- 1Faculty of Medicine, Federal University of Ceará, Fortaleza, Brazil
- 2Department of Medicine, Discipline of Infectious Diseases, Federal University of São Paulo, São Paulo, Brazil
- 3Tropical Medicine Department, Federal University of Pernambuco, Recife, Brazil
- 4College of Veterinary, State University of Ceará, Fortaleza, Brazil
Persister cells are metabolically inactive dormant cells that lie within microbial biofilms. They are phenotypic variants highly tolerant to antimicrobials and, therefore, associated with recalcitrant infections. In the present study, we investigated if Trichosporon asahii and T. inkin are able to produce persister cells. Trichosporon spp. are ubiquitous fungi, commonly found as commensals of the human skin and gut microbiota, and have been increasingly reported as agents of fungemia in immunocompromised patients. Biofilms derived from clinical strains of T asahii (n=5) and T. inkin (n=7) were formed in flat-bottomed microtiter plates and incubated at 35°C for 48 h, treated with 100 μg/ml amphotericin B (AMB) and incubated at 35°C for additional 24 h. Biofilms were scraped from the wells and persister cells were assayed for susceptibility to AMB. Additionally, we investigated if these persister cells were able to generate new biofilms and studied their ultrastructure and AMB susceptibility. Persister cells were detected in both T asahii and T. inkin biofilms and showed tolerance to high doses of AMB (up to 256 times higher than the minimum inhibitory concentration). Persister cells were able to generate biofilms, however they presented reduced biomass and metabolic activity, and reduced tolerance to AMB, in comparison to biofilm growth control. The present study describes the occurrence of persister cells in Trichosporon spp. and suggests their role in the reduced AMB susceptibility of T. asahii and T. inkin biofilms.
Introduction
Trichosporon spp. are ubiquitous fungi, commonly found as commensals of the human skin and gastrointestinal tract (Duarte-Oliveira et al., 2017). Trichosporon species are emerging opportunistic fungi that have been increasingly reported as agents of fungemia in the last years, especially in immunocompromised patients (Challapilla et al., 2019; Sah et al., 2019; Alp et al., 2020). Furthermore, Trichosporon species have been reported as the second leading cause of invasive yeast infection in patients with malignant hematological diseases (Almeida and Hennequin, 2016).
The ability of Trichosporon species to cause systemic infections is likely associated with the expression of virulence factors, such as extracellular lytic compounds, and, most importantly, biofilms (Montoya et al., 2018), microbial communities surrounded by a polymeric extracellular matrix (Zhao et al., 2017). This structure expresses several mechanisms to escape the action of antimicrobials, as well as to resist physical stress, desiccation, UV radiation, and host immune system (Martinez and Casadevall, 2007; Polke et al., 2015). Previous studies have shown that T. asahii and T. inkin biofilms produce extracellular protease (Cordeiro et al., 2015) and also show increased tolerance to antifungals (Cordeiro et al., 2015; Almeida and Hennequin, 2016; Montoya et al., 2018; Cordeiro et al., 2019). Indeed, T. asahii biofilms may be up to 16,000 times more resistant to voriconazole, the most active antifungal against Trichosporon planktonic cells (Di Bonaventura et al., 2006).
Fungal biofilms tolerate high antifungal concentrations by many simultaneous mechanisms (Taff et al., 2013). Previous studies conducted with Candida albicans have shown that antifungal tolerance may be related to the development of a subpopulation of dormant cells within biofilms, known as persister cells (LaFleur et al., 2006). Such persister cells have been related to relapsing infections, as they tolerate antifungal exposure and can revert to an actively growing state, repopulating the biofilm, once treatment is ceased (Del Pozo, 2018).
Despite their relevance as emergent opportunistic species, few studies have addressed mechanisms of antifungal resistance of Trichosporon species in planktonic or sessile forms (Kushima et al., 2012; Kushima et al., 2015; Kushima et al., 2017; Li et al., 2017; Padovan et al., 2019). The present study aimed to evaluate if T. asahii and T. inkin are prone to develop dormant persister cells within biofilms and to suggest their importance in antifungal tolerance.
Material and Methods
Microorganisms
A total of 12 strains of Trichosporon spp. were evaluated in this study (Table 1). Strain identification was based on analysis of micromorphological features on malt agar (De Hoog et al., 2000) and sequencing of intergenic spacer region IG1 of rDNA (Silvestre Junior et al., 2010). Strains were recovered from storage and maintained on potato dextrose agar (PDA; Himedia, India), at 35°C, for 48 h. The isolates belong to the culture collection of the Specialized Medical Mycology Center of Federal University of Ceará, Brazil. The chosen T. asahii strains do not have mechanisms of acquired resistance to amphotericin B (wild-type), according to the epidemiological cutoff values (ECVs), proposed by Francisco et al. (2019) for the species.
C. albicans ATCC 10231 was included as internal control for persister cell isolation; moreover, C. krusei ATCC 6258 and C. parapsilosis ATCC 22019 were used as quality controls for susceptibility assays (CLSI, 2008).
Drugs
Stock solution of amphotericin B (AMB; Sigma Chemical Co., St. Louis, MO, United States) was prepared as recommended by the document M27-A3 (CLSI, 2008). Serial two-fold dilutions of AMB were performed in RPMI 1640 medium (Sigma-Aldrich, MO, United States), buffered to pH 7.0 with 0.165 M morpholinepropanesulfonic acid (MOPS; Sigma-Aldrich, MO, USA).
Biofilm Formation
Biofilm growth was induced as described by Cordeiro et al. (2015). Strains of T. asahii (n=5) and T. inkin (n=7) were grown on potato dextrose agar (PDA, Himedia, Mumbai, India) at 35°C for 48 h. Aliquots of 200 μl of fungal suspension adjusted to 2 x 106 cells/ml in RPMI 1640 medium were added to flat-bottomed 96-well polystyrene microplates and incubated at 35°C for 6 h (adhesion period) at 80 rpm. After incubation, non-adherent cells were removed by washing with sterile phosphate buffer saline with 0.05% Tween 20 (PBS-Tween 20). Afterwards, the wells were filled with RPMI medium and incubated at 35°C for 48 h (maturation period) at 80 rpm.
Detection of Persister Cells
The presence of persister cells in Trichosporon spp. biofilms was evaluated according to the methodology described by LaFleur et al. (2006) for C. albicans biofilms. Mature biofilms were washed with sterile PBS-Tween 20 and then treated with 100 μg/ml AMB and incubated at 35°C for additional 24 h (La Fleur et al., 2006). Thereafter, the wells were washed with sterile PBS-Tween 20, scraped with a pipette tip and the cells were resuspended in 100 μl in sterile PBS. Suspensions were serially diluted, plated on PDA agar and incubated at 35°C for 24 h for viable cell counting. Cells that survived exposure to 100 μg/mL AMB treatment were considered persister cells (LaFleur et al., 2006) Biofilms formed in RPMI 1640 medium without antifungal drug were included as biofilm growth control. C. albicans ATCC 10231 was included as control for validation of the methodology described by LaFleur et al. (2006), as it has been shown to produce dormant cells in the presence of AMB (Boucherit et al., 2007). All assays were performed in triplicate, at two independent experiments.
Regeneration of Biofilms by Persister Cells
After observing the presence of persister cells in Trichosporon biofilms, we tested their ability to regenerate biofilms on an abiotic surface. Persister cells were induced and isolated as described above. Thereafter, cell suspensions were adjusted in RPMI medium, transferred to flat-bottomed 96-well polystyrene plates and incubated at 35°C for 48 h at 80 rpm order to form progeny biofilms. Results were compared with the following paired controls: (1) mature biofilms produced by planktonic cells; and (2) progeny biofilms produced by cells detached from mature biofilms. Biofilms were evaluated for metabolic activity and biomass, by the XTT reduction (Cordeiro et al., 2015) and crystal violet staining assays (Pierce et al., 2015), respectively.
AMB Susceptibility of Persister Cells, Biofilm Cells, and Planktonic Cells
Susceptibility of persister cells to AMB was accessed by the broth microdilution method (CLSI, 2008). For isolation of persister cells, 48-h mature biofilms grown in RPMI-medium without antifungals were incubated with 100 µg/ml AMB for 24 h. Progeny biofilms were formed by inoculating persister cells into drug-free RPMI medium and repeating the biofilm growth procedure described above. Results were compared with the following paired controls: (1) planktonic cells; (2) mature biofilms produced by planktonic cells; (3) progeny biofilms produced by cells detached from mature biofilms; and (4) progeny biofilms produced by persister cells. Planktonic cells were obtained from 48-h culture on PDA at 35°C. Cell suspensions from biofilms were obtained after scraping of the wells and centrifugation at 9,167 xg for 10 min. Inocula were prepared in sterile saline solution and adjusted to a final concentration of 0.5–2.5 × 103 cells/ml in RPMI 1640 medium buffered to pH 7.0 with 0.165 M MOPS. AMB was tested in concentrations ranging from 0.25 to 128 µg/ml (Cordeiro et al., 2015). Plates were incubated at 35°C for 48 h and fungal growth was visually determined analyzed. The minimum inhibitory concentration (MIC) was defined as the lowest concentration able of inhibiting 100% of visual fungal growth. Isolates were tested in triplicate, at two independent experiments. Controls were grown in RPMI medium without AMB. C. krusei ATCC 6258 and C. parapsilosis ATCC 22019 were included as quality controls.
Biofilm Structure and Morphology
Structural analysis of Trichosporon biofilms (T. asahii, CEMM 05-6-072, urine; T. inkin, CEMM 05-6-074, urine) was performed by scanning electron microscopy (SEM) and Confocal Laser Scanning Microscopy (CLSM), as described elsewhere (Di Bonaventura et al., 2006; Cordeiro et al., 2017). Biofilms were formed on Thermanox® slides (Thermo Fisher Scientific, NY, USA) in 12-well polystyrene plates with RPMI medium as described above and incubated at 35°C for 48 h at 80 rpm. Paired controls were grown in RPMI medium without antimicrobials. For SEM analysis, biofilms were fixed with 2.5% glutaraldehyde in 0.15M sodium cacodylate buffer and incubated overnight at 4°C. Biofilms were washed twice with 0.15M cacodylate buffer for 5 min and dehydrated in ethanol. Slides were dried with hexamethyldisilazane (Polysciences Europe, Germany) for 30 min, coated with 10nm gold (Emitech Q150T, Lewes, UK) and observed in a SEM (FEI Inspect S50, Thermo Fisher Scientific, Hillsboro, Oregon, USA) in the high vacuum mode at 15 kV. For CLSM, biofilms were stained with the Live/DeadTM (Invitrogen, Molecular Probes, Carlsbad, CA, USA) and evaluated with a confocal Nikon C2 C microscope (Nikon, Melville, NY, USA), at 488 nm, for the detection of SYTO 9 (live cells) and at 561 nm, for the detection of propidium iodide (dead/damaged cells). Images were processed using ImageJ Software (Collins, 2007). The software COMSTAT was used for quantitative analysis of the image stacks produced by CLSM.
Statistical Analysis
Parametric data were analyzed using Student’s t-test or one-way analysis of variance (ANOVA) followed by Tukey’s post-hoc test. For data with asymmetry, Wilcoxon’s or Friedman’s nonparametric tests followed by Dunn’s post-hoc test were applied. P-values < 0.05 were considered statistically significant. Statistical Analysis was performed using the software GraphPad Prism 7.0 (GraphPad Software, CA, USA).
Results
Presence of Persister Cells in Trichosporon Biofilms
The colony-forming unit (CFU) counts showed the presence of viable cells in Trichosporon biofilms after exposure to 100 μg/mL AMB. Counts ranged from 1.1 x 105 to 2.26 x 105 CFU/mL for T. asahii and 2.6 x 104 to 2.5 x 105 T. inkin strains (Figure 1A). Biofilm growth controls ranged from 3.53 x 106 to 7.7 x 106 CFU/mL and 1.12 x 106 to 5.75 x 106, for T. asahii and T. inkin, respectively. Persister cells consisted approximately 4% of viable cells for both T. asahii and T. inkin biofilms (Figure 1B). Statistically significant differences were observed when compared to drug-free growth control (P<0.05). The number of persister cells of C. albicans (2 x 104 CFU/ml) corresponds to approximately 1.45% of the total number of cells within biofilm growth control (1.38 x 106 CFU/ml).
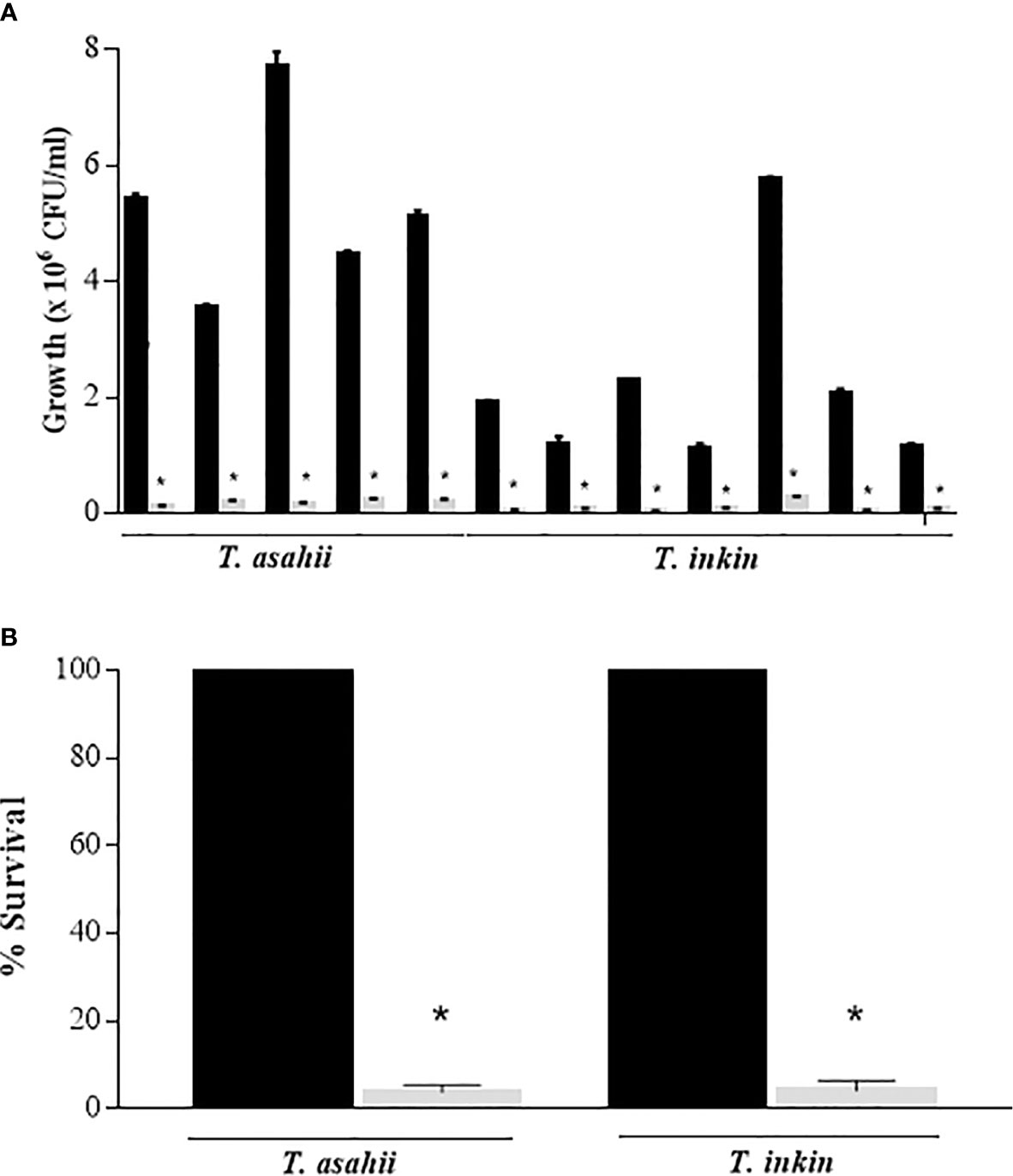
Figure 1 Detection of persister cells in T. asahii and T. inkin biofilms (grey bars). (A) Mature biofilms formed in RPMI medium were treated with 100 µg/mL AMB. (B) Survival of T. asahii and T. inkin biofilms challenged with 100 µg/mL AMB. Biofilm controls were grown in drug-free RPMI medium (black bars). Values are shown as mean ± SD. *Statistically significant differences when compared to the respective drug-free control (P < 0.05).
Persister Cells of Trichosporon Can Form New Biofilms
Mature Trichosporon biofilms were challenged with AMB (100 µg/ml), then, progeny biofilms were formed by inoculating persister cells into RPMI medium, as previously described. Persister cells from each strain were able to form progeny biofilms. In general, a significant reduction in metabolic activity and biomass was observed for both species, when compared to progeny biofilms produced by cells detached from mature biofilms (P<0.05), as shown in Figure 2.
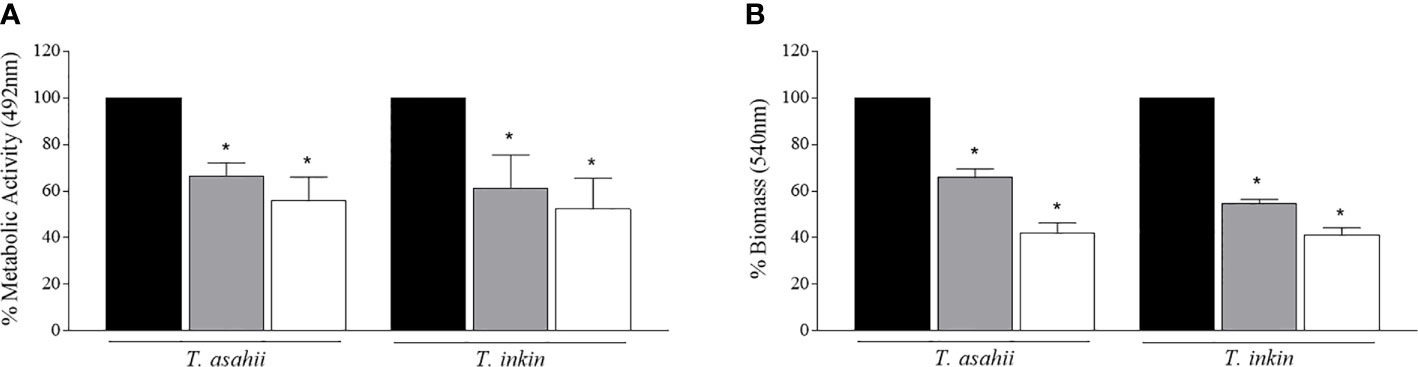
Figure 2 Regeneration of T. asahii and T inkin biofilms. Biofilms were formed in RPMI medium and produced by planktonic cells (black bars), cells detached from mature biofilms (grey bars) or persister cells (white bars). Metabolic activity and total biomass were expressed as relative percentages of absorbance by the XTT reduction (A) and crystal violet staining (B) assays. *Statistically significant differences when compared to the biofilm growth control produced by planktonic cells (P < 0.05).
Persister Cells Showed Tolerance to AMB
Persister cells presented increased tolerance to AMB, compared to planktonic cells and biofilm-detached cells (Table 2). MICs against persister cells from T. asahii and T. inkin biofilms ranged from 64 to >128 µg/mL (geometric mean: 111.43 µg/mL and 115.93 µg/mL, respectively). MICs against planktonic cells ranged from 0.25 to 2.0 µg/mL for T. asahii (geometric mean: 1.41 µg/mL) and 0.5 µg/mL to 1.0 µg/mL for T. inkin (geometric mean: 1.0 µg/mL). MICs against mature biofilm-detached cells ranged from 16 to 64 µg/mL (geometric mean: 36.75 µg/mL and 28.98 µg/mL, for T. asahii and T. inkin, respectively). MICs against progeny biofilm-detached cells ranged from 8 to 32 µg/mL (geometric mean: 21.11 µg/mL and 14.5 µg/mL, for T. asahii and T. inkin, respectively). Cells from progeny biofilms from persister cells showed increased susceptibility to AMB, when compared to other biofilm-derived cells, displaying MICs ranging from 2 to 8 µg/mL (geometric mean: 5 µg/mL for both species). AMB MIC of control strains C. krusei ATCC and C. parapsilosis ATCC 22019 were according CLSI guidelines (CLSI, 2008).
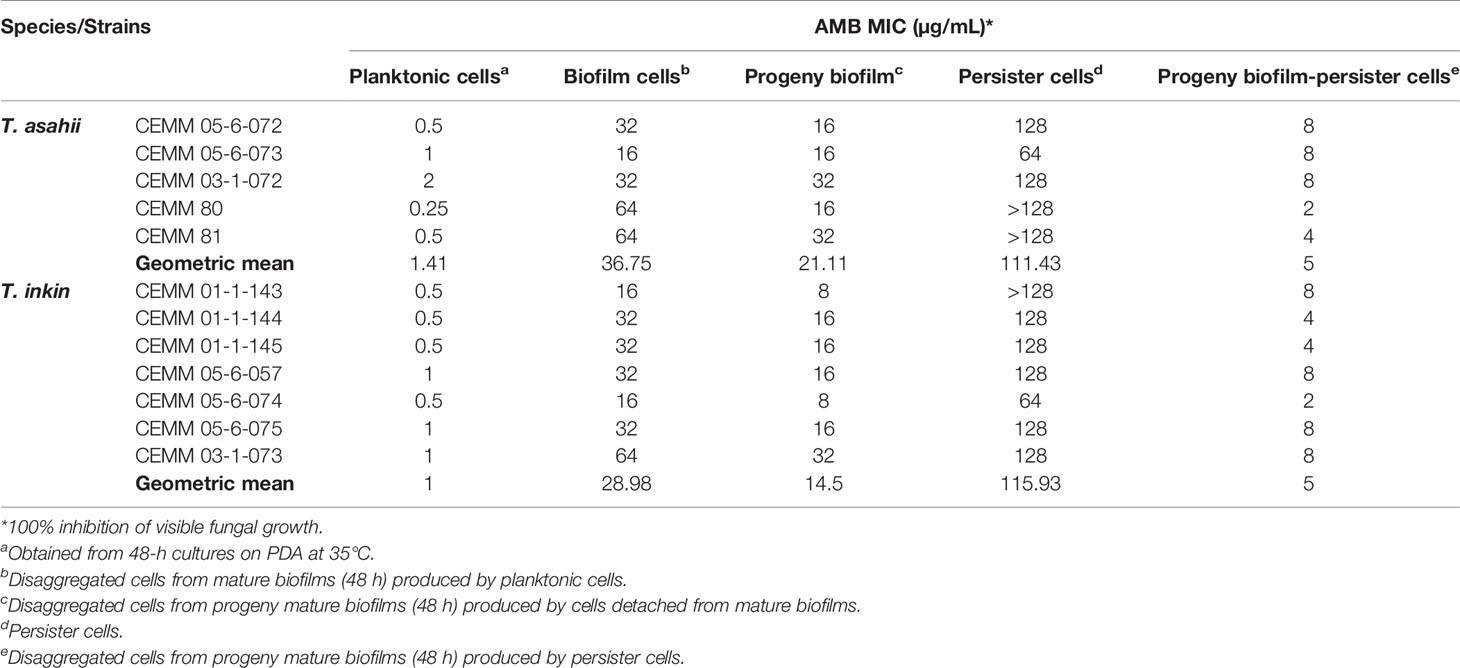
Table 2 Minimum inhibitory concentration (MIC) of amphotericin B (AMB) against persister cells, biofilm cells and planktonic cells of T. asahii and T. inkin strains.
Biofilm Ultrastructure
Persister cells were compared to biofilm growth control by SEM (Figure 3) and CLSM (Figure 4). Structured biofilms with high cell density enclosed by a dense extracellular material were seen in drug-free growth controls (Figures 3A, B, and 4A, B). AMB (100 µg/mL) was able to disrupt the three-dimensional structure of mature biofilms, leaving deformed fungal structures (Figures 3C, D) and rare live cells (Figures 4C, D). Persister cells were able to form progeny biofilms with fewer filaments and less extracellular matrix (Figures 3E, F and 4E, F) than biofilm growth control. Progeny biofilms produced by cells detached from mature biofilms also showed fewer filaments and less extracellular matrix than the drug-free growth control (Figures 3G, H and 4G, H).
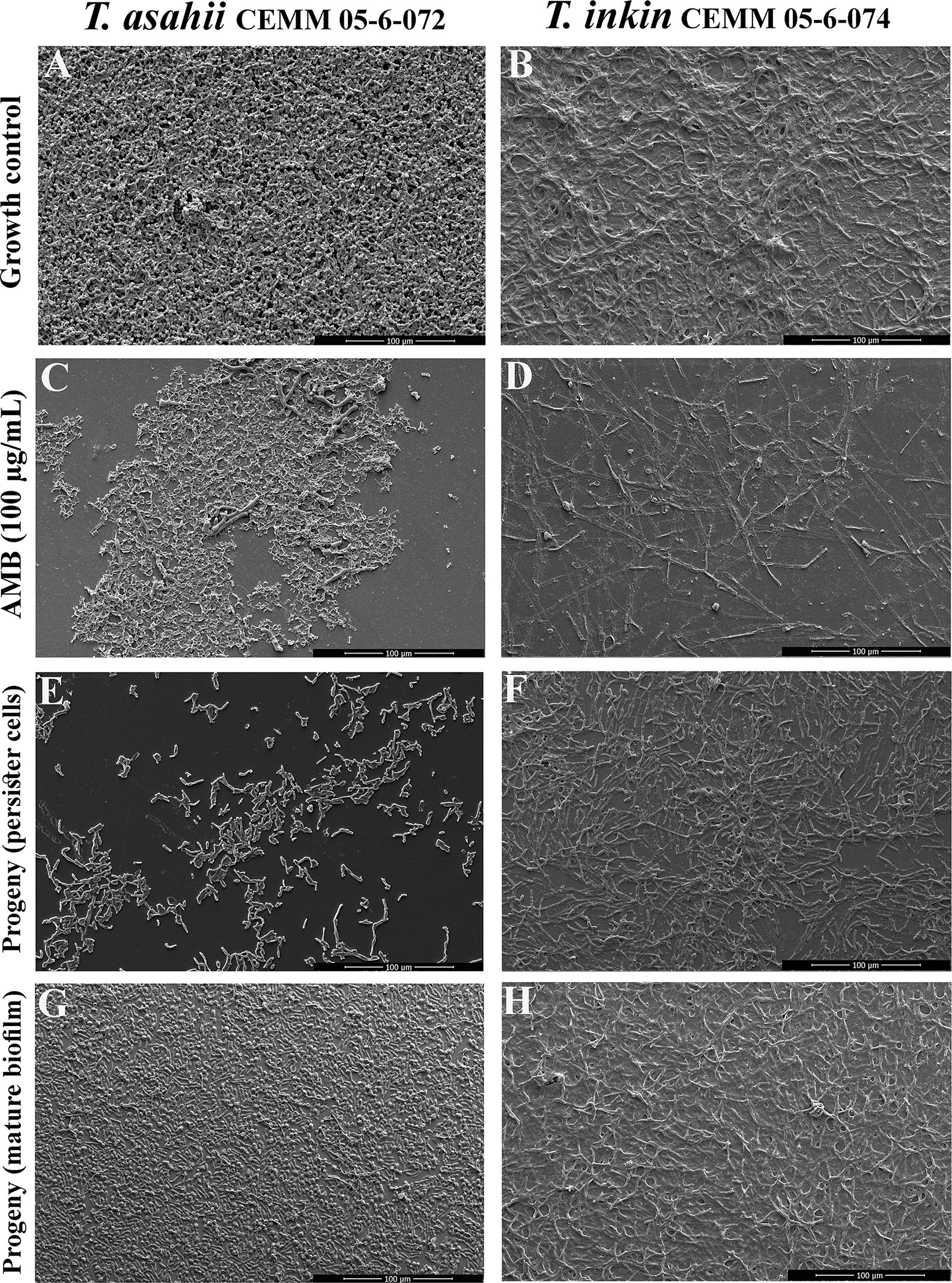
Figure 3 Ultrastructure of T. asahii CEMM 05-6-072 and T. inkin CEMM 05-6-074 biofilms by SEM. Sessile cells were formed on Thermanox™ coverslips in drug-free RPMI medium as controls (A, B). Mature biofilms (48 h) were challenged with 100 μg/mL AMB and incubated at 35°C for 24 h (C, D). Surviving cells were considered persisters. Biofilms were then scrapped with a pipette tip and inocula were prepared in RPMI medium. Progeny biofilms produced by persister cells (E, F) or mature biofilms-detached cells (G, H) were formed in RPMI medium.
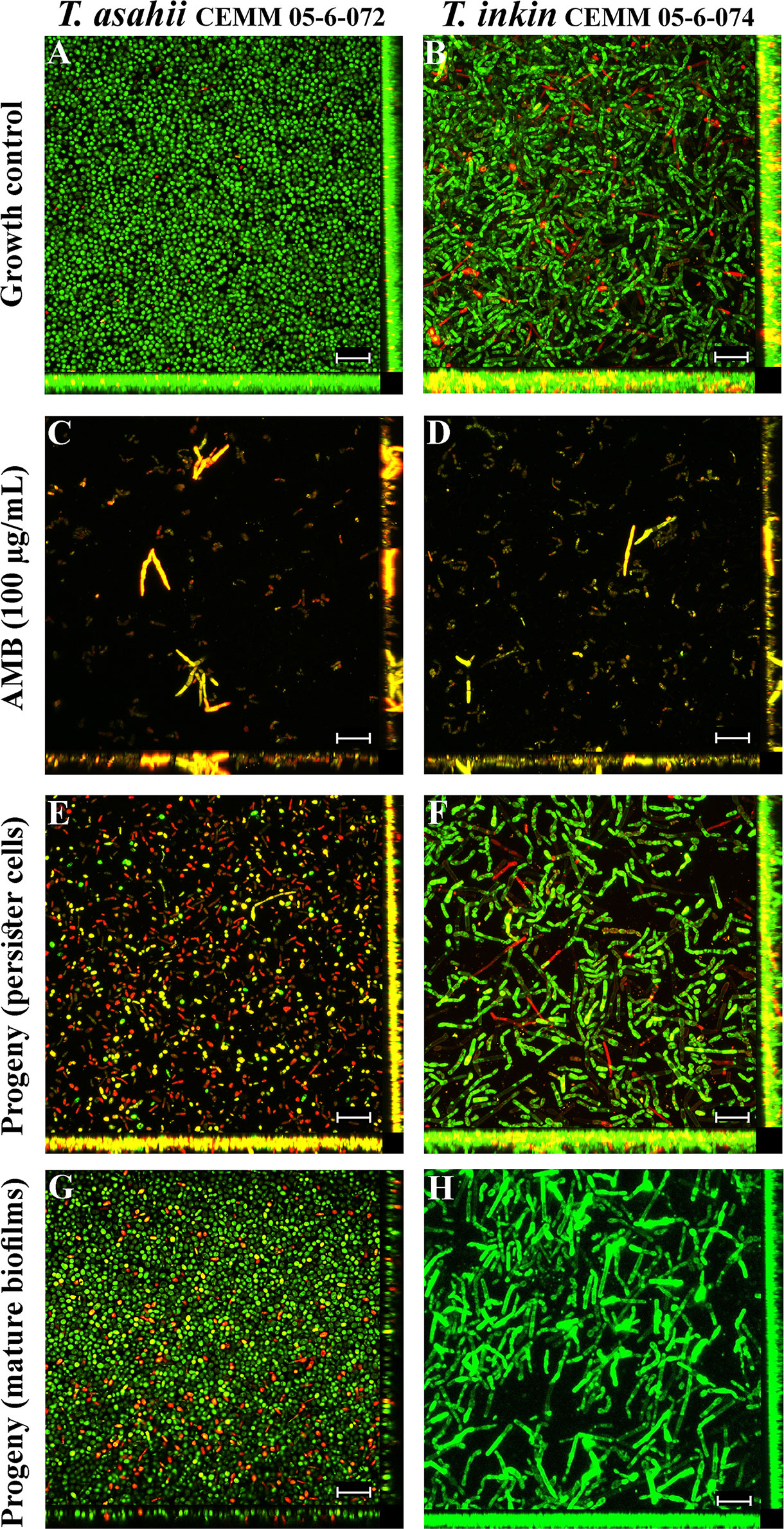
Figure 4 Scanning electron microscopy of T. asahii CEMM 05-6-072 and T. inkin CEMM 05-6-074 biofilms. Sessile cells were formed on Thermanox™ coverslips in drug-free RPMI medium as controls (A, B). Mature biofilms (48 h) were challenged with 100 μg/mL AMB (C, D) for detection of persister cells. Progeny biofilms produced by persister cells (E, F) or mature biofilms-detached cells were formed in RPMI medium (G, H). Live cells are shown in green and dead/damaged cells are shown in yellow/red after stain with SYTO9 and propidium iodide, respectively. Magnification: 400x. Bar: 100 μm.
Discussion
Mechanisms associated with antifungal tolerance have already been described in Candida biofilms and include increased efflux pump activity; drug sequestration by extracellular matrix (Cohen et al., 2013; Wuyts et al., 2018); expression of resistance genes (Li et al., 2015); reduction of cell ergosterol concentration (Mukherjee et al., 2003) and production of persistent cells (LaFleur et al., 2006; Al-Dhaheri and Douglas, 2008; Delarze and Sanglard, 2015; Wuyts et al., 2018). Although the antifungal tolerance in Trichosporon biofilms is widely reported (Falk et al., 2003; Di Bonaventura et al., 2006; Iturrieta-González et al., 2014; Cordeiro et al., 2015), the mechanisms underlying this phenotype have not been described.
LaFleur et al. (2006) were the first to describe the occurrence of persister cells in fungal biofilms, presenting evidence that this phenomenon occurs in C. albicans biofilms. Authors challenged mature biofilms with up to 900 µg/mL of AMB and showed that most of the population was killed by AMB at low concentrations but nearly 1% of the cells seemed to be completely invulnerable to higher concentrations of the drug. Since then, persister cells have been described in biofilms of C. parapsilosis, C. krusei (Al-Dhaheri and Douglas, 2008) and Saccharomyces cerevisiae (Bojsen et al., 2014).
Persister cells are phenotypic variants that are maintained in a state of dormancy, being able to survive the oxidative stress induced by high concentrations of antifungals through the activation of energy storage pathways (Wuyts et al., 2018). As these dormant cells have low metabolic activity, they have been detected by counting colony-forming units, instead of using viability reagents (LaFleur et al., 2006; Al-Dhaheri and Douglas, 2008; LaFleur et al., 2010; Li et al., 2015; Sun et al., 2016; Wu et al., 2019; Yee et al., 2019; Galdiero et al., 2020; Wu et al., 2020). In the present study, we observed that clinical strains of T. asahii and T. inkin produced persister cells inside their biofilms. Using the methodology originally proposed by LaFleur for the detection of persister cells in C. albicans biofilms, it was observed that the proportion of persister cells varied from 2 to 5% for T. asahii and from 2 to 6% for T. inkin. These values are higher than those found in C. albicans (LaFleur et al., 2006), C. parapsilosis and C. krusei biofilms (Al-Dhaheri and Douglas, 2008), which usually comprise up to 2% of the total population. We do not know if these results indicate intrinsic properties of Trichosporon biofilms or if they are derived from the small number of strains investigated in the present study.
The concept of persister cells also encompass a biphasic pattern of killing when a sessile population is challenged with an antifungal drug at concentrations well above MIC (Wuyts et al., 2018). In our study, mature biofilms of Trichosporon were challenged with increased concentrations of AMB (10, 50 and 100 µg/mL) and a biphasic pattern of killing was seen: whereas 50 µg/mL AMB suppressed Trichosporon biofilms (data not shown), few cells remained alive after challenge with 100 µg/mL of AMB, as shown by CFU counting and confocal microscopy. Previous studies have shown that MIC values for AMB against Trichosporon biofilm-cells were, in general, below 50 µg/mL (Iturrieta-González et al., 2014; Cordeiro et al., 2015; Montoya et al., 2018).
In the present study, persister cells of T. asahii and T. inkin were able to originate progeny biofilms, however, these biofilms presented less biomass and reduced metabolic activity when compared to the progeny biofilms produced by cells detached from mature biofilms. Such results were corroborated by the ultrastructural analysis, which revealed that the biofilms deriving from persister cells have lower cell density. Detached cells from these progeny biofilms were less tolerant to AMB than persister cells contained in the starter inoculum. These results suggest that Trichosporon persister cells are not mutants but phenotypic variants of regular cells, as previously demonstrated for other fungal species (LaFleur et al., 2006; Al-Dhaheri and Douglas, 2008; Wuyts et al., 2018).
Besides the intracellular accumulation of energy storage molecules (Li et al., 2015; Wuyts et al., 2018), it is supposed that persister cells may have a different cell wall composition (Wuyts et al., 2018) and increased extracellular matrix production (Li et al., 2015). Previous studies have shown that the extracellular matrix actively contributes to the antifungal resistance of biofilms, by inducing drug-sequestration and harboring drug-efflux proteins (Li et al., 2015; Wuyts et al., 2018; Berman and Krysan, 2020). Although we were not able to study the cell wall properties of persister cells of T. asahii and T. inkin, microscopy analyses showed that these cells and their progeny biofilms had less extracellular matrix, resulting in thinner biofilms. The reduced amount of extracellular matrix observed in our results reinforces the hypothesis that the tolerance of Trichosporon biofilms to AMB at 100 µg/mL is derived from the presence of persister cells.
Experimental evidences suggest that persister cells are directly related to the occurrence of recalcitrant microbial infections. Unequivocal proof was first presented by Mulcahy et al. (2010) who showed a direct relationship between the presence of persister cells of Pseudomonas aeruginosa in patients with cystic fibrosis pneumonia. Studies performed with persister cells from bacterial biofilms prove that these cells, besides presenting reduced susceptibility to antibiotics, are better suited to survive the host immune system, persist within catheter-associated biofilm infection (Daubert et al., 2020) and cause more severe disease (Yee et al., 2019). Regarding fungal infections, LaFleur et al. (2010) showed that in vivo selection for high-persister mutants occurs in cancer patients that harbor Candida. Recently, it was hypothesized that persister cells inside biotic biofilms formed on vaginal epithelium and underlying tissues are related to recalcitrant vulvovaginal candidiasis (Wu et al., 2019; Wu et al., 2020). Although the virulence of fungal persister cells is not fully understood, the occurrence of dormant cells in biotic biofilms demands the attention of clinicians and researchers, since the available antifungals have low activity against biofilms and persister cells (Galdiero et al., 2020).
In conclusion, the results presented here show that T. asahii and T. inkin produce persister cells in their biofilms. These cells have a high tolerance to AMB and provide an inoculum for cell growth and new biofilm formation. Future studies should be conducted in order to understand the genetic regulation associated with the production of persister cells in Trichosporon biofilms and their phenotypic tolerance to AMB. Physicians should be aware that the production of persister cells within biofilms may be related to therapeutic failure in invasive trichosporonosis.
Data Availability Statement
The original contributions presented in the study are included in the article/supplementary material. Further inquiries can be directed to the corresponding author.
Author Contributions
RC and AA designed the research, analyzed the data and wrote the manuscript. AA, BS, LP, and FP performed the experiments. DC-B captured CLSM images. DC-B, ZC, RL-N, MR, and JC critically revised the manuscript. All authors contributed to the article and approved the submitted version.
Conflict of Interest
The authors declare that the research was conducted in the absence of any commercial or financial relationships that could be construed as a potential conflict of interest.
Acknowledgments
This study was supported by Conselho Nacional de Desenvolvimento Científico e Tecnológico, Brazil (Process 430193/2018-1). The authors are grateful to Central Analítica-UFC/CT-INFRA/MCTI-SISNANO/Pró-Equipamentos CAPES and Centro de Tecnologias Estratégicas do Nordeste-MCTIC.
References
Al-Dhaheri R. S., Douglas L. J. (2008). Absence of amphotericin B-tolerant persister cells in biofilms of some Candida species. Antimicrob. Agents Chemother. 52, 1884–1887. doi: 10.1128/AAC.01473-07
Almeida J. N. Jr., Hennequin C. (2016). Invasive Trichosporon Infection: a systematic review on a re-emerging fungal pathogen. Front. Microbiol. 7, 1629. doi: 10.3389/fmicb.2016.01629
Alp S., Gulmez D., Ayaz C. M., Arıkan-Akdaglı S., Akova M. (2020). Fungemia due to rare yeasts in a tertiary care university center within 18 years. Mycoses 63, 488–493. doi: 10.1111/myc.13072
Berman J., Krysan D. J. (2020). Drug resistance and tolerance in fungi. Nat. Rev. Microbiol. 18, 319–331. doi: 10.1038/s41579-019-0322-2
Bojsen R., Regenberg B., Folkesson A. (2014). Saccharomyces cerevisiae biofilm tolerance towards systemic antifungals depends on growth phase. BMC Microbiol. 14, 305. doi: 10.1186/s12866-014-0305-4
Boucherit Z., Seksek O., Bolard J. (2007). Dormancy of Candida albicans cells in the presence of the polyene antibiotic amphotericin B: simple demonstration by flow cytometry. Med. Mycol. 45 (6), 525–533. doi: 10.1080/13693780701487821
Challapilla M., Patel K., Patel B., Soman R., Rodrigues C., Shetty A. (2019). Trichosporon-blood stream infection. J. Assoc. Physicians India 67, 19–21.
CLSI (2008). “Reference Method for Broth Dilution Antifungal Susceptibility Testing of Yeasts; Approved Standard - Third Edition,” in CLSI document M27-A3. Wayne, PA, USA.
Cohen N. R., Lobritz M. A., Collins J. J. (2013). Microbial persistence and the road to drug resistance. Cell Host Microbe 13, 632–642. doi: 10.1016/j.chom.2013.05.009
Cordeiro R., Serpa R., Alexandre C. F. U., Marques F. J. F., Melo C. V. S., Franco J. S., et al. (2015). Trichosporon inkin biofilms produce extracellular proteases and exhibit resistance to antifungals. J. Med. Microbiol. 64, 1277–1286. doi: 10.1099/jmm.0.000159
Cordeiro R. A., Serpa R., Mendes P. B. L., Evangelista A. J. J., Colares A. R. C., Franco J. S., et al. (2017). The HIV aspartyl protease inhibitor ritonavir impairs planktonic growth, biofilm formation and proteolytic activity in Trichosporon spp. Biofouling 33, 640–650. doi: 10.1080/08927014.2017.1350947
Cordeiro R. A., Aguiar A. L. R., Pereira V. S., Pereira L. M. G., Portela F. V. M., Brilhante R. S. N., et al. (2019). Sodium butyrate inhibits planktonic and sessile cells of Trichosporon spp. Microb. Pathog. 130, 219–225. doi: 10.1016/j.micpath.2019.03.013
Daubert T. A., Kluthe K., Nuxoll A. (2020). S. aureus persister cells have increased survival to components of innate immunity, leading to persistence within a catheter-associated biofilm infection. J. Immunol. 204 (1 Supplement), 156.11.
De Hoog G. S., Guarro J., Gene´ J., Figueiras M. J. (2000). Atlas of Clinical Fungi. 2nd ed (Washington: ASM Press).
Del Pozo J. L. (2018). Biofilm-related disease. Expert. Rev. Anti. Infect. Ther. 16, 51–65. doi: 10.1080/14787210.2018.1417036
Delarze E., Sanglard D. (2015). Defining the frontiers between antifungal resistance, tolerance and the concept of persistence. Drug Resist. Update 23, 12–19. doi: 10.1016/j.drup.2015.10.001
Di Bonaventura G., Pompilio A., Picciani C., Iezzi M., D’antonio D., Piccolomini R. (2006). Biofilm formation by the emerging fungal pathogen Trichosporon asahii: development, architecture and antifungal resistance. Antimicrob. Agents Chemother. 50, 3269–3276. doi: 10.1128/AAC.00556-06challa
Duarte-Oliveira C., Rodrigues F., Gonçalves S. M., Goldman G. H., Carvalho A., Cunha C. (2017). The cell biology of the Trichosporon-host interaction. Front. Cell Infect. Microbiol. 7, 118. doi: 10.3389/fcimb.2017.00118
Falk R., Wolf D. G., Shapiro M., Polacheck I. (2003). Multidrug-resistant Trichosporon asahii isolates are susceptible to voriconazole. J. Clin. Microbiol. 41, 911. doi: 10.1128/JCM.41.2.911.2003
Francisco E. C., de Almeida Junior J. N., de Queiroz Telles F., Aquino V. R., Mendes A. V. A., de Andrade Barberino M. G. M., et al. (2019). Species distribution and antifungal susceptibility of 358 Trichosporon clinical isolates collected in 24 medical centres. Clin. Microbiol. Infect. 25, 909.e1–909.e5. doi: 10.1016/j.cmi.2019.03.026
Galdiero E., de Alteriis E., De Natale A., Siciliano A., Guida M., Lombardi L., et al. (2020). Eradication of Candida albicans persister cell biofilm by the membranotropic peptide gH625. Sci. Rep. 10, 5780. doi: 10.1038/s41598-020-62746-w
Iturrieta-González I. A., Padovan A. C. B., Bizerra F. C., Hahn R. C., Colombo A. N. (2014). Multiple species of Trichosporon produce biofilms highly resistant to triazoles and amphotericin B. PLoS One 9, e109553. doi: 10.1371/journal.pone.0109553
Kushima H., Tokimatsu I., Ishii H., Kawano R., Shirai R., Kishi K., et al. (2012). Cloning of the lanosterol 14-α-demethylase (ERG11) gene in Trichosporon asahii: a possible association between G453R amino acid substitution and azole resistance in T. asahii. FEMS Yeast Res. 12, 662–667. doi: 10.1111/j.1567-1364.2012.00816.x
Kushima H., Tokimatsu I., Ishii H., Kadota J. (2015). Antifungal susceptibility and drug-resistant mechanism of Trichosporon. Med. Mycol. J. 56, J123–J128. doi: 10.3314/mmj.56.J123
Kushima H., Tokimatsu I., Kawano R., Watanabe K., Kadota J.-i. (2017). A new amino acid substitution at G150S in lanosterol 14-α demethylase (Erg11 protein) in multi-azole-resistant Trichosporon asahii. Med. Mycol. J. 58, E23–E28. doi: 10.3314/mmj.16-00027
LaFleur M. D., Kumamoto C. A., Lewis K. (2006). Candida albicans biofilms produce antifungal-tolerant persister cells. Antimicrob. Agents Chemother. 50, 3839–3846. doi: 10.1128/AAC.00684-06
LaFleur M. D., Qi Q., Lewis K. (2010). Patients with long-term oral carriage harbor high-persister mutants of Candida albicans. Antimicrob. Agents Chemother. 54, 39–44. doi: 10.1128/AAC.00860-09
Li H., Wang C., Chen Y., Zhang S., Yang R. (2017). Integrated transcriptomic analysis of Trichosporon Asahii uncovers the core genes and pathways of fluconazole resistance. Sci. Rep. 7, 17847. doi: 10.1038/s41598-017-18072-9
Li P., Seneviratne C. J., Alpi E., Vizcaino J. A., Jin L. (2015). Delicate metabolic control and coordinated stress response critically determine antifungal tolerance of Candida albicans biofilm persisters. Antimicrob. Agents Chemother. 59, 6101–6112. doi: 10.1128/AAC.00543-15hoog
Martinez L. R., Casadevall A. (2007). Cryptococcus neoformans biofilm formation depends on surface support and carbon source and reduces fungal cell susceptibility to heat, cold, and UV light. Appl. Environ. Microbiol. 73, 4592–4601. doi: 10.1128/AEM.02506-06
Montoya A. M., Elizondo-Zertucge M., Treviño-Rangel R. J., Becerril-Gargia M., González G. M. (2018). Biofilm formation and antifungal susceptibility of Trichosporon asahii isolates from Mexican patients. Rev. Iberoam Micol. 35, 22–26. doi: 10.1016/j.riam.2017.02.008
Mukherjee P. K., Chandra J., Kuhn D. M., Ghannoum M. A. (2003). Mechanism of fluconazole resistance in Candida albicans biofilms: phase-specific role of efflux pumps and membrane sterols. Infect. Immun. 71, 4333–4340. doi: 10.1128/iai.71.8.4333-4340.2003
Mulcahy L. R., Burns J. L., Lory S., Lewis K. (2010). Emergence of Pseudomonas aeruginosa strains producing high levels of persister cells in patients with cystic fibrosis. J. Bacteriol. 192, 6191–6199. doi: 10.1128/JB.01651-09
Padovan A. C. B., Rocha W. P. D. S., Toti A. C. M., Jesus D. F. F., Chaves G. M., Colombo A. L. (2019). Exploring the resistance mechanisms in Trichosporon asahii: Triazoles as the last defense for invasive trichosporonosis. Fungal Genet. Biol. 133, 103267. doi: 10.1016/j.fgb.2019.103267
Pierce C. G., Chaturvedi A. K., Lazzell A. L., Powell A. T., Saville S. P., McHardy S. F., et al. (2015). A novel small molecule inhibitor of Candida albicans biofilm formation, filamentation and virulence with low potential for the development of resistance. NPJ Biofilms Microbiomes 1, 15012. doi: 10.1038/npjbiofilms.2015.12
Polke M., Hube B., Jacobsen I. D. (2015). Candida survival strategies. Adv. Appl. Microbiol. 91, 139–235. doi: 10.1016/bs.aambs.2014.12.002
Sah R., Soin A. S., Chawla S., Wadhwa T., Gupta N. (2019). Disseminated Trichosporon asahii infection in a combined liver-kidney transplant recipient successfully treated with voriconazole. Immun. Inflamm. Dis. 7, 125–129. doi: 10.1002/iid3.250
Silvestre Junior A. M., Miranda M. A. B. R., Camargo Z. P. (2010). Trichosporon species isolated from the perigenital region, urine and catheters of a Brazilian population. Braz. J. Microbiol. 41, 628–634. doi: 10.1590/S1517-83822010000300013
Sun J., Li Z., Chu H., Guo J., Jiang G. (2016). Candida albicans amphotericin B-tolerant persister formation is closely related to surface adhesion. Mycopathologia 181, 41–49. doi: 10.1007/s11046-015-9894-1
Taff H. T., Mitchell K. F., Edward J. A., Andes D. R. (2013). Mechanisms of Candida biofilm drug resistance. Future Microbiol. 8, 1325–1337. doi: 10.2217/fmb.13.101
Wu X., Zhang S., Xu X., Shen L., Xu B., Qu W., et al. (2019). RAFT-derived polymethacrylates as a superior treatment for recurrent vulvovaginal candidiasis by targeting biotic biofilms and persister cells. Front. Microbiol. 10, 2592. doi: 10.3389/fmicb.2019.02592
Wu X., Zhang S., Li H., Shen L., Dong C., Sun Y., et al. (2020). Biofilm formation of Candida albicans facilitates fungal infiltration and persister cell formation in vaginal candidiasis. Front. Microbiol. 11, 1117. doi: 10.3389/fmicb.2020.01117
Wuyts J., Van Dijck P., Holtappels M. (2018). Fungal persister cells: the basis for recalcitrants infections? PLoS Pathog. 14, e1007301. doi: 10.1371/journal.ppat.1007301
Yee R., Yuan Y., Shi W., Brayton C., Tarff A., Feng J., et al. (2019). Infection with persister forms of Staphylococcus aureus causes a persistent skin infection with more severe lesions in mice: failure to clear the infection by the current standard of care treatment. Discov. Med. 28, 7–16.
Keywords: antifungal susceptibility, dormant cells, Trichosporon spp., amphotericin B, trichosporonosis
Citation: Cordeiro RdA, Aguiar ALR, Silva BN, Pereira LMG, Portela FVM, Camargo ZP, Lima-Neto RG, Castelo-Branco DdSCM, Rocha MFG and Sidrim JJC (2021) Trichosporon asahii and Trichosporon inkin Biofilms Produce Antifungal-Tolerant Persister Cells. Front. Cell. Infect. Microbiol. 11:645812. doi: 10.3389/fcimb.2021.645812
Received: 24 December 2020; Accepted: 17 February 2021;
Published: 22 April 2021.
Edited by:
Maria José Soares Mendes Giannini, São Paulo State University, BrazilReviewed by:
Aylin Döğen, Mersin University, TurkeyTaissa Villa, Federal University of Rio de Janeiro, Brazil
Copyright © 2021 Cordeiro, Aguiar, Silva, Pereira, Portela, Camargo, Lima-Neto, Castelo-Branco, Rocha and Sidrim. This is an open-access article distributed under the terms of the Creative Commons Attribution License (CC BY). The use, distribution or reproduction in other forums is permitted, provided the original author(s) and the copyright owner(s) are credited and that the original publication in this journal is cited, in accordance with accepted academic practice. No use, distribution or reproduction is permitted which does not comply with these terms.
*Correspondence: Rossana de Aguiar Cordeiro, cm9zc2FuYWNvcmRlaXJvQHVmYy5icg==
†These authors have contributed equally to this work