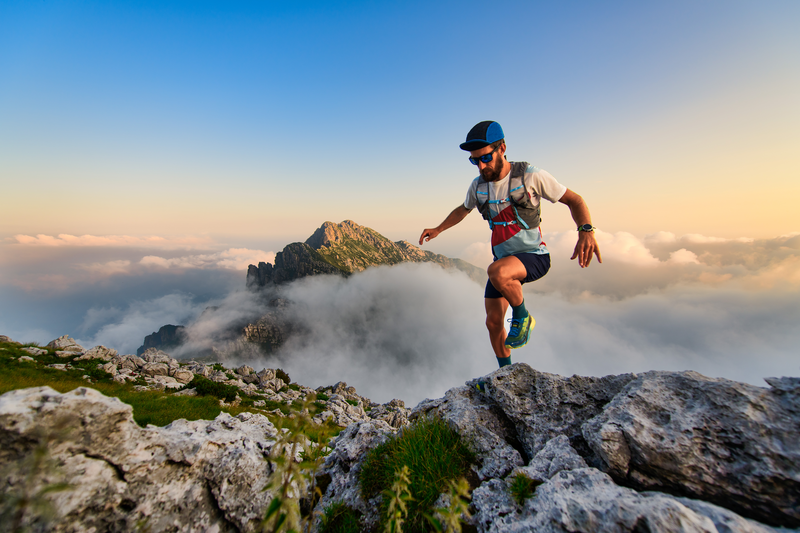
94% of researchers rate our articles as excellent or good
Learn more about the work of our research integrity team to safeguard the quality of each article we publish.
Find out more
ORIGINAL RESEARCH article
Front. Cell. Infect. Microbiol. , 15 June 2021
Sec. Clinical Microbiology
Volume 11 - 2021 | https://doi.org/10.3389/fcimb.2021.690799
This article is part of the Research Topic Foodborne Enterobacteriaceae of Animal Origin: Epidemic Characteristics of Drug Resistance, Pathogenic Mechanisms, and Novel Control Measures View all 14 articles
In this study, a detailed genetic dissection of the huge and complex blaNDM-carrying genetic elements and their related mobile genetic elements was performed in Enterobacteriaceae. An extensive comparison was applied to 12 chromosomal genetic elements, including six sequenced in this study and the other six from GenBank. These 12 genetic elements were divided into five groups: a novel IME Tn6588; two related IMEs Tn6523 (SGI1) and Tn6589; four related ICEs Tn6512 (R391), Tn6575 (ICEPvuChnBC22), Tn6576, and Tn6577; Tn7 and its derivatives Tn6726 and 40.7-kb Tn7-related element; and two related IMEs Tn6591 (GIsul2) and Tn6590. At least 51 resistance genes, involved in resistance to 18 different categories of antibiotics and heavy metals, were found in these 12 genetic elements. Notably, Tn6576 carried another ICE Tn6582. In particular, the six blaNDM-carrying genetic elements Tn6588, Tn6589, Tn6575, Tn6576, Tn6726, and 40.7-kb Tn7-related element contained large accessory multidrug resistance (MDR) regions, each of which had a very complex mosaic structure that comprised intact or residual mobile genetic elements including insertion sequences, unit or composite transposons, integrons, and putative resistance units. Core blaNDM genetic environments manifested as four different Tn125 derivatives and, notably, two or more copies of relevant Tn125 derivatives were found in each of Tn6576, Tn6588, Tn6589, and 40.7-kb Tn7-related element. The huge and complex blaNDM-carrying genetic elements were assembled from complex transposition and homolog recombination. Firstly identified were eight novel mobile elements, including three ICEs Tn6576, Tn6577, and Tn6582, two IMEs, Tn6588 and Tn6589, two composite transposons Tn6580a and Tn6580b, and one integron In1718.
New Delhi metallo-β-lactamase (NDM) is able to hydrolyze nearly all β-lactams except aztreonam and thus mediates resistance to penicillins, cephalosporins, and carbapenems (Yong et al., 2009). It is hypothesized that the blaNDM gene is originally integrated into the Acinetobacter chromosome from an unknown environmental species and then captured by two copies of ISAba125, giving rise to ISAba125-composite transposon Tn125 (Poirel et al., 2012). With the transposition of Tn125, blaNDM is disseminated among Acinetobacter, Enterobacteriaceae and Pseudomonas species; Tn125 and its blaNDM-carrying derivatives, with various truncations and deletions, can be found in the accessory resistance regions of bacterial plasmids or chromosomes (Wu et al., 2019). There are reports of chromosomal location of blaNDM in Enterobacteriaceae species including Escherichia coli (Pfeifer et al., 2011; Poirel et al., 2011; Shen et al., 2017; Reynolds et al., 2019), Providencia stuartii (Poirel et al., 2011), Proteus mirabilis (Girlich et al., 2015), Klebsiella pneumoniae (Sakamoto et al., 2018), and Proteus vulgaris (Kong et al., 2020), but few of them are subjective to detailed genetic dissection of blaNDM-carrying accessory resistance regions (Girlich et al., 2015; Sakamoto et al., 2018; Reynolds et al., 2019; Kong et al., 2020).
Integrative and conjugative elements (ICEs) and integrative and mobilizable elements (IMEs) (Bellanger et al., 2014; Delavat et al., 2017; Botelho and Schulenburg, 2021) are two different types of mobile genetic elements which are frequently integrated into bacterial chromosome, contributing to dissemination of resistance genes. ICEs have the ability to transfer between cells because of their self-encoded conjugation function. It is typically composed of attL (attachment site at the left end), int (integrase), xis (excisionase), rlx (relaxase), oriT (origin of conjugative replication), cpl (coupling protein), a P (TivB)- or F (TivF)-type T4SS machinery (mating pair formation), and attR (attachment site at the right end). IMEs are not self-transmissible, and they achieve the intercellular mobility with the help of other conjugative elements that encode proteins involved in complete conjugation function. IMEs typically have attL, int, rlx, oriT, and attR, but contained no conjugal transfer genes. Tn7 is a unit transposon with the ability to integrate into bacterial chromosomes and plasmids, and it encodes five core transposition determinants TnsA and TnsB (transposases), TnsC (regulator), and TnsD and TnsE (DNA-binding proteins), as well as three TnsB-binding sites and four TnsB-binding sites at its left and right ends, respectively (Peters, 2014).
In this work, whole-genome sequencing identified four blaNDM-1/-3-carrying genetic elements plus two additional genetic elements harboring other resistance genes in the chromosomes of four isolates of Providencia rettgeri, Proteus mirabilis, and K. pneumoniae. An extension sequence comparison was then applied to a collection of 12 chromosomal genetic elements (including the above six ones sequenced in this work) that could be grouped into ICEs, IMEs, and Tn7 unit transposon and its derivatives. Data presented here gave a detailed genetic dissection of the huge and complex blaNDM-carrying genetic elements and their related mobile genetic elements in multiple Enterobacteriaceae species.
The four chromosomal blaNDM-carrying isolates (Table S1) were screened from more than two hundred blaNDM-carrying Enterobacteriaceae isolates routinely collected from China hospitals and livestock farms. Providencia rettgeri 1701091 and Proteus mirabilis 1701092 (Table S1) were recovered in 2017 from the chicken intestinal contents in two different China livestock farms. K. pneumoniae QD23 and Providencia rettgeri 51003 were recovered from the urine specimens of two different patients with nosocomial infections in two Chinese public hospitals in 2015 and 2017, respectively. Bacterial species identification was performed using genome sequence-based average nucleotide identity analysis (http://www.ezbiocloud.net/tools/ani) (Richter and Rosselló-Móra, 2009).
Bacterial genomic DNA was isolated using the UltraClean Microbial Kit (Qiagen, NW, Germany) and sequenced from a sheared DNA library with average size of 15 kb (ranged from 10 to 20 kb) on a PacBio RSII sequencer (Pacific Biosciences, CA, USA), as well as a paired-end library with an average insert size of 350 bp (ranged from 150 to 600 kb) on a HiSeq sequencer (Illumina, CA, USA). The paired-end short Illumina reads were used to correct the long PacBio reads utilizing proovread (Hackl et al., 2014), and then the corrected PacBio reads were assembled de novo utilizing SMARTdenovo (https://github.com/ruanjue/smartdenovo).
Open reading frames (ORFs) and pseudogenes were predicted using RAST 2.0 (https://rast.nmpdr.org/) (Brettin et al., 2015) combined with BLASTP/BLASTN searches (Boratyn et al., 2013) against the UniProtKB/Swiss-Prot database (https://web.expasy.org/docs/swiss-prot_guideline.html) (Boutet et al., 2016) and the RefSeq database (https://www.ncbi.nlm.nih.gov/refseq/) (O’Leary et al., 2016). Annotation of resistance genes, mobile elements, and other features were carried out using the online databases including CARD (https://card.mcmaster.ca/browse) (Jia et al., 2017), ResFinder (https://cge.cbs.dtu.dk/services/ResFinder/) (Zankari et al., 2012), ISfinder (https://www-is.biotoul.fr/) (Siguier et al., 2006), INTEGRALL (http://integrall.bio.ua.pt/)? (Moura et al., 2009) and Tn Number Registry (https://www.ucl.ac.uk/eastman/tn-number-registry) (Roberts et al., 2008). Multiple and pairwise sequence comparisons were performed using MUSCLE 3.8.31 (Edgar, 2004) and BLASTN, respectively. Gene organization diagrams were drawn in Inkscape 1.0 (https://inkscape.org/en/). Heatmaps were plotted with MeV 4.9.0 (Saeed et al., 2003).
Conjugal transfer experiments were carried out with rifampin-resistant Escherichia coli EC600 or sodium azide-resistant E. coli J53 being used as a recipient, and the 1701092 or QD23 isolate as a donor. Three milliliters of overnight cultures of each of donor and recipient bacteria were mixed together, harvested and resuspended in 80 μl of Brain Heart Infusion (BHI) broth (BD Biosciences). The mixture was spotted on a 1 cm2 hydrophilic nylon membrane filter with a 0.45 µm pore size (Millipore) that was placed on BHI agar (BD Biosciences) plate and then incubated for mating at 37°C for 12 to 18 h. Bacteria were washed from filter membrane and spotted on Muller–Hinton (MH) agar (BD Biosciences) plates, for selecting an E. coli transconjugant carrying blaNDM or carrying tetA(C). Then 200 mg/L sodium azide (for J53) or 1,000 mg/L rifampin (for EC600), together with 4 mg/L imipenem (for blaNDM) or 8 mg/L tetracycline [for tetA(C)] was used for transconjugant selection.
All the wild-type and transconjugant strains was subjected to PCR amplification followed by amplicon sequencing, for determining the sequences of bacterial 16S rRNA genes (Frank et al., 2008), the presence of key markers such as blaNDM, tetA(C), int, and parM, and also the location/boundary of mobile genetic elements such as Tn6588, Tn6589, Tn6576, Tn6577, and Tn6590 (data not shown).
Activity of Ambler class A/B/D carbapenemases in bacterial cell extracts was determined by a modified CarbaNP test (Feng et al., 2016). Bacterial antimicrobial susceptibility was tested by BioMérieux VITEK 2 and interpreted as per the Clinical and Laboratory Standards Institute (CLSI) guidelines (CLSI, 2020).
The complete chromosome sequences of the 1701091, 1701092, QD23, and 51003 isolates were submitted to GenBank under accession numbers CP042860, CP042857, CP042858, and CP042861 respectively.
The complete genome sequences of four blaNDM-carrying isolates Providencia rettgeri 1701091, Proteus mirabilis 1701092, K. pneumoniae QD23, and Providencia rettgeri 51003 were determined in this work through high-throughput genome sequencing. A total of six chromosome-borne accessory resistance regions were identified: blaNDM-1/-3-carrying Tn6588, Tn6589, Tn6576, and 40.7-kb Tn7-related element from strains 1701091, 1701092, QD23, and 51003, respectively; tetA(C)- and blaCTX-M-14-carrying Tn6577 were from strain 1701092; and strAB-carrying Tn6590 was from strain 51003.
A detailed sequence comparison was applied to a collection of 12 chromosomal genetic elements, which included the above mentioned six genetic elements sequenced in this study, together with six additional ones from GenBank (four reference/prototype ones Tn6523, Tn6512, Tn7, and Tn6591, and two blaNDM-carrying ones Tn6575 and Tn6726). These 12 genetic elements could be further divided into five distinct groups: a novel IME Tn6588; two related IMEs Tn6523 and Tn6589; four related ICEs Tn6512, Tn6575, Tn6576 and Tn6577; Tn7 and its two derivatives Tn6726 and 40.7-kb Tn7-related element; and two related IMEs Tn6591 and Tn6590 (Table 1). Six (Tn6588, Tn6589, Tn6575, Tn6576, Tn6726, and 40.7-kb Tn7-related element) of them harbored blaNDM. At least 51 resistance genes, involved in resistance to 18 different categories of antibiotics and heavy metals, were identified in these 12 elements (Figure 1 and Table S2).
Figure 1 A heatmap of prevalence of resistance genes. The original data are shown in Table S2.
Tn6588 (100.0 kb in length) was inserted into the chromosomal orf1407 gene (cytochrome c551 peroxidase). Tn6588 had a 9.2-kb backbone (containing int) with insertion of two accessory modules: IS1A and a 90.1-kb multidrug resistance (MDR) region, and it had terminal 35-bp attL/attR pairs and were further bracketed by 5-bp direct repeats (DRs; target site duplication signals for transposition) (Figure 2A). The MDR region contained a total of 19 resistance genes including blaNDM-1 (Figure 1 and Table S2), which were located at eight different resistance loci: In1718, IS26–mph(E)–IS26 unit, In1247, a truncated ISCR2–floR unit, ISCR2–sul2 unit, ISEc59–aph(4)-Ia–aacC4–IS26 unit, ΔTn4352 containing aphA1, and a 6.8-kb In27-carrying Tn6909-related region (Figure 2B).
Figure 2 Shown are the organization of Tn6588 (A), and MDR region from Tn6588 (B). Genes are denoted by arrows. Genes, mobile elements, and other features are colored based on their functional classification. Shading denotes regions of homology (nucleotide identity ≥95%). Numbers in brackets indicate nucleotide positions within the chromosome of strain 1701091. The accession numbers of Tn6727, ISCR2–floR unit, Tn4352 (Wrighton and Strike, 1987), Tn6581c, and Tn6909 used as reference are CP047346, CP042857, CP042858, CP042857, and CP032168, respectively.
In1718 was a concise class 1 integron with a gene cassette array (GCA) aacA4cr–blaOXA-1–catB3–arr-3. In1247 was a complex class 1 integron containing VR1 (variable region 1=GCA: aadA2–lnuF), five copies of 6.4-kb repeat [VR2 (ISCR1–bleMBL–blaNDM-1–arr-3 unit) plus 3′-CS2 (a second 3′-conseved segment)], and VR3 (ISCR1–aphA6 unit). In1247 plus a Tn21 core transposition module tnpAR–res–tnpM in this MDR region were genetically related to the Tn3-family unit transposon Tn6727 (Partridge et al., 2001), which was initially found in Proteus vulgaris and originally associated with Tn21. The 6.8-kb Tn6909-related region looked like a truncated version of Tn3-family unit transposon Tn6909 that was originally associated with Tn1696 and Tn21 (Partridge et al., 2001).
Tn6523, a 42.4-kb IME initially found in Salmonella enterica serovar Typhimurium DT104 (Boyd et al., 2000), had a 27.2-kb backbone (containing attL, int, xis, rlx, oriT, and attR) with insertion of a single accessory module In127. Besides the GCA (aadA2), In127 captured additional two resistance loci: ISCR3–tetA(G)–floR unit and a blaCARB-2-carrying In167. The backbone of Tn6589 was almost identical to Tn6523 but integrated with a 66.4-kb MDR region instead of In127 (Figure 3A). This MDR region contained a total of 16 resistance genes including blaNDM-1 (Figure 1 and Table S2), which were located at seven different resistance loci: In27, ISCR3–tetA(G)–floR unit, Tn2–rmtB region, ISCR2–floR unit, ISPa13–erm42–IS26 unit, aacC2–tmrB region, and In363 with a GCA dfrA1–gcuC (Figure 3B). In27 in this MDR region was a complex class 1 integron, which carried VR1 (GCA: dfrA12–gcuF–aadA2), and two copies of 11.8-kb repeat region [VR2 (ISCR1–bleMBL–blaNDM-1–arr-3 unit) + 3′-CS2 + VR3 (ISCR1–qnrA1 unit) + 3′-CS3] (Figure 3B).
Figure 3 Shown are two Tn6523-related transposons (A), and MDR region from Tn6589 and In127 from Tn6523 (B). Genes are denoted by arrows. Genes, mobile elements, and other features are colored based on their functional classification. Shading denotes regions of homology (nucleotide identity ≥95%). Numbers in brackets indicate nucleotide positions within Tn6523 (Boyd et al., 2000) and the chromosome of strain 1701092. The accession number of Tn6523 used as reference is AF261825.
Tn6512, an 88.5-kb ICE initially found in Providencia rettgeri 107 (Boltner et al., 2002), was composed of an 82.4-kb backbone with insertion of three accessory modules: IS15DI-composite transposon Tn6578 (containing aphA1), ISPrre1 and ISPrre2 (Figure S1). Tn6512, Tn6575 (146.9 kb in length) (Kong et al., 2020), Tn6576 (534.1 kb in length), and Tn6577 (137.5 kb in length) had similar backbones and, especially, shared the core backbone genes attL, int, xis, rlx, ori, cpl, a TivF-type T4SS gene set, and attR (Figure S1). All these four ICEs were integrated at the same site within the chromosomal gene prfC (peptide chain release factor 3).
Each of Tn6575, Tn6576, and Tn6577 had two accessory modules. Firstly, a 85.0-kb MDR region, a novel 406.4-kb ICE Tn6582, and a 11.1-kb blaHMS-sul2 region were inserted at the same site within umuC of Tn6575, Tn6576, and Tn6577, respectively, which led to different truncations of surrounding backbone regions of Tn6575 and Tn6577 but not Tn6576. Secondly, ISPpu12, Tn6580b (55.2 kb in length), and Tn6580a (50.0 kb in length) were integrated at the same site downstream of orf714 of Tn6575, Tn6576, and Tn6577, respectively (Figure S1).
The 85.0-kb MDR region of Tn6575 contained a total of 22 resistance genes including blaNDM-1 (Figure 1 and Table S2), which were located at 12 different resistance loci: two copies of a truncated ISEc59–aph(4)-Ia–aacC4–IS26 unit, an unnamable In element (harboring a long GCA aacA4cr–blaOXA-1–catB3–arr-3–aacA4cr–arr-3 but lacking the whole 5′-CS), aphA1-containing Tn4352, IS26–cfr–IS26 unit, IS26-composite transposon Tn6581a containing bleO, In525 (GCA: dfrA32–ereA–aadA2), tetA(C)-containing ΔTn6309, blaNDM-1-containing ΔTn125-2, a 6.6-kb In0-carrying Tn6911-related region, a truncated ISCR2–floR unit, a truncated ISCR2–sul2 unit, and strAB-containing ΔTn5393c (Figure 4). The Tn6911-related region in this MDR region looked like a truncated version of Tn3-family unit transposon Tn6911 that was originally associated with Tn21 (Partridge et al., 2001).
Figure 4 Organization of MDR region from Tn6575, and comparison to related regions. Genes are denoted by arrows. Genes, mobile elements, and other features are colored based on their functional classification. Shading denotes regions of homology (nucleotide identity ≥95%). Numbers in brackets indicate nucleotide positions within Tn6575. The accession numbers of IS26–aacC4–aph(4)-Ia–ISEc59 unit, Tn6309 (Sun et al., 2016), Tn6911, Tn5393c (L’Abée-Lund and Sørum, 2000), ISCR2–floR unit, ISCR2–sul2 unit used as reference are CP042857, KX710094, KT033469, AF262622, CP042857, and AE014073, respectively.
Tn6582 had a complete set of core ICE backbone determinants and, moreover, a lot of accessory modules: two MDR (MDR-1 and MDR-2) regions, sil–cop region as found in IncHI2 plasmid R478 (Gilmour et al., 2004), floR–strAB–sul2 region (containing ΔTn5393c and a truncated ISCR2–floR unit), and multiple intact or residue IS elements (Figure S2). The 38.9-kb MDR-1 region (Figure 5) comprised ΔTn6029 (containing sul2 and strAB), Tn6581b, an unnamable In37-like element (harboring the In37 GCA aacA4cr–blaOXA-1–catB3–arr-3 but lack of the whole 5′-CS), In27 (GCA: dfrA12–gcuF–aadA2), and two copies of 11.0-kb repeat (each copy harbored a truncated aacC2–tmrB region, Tn4352, IS26–mph(A)–IS6100 unit, and chrA-orf98 unit). The 43.7-kb MDR-2 region (Figure 6) comprised ΔTn21 (containing mer), aacC2–tmrB region, and two copies of 21.4-kb repeat (each copy harbored IS26–mph(A)–IS6100 unit, chrA-orf98 unit, In1021, and a truncated aacC2–tmrB region). In1021 was a complex class 1 integron containing VR1 (GCA: aacA4cr–arr3–dfrA27–aadA16) and VR2 (ISCR1–bleMBL–blaNDM-3–arr-3 unit).
Figure 5 Organization of MDR-1 region from Tn6582, and comparison to related regions. Genes are denoted by arrows. Genes, mobile elements, and other features are colored based on their functional classification. Shading denotes regions of homology (nucleotide identity ≥95%). Numbers in brackets indicate nucleotide positions within the chromosome of strain QD23. The accession numbers of Tn6029 (Cain et al., 2010), aacC2–tmrB region (Partridge et al., 2012), and In37 (Wang et al., 2003) used as reference are GQ150541, JX101693, and AY259086, respectively.
Figure 6 Organization of MDR-2 region from Tn6582. Genes are denoted by arrows. Genes, mobile elements, and other features are colored based on their functional classification. Shading denotes regions of homology (nucleotide identity ≥95%). Numbers in brackets indicate nucleotide positions within the chromosome of strain QD23.
The ISPpu12-composite transposon Tn6580a contained a total of 17 resistance genes (Figure 1 and Table S2), which were located at nine different resistance loci: In525 (GCA: dfrA32–ereA–aadA2), a truncated chrA–orf98 unit, Tn6581c, the unnamable In37-like element as described above, ISEc59–aph(4)-Ia–aacC4–IS26 unit, ISCR2–sul2 unit, ISCR2–floR unit, blaCTX-M-14-containing ΔTn6503a, and tetA(C)-containing ΔTn6309 (Figure 7). Three major modular differences were recognized in Tn6580b relative to Tn6580a: i) replacement of Tn6581c by Tn6581b; ii) inversion of the unnamable In37-like element; and iii) insertion of IS26–fosA3–IS26 unit together with Tn4352 at a site between ΔTn6503a and ΔTn6309.
Figure 7 Comparison of Tn6580a from Tn6577 and Tn6580b from Tn6576, and related regions. Genes are denoted by arrows. Genes, mobile elements, and other features are colored based on their functional classification. Shading denotes regions of homology (nucleotide identity ≥95%). Numbers in brackets indicate nucleotide positions within the chromosomes of strains 1701092 and QD23. The accession numbers of Tn6503a (Feng et al., 2015) and Tn6309 (Sun et al., 2016) used as reference are KP987215 and KX710094, respectively.
All of Tn7 (14.1 kb in length), Tn6726 (175.8 kb in length) (Sakamoto et al., 2018), and 40.7-kb Tn7-related element were integrated at a site downstream of the chromosomal gene glmS (glutamine-fructose-6-phosphate aminotransferase). Tn6726 differed from Tn7 by acquisition of In2-3 (GCA: dfrA1) instead of In2-4 (GCA: dfrA1–aadA1) and, moreover, a 162.6-kb ISKpn26-composite transposon Tn6728 was inserted at a site within intI2 of In2-3 (Figure 8). Tn6728 harbored a 40.9-kb MDR region as well as an array of IncHI3 core backbone genes (Figure S3). This 40.9-kb MDR region included a 9.4-kb Tn6909-related region together with ΔTn1548 (these two shared In27), a truncated ISAba14–aphA6–ISAba14 unit, and blaNDM-1-carrying ΔTn125-1 (Figure 9).
Figure 8 Comparison of Tn7 and its two derivatives Tn6726 and 40.7-kb Tn7-related element. Genes are denoted by arrows. Genes, mobile elements, and other features are colored based on their functional classification. Shading denotes regions of homology (nucleotide identity ≥95%). Numbers in brackets indicate nucleotide positions within the chromosomes of strains KP64 and 51003. The accession number of Tn7 used as reference is KX117211.
Figure 9 Organization of MDR region from Tn6728, and comparison to related regions. Genes are denoted by arrows. Genes, mobile elements, and other features are colored based on their functional classification. Shading denotes regions of homology (nucleotide identity ≥95%). Numbers in brackets indicate nucleotide positions within the chromosome of strain KP64. The accession numbers of Tn1548 (Galimand et al., 2005), Tn6909, and ISAba14–aphA6–ISAba14 unit used as reference are AF550415, CP032168, and CP046406, respectively.
Compared with Tn7, 40.7-kb Tn7-related element underwent two insertion events: i) tnsABCDE was truncated by insertion of a 33.6-kb MDR region; and ii) tnsB was interrupted by insertion of ISPrst3; this element could not be discriminated as an intact Tn7-like transposon due to the presence of an incomplete tnsABCDE module (Figure 8). This 33.6-kb MDR region contained In2-16, ΔTn1548, and aphA1-carrying ΔTn4352. In2-16 carried VR1 (GCA: lnu(F)–dfrA1–aadA1a) and two copies of 5.6-kb repeat [VR2 (ISCR1–bleMBL–blaNDM-1 unit) plus Δ3′-CS2] (Figure 10).
Figure 10 Organization of MDR region from 40.7-kb Tn7-related element, and comparison to related regions. Genes are denoted by arrows. Genes, mobile elements, and other features are colored based on their functional classification. Shading denotes regions of homology (nucleotide identity ≥95%). Numbers in brackets indicate nucleotide positions within the chromosome of strain 51003. The accession number of Tn1548 (Galimand et al., 2005) used as reference is AF550415.
ISEc29–mph(E)–IS26/IS15DI unit and ISCR1–armA unit were presented in both ΔTn1548 from Tn6728 and that from 40.7-kb Tn7-related element, whereas In27 was found in the former ΔTn1548 rather than the later one; in addition, ISEc29–mph(E)–IS26/IS15DI unit was interrupted by insertion of two different IS elements ISKpn21 and ISAba24, respectively, in these two ΔTn1548 (Figures 9 and 10).
The 15.5-kb IME Tn6591 (GIsul2) (Wei et al., 2003), initially found in Shigella flexneri 2457T, was integrated into the chromosomal gene guaA (glutamine-hydrolyzing GMP synthase) and had a 12.6-kb backbone (containing attL, int, oriT and attR) with insertion of a single accessory module ISCR2-sul2 unit (Figure 11). Tn6590 (15.6 kb in length) was integrated at the same chromosomal site, and Tn6590 differed from Tn6591 by only truncation of ISCR2–sul2 unit due to insertion of strAB-carrying ΔTn5393c.
Figure 11 Organization of two related IMEs Tn6590 and Tn6591, and comparison to related regions. Genes are denoted by arrows. Genes, mobile elements, and other features are colored based on their functional classification. Shading denotes regions of homology (nucleotide identity ≥95%). Numbers in brackets indicate nucleotide positions within the chromosome of strain 51003. The accession number of Tn5393c (L’Abée-Lund and Sørum, 2000) used as reference is AF262622.
Proteus mirabilis 1701092 carried no plasmids, and all accessory resistance regions (Tn6577 and Tn6589) were located in the chromosome. Besides chromosome-borne accessory resistance regions, an IncFII plasmid p701091-FII (carrying no resistance genes), an IncI plasmid pQD23-CTXM [harboring blaCTX-M-104 and erm(B)], and an IncFII plasmid p51003-FII (containing blaTEM-1B and blaCTX-M-3) together with an Col3M plasmid p51003-qnrD (having qnrD) were identified in Providencia spp. 1701091, K. pneumoniae QD23, and Providencia spp. 51003, respectively. Coexistance of a large array of resistance genes in both chromosome and plasmids of a single bacterial isolate makes it tends to become extensively resistant.
This work identified three ICEs Tn6577, Tn6582 and Tn6576 in total, all of which had essential conjugal transfer genes. Notably, Tn6582 was located within Tn6576. As for conjugation experiments, Tn6577 was transferred from the wild-type isolate (susceptible to rifampin) into E. coli EC600, generating the transconjugant Tn6577–TETA(C)–EC600; Tn6582 could be transferred from the wild-type isolate (non-susceptible to rifampin but susceptible to sodium azide) in E. coli J53 to obtain Tn6582–NDM–J53, but repeated conjugation attempts failed to transfer Tn6576 into E. coli J53. Tn6577–TETA(C)–EC600 was highly resistant to tetracycline and ceftriaxone owing to presence of tetA(C) and blaCTX-M-14. Tn6582–NDM–J53 was highly resistant to ceftriaxone and imipenem resulted from production of NDM enzyme (data not shown). The Ambler class B carbapenemase activity was detected in Tn6582–NDM–J53 and its wild-type isolate.
Since the blaNDM gene was initially identified in India in 2009 (Yong et al., 2009), it spread rapidly all over the world (Dortet et al., 2014). Although blaNDM was initially discovered in a plasmid of K. pneumoniae (Yong et al., 2009), the chromosomal location of blaNDM in Enterobacteriaceae species has been reported in recent years (Pfeifer et al., 2011; Poirel et al., 2011; Girlich et al., 2015; Shen et al., 2017; Sakamoto et al., 2018; Reynolds et al., 2019; Kong et al., 2020). There were few reports related to a detailed genetic dissection of different kinds of blaNDM-carrying accessory resistance regions in the chromosomes (Girlich et al., 2015; Sakamoto et al., 2018; Reynolds et al., 2019; Kong et al., 2020), but none of them had a systematic summary for these blaNDM-carrying mobile genetic elements.
Data presented here involved a total of six chromosomal blaNDM-carrying genetic elements Tn6575, Tn6726, Tn6588, Tn6589, Tn6576, and 40.7-kb Tn7-related element, and the last four were sequenced in this work. These six genetic elements belonged to three different categories: ICEs (Tn6575 and Tn6576), IMEs (Tn6588 and Tn6589), and two derivatives (Tn6726 and 40.7-kb Tn7-related element) of Tn7 unit transposon. Notably, Tn6576 carried another ICE Tn6582. These ICEs and IMEs would have the intercellular self-mobility as they carried essential conjugal transfer genes (Bellanger et al., 2014; Botelho and Schulenburg, 2021). Tn6726 would have the intracellular mobility as it had a complete core transposition module tnsABCDE, while 40.7-kb Tn7-related element would loss its mobility due to lesion in tnsABCDE.
Tn6512-related ICEs were frequently reported in Vibrio, Proteus, and Shewanella (Burrus et al., 2006; Nonaka et al., 2012; Lei et al., 2016; Fang et al., 2018). Tn6575 and Tn6576 were the only two blaNDM-carrying Tn6512-related ICEs (last accessed 15 December 2019). Tn6523-related IMEs were frequently reported in Salmonella and Proteus mirabilis (Hall, 2010; Siebor and Neuwirth, 2013; Sung et al., 2017). This study presented Tn6589, the first blaNDM-carrying Tn6523-related IME. Tn7, and its derivatives had the ability to integrate into bacterial plasmids and chromosomes (Peters, 2014). There were several reports of Tn7 derivatives located in bacterial chromosomes (Chen et al., 2018; Chen et al., 2019). To date, Tn6726 and 40.7-kb Tn7-related element were the only two blaNDM-carrying Tn7 derivatives integrated into chromosomes. Different to 40.7-kb Tn7-related element, Tn6726 carried a series of backbone genes of IncHI3 plasmid, which means that blaNDM together with its surrounding genetic environment in Tn6726 might be originated from a IncHI3 plasmid. In summary, Tn6512-related ICEs, Tn6523-related IMEs, and Tn7 derivatives recently began to be a reservoir of blaNDM genes in Enterobacteriaceae.
Each of these six blaNDM-carrying genetic elements had large accessory resistance regions: i) Tn6575, Tn6588, Tn6589, and 40.7-kb Tn7-related element; each had a single MDR region, 85.0 kb, 90.1 kb, 66.4 kb, and 33.8 kb in length, respectively; ii) Tn6726 contained a 162.6-kb ISKpn26-composite transposon Tn6728 integrated with a 40.9-kb MDR region; and iii) Tn6576 harbored a 406.4-kb ICE Tn6582 (containing two distinct MDR-1 and MDR-2 regions, 38.9 kb and 43.7 kb in length, respectively), and additionally a 55.2-kb ISPpu12-composite Tn6580b that as a whole could be considered as a MDR region. Each of these large MDR regions had a very complex mosaic structure, which was composed of intact or residue mobile genetic elements including ISs, unit or composite transposons, integrons and putative resistance units, and likely assembled from complex transposition and homologous recombination.
Four different Tn125 derivatives, namely ΔTn125-1, ΔTn125-2, ΔTn125-3, and ΔTn125-4 (Figure 12), were identified from the relevant MDR regions of these six blaNDM-carrying genetic elements. ΔTn125-1 from Tn6726 and ΔTn125-2 from Tn6575 highly resembled the prototype Tn125: ΔTn125-1 resulted from the insertion of ISEc33 into the left copy of ISAba125, while terminal truncation of both copies of ISAba125 generated ΔTn125-2. It was thought that ΔTn125-1 and ΔTn125-2 were generated from transposition of Tn125 into Tn6726 and Tn6575, followed by further modular modifications such as insertion and truncation. ΔTn125-3 from 40.7-kb Tn7-related element and ΔTn125-4 from Tn6588, Tn6589 and Tn6576 had very short blaNDM-1/-3-carrying structures. ΔTn125-3 or ΔTn125-4 was captured by ISCR1, generating ISCR1–bleMBL–blaNDM-1 or ISCR1–bleMBL–blaNDM-1/-3–arr-3 unit, respectively. Furthermore, the former unit was integrated into In2-16 (Figure 10) while the later one into In1247Tn6588 (Figure 2), In27Tn6589 (Figure 3) and In1021Tn6576 (Figure 6), manifesting as the VR2 regions of these integrons. Notably, two or more copies of blaNDM-1/-3 genes were found in each of Tn6576, Tn6588, Tn6589, and 40.7-kb Tn7-related element, which resulted from the presence of multiple ≥5.6-kb repeats (each harboring a Tn125 derivative and the other components) in these four genetic elements.
Figure 12 Comparison of Tn125 and its four derivatives. Genes are denoted by arrows. Genes, mobile elements, and other features are colored based on their functional classification. Shading denotes regions of homology (nucleotide identity ≥95%). Numbers in brackets indicate nucleotide positions within Tn6575 and the chromosomes of strains KP64, 51003, 1701091, 1701092, and QD23, respectively. The accession number of Tn125 (Poirel et al., 2012) used as reference is JN872328.
Multiple copies of blaNDM located in a single plasmid or chromosome were reported in previous studies (Jovcić et al., 2013; Shen et al., 2017; Feng et al., 2018), and all these blaNDM genes were around ISCR1. Similarly, Tn6576, Tn6588, Tn6589, and 40.7-kb Tn7-related element in this study also contained ISCR1-around blaNDM genes. It was confirmed that ISCR1 captured adjacent genes (frequently including antibiotic resistance genes) at the end of its initiation of replication (oriIS) through rolling-circle transposition (Toleman et al., 2006). Our sequencing data suggested that ISCR1 might experience multiple rounds of capturing blaNDM and further integrating blaNDM into the integrons, resulting in the presence of multiple copies of ISCR1-accociated blaNDM genes in a single genetic element.
There were eight novel (firstly identified in this study) mobile genetic elements, including three ICEs Tn6576, Tn6577, and Tn6582, two IMEs Tn6588 and Tn6589, two composite transposons Tn6580a and Tn6580b, and one integron In1718. Additional 12 genetic elements (IME: Tn6590; composite transposons: Tn6578, Tn6581a, Tn6581b, Tn6581c, and Tn6728; unit transposons: Tn6726, Tn6727, Tn6909, and Tn6911; IS: ISPvu1; and 40.7-kb Tn7-related element) were newly designated (firstly designated in this study, but with previously determined sequences). The four previously designated ICEs/IMEs SGI1, R391, ICEPvuChnBC22, and GIsul2 were renamed as standard Tn designations Tn6523, Tn6512, Tn6575, and Tn6591, respectively. All the putative resistance units presented in this work were annotated and collected in a custom and yet unpublished database.
This study dealt with an extensive sequence comparison of 12 chromosomal genetic elements, including six blaNDM-carrying ones. All these blaNDM-carrying genetic elements had huge and complex MDR regions. The core blaNDM genetic environments manifested as four different Tn125 derivatives. Notably, two or more copies of blaNDM were found in each of the four genetic elements. Egiht novel mobile elements were firstly identified, including three ICEs Tn6576, Tn6577, and Tn6582, two IMEs Tn6588 and Tn6589, two composite transposons Tn6580a and Tn6580b, and one integron In1718. This study would provide a deeper genetic insight into the chromosomal integration of blaNDM-carrying genetic elements in Enterobacteriaceae.
The datasets generated for this study can be found in the complete chromosomal nucleotide sequences of 1701091, 1701092, QD23 and 51003, which were submitted to GenBank under accession numbers CP042860, CP042857, CP042858 and CP042861, respectively.
This study uses the bacterial isolates obtained from the Chinese livestock farm and public hospitals as listed in Table S1. The local legislation did not require the study to be reviewed or approved by an ethics committee, because the bacterial isolates involved in this study was part of the routine laboratory procedures. The research involving biohazards and all related procedures were approved by the Biosafety Committee of the Beijing Institute of Microbiology and Epidemiology.
DZ and HY conceived the study and designed experimental procedures. XL, YJ, and FC performed the experiments. XL, XJ, and LZ analyzed the data. LH, DW and YS contributed to reagents and materials. XL and ZY wrote the original draft. DZ and HY reviewed the manuscript. All authors contributed to the article and approved the submitted version.
This work was supported by the National Science and Technology Major Project (2018ZX10733402) of China, and the Foundation of the Public Welfare Program of Natural Science Foundation (LGF19H200006) of Zhejiang.
The authors declare that the research was conducted in the absence of any commercial or financial relationships that could be construed as a potential conflict of interest.
The Supplementary Material for this article can be found online at: https://www.frontiersin.org/articles/10.3389/fcimb.2021.690799/full#supplementary-material
Bellanger, X., Payot, S., Leblond-Bourget, N., Guedon, G. (2014). Conjugative and Mobilizable Genomic Islands in Bacteria: Evolution and Diversity. FEMS Microbiol. Rev. 38 (4), 720–760. doi: 10.1111/1574-6976.12058
Boltner, D., MacMahon, C., Pembroke, J. T., Strike, P., Osborn, A. M. (2002). R391: A Conjugative Integrating Mosaic Comprised of Phage, Plasmid, and Transposon Elements. J. Bacteriol. 184 (18), 5158–5169. doi: 10.1128/jb.184.18.5158-5169.2002
Boratyn, G. M., Camacho, C., Cooper, P. S., Coulouris, G., Fong, A., Ma, N., et al. (2013). BLAST: A More Efficient Report With Usability Improvements. Nucleic Acids Res. 41 (Web Server issue), W29–W33. doi: 10.1093/nar/gkt282
Botelho, J., Schulenburg, H. (2021). The Role of Integrative and Conjugative Elements in Antibiotic Resistance Evolution. Trends Microbiol. 29 (1), 8–18. doi: 10.1016/j.tim.2020.05.011
Boutet, E., Lieberherr, D., Tognolli, M., Schneider, M., Bansal, P., Bridge, A. J., et al. (2016). UniProtKB/Swiss-Prot, the Manually Annotated Section of the UniProt KnowledgeBase: How to Use the Entry View. Methods Mol. Biol. 1374, 23–54. doi: 10.1007/978-1-4939-3167-5_2
Boyd, D. A., Peters, G. A., Ng, L., Mulvey, M. R. (2000). Partial Characterization of a Genomic Island Associated With the Multidrug Resistance Region of Salmonella Enterica Typhymurium Dt104. FEMS Microbiol. Lett. 189 (2), 285–291. doi: 10.1111/j.1574-6968.2000.tb09245.x
Brettin, T., Davis, J. J., Disz, T., Edwards, R. A., Gerdes, S., Olsen, G. J., et al. (2015). Rasttk: A Modular and Extensible Implementation of the RAST Algorithm for Building Custom Annotation Pipelines and Annotating Batches of Genomes. Sci. Rep. 5, 8365. doi: 10.1038/srep08365
Burrus, V., Marrero, J., Waldor, M. K. (2006). The Current ICE Age: Biology and Evolution of SXT-Related Integrating Conjugative Elements. Plasmid 55 (3), 173–183. doi: 10.1016/j.plasmid.2006.01.001
Cain, A., Liu, X., Djordjevic, S., Hall, R. (2010). Transposons Related to Tn1696 in IncHI2 Plasmids in Multiply Antibiotic Resistant Salmonella Enterica Serovar Typhimurium From Australian Animals. Microbial Drug Resistance (Larchmont N. Y.) 16 (3), 197–202. doi: 10.1089/mdr.2010.0042
Chen, Y. P., Lei, C. W., Kong, L. H., Zeng, J. X., Zhang, X. Z., Liu, B. H., et al. (2018). Tn6450, A Novel Multidrug Resistance Transposon Characterized in a Proteus Mirabilis Isolate From Chicken in China. Antimicrob. Agents Chemother. 62 (4), e02192–17. doi: 10.1128/aac.02192-17
Chen, Y., Lei, C., Zuo, L., Kong, L., Kang, Z., Zeng, J., et al. (2019). A Novel Cfr-Carrying Tn7 Transposon Derivative Characterized in Morganella Morganii of Swine Origin in China. J. Antimicrob. Chemother. 74 (3), 603–606. doi: 10.1093/jac/dky494
CLSI (2020). Performance Standards for Antimicrobial Susceptibility Testing (Wayne, PA: Clinical and Laboratory Standards Institute).
Delavat, F., Miyazaki, R., Carraro, N., Pradervand, N., van der Meer, J. R. (2017). The Hidden Life of Integrative and Conjugative Elements. FEMS Microbiol. Rev. 41 (4), 512–537. doi: 10.1093/femsre/fux008
Dortet, L., Poirel, L., Nordmann, P. (2014). Worldwide Dissemination of the NDM-Type Carbapenemases in Gram-Negative Bacteria. BioMed. Res. Int., 249856. doi: 10.1155/2014/249856
Edgar, R. C. (2004). MUSCLE: Multiple Sequence Alignment With High Accuracy and High Throughput. Nucleic Acids Res. 32 (5), 1792–1797. doi: 10.1093/nar/gkh340
Fang, Y., Wang, Y., Li, Z., Liu, Z., Li, X., Diao, B., et al. (2018). Distribution and Genetic Characteristics of SXT/R391 Integrative Conjugative Elements in Shewanella Spp. From China. Front. Microbiol. 9, 920. doi: 10.3389/fmicb.2018.00920
Feng, Y., Liu, L., McNally, A., Zong, Z. (2018). Coexistence of Two blaNDM-5 Genes on an IncF Plasmid as Revealed by Nanopore Sequencing. Antimicrob. Agents Chemother. 62 (5), e00110–18. doi: 10.1128/AAC.00110-18
Feng, J., Qiu, Y., Yin, Z., Chen, W., Yang, H., Yang, W., et al. (2015). Coexistence of a Novel KPC-2-Encoding MDR Plasmid and an NDM-1-Encoding pNDM-HN380-Like Plasmid in a Clinical Isolate of Citrobacter Freundii. J. Antimicrob. Chemother. 70 (11), 2987–2991. doi: 10.1093/jac/dkv232
Feng, W., Zhou, D., Wang, Q., Luo, W., Zhang, D., Sun, Q., et al. (2016). Dissemination of IMP-4-Encoding pIMP-HZ1-Related Plasmids Among Klebsiella Pneumoniae and Pseudomonas Aeruginosa in a Chinese Teaching Hospital. Sci. Rep. 6, 33419. doi: 10.1038/srep33419
Frank, J. A., Reich, C. I., Sharma, S., Weisbaum, J. S., Wilson, B. A., Olsen, G. J. (2008). Critical Evaluation of Two Primers Commonly Used for Amplification of Bacterial 16S rRNA Genes. Appl. Environ. Microbiol. 74 (8), 2461–2470. doi: 10.1128/aem.02272-07
Galimand, M., Sabtcheva, S., Courvalin, P., Lambert, T. (2005). Worldwide Disseminated Arma Aminoglycoside Resistance Methylase Gene Is Borne by Composite Transposon Tn1548. Antimicrob. Agents Chemother. 49 (7), 2949–2953. doi: 10.1128/aac.49.7.2949-2953.2005
Gilmour, M. W., Thomson, N. R., Sanders, M., Parkhill, J., Taylor, D. E. (2004). The Complete Nucleotide Sequence of the Resistance Plasmid R478: Defining the Backbone Components of Incompatibility Group H Conjugative Plasmids Through Comparative Genomics. Plasmid 52 (3), 182–202. doi: 10.1016/j.plasmid.2004.06.006
Girlich, D., Dortet, L., Poirel, L., Nordmann, P. (2015). Integration of the blaNDM-1 Carbapenemase Gene Into Proteus Genomic Island 1 (PGI1-PmPEL) in a Proteus Mirabilis Clinical Isolate. J. Antimicrob. Chemother. 70 (1), 98–102. doi: 10.1093/jac/dku371
Hackl, T., Hedrich, R., Schultz, J., Forster, F. (2014). Proovread: Large-Scale High-Accuracy PacBio Correction Through Iterative Short Read Consensus. Bioinformatics 30 (21), 3004–3011. doi: 10.1093/bioinformatics/btu392
Hall, R. M. (2010). Salmonella Genomic Islands and Antibiotic Resistance in Salmonella Enterica. Future Microbiol. 5 (10), 1525–1538. doi: 10.2217/fmb.10.122
Jia, B., Raphenya, A. R., Alcock, B., Waglechner, N., Guo, P., Tsang, K. K., et al. (2017). CARD 2017: Expansion and Model-Centric Curation of the Comprehensive Antibiotic Resistance Database. Nucleic Acids Res. 45 (D1), D566–D573. doi: 10.1093/nar/gkw1004
Jovcić, B., Lepsanović, Z., Begović, J., Rakonjac, B., Perovanović, J., Topisirović, L., et al. (2013). The Clinical Isolate Pseudomonas Aeruginosa MMA83 Carries Two Copies of the blaNDM-1 Gene in a Novel Genetic Context. Antimicrob. Agents Chemother. 57 (7), 3405–3407. doi: 10.1128/aac.02312-12
Kong, L. H., Xiang, R., Wang, Y. L., Wu, S. K., Lei, C. W., Kang, Z. Z., et al. (2020). Integration of the blaNDM-1 Carbapenemase Gene Into a Novel SXT/R391 Integrative and Conjugative Element in Proteus Vulgaris. J. Antimicrob. Chemother. 75 (6), 1439–1442. doi: 10.1093/jac/dkaa068
L’Abée-Lund, T. M., Sørum, H. (2000). Functional Tn5393-like Transposon in the R Plasmid pRAS2 From the Fish Pathogen Aeromonas Salmonicida Subspecies Salmonicida Isolated in Norway. Appl. Environ. Microbiol. 66 (12), 5533–5535. doi: 10.1128/aem.66.12.5533-5535.2000
Lei, C. W., Zhang, A. Y., Wang, H. N., Liu, B. H., Yang, L. Q., Yang, Y. Q. (2016). Characterization of SXT/R391 Integrative and Conjugative Elements in Proteus Mirabilis Isolates From Food-Producing Animals in China. Antimicrob. Agents Chemother. 60 (3), 1935–1938. doi: 10.1128/aac.02852-15
Moura, A., Soares, M., Pereira, C., Leitão, N., Henriques, I., Correia, A. (2009). INTEGRALL: A Database and Search Engine for Integrons, Integrases and Gene Cassettes. Bioinformatics 25 (8), 1096–1098. doi: 10.1093/bioinformatics/btp105
Nonaka, L., Maruyama, F., Miyamoto, M., Miyakoshi, M., Kurokawa, K. (2012). And Masuda, MNovel Conjugative Transferable Multiple Drug Resistance Plasmid pAQU1 From Photobacterium Damselae Subsp. Damselae Isolated From Marine Aquaculture Environment. Microbes Environ. 27 (3), 263–272. doi: 10.1264/jsme2.me11338
O’Leary, N. A., Wright, M. W., Brister, J. R., Ciufo, S., Haddad, D., McVeigh, R., et al. (2016). Reference Sequence (RefSeq) Database at NCBI: Current Status, Taxonomic Expansion, and Functional Annotation. Nucleic Acids Res. 44 (D1), D733–D745. doi: 10.1093/nar/gkv1189
Partridge, S. R., Brown, H. J., Stokes, H. W., Hall, R. M. (2001). Transposons Tn1696 and Tn21 and Their Integrons In4 and In2 Have Independent Origins. Antimicrob. Agents Chemother. 45 (4), 1263–1270. doi: 10.1128/aac.45.4.1263-1270.2001
Partridge, S. R., Ginn, A. N., Paulsen, I. T., Iredell, J. R. (2012). Pel1573 Carrying blaIMP-4, From Sydney, Australia, Is Closely Related to Other IncL/M Plasmids. Antimicrob. Agents Chemother. 56 (11), 6029–6032. doi: 10.1128/aac.01189-12
Peters, J. E., Craig, N. L. (2001). Tn7: Smarter Than We Thought. Nat. Rev. Mol. Cell Biol. 2 (11), 806–814. doi: 10.1038/35099006
Pfeifer, Y., Witte, W., Holfelder, M., Busch, J., Nordmann, P., Poirel, L. (2011). NDM-1-Producing Escherichia Coli in Germany. Antimicrob. Agents Chemother. 55 (3), 1318–1319. doi: 10.1128/AAC.01585-10
Poirel, L., Bonnin, R. A., Boulanger, A., Schrenzel, J., Kaase, M., Nordmann, P. (2012). Tn125-Related Acquisition of blaNDM-Like Genes in Acinetobacter Baumannii. Antimicrob. Agents Chemother. 56 (2), 1087–1089. doi: 10.1128/aac.05620-11
Poirel, L., Dortet, L., Bernabeu, S., Nordmann, P. (2011). Genetic Features of blaNDM-1-Positive Enterobacteriaceae. Antimicrob. Agents Chemother. 55 (11), 5403–5407. doi: 10.1128/AAC.00585-11
Reynolds, M. E., Phan, H. T. T., George, S., Hubbard, A. T. M., Stoesser, N., Maciuca, I. E., et al. (2019). Occurrence and Characterization of Escherichia Coli ST410 Co-Harbouring blaNDM-5, blaCMY-42 and blaTEM-190 in a Dog From the UK. J. Antimicrob. Chemother. 74 (5), 1207–1211. doi: 10.1093/jac/dkz017
Richter, M., Rosselló-Móra, R. (2009). Shifting the Genomic Gold Standard for the Prokaryotic Species Definition. Proc. Natl. Acad. Sci. U. S. A. 106 (45), 19126–19131. doi: 10.1073/pnas.0906412106
Roberts, A. P., Chandler, M., Courvalin, P., Guedon, G., Mullany, P., Pembroke, T., et al. (2008). Revised Nomenclature for Transposable Genetic Elements. Plasmid 60 (3), 167–173. doi: 10.1016/j.plasmid.2008.08.001
Saeed, A. I., Sharov, V., White, J., Li, J., Liang, W., Bhagabati, N., et al. (2003). TM4: A Free, Open-Source System for Microarray Data Management and Analysis. Biotechniques 34 (2), 374–378. doi: 10.2144/03342mt01
Sakamoto, N., Akeda, Y., Sugawara, Y., Takeuchi, D., Motooka, D., Yamamoto, N., et al. (2018). Genomic Characterization of Carbapenemase-Producing Klebsiella Pneumoniae With Chromosomally Carried Blandm-1. Antimicrob. Agents Chemother. 62 (12), e01520–18. doi: 10.1128/aac.01520-18
Shen, P., Yi, M., Fu, Y., Ruan, Z., Du, X., Yu, Y., et al. (2017). Detection of an Escherichia Coli Sequence Type 167 Strain With Two Tandem Copies of blaNDM-1 in the Chromosome. J. Clin. Microbiol. 55 (1), 199–205. doi: 10.1128/jcm.01581-16
Siebor, E., Neuwirth, C. (2013). Emergence of Salmonella Genomic Island 1 (SGI1) Among Proteus Mirabilis Clinical Isolates in Dijon, France. J. Antimicrob. Chemother. 68 (8), 1750–1756. doi: 10.1093/jac/dkt100
Siguier, P., Perochon, J., Lestrade, L., Mahillon, J., Chandler, M. (2006). Isfinder: The Reference Centre for Bacterial Insertion Sequences. Nucleic Acids Res. 34 (Database issue), D32–D36. doi: 10.1093/nar/gkj014
Sung, J. Y., Kim, S., Kwon, G., Koo, S. H. (2017). Molecular Characterization of Salmonella Genomic Island 1 in Proteus Mirabilis Isolates From Chungcheong Province, Korea. J. Microbiol. Biotechnol. 27 (11), 2052–2059. doi: 10.4014/jmb.1708.08040
Sun, F., Zhou, D., Sun, Q., Luo, W., Tong, Y., Zhang, D., et al. (2016). Genetic Characterization of Two Fully Sequenced Multi-Drug Resistant Plasmids pP10164-2 and pP10164-3 From Leclercia Adecarboxylata. Sci. Rep. 6, 33982. doi: 10.1038/srep33982
Toleman, M. A., Bennett, P. M., Walsh, T. R. (2006). ISCR Elements: Novel Gene-Capturing Systems of the 21st Century? Microbiol. Mol. Biol. Rev. 70 (2), 296–316. doi: 10.1128/MMBR.00048-05
Wang, M., Tran, J. H., Jacoby, G. A., Zhang, Y., Wang, F., Hooper, D. C. (2003). Plasmid-Mediated Quinolone Resistance in Clinical Isolates of Escherichia Coli From Shanghai, China. Antimicrob. Agents Chemother. 47 (7), 2242–2248. doi: 10.1128/aac.47.7.2242-2248.2003
Wei, J., Goldberg, M. B., Burland, V., Venkatesan, M. M., Deng, W., Fournier, G., et al. (2003). Complete Genome Sequence and Comparative Genomics of Shigella Flexneri Serotype 2a Strain 2457T. Infection Immun. 71 (5), 2775–2786. doi: 10.1128/iai.71.5.2775-2786.2003
Wrighton, C. J., Strike, P. (1987). A Pathway for the Evolution of the Plasmid NTP16 Involving the Novel Kanamycin Resistance Transposon Tn4352. Plasmid 17 (1), 37–45. doi: 10.1016/0147-619x(87)90006-0
Wu, W., Feng, Y., Tang, G., Qiao, F., McNally, A., Zong, Z. (2019). NDM Metallo-β-Lactamases and Their Bacterial Producers in Health Care Settings. Clin. Microbiol. Rev. 32 (2), e00115–18. doi: 10.1128/cmr.00115-18
Yong, D., Toleman, M. A., Giske, C. G., Cho, H. S., Sundman, K., Lee, K., et al. (2009). Characterization of a New Metallo-Beta-Lactamase Gene, blaNDM-1, and a Novel Erythromycin Esterase Gene Carried on a Unique Genetic Structure in Klebsiella Pneumoniae Sequence Type 14 From India. Antimicrob. Agents Chemother. 53 (12), 5046–5054. doi: 10.1128/AAC.00774-09
Keywords: Enterobacteriaceae, chromosomal integration, blaNDM, multidrug resistance, mobile genetic elements
Citation: Luo X, Yin Z, Zeng L, Hu L, Jiang X, Jing Y, Chen F, Wang D, Song Y, Yang H and Zhou D (2021) Chromosomal Integration of Huge and Complex blaNDM-Carrying Genetic Elements in Enterobacteriaceae. Front. Cell. Infect. Microbiol. 11:690799. doi: 10.3389/fcimb.2021.690799
Received: 04 April 2021; Accepted: 17 May 2021;
Published: 15 June 2021.
Edited by:
Zhang Wan Jiang, Chinese Academy of Agricultural Sciences (CAAS), ChinaReviewed by:
Ruichao Li, Yangzhou University, ChinaCopyright © 2021 Luo, Yin, Zeng, Hu, Jiang, Jing, Chen, Wang, Song, Yang and Zhou. This is an open-access article distributed under the terms of the Creative Commons Attribution License (CC BY). The use, distribution or reproduction in other forums is permitted, provided the original author(s) and the copyright owner(s) are credited and that the original publication in this journal is cited, in accordance with accepted academic practice. No use, distribution or reproduction is permitted which does not comply with these terms.
*Correspondence: Dongsheng Zhou, ZG9uZ3NoZW5nemhvdTE5NzdAZ21haWwuY29t; Huiying Yang, eWh5MzI0QGFsaXl1bi5jb20=
†These authors have contributed equally to this work
Disclaimer: All claims expressed in this article are solely those of the authors and do not necessarily represent those of their affiliated organizations, or those of the publisher, the editors and the reviewers. Any product that may be evaluated in this article or claim that may be made by its manufacturer is not guaranteed or endorsed by the publisher.
Research integrity at Frontiers
Learn more about the work of our research integrity team to safeguard the quality of each article we publish.