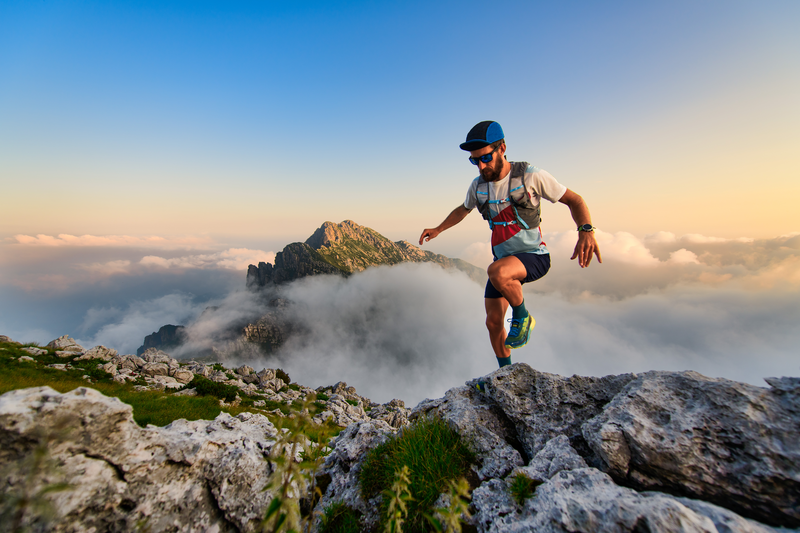
94% of researchers rate our articles as excellent or good
Learn more about the work of our research integrity team to safeguard the quality of each article we publish.
Find out more
ORIGINAL RESEARCH article
Front. Cell. Infect. Microbiol. , 30 April 2021
Sec. Molecular Bacterial Pathogenesis
Volume 11 - 2021 | https://doi.org/10.3389/fcimb.2021.673222
This article is part of the Research Topic Vibrio Virulence Regulation and Host Interactions View all 13 articles
RtxA1 is a major cytotoxin of Vibrio vulnificus (V. vulnificus) causing fatal septicemia and necrotic wound infections. Our previous work has shown that RpoS regulates the expression and secretion of V. vulnificus RtxA1 toxin. This study was conducted to further investigate the potential mechanisms of RpoS on RtxA1 secretion. First, V. vulnificus TolCV1 and TolCV2 proteins, two Escherichia coli TolC homologs, were measured at various time points by Western blotting. The expression of TolCV1 was increased time-dependently, whereas that of TolCV2 was decreased. Expression of both TolCV1 and TolCV2 was significantly downregulated in an rpoS deletion mutation. Subsequently, we explored the roles of TolCV1 and TolCV2 in V. vulnificus pathogenesis. Western blot analysis showed that RtxA1 toxin was exported by TolCV1, not TolCV2, which was consistent with the cytotoxicity results. Furthermore, the expression of TolCV1 and TolCV2 was increased after treatment of the host signal bile salt and the growth of tolCV1 mutant was totally abolished in the presence of bile salt. A tolCV1 mutation resulted in significant reduction of V. vulnificus induced-virulence in mice. Taken together, TolCV1 plays key roles in RtxA1 secretion, bile salt resistance, and mice lethality of V. vulnificus, suggesting that TolCV1 could be an attractive target for the design of new medicines to treat V. vulnificus infections.
Vibrio vulnificus (V. vulnificus) is a halophilic Gram-negative bacterium that causes fatal primary septicemia and necrotizing wound infections, and is commonly transmitted by seawater exposure or contaminated seafood consumption (Baker-Austin et al., 2018; Park and Lee, 2018). V. vulnificus infections usually occur in individuals with underlying conditions such as liver diseases, diabetes, and immune disorder (Baker-Austin et al., 2018). RtxA1, a member of multifunctional autoprocessing repeats-in-toxin (MARTX) family, is a major cytotoxin of V. vulnificus (Kwak et al., 2011; Roig et al., 2011). Our previous studies have demonstrated that the expression of RtxA1 is dramatically increased after the close contact of V. vulnificus with host cells (Kim et al., 2008), and RtxA1 toxin induces the acute cell death by forming pores in the cellular membrane (Kim et al., 2013). Additionally, the expression and secretion of RtxA1 toxin are regulated by the sigma factor RpoS (Guo et al., 2018).
V. vulnificus TolC, an outer membrane channel protein that participates in the assembly of tripartite efflux pumps, has been reported to be involved in the secretion of RtxA1 toxin (Hwang et al., 2011). In V. vulnificus, there are two Escherichia coli (E.coli) TolC homologs, TolCV1 and TolCV2 (VVM0602608 and VVM0604400), showing 51.3% and 29.6% sequence identity, respectively (Lee et al., 2013). TolC is able to co-operate with several inner membrane complexes and thereby participates in the assembly of different tripartite efflux pumps, such as AcrAB-TolC (Du et al., 2014), MacAB-TolC (Du et al., 2015), EmrAB-TolC (Puértolas-Balint et al., 2020), and HlyBD-TolC (Kanonenberg et al., 2019). Some studies have demonstrated that V. vulnificus TolCV1 and TolCV2 can interact with E.coli membrane fusion protein AcrA and MacA to partially assume the efflux pump function of E.coli TolC (Lee et al., 2013; Lee et al., 2014b). A wide variety of substrates are directly transported across the envelope through TolC-dependent export and efflux system, which endues TolC with multiple functions and makes it to be critical for bacterial survival in the environment rich with pernicious agents or under extremal conditions (Langevin and Dunlop, 2018). Recent studies have demonstrated that AcrAB-TolC and its homologs are crucial for the drug-resistance acquisition in Gram-negative bacteria (El Meouche and Dunlop, 2018; Nolivos et al., 2019). Interestingly, V. vulnificus TolCV1 and TolCV2 are also associated with the efflux of diverse antibiotics (Lee et al., 2014a; Lee et al., 2015), biofilm formation (Lee et al., 2007), and iron-uptake system (Kawano et al., 2014). Several lines of evidence indicate that TolC affects virulence expression in Vibrio cholerae, Francisella tularensis and Enterobacter cloacae (Gil et al., 2006; Minato et al., 2011; Pérez et al., 2012) and is indispensable for bile salt resistance and colonization in Vibrio cholerae (Bina and Mekalanos, 2001). These fundings indicate that outer membrane TolC possesses multiple functions in various strains, which drove us to further explore more functions of TolCV1 and TolCV2 in V. vulnificus.
This study was conducted to further investigate the potential mechanisms of RpoS on RtxA1 secretion. First, we measured the effect of rpoS mutation on TolCV1 and TolCV2 expression by Western blotting. We also examined the roles of TolCV1 and TolCV2 in host factor-induced RtxA1 toxin secretion and expression, cytotoxicity to host cells, bile salt resistance and mice lethality.
The bacterial strains and plasmids used in this study are listed in Table 1. Bacterial strains were reserved at −80°C in growth medium with 20% (vol/vol) glycerol. Unless stated otherwise, all V. vulnificus strains were propagated in Luria-Bertani broth (LB broth, Difco, Becton-Dickinson, Sparks, MD, USA) at 37°C in a shaking incubator (200 rpm).
The suicide plasmid pDM4 was used to construct an in-frame tolCV2 deletion mutant of V. vulnificus MO6-24/O as described previously (Guo et al., 2020). The upstream and downstream DNA fragments of tolCV2 were amplified by PCR from V. vulnificus MO6-24/O chromosomal DNA (accession number NC_014966.1) (Park et al., 2011) using the primer pairs (tolCV2-1: 5′-GGAATTCTCTGCTGTGAGCGTTGCGCT-3′; tolCV2-2: 5′-GTTGCAATAATTAACCATGCGCGCCTCCCATCATC-3′) and (tolCV2-3: 5′-GCATGGTTAATTATTGCAACAAACATGGCAAACG-3′; tolCV2-4: 5′-GCTCTAGAATATCCCGTGATCACCGG-3′), respectively. These two DNA fragments were used as templates for the second crossover PCR with tolCV2-1 and tolCV2-4 as primers. The resulting PCR products were ligated into suicide plasmid pDM4 and transformed into E. coli SY327λpir and E. coli SM10λpir, generating the pDM4::△tolCV2, which was conjugally transferred into MO6-24/O via triparental mating. The stable transconjugants were selected on TCBS agar plates with chloramphenicol and then heart infusion (HI) agar plates with 10% sucrose. The mutation was verified via PCR and Western blot analysis.
The complementation of tolCV2 mutant was constructed using plasmid pLAFR3 with a primer pair (tolCV2-F-EcoRI: 5′-CGGAATTCGTCCAGACATTAAAGCCG-3′; tolCV2-R-PstI: 5′-AAAACTGCAGGTTGCAATAACGCGCTC-3′). The DNA fragment containing tolCV2 gene and flanking DNA sequence was amplified by PCR and then cloned into pLAFR3, resulting in the pLAFR3::tolCV2, which was introduced into tolCV2 mutant strain by triparental mating. Stable transconjugants were selected and confirmed by PCR and Western blot analysis.
Rabbit polyclonal anti-TolCV2 antibody was produced as described in our previous study (Guo et al., 2020). The DNA fragment encoding tolCV2 was amplified from V. vulnificus MO6-24/O chromosomal DNA by PCR with the following primer pair (tolCV2-F-EcoRI: 5′-CGGAATTCATGGTTAACAAGCACCTATC-3′; tolCV2-R-XhoI: 5′-CCGCTCGAGTCATGAATGAAAAGCTCGG-3′). The resulting PCR products were then inserted into the expression vector PGEX-4T-1 (Amersham Pharmacia Biotech Inc., Piscataway, NJ) and the GST-TolCV2 fusion protein was purified by GST SpinTrap columns (GE Healthcare Life Science, Buckinghamshire, UK). The rabbit polyclonal anti-TolCV2 antibody was produced using New Zealand white rabbits, and the specificity of the polyclonal antibody against TolCV2 was confirmed by Western blotting.
Single colony of V. vulnificus strain was inoculated into LB broth and cultured overnight at 37°C in a shaking incubator (200 rpm). The overnight cultures were diluted 200-fold with fresh LB medium and subsequently cultured at 37°C. The pellets were washed twice with DPBS (Welgene, Gyeongsan-si, Gyeongsangbuk-do, South Korea). Bacterial cells (2×108 CFU) resuspended in SDS-PAGE sample buffer were boiled at 100°C for 10 min and separated by 10% SDS-PAGE gels before being transferred to polyvinylidene fluoride (PVDF) membranes (Millipore, Bedford, MA, USA). The membranes were then blocked with 5% skim milk in Tris‐buffered saline containing 0.05% Tween 20 (TBS/T) for 2 h at room temperature and incubated with primary antibodies specific to TolCV1 (Guo et al., 2020), TolCV2 or RtxA1-D2 (Kim et al., 2013) at 4°C overnight. The membranes were rinsed with TBS/T for 1 h, incubated with horseradish peroxidase (HRP) linked anti-rabbit secondary antibody (Jackson ImmunoResearch, West Grove, PA, USA) for 1 h, and washed again with TBS/T for another 1 h. Protein bands were detected by the ECL Western blot analysis system (Advansta, Menlo Park, CA, USA). The intensity of bands was measured in arbitrary units (AU) by using ImageJ 1.50i software (National Institute of Health, USA).
HeLa cells (Korea Cell Line Bank, Seoul, Korea) were cultured in Dulbecco’s modified Eagle’s medium (DMEM, Welgene, Daegu, Korea) containing 10% fetal bovine serum (ThermoFisher Scientific, Waltham, MA, US). V. vulnificus strains from cultures grown overnight in LB broth were diluted 200-fold with 10 mL of fresh LB broth in a shaking incubator at 37°C for another 3 h. HeLa cells (5×105 cells/well) grown overnight in 6-well plates were washed with serum free DMEM medium before being infected with bacteria at an MOI of 20 for 120, 150, or 180 min. The supernatants (300 µL) were precipitated by the addition of 3-fold ice-cold acetone. Bacterial pellets of the supernatants and HeLa cells were lysed with the cell lysis buffer (Promega, Madison, WI, USA) containing protease inhibitor cocktail (Sigma-Aldrich, St. Louis, MO, USA) shaking on ice for 30 min and harvested by centrifugation at 13000 rpm for 10 min after scrapping. The protein concentration was quantified using the Bradford’s reagent (Bio-Rad Laboratories, USA). Equal amounts of protein were separated by NuPAGE™ 3%-8% Tris-Acetate gels (Thermo Fisher Scientific, Carlsbad, CA, USA) and subsequently subjected to Western blotting using an anti-RtxA1 antibody specific to amino acid 1492-1970 (RTX-D2 domain). Western blotting was performed as described above.
HeLa cells seeded into 48-well plates (5×104 cells/well) overnight were washed with serum-free DMEM before being infected with 3 h or 9 h cultured bacterial cells of V. vulnificus strains at an MOI of 20 for 120, 150, or 180 min. A CytoTox96 Non-Radioactive Cytotoxicity Assay kit (Promega, Madison, WI, USA) was used to measure the amount of lactate dehydrogenase (LDH) released in the supernatants.
V. vulnificus wild-type grown overnight in LB broth were diluted 200-fold with fresh LB medium and then cultured with or without 0.02% bile salt at 37°C for 3 h or 9 h. Equal number of bacterial cells (2×108 CFU) were harvested and resolved in sample buffer for Western blotting, which was performed as described above.
Thiosulphate-citrate-bile salt sucrose (TCBS) agar plate (Difco, Becton-Dickinson, Sparks, MD, USA) is usually used for the selective isolation of Vibrio species (Di Pinto et al., 2011), which contains bile salts as one of the main ingredients. To verify the growth conditions of V. vulnificus strains in the presence of bile salt, their overnight cultures (3 µL) were dropped on TCBS agar and then incubated at 37°C overnight.
Overnight cultures of V. vulnificus strains were diluted 200-fold with fresh LB media in the presence or absence of 0.02% bile salt. Bacterial cells were cultured in a 37°C shaking incubator and the growth was measured using a spectrophotometer at 600 nm every 2 h.
Eight-week-old female ICR mice (DBL, Umsung, South Korea) were kept under specific-pathogen-free conditions. Mice were infected with V. vulnificus wild-type, tolCV1 mutant, tolCV2 mutant, or rtxA1 mutant strains (1×107 CFU/mouse) through intraperitoneal (i.p.) injection. Five mice were tested for each group and infected mice were subsequently observed for 72 h. All procedures involving animals were performed in accordance with the guidelines of the Chonnam National University Animal Care and Use Committee (IACUC-YB-2020-81).
We previously reported that sigma factor RpoS regulates RtxA1 expression and secretion to influence V. vulnificus pathogenesis (Guo et al., 2018). Hence, the current study was designed to explore whether RpoS represses RtxA1 secretion associated with outer membrane proteins TolCV1 and TolCV2. First, the TolCV1 and TolCV2 expression levels were measured at various time points and results showed that the expression levels of TolCV1 were time-dependently increased, unlike those of TolCV2, which were decreased (Figure 1A). Subsequently, the expression levels of TolCV1 and TolCV2 were compared between V. vulnificus wild-type and rpoS mutant strains at 3 and 9 h. Results showed that both TolCV1 and TolCV2 expression levels in the rpoS mutant strain were significantly lower than those in the wild-type (Figure 1B), indicating that RpoS acts as a positive regulator in TolCV1 and TolCV2 expressions. These data suggest that RpoS plays an essential role in the time-dependent expression of the outer membrane proteins TolCV1 and TolCV2 in V. vulnificus.
Figure 1 Effect of rpoS mutation on the expression of outer membrane proteins TolCV1 and TolCV2. Overnight cultures of each V. vulnificus strains were diluted 200-fold with fresh LB broth and grown in a 37°C shaking incubator. Equivalent number of bacterial cells (2×108 CFU) were harvested at indicated time points followed by Western blotting with anti-TolCV1 or anti-TolCV2 primary antibodies. (A) Western blot analysis of V. vulnificus wild-type cells harvested at 3, 6, 9, 12, and 24 h cultures (B) Western blot analysis of V. vulnificus wild-type and its rpoS mutant cells collected at 3 h and 9 h cultures. Relative protein levels were quantified using ImageJ software. Results are representative of at least three independent experiments. Abbreviation: wt, wild-type; tolCV1-: tolCV1 mutant of MO6-24/O; tolCV1- + pLAFR3::tolCV1: complementary strain of tolCV1 mutant; tolCV2-: tolCV2 mutant of MO6-24/O; tolCV2- + pLAFR3::tolCV2: complementary strain of tolCV2 mutant; rpoS-: rpoS mutant of MO6-24/O.
Only when the close contact of V. vulnificus with host cells was allowed, the expression of RtxA1 toxin would be dramatically upregulated to induce host cell death within a short time (Kim et al., 2008). To explore if TolCV1 and TolCV2 are required for the host factor induced-RtxA1 expression and secretion, the tolCV1 and tolCV2 mutant strains were constructed in V. vulnificus MO6-24/O strain. HeLa cells were infected with either V. vulnificus wild-type, rpoS mutant, tolCV1 mutant, tolCV2 mutant, or rtxA1 mutant strains at an MOI of 20 for 120, 150, or 180 min. The protein levels of RtxA1 in the supernatants and HeLa cell lysates were determined by Western blotting. Similar to our previous report (Guo et al., 2018), the rpoS mutation resulted in a decreased level of host factor-induced RtxA1 expression in the cell lysates (Figure 2). RtxA1 proteins in HeLa cell lysates was detected without differences in infection with V. vulnificus wild-type, tolCV1 mutant, or tolCV2 mutant at any time (Figure 2). Additionally, RtxA1 protein was completely vanished in the supernatants of tolCV1 mutant-infected HeLa cells, suggesting that RtxA1 toxin was exported by TolCV1 only, not TolCV2. Therefore, we can draw a conclusion that TolCV1 and TolCV2 do not affect the host factor-induced RtxA1 expression and TolCV1 is responsible for RtxA1 secretion.
Figure 2 Effect of tolCV1 or tolCV2 mutation on RtxA1 expression and secretion after contact with host cells. HeLa cells cultured overnight in 6-well plates (5×105 cells/well) were infected with either V. vulnificus wild-type (wt), rpoS mutant (rpoS-), tolCV1 mutant (tolCV1-), tolCV2 mutant (tolCV2-), or rtxA1 mutant (rtxA1-) strains at an MOI of 20 for 120, 150, or 180 min. RtxA1 protein in the supernatants and cell lysates were detected by Western blot analysis with an RtxA1 antibody specific to amino acids 1492-1970 (RtxA1-D2 domain). Relative protein levels were quantified using ImageJ software. Results are representative of at least three independent experiments.
To determine the roles of TolCV1 and TolCV2 in V. vulnificus virulence, the cytotoxicity of these strains to HeLa cells was measured by LDH assay. Consistent with Western blotting results, the rpoS mutant strain exhibited a decreased and delayed cytotoxicity to HeLa cells (Figure 3). The mutation of tolCV1 considerably decreased cell cytotoxicity of V. vulnificus, but that of tolCV2 did not exhibit a significant effect (Figure 3), suggesting that TolCV2 was not involved in V. vulnificus-induced cytotoxicity to HeLa cells. In conclusion, TolCV1 affects V. vulnificus cytotoxicity by controlling RtxA1 secretion, which is regulated by RpoS.
Figure 3 Effect of tolCV1 or tolCV2 mutation on V. vulnificus cytotoxicity to host cells. HeLa cells cultured overnight in 48-well plates (5×104 cells/well) were infected with 3 h or 9 h cultured bacterial cells of V. vulnificus wild-type (wt), rpoS mutant (rpoS-), tolCV1 mutant (tolCV1-), tolCV2 mutant (tolCV2-), or rtxA1 mutant (rtxA1-) strains at an MOI of 20 for 120, 150, or 180 min. The cytotoxicity of V. vulnificus strains was determined by measuring the amount of lactate dehydrogenase (LDH) released in the cell culture supernatants. Results are representative of at least three independent experiments. The statistical differences were analyzed by Student’s t-test (*** and ** indicate P < 0.001 and P < 0.01 versus the wild-type treated group, respectively).
To investigate the potential roles of TolCV1 and TolCV2 after bacteria enter the human body, we measured TolCV1 and TolCV2 expression levels after treatment with the host signal bile salt. The expression of TolCV1 and TolCV2 was upregulated in LB broth with 0.02% bile salt (Figure 4A). Consequently, V. vulnificus wild-type, rpoS mutant, tolCV1 mutant, tolCV2 mutant, or rtxA1 mutant strains were cultured on TCBS agar plates to verify their growth conditions in the presence of bile salt. The tolCV1 mutant strain showed growth defect on TCBS agar plate, which was restored by the in trans complementation with a plasmid-encoded wild-type allele (Figure 4B). Furthermore, we monitored the growth rates of the wild-type, rpoS mutant, tolCV mutant strains in LB broth containing 0.02% bile salt. Results indicated that the growth of tolCV1 mutant was totally abolished and that of rpoS mutant was slightly suppressed in LB broth with 0.02% bile salt (Figure 4C). Based on these results, we concluded that TolCV1 is responsible for V. vulnificus growth in the presence of bile salt, and is crucial for V. vulnificus successful infection within the host.
Figure 4 Effect of tolCV1 or tolCV2 mutation on V. vulnificus resistance to bile salt. (A) V. vulnificus cells grown overnight in LB broth were diluted 200-fold with fresh LB medium containing 0.02% bile salt for 3 or 9 h. Equivalent number of bacterial cells (2×108 CFU) collected at 3 or 9 h cultures were subjected to Western blotting using anti-TolCV1 or anti-TolCV2 primary antibodies. Relative protein levels were quantified using ImageJ software. (B) Three microliters of overnight cultures of V. vulnificus strains were dropped on TCBS agar and then incubated overnight at 37°C. (C) Overnight cultures of V. vulnificus wild-type, rpoS mutant, or tolCV1 mutant strains were diluted 200-fold with fresh LB media in the presence or absence of 0.02% bile salt. Bacterial cells were cultured in a shaking incubator at 37°C and the growth was measured using a spectrophotometer at 600 nm every 2 h. Results are representative of at least three independent experiments. Abbreviation: wt, wild-type; tolCV1-: tolCV1 mutant of MO6-24/O; tolCV1- + pLAFR3::tolCV1: complementary strain of tolCV1 mutant; tolCV2-: tolCV2 mutant of MO6-24/O; rpoS-: rpoS mutant of MO6-24/O; rtxA1-: rtxA1 mutant of MO6-24/O.
To further study the roles of TolC proteins in vivo, mice were infected with either V. vulnificus wild-type, tolCV1 mutant, tolCV2 mutant, or rtxA1 mutant strains by intraperitoneal injection and their survival times were observed in the following 72 h. As previously reported, the mice infected with V. vulnificus wild-type exhibited lower activity, rapid mortality, and breathing difficultly after injection, while the rtxA1 mutant-infected mice had improved survival rates (Figure 5). Moreover, the mice infected with tolCV1 mutant performed better than mice infected with rtxA1 mutant, showing a longer survival time and higher survival rate (Figure 5). In contrast, the tolCV2 mutant-infected mice were expeditiously dead and indistinguishable from the wild-type-infected mice (Figure 5).
Figure 5 Effect of tolCV1 or tolCV2 mutation on mice lethality caused by V. vulnificus. Eight-week-old female ICR mice were infected with V. vulnificus wild-type (wt), tolCV1 mutant (tolCV1-), tolCV2 mutant (tolCV2-), or rtxA1 mutant (rtxA1-) strains (1×107 CFU/mouse) via intraperitoneal injection. Infected mice were observed for 72 h after injection and their survival times were recorded. Five mice were tested for each group.
In this study, we first found that RpoS is a positive regulatory factor of TolCV1 and TolCV2 expression (Figure 1B) and TolCV1 is responsible for exporting RtxA1 (Figure 2). Therefore, we concluded that the regulatory effect of RpoS on RtxA1 secretion was achieved by regulating the expression of TolCV1. In a previous report (Boardman and Satchell, 2004), it was suggested that RtxA1 was secreted by a Type I secretion system constituted by RtxB, RtxE, RtxD, and TolC in Vibrio cholerae. Therefore, RtxB, RtxE, RtxD may be the potential partners of TolCV1 collaboratively participated in the secretion of RtxA1 in V. vulnificus, which needs to be further confirmed. Additionally, the secretion of V. vulnificus hemolysin (VvhA) has been reported to be mediated by the Type II secretion system, and irrelevant with regard to TolC (Hwang et al., 2011). Subsequently, we explored the roles of TolCV1 and TolCV2 in V. vulnificus infection. The tolCV1 mutation resulted in V. vulnificus growth defect in the presence of host signal bile salt (Figures 4B, C), which possibly explains why tolCV1 mutation significantly reduced V. vulnificus-induced virulence in mice (Figure 5). RpoS is a stress sigma factor that is strongly induced during bacterial growth into stationary phase (Battesti et al., 2011). In the present study, we showed that TolCV1 expression time-dependently increased (Figure 1A), which may be caused by the accumulation of intracellular RpoS. This regulation presumably enables the bacteria to be more adaptive to stressful conditions in the stationary phase. In contrast, TolCV2 showed a time-dependently decreased expression trend (Figure 1A), suggesting that the expression of TolCV1 and TolCV2 was cooperatively regulated by several factors. A recently reported study revealed that the dead cells of bacterial swarms served as an “alarm signal” for live cells to increase their antibiotic resistance by releasing AcrA protein to bind with TolC located on the outer membrane of alive cells (Bhattacharyya et al., 2020). As the number of dead cells increased over time, we speculate that the gradually increased dead bacterial cells release some substances into the cultures that stimulate the expression of TolCV1 and TolCV2; however, this merits further research.
As previously reported, the outer membrane protein TolC affects virulence expression in Vibrio cholerae, Francisella tularensis and Enterobacter cloacae (Gil et al., 2006; Minato et al., 2011; Pérez et al., 2012). V. vulnificus TolCV1 and TolCV2 proteins display 78.65% and 44.44% sequence identity with Vibrio cholerae TolC, respectively. However, in V. vulnificus, the loss of outer membrane proteins TolCV1 and TolCV2 did not exhibit significant effect on the host factor-induced expression of RtxA1 toxin (Figure 2). Although Hwang et al. (Hwang et al., 2011) stated that TolC is responsible for RtxA1 secretion in V. vulnificus, whether TolCV1 or TolCV2 performed this task remained unclear. Here, we confirmed that RtxA1 toxin was exported by TolCV1 only, not TolCV2 (Figure 2). Besides, tolCV1 and tolCV2 mutant strains exhibited no difference with the wild-type strain in adhesion, and tolCV1 mutation resulted in mild decreased motility of V. vulnificus in LB with 0.3% agar (data not shown).
V. vulnificus infections affect many people due to the intake of contaminated seafood (Chung et al., 2006). Large amounts of bile salt are distributed in the human intestinal environment, and the gastrointestinal tract is one of the most important ways for V. vulnificus to enter the human body. Existing evidence that TolC is indispensable for bile salt resistance and colonization in Vibrio cholerae (Bina and Mekalanos, 2001) encouraged us to explore the effect of TolCV1 and TolCV2 on bile salt resistance in V. vulnificus. The results indicated that TolCV1 is also responsible for maintaining V. vulnificus survival in the presence of bile salt (Figures 4B, C). Furthermore, a previous study revealed that the deletion of rpoS resulted in V. vulnificus delayed adaptation to bile salt (Chen et al., 2010), which can be explained by the downregulation of TolCV1 expression. Moreover, the mice infected with tolCV1 mutant showed a longer survival time and higher survival rate than mice infected with rtxA1 mutant (Figure 5), suggesting that TolCV1 is required for V. vulnificus pathogenesis and survival under in vivo conditions. Despite the fact that both TolCV1 and TolCV2 exhibit sequence identity in some degree to E. coli TolC, TolCV2 did not exhibit any comparative capacities to TolCV1. There exists an evidence that TolC is involved in the vulnibactin export of V. vulnificus (Kawano et al., 2014). Therefore, it is conceivable that TolCV1 and TolCV2 can also secrete other proteins or metabolites that are vital for V. vulnificus pathogenesis or survival. Further studies are required to comprehensively elucidate the functions of TolCV1 and TolCV2.
In the present study, we demonstrated that TolCV1 significantly influences RtxA1 secretion, bile salt resistance, and mice lethality of V. vulnificus. The results obtained in these experiments are encouraging, since our findings determined TolCV1 as an attractive target for developing drugs to treat V. vulnificus infections, which might someday lead to clinical applications.
Publicly available datasets were analyzed in this study. This data can be found here: https://www.ncbi.nlm.nih.gov/nuccore/NC_014966.1.
The animal study was reviewed and approved by Chonnam National University Animal Care and Use Committee (IACUC-YB-2020-81).
YG and RG performed the experiment and analyzed the data. YG wrote the manuscript. JR contributed to the conceptual design. YK conceived and designed the study, wrote and reviewed the manuscript. All authors contributed to the article and approved the submitted version.
This work was supported by a National Research Foundation of Korean (NRF) grant funded by the Korean government (Nos. 2018R1D1A3B07045194 and 2019R1A2C1005884).
The authors declare that the research was conducted in the absence of any commercial or financial relationships that could be construed as a potential conflict of interest.
We would like to thank Editage (www.editage.co.kr) for English language editing.
Baker-Austin C., Oliver J. D., Alam M., Ali A., Waldor M. K., Qadri F., et al. (2018). Vibrio Spp. Infections. Nat. Rev. Dis. Primers. 4, 1–19. doi: 10.1038/s41572-018-0005-8
Battesti A., Majdalani N., Gottesman S. (2011). The Rpos-Mediated General Stress Response in Escherichia Coli. Annu. Rev. Microbiol. 65, 189–213. doi: 10.1146/annurev-micro-090110-102946
Bhattacharyya S., Walker D. M., Harshey R. M. (2020). Dead Cells Release a ‘Necrosignal’that Activates Antibiotic Survival Pathways in Bacterial Swarms. Nat. Commun. 11, 1–12. doi: 10.1038/s41467-020-17709-0
Bina J. E., Mekalanos J. J. (2001). Vibrio Cholerae Tolc is Required for Bile Resistance and Colonization. Infect. Immun. 69, 4681–4685. doi: 10.1128/iai.69.7.4681-4685.2001
Boardman B. K., Satchell K. J. F. (2004). Vibrio Cholerae Strains With Mutations in an Atypical Type I Secretion System Accumulate RTX Toxin Intracellularly. J. bacteriology. 186, 8137–8143. doi: 10.1128/JB.186.23.8137-8143.2004
Chen W.-L., Oliver J. D., Wong H.-C. (2010). Adaptation of Vibrio Vulnificus and an Rpos Mutant to Bile Salts. Int. J. Food Microbiol. 140, 232–238. doi: 10.1016/j.ijfoodmicro.2010.03.027
Chung P., Chuang S., Tsang T., Wai-Man L., Yung R., Lo J., et al. (2006). Cutaneous Injury and Vibrio Vulnificus Infection. Emerg. Infect. Dis. 12, 1302. doi: 10.3201/eid1208.051495
Di Pinto A., Terio V., Novello L., Tantillo G. (2011). ). Comparison Between Thiosulphate-Citrate-Bile Salt Sucrose (TCBS) Agar and Chromagar Vibrio for Isolating Vibrio Parahaemolyticus. Food Control. 22, 124–127. doi: 10.1016/j.foodcont.2010.06.013
Ditta G., Stanfield S., Corbin D., Helinski D. R. (1980). Broad Host Range DNA Cloning System for Gram-Negative Bacteria: Construction of a Gene Bank of Rhizobium Meliloti. Proc. Natl. Acad. Sci. U. S. A. 77, 7347–7351. doi: 10.1073/pnas.77.12.7347
Du D., Van Veen H. W., Luisi B. F. (2015). Assembly and Operation of Bacterial Tripartite Multidrug Efflux Pumps. Trends Microbiol. 23, 311–319. doi: 10.1016/j.tim.2015.01.010
Du D., Wang Z., James N. R., Voss J. E., Klimont E., Ohene-Agyei T., et al. (2014). Structure of the Acrab–Tolc Multidrug Efflux Pump. Nature. 509, 512–515. doi: 10.1038/nature13205
El Meouche I., Dunlop M. J. (2018). Heterogeneity in Efflux Pump Expression Predisposes Antibiotic-Resistant Cells to Mutation. Science. 362, 686–690. doi: 10.1126/science.aar7981
Gil H., Platz G. J., Forestal C. A., Monfett M., Bakshi C. S., Sellati T. J., et al. (2006). Deletion of Tolc Orthologs in Francisella Tularensis Identifies Roles in Multidrug Resistance and Virulence. Proc. Natl. Acad. Sci. U. S. A. 103, 12897–12902. doi: 10.1073/pnas.0602582103
Guo R. H., Gong Y., Kim S. Y., Rhee J. H., Kim Y. R. (2020). DIDS Inhibits Vibrio Vulnificus Cytotoxicity by Interfering With Tolc-Mediated Rtxa1 Toxin Secretion. Eur. J. Pharmacol. 884, 173407. doi: 10.1016/j.ejphar.2020.173407
Guo R. H., Lim J. Y., Tra My D. N., Jo S. J., Park J. U., Rhee J. H., et al. (2018). Vibrio Vulnificus Rtxa1 Toxin Expression Upon Contact With Host Cells is Rpos-Dependent. Front. Cell Infect. Microbiol. 8, 70. doi: 10.3389/fcimb.2018.00070
Hwang W., Lee N. Y., Kim J., Lee M.-A., Kim K.-S., Lee K.-H., et al. (2011). Functional Characterization of Epsc, a Component of the Type II Secretion System, in the Pathogenicity of Vibrio Vulnificus. Infect. Immun. 79, 4068–4080. doi: 10.1128/IAI.05351-11
Kanonenberg K., Smits S. H., Schmitt L. (2019). Functional Reconstitution of Hlyb, a Type I Secretion ABC Transporter, in Saposin-a Nanoparticles. Sci. Rep. 9, 1–12. doi: 10.1038/s41598-019-44812-0
Kawano H., Miyamoto K., Yasunobe M., Murata M., Myojin T., Tsuchiya T., et al. (2014). The RND Protein is Involved in the Vulnibactin Export System in Vibrio Vulnificus M2799. Microb. Pathog. 75, 59–67. doi: 10.1016/j.micpath.2014.09.001
Kim Y. R., Lee S. E., Kang I.-C., Nam K. I., Choy H. E., Rhee J. H. (2013). A Bacterial Rtx Toxin Causes Programmed Necrotic Cell Death Through Calcium-Mediated Mitochondrial Dysfunction. J. Infect. Dis. 207, 1406–1415. doi: 10.1093/infdis/jis746
Kim Y. R., Lee S. E., Kook H., Yeom J. A., Na H. S., Kim S. Y., et al. (2008). Vibrio Vulnificus RTX Toxin Kills Host Cells Only After Contact of the Bacteria With Host Cells. Cell Microbiol. 10, 848–862. doi: 10.1111/j.1462-5822.2007.01088.x
Kwak J. S., Jeong H.-G., Satchell K. J. (2011). Vibrio Vulnificus Rtxa1 Gene Recombination Generates Toxin Variants With Altered Potency During Intestinal Infection. Proc. Natl. Acad. Sci. 108, 1645–1650. doi: 10.1073/pnas.1014339108
Langevin A. M., Dunlop M. J. (2018). Stress Introduction Rate Alters the Benefit of Acrab-Tolc Efflux Pumps. J. Bacteriol. 200, 1–11. doi: 10.1128/JB.00525-17
Lee K.-E., Bang J.-S., Baek C.-H., Park D.-K., Hwang W., Choi S.-H., et al. (2007). IVET-Based Identification of Virulence Factors in Vibrio Vulnificus MO6-24/O. J. Microbiol. Biotechnol. 17, 234–243.
Lee M., Kim H.-L., Song S., Joo M., Lee S., Kim D., et al. (2013). The A-Barrel Tip Region of Escherichia Coli Tolc Homologs of Vibrio Vulnificus Interacts With the Maca Protein to Form the Functional Macrolide-Specific Efflux Pump Macab-Tolc. J. Microbiol. 51, 154–159. doi: 10.1007/s12275-013-2699-3
Lee S., Song S., Lee K. (2014a). Functional Analysis of Tolc Homologs in Vibrio Vulnificus. Curr. Microbiol. 68, 729–734. doi: 10.1007/s00284-014-0537-4
Lee S., Song S., Lee M., Hwang S., Kim J.-S., Ha N.-C., et al. (2014b). Interaction Between the A-Barrel Tip of Vibrio Vulnificus Tolc Homologs and Acra Implies the Adapter Bridging Model. J. Microbiol. 52, 148–153. doi: 10.1007/s12275-014-3578-2
Lee S., Yeom J.-H., Seo S., Lee M., Kim S., Bae J., et al. (2015). Functional Analysis of Vibrio Vulnificus RND Efflux Pumps Homologous to Vibrio Cholerae Vexab and Vexcd, and to Escherichia Coli Acrab. J. Microbiol. 53, 256–261. doi: 10.1007/s12275-015-5037-0
Miller V. L., Mekalanos J. J. (1988). A Novel Suicide Vector and Its Use in Construction of Insertion Mutations: Osmoregulation of Outer Membrane Proteins and Virulence Determinants in Vibrio Cholerae Requires Toxr. J. Bacteriol. 170, 2575–2583. doi: 10.1128/jb.170.6.2575-2583.1988
Milton D. L., O’toole R., Horstedt P., Wolf-Watz H. (1996). Flagellin a is Essential for the Virulence of Vibrio Anguillarum. J. Bacteriol. 178, 1310–1319. doi: 10.1128/jb.178.5.1310-1319.1996
Minato Y., Siefken R. L., Häse C. C. (2011). Tolc Affects Virulence Gene Expression in Vibrio Cholerae. J. Bacteriol. 193, 5850–5852. doi: 10.1128/jb.05222-11
Nolivos S., Cayron J., Dedieu A., Page A., Delolme F., Lesterlin C. (2019). Role of Acrab-Tolc Multidrug Efflux Pump in Drug-Resistance Acquisition by Plasmid Transfer. Science. 364, 778–782. doi: 10.1126/science.aav6390
Park J. H., Cho Y.-J., Chun J., Seok Y.-J., Lee J. K., Kim K.-S., et al. (2011). Complete Genome Sequence of Vibrio Vulnificus MO6-24/O. J. Bacteriol 193, 2062–2063. doi: 10.1128/JB.00110-11
Park J., Lee C. (2018). Vibrio Vulnificus Infection. N Engl. J. Med. 379, 375. doi: 10.1056/NEJMicm1716464
Pérez A., Poza M., Fernández A., Del Carmen Fernández M., Mallo S., Merino M., et al. (2012). Involvement of the Acrab-Tolc Efflux Pump in the Resistance, Fitness, and Virulence of Enterobacter Cloacae. Antimicrob. Agents Chemother. 56, 2084–2090. doi: 10.1128/aac.05509-11
Puértolas-Balint F., Warsi O., Linkevicius M., Tang P.-C., Andersson D. I. (2020). Mutations That Increase Expression of the Emrab-Tolc Efflux Pump Confer Increased Resistance to Nitroxoline in Escherichia Coli. J. Antimicrob. Chemother. 75, 300–308. doi: 10.1093/jac/dkz434
Reddy G., Hayat U., Abeygunawardana C., Fox C., Wright A., Maneval D., et al. (1992). Purification and Determination of the Structure of Capsular Polysaccharide of Vibrio Vulnificus M06-24. J. Bacteriol. 174, 2620–2630. doi: 10.1128/jb.174.8.2620-2630.1992
Roig F. J., González-Candelas F., Amaro C. (2011). Domain Organization and Evolution of Multifunctional Autoprocessing Repeats-in-Toxin (MARTX) Toxin in Vibrio Vulnificus. Appl. Environ. Microbiol. 77, 657–668. doi: 10.1128/AEM.01806-10
Keywords: TolC, RpoS, bile salt resistance, RtxA1 secretion, Vibrio vulnificus
Citation: Gong Y, Guo RH, Rhee JH and Kim YR (2021) TolCV1 Has Multifaceted Roles During Vibrio vulnificus Infection. Front. Cell. Infect. Microbiol. 11:673222. doi: 10.3389/fcimb.2021.673222
Received: 27 February 2021; Accepted: 12 April 2021;
Published: 30 April 2021.
Edited by:
Lixing Huang, Jimei University, ChinaReviewed by:
Nam-chul Ha, Seoul National University, South KoreaCopyright © 2021 Gong, Guo, Rhee and Kim. This is an open-access article distributed under the terms of the Creative Commons Attribution License (CC BY). The use, distribution or reproduction in other forums is permitted, provided the original author(s) and the copyright owner(s) are credited and that the original publication in this journal is cited, in accordance with accepted academic practice. No use, distribution or reproduction is permitted which does not comply with these terms.
*Correspondence: Young Ran Kim, a2lteXJAam51LmFjLmty
Disclaimer: All claims expressed in this article are solely those of the authors and do not necessarily represent those of their affiliated organizations, or those of the publisher, the editors and the reviewers. Any product that may be evaluated in this article or claim that may be made by its manufacturer is not guaranteed or endorsed by the publisher.
Research integrity at Frontiers
Learn more about the work of our research integrity team to safeguard the quality of each article we publish.