the regulation of virulence factor synthesis. Cysteamine impaired chemotaxis, lowered pyocyanin, pyoverdine and exopolysaccharide production, and reduced the toxicity of P. aeruginosa secreted factors in a Galleria mellonella infection model. Thus, cysteamine has additional potent anti-virulence properties targeting P. aeruginosa, further supporting its therapeutic potential in CF and other infections.
Introduction
Pseudomonas aeruginosa is a versatile and opportunistic human pathogen and often recalcitrant to antibiotic therapy. It is a major cause of ventilator-associated pneumonia (VAP) in hospitals (Koulenti et al., 2009), a less common cause of community acquired pneumonia (CAP) and can also cause infections at other body sites such as wound infections, urinary tract catheter-associated infections and gangrenous diabetic foot ulcer, (Gjødsbol et al., 2006; Nathwani et al., 2014; Trubiano and Padiglione, 2015; Kim et al., 2020). Acute P. aeruginosa pneumonia can occur in immunocompetent patients but susceptible and immunocompromised patients such as those receiving mechanical ventilation, or the very young and old are most at risk. Cystic fibrosis (CF) patients have dysfunctional mucocilliary clearance due to thick, dehydrated mucus, and are at risk of lung colonisation by P. aeruginosa. Despite recent falls in prevalence, it is still a common species found in the sputum of adults with CF (Cystic Fibrosis Foundation Patient Registry: 2018 Annual Data Report, 2019). This Gram negative motile rod-shaped bacterium was thought to be intrinsically resistant to macrolide antibiotics, though recent evidence suggests that the high minimal inhibitory concentrations (MICs) found in in vitro susceptibility testing media were less reflective of antimicrobial activity in physiological conditions (Buyck et al., 2012). Nevertheless, the rising incidence of acquired resistance, particularly to carbapenems has led to its designation as a critical priority pathogen of concern by WHO (World Health Organisation (WHO), 2017).
P. aeruginosa can produce a wide array of virulence factors, such as phenazine pigments, as well as cytotoxins, exotoxins, elastase, phospholipase C, and protease A (Azam and Khan, 2019). In CF, opportunistic isolates sometimes establish chronic infection which can be very difficult to eradicate (Lyczak et al., 2000; Gibson et al., 2003). During early infection P. aeruginosa isolates are usually highly motile, express type III secretion system effector molecules and the LPS O antigen. Adaptation to chronic infection, often seen within the airways of adults with CF, leads toward a mucoid phenotype, alginate production, acquisition of antibiotic resistance and loss of motility (Smith et al., 2006; Mowat et al., 2011). Evolutionary divergence and adaptation to certain microenvironments can even take place within specific parts of the CF lung (Winstanley et al., 2016). The volatile, and highly toxic compound HCN can be detected in the breath of people with CF colonised with P. aeruginosa (Gilchrist et al., 2013; Gilchrist et al., 2015) and its presence in sputum significantly correlates with poorer lung function in individuals with CF and non-CF bronchiectasis (Ryall et al., 2008). Over time, P. aeruginosa colonisation is associated with damage to the lung architecture and declining lung function in CF (Kerem et al., 2014). Most of the virulence traits of P. aeruginosa are secreted factors under the control of complex, overlapping quorum sensing systems which include those regulated by LasR, RhlR, and MvfR (PqsR) transcriptional regulators. Although these systems have been described as hierarchical (with LasR system at the top) and interdependent (Pesci et al., 1999; McGrath et al., 2004; Heeb et al., 2011), LasR loss of function mutations often arise in chronic CF infections and both RhlR and PQS can function independently (Kostylev et al., 2019).
Cysteamine is a simple aminothiol, produced endogenously through the action of pantetheinases such as vanin-1 in the metabolism of co-enzyme A (Pitari et al., 2000) and is a molecule which may have an underappreciated role in immunity to infection (Fraser-Pitt and Mercer, 2021). It is also a therapeutic licensed for many years for the treatment of the lysosomal storage disorder, cystinosis (Schneider et al., 1995). Cysteamine may also have therapeutic potential as both an oral adjunct to standard of care therapy (SOCT) during infectious exacerbations in CF and as an inhaled maintenance therapy adjunct to antimicrobial therapy. It potentiates antibiotic activity in vitro (Charrier et al., 2014; Devereux et al., 2015; Fraser-Pitt et al., 2016; Fraser-Pitt et al., 2018), as well as in murine models of infection (Fraser-Pitt et al., 2018). Oral cysteamine bitartrate, adjunct to SOCT, resulted in statistically significant improvements in patient reported outcome measures and biomarkers of inflammation and infection including reduced white blood cell count, and CRP in patients experiencing an infectious pulmonary exacerbation (Devereux et al., 2020). Cysteamine may also stimulate autophagy, and reduce proteostasis, and some groups have shown that this increases trafficking of the cystic fibrosis transmembrane conductance regulator (CFTR) to the plasma membrane (De Stefano et al., 2014), although other groups have questioned this (Armirotti et al., 2019). Cysteamine has been demonstrated to improve the clearance of intracellular pathogens including P. aeruginosa and Burkholderia cenocepacia from CF macrophages (Ferrari et al., 2017; Shrestha et al., 2017; Shrestha et al., 2020). Cystic fibrosis has recently seen a paradigm shift in terms of care with the introduction of combinatorial modulator therapies which address the underlying cause of the condition (Middleton et al., 2019). Nonetheless, infectious exacerbations and infection remain as major challenges in CF clinical care and there is still a critical need for novel and effective interventions that target CF symptomology. The broad-spectrum antibiotic potentiation, mucolytic activities, and anti-inflammatory properties of cysteamine may be of benefit to all CF genotypes.
Cysteamine interferes with the REDOX balance of the bacterial cell through conversion to the disulphide cystamine, which depletes intracellular NADPH and cellular reducing power. It can also disrupt the metabolism of P. aeruginosa, and it was noted that sub-lethal concentrations of cysteamine could sensitise it to other antimicrobial agents. Cysteamine has a marked effect on pigment production in numerous strains, and the export of pyomelanin from B. cenocepacia (Fraser-Pitt et al., 2018). Cysteamine is a highly reactive small molecule, so the precise bacterial cell target (or more likely, targets) that are responsible for such pleiotropic activity remain to be elucidated. As P. aeruginosa is a versatile pathogen with a highly flexible metabolism (Dolan et al., 2020), extensive metabolic redundancy can hinder the identification of the precise target of antimicrobials used against this organism. Here we explored further the mechanisms of cysteamine-mediated interference in bacterial metabolism and undermining of virulence traits of P. aeruginosa.
Cysteamine has long been known to inhibit the glycine cleavage system found in the mitochondria of eukaryotic cells (Yudkoff et al., 1981) through inhibition of the glycine cleavage system found in the mitochondria (Hayasaka and Tada, 1983). This multienzyme system has a role in glycine and serine catabolism as well as the provision of methyl groups during the biosynthesis of methionine and purines. In mammals the system includes the P-protein, H-protein, L-protein and T-protein - the H-protein is one of the very few lipoylated proteins and the P-protein requires the pyridoxal phosphate (PLP) cofactor. The system is widely evolutionarily conserved in mammalian, plant and many bacterial species. The glycine decarboxylase enzyme is exquisitely sensitive to competitive inhibition by cysteamine with a Ki of 5 µM, which is thought to act via interaction with PLP (Lowry et al., 1986). Cysteamine (in common with some other aminothiols) has long been known to react with PLP (Buell and Hansen, 1960) by forming thiazolidine ring. It is known to interfere with PLP-dependent enzyme kinetics (De Marco et al., 1965), and as an endogenous molecule there has been speculation as to the biological role (Terzouli et al., 1998). P. aeruginosa possesses two copies of glycine dehydrogenase (decarboxylating) genes, gcvP1 and gcvP2. The GcvP1 and GcvP2 proteins of P. aeruginosa are also PLP-dependent enzymes and GcvP2 belongs to the gcs2 cluster required for glycine catabolism (Lundgren et al., 2013). Glycine is the precursor for HCN production, first demonstrated in P. fluorescens (Laville et al., 1998), and the glycine cleavage system has been implicated in HCN production in P. aeruginosa too (Sarwar et al., 2016). Inhibition of glycine catabolism caused by increases in ammonia (a product of the reaction) has been reported to increase the abundance of the glycine substrate which in turn raises the amount utilised to make HCN via the gene cluster hcnABC (Yan et al., 2018).
Here, we demonstrate that cysteamine inhibits glycine utilisation by P. aeruginosa, and that the glycine cleavage system has unexpected inhibitory activity on HCN production. We also show for the first time that cysteamine disrupts chemotaxis and swarming motility which may be linked to disruption of metabolism, but the glycine cleavage system is not the only target. One of the most striking impacts of cysteamine treatment on P. aeruginosa is the inhibition of pigment production. Pseudomonas sp. produce a wide array of pigments, including pyocyanin, a blue REDOX active compound and toxic virulence factor. We have reported qualitative observations on cysteamine-mediated changes in pigment production on a wide range of P. aeruginosa strains previously (Fraser-Pitt et al., 2018) and here we quantify the effects on pyocyanin. P. aeruginosa also secretes the fluorescent green-yellow iron-chelating siderophore, pyoverdine. Pyoverdine is necessary for virulence in numerous infection models and chemical inhibitors of the production of this siderophore have demonstrated potential in mouse models of infection (Kang et al., 2019) therefore we also investigated the impact of cysteamine on pyoverdine fluorescence. Whilst inhibition of biofilm formation by cysteamine has been demonstrated previously against a wide range of bacteria (Charrier et al., 2014; Fraser-Pitt et al., 2016; Fraser-Pitt et al,. 2018), here we demonstrate that it can reduce exopolysaccharide production in an alginate-producing strain and inhibits the induction of biofilm formation by the aminoglycoside, tobramycin. These data illustrate that cysteamine can dysregulate multiple aspects of P. aeruginosa virulence.
Materials and Methods
Unless otherwise stated, chemicals, reagents and media were purchased from Sigma-Aldrich (MO, USA).
Bacterial Strains, Growth Media and Culture Conditions
P. aeruginosa PAO1 a commonly used type strain (Holloway, 1955) was purchased from the American Type Culture Collection. PA14 (UCBPP-PA14) is a clinical burns isolate (Rahme et al., 1995) first sequenced in 2004 (He et al., 2004) and representative of common clonal isolates found worldwide in numerous disease states including CF (Wiehlmann et al., 2007). NH57388A is a stable mucA mucoid isolate derived from CF sputum which produces pyocyanin (Norman et al., 2016) and NH57388B is a non-mucoid quorum-sensing deficient strain that does not produce N-acylhomoserine lactone signalling molecules (Hoffmann et al., 2005).
The ΔgcvP2 PAO1 mutant was created by PCR amplifying flanking regions 800-1000 bp upstream and downstream of the gcvP2 ORF. Upstream and downstream regions were then overlapped and cloned into the suicide vector pEX19Gm using Gibson assembly as described previously (Huang and Wilks, 2017). The resulting deletion plasmid was then transformed into P. aeruginosa PAO1 by electroporation and selected for on LB plates containing 50 µg/ml gentamycin. Deletion mutants were identified via sacB mediated sucrose counter-selection and confirmed by PCR. Primers used are described in Table S1.
Growth media included cation-adjusted Müller-Hinton broth 2 (MHB). M9 minimal media was prepared (Miller, 1972) with 20 mM carbon sources as described previously (Sarwar et al., 2016). Growth was assessed by optical density (O.D.) of 100 µl volumes at 625 nm over time at 37°C in 96-well flat-bottomed plates (Thermo-Fisher, MA, USA) using a Synergy (Biotek, VT, USA) plate reader. When not in routine use strains were cryopreserved in 20% glycerol (80% Müller-Hinton broth) at -80°C for long term storage.
Susceptibility (MIC) and Chemotaxis Experiments
The MIC (minimum inhibitory concentration at which 100% of bacterial growth is prevented) of cysteamine in different media was calculated as described using the CLSI broth microdilution procedure M07-A10 (Wayne, 2015). The standard solid media used to maintain strains was Müller-Hinton agar (MHA) containing 1.5% granulated agar (Melford Laboratories, UK), Chemotaxis swimming and swarming assessments were performed on M9 minimal media containing 0.3% granulated agar, and 20 mM glucose, or 0.2% w/v casamino acids +/- cysteamine at 200 mg/L in 2-compartment plates. Overnight cultures grown in MHB were pelleted, washed and resuspended in M9 minimal salts to achieve uniform OD600 = 0.4 ± 0.02 and 2 µl volumes were spotted onto the plate surface in a sterile class II containment hood prior to incubation at 37°C (Parales and Ditty, 2018). Images were captured at 24 and 48 h using a Nikon D5100 (Japan) at the same conditions and settings (f/5.3, 1/50 s, ISO-1600) and a Samsung Galaxy S20 (Samsung, South Korea) for colour images to demonstrate inhibition in pigment production. Colony area was calculated from colony radii. The experiment was conducted on three occasions.
Determination of Glycine in Culture Supernatants and Bacterial Cell Lysates
Bacterial cultures were prepared in Müller Hinton broth of each strain (PAO1 parent, and ΔgcvP2 strain) using standardised inoculums of 1 in 150 dilution of 0.5 McFarland standard from overnight cultures. Three 10 ml cultures of each strain were used as controls (no exposure to cysteamine) and 3 cultures were treated with 200 mg/L cysteamine. After 24 h at 37°C aliquots of bacterial cultures were serially diluted to determine final cfu/ml and then pelleted at 5,000 g for 5 min. Clarified supernatants were carefully removed without disturbing the pellets and filter sterilised using syringe-driven 0.22 µM PES filter and stored at -80°C prior to analysis. Bacterial pellets were washed 1 x in ice-cold PBS and centrifuged again before being resuspended in 1 ml sterile distilled water and transferred to a heating block and heated at 90°C to lyse bacteria. Lysates were also stored at -80°C prior to analysis. Glycine concentrations were determined fluorometrically using a glycine assay kit ab211000 (Abcam, Cambridge UK) according to the manufacturer’s instructions on a black-walled 96-well microtitre plate and read using Biotek Synergy plate-reader (Biotek, VT, USA). Uninoculated Müller-Hinton broth (both treated and untreated) were used as controls and the addition of cysteamine did not affect the assay or the concentration of glycine in the media which was 2871.42 ±121.63 ng/ml (standard deviation).
Qualitative Hydrogen Cyanide Detection
Volatile HCN production was determined qualitatively via colorimetric detection using Whatman ashless 125 mm diameter filter paper discs (Whatman, UK) cut to fit above the surface of a two-compartment culture dish and impregnated with a solution containing 5 mg of copper (II) ethylacetoacetate and 5 mg 4,4’methylenebis-(N,N-dimethylaniline) in chloroform (Castric and Castric, 1983). Discs were left to dry and stored at 4°C before use. Bacterial lawns were prepared from overnight cultures resuspended to a uniform 0.5 McFarland cell density and seeded onto two-compartment plates containing MHA or MHA with 200 mg/L cysteamine and allowed to dry in a class II containment hood. HCN detection discs were overlain above both compartments and sterile modified microfuge tube lids were used to keep the discs from touching the culture surface and to prevent condensation on the lids. Plates were incubated upright at 37°C. PAO1, and ΔgcvP2 PAO1 cultures tended to produce HCN quicker and were examined 18 h post inoculation, whereas PA14, and NH57388A and B were examined at 24 h. Experiments were conducted on three occasions.
C. elegans Assessment of HCN Mediated Paralysis
C. elegans was maintained under standard conditions (Wood, 1988). Worms were synchronised to achieve a population of young adults using the bleaching method described by Stiernagle (Stiernagle, 2006).
Bacteria were grown overnight on MHA, cultures were then diluted 1:100 in MHA and spread onto new MHA plates with or without the incorporation of cysteamine at the sub-inhibitory concentrations indicated in the agar and cultured for a further 24 h at 37°C. Bacterial lawns were overlain with nitrocellulose membranes in a method adapted from Darby (Darby et al., 1999). Synchronized worms were then transferred to the nitrocellulose membrane and incubated at room temperature for 6 h. Worms were carefully lifted from the hydrophobic nitrocellulose membrane by 100 µl M9 buffer and transferred to optically clear 96-well flat-bottomed plates to be examined for paralysis and scored for survival every hour, for 6 hours, by inspection under an Axiovert 40 CFL Microscope (Zeiss, Germany) at x 100 magnification. Worm paralysis was defined as no movement after mechanical stimulation.
Quantification of Pyocyanin
Pyocyanin was extracted from 12 ml P. aeruginosa cultures grown statically in MHB for 24 hours at 37°C following inoculation with standardised 1:150 dilutions (80 µl) of 0.5 McFarland suspensions of bacterial cells +/- cysteamine at stated concentrations. Following incubation bacterial cells were removed by centrifugation at 5,000 g for 5 min. Supernatants were then transferred to a fresh tube and further clarified by passing through a 0.22 µM PES filter. Pyocyanin was extracted via liquid phase partitioning first with chloroform and then again with 0.2 N HCl and quantified by measuring the absorbance of 100 µl volumes on 96-well flat-bottomed plates at 520 nm using a Synergy plate reader, concentrations were calculated using the extinction coefficient 17.072 (Kurachi, 1958; Essar et al., 1990).
Quantification of Exopolysaccharide Production
The effect of cysteamine on the production of exopolysaccharide by the stable mucoid P. aeruginosa NH57388A was compared with the non-mucoid AHL-deficient NH57388B strain. Exopolysaccharide production was determined in a method adapted from that devised by Ramus (1977). Each 12 ml culture was inoculated with standardised 1:150 dilutions (80 µl) of 0.5 McFarland suspensions of bacterial cells from overnight cultures on MHA plates and cysteamine was added to final concentrations of 2 - 200 mg/L. Cultures were incubated statically for 120 h at 37°C. Following incubation bacterial cells were removed by centrifugation at 5,000 g for 5 min at 4°C, 1 ml volumes were transferred to sterile microfuge tubes and 100 µl of acidified Alcian blue (1 mg/ml) was added which precipitates polyanionic exopolysaccharides such as alginate. Tubes were briefly vortexed (10 sec) to mix before further centrifugation at 5,000 g for 5 min at 4°C which pellets the precipitate. Aliquots of supernatant (100 µl) were then transferred to clear 96-well flat-bottomed plate and absorbance recorded at 610 nm using a Synergy plate reader. EPS concentration is inversely proportionate to absorbance of the unbound dye, and samples were quantified by comparison to a standard curve prepared using known concentrations of sodium alginate.
Biofilm Assay
Attachment of P. aeruginosa Pa14 biomass to 6-well plates was measured using an adapted crystal violet biofilm assay (O’Toole et al., 1999) using standardised inoculum and MHB growth media ± cysteamine and/or tobramycin as described in Figure 4. Planktonic growth in culture supernatants was measured after 24 h at 37°C by transferring 100 µl of each replicate to optically clear microtitre plates and recording absorbance at 625 nm. Crystal violet was released from washed surface-attached cells using 100% ethanol and 100 µl of each replicate transferred to microtitre plates quantified at 595 nm.
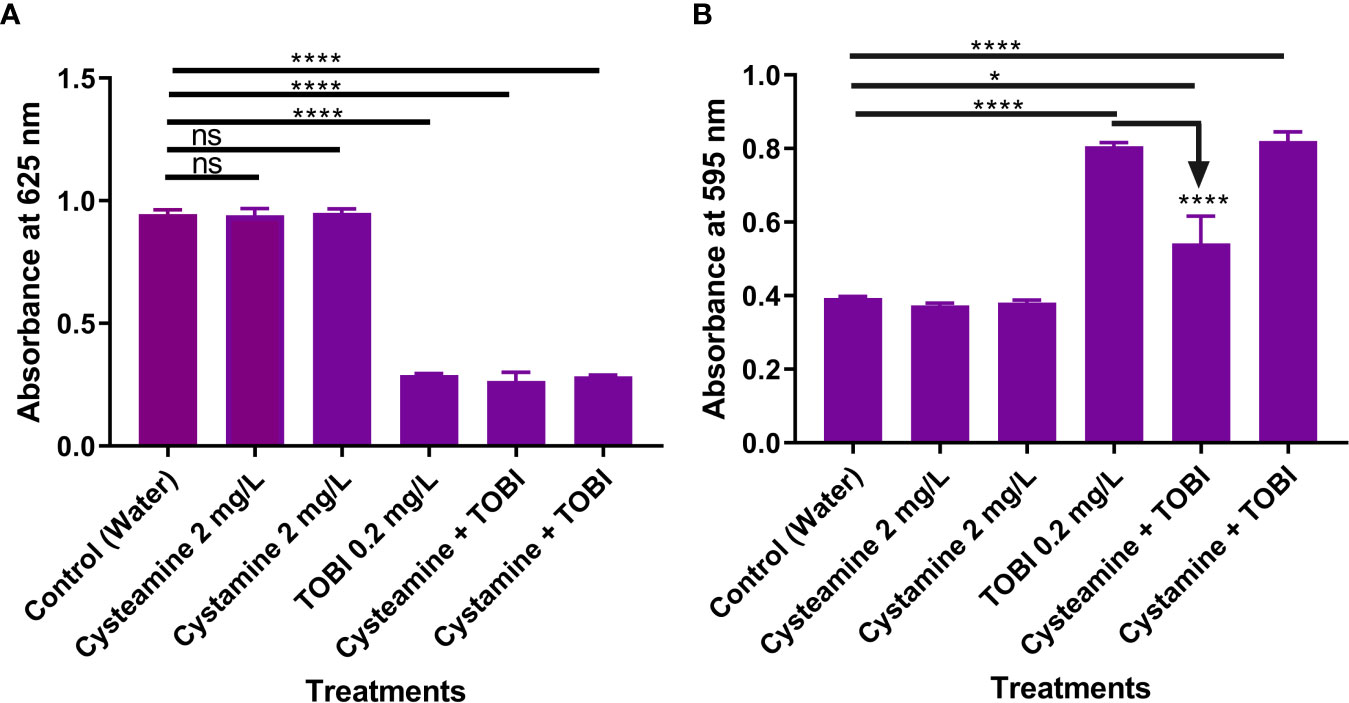
Figure 4 Cysteamine and cystamine do not inhibit growth of planktonic cells (A) at 2 mg/L. Whilst sub-lethal concentrations of tobramycin (TOBI) reduced the number of planktonic cells at 24 h (A) induced biofilm formation over the same time period (B) and this is significantly reduced by adjunct treatment with cysteamine but not the disulfide, cystamine (n=9). ****p ≤ 0.0001 one-way ANOVA followed by Dunnet’s post-hoc analysis. ns, not significant. *p is less than or equal to 0.05.
Fractionation of P. aeruginosa Whole Cell Proteome Liquid Chromatography and Electrospray Ionisation Tandem Mass Spectrometry (LC-ESI-MS/MS) Analysis
Sample Preparation and Separation by SDS PAGE
Six separate 10 ml cultures of P. aeruginosa PAO1 in MHB were prepared in sterile Universal tubes using single colonies grown from frozen stock revived overnight on MHA plates. Cultures were incubated statically for 20 hours and diluted in fresh media if necessary, to achieve growing cultures with an optical density of 0.3 at 625 nm. Each culture was then sub-cultured 1 ml into 9 ml of fresh MHB and incubated for 4 hours at 37°C to achieve mid-logarithmic growth. Bacterial cells were pelleted by centrifugation at 5,000 g for 5 min. Following this, three of these cultures were resuspended in MHB media alone and three were resuspended in media containing sub-inhibitory 250 mg/L cysteamine and all cultures were incubated for a further 4 hours at 37°C. Bacterial cells were then pelleted by centrifugation at 5,000 g for 5 min at 4°C and washed once with ice cold PBS. Bacterial cell pellets were resuspended and lysed in 200 µl of BugBuster (Merck Millipore, MA, USA) containing a protease inhibitor cocktail (Complete Mini, Roche Diagnostics GmbH, Basel, Switzerland) at the manufacturer’s recommended concentration. Lysate protein concentrations were determined by bicinchoninic acid (BCA) assay (Smith et al., 1985) and equal amounts 5 mg total protein of whole cell lysates were separated by one-dimensional sodium dodecyl sulfate polyacrylamide gel electrophoresis (SDS-PAGE) using a precast 4-20% gradient Tris-glycine gel. Gel was visualised with Coomassie based EZBlue (Sigma, MO, USA) stain.
LC-ESI-MS/MS Analysis
Each of 6 lanes of whole cell proteins was fractionated into 21 slices. Each slice was subjected to in-gel tryptic digestion, reduction, alkylation and trypsinolysis, followed by reversed-phase liquid chromatographic separation of tryptic peptides using rapid monolithic column chromatography and ESI-MS/MS using a fast-scanning three-dimensional ion trap tandem mass spectrometer (Bruker Daltonics amaZon-ETD).
LC was performed using an Ultimate 3000 nano-HPLC system (Dionex, LC-Packings) using the method described previously (Burgess et al., 2009).
Mass spectrum peak lists for peptides and fragmentation ions were compared with in-silico published sequence for P. aeruginosa PAO1 utilising the Mascot™ V2.4.1 (Matrix Science) search engine. Mascot search parameters were set in accordance with published guidelines (Taylor and Goodlett, 2005) and to this end, fixed (carbamidomethyl “C”) and variable (oxidation “M” and deamidation “N,Q”) modifications were selected along with peptide (MS) and secondary fragmentation (MS/MS) tolerance values of 0.5Da whilst allowing for a single 13C isotope. Protein identifications obtained from each of the 21 individual gel slices per lane were compiled using the Meta-score protein compilation feature within the ProteinScape bioinformatics platform (Chamrad et al., 2003). From the compiled protein lists individual protein identifications were inspected manually and considered significant only if a) two peptides were matched for each protein, b) peptides were represented by a sequence coverage of >5% and c) each matched peptide contained an unbroken “b” or “y” ion series represented by a minimum of four contiguous amino acid residues. The identification or absence of a protein in each treatment condition is only a semi-quantitative indication of relative abundance of proteins and individual leads need to be confirmed with further analysis.
Preparation of Sterile P. aeruginosa Conditioned Media
P. aeruginosa NH57388A (A) was cultured for 24 h at 37°C in the presence of sub-inhibitory concentrations of cysteamine at 0, 2, 10, 20, 100 and 200 mg/L in cation-adjusted MHB (Thermo-Fisher, MA, USA). Culture supernatants were then clarified by centrifugation at 5,000 g for 5 min and then filter-sterilised by passing through a 0.22 µM syringe-driven PES filter.
Assessment of the Toxicity of Secreted P. aeruginosa Factors Produced in Conditioned Media or Sputum in Galleria mellonella
All Galleria mellonella larvae were purchased from Live Food UK Ltd (Rooks Bridge, UK) and, upon arrival, kept in wood shavings at 20°C in the dark for 3 days prior to challenge. Larvae with approximate weight of 0.25–0.30 g, which were not already melanized were assigned randomly to the different challenge groups or unmanipulated or vehicle control cohorts. Larvae were surface-sterilised with 70% ethanol. Ten microlitre volumes of vehicle control, or conditioned media was injected into the last left proleg as appropriate for each treatment using a 100 microlitre Hamilton syringe which was sterilized with ethanol and larvae were placed into separate sterile petri dishes at 37°C. A melanisation score was determined by observation over time according to criteria described by Kay et al. (2019), adapted from Senior et al. (2011). Insects were considered dead when they become unresponsive to physical stimuli and turned black in colour. The toxicity of P. aeruginosa conditioned media was assessed with 10 larvae per treatment group. Survival was plotted using Kaplan-Meier survival distribution method and tested for statistical significance using Log-rank (Mantel-Cox) test.
Statistical Analysis
All statistical analysis was performed using GraphPad Prism 9.0.0. software using appropriate tests outlined in in the methods section above or in the figure legend.
Results
Cysteamine Inhibited the Utilisation of Glycine in Pseudomonas aeruginosa
Cysteamine has a high minimal inhibitory concentration (MIC) of 500 mg/L in glucose minimal media against P. aeruginosa strains (Fraser-Pitt et al., 2018) and Table S2. Here we show that 200 mg/L has little effect on growth in either the ΔgcvP2 mutant or PAO1 parent strain (Figure 1A). However, cysteamine prevented the utilisation of glycine as a sole carbon source by P. aeruginosa PAO1 (Figure 1B) as treatment with as little as 4 mg/L cysteamine completely prevented growth. As the gcs2 cluster is responsible for glycine utilisation in P. aeruginosa and glycine decarboxylase is the target in mammalian cells we characterised the growth of ΔgcvP2 mutant and PAO1 parent strain in glucose and glycine minimal media. As expected ΔgcvP2 did not grow in glycine minimal media. The addition of high sub-inhibitory concentrations of cysteamine (200 mg/L) to growing cultures of the PAO1 parent and ΔgcvP2 strains in Müller-Hinton broth caused glycine to accumulate in both the supernatant (Figure 1C) and bacterial lysates (Figure 1D), but significant increases were only seen in the parent strain. Levels of glycine, in cysteamine-treated supernatants only, were above those of uninoculated Müller-Hinton broth, indicating that glycine is created during metabolism in this media but that it does not ordinarily accumulate. The ΔgcvP2 strain had higher mean concentrations of glycine in the untreated pellets than the parent strain, though this was not significant (p=0.067).
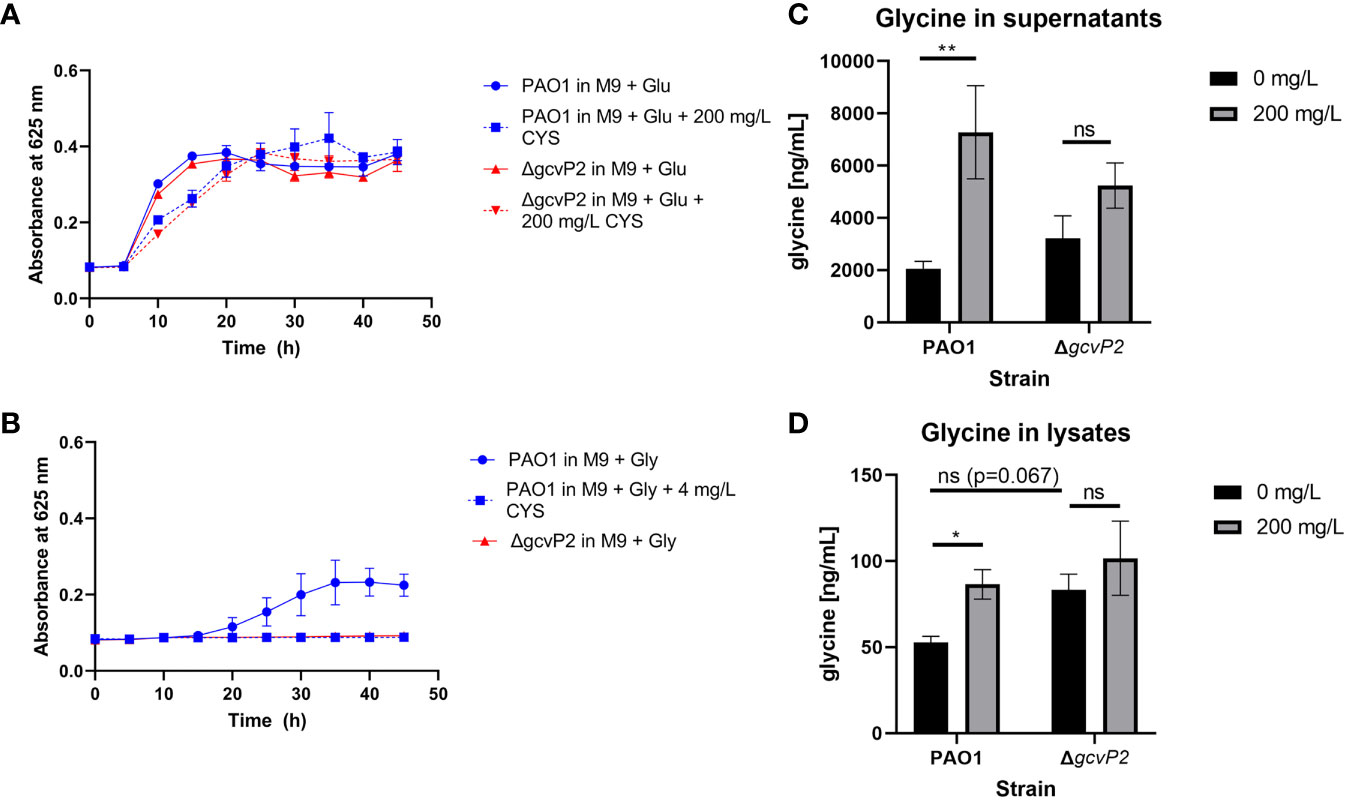
Figure 1 (A) P. aeruginosa PAO1 and ΔgcvP2 growth over time at 37°C in M9 minimal media supplemented with 20 mM glucose with or without the addition of 200 mg/L cysteamine (n=3). (B) Only the PAO1 parent strain can grow in M9 supplemented with 20 mM glycine as the sole carbon source and 4 mg/L cysteamine prevents growth of the parent strain on this carbon source (n=3). (C) Glycine concentrations in bacterial culture supernatants grown for 24 h in cation-adjusted Müller-Hinton broth at 37°C with or without the addition of 200 mg/L cysteamine (n=3). Glycine levels in media alone were unaffected by cysteamine and were calculated as 2871.42 ±121.63 ng/ml (standard deviation). **p ≤ 0.01, two-way ANOVA with Tukey’s test. ns, not significant (D) Glycine concentrations in bacterial lysates from cultures grown for 24 h in cation-adjusted Müller-Hinton broth at 37°C with or without the addition of 200 mg/L cysteamine (n=3). *p ≤ 0.05, Two-way ANOVA with Tukey’s test. ns, not significant.
Electrospray Ionisation Tandem Mass Spectrometry Proteome Analysis of P. aeruginosa Exposed to Cysteamine
Whole cell proteomic analysis was conducted to examine the effect of cysteamine-mediated blockade on glycine utilisation by sub-inhibitory concentrations of cysteamine.
365 proteins were confidently identified by ESI tandem MS according to criteria outlined above. Fifteen proteins were found only in cysteamine-treated conditions across all replicates, and 66 proteins were confidently identified only in control conditions. The remaining 284 were confidently identified in both conditions. Table 1 lists proteins confidently identified only in cysteamine treated P. aeruginosa cells and Table 2 shows proteins only confidently identified in control conditions.
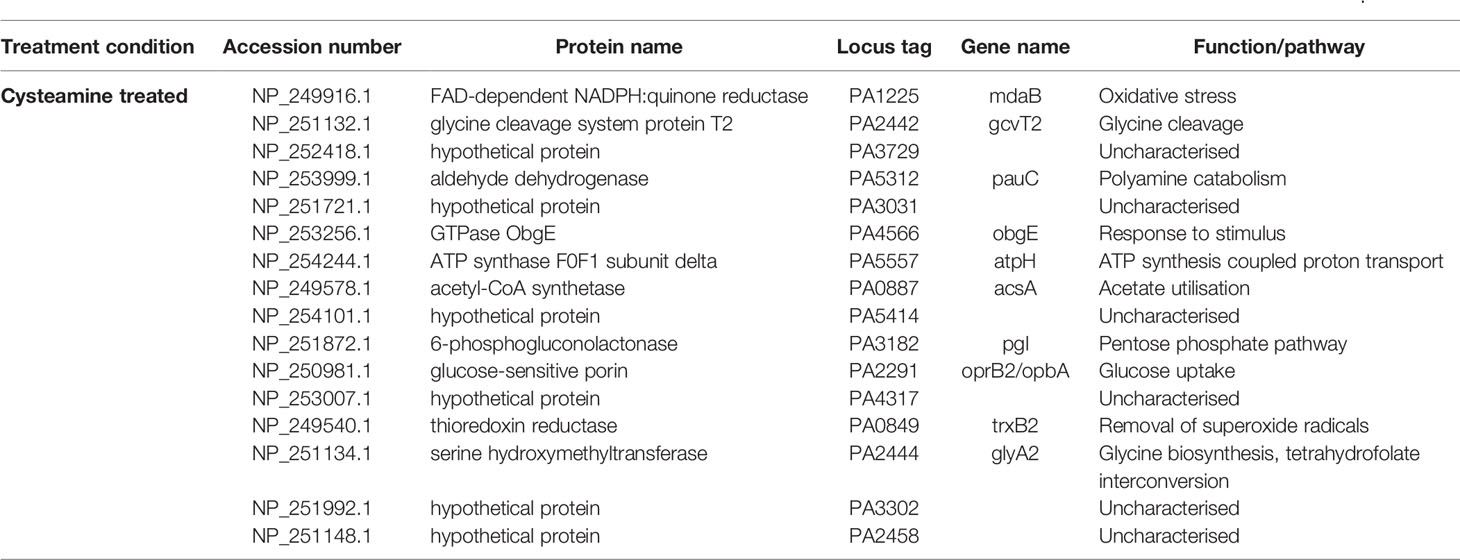
Table 1 Proteins confidently identified only in the cysteamine-treated P. aeruginosa PAO1 cells according to criteria outlined by Taylor and Goodlett, 2005.
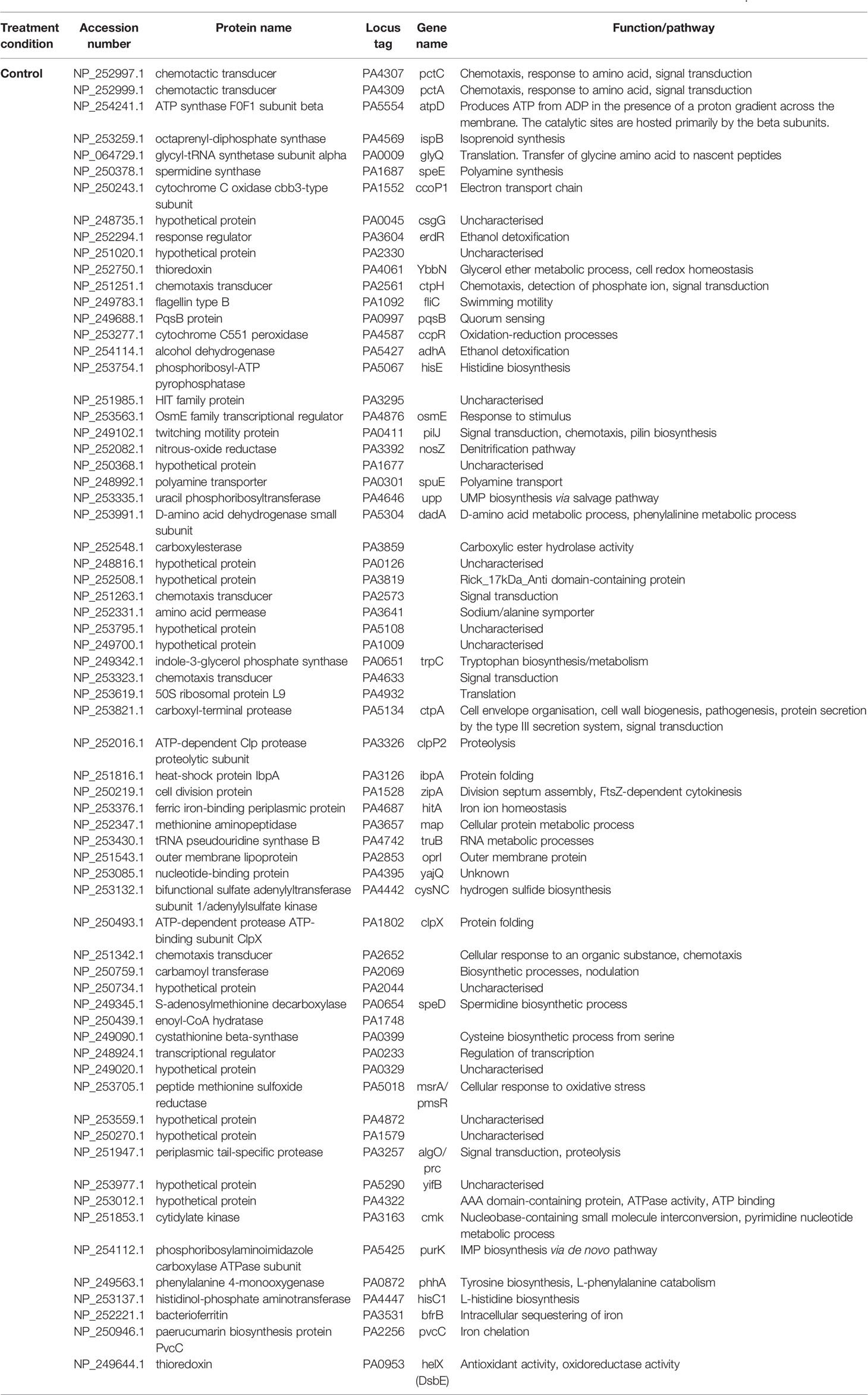
Table 2 Proteins confidently identified only in the control P. aeruginosa PAO1 cells according to criteria outlined by Taylor and Goodlett, 2005.
Although the glycine dehydrogenase (decarboxylating) proteins encoded by gcvP1 and gcvP2 were not amongst the proteins detected with confidence in either cysteamine treated or controls, the neighbouring serine hydroxymethyltransferase (GlyA2) and glycine cleavage system protein T2 (GcvT2) were only confidently detected in cysteamine treated cells, suggesting an increased protein abundance in this condition. The glycyl tRNA synthetase (glyQ) was amongst the proteins found only in the control condition.
Also of interest, the FAD-dependent NADPH:quinone reductase, MdaB (modulator of drug activity B), was identified only in the cysteamine treated condition. This has been implicated in the detoxification of certain compounds and it is linked to quinone and REDOX cycling (Adams et al., 2005). It is not known if this is involved in a shift in NADP : NADPH ratio previously reported to be induced by cysteamine (Fraser-Pitt et al., 2018).
Chemotaxis proteins PctA, PctC, CtpH, PA4633 and PA2652 were amongst those identified only in control conditions. Flagellin (the structural unit of the bacterial flagellum) and the twitching motility protein PilJ, were also only detected in the control condition. This provided the rationale to investigate the potential for cysteamine to inhibit chemotaxis and chemotactic swimming and swarming motility (Figure 2).
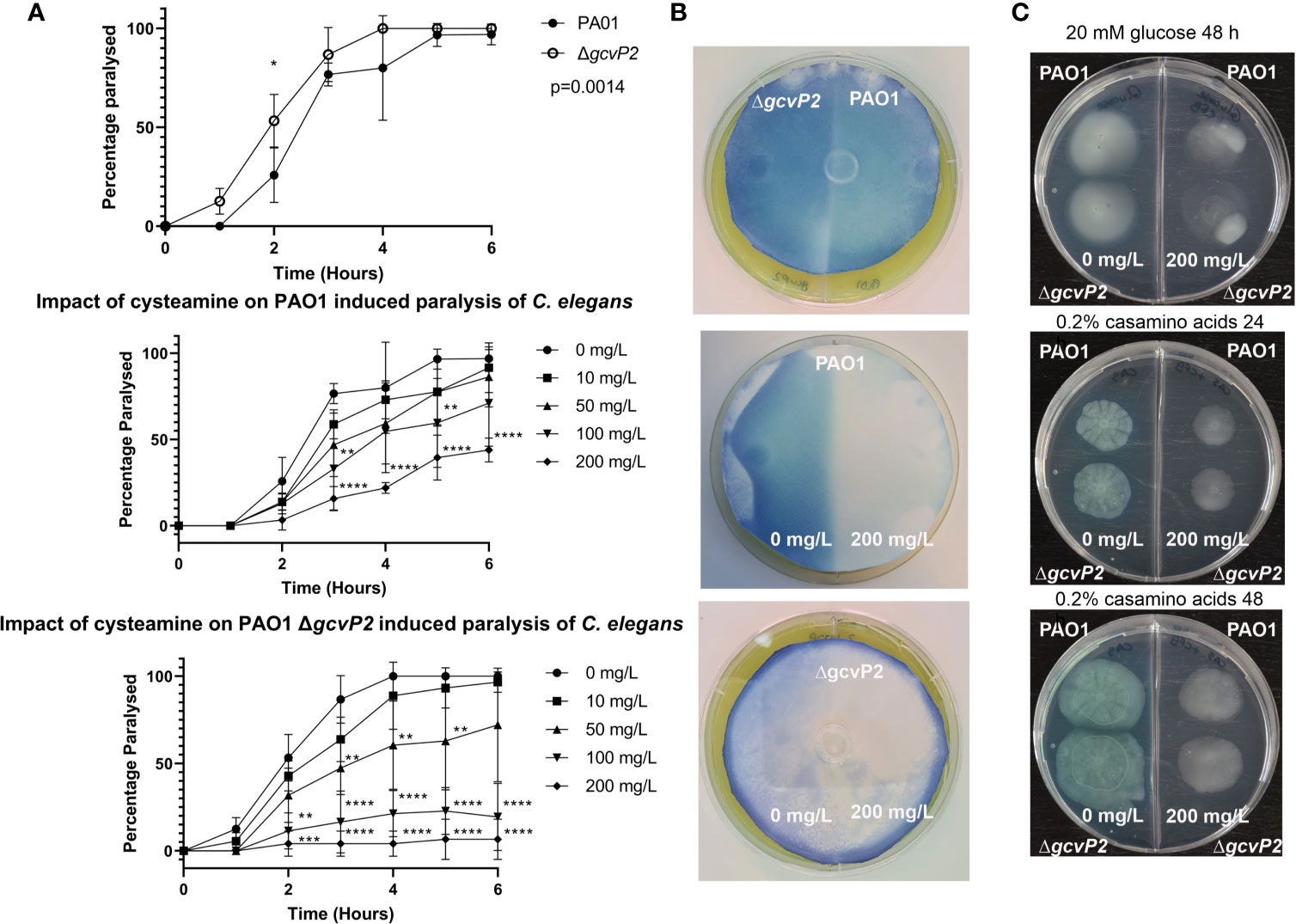
Figure 2 C. elegans paralysis assay, HCN production, and chemotaxis and motility assays. (A) Both the PAO1 parent strain and the ΔgcvP2 mutant induced paralysis in C. elegans over time. The ΔgcvP2 mutant produced significantly higher levels of paralysis than the parent strain. Cysteamine provided dose-dependent protection from paralysis in both mutant and parent strain, suggesting cysteamine inhibited the HCN produced by P. aeruginosa cultures. *p ≤ 0.05, **p ≤ 0.01, ***p ≤ 0.001, ****p ≤ 0.0001 two-way ANOVA and Šidák’s multiple comparisons test. (B) Colorimetric detection of volatile HCN produced by P. aeruginosa PAO1 and ΔgcvP2 mutant grown for the same time (n=3) showed that the mutant liberated more HCN. Experiments on 2-compartment agar plates with either MHA alone (-) or supplemented (+) with sub-inhibitory 200 mg/L cysteamine showed that HCN production in both the mutant and the parent strain were inhibited by cysteamine. (C) Chemotactic swimming motility of both PAO1 and the ΔgcvP2 mutant on 0.3% M9 agar containing 20 mM glucose was inhibited by the addition of 200 mg/L cysteamine at 48 h, as demonstrated by the abolition of concentric rings. Swarming motility, as defined by dendritic outgrowth, was also inhibited on 0.3% agar containing 0.2% w/v casamino acids at 24 h and 48 h, and the effect on pigment production is also evident (Representative images from n=3).
Cysteamine Inhibits HCN Production
Glycine is converted into HCN by P. aeruginosa by the contiguous HCN synthase genes hcnABC. A blockade of glycine cleavage by cysteamine in P. aeruginosa might be expected to raise glycine levels, as it does when administered to rats (Iwama et al., 1997). Mutation in the gcsR gene, a TyrR-like enhancer-binding protein of the alternative sigma factor RpoN (σ54) which regulates the glycine cleavage system in P. aeruginosa PAO1 (Sarwar et al., 2016), was demonstrated to enhance HCN-dependent paralytic-killing in the Caenorhabditis elegans model. HCN production is also known to be under the control of the hierarchical quorum sensing system in P. aeruginosa.
We examined the effects of cysteamine on the production of HCN by the ΔgcvP2 mutant and the parent PAO1 We did this by comparing the ability of PAO1 and ΔgcvP2 to induce paralysis in a C. elegans model (Darby et al., 1999), and examined any effect of the incorporation of cysteamine into the growth media on the ability of each strain to induce paralysis (Figure 2A). We also examined HCN production directly by incorporating sub-inhibitory concentrations of cysteamine into agar plates seeded with lawns of each isolate and detecting volatile HCN produced during culture over time (Figure 2B).
Despite effective blockade of glycine utilisation by cysteamine in P. aeruginosa at 4 mg/L (Figure 1B), the incorporation of cysteamine (at 200 mg/L) into MHA plates surprisingly inhibits the production of HCN, despite having no effect on bacterial growth. The ΔgcvP2 mutant produced more HCN than the PAO1 parent strain over the same incubation time, and induced paralysis in C. elegans quicker than the parent strain, probably due to increased substrate availability. Cysteamine also dose-dependently protected C. elegans from paralysis (Figures 2A, B).
Cysteamine also had a marked effect on chemotaxis on semi-solid (0.3% agar) media containing glucose as the sole carbon source. Radial growth was apparent in control conditions at 48 h for both the parent strain and ΔgcvP2 and both were impacted by the incorporation of cysteamine into the growth media, as surface growth out from the central inoculum was reduced and disorganised. When grown on 0.2% w/v casamino acids medium there is evidence of swarming motility, indicated by dendritic outgrowth from the inoculum, in both mutant and parent strain which is inhibited by cysteamine. The impact on pigment production was also particularly evident when grown on casamino acids (Figure 2C). The inclusion of cysteamine also impacted on the area of growth, with significant reductions in growth area for both the parent strain and ΔgcvP2 mutant on agar containing 0.2% w/v casamino acids at 24 and 48 h. Cysteamine reductions in growth on 20 mM glucose were less evident at 24 h and only significant for the ΔgcvP2 mutant at 48 h (Figure S1).
Inhibition of Exopolysaccharide Production
Exopolysaccharide (EPS) production is important for biofilm formation which is critical for P. aeruginosa chronic infection of the CF lung (Høiby, Ciofu and Bjarnsholt, 2010), at least three exopolysaccharides contribute toward biofilm production, including Psl, Pel and alginate (Reviewed by Ghafoor et al., 2011). Cysteamine significantly inhibited the amount of EPS secreted by mucoid P. aeruginosa strain NH57388A into (and precipitated from) culture media and, as expected, the non-mucoid quorum sensing mutant NH57388B produced significantly less exopolysaccharide than NH57388A (Figure 3A). Cysteamine had a significant effect on the exopolysaccharide secreted by both the alginate-producing NH57388A strain and the non-mucoid NH57388B at high sub-inhibitory concentrations.
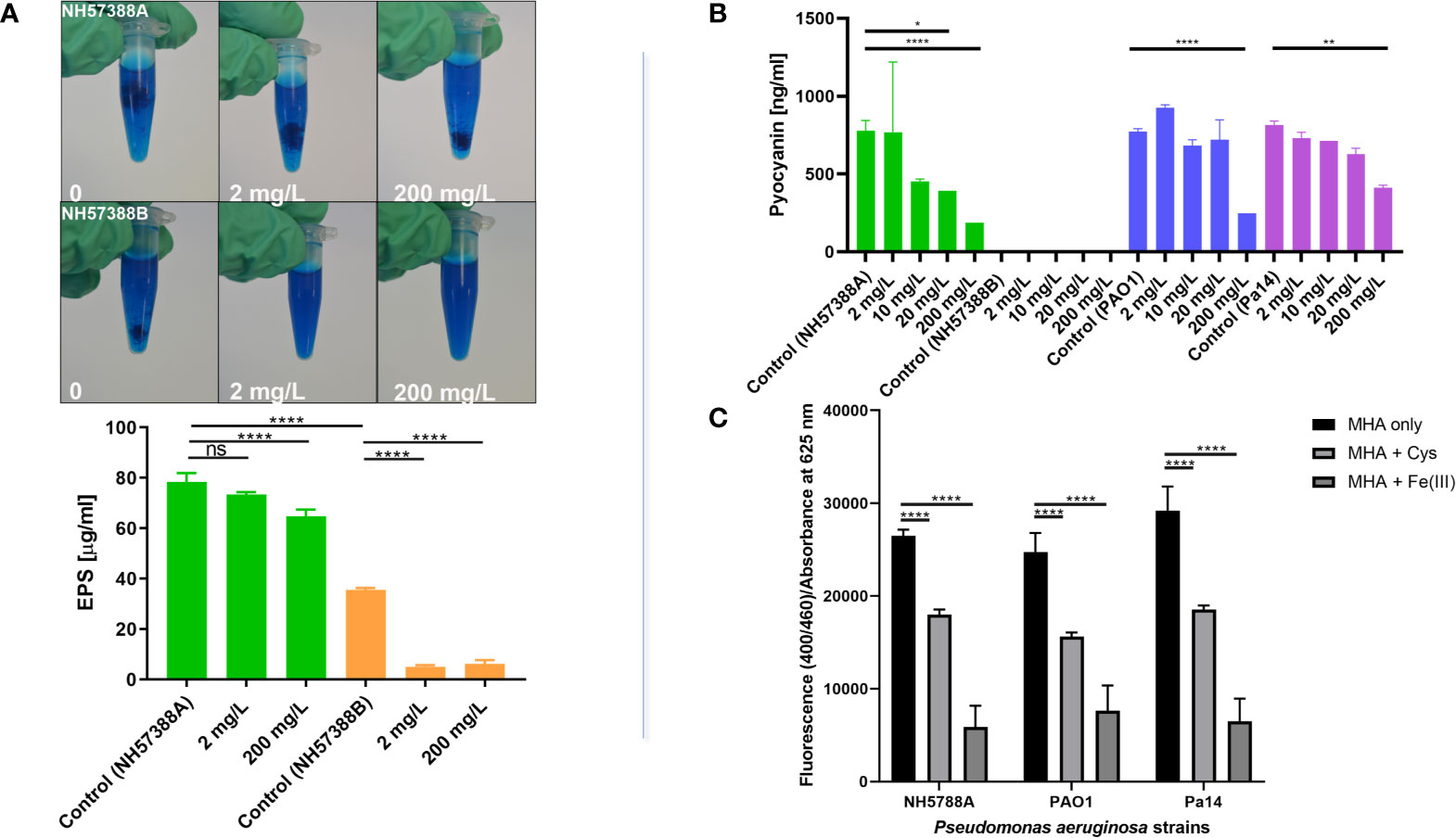
Figure 3 Cysteamine inhibits exopolysaccharide, pyoverdine and pyocyanin production. (A) Exopolysaccharide precipitated from sterile-filtered MHB culture supernatants of P. aeruginosa strains NH57388A and NH57388B with acidified Alcian blue following culture for 48 h at 37°C. Quantification of exopolysaccharide secreted into culture supernatant by NH57388A mucoid strain treated with cysteamine at 2 and 200 mg/L for 48 h at 37°C. ****p ≤ 0.0001 one-way ANOVA and Dunnet’s multiple comparison test, ns, not significant (n=3). Cysteamine had a dose-dependent inhibitory effect on exopolysaccharide secreted from mucoid NH57388A and non-mucoid quorum sensing deficient NH57388B strains. (B) Cysteamine-mediated inhibition of pyocyanin production by P. aeruginosa strains PAO1, PA14 and NH57388A following 20 h treatment at 37°C with the concentration range shown. *p ≤ 0.05, **p ≤ 0.01, ****p = < 0.0001, One-way ANOVA and Tukey’s test (n=3). (C) Inhibition of pyoverdine expression after 20 h growth in MHB by cysteamine at 200 mg/L in multiple strains as detected by fluorescence at 400/460 ex/em normalised to absorbance at 625 nm and compared with quenching induced by excess iron (1 mM Fe(III)Cl3). ****p = < 0.0001, Two-way ANOVA and Tukey’s test (n=3).
Cysteamine Inhibits the Production of Pyocyanin and Pyoverdine
Here we quantify the effect of a range of cysteamine doses on pyocyanin (Figure 3B) secreted by P. aeruginosa PAO1 type strain, NH57388A alginate-producing strain and PA14 clinical strains grown in MHB. We also demonstrate that sub-inhibitory concentrations of cysteamine inhibit pyoverdine fluorescence (Figure 3C).
Cysteamine Reduces Tobramycin-Mediated Biofilm Induction
Aminoglycosides are routinely used for the control of P. aeruginosa in CF patients, including short-term parenteral administration during pulmonary exacerbations and chronic use as inhalation therapies. The tobramycin inhalation powder is safe, efficacious (Geller et al., 2011; Konstan et al., 2011) and has been adopted for routine chronic use for patients colonised with P. aeruginosa for some time, mainly owing to superior convenience, and therefore improved patient compliance, over nebulised therapies (Blasi et al., 2018). Tobramycin inhalation is known to improve lung function over time, and reduce the exacerbation rate (Smith et al., 2018). At the same time exposure to sub-inhibitory concentrations of tobramycin is known to induce biofilm formation by P. aeruginosa (Hoffman et al., 2005) including in CF isolates, independent of minimum inhibitory concentration (MIC) and resistance status (Elliott et al., 2010). RpoN is known to play a role in tobramycin tolerance and biofilm formation (Lloyd et al., 2017; Viducic et al., 2017; Shao et al., 2018).
Here we examined the effect of combining sub-inhibitory concentrations of cysteamine and tobramycin together on the attachment of P. aeruginosa clinical strain PA14 to polystyrene plates using the modified crystal violet method (O’Toole, et al, 1999). Although sub-inhibitory concentrations of some other antibiotics can induce biofilm formation, tobramycin was chosen due to its use as an inhalation maintenance therapy in CF, and PA14 was selected due to clinical relevance. Tobramycin impaired planktonic growth at 0.2 mg/L but encouraged biofilm formation, whereas cysteamine at 2 mg/L had no impact on planktonic growth at all, alone or in combination with tobramycin at this concentration. However, the combination of tobramycin and cysteamine significantly reduced the biofilm formation (Figure 4). The disulfide, cystamine, had no effect on biofilm formation at 24 h.
Cysteamine Inhibited P. aeruginosa Virulence in G. mellonella Model
We have already demonstrated potentiation of ciprofloxacin and tobramycin against P. aeruginosa in different murine infection models (Fraser-Pitt et al., 2018), but the degree to which inhibition of bacterial virulence factor production contributed to enhanced antimicrobial efficacy is difficult to assess and was not examined at the time. The larvae of the wax moth G. mellonella have been developed as a non-mammalian in vivo infection model which has now been used extensively to test antimicrobial agents. P. aeruginosa has strain-dependent virulence in this model, which correlates well to virulence in mice (Jander et al., 2000). Although some P. aeruginosa virulence mechanisms are contact-dependent, many are secreted, so we have assessed the toxicity of sterile-filtered culture supernatants derived from P. aeruginosa strains at equal cell densities, treated with different sub-inhibitory concentrations of cysteamine for 20 h at 37°C prior to injection into the insect haemolymph. The lethality of Pseudomonas infection in G. mellonella can be extreme and varies widely between strains (Cullen et al., 2015). Therefore, we decided to examine sterile-filtered supernatants from cultures of P. aeruginosa, using the less virulent mucoid strain, NH57388A
Exposure to 2, 20, 100 and 200 mg/L cysteamine significantly reduced the toxicity of sterile-filtered supernatants of NH57388A in G. mellonella (Figure 5) and reduced the rapid melanisation seen at 1 h post injection.
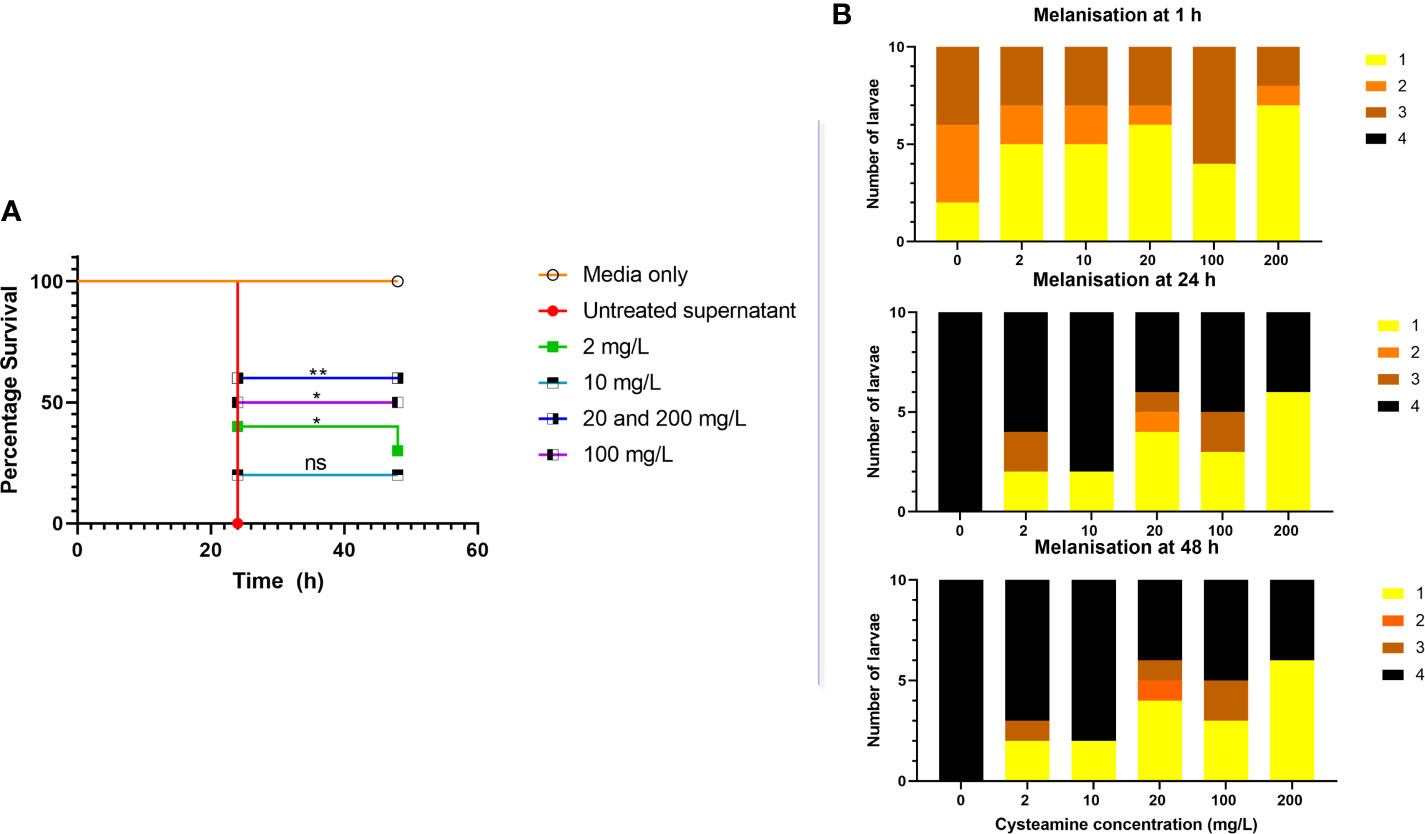
Figure 5 (A) Cysteamine inhibited the toxicity of microbial secreted factors toward G. mellonella produced in culture. P. aeruginosa NH57388A was cultured for 24 h at 37°C in the presence of cysteamine at 0, 2, 10, 20, 100 and 200 mg/L and 10 µl volumes of sterile-filtered supernatants were injected into G. mellonella larvae and survival recorded over 48 h. *p ≤ 0.05, **p ≤ 0.01 log-rank (Mantel-Cox) test, ns, not significant. (B) Melanisation in response to P. aeruginosa secreted factors was rapid as illustrated by the change in melanisation score over time and inhibited by cysteamine. Scoring criteria developed by Kay et al. (2019) are as follows: 1. No signs of melanisation, 2. Signs of nodulation and lateral line melanisation, 3. Systemic melanisation (>50%), 4. Deceased (no movement for 20 min) with full systemic melanisation).
Discussion
Cysteamine was found to inhibit multiple aspects of P. aeruginosa virulence building upon previous data demonstrating antibiofilm activity and the ability to reduce pigment production. Results presented here demonstrate for the first time that cysteamine can inhibit glycine utilisation in prokaryotic cells, but the links between this metabolic disruption and virulence factor production are not known. We also demonstrate inhibition of HCN and exopolysaccharide production and that cysteamine (at therapeutically achievable doses) hinders biofilm formation induced by tobramycin.
Inhibition of Glycine Utilisation and Links to Virulence
Cysteamine inhibits the evolutionary well-conserved glycine cleavage system in mammalian cells. This system is found on the inner membrane of mitochondria in mammalian cells and is composed of H, P, L and T proteins. It has been reported that cysteamine increases the Km for glycine and Vmax for the P-protein (Hayasaka and Tada, 1983), and is reported to prevent both glycine oxidation and synthesis (Yudkoff et al., 1981). P. aeruginosa PAO1 encodes two copies of gcvH, gcvP and gcvT genes and has three copies of the serine hydroxymethyltransferase gene glyA which converts glycine to serine and plays an important role in the generation of one carbon units for methylation as well as (together with serine dehydratase) conversion to pyruvate for utilisation as a sole carbon (or nitrogen) source (Sarwar et al., 2016). The inability to utilise glycine in the presence of small concentrations of cysteamine (Figure 1) could be indicative of a similar blockade.
Inhibition of glycine cleavage could be expected to interfere with bacterial C1 metabolism and methylation. The relative abundance of methyl-accepting chemotaxis proteins (MCPs) may be reduced by cysteamine, with the proteins CtpH, PctA, and PctB are amongst those identified by proteomics only in control conditions (Table 2). The same chemotaxis proteins have been implicated in virulence by Sheng et al., 2019, which demonstrate inhibition of these MCPs (plus CtpM and others) by overexpression of MapZ, a c-di-GMP-dependent inhibitor of methylation of MCPs protected mice in an intraperitoneal model of acute infection. Generation of the second messenger c-di-GMP is directly linked to the glycine cleavage system via serine hydroxymethyltransferase (ShrA/GlyA2) in P. aeruginosa (Pu et al., 2018) and high levels of c-di-GMP are linked to chronic virulence traits including exopolysaccharide production (Lee et al., 2007; Chung et al., 2008), alginate polymerisation and export via Alg44 (Amikam and Galperin, 2006; Merighi et al., 2007; Oglesby et al., 2008), biofilm formation (Hickman et al., 2005), and antibiotic efflux (Nicastro et al., 2014; Poudyal and Sauer, 2018; Wang et al., 2018).
In the proteomics data GcvT2 and GlyA2 were only identified in the cysteamine-treated condition (Table 1). In combination with a lack of detectable glycyl tRNA synthetase (GlyQ) in cysteamine treated conditions, it is tempting to speculate that these alterations are a direct feedback response to the inhibition of glycine decarboxylation, and the corresponding increase in glycine abundance (Table 2). Whilst we confirmed cysteamine has impacts on swimming and swarming chemotactic behaviours (Figure 2) there was an equal effect on both the ΔgcvP2 and parent strain. P. aeruginosa possesses two glycine cleavage operons therefore it could be that any impact on chemotaxis in the ΔgcvP2 mutant is compensated by secondary activity of gcvP1, or that cysteamine disrupts chemotaxis via a different mechanism. The measurement of glycine in bacterial lysates and supernatants confirmed cysteamine-mediated disruption of glycine utilisation as glycine was increased in cysteamine treated PAO1 cells (Figures 1C, D). Although the increase was only significant in the parent strain, mean increases in glycine were seen in the mutant also, which may suggest the gcvP1 has residual activity impaired by cysteamine treatment and this is worthy of future investigation.
The glycine cleavage system was initially linked to the production of pyocyanin via the TyrR-like enhancer-binding protein (EBP) GcsR (PA2449) believed to regulate the gsc2 operon, which includes gcvH2, gcvP2, glyA2, sdaA, and gcvT2 encoding some of the proteins mentioned above found in cysteamine-treated cells (Lundgren et al., 2013). However, this was subsequently disproved by the same group who determined the inhibition of pyocyanin in the initial study was due to unrelated mutations. This study confirmed the EBP nature of GcsR which enhances the transcriptional activity of RpoN upstream of the gsc2 operon. As discussed above, the ΔgscR strain increased the paralytic killing of C. elegans postulated to be caused by diverting glycine into HCN production, although the authors did not look at HCN production directly and saw no changes in expression of hcnABC genes (Sarwar et al., 2016). Our study shows the ΔgcvP2 strain does indeed produce more HCN than the parent strain, confirming increased substrate availability can drive increases in hcnABC conversion of glycine to HCN. It is therefore surprising that we see cysteamine-mediated decreases in HCN production and reduced paralysis in C. elegans, (Figure 2) and reduced virulence in the G. mellonella model (Figure 5) despite inhibition of glycine catabolism.
Transcriptional control of hcnABC, which converts glycine into HCN and CO2, is complex (Figure 6). HCN formation is greater under low oxygen tension and the anaerobic regulator of arginine deiminase and nitrate reductase (ANR) protein is one of a few transcriptional regulators which influence hcnABC (Zimmermann et al., 1991; Laville et al., 1998). ANR, and the E. coli homologue FNR, sense oxygen via an iron-sulfur cluster, and DNA binding is maximal under reducing conditions (Ye et al., 1995; Lazazzera et al., 1996), and iron availability also impacts HCN expression via ANR which is abolished in P. fluorescens when iron is depleted (Blumer and Haas, 2000). The quorum sensing response activators LasR and RhlR also regulate HCN production in P. aeruginosa (Latifi et al., 1995; Winson et al., 1995; Reimmann et al., 1997; Whiteley et al¸1999) and can act in concert to maximise HCN production (Pessi and Haas, 2000). The GacA two-component system via two regulatory RNAs, RsmZ and RsmY, also influences HCN expression indirectly via quorum sensing due to enhanced expression of rhlI, or directly post-transcriptionally, by enhancing the translation of hcnABC (Pessi and Haas, 2001).
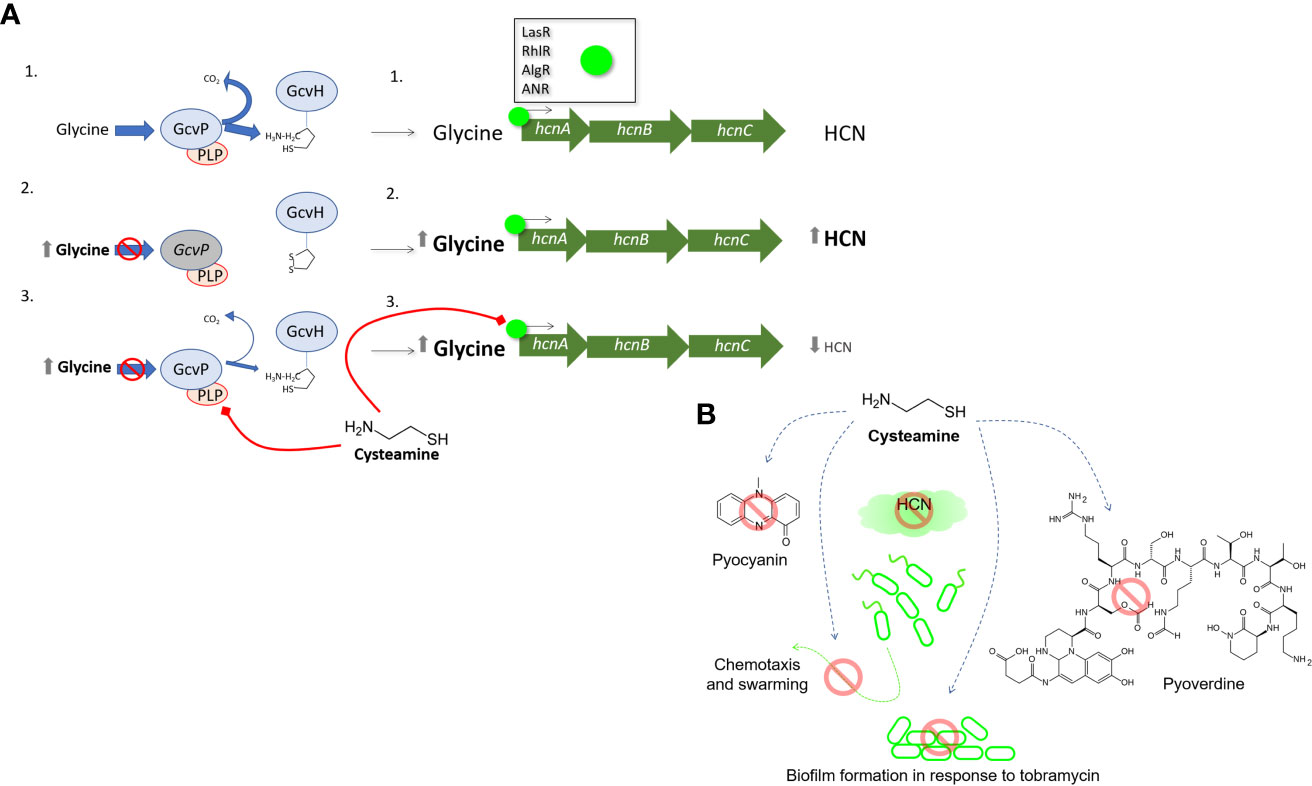
Figure 6 The effect of cysteamine on HCN production (A) provides clues to the anti-virulence activities of cysteamine. In untreated P. aeruginosa 1. Glycine cleavage is uninterrupted and normal levels of glycine are available as precursors for hcnABC gene cluster for the generation of HCN which is under varying levels of regulatory control by LasR, RhlR, AlgR, MvfR and ANR. In scenario 2. inactivation of glycine decarboxylase (GcvP2) prevents glycine utilisation, and higher concentrations of glycine are available for the synthesis of HCN by HcnABC and an increase of HCN is detected. In scenario 3. cysteamine reversibly inactivates GcvP2 via thiazolidine formation with the cofactor PLP. More glycine is available for HCN synthesis, but less HCN synthesis occurs, indicating cysteamine also impairs the regulation of one or more of the regulators listed above. Panel (B) summarises the anti-virulence activities reported in this manuscript.
Therefore, we have uncovered that cysteamine blocks the glycine cleavage system and this should, in theory, increase HCN production as shown with the ΔgcvP2 mutant. Instead, cysteamine must also simultaneously act upon one or more of the P. aeruginosa global regulatory systems to impair HCN production, despite the presumed backup of cellular glycine. These data highlight that precursor availability is a key cue in HCN production in P. aeruginosa, yet a cue which is still subordinate to the additional regulatory mechanisms detailed above. Thus, the dissection of a single, core metabolic target of cysteamine has unexpectedly shed light into the regulatory hierarchy of HCN production in P. aeruginosa – a vital virulence factor in this organism.
Inhibition of Multiple Virulence Factors by Cysteamine
Quorum sensing systems coordinate the production of various virulence factors in P. aeruginosa including the production of phenazines. Our previous work demonstrated inhibition of pigment production in multiple P. aeruginosa strains and inhibition of the export of the pigment pyomelanin in B. cenocepacia strains (Fraser-Pitt et al., 2018). Here we used the chloroform extraction method to confirm the dose-dependent reduction in pyocyanin production by P. aeruginosa in response to cysteamine. Similarly, cysteamine interferes with the production of the fluorescent, iron-chelating pyoverdine virulence factor. Pyoverdine production has been described as essential for virulence in in vivo models both with mutants of type strains (Meyer et al., 1996) and in CF isolates (Kang et al., 2019).
Anecdotal evidence from the CARE-CF-1 trial led us to also examine the effect of cysteamine on exopolysaccharide production. In the trial, a dominant pyomelanin-producing sputum isolate of P. aeruginosa was mucoid at day 0, (before treatment) and non-mucoid at day 14 following exposure to cysteamine. It is already known that the thiol, N-acetylcysteine (already used as a mucolytic treatment in CF), can reduce extracellular polysaccharide production in various bacteria (Olofsson et al., 2003). Cysteamine also demonstrated a significant, though modest, reduction in secreted exopolysaccharide produced by the mucoid alginate-producing NH57388A strain.
The anti-biofilm activity of cysteamine has been reported before. High concentrations can disrupt biofilms, whereas sub-MIC concentrations can prevent biofilms by P. aeruginosa and B. cepacia complex strains (Charrier et al., 2014; Fraser-Pitt et al., 2016; Fraser-Pitt et al., 2018). Here we demonstrate that concentrations of cysteamine that are therapeutically achievable via systemic dosing can also reduce the biofilm promoting activity of another agent, tobramycin. This antibiotic is frequently employed prophylactically in adults with CF known to be colonised with P. aeruginosa to suppress their growth, but sub-lethal concentrations of the antibiotic have long been known to encourage biofilm formation (Hoffman et al., 2005). A recent study (Tahrioui et al., 2019) demonstrated the adaptive response mechanism is thought to involve extracellular DNA release, PrrF small regulatory RNAs and quorum sensing via the pseudomonas quinolone signal (PQS).
The results above show cysteamine was capable of disrupting metabolism and a number of secreted virulence factors in P. aeruginosa. This was confirmed using the G. mellonella invertebrate model. Larvae melanised rapidly in response to P. aeruginosa NH57388A supernatants and died within 24 h. Cysteamine provided some protection against this toxic response and the results were evident quickly due to the effect on reducing melanisation at 1 h. Most deaths in the larvae injected with supernatants from treated P. aeruginosa occurred at 24 h with few thereafter indicating a protection from toxicity.
In conclusion, cysteamine inhibits glycine utilisation and dysregulates virulence factor production in P. aeruginosa at sub-inhibitory concentrations and reduces the secretion of toxic factors by P. aeruginosa. The strategic development of novel therapeutics which have anti-virulence properties and operate differently to conventional antibiotics has been described as essential for our progress in combating antimicrobial resistance (Fleitas Martínez et al., 2019). Compounds which can improve the effectiveness of other agents and at the same time reduce virulence factor production at sub-lethal concentrations are less likely to drive the emergence of resistance (Vale et al., 2016; Munguia and Nizet, 2017). As an endogenously produced molecule, expressed at sites of inflammation and infection, it maybe that cysteamine has an underappreciated role in innate defence against infection. In common with many innate immune effectors, it may work best in concert with other agents, particularly at therapeutic doses where the effects on individual virulence factors (except for the reduction in tobramycin mediated biofilm induction) are less easy to demonstrate. However, the ability to reduce several virulence factors at once in this versatile pathogen likely means the whole effect is greater than the sum of its parts. There is clearly more than one bacterial cell target for cysteamine and given the common effects on eukaryotic and prokaryotic glycine cleavage systems, cysteamine will affect both the host and the pathogen. Critical regulatory systems in P. aeruginosa are clearly disrupted by cysteamine and a systems-based approach may be useful in further understanding the impact of this aminothiol on microbial virulence and metabolism. The positive impact of cysteamine on several virulence factors in this still-common CF pathogen combined with the multivalent properties of this molecule (mucolysis, antibiotic-potentiation etc.) add to the potential utility for this endogenous molecule as a therapeutic in CF, and potentially beyond as we continue the search for agents to tackle the antimicrobial resistance crisis.
Data Availability Statement
The original contributions presented in the study are publicly available. This data can be found here: [https://www.ebi.ac.uk/pride/archive/projects/PXD027156].
Author Contributions
DF-P is the corresponding author and wrote the original draft and submitted manuscript, had roles in supervision, conceptualisation, investigation, data curation and analysis of the project. SD made significant contributions to the conceptualisation of the project and was involved in investigation and analysis of data and reviewing/editing of the manuscript. DT-A is an investigating scientist and was involved in generating data and analysis and reviewing/editing. JH was involved in C. elegans investigation and data analysis. DS has made significant contributions to conceptualisation of the project and in G. mellonella method development. NL-R was a visiting student involved in investigation, data curation and analysis. PN was a visiting student involved in investigation, data curation and analysis. TS provided expertise for C. elegans method development and the review/editing of the manuscript. EM provided mass spectrometry expertise, and was involved in investigation and data generation. KM provided mass spectrometry expertise and was involved in investigation and data generation, reviewing/editing. NI supervised proteomics investigation. DM conceptualisation, review/editing. DO supervision, conceptualisation, review/editing. All authors contributed to the article and approved the submitted version.
Funding
We would like to thank the Society for Applied Microbiology (Sfam) for funding the proteomics work contained in this manuscript via a New Lecturer Award (awarded to DF-P whilst a Lecturer at Edinburgh Napier University) in 2011. NL-R and PN had student placements at NovaBiotics Ltd and were generously supported by Equate Scotland Careerwise programme for women in STEM. SD was generously supported by a BBSRC Flexible Talent Mobility Account 2020-2021. All other work was commercially funded by NovaBiotics Ltd. NovaBiotics Ltd provided support in the form of salaries for authors DF-P, DT-A, JH, DS, DM, and DO.
Conflict of Interest
DF-P, DT-A, JH, DS, and DM are all employees of NovaBiotics Ltd. DAO is the CEO, CSO and a shareholder of NovaBiotics Ltd. DF-P and DO are authors of patent; WO2016198842A1, IL256162D0 - An amino thiol for use in the treatment of an infection caused by the bacterium mycobacterium spp. DO is also author of the following patents; US9364491B2 (and other territories) - Antimicrobial compositions with cysteamine/A composition comprising an antibiotic and a dispersant; WO2016046524A1 - Use of cysteamine in treating infections caused by yeasts/moulds; US9782423B2 (and other territories) - Antibiotic compositions comprising an antibiotic agent and cysteamine; US9339525B2 (and other territories) - Inhibition of biofilm organisms; and US20190175501A1 pending (and other territories) - Microparticles comprising a sulphur-containing compound. This does not alter our adherence to Frontiers policies on sharing data and materials.
The remaining authors declare that the research was conducted in the absence of any commercial or financial relationships that could be construed as a potential conflict of interest.
Publisher’s Note
All claims expressed in this article are solely those of the authors and do not necessarily represent those of their affiliated organizations, or those of the publisher, the editors and the reviewers. Any product that may be evaluated in this article, or claim that may be made by its manufacturer, is not guaranteed or endorsed by the publisher.
Supplementary Material
The Supplementary Material for this article can be found online at: https://www.frontiersin.org/articles/10.3389/fcimb.2021.718213/full#supplementary-material
References
Adams, M. A., Iannuzzi, P., Jia, Z. (2005). Mdab From Escherichia Coli: Cloning, Purification, Crystallization and Preliminary X-Ray Analysis. Acta Crystallogr. Sect. F Struct. Biol. Cryst Commun. 61 (Pt 2), 235–238. doi: 10.1107/S1744309105001545
Amikam, D., Galperin, M. Y. (2006). Pilz Domain is Part of the Bacterial C-Di-GMP Binding Protein. Bioinformatics 22 (1), 3–6. doi: 10.1093/bioinformatics/bti739
Armirotti, A., Tomati, V., Matthes, E., Veit, G., Cholon, D. M., Phuan, P. W., et al. (2019). Bioactive Thymosin Alpha-1 Does Not Influence F508del-CFTR Maturation and Activity. Sci. Rep. 9 (1), 10310. doi: 10.1038/s41598-019-46639-1
Azam, M. W., Khan, A. U. (2019). Updates on the Pathogenicity Status of Pseudomonas Aeruginosa. Drug Discov Today 24 (1), 350–359. doi: 10.1016/j.drudis.2018.07.003
Blasi, F., Carnovale, V., Cimino, G., Lucidi, V., Salvatore, D., Messore, B., et al. (2018). Treatment Compliance in Cystic Fibrosis Patients With Chronic Pseudomonas Aeruginosa Infection Treated With Tobramycin Inhalation Powder: The FREE Study. Respir. Med. 138, 88–94. doi: 10.1016/j.rmed.2018.03.034
Blumer, C., Haas, D. (2000). Iron Regulation of the Hcnabc Genes Encoding Hydrogen Cyanide Synthase Depends on the Anaerobic Regulator ANR Rather Than on the Global Activator Gaca in Pseudomonas Fluorescens CHA0. Microbiol. (Reading) 146 (Pt 10), 2417–2424. doi: 10.1099/00221287-146-10-2417
Buell, M. V., Hansen, R. E. (1960). Reaction of Pyridoxal-5-Phosphate With Aminothiols. J. Am. Chem. Soc. 82 (23), 6042–6049. doi: 10.1021/ja01508a018
Burgess, K. E., Lainson, A., Imrie, L., Fraser-Pitt, D., Yaga, R., Smith, D. G., et al. (2009). Performance of Five Different Electrospray Ionisation Sources in Conjunction With Rapid Monolithic Column Liquid Chromatography and Fast MS/MS Scanning. Proteomics 9 (6), 1720–1726. doi: 10.1002/pmic.200800200
Buyck, J. M., Plesiat, P., Traore, H., Vanderbist, F., Tulkens, P. M., Van Bambeke, F. (2012). Increased Susceptibility of Pseudomonas Aeruginosa to Macrolides and Ketolides in Eukaryotic Cell Culture Media and Biological Fluids Due to Decreased Expression of Oprm and Increased Outer-Membrane Permeability. Clin. Infect. Dis. 55, 534–542. doi: 10.1093/cid/cis473
Castric, K. F., Castric, P. A. (1983). Method for Detection of Cyanogenic Bacteria. Appl. Environ. Microbiol. 45, 701–702. doi: 10.1128/aem.45.2.701-702.1983
Chamrad, D. C., Koerting, G., Gobom, J., Thiele, H., Klose, J., Meyer, H. E., et al. (2003). Interpretation of Mass Spectrometry Data for High-Throughput Proteomics. Anal. Bioanal Chem. 376 (7), 1014–1022. doi: 10.1007/s00216-003-1995-x
Charrier, C., Rodger, C., Robertson, J., Kowalczuk, A., Shand, N., Fraser-Pitt, D., et al. (2014). Cysteamine (Lynovex®), a Novel Mucoactive Antimicrobial & Antibiofilm Agent for the Treatment of Cystic Fibrosis. Orphanet J. Rare Dis. 9, 189. doi: 10.1186/s13023-014-0189-2
Chung, I. Y., Choi, K. B., Heo, Y. J., Cho, Y. H. (2008). Effect of PEL Exopolysaccharide on the Wspf Mutant Phenotypes in Pseudomonas Aeruginosa PA14. J. Microbiol. Biotechnol. 18 (7), 1227–1234.
Cullen, L., Weiser, R., Olszak, T., Maldonado, R. F., Moreira, A. S., Slachmuylders, L., et al. (2015). Phenotypic Characterization of an International Pseudomonas Aeruginosa Reference Panel: Strains of Cystic Fibrosis (CF) Origin Show Less In Vivo Virulence Than Non-CF Strains. Microbiol. (Reading) 161 (10), 1961–1977. doi: 10.1099/mic.0.000155
Cystic Fibrosis Foundation Patient Registry. (2019). 2018 Annual Data Report (Cystic Fibrosis Foundation). Available at: https://www.cff.org/Research/Researcher-Resources/Patient-Registry/2018-Patient-Registry-Annual-Data-Report.pdf (Accessed April 2021).
Darby, C., Cosma, C. L., Thomas, J. H., Manoil, C. (1999). Lethal Paralysis of Caenorhabditis Elegans by Pseudomonas Aeruginosa. Proc. Natl. Acad. Sci. U. S. A. 96 (26), 15202–15207. doi: 10.1073/pnas.96.26.15202
De Marco, C., Coletta, M., Bombardieri, G. (1965). Inhibition of Plasma Monoamine Oxidase by Cysteamine. Nature 205, 176. doi: 10.1038/205176a0
De Stefano, D., Villella, V. R., Esposito, S., Tosco, A., Sepe, A., De Gregorio, F., et al. (2014). Restoration of CFTR Function in Patients With Cystic Fibrosis Carrying the F508del-CFTR Mutation. Autophagy 10 (11), 2053–2074. doi: 10.4161/15548627.2014.973737
Devereux, G., Fraser-Pitt, D., Robertson, J., Devlin, E., Mercer, D., O’Neil, D. (2015). Cysteamine as a Future Intervention in Cystic Fibrosis Against Current and Emerging Pathogens: A Patient-Based Ex Vivo Study Confirming its Antimicrobial and Mucoactive Potential in Sputum. EBiomedicine 2 (10), 1507–1512. doi: 10.1016/j.ebiom.2015.08.018
Devereux, G., Wrolstad, D., Bourke, S. J., Daines, C. L., Doe, S., Dougherty, R., et al. (2020). Oral Cysteamine as an Adjunct Treatment in Cystic Fibrosis Pulmonary Exacerbations: An Exploratory Randomized Clinical Trial. PloS One 15 (12), e0242945. doi: 10.1371/journal.pone.0242945
Dolan, S. K., Kohlstedt, M., Trigg, S., Vallejo Ramirez, P., Kaminski, C. F., Wittmann, C., et al. (2020). Contextual Flexibility in Pseudomonas Aeruginosa Central Carbon Metabolism During Growth in Single Carbon Sources. mBio 11 (2), e02684–e02619. doi: 10.1128/mBio.02684-19
Elliott, D., Burns, J. L., Hoffman, L. R. (2010). Exploratory Study of the Prevalence and Clinical Significance of Tobramycin-Mediated Biofilm Induction in Pseudomonas Aeruginosa Isolates From Cystic Fibrosis Patients. Antimicrob. Agents Chemother. 54 (7), 3024–3026. doi: 10.1128/AAC.00102-10
Essar, D. W., Eberly, L., Hadero, A., Crawford, I. P. (1990). Identification and Characterization of Genes for a Second Anthranilate Synthase in Pseudomonas Aeruginosa: Interchangeability of the Two Anthranilate Synthases and Evolutionary Implications. J. Bacteriol. 172 (2), 884–900. doi: 10.1128/jb.172.2.884-900.1990
Ferrari, E., Monzani, R., Villella, V. R., Esposito, S., Saluzzo, F., Rossin, F., et al. (2017). Cysteamine Re-Establishes the Clearance of Pseudomonas Aeruginosa by Macrophages Bearing the Cystic Fibrosis-Relevant F508del-CFTR Mutation. Cell Death Dis. 8 (1), e2544. doi: 10.1038/cddis.2016.476
Fleitas Martínez, O., Cardoso, M. H., Ribeiro, S. M., Franco, O. L. (2019). Recent Advances in Anti-Virulence Therapeutic Strategies With a Focus on Dismantling Bacterial Membrane Microdomains, Toxin Neutralization, Quorum-Sensing Interference and Biofilm Inhibition. Front. Cell Infect. Microbiol. 9, 74. doi: 10.3389/fcimb.2019.00074
Fraser-Pitt, D., Mercer, D. (2021). What Role for Cysteamine in the Defence Against Infection? Emerg. Top. Life Sci. ETLS20200351. doi: 10.1042/ETLS20200351
Fraser-Pitt, D., Mercer, D., Lovie, E., Robertson, J., O’Neil, D. (2016). Activity of Cysteamine Against the Cystic Fibrosis Pathogen Burkholderia Cepacia Complex. Antimicrob. Agents Chemother. 60 (10), 6200–6206. doi: 10.1128/AAC.01198-16
Fraser-Pitt, D. J., Mercer, D. K., Smith, D., Kowalczuk, A., Robertson, J., Lovie, E., et al. (2018). Cysteamine, an Endogenous Aminothiol, and Cystamine, the Disulfide Product of Oxidation, Increase Pseudomonas Aeruginosa Sensitivity to Reactive Oxygen and Nitrogen Species and Potentiate Therapeutic Antibiotics Against Bacterial Infection. Infect. Immun. 86 (6), e00947–e00917. doi: 10.1128/IAI.00947-17
Geller, D. E., Weers, J., Heuerding, S. (2011). Development of an Inhaled Dry-Powder Formulation of Tobramycin Using Pulmosphere™ Technology. J. Aerosol Med. Pulm Drug Deliv. 24 (4), 175–182. doi: 10.1089/jamp.2010.0855
Ghafoor, A., Hay, I. D., Rehm, B. H. (2011). Role of Exopolysaccharides in Pseudomonas Aeruginosa Biofilm Formation and Architecture. Appl. Environ. Microbiol. 77 (15), 5238–5246. doi: 10.1128/AEM.00637-11
Gibson, R. L., Burns, J. L., Ramsey, B. W. (2003). Pathophysiology and Management of Pulmonary Infections in Cystic Fibrosis. Am. J. Respir. Crit. Care Med. 168, 918–951. doi: 10.1164/rccm.200304-505SO
Gilchrist, F. J., Bright-Thomas, R. J., Jones, A. M., Smith, D., Spaněl, P., Webb, A. K., et al. (2013). Hydrogen Cyanide Concentrations in the Breath of Adult Cystic Fibrosis Patients With and Without Pseudomonas aeruginosa Infection. J. Breath Res. 7 (2), 026010.
Gilchrist, F. J., Belcher, J., Jones, A. M., Smith, D., Smyth, A. R., Southern, K. W., et al. (2015). Exhaled Breath Hydrogen Cyanide as a Marker of Early Pseudomonas Aeruginosa Infection in Children With Cystic Fibrosis. ERJ Open Res. 1 (2), 00044–02015. doi: 10.1183/23120541.00044-2015
Gjødsbol, K., Christiensen, J. J., Karlsmark, T., Jørgensen, B., Klein, B. M., Krogfelt, K. A. (2006). Multiple Bacterial Species Reside in Chronic Wounds: A Longitudinal Study. Int. Wound J. 3, 225–231. doi: 10.1111/j.1742-481X.2006.00159.x
Hayasaka, K., Tada, K. (1983). Effects of the Metabolites of the Branched-Chain Amino Acids and Cysteamine on the Glycine Cleavage System. Biochem. Int. 6 (2), 225–230.
He, J., Baldini, R. L., Déziel, E., Saucier, M., Zhang, Q., Liberati, N. T., et al. (2004). The Broad Host Range Pathogen Pseudomonas Aeruginosa Strain PA14 Carries Two Pathogenicity Islands Harboring Plant and Animal Virulence Genes. Proc. Natl. Acad. Sci. U. S. A. 101 (8), 2530–2535. doi: 10.1073/pnas.0304622101
Heeb, S., Fletcher, M. P., Chhabra, S. R., Diggle, S. P., Williams, P., Camara, M. (2011). Quinolones: From Antibiotics to Autoinducers. FEMS Microbiol. Rev. 35, 247–274. doi: 10.1111/j.1574-6976.2010.00247.x
Hickman, J. W., Tifrea, D. F., Harwood, C. S. (2005). A Chemosensory System That Regulates Biofilm Formation Through Modulation of Cyclic Diguanylate Levels. Proc. Natl. Acad. Sci. U. S. A. 102 (40), 14422–14427. doi: 10.1073/pnas.0507170102
Hoffman, L. R., D’Argenio, D. A., MacCoss, M. J., Zhang, Z., Jones, R. A., Miller, S. I. (2005). Aminoglycoside Antibiotics Induce Bacterial Biofilm Formation. Nature 436 (7054), 1171–1175. doi: 10.1128/IAI.73.4.2504-2514.2005
Hoffmann, N., Rasmussen, T. B., Jensen, PØ, Stub, C., Hentzer, M., Molin, S., et al. (2005). Novel Mouse Model of Chronic Pseudomonas Aeruginosa Lung Infection Mimicking Cystic Fibrosis. Infect. Immun. 73 (4), 2504–2514. doi: 10.1128/IAI.73.4.2504-2514.2005
Holloway, B. W. (1955). Genetic Recombination in Pseudomonas Aeruginosa. J. Gen. Microbiol. 13, 572–581. doi: 10.1099/00221287-13-3-572
Huang, W., Wilks, A. (2017). A Rapid Seamless Method for Gene Knockout in Pseudomonas Aeruginosa. BMC Microbiol. 17 (1), 199. doi: 10.1186/s12866-017-1112-5
Iwama, H., Takahashi, K., Kure, S., Hayashi, F., Narisawa, K., Tada, K., et al. (1997). Depletion of Cerebral D-Serine in non-Ketotic Hyperglycinemia: Possible Involvement of Glycine Cleavage System in Control of Endogenous D-Serine. Biochem. Biophys. Res. Commun. 231 (3), 793–796. doi: 10.1006/bbrc.1997.6184
Jander, G., Rahme, L. G., Ausubel, F. M. (2000). Positive Correlation Between Virulence of Pseudomonas Aeruginosa Mutants in Mice and Insects. J. Bacteriol. 182 (13), 3843–3845. doi: 10.1128/JB.182.13.3843-3845.2000
Kang, D., Revtovich, A. V., Chen, Q., Shah, K. N., Cannon, C. L., Kirienko, N. V. (2019). Pyoverdine-Dependent Virulence of Pseudomonas Aeruginosa Isolates From Cystic Fibrosis Patients. Front. Microbiol. 10, 2048. doi: 10.3389/fmicb.2019.02048
Kay, S., Edwards, J., Brown, J., Dixon, R. (2019). Galleria Mellonella Infection Model Identifies Both High and Low Lethality of Clostridium Perfringens Toxigenic Strains and Their Response to Antimicrobials. Front. Microbiol. 10, 1281. doi: 10.3389/fmicb.2019.01281
Kerem, E., Viviani, L., Zolin, A., MacNeill, S., Hatziagorou, E., Ellemunter, H., et al. (2014). Factors Associated With FEV1 Decline in Cystic Fibrosis: Analysis of the ECFS Patient Registry. Eur. Respir. J. 43 (1), 125–133. doi: 10.1183/09031936.00166412
Kim, J. J., Lydecker, A., Davé, R., Bork, J. T., Roghmann, M. C. (2020). Diabetic Foot Infections: Local Prevalence of and Case-Control Study of Risk Factors for Methicillin-Resistant Staphylococcus Aureus and Pseudomonas Aeruginosa. Open Forum Infect. Dis. 7 (10), ofaa412. doi: 10.1093/ofid/ofaa412
Konstan, M. W., Flume, P. A., Kappler, M., Chiron, R., Higgins, M., Brockhaus, F., et al. (2011). Safety, Efficacy and Convenience of Tobramycin Inhalation Powder in Cystic Fibrosis Patients: The EAGER Trial. J. Cyst. Fibros. 10 (1), 54–61. doi: 10.1016/j.jcf.2010.10.003
Kostylev, M., Kim, D. Y., Smalley, N. E., Salukhe, I., Greenberg, E. P., Dandekar, A. A. (2019). Evolution of the Pseudomonas Aeruginosa Quorum-Sensing Hierarchy. Proc. Natl. Acad. Sci. U. S. A. 116 (14), 7027–7032. doi: 10.1073/pnas.1819796116
Koulenti, D., Lisboa, T., Brun-Buisson, C., Krueger, W., Macor, A., Sole-Violan, J., et al. (2009). EU-VAP/CAP Study Group. Spectrum of Practice in the Diagnosis of Nosocomial Pneumonia in Patients Requiring Mechanical Ventilation in European Intensive Care Units. Crit. Care Med. 37 (8), 2360–2368. doi: 10.1097/CCM.0b013e3181a037ac
Kurachi, M. (1958). Studies on the Biosynthesis of Pyocyanine (II): Isolation and Determination of Pyocyanine. Bull. Inst. Chem. Res. 36 (6), 174–187.
Latifi, A., Winson, M. K., Foglino, M., Bycroft, B. W., Stewart, G. S., Lazdunski, A., et al. (1995). Multiple Homologues of Luxr and Luxi Control Expression of Virulence Determinants and Secondary Metabolites Through Quorum Sensing in Pseudomonas Aeruginosa PAO1. Mol. Microbiol. 17 (2), 333–343. doi: 10.1111/j.1365-2958.1995.mmi_17020333.x
Laville, J., Blumer, C., Von Schroetter, C., Gaia, V., Défago, G., Keel, C., et al. (1998). Characterization of the Hcnabc Gene Cluster Encoding Hydrogen Cyanide Synthase and Anaerobic Regulation by ANR in the Strictly Aerobic Biocontrol Agent Pseudomonas Fluorescens CHA0. J. Bacteriol. 180 (12), 3187–3196. doi: 10.1128/JB.180.12.3187-3196.1998
Lazazzera, B. A., Beinert, H., Khoroshilova, N., Kennedy, M. C., Kiley, P. J. (1996). DNA Binding and Dimerization of the Fe-s-Containing FNR Protein From Escherichia Coli Are Regulated by Oxygen. J. Biol. Chem. 271 (5), 2762–2768. doi: 10.1074/jbc.271.5.2762
Lee, V. T., Matewish, J. M., Kessler, J. L., Hyodo, M., Hayakawa, Y., Lory, S. (2007). A Cyclic-Di-GMP Receptor Required for Bacterial Exopolysaccharide Production. Mol. Microbiol. 65 (6), 1474–1484. doi: 10.1111/j.1365-2958.2007.05879.x
Lloyd, M. G., Lundgren, B. R., Hall, C. W., Gagnon, L. B., Mah, T. F., Moffat, J. F., et al. (2017). Targeting the Alternative Sigma Factor Rpon to Combat Virulence in Pseudomonas Aeruginosa. Sci. Rep. 7 (1), 12615. doi: 10.1038/s41598-017-12667-y
Lowry, M., Hall, M. S., Brosnan, J. T. (1986). Specificity and Mechanism of the Inhibition by Cysteamine of the Renal Glycine-Cleavage Complex. Biochem. Soc Trans. 14, 131– 132. doi: 10.1042/bst0140131
Lundgren, B. R., Thornton, W., Dornan, M. H., Villegas-Peñaranda, L. R., Boddy, C. N., Nomura, C. T. (2013). Gene PA2449 is Essential for Glycine Metabolism and Pyocyanin Biosynthesis in Pseudomonas Aeruginosa PAO1. J. Bacteriol. 195 (9), 2087–2100. doi: 10.1128/JB.02205-12
Lyczak, J. B., Cannon, C. L., Pier, G. B. (2000). Establishment of Pseudomonas Aeruginosa Infection: Lessons From a Versatile Opportunist. Microbes Infect. 2, 1051–1060. doi: 10.1016/S1286-4579(00)01259-4
McGrath, S., Wade, D. S., Pesci, E. C. (2004). Dueling Quorum Sensing Systems in Pseudomonas Aeruginosa Control the Production of the Pseudomonas Quinolone Signal (PQS). FEMS Microbiol. Lett. 230, 27–34. doi: 10.1016/S0378-1097(03)00849-8
Merighi, M., Lee, V. T., Hyodo, M., Hayakawa, Y., Lory, S. (2007). The Second Messenger Bis-(3’-5’)-Cyclic-GMP and its Pilz Domain-Containing Receptor Alg44 Are Required for Alginate Biosynthesis in Pseudomonas Aeruginosa. Mol. Microbiol. 65 (4), 876–895. doi: 10.1111/j.1365-2958.2007.05817.x
Meyer, J. M., Neely, A., Stintzi, A., Georges, C., Holder, I. A. (1996). Pyoverdin Is Essential for Virulence of Pseudomonas Aeruginosa. Infect. Immun. 64 (2), 518–523. doi: 10.1128/iai.64.2.518-523.1996
Middleton, P. G., Mall, M. A., Dřevínek, P., Lands, L. C., McKone, E. F., Polineni, D., et al. (2019). Elexacaftor-Tezacaftor-Ivacaftor for Cystic Fibrosis With a Single Phe508del Allele. N. Engl. J. Med. 381 (19), 1809–1819. doi: 10.1056/NEJMoa1908639
Miller, J. H. (1972). Experiments in Molecular Genetics (Cold Spring Harbor, NY: Cold Spring Harbor Laboratory).
Mowat, E., Paterson, S., Fothergill, J. L., Wright, E. A., Ledson, M. J., Walshaw, M. J., et al. (2011). Pseudomonas Aeruginosa Population Diversity and Turnover in Cystic Fibrosis Chronic Infections. Am. J. Respir. Crit. Care Med. 183, 1674–1679. doi: 10.1164/rccm.201009-1430OC
Munguia, J., Nizet, V. (2017). Pharmacological Targeting of the Host-Pathogen Interaction: Alternatives to Classical Antibiotics to Combat Drug-Resistant Superbugs. Trends Pharmacol. Sci. 38 (5), 473–488. doi: 10.1016/j.tips.2017.02.003
Nathwani, D., Raman, G., Sulham, K., Gavaghan, M., Menon, V. (2014). Clinical and Economic Consequences of Hospital-Acquired Resistant and Multidrug-Resistant Pseudomonas Aeruginosa Infections: A Systematic Review and Meta-Analysis. Antimicrob. Resist. Infect. Control 3, 32. doi: 10.1186/2047-2994-3-32
Nicastro, G. G., Kaihami, G. H., Pereira, T. O., Meireles, D. A., Groleau, M. C., Déziel, E., et al. (2014). Cyclic-Di-GMP Levels Affect Pseudomonas Aeruginosa Fitness in the Presence of Imipenem. Environ. Microbiol. 16 (5), 1321–1333. doi: 10.1111/1462-2920.12422
Norman, A., Ciofu, O., Amador, C. I., Høiby, N., Jelsbak, L. (2016). Genome Sequence of Pseudomonas Aeruginosa Strain DK1-NH57388A, a Stable Mucoid Cystic Fibrosis Isolate. Genome Announc. 4 (1), e00008–e00016. doi: 10.1128/genomeA.00008-16
Oglesby, L. L., Jain, S., Ohman, D. E. (2008). Membrane Topology and Roles of Pseudomonas Aeruginosa Alg8 and Alg44 in Alginate Polymerization. Microbiol. (Reading) 154 (Pt 6), 1605–1615. doi: 10.1099/mic.0.2007/015305-0
Olofsson, A. C., Hermansson, M., Elwing, H. (2003). N-Acetyl-L-Cysteine Affects Growth, Extracellular Polysaccharide Production, and Bacterial Biofilm Formation on Solid Surfaces. Appl. Environ. Microbiol. 69 (8), 4814–4822. doi: 10.1128/AEM.69.8.4814-4822.2003
O’Toole, G. A., Pratt, L. A., Watnick, P. I., Newman, D. K., Weaver, V. B., Kolter, R. (1999). Genetic Approaches to Study of Biofilms. Methods Enzymol. 310, 91–109. doi: 10.1016/S0076-6879(99)10008-9
Parales, R. E., Ditty, J. L. (2018). Chemotaxis to Atypical Chemoattractants by Soil Bacteria. Methods Mol. Biol. 1729, 255–280. doi: 10.1007/978-1-4939-7577-8_21
Pesci, E. C., Millbank, J. B., Pearson, P., McKnight, S., Kende, A. S., Greenberg, E. P., et al. (1999). Quinolone Signaling in the Cell-to-Cell Communication System of Pseudomonas Aeruginosa. Proc. Natl. Acad. Sci. U. S. A. 96, 11229–11234. doi: 10.1073/pnas.96.20.11229
Pessi, G., Haas, D. (2000). Transcriptional Control of the Hydrogen Cyanide Biosynthetic Genes Hcnabc by the Anaerobic Regulator ANR and the Quorum-Sensing Regulators Lasr and Rhlr in Pseudomonas Aeruginosa. J. Bacteriol. 182 (24), 6940–6949. doi: 10.1128/JB.182.24.6940-6949.2000
Pessi, G., Haas, D. (2001). Dual Control of Hydrogen Cyanide Biosynthesis by the Global Activator Gaca in Pseudomonas Aeruginosa PAO1. FEMS Microbiol. Lett. 200 (1), 73–78. doi: 10.1111/j.1574-6968.2001.tb10695.x
Pitari, G., Malergue, F., Martin, F., Philippe, J. M., Massucci, M. T., Chabret, C., et al. (2000). Pantetheinase Activity of Membrane-Bound Vanin-1: Lack of Free Cysteamine in Tissues of Vanin-1 Deficient Mice. FEBS Lett. 483 (2-3), 149–154. doi: 10.1016/S0014-5793(00)02110-4
Poudyal, B., Sauer, K. (2018). The ABC of Biofilm Drug Tolerance: The Merr-Like Regulator Brlr is an Activator of ABC Transport Systems, With PA1874-77 Contributing to the Tolerance of Pseudomonas Aeruginosa Biofilms to Tobramycin. Antimicrob. Agents Chemother. 62 (2), e01981–e01917. doi: 10.1128/AAC.01981-17
Pu, M., Sheng, L., Song, S., Gong, T., Wood, T. K. (2018). Serine Hydroxymethyltransferase Shra (PA2444) Controls Rugose Small-Colony Variant Formation in Pseudomonas Aeruginosa. Front. Microbiol. 9, 315. doi: 10.3389/fmicb.2018.00315
Rahme, L. G., Stevens, E. J., Wolfort, S. F., Shao, J., Tompkins, R. G., Ausubel, F. M. (1995). Common Virulence Factors for Bacterial Pathogenicity in Plants and Animals. Science 268 (5219), 1899–1902. doi: 10.1126/science.7604262
Ramus, J. (1977). Alcian Blue: A Quantitative Aqueous Assay for Algal Acid and Sulfated Polysaccharides. J. Phycol. 13, 345–348. doi: 10.1111/j.1529-8817.1977.tb02939.x
Reimmann, C., Beyeler, M., Latifi, A., Winteler, H., Foglino, M., Lazdunski, A., et al. (1997). The Global Activator Gaca of Pseudomonas Aeruginosa PAO Positively Controls the Production of the Autoinducer N-Butyryl-Homoserine Lactone and the Formation of the Virulence Factors Pyocyanin, Cyanide, and Lipase. Mol. Microbiol. 24 (2), 309–319. doi: 10.1046/j.1365-2958.1997.3291701.x
Ryall, B., Davies, J. C., Wilson, R., Shoemark, A., Williams, H. D. (2008). Pseudomonas Aeruginosa, Cyanide Accumulation and Lung Function in CF and non-CF Bronchiectasis Patients. Eur. Respir. J. 32, 740–747. doi: 10.1183/09031936.00159607
Sarwar, Z., Lundgren, B. R., Grassa, M. T., Wang, M. X., Gribble, M., Moffat, J. F., et al. (2016). Gcsr, A Tyrr-Like Enhancer-Binding Protein, Regulates Expression of the Glycine Cleavage System in Pseudomonas Aeruginosa PAO1. mSphere 1 (2), e00020–e00016. doi: 10.1128/mSphere.00020-16
Schneider, J. A., Clark, K. F., Greene, A. A., Reisch, J. S., Markello, T. C., Gahl, W. A., et al. (1995). Recent Advances in the Treatment of Cystinosis. J. Inherit. Metab. Dis. 18 (4), 387–397. doi: 10.1007/BF00710051
Senior, N. J., Bagnall, M. C., Champion, O. L., Reynolds, S. E., La Ragione, R. M., Woodward, M. J., et al. (2011). Galleria Mellonella as an Infection Model for Campylobacter Jejuni Virulence. J. Med. Microbiol. 60 (Pt 5), 661–669. doi: 10.1099/jmm.0.026658-0
Shao, X., Zhang, X., Zhang, Y., Zhu, M., Yang, P., Yuan, J., et al. (2018). Rpon-Dependent Direct Regulation of Quorum Sensing and the Type VI Secretion System in Pseudomonas Aeruginosa PAO1. J. Bacteriol. 200 (16), e00205–e00218. doi: 10.1128/JB.00205-18
Shrestha, C. L., Assani, K. D., Rinehardt, H., Albastroiu, F., Zhang, S., Shell, R., et al. (2017). Cysteamine-Mediated Clearance of Antibiotic-Resistant Pathogens in Human Cystic Fibrosis Macrophages. PloS One 12 (10), e0186169. doi: 10.1371/journal.pone.0186169
Shrestha, C. L., Zhang, S., Wisniewski, B., Häfner, S., Elie, J., Meijer, L., et al. (2020). (R)-Roscovitine and CFTR Modulators Enhance Killing of Multi-Drug Resistant Burkholderia Cenocepacia by Cystic Fibrosis Macrophages. Sci. Rep. 10 (1), 21700. doi: 10.1038/s41598-020-78817-x
Smith, E. E., Buckley, D. G., Wu, Z., Saenphimmachak, C., Hoffman, L. R., D'Argenio, D. A., et al. (2006). Genetic Adaptation by Pseudomonas Aeruginosa to the Airways of Cystic Fibrosis Patients. Proc. Natl. Acad. Sci. U. S. A. 103, 8487–8492. doi: 10.1073/pnas.0602138103
Smith, P. K., Krohn, R. I., Hermanson, G. T., Mallia, A. K., Gartner, F. H., Provenzano, M. D., et al. (1985). Measurement of Protein Using Bicinchoninic Acid. Anal. Biochem. 150 (1), 76–85. doi: 10.1016/0003-2697(85)90442-7
Smith, S., Rowbotham, N. J., Charbek, E. (2018). Inhaled Antibiotics for Pulmonary Exacerbations in Cystic Fibrosis. Cochrane Database Syst. Rev. 10 (10), CD008319. doi: 10.1002/14651858.CD008319.pub3
Tahrioui, A., Duchesne, R., Bouffartigues, E., Rodrigues, S., Maillot, O., Tortuel, D., et al. (2019). Extracellular DNA Release, Quorum Sensing, and Prrf1/F2 Small Rnas are Key Players in Pseudomonas Aeruginosa Tobramycin-Enhanced Biofilm Formation. NPJ Biofilms Microbiomes 5 (1), 15. doi: 10.1038/s41522-019-0088-3
Taylor, G. K., Goodlett, D. R. (2005). Rules Governing Protein Identification by Mass Spectrometry. Rapid Commun. Mass Spectrom. 19 (23), 3420. doi: 10.1002/rcm.2225
Terzuoli, L., Leoncini, R., Pagani, R., Guerranti, R., Vannoni, D., Ponticelli, F., et al. (1998). Some Chemical Properties and Biological Role of Thiazolidine Compounds. Life Sci. 63 (14), 1251–1267. doi: 10.1016/S0024-3205(98)00387-7
Trubiano, J. A., Padiglione, A. A. (2015). Nosocomial Infections in the Intensive Care Unit. Anaesth. Intensive Care 16, 598–602. doi: 10.1016/j.mpaic.2015.09.010
Vale, P. F., McNally, L., Doeschl-Wilson, A., King, K. C., Popat, R., Domingo-Sananes, M. R., et al. (2016). Beyond Killing Can We Find New Ways to Manage Infection? Evol. Med. Public Health 2016 (1), 148–157. doi: 10.1093/emph/eow012
Viducic, D., Murakami, K., Amoh, T., Ono, T., Miyake, Y. (2017). Rpon Promotes Pseudomonas Aeruginosa Survival in the Presence of Tobramycin. Front. Microbiol. 8, 839. doi: 10.3389/fmicb.2017.00839
Wang, F., He, Q., Yin, J., Xu, S., Hu, W., Gu, L. (2018). Brlr From Pseudomonas Aeruginosa is a Receptor for Both Cyclic Di-GMP and Pyocyanin. Nat. Commun. 9 (1), 2563. doi: 10.1038/s41467-018-05004-y
Wayne, P. A., Clinical and Laboratory. Standards Institute (2015). Methods for Dilution Antimicrobial Susceptibility Tests for Bacteria That Grow Aerobically, Approved Standard—Tenth Edition. CLSI document M07-A10. The Clinical and Laboratory Standards Institute (CLSI).
Whiteley, M., Lee, K. M., Greenberg, E. P. (1999). Identification of Genes Controlled by Quorum Sensing in Pseudomonas Aeruginosa. Proc. Natl. Acad. Sci. U S A. 96 (24), 13904–13909. doi: 10.1073/pnas.96.24.13904
World Health Organisation (WHO). (2017). Global Priority List of Antibiotic-Resistant Bacteria to Guide Research, Discovery, and Development of New Antibiotics. (Geneva, Switzerland: World Health Organization). Available at: https://www.who.int/medicines/publications/WHO-PPL-Short_Summary_25Feb-ET_NM_WHO.pdf (Accessed 22 March 2021).
Wiehlmann, L., Wagner, G., Cramer, N., Siebert, B., Gudowius, P., Morales, G., et al. (2007). Population Structure of Pseudomonas Aeruginosa. Proc. Natl. Acad. Sci. U. S. A. 104 (19), 8101–8106. doi: 10.1073/pnas.0609213104
Winson, M. K., Camara, M., Latifi, A., Foglino, M., Chhabra, S. R., Daykin, M., et al. (1995). Multiple N-Acyl-L-Homoserine Lactone Signal Molecules Regulate Production of Virulence Determinants and Secondary Metabolites in Pseudomonas Aeruginosa. Proc. Natl. Acad. Sci. U. S. A. 92 (20), 9427–9431. doi: 10.1073/pnas.92.20.9427
Winstanley, C., O’Brien, S., Brockhurst, M. A. (2016). Pseudomonas Aeruginosa Evolutionary Adaptation and Diversification in Cystic Fibrosis Chronic Lung Infections. Trends Microbiol. 24 (5), 327–337. doi: 10.1016/j.tim.2016.01.008
Wood, W. B. (1988). “Introduction to C. Elegans Biology,” in The Nematode Caenorhabditis Elegans. Ed. Wood, W. B. (Cold Spring Harbor, NY: Cold Spring Harbor Laboratory), 1–16.
Yan, H., Wang, M., Sun, F., Dandekar, A. A., Shen, D., Li, N. (2018). A Metabolic Trade-Off Modulates Policing of Social Cheaters in Populations of Pseudomonas Aeruginosa. Front. Microbiol. 9, 337. doi: 10.3389/fmicb.2018.00337
Ye, R. W., Haas, D., Ka, J. O., Krishnapillai, V., Zimmermann, A., Baird, C., et al. (1995). Anaerobic Activation of the Entire Denitrification Pathway in Pseudomonas Aeruginosa Requires Anr, an Analog of Fnr. J. Bacteriol. 177 (12), 3606–3609. doi: 10.1128/jb.177.12.3606-3609.1995
Yudkoff, M., Nissim, I., Schneider, A., Segal, S. (1981). Cysteamine Inhibition of [15N]-Glycine Turnover in Cystinosis and of Glycine Cleavage In Vitro. Metab. Clin. Exp. 30 (11), 1096–1103. doi: 10.1016/0026-0495(81)90054-8
Keywords: virulence, biofilm, Pseudomonas aeruginosa, novel therapeutic, glycine cleavage complex
Citation: Fraser-Pitt DJ, Dolan SK, Toledo-Aparicio D, Hunt JG, Smith DW, Lacy-Roberts N, Nupe Hewage PS, Stoyanova TN, Manson E, McClean K, Inglis NF, Mercer DK and O’Neil DA (2021) Cysteamine Inhibits Glycine Utilisation and Disrupts Virulence in Pseudomonas aeruginosa. Front. Cell. Infect. Microbiol. 11:718213. doi: 10.3389/fcimb.2021.718213
Received: 31 May 2021; Accepted: 11 August 2021;
Published: 22 September 2021.
Edited by:
Ruggero La Rosa, Novo Nordisk Foundation Center for Biosustainability (DTU Biosustain), DenmarkReviewed by:
Erin Samantha Gloag, The Ohio State University, United StatesVolker Behrends, University of Roehampton London, United Kingdom
Copyright © 2021 Fraser-Pitt, Dolan, Toledo-Aparicio, Hunt, Smith, Lacy-Roberts, Nupe Hewage, Stoyanova, Manson, McClean, Inglis, Mercer and O’Neil. This is an open-access article distributed under the terms of the Creative Commons Attribution License (CC BY). The use, distribution or reproduction in other forums is permitted, provided the original author(s) and the copyright owner(s) are credited and that the original publication in this journal is cited, in accordance with accepted academic practice. No use, distribution or reproduction is permitted which does not comply with these terms.
*Correspondence: Douglas J. Fraser-Pitt, ZG91Z0Bub3ZhYmlvdGljcy5jby51aw==