- 1USDA-ARS Knipling-Bushland U.S. Livestock Insects Research Laboratory, Kerrville, TX, United States
- 2Veterinary Pest Genomics Center, Kerrville, TX, United States
This study was conducted to enhance the identification of novel targets to develop acaricides that can be used to advance integrated tick-borne disease management. Drivers for the emergence and re-emergence of tick-borne diseases affecting humans, livestock, and other domestic animals in many parts of the world include the increased abundance and expanded geographic distribution of tick species that vector pathogens. The evolution of resistance to acaricides among some of the most important tick vector species highlights the vulnerability of relying on chemical treatments for tick control to mitigate the health burden of tick-borne diseases. The involvement of inward rectifier potassium (Kir) channels in homeostasis, diuresis, and salivary gland secretion in ticks and other pests identified them as attractive targets to develop novel acaricides. However, few studies exist on the molecular characteristics of Kir channels in ticks. This bioinformatic analysis described Kir channels in 20 species of hard and soft ticks. Summarizing relevant investigations on Kir channel function in invertebrate pests allowed the phylogenomic study of this class of ion channels in ticks. How this information can be adapted to innovate tick control technologies is discussed.
Introduction
Ticks (Acari) are obligate blood feeding parasites and vectors of a diverse array of pathogens including bacteria, protozoa, and viruses that cause diseases among humans, livestock, and other domestic animals (Sonenshine and Roe, 2013). The health burden of tick-borne disease (TBD) increased globally (Paules et al., 2018; Madison-Antenucci et al., 2020). In the United States alone, the number of human TBD cases more than doubled increasing from 22,527 to 48,610 between 2004 and 2016 (Rosenberg et al., 2018). Several species of hard and soft ticks are vectors of the diverse pathogens causing tick-borne diseases (Brites-Neto et al., 2015). Recent studies have implicated ticks in Alpha-Gal Syndrome, a potentially life-threatening allergy to red meat that is induced by the sugar alpha-gal from a feeding tick (Crispell et al., 2019). The application of technological advances is helping explain the global diversity of ticks and their ability to transmit pathogens (Dantas-Torres, 2018; Yang and Han, 2018).
Drivers for the emergence and re-emergence of tick-borne diseases affecting humans, livestock, and other domestic animals in many parts of the world include the increased abundance and expanded geographic distribution of tick species that vector pathogens. Dermacentor variabilis, the principal vector of Rickettsia rickettsii causing Rocky Mountain spotted fever in North America, expanded its range northward into Canada surviving the milder winters associated with variability in climatic patterns (Sonenshine, 2018). Due to its expanding geographic distribution and vector biology, Ixodes scapularis has become one of the most important disease vectors in North America where it transmits Borrelia burgdorferi, Babesia microti, and Powassan virus, causing Lyme disease, human babesiosis, and Powassan virus disease, respectively (Eisen and Eisen, 2018).
In addition, Amblyomma americanum and Amblyomma maculatum have expanded their range northward, extending the geographic range of additional tick species that threaten livestock (Sonenshine, 2018). Detection of the Asian longhorned tick, Haemaphysalis longicornis, in the United States (U.S.) in 2017 highlights the involvement of invasive tick species in the emergence of tick-borne diseases (Rainey et al., 2018). Ha. longicornis is a known vector of pathogens that affect humans, livestock, and other domestic animals (Beard et al., 2018). Control of tick populations, treatment of host infestations, and reduction of exposure to tick bites are critical for tick-borne disease management (Pérez De León et al., 2014).
The evolution of resistance to acaricides among some of the most important tick vector species highlights the vulnerability of relying on chemical treatments for tick control to mitigate the health burden of tick-borne diseases. In the U.S. for example, bovine babesiosis, or cattle tick fever, was eradicated by eliminating the one-host fever tick vectors, Rhipicephalus microplus and R. annulatus, through efforts of the Cattle Fever Tick Eradication Program including the systematic treatment of cattle with acaricides (Guerrero et al., 2014). However, the spread of resistance to acaricides approved for use by the Cattle Fever Tick Eradication Program among fever ticks causing outbreaks raises the specter for the emergence of bovine babesiosis in the U.S. (Thomas et al., 2020). Resistance to commonly used commercial acaricides in the three-host ticks R. sanguineus and Amblyomma mixtum, which are known vectors of human tick-borne diseases, stresses the need to innovate tick control technologies (Rodriguez-Vivas et al., 2017; Higa et al., 2020).
Discovering unique molecular targets facilitates the development of safer acaricides with new modes of action (Meng and Sluder, 2018). Previous research identified the inward rectifier potassium (Kir) channels as attractive targets to develop novel acaricides because of their involvement in homeostasis, diuresis, and salivary gland secretion in ticks and other pests (Li et al., 2019; Li et al., 2020). However, few studies exist on the molecular characteristics of Kir channels in ticks. Here, a bioinformatic analysis described Kir channels in 20 species of hard and soft ticks. Summarizing relevant investigations on Kir channel function in invertebrate pests allowed the phylogenomic study of this class of ion channels in ticks. The adaptation of this information to innovate tick control technologies is discussed.
Kir Channel Structure and Genome Repertoires
Inward rectifier potassium (Kir) channels were named due to their ability to facilitate the inward movement of K+ ions at hyperpolarizing membrane voltages more readily than the outward movement of K+ ions at depolarizing membrane voltages, a function which has been compared to an electrical diode wherein current flows in only one direction (Nishida and Mackinnon, 2002; Tao et al., 2009). The S4 voltage sensor region found in voltage-gated K+, Na+, and Ca2+ channels is absent in Kir channels; therefore, Kir channels are refractory to changes in membrane potential associated with the inward movement of other cations (Nishida and Mackinnon, 2002; Hibino et al., 2010). The outward movement of K+ is inhibited by intracellular cations and polyamines that enter the pore from the cytoplasmic side but cannot pass through, thus blocking the channel and preventing the outward flow of K+ (Nishida and Mackinnon, 2002; Hibino et al., 2010). These properties allow Kir channels to maintain resting membrane potential and regulate action potential duration (Hibino et al., 2010).
Kir channels are formed by the assembly of four identical or similar protein subunits, each with two transmembrane domains flanking a re-entrant loop region forming the channel pore, and N- and C-terminal cytoplasmic regions (Nichols and Lopatin, 1997; Hibino et al., 2010). The subunits are organized such that one transmembrane α-helix (TM1) is on the outside of the channel while the other (TM2) is on the inside near the pore (Hibino et al., 2010). The pore-forming region includes a selectivity filter that confers passage of K+ but excludes Na+ (Doyle et al., 1998). In Eukaryotes, the selectivity filter is characterized by a seven-residue motif TXGYGFR, where X is an aliphatic amino acid, and F is sometimes replaced by a different residue (Tao et al., 2009).
Sixteen genes encoding Kir channel subunits have been identified in mammals. They belong to seven subfamilies (Kir1 to Kir7) comprising four functional groups: classical, G protein-gated, ATP-sensitive, and K+-transport channels (Hibino et al., 2010; Walsh, 2020). In mammals, Kir channels are expressed by diverse tissues comprising the nervous, muscle, cardiovascular, and urinary systems (Walsh, 2020). Consequently, abnormal function of Kir channels has been implicated in human diseases affecting these organ systems (Zangerl-Plessl et al., 2019). Mutations that cause trafficking defects associated with Kir channel dysfunction have been identified and primarily occur in two “hotspots”: in and around the TM1 domain and in a segment of the C-terminal cytoplasmic region (Zangerl-Plessl et al., 2019).
Compared to mammals, insects possess a smaller repertoire of Kir channels, ranging in number from three to six genes in the species studied thus far (Lai et al., 2020). Based on phylogenetic analysis, there are at least three different subtypes of insect Kir channels. Drosophila melanogaster (Luan and Li, 2012) and Nilaparvata lugens (Ren et al., 2018) each have three (Kir1-Kir3), while Aedes aegypti has four due to a duplication in Kir2 (Kir2A and Kir2B) (Piermarini et al., 2013). However, the aphids Acyrthosiphon pisum and Aphis glycines have only two (Kir1 and Kir2), suggesting a loss of Kir3 during aphid evolution (Piermarini et al., 2018). A recent study of Kir channels revealed a possible fourth subtype in Lepidoptera (Kir4), which was not found in the Diptera, Heteroptera, and Homoptera included in the study (Lai et al., 2020).
Bioinformatics of Kir Channels in Ticks
Screening of the NCBI databases produced a single Kir channel subunit gene in 20 tick species ranging from 474 to 492 amino acids in length (Table 1). All are apparently full-length, having start and stop codons, except for Ornithodoros erraticus and O. moubata, which did not have stop codons. Their length, however, was similar to the Kir channels in O. rostratus and O. turicata and likely represent full-length or near full-length proteins. Kir channels in R. annulatus and R. microplus were manually annotated from their respective genome assemblies and have coding sequences with six exons spanning 7,014 and 9,318 nucleotides, respectively. Despite improvements in sequencing technologies that have led to the generation of several high-quality tick genome assemblies in recent years (Miller et al., 2018; Jia et al., 2020), the lack of genome assemblies prevented the screening of all 20 tick species for additional Kir channel genes.
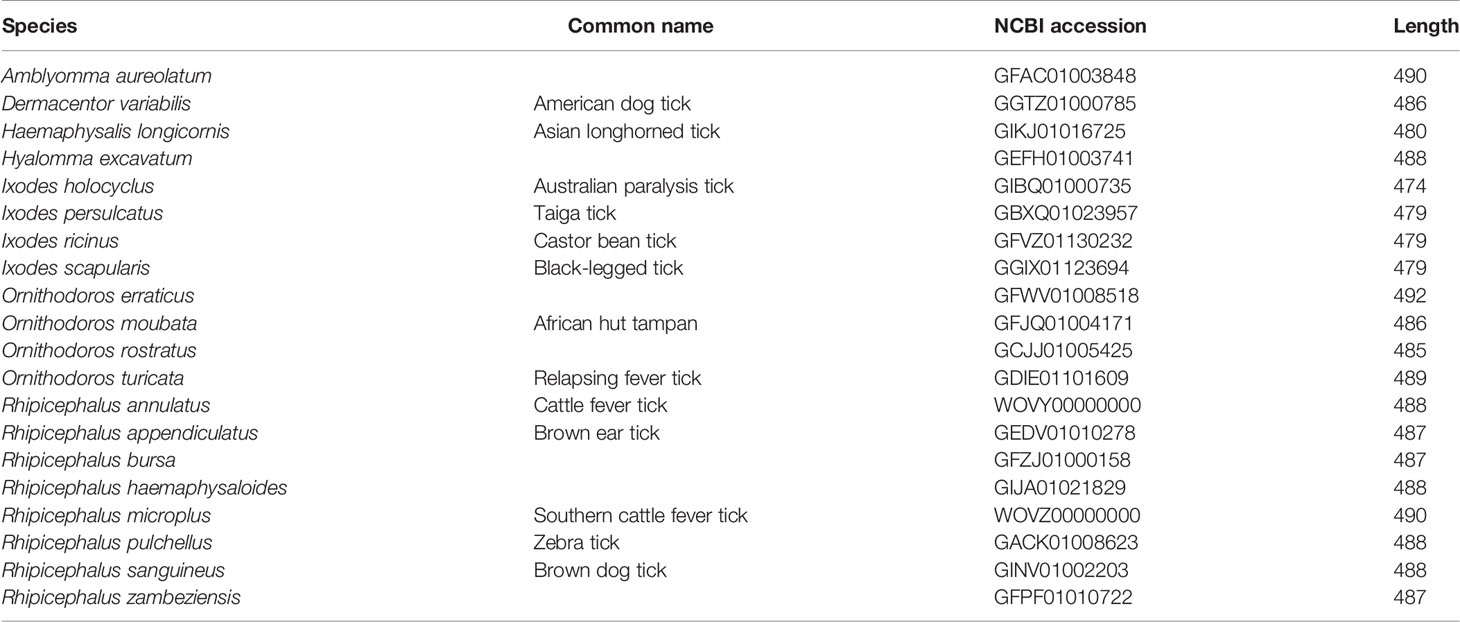
Table 1 Summary of tick Kir channel proteins identified in the NCBI databases. All were from transcriptome shotgun assemblies (TSA database) except Rh. annulatus and Rh. Microplus, which were acquired from their respective genome assemblies.
Based on phylogenetic analysis of the Kir channel subunits in a soft tick (O. turicata), two hard ticks (I. scapularis and R. microplus), two Diptera (D. melanogaster and Ae. aegypti) and two Lepidoptera (Manduca sexta and Danaus plexippus), the tick Kir channels belong to the Kir1 clade in insects (Figure 1A). Amino acid percent identity of tick Kirs to D. melanogaster Kir1 ranged from 50 to 51%. The relationships among all 20 tick Kir channel subunits are consistent with recent systematic analysis of ticks based on 18S and 28S rRNA genes and whole mitochondrial genomes (Mans et al., 2019). The soft ticks (Argasidae) and hard ticks (Ixodidae) are separated into two distinct clades, with Ornithodoros spp. representing the soft ticks and the remaining being hard ticks (Figure 1B). The soft and hard ticks differ in their feeding strategies, wherein the soft ticks are fast-feeding, and the hard ticks are long-feeding, and adaptations associated with these different feeding strategies have been described (Mans and Neitz, 2004). The divergence observed between the hard and soft tick Kir channels could reflect the physiological demands of the different feeding strategies. The Ixodidae Kir subunits formed two clades representing the Prostriata (Ixodes) and the Metastriata (all other Ixodidae), which can be separated based on morphological characteristics of the anal grooves.
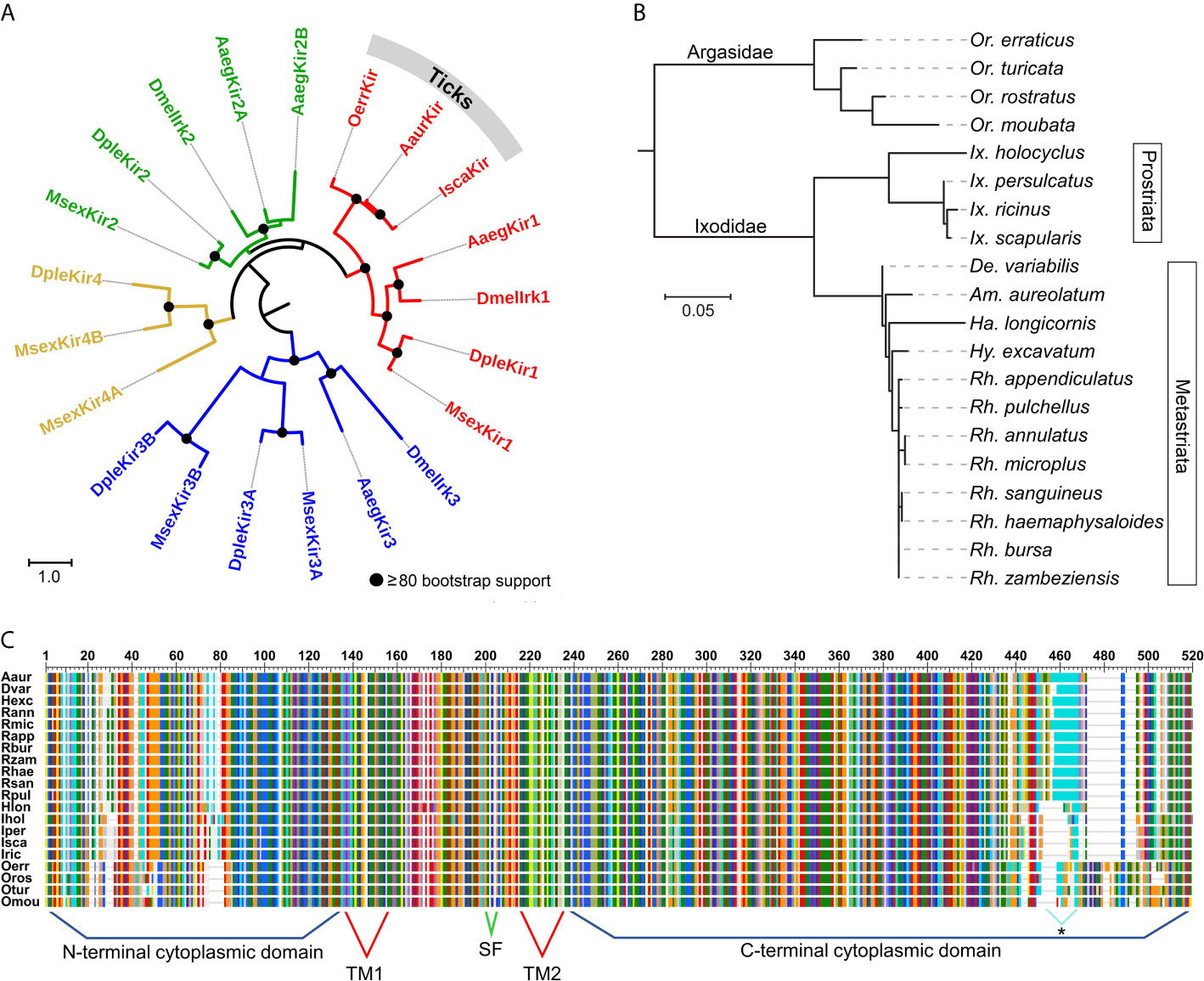
Figure 1 Phylogenetic relationships and protein features of tick and insect Kir channels. (A) Estimated phylogenetic relationships of three tick Kir channels with those in D. melanogaster (Dmel), Aedes aegypti (Aaeg), Manduca sexta (Msex) and Danaus plexippus (Dple). The multiple alignment was conducted using the L-INS-I method in MAFFT v7.475 (Katoh et al., 2019). The phylogenies (A,B) were estimated using the maximum likelihood method and LG substitution model in RaxML v8.2.11 (Stamatakis, 2014). Bootstrap support was estimated using 500 replications. The trees are rooted at the midpoint. (B) Estimated phylogenetic relationships among 20 tick Kir channel proteins. (C) Multiple sequence alignment of 20 tick Kir channels illustrating the predicted transmembrane (TM) and cytoplasmic domains, and the K+ selectivity filter (SF). The C-terminus of the Metastriata ticks, except Ha. longicornis (Hlon) contains long stretches of glutamine repeats. Multiple alignment conducted using MAFFT v7.475 (Katoh et al., 2019).
A multiple sequence alignment shows the vast majority of variation to be in the N- and C-terminal domains of the protein, which flank the more highly conserved transmembrane region (Figure 1C). All of the tick Kir channels examined in the present study have the K+ selectivity filter motif of TIGYGSR, except for O. moubata which has the motif TIGYGFR (Figure S1). The C-terminal domain of the Prostriata ticks, with the exception of Ha. longicornis, is characterized by microsatellite repeats (CAG and CAA) coding for runs of glutamine (Q), ranging from 10 to 13 residues in length (Figures 1C and S1). The role of this highly variable region of the N-terminal domain has yet to be determined.
Kir Channels as Targets for Novel Control Measures
Hematophagous arthropods overcome extreme physiological challenges during and after blood feeding, most notably, post-prandial diuresis (Beyenbach, 2003; Benoit and Denlinger, 2010); therefore, inhibition of renal function could provide a means to control blood feeding arthropods and the pathogens they transmit (Piermarini et al., 2017). A series of experiments aimed at understanding insect renal physiology led to the discovery of Kir channels as key components of diuresis in insect Malpighian tubules. Among these studies were gene expression analyses showing relatively high expression of Kir channels in Malpighian tubules and functional characterization of Kir1 and Kir2 subtypes using Drosophila S2 and Xenopus oocyte heterologous expression systems (Döring et al., 2002; Piermarini et al., 2013).
The identification of small molecule inhibitors has proven to be invaluable for in vivo and in vitro analyses of Kir channels and could lead to the development of novel insecticides. The small molecule inhibitors of mammalian Kir channels, VU590 and VU573, (Raphemot et al., 2011; Denton et al., 2013) were found to modulate the activity of Ae. aegypti Kir1 (VU590 and VU573) and Kir2B (VU573) and cause renal failure and/or mortality in Ae. aegypti (Piermarini et al., 2013; Raphemot et al., 2014). One of the major concerns during the development of insecticides is the effect on non-target species such as humans and beneficial insects. A small molecule inhibitor of mosquito Kir1 (VU041) was identified by high-throughput screening that does not inhibit most mammalian Kir channels (exception Kir2.1) and is not lethal to honey bees when applied topically at the concentrations tested (Swale et al., 2016). Further, VU041 was effective in reducing fecundity in insecticide resistant and wild-type strains of Ae. aegypti and An. gambiae (Swale et al., 2016).
In addition to Malpighian tubules, there is growing evidence that Kir channels perform vital functions in the salivary glands, which are essential for osmoregulation, blood feeding, and pathogen transmission in hematophagous arthropods, making them attractive targets for disruption (Ribeiro, 1987; Sauer et al., 1995; Sauer et al., 2000; Swale et al., 2017; Nuttall, 2019). Bioactive factors produced in the salivary glands enable the acquisition of a blood meal at the host interface and facilitate the transmission of tick-borne pathogens to vertebrate hosts (Kazimírová and Stibraniova, 2013).
A recent study on the role of Kir channels in tick salivary gland function provided evidence that pharmacological inhibition of these ion channels reduces the secretory activity of salivary glands in the lone star tick, Amblyomma americanum (Li et al., 2019). The reduced secretory capacity of the salivary gland was directly correlated with a dramatic reduction of blood ingestion during feeding. This study identified small-molecule modulators of Kir channel function (VU041, VU625, and VU688) that were previously shown to be inhibitors of mosquito Kir channels (Raphemot et al., 2014; Swale et al., 2016). Similarly, small molecule inhibitors reduced salivary gland secretion (VU041, VU590, VU937, and VU063) and blood meal ingestion (VU041 and VU063) in the horn fly, Haematobia irritans (Li et al., 2020). Although four inhibitors (VU041, VU590, VU937, and VU063) caused mortality, VU041 was the highest at 82 ± 11% (Li et al., 2020).
A recent study suggests an insecticide targeting a Kir channel has been developed and been in use for several years. Flonicamid (N‐cyanomethyl‐4‐trifluoromethylnicotinamide) is an insecticide that is highly effective against aphids, but not against other insects, including some coleopterans, lepidopterans, and dipterans (Morita et al., 2014). It is structurally similar to neonicotinoid insecticides, which target nicotinic acetylcholine receptors (Ren et al., 2018). However, flonicamid had no activity against nicotinic acetylcholine receptors, acetylcholine esterase or sodium channels (Morita et al., 2014). The mode of action remained elusive until recently, when a study showed flonicamid had a similar effect on the Nilaparvata lugens Kir1 channel as VU590 (Ren et al., 2018), which is a potent inhibitor of Kir1 in Ae. aegypti (Rouhier et al., 2014). Flonicamid also inhibited renal excretion in Culex pipiens and, taken together, suggests its mode of action is by inhibiting the insect Kir1 subtype (Ren et al., 2018). Curiously, flonicamid showed little efficacy against Ha. longicornis after spray application at 50 ppm concentration (Park et al., 2019). This lack of activity is likely due to a failure to inhibit the Ha. longicornis Kir channel, thus demonstrating the need for identification of tick Kir channel inhibitors.
Conclusion
This review highlights the importance of Kir channels as potential targets for inhibition of renal and salivary gland function in arthropod pests and vectors of disease. Furthermore, we describe the Kir channel subunits in 20 tick species, thus providing the framework for in vitro functional analyses and screening of small molecule inhibitors. Recent efforts have only begun to shed light on the importance of Kir channels in tick physiology, especially their role in the regulation of blood feeding. Many gaps in our knowledge regarding the functional, temporal, spatial, and molecular characteristics of ticks still exist today. This review highlights the critical need for follow-up studies that can help elucidate these key aspects of tick Kir channel biology. The evidence for Kir channels as potential targets of insecticides and acaricides is mounting. These findings hold the potential to identifying targets for tick-borne pathogen intervention and control measures. Continued research elucidating the functional mechanisms of Kir channels and other membrane bound proteins, promises to bring an exciting new era in targeted pharmacological interventions. Although studies on the physiological role and molecular basis of Kir channel function in mammals and insects have provided critical groundwork, Kir channels in ticks remain understudied.
Author Contributions
PS and AL conceived this work. PS, PH, and AL wrote the manuscript. PS, PH, and KB screened the NCBI databases for Kir sequences. All authors contributed to the article and approved the submitted version.
Funding
This study was funded by the United States Department of Agriculture’s Agricultural Research Service (USDA-ARS) under project number: 3094-32000-042-00D.
Conflict of Interest
Any mention of trade names or commercial products in this publication is solely for the purpose of providing specific information and does not imply a recommendation or endorsement by the U.S. Department of Agriculture. The USDA is an equal opportunity provider and employer.
The authors declare that the research was conducted in the absence of any commercial or financial relationships that could be construed as a potential conflict of interest.
Acknowledgments
USDA is an equal opportunity employer and provider.
Supplementary Material
The Supplementary Material for this article can be found online at: https://www.frontiersin.org/articles/10.3389/fcimb.2021.647020/full#supplementary-material
References
Beard, C. B., Occi, J., Bonilla, D. L., Egizi, A. M., Fonseca, D. M., Mertins, J. W., et al. (2018). Multistate infestation with the exotic disease–vector tick Haemaphysalis longicornis—United States, August 2017–September 2018. Morbid Mortal. Wkly. Rep. 67, 1310–1313. doi: 10.15585/mmwr.mm6747a3
Benoit, J. B., Denlinger, D. L. (2010). Meeting the challenges of on-host and off-host water balance in blood-feeding arthropods. J. Insect Physiol. 56, 1366–1376. doi: 10.1016/j.jinsphys.2010.02.014
Beyenbach, K. W. (2003). Transport mechanisms of diuresis in Malpighian tubules of insects. J. Exp. Biol. 206, 3845–3856. doi: 10.1242/jeb.00639
Brites-Neto, J., Duarte, K. M. R., Martins, T. F. (2015). Tick-borne infections in human and animal population worldwide. Vet. World 8, 301. doi: 10.14202/vetworld.2015.301-315
Crispell, G., Commins, S. P., Archer-Hartman, S. A., Choudhary, S., Dharmarajan, G., Azadi, P., et al. (2019). Discovery of alpha-gal-containing antigens in North American tick species believed to induce red meat allergy. Front. Immunol. 10, 1056. doi: 10.3389/fimmu.2019.01056
Dantas-Torres, F. (2018). Species concepts: what about ticks? Trends Parasitol. 34, 1017–1026 doi: 10.1016/j.pt.2018.09.009.
Denton, J. S., Pao, A. C., Maduke, M. (2013). Novel diuretic targets. Am. J. Physiol. Renal Physiol. 305, F931–F942. doi: 10.1152/ajprenal.00230.2013
Döring, F., Wischmeyer, E., Kühnlein, R. P., Jäckle, H., Karschin, A. (2002). Inwardly Rectifying K+ (Kir) Channels in Drosophila a crucial role of cellular milieu factors for kir channel function. J. Biol. Chem. 277, 25554–25561. doi: 10.1074/jbc.M202385200
Doyle, D. A., Cabral, J. M., Pfuetzner, R. A., Kuo, A., Gulbis, J. M., Cohen, S. L., et al. (1998). The structure of the potassium channel: molecular basis of K+ conduction and selectivity. Science 280, 69–77. doi: 10.1126/science.280.5360.69
Eisen, R. J., Eisen, L. (2018). The blacklegged tick, Ixodes scapularis: an increasing public health concern. Trends Parasitol. 34, 295–309. doi: 10.1016/j.pt.2017.12.006
Guerrero, F. D., Pérez De León, A., Rodriguez-Vivas, R., Jonsson, N., Miller, R., Andreotti, R. (2014). “Acaricide research and development, resistance and resistance monitoring,” in Biology of Ticks, eds. Sonenshine, D, Roe, R. (New York: Oxford University Press)353–374.
Hibino, H., Inanobe, A., Furutani, K., Murakami, S., Findlay, I., Kurachi, Y. (2010). Inwardly rectifying potassium channels: their structure, function, and physiological roles. Physiol. Rev. 90, 291–366. doi: 10.1152/physrev.00021.2009
Higa, L. D. O. S., Piña, F. T. B., Da Silva Rodrigues, V., Garcia, M. V., Salas, D. R., Miller, R. J., et al. (2020). Evidence of acaricide resistance in different life stages of Amblyomma mixtum and Rhipicephalus microplus (Acari: Ixodidae) collected from the same farm in the state of Veracruz, Mexico. Prev. Vet Med. 174, 104837. doi: 10.1016/j.prevetmed.2019.104837
Jia, N., Wang, J., Shi, W., Du, L., Sun, Y., Zhan, W., et al. (2020). Large-scale comparative analyses of tick genomes elucidate their genetic diversity and vector capacities. Cell 182, 1328–1340. e1313. doi: 10.1016/j.cell.2020.07.023
Katoh, K., Rozewicki, J., Yamada, K. D. (2019). MAFFT online service: multiple sequence alignment, interactive sequence choice and visualization. Briefings in bioinformatics 20, 1160–1166. doi: 10.1093/bib/bbx108
Kazimírová, M., Stibraniova, I. (2013). Tick salivary compounds: their role in modulation of host defences and pathogen transmission. Front. Cell. Infect. Microbiol. 3, 43.
Lai, X., Xu, J., Ma, H., Liu, Z., Zheng, W., Liu, J., et al. (2020). Identification and Expression of Inward-Rectifying Potassium Channel Subunits in Plutella xylostella. Insects 11, 461. doi: 10.3390/insects11080461
Li, Z., Macaluso, K. R., Foil, L. D., Swale, D. R. (2019). Inward rectifier potassium (Kir) channels mediate salivary gland function and blood feeding in the lone star tick, Amblyomma americanum. PLoS Neglect. Trop. Dis. 13, e0007153. doi: 10.1371/journal.pntd.0007153
Li, Z., Guerrero, F., Pérez De León, A. A., Foil, L. D., Swale, D. R. (2020). Small-Molecule Inhibitors of Inward Rectifier Potassium (Kir) Channels Reduce Bloodmeal Feeding and Have Insecticidal Activity Against the Horn Fly (Diptera: Muscidae). J. Med. Entomol. 57, 1131–1140 doi: 10.1093/jme/tjaa015
Luan, Z., Li, H.-S. (2012). Inwardly rectifying potassium channels in Drosophila. Acta Physiologica Sinica 64, 515–519.
Madison-Antenucci, S., Kramer, L. D., Gebhardt, L. L., Kauffman, E. (2020). Emerging Tick-Borne Diseases. Clin. Microbiol. Rev. 33, e00083. doi: 10.1128/CMR.00083-18
Mans, B. J., Featherston, J., Kvas, M., Pillay, K.-A., De Klerk, D. G., Pienaar, R., et al. (2019). Argasid and ixodid systematics: implications for soft tick evolution and systematics, with a new argasid species list. Ticks tick-borne Dis. 10, 219–240. doi: 10.1016/j.ttbdis.2018.09.010
Mans, B. J., Neitz, A. W. (2004). Adaptation of ticks to a blood-feeding environment: evolution from a functional perspective. Insect Biochem. Mol. Biol. 34, 1–17. doi: 10.1016/j.ibmb.2003.09.002
Meng, C. Q., Sluder, A. E. (2018). Ectoparasites: drug discovery against moving targets (Weinheim, Germany: Wiley Online Library).
Miller, J. R., Koren, S., Dilley, K. A., Harkins, D. M., Stockwell, T. B., Shabman, R. S., et al. (2018). A draft genome sequence for the Ixodes scapularis cell line, ISE6. F1000Research 7. doi: 10.12688/f1000research.13635.1
Morita, M., Yoneda, T., Akiyoshi, N. (2014). Research and development of a novel insecticide, flonicamid. J. Pesticide Sci. 39, 179–180. doi: 10.1584/jpestics.J14-05
Nichols, C., Lopatin, A. (1997). Inward rectifier potassium channels. Annu. Rev. Physiol. 59, 171–191. doi: 10.1146/annurev.physiol.59.1.171
Nishida, M., Mackinnon, R. (2002). Structural basis of inward rectification: cytoplasmic pore of the G protein-gated inward rectifier GIRK1 at 1.8 Å resolution. Cell 111, 957–965. doi: 10.1016/S0092-8674(02)01227-8
Nuttall, P. A. (2019). Tick saliva and its role in pathogen transmission. Wiener klinische Wochenschrift, 1–12. doi: 10.1007/s00508-019-1500-y
Park, G. H., Kim, H. K., Lee, W. G., Cho, S. H., Kim, G. H. (2019). Evaluation of the acaricidal activity of 63 commercialized pesticides against Haemaphysalis longicornis (Acari: Ixodidae). Entomological Res. 49, 330–336. doi: 10.1111/1748-5967.12367
Paules, C. I., Marston, H. D., Bloom, M. E., Fauci, A. S. (2018). Tickborne diseases—confronting a growing threat. N. Engl. J. Med. 379, 701–703. doi: 10.1056/NEJMp1807870
Pérez De León, A. A., Teel, P. D., Li, A., Ponnusamy, L., Roe, R. M. (2014). Advancing integrated tick management to mitigate burden of tick-borne diseases. Outlooks Pest Manage. 25, 382–389. doi: 10.1564/v25_dec_10
Piermarini, P. M., Rouhier, M. F., Schepel, M., Kosse, C., Beyenbach, K. W. (2013). Cloning and functional characterization of inward-rectifying potassium (Kir) channels from Malpighian tubules of the mosquito Aedes aegypti. Insect Biochem. Mol. Biol. 43, 75–90. doi: 10.1016/j.ibmb.2012.09.009
Piermarini, P. M., Esquivel, C. J., Denton, J. S. (2017). Malpighian tubules as novel targets for mosquito control. Int. J. Environ. Res. Public Health 14, 111. doi: 10.3390/ijerph14020111
Piermarini, P. M., Inocente, E. A., Acosta, N., Hopkins, C. R., Denton, J. S., Michel, A. P. (2018). Inward rectifier potassium (Kir) channels in the soybean aphid Aphis glycines: Functional characterization, pharmacology, and toxicology. J. Insect Physiol. 110, 57–65. doi: 10.1016/j.jinsphys.2018.09.001
Rainey, T., Occi, J. L., Robbins, R. G., Egizi, A. (2018). Discovery of Haemaphysalis longicornis (Ixodida: Ixodidae) parasitizing a sheep in New Jersey, United States. J. Med. Entomol. 55, 757–759. doi: 10.1093/jme/tjy006
Raphemot, R., Lonergan, D. F., Nguyen, T. T., Utley, T., Lewis, L. M., Kadakia, R., et al. (2011). Discovery, Characterization, and Structure–Activity Relationships of an Inhibitor of Inward Rectifier Potassium (Kir) Channels with Preference for Kir2. 3, Kir3. X, and Kir7. 1. Front. Pharmacol. 2, 75.
Raphemot, R., Rouhier, M. F., Swale, D. R., Days, E., Weaver, C. D., Lovell, K. M., et al. (2014). Discovery and Characterization of a Potent and Selective Inhibitor of Aedes aegypti Inward Rectifier Potassium Channels. PLoS One 9, e110772. doi: 10.1371/journal.pone.0110772
Ren, M., Niu, J., Hu, B., Wei, Q., Zheng, C., Tian, X., et al. (2018). Block of Kir channels by flonicamid disrupts salivary and renal excretion of insect pests. Insect Biochem. Mol. Biol. 99, 17–26. doi: 10.1016/j.ibmb.2018.05.007
Ribeiro, J. (1987). Role of saliva in blood-feeding by arthropods. Annu. Rev. Entomol. 32, 463–478. doi: 10.1146/annurev.en.32.010187.002335
Rodriguez-Vivas, R., Ojeda-Chi, M., Trinidad-Martinez, I., De León, A. P. (2017). First documentation of ivermectin resistance in Rhipicephalus sanguineus sensu lato (Acari: Ixodidae). Vet. Parasitol. 233, 9–13. doi: 10.1016/j.vetpar.2016.11.015
Rosenberg, R., Lindsey, N. P., Fischer, M., Gregory, C. J., Hinckley, A. F., Mead, P. S., et al. (2018). Vital signs: trends in reported vectorborne disease cases—United States and Territories 2004–2016. Morbid, Mortal. Weekly Rep. 67, 496. doi: 10.15585/mmwr.mm6717e1
Rouhier, M. F., Raphemot, R., Denton, J. S., Piermarini, P. M. (2014). Pharmacological validation of an inward-rectifier potassium (Kir) channel as an insecticide target in the yellow fever mosquito Aedes aegypti. PLoS One 9, e100700. doi: 10.1371/journal.pone.0100700
Sauer, J., Mcswain, J., Bowman, A., Essenberg, R. (1995). Tick salivary gland physiology. Annu. Rev. Entomol. 40, 245–267. doi: 10.1146/annurev.en.40.010195.001333
Sauer, J., Essenberg, R., Bowman, A. (2000). Salivary glands in ixodid ticks: control and mechanism of secretion. J. Insect Physiol. 46, 1069–1078. doi: 10.1016/S0022-1910(99)00210-3
Sonenshine, D. E. (2018). Range expansion of tick disease vectors in North America: implications for spread of tick-borne disease. Int. J. Environ. Res. Public Health 15, 478. doi: 10.3390/ijerph15030478
Sonenshine, D. E., Roe, R. M. (2013). Ticks, people, and animals. Biology of Ticks (New York: Oxford University Press), 3–16.
Stamatakis, A. (2014). RAxML version 8: a tool for phylogenetic analysis and post-analysis of large phylogenies. Bioinformatics 30, 1312–1313. doi: 10.1093/bioinformatics/btu033
Swale, D. R., Engers, D. W., Bollinger, S. R., Gross, A., Inocente, E. A., Days, E., et al. (2016). An insecticide resistance-breaking mosquitocide targeting inward rectifier potassium channels in vectors of Zika virus and malaria. Sci. Rep. 6, 36954–36954. doi: 10.1038/srep36954
Swale, D. R., Li, Z., Guerrero, F., De León, A., Foil, L. D. (2017). Role of inward rectifier potassium channels in salivary gland function and sugar feeding of the fruit fly, Drosophila melanogaster. Pesticide Biochem. Physiol. 141, 41–49. doi: 10.1016/j.pestbp.2016.11.005
Tao, X., Avalos, J. L., Chen, J., Mackinnon, R. (2009). Crystal structure of the eukaryotic strong inward-rectifier K+ channel Kir2. 2 at 3.1 Å resolution. Science 326, 1668–1674.
Thomas, D. B., Klafke, G., Busch, J. D., Olafson, P. U., Miller, R. A., Mosqueda, J., et al. (2020). Tracking the increase of acaricide resistance in an invasive population of Cattle Fever Ticks (Acari: Ixodidae) and implementation of real-time PCR assays to rapidly genotype resistance mutations. Ann. Entomological Soc. America 113, 298–309. doi: 10.1093/aesa/saz053
Walsh, K. B. (2020). Screening Technologies for Inward Rectifier Potassium Channels: Discovery of New Blockers and Activators. SLAS DISCOVERY: Advancing Sci. Drug Discov. 25, 420–433.
Yang, L. H., Han, B. A. (2018). Data-driven predictions and novel hypotheses about zoonotic tick vectors from the genus Ixodes. BMC Ecol. 18, 1–6. doi: 10.1186/s12898-018-0163-2
Keywords: phylogenomic, tick, Kir, Acari, evolution
Citation: Saelao P, Hickner PV, Bendele KG and Pérez de León AA (2021) Phylogenomics of Tick Inward Rectifier Potassium Channels and Their Potential as Targets to Innovate Control Technologies. Front. Cell. Infect. Microbiol. 11:647020. doi: 10.3389/fcimb.2021.647020
Received: 28 December 2020; Accepted: 16 February 2021;
Published: 19 March 2021.
Edited by:
Jun Feng, National Institute of Parasitic Diseases, ChinaReviewed by:
Shahid Karim, University of Southern Mississippi, United StatesCarlos Termignoni, Federal University of Rio Grande do Sul, Brazil
Copyright © 2021 Saelao, Hickner, Bendele and Pérez de León. This is an open-access article distributed under the terms of the Creative Commons Attribution License (CC BY). The use, distribution or reproduction in other forums is permitted, provided the original author(s) and the copyright owner(s) are credited and that the original publication in this journal is cited, in accordance with accepted academic practice. No use, distribution or reproduction is permitted which does not comply with these terms.
*Correspondence: Perot Saelao, UGVyb3QuU2FlbGFvQHVzZGEuZ292
†These authors have contributed equally to this work
‡Present address: Adalberto A. Pérez de León, USDA-ARS San Joaquin Valley Agricultural Sciences Center, Parlier, CA, United States