- 1Liaoning Provincial Key Laboratory of Oral Diseases, Department of Oral Biology, School and Hospital of Stomatology, China Medical University, Shenyang, China
- 2Liaoning Provincial Key Laboratory of Oral Diseases, Department of Periodontics, School and Hospital of Stomatology, China Medical University, Shenyang, China
Chronic obstructive pulmonary disease (COPD) is the third leading cause of mortality worldwide, and inflammatory damage induced by bacterial infections is an important contributor to the etiology of COPD. Fusobacterium nucleatum, a recognized periodontal pathogen, is considered as a biomarker of lung function deterioration of COPD patients coinfected with Pseudomonas aerugionsa, but the underlying mechanism is still unclear. This study established single- and dual-species infection models, bacterial simultaneous and sequential infection models, and found that F. nucleatum could coaggregate with P. aeruginosa to synergistically invade into pulmonary epithelial cells and transiently resist P. aeruginosa-induced cytotoxic damage to amplify IL-6 and TNF-α associated inflammation in pulmonary epithelial cells simultaneously infected with P. aeruginosa and F. nucleatum. Furthermore, F. nucleatum pretreatment or subsequential infection could maintain or even aggravate P. aeruginosa-induced inflammatory cytotoxicity of pulmonary epithelial cells. These results indicate that oral pathogen F. nucleatum coaggregates with P. aeruginosa to facilitate bacterial invasion and modulates the inflammatory cytotoxicity of pulmonary epithelial cells, which may contribute to lung function deterioration of COPD patients accompanied with P. aeruginosa and F. nucleatum coinfection.
Introduction
Chronic obstructive pulmonary disease (COPD) is the third leading cause of mortality worldwide, characterized by persistent airflow limitation and respiratory symptoms including dyspnea, cough and/or sputum production (Singh et al., 2019). 54.7% of patients with COPD exacerbations admitted to the hospital are associated with respiratory bacterial infection, (Papi et al., 2006). Pseudomonas aeruginosa, a ubiquitous opportunistic pathogen, is one of the most prevalent bacteria responsible for respiratory infection in COPD patients (Choi et al., 2019). P. aeruginosa is associated with prolonged hospitalization, increased exacerbation rate and poor lone-term prognosis in COPD patients (Garcia-Vidal et al., 2009; Almagro et al., 2012; Eklof et al., 2020).
In 1999, Dr. Scannapieco first proposed that oral microbiota is closely related to respiratory infections (Scannapieco, 1999). The dynamic and polymicrobial dental plaque biofilm has been proved as a reservoir of respiratory pathogens, direct inhalation of the shedding of dental plaque colonized by respiratory pathogens into lung is one of the most possible mechanisms involved in the association between oral microbiota and respiratory diseases (Tan et al., 2014; Sands et al., 2017). Fusobacterium nucleatum, an oral commensal and periodontal pathogen, is ubiquitous in the oral cavity of healthy and diseased individuals. F. nucleatum acts as a coaggregation bridge between early and late colonizing bacteria in the polymicrobial dental plaque biofilm, and affects the composition and architecture of supra- and subgingival biofilm (Thurnheer et al., 2019). Recent studies demonstrate that F. nucleatum colonizes in the respiratory tract, not only leads to respiratory infection in patients with chronic diseases such as tumors and diabetes (Yang et al., 2011; Bailhache et al., 2013; Shamriz et al., 2015), but also causes endobronchial lesion in healthy children (Gedik et al., 2014). Meanwhile, our previous study finds that F. nucleatum and P. aeruginosa frequently coexisted in the respiratory tract of patients with COPD exacerbation, and as the number of F. nucleatum in the respiratory tract microbiota increases, their lung function declines (Li et al., 2020). These results suggest that F. nucleatum and P. aeruginosa may play a synergistic role in respiratory infection of COPD patients, but the mechanism underlying this is still unclear.
The ability to adhere and invade host cells is a virulence property of certain bacteria. Although P. aeruginosa is usually considered an extracellular pathogen, some studies have demonstrated that P. aeruginosa can invade a variety of epithelial cells via an endocytic process dependent on actin microfilaments (Fleiszig et al., 1994; Fleiszig et al., 1995). The invasive P. aeruginosa induces membrane blebs in epithelial cells, which are utilized as a niche for bacterial intracellular replication and motility (Angus et al., 2008). In contrast, F. nucleatum adheres to and invades epithelial cells via a zipping mechanism relying on its outer membrane proteins to bind to the cell-surface receptors (Han et al., 2000). At the same time, F. nucleatum can coaggregate with almost all of the oral bacteria dependent on its outer membrane proteins (Kaplan et al., 2009; Loozen et al., 2014; Coppenhagen-Glazer et al., 2015). F. nucleatum not only enhances the adhesion and invasion of invasive bacteria such as Porphyromonas gingivalis and Aggregatibacter actinomycetemcomitans to tissue cells (Li et al., 2015), but also transports noninvasive bacteria such as Streptococcus cristatus and Streptococcus sanguis into epithelial cells (Edwards et al., 2006). It is still unclear whether F. nucleatum will affect the infection of P. aeruginosa to pulmonary epithelial cells due to interspecies coaggregation in COPD patients accompanied with coinfection of F. nucleatum and P. aeruginosa.
Mucosal epithelium is not only the first physical barrier between host and bacteria, but also an active participant in natural immunity and inflammation (Proud and Leigh, 2011). IL-1β is considered a biomarker of bacteria-associated exacerbation of COPD, and TNF-α and IL-6 are the potential markers of IL-1β-associated exacerbation of COPD (Bafadhel et al., 2011; Damera et al., 2016). Vos et al. found that P. aeruginosa infection of human bronchial epithelial cells can significantly induce the expression of IL-1 family members IL-1β and IL-1F9 (Vos et al., 2005). P. aeruginosa-derived flagellin induces IL-6 and IL-8 production in bronchial epithelial cells through the phosphorylation of p38, ERK and JNK, which partially explains the underlying mechanism of P. aeruginosa causing acute exacerbation of COPD (Nakamoto et al., 2019). Hayata et al. report that F. nucleatum induces the productions of proinflammatory cytokines IL-6 and IL-8 by the bronchial and pharyngeal epithelial cells, which may trigger exacerbation of COPD (Hayata et al., 2019). Therefore, we speculate that coinfection of P. aeruginosa and F. nucleatum may induce cytokine release dysregulation in pulmonary epithelial cells, we plan to establish bacterial simultaneous and sequential infection models to simulate three types of clinical mixed-infection modes in order to fully confirm our scientific hypothesis, and to further reveal the possible mechanism of P. aeruginosa and F. nucleatum coinfection causing lung function decline in COPD patients.
On the basis of the above thinking and hypothesis, the aim of this study was to explore the potential mechanism by which F. nucleatum cooperated with P. aeruginosa to exacerbate COPD. First, we investigated the effect of bacterial coinfection on bacterial adhesion and invasion ability, and the role of bacterial coaggregation in this process. Second, we established bacterial simultaneous and sequential infection models to simulate three types of clinical mixed-infection modes, and detected the effect of the combined infection with P. aeruginosa and F. nucleatum on inflammation and damage of pulmonary epithelial cells.
Materials and Methods
Bacteria Culture
F. nucleatum (ATCC 25586) and P. aeruginosa PAO1 strain (ATCC BAA-47) were obtained from American Type Culture Collection (ATCC). F. nucleatum was grown in tryptic soy broth (TSB. Becton, Dickinson and Company, Sparks, MD, USA) supplemented with 5% defibrinated sheep blood, 10mg/ml hemin and 5mg/ml menadione in anaerobic atmosphere (10% H2, 10% CO2, 80% N2) at 37 °C (Yu et al., 2017; Wu et al., 2018). P. aeruginosa was cultured in TSB under aerobic conditions at 37°C.
Bacterial Aggregation Assay
P. aeruginosa and F. nucleatum were standardized in sterile coaggregation buffer (150 mM NaCl, 1 mM Tris, 0.1 mM CaCl2 and 0.1 mM MgCl2) to give a final cell density of 1×109 colony-forming units per mL (CFUs/mL). Equal numbers of single- or dual-species bacterial cells were suspended and vortexed for 30 s in a reaction tube (T=0 h). The tubes were incubated at room temperature for 1 h to allow aggregation (T=1 h). A visual rating scale of 0-4 was used to grade the reaction. “0” indicates an evenly turbid suspension with no visible aggregates, meaning no coaggregation; “1+” indicates turbid supernatant with finely dispersed coaggregates; “2+” indicates definite coaggregates that do not precipitate immediately; “3+” indicates slightly turbid supernatant with formation of large precipitating coaggregates; and “4+” indicates complete sedimentation with a clear supernatant (Cisar et al., 1979; Shimazu et al., 2016). In addition, a spectrophotometric assay was employed to determine the percentage of bacterial auto- and coaggregation (Cisar et al., 1979; Kaplan et al., 2009). The optical density of bacterial suspensions at 600 nm wavelength (OD600) were measured at the time points of 0 and 1 h. Percentage aggregation was calculated using the following equation: % autoaggregation or coaggregation =(OD600(T0)-OD600(T1))/OD600(T0) ×100. Percentage aggregation were classified as high (more than 40%), intermediate (30-40%) and low aggregation (less than 30%).
Phase Contrast Microscopy
Auto- and coaggregation of the test bacterial species were also studied by phase contrast microscopy. After preparing bacterial suspensions with or without partner species in coaggregation buffer for 1 h as described above, 10 μL from each preparation was mounted on a microscopic glass slide with a coverslip and photographed by phase contrast microscope (Olympus, Japan).
Cell Culture
Human pulmonary epithelial cell line A549 was obtained from ATCC and cultured in Dulbecco’s modified Eagle’s medium (DMEM. HyClone Laboratories, Logan, UT, USA) supplemented with 10% fetal bovine serum (FBS. HyClone Laboratories, Logan, UT, USA), 100 U/mL penicillin and 100 mg/mL streptomycin in a humidified 37°C incubator with 5% CO2.
Bacterial Infection of Pulmonary Epithelial Cells
The in vitro bacterial infection model used in this study was performed as described previously (Pan et al., 2009; Li et al., 2019). Briefly, A549 cells were seeded into 24-well tissue culture plates at a density of 105 cells/well. Cells were inoculated and grown for 24 h to allow the cells to reach confluence. Logarithmic growth phase bacteria were harvested and resuspended to 107 CFUs/mL in DMEM without antibiotics. A549 cells were incubated with F. nucleatum and P. aeruginosa alone or together at multiplicity of infection (MOI, bacteria: epithelial cells) of 10, 50 or 100 in DMEM without antibiotics. For bacterial simultaneous infection model, A549 cells were incubated with both F. nucleatum (MOI 100) and P. aeruginosa (MOI 100) at the same times in DMEM without antibiotics. For bacterial sequential infection model, A549 cells were treated with F. nucleatum (MOI 100) or P. aeruginosa (MOI 100) in DMEM without antibiotics for 12 h, and then added the other bacteria (MOI 100) to continue infection for 12 h. In order to exclude the effect of the increased bacterial load, the single P. aeruginosa or F. nucleatum infection groups at a MOI of 200 were used as controls in the bacterial simultaneous infection assay, and the sequential inoculation of the same bacteria were used as controls in the bacterial sequential infection assay.
Antibiotic Protection Adhesion and Invasion Assay
Assays of bacterial adherence and invasion were performed using methods previously described (Pan et al., 2009). Briefly, bacteria were harvested, washed, and resuspended in DMEM without antibiotics. A549 cells were infected with bacteria in DMEM without antibiotics in the humidified 37°C incubator with 5% CO2 for 3 h, and washed with phosphate-buffered saline (PBS). For determining total adhesion and invasion levels (attachment levels), cells were lysed with sterile distilled water for 30 min, then dilutions of the lysate were plated and cultured anaerobically on TSB agar supplemented with 5% defibrinated sheep blood, 10mg/ml hemin and 5mg/ml menadione to determine CFUs for F. nucleatum, or cultured aerobically on TSB agar to determine CFUs for P. aeruginosa. For invasion assay, extracellular bacteria were killed with 200 μg/mL gentamicin (Sigma, St. Louis, MO, USA) and 300 μg/mL metronidazole (Sigma, St. Louis, MO, USA) for 1 h. Cellular lysate were diluted and cultured to determine CFUs. The number of bacterial attachment or invasion is equal to CFUs divided by the number of cells.
Scanning Electron Microscopy (SEM)
A549 cells were infected with P. aeruginosa (MOI 100) and F. nucleatum (MOI 100) alone or together in DMEM without antibiotics for 3 h, washed with PBS, and fixed with 2.5% glutaraldehyde (BioChemika, Fluka, Switzerland). A549 cells were gradually dehydrated with ethanol and smeared onto copper plates followed by gold sputtering, and images were acquired using SEM (Inspect F50, FEI Company, USA) to observe the bacterial adhesion and cell morphology.
CCK-8 Cell Viability Assay
Cell counting kit (Dojindo Molecular Technologies, Inc., Kumamoto, Japan) was used to assess cell proliferation according to the manufacturer’s manual. Briefly, A549 cells were infected with P. aeruginosa and F. nucleatum alone (MOI 10, 50 or 100) or together (MOI 100) in DMEM without antibiotics for 4, 8, 16 and 24 h. 10 μL of CCK-8 solution was added to 100 μL of medium solution and incubated for 1 h at 37°C. OD450 was measured with a microplate reader (Infinite M200, Tecan, Austria) to reflect the cell proliferation.
Cytotoxicity Assessment by Lactate Dehydrogenase (LDH) Leakage Assay
LDH activity was monitored by the Pierce™ LDH cytotoxicity assay kit (Thermo Scientific, USA) in accordance with the manufacturer’s recommendations. A549 cells were exposed to P. aeruginosa and F. nucleatum alone (MOI 10, 50 or 100) or together (MOI 100) in DMEM without antibiotics for the desired time, the culture supernatant was harvested and centrifuged at 12000 g for 5 min, and 50 μL supernatant was mixed with 50 μL Reaction mixture and incubated at room temperature in the dark for 30 min. 50 μL stop solution was added to stop reaction. OD490 and OD680 were measured with a microplate reader (Infinite M200, Tecan, Austria). LDH activity = OD490-OD680.
Live/Dead Assay by Calcein Acetoxymethyl Ester/Propidium Iodide (Calcein-AM/PI) Staining
The effects of P. aeruginosa and F. nucleatum on the viability or cytotoxicity of pulmonary epithelial cells were evaluated using the Calcein-AM/PI Double Stain Kit (Molecular Probes, Eugene, OR, USA). In brief, A549 cells were exposed to P. aeruginosa and F. nucleatum alone or together in DMEM without antibiotics for the desired time, washed with PBS. Cells were stained with 200 μL Calcein-AM/PI stain working solution at 37°C for 15 min. Living cells (green cytoplasmic fluorescence) and dead cells (red nucleus fluorescence) were immediately observed by fluorescence microscope.
Enzyme-Linked Immunosorbent Assay (ELISA)
A549 cells were exposed to P. aeruginosa and F. nucleatum alone or together in DMEM without antibiotics for the desired time, the culture supernatant was harvested and centrifuged at 12000 g for 5 min. IL-1β, IL-6 and TNF-α levels in the culture supernatant were determined via ELISA kits (Invitrogen, Cambrillo, USA) according to the manufacturer’s protocol. After the procedure, plates were read on the spectrometer at 450 nm wavelength. The results were converted to numeric values using standard curves.
Statistical Analysis
All experiments were performed in triplicate and repeated three times. All values were presented as mean ± standard deviation (SD) and analyzed by analysis of variance followed by multiple comparisons test using GraphPad Prism version 7.00 (GraphPad Software, La Jolla, CA, USA). Differences were considered statistically significant at P<0.05.
Results
The Interaction Between F. nucleatum and P. aeruginosa: F. nucleatum Coaggregates With P. aeruginosa and Adheres to Pulmonary Epithelial Cells
To detect the relationship between P. aeruginosa and F. nucleatum, bacterial aggregation assay was performed. The profiles of microbial aggregation of P. aeruginosa and F. nucleatum were showed in Figures 1A–C. P. aeruginosa displayed a poor autoaggregation with an autoaggregation score of “0” and a low percentage autoaggregation (8.1 ± 3.66%) (Figures 1A–C). F. nucleatum showed a strong autoaggregation with an autoaggregation score of “4+” and a high percentage autoaggregation (72.99 ± 9.43%) (Figures 1A–C). Moreover, F. nucleatum and P. aeruginosa were intermediate coaggregators with an autoaggregation score of “2+” and a percentage autoaggregation of 30.52 ± 7.28% (Figures 1A–C).
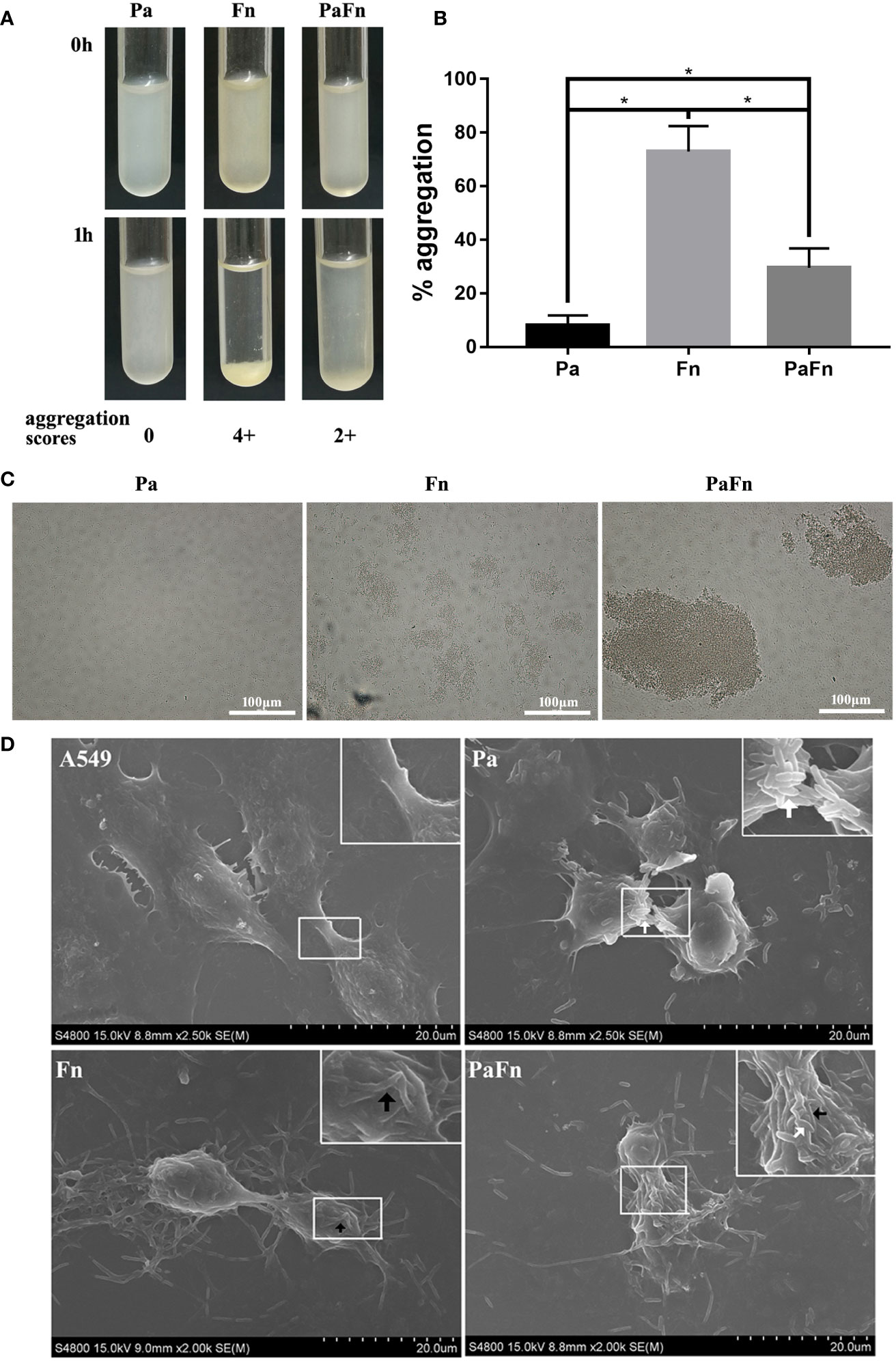
Figure 1 F. nucleatum coaggregates with P. aeruginosa and adheres to pulmonary epithelial cells. (A) Photographs of autoaggregation and coaggregation of P. aeruginosa and F. nucleatum. The aggregation score of each bacterial group was indicated below the photograph. (B) Percentage aggregation of P. aeruginosa and F. nucleatum. *P < 0.05, statistics were achieved by analysis of variance followed by Tukey’s multiple comparisons test. (C) Representative images of auto- and coaggregation of P. aeruginosa and F. nucleatum observed by phase contrast microscope. (D) Representative SEM images of pulmonary epithelial cells infected with P. aeruginosa and F. nucleatum alone or together. A549 cells were infected with P. aeruginosa (MOI 100) and F. nucleatum (MOI 100) alone or together for 3 h. White arrow indicates P. aeruginosa, and black arrow indicates F. nucleatum. Pa, P. aeruginosa; Fn, F. nucleatum.
To explore whether bacterial coaggregation affect the form of bacterial adherence to host cells, bacterial adhesion assay and SEM were performed. Figure 1D demonstrated that P. aeruginosa mainly attached to the cellular junction of pulmonary epithelial cells, which shrunk and became round (Figure 1D). F. nucleatum auto-aggregated to form a bacterial network and attached to the cellular surface of pulmonary epithelial cells, which remained stretched (Figure 1D). When P. aeruginosa and F. nucleatum coinfected pulmonary epithelial cells, F. nucleatum coaggregated with P. aeruginosa and adhered to the cellular junction and surface of pulmonary epithelial cells, which shrunk and became round (Figure 1D).
The Model of Pulmonary Epithelial Cells Simultaneously Infected With P. aeruginosa and F. nucleatum
Coinfection with P. aeruginosa and F. nucleatum Promotes Each Bacterial Invasion of Pulmonary Epithelial Cells
To explore the effect of P. aeruginosa and F. nucleatum coinfection on bacterial attachment and invasion, pulmonary epithelial cells were infected with P. aeruginosa and/or F. nucleatum at different MOI (10, 50, 100). Figures 2A, B demonstrated that both P. aeruginosa and F. nucleatum could adhere to and invade the pulmonary epithelial cells, and as the bacterial MOI value increased, the number of P. aeruginosa and F. nucleatum attaching to and invading pulmonary epithelial cells increased. When the pulmonary epithelial cells were simultaneously infected with P. aeruginosa and F. nucleatum at different MOI (10, 50, 100), F. nucleatum did not affect the attachment number of P. aeruginosa to pulmonary epithelial cells, but F. nucleatum (MOI=100) significantly enhanced the invasion number of P. aeruginosa into pulmonary epithelial cells (Figure 2C). Meanwhile, P. aeruginosa did not affect the attachment number of F. nucleatum to pulmonary epithelial cells, but P. aeruginosa (MOI=100) significantly enhanced the invasion number of F. nucleatum into pulmonary epithelial cells (Figure 2D). In order to prove that interaction between these two bacterial species promotes each bacterial invasion of pulmonary epithelial cells, the single P. aeruginosa or F. nucleatum infection groups at a MOI of 200 were used as controls. Figures 2C, D demonstrated that there was not significantly difference in the number of bacterial attachment and invasion between Pa100 and Pa200 groups, and between Fn100 and Fn200 groups.
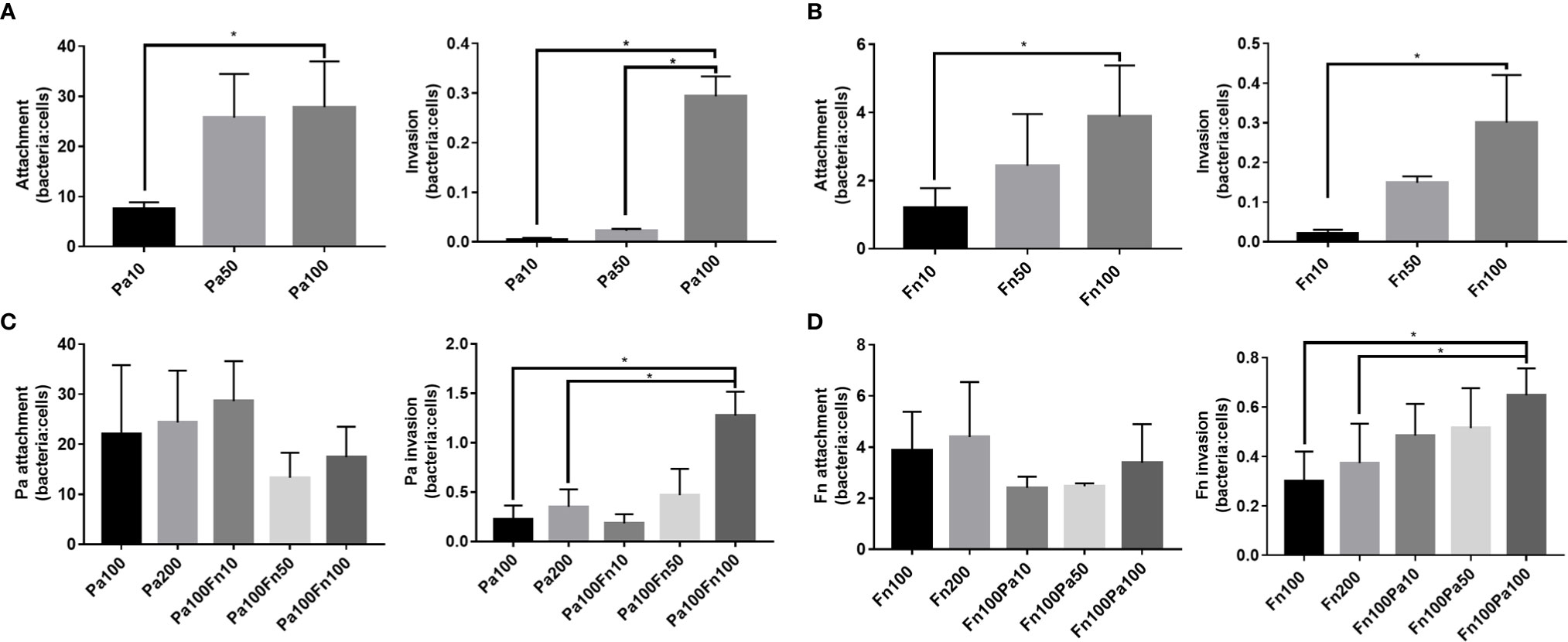
Figure 2 The adherence and invasion of P. aeruginosa and F. nucleatum to pulmonary epithelial cells. (A) The attachment and invasion of P. aeruginosa to pulmonary epithelial cells. A549 cells were infected with different MOI (10, 50, 100) of P. aeruginosa. (B) The attachment and invasion of F. nucleatum to pulmonary epithelial cells. A549 cells were infected with different MOI (10, 50, 100) of F. nucleatum. (C) The effect of F. nucleatum on the attachment and invasion of P. aeruginosa. A549 cells were simultaneously infected with P. aeruginosa (MOI 100) and F. nucleatum (MOI 10, 50, 100), and the single P. aeruginosa infection groups (MOI 100, 200) were used as control. (D) The effect of P. aeruginosa on the attachment and invasion of F. nucleatum. A549 cells were simultaneously infected with F. nucleatum (MOI 100) and P. aeruginosa (MOI 10, 50, 100), and the single F. nucleatum infection groups (MOI 100, 200) were used as control. *P < 0.05, statistics were achieved by analysis of variance followed by Sidak’s multiple comparisons test. Pa, P. aeruginosa; Fn, F. nucleatum.
F. nucleatum Coinfection Transiently Resists P. aeruginosa-Induced Cytotoxicity of Pulmonary Epithelial Cells
To exploit the effect of P. aeruginosa and F. nucleatum coinfection on the biological activity of pulmonary epithelial cells, cell proliferation and cytotoxicity were examined by CCK8 assay and LDH activity assay, respectively. Compared with the control (A549), P. aeruginosa (MOI 10, 50, 100) significantly inhibited the proliferation of pulmonary epithelial cells (Figure 3A). When the MOI of P. aeruginosa were 50 and 100, P. aeruginosa significantly induced cytotoxicity of pulmonary epithelial cells, and the cytotoxicity increased with the extension of infection time (Figure 3A). In contrast, F. nucleatum had no effect on the cytotoxicity of pulmonary epithelial cells, but enhanced the proliferation of pulmonary epithelial cells, which were infected by F. nucleatum at MOI of 100 at 8 h (Figure 3B). However, when pulmonary epithelial cells were simultaneously infected with P. aeruginosa and F. nucleatum, cell proliferation activity was lower than the single F. nucleatum-infected groups but higher than the single P. aeruginosa-infected groups after 12 h (Figure 3C). Meanwhile, the combined infection of P. aeruginosa and F. nucleatum significantly enhanced the cytotoxic response compared to A549 and single F. nucleatum-infected groups, but the cytotoxicity level of the combined infection group was lower than that of the single P. aeruginosa groups after 12 h (Figure 3C). In order to eliminate the effect of the increased bacterial load on cell proliferation and cytotoxicity, the single P. aeruginosa or F. nucleatum infection groups at a MOI of 200 were used as controls. Figure 3C demonstrated there was not significantly difference in cell proliferation and cytotoxicity of pulmonary epithelial cells between Pa100 and Pa200 groups, and between Fn100 and Fn200 groups.
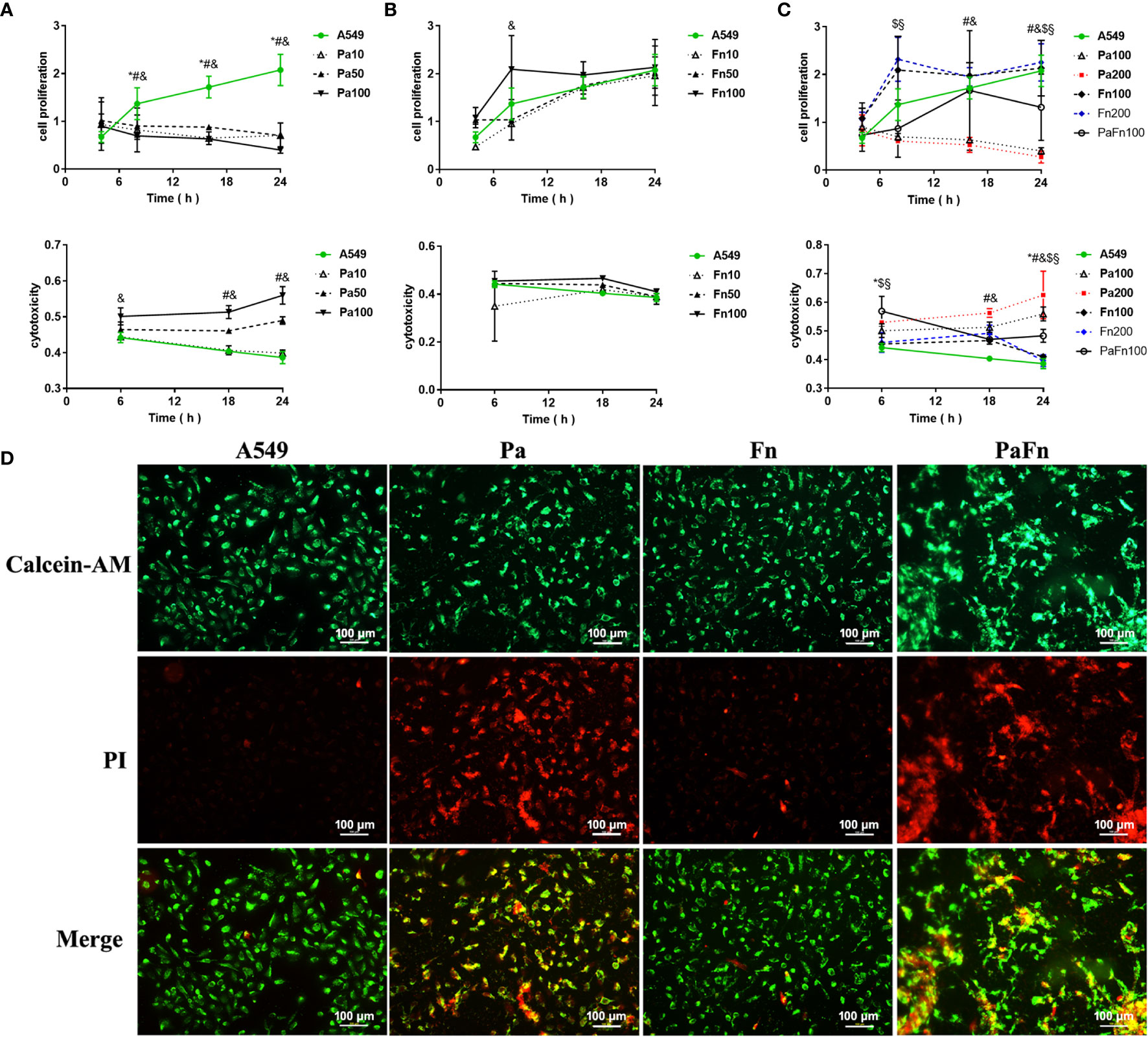
Figure 3 The effect of P. aeruginosa and F. nucleatum on cellular proliferation and cytotoxicity. (A) The effect of P. aeruginosa on cell proliferation and cytotoxicity of pulmonary epithelial cells. A549 cells were infected with different MOI (10, 50, 100) of P. aeruginosa. * Pa10 compared with A549, # Pa50 compared with A549, & Pa100 compared with A549. (B) The effect of F. nucleatum on cell proliferation and cytotoxicity of pulmonary epithelial cells. A549 cells were infected with different MOI (10, 50, 100) of F. nucleatum. * Fn10 compared with A549, # Fn50 compared with A549, & Fn100 compared with A549. (C) The effect of the simultaneous infection of P. aeruginosa and F. nucleatum on cell proliferation and cytotoxicity of pulmonary epithelial cells. A549 cells were simultaneously infected with P. aeruginosa (MOI 100) and F. nucleatum (MOI 100), and the single P. aeruginosa or F. nucleatum infection groups (MOI 100, 200) were used as control. * A549 compared with PaFn100, # Pa100 compared with PaFn100, & Pa200 compared with PaFn100, $ Fn100 compared with PaFn100, § Fn200 compared with PaFn100. */#/&/$/§ P<0.05, statistics were achieved by analysis of variance followed by Dunnett’s multiple comparisons test. (D) Representative fluorescence images of pulmonary epithelial cells infected with P. aeruginosa and F. nucleatum alone or together. A549 cells were infected with P. aeruginosa (MOI 100) and F. nucleatum (MOI 100) alone or together for 24 h. Pa, P. aeruginosa; Fn, F. nucleatum.
In order to observe the cell viability or cytotoxicity intuitively, pulmonary epithelial cells were labeled with Calcein-AM and PI dyes. Figure 3D demonstrated that pulmonary epithelial cells infected with F. nucleatum were mainly live, but the P. aeruginosa alone or combination with F. nucleatum groups were mainly dead cells at 24 h.
P. aeruginosa and F. nucleatum Coinfection Enhances IL-6 and TNF-α Expression in Pulmonary Epithelial Cells Compared With Single P. aeruginosa Infection
To detect the effect of P. aeruginosa and F. nucleatum coinfection on inflammatory response of pulmonary epithelial cells, the expressions of IL-1β, IL-6 and TNF-α were examined by ELISA. Compared with the control group (A549), P. aeruginosa significantly increased the secretion of IL-1β and IL-6 in pulmonary epithelial cells, but there was not significant difference in IL-1β and IL-6 secretion levels between different MOI groups (Figure 4A). P. aeruginosa had no significant effect on TNF-α secretion of pulmonary epithelial cells (Figure 4A). Figure 4B showed that compared with the control group (A549), F. nucleatum (MOI 100) significantly enhanced the secretion of IL-1β, IL-6 and TNF-α, and the secretion levels of IL-1β, IL-6 and TNF-α in F. nucleatum MOI 100 group were higher than those of F. nucleatum MOI 10 and/or MOI 50 groups. Moreover, P. aeruginosa and F. nucleatum coinfection significantly enhanced the secretion of IL-1β, IL-6 and TNF-α in pulmonary epithelial cells compared to A549 group, and the secretion levels of IL-6 and TNF-α in the combined infection group were significantly higher than those of P. aeruginosa alone groups (Figure 4C). In addition, the single P. aeruginosa or F. nucleatum infection groups at a MOI of 200 were also used as controls. Figure 4C demonstrated that there was not significantly difference in the expressions of IL-1β, IL-6 and TNF-α in pulmonary epithelial cells between Pa100 and Pa200 groups, and between Fn100 and Fn200 groups.
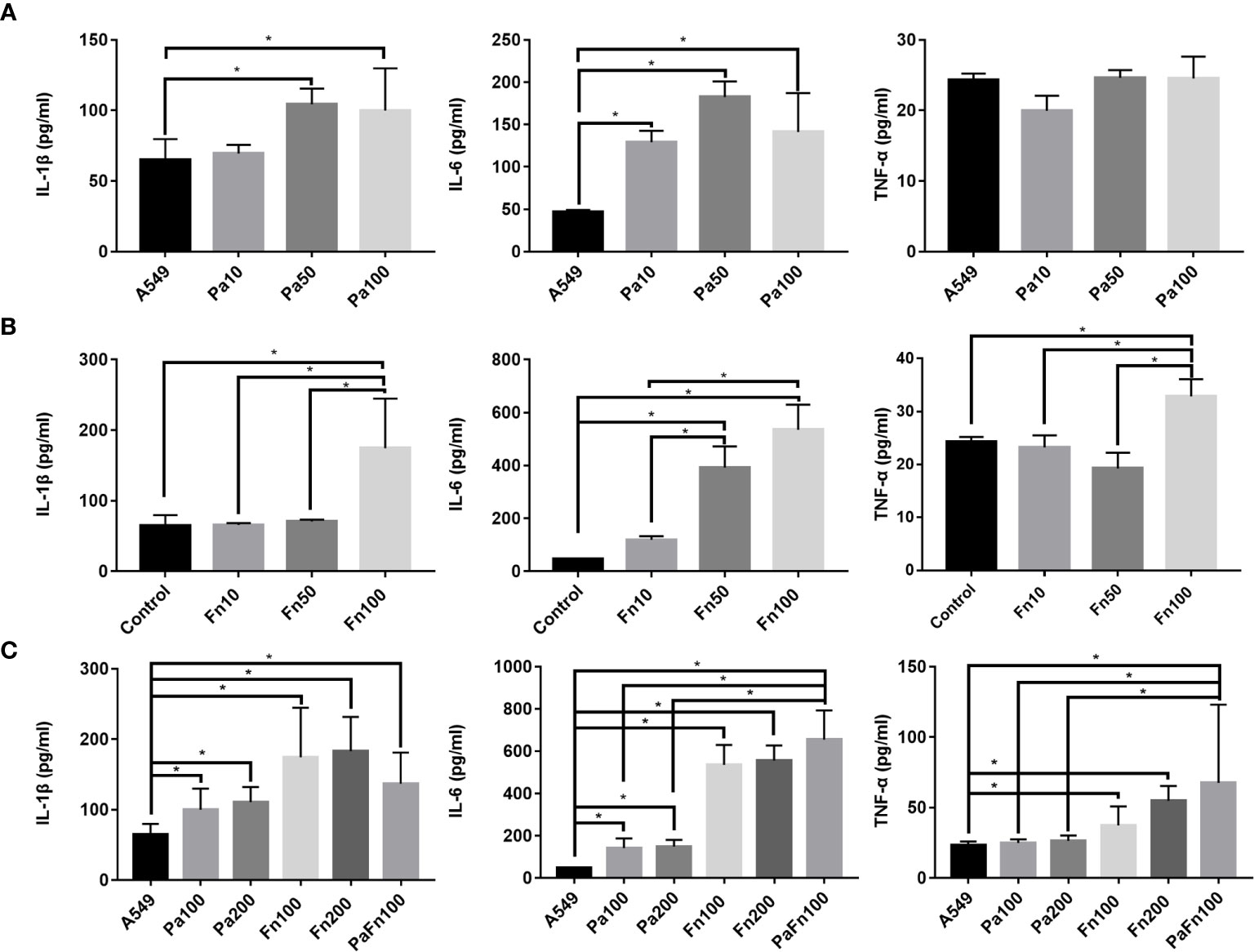
Figure 4 The effect of P. aeruginosa and F. nucleatum on IL-1β, IL-6 and TNF-α productions. (A) The effect of P. aeruginosa on IL-1β, IL-6 and TNF-α productions of pulmonary epithelial cells. A549 cells were infected with different MOI (10, 50, 100) of P. aeruginosa for 24 h. (B) The effect of F. nucleatum on IL-1β, IL-6 and TNF-α productions of pulmonary epithelial cells. A549 cells were infected with different MOI (10, 50, 100) of F. nucleatum for 24 h. (C) The effect of the simultaneous infection of P. aeruginosa and F. nucleatum on IL-1β, IL-6 and TNF-α productions of pulmonary epithelial cells. A549 cells were simultaneously infected with P. aeruginosa (MOI 100) and F. nucleatum (MOI 100) for 24 h, and the single P. aeruginosa or F. nucleatum infection groups (MOI 100, 200) were used as control. *P < 0.05, statistics were achieved by analysis of variance followed by Sidak’s multiple comparisons test. Pa, P. aeruginosa; Fn, F. nucleatum.
The Model of Pulmonary Epithelial Cells Pretreated With P. aeruginosa: F. nucleatum Subsequential Infection Maintains P. aeruginosa-Induced Inflammatory Cytotoxicity of Pulmonary Epithelial Cells
To determine the role of F. nucleatum in the case where respiratory P. aeruginosa infection has already occurred, pulmonary epithelial cells were pretreated with P. aeruginosa followed with F. nucleatum infection (Figure 5A). Figures 5B, C demonstrated that single F. nucleatum infection did not affect the proliferation and cytotoxicity of pulmonary epithelial cell (Fn versus A549), while P. aeruginosa significantly inhibited the proliferation and induced cytotoxicity of pulmonary epithelial cells no matter with or without bacterial subsequential infection (Pa/Pa-Pa/Pa-Fn versus A549, Figures 5B, C). But, either F. nucleatum or P. aeruginosa subsequential infection just maintained the inhibition of cell proliferation and the induction of cytotoxicity caused by P. aeruginosa (Pa-Fn/Pa-Pa versus Pa, Figures 5B, C). In addition, although single F. nucleatum infection significantly induced the secretions of IL-1β, IL-6 and TNF-α in pulmonary epithelial cells (Fn versus A549), P. aeruginosa just upregulated the expressions of IL-1β and IL-6 no matter with or without bacterial subsequential infection (Pa/Pa-Pa/Pa-Fn versus A549, Figure 5D). The expression levels of IL-1β and IL-6 in P. aeruginosa and F. nucleatum sequential infection group were consistent with those of single P. aeruginosa infection group and P. aeruginosa subsequential infection group (Pa-Fn versus Pa, Pa-Fn versus Pa-Pa), but higher than those of single F. nucleatum infection group (Pa-Fn versus Fn, Figure 5D).
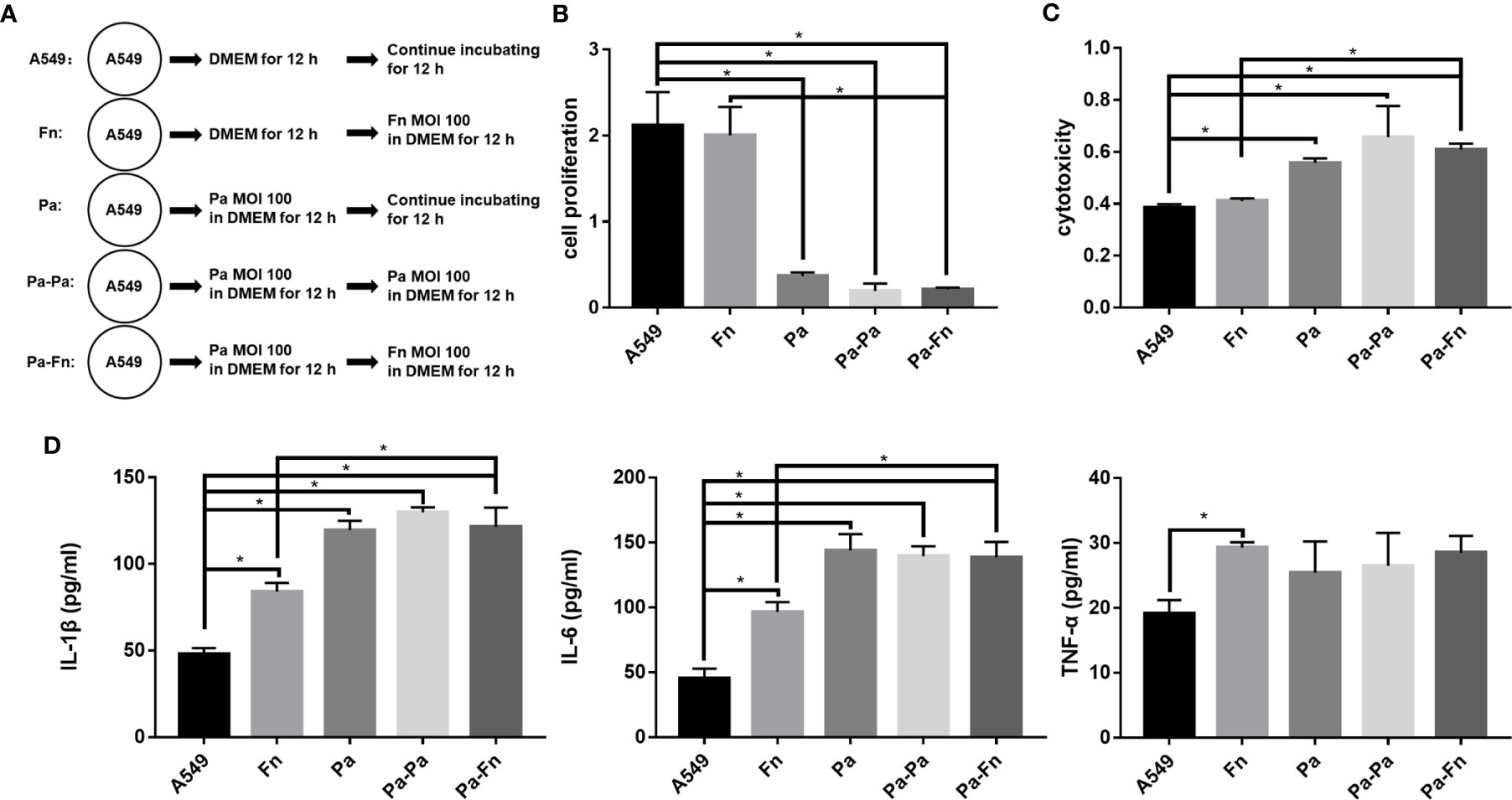
Figure 5 The effect of F. nucleatum subsequential infection on cellular proliferation, cytotoxicity and inflammation. (A) Schematic representation of cell assay. A549 cells cultured in DMEM for 24 h were the blank control. Fn represented A549 cells precultured in DMEM for 12 h followed by F. nucleatum (MOI 100) infection for other 12 h. Pa represented A549 cells treated with P. aeruginosa (MOI 100) for 24 h. Pa-Pa means that A549 cells pretreated with P. aeruginosa (MOI 100) for 12 h continue to be infected with another P. aeruginosa (MOI 100) for 12 h. Pa-Fn means that A549 cells pretreated with P. aeruginosa (MOI 100) for 12 h continue to be infected with F. nucleatum (MOI 100) for 12 h. (B) The effect of F. nucleatum subsequential infection on cellular proliferation of pulmonary epithelial cells. (C) The effect of F. nucleatum subsequential infection on cytotoxicity of pulmonary epithelial cells. (D) The effect of F. nucleatum subsequential infection on IL-1β, IL-6 and TNF-α productions of pulmonary epithelial cells. *P < 0.05, statistics were achieved by analysis of variance followed by Sidak’s multiple comparisons test.
The Model of Pulmonary Epithelial Cells Pretreated With F. nucleatum: F. nucleatum Pretreatment Enlarged TNF-α Associated Cytotoxicity of Pulmonary Epithelial Cells Subsequentially Infected With P. aeruginosa
In order to explore whether the presence of F. nucleatum would affect the progress of respiratory P. aeruginosa infection, pulmonary epithelial cells were pretreated with F. nucleatum followed with P. aeruginosa infection (Figure 6A). Figures 6B, C demonstrated that single F. nucleatum infection did not affect the proliferation and cytotoxicity of pulmonary epithelial cell (Fn versus A549), but P. aeruginosa subsequential infection significantly inhibited the proliferation and induced cytotoxicity of pulmonary epithelial cells, no matter which were pretreated with F. nucleatum or not (Pa versus A549, Fn-Pa versus A549/Fn). In addition, F. nucleatum alone significantly upregulated the secretions of IL-1β, IL-6 and TNF-α (Fn versus A549), while P. aeruginosa alone significantly upregulated the secretions of IL-1β and IL-6 without affecting TNF-α expression (Pa versus A549, Figure 6D). However, P. aeruginosa subsequential infection significantly enhanced the secretions of IL-1β, IL-6 and TNF-α in pulmonary epithelial cells pretreated with F. nucleatum compared with A549 and single P. aeruginosa group (Fn-Pa versus A549/Pa), but only TNF-α secretion in F. nucleatum and P. aeruginosa sequential infection group was also higher than that of F. nucleatum alone group (Fn-Pa versus Fn, Figure 6D). In order to eliminate the effect of the increment in bacterial loads on the sequential infection assay, F. nucleatum subsequential infection group (Fn-Fn) was included. Figures 6B–D demonstrated that there was not significantly difference in cell proliferation, cytotoxicity and the secretions of IL-1β, IL-6 and TNF-α in pulmonary epithelial cell between Fn group and Fn-Fn group.
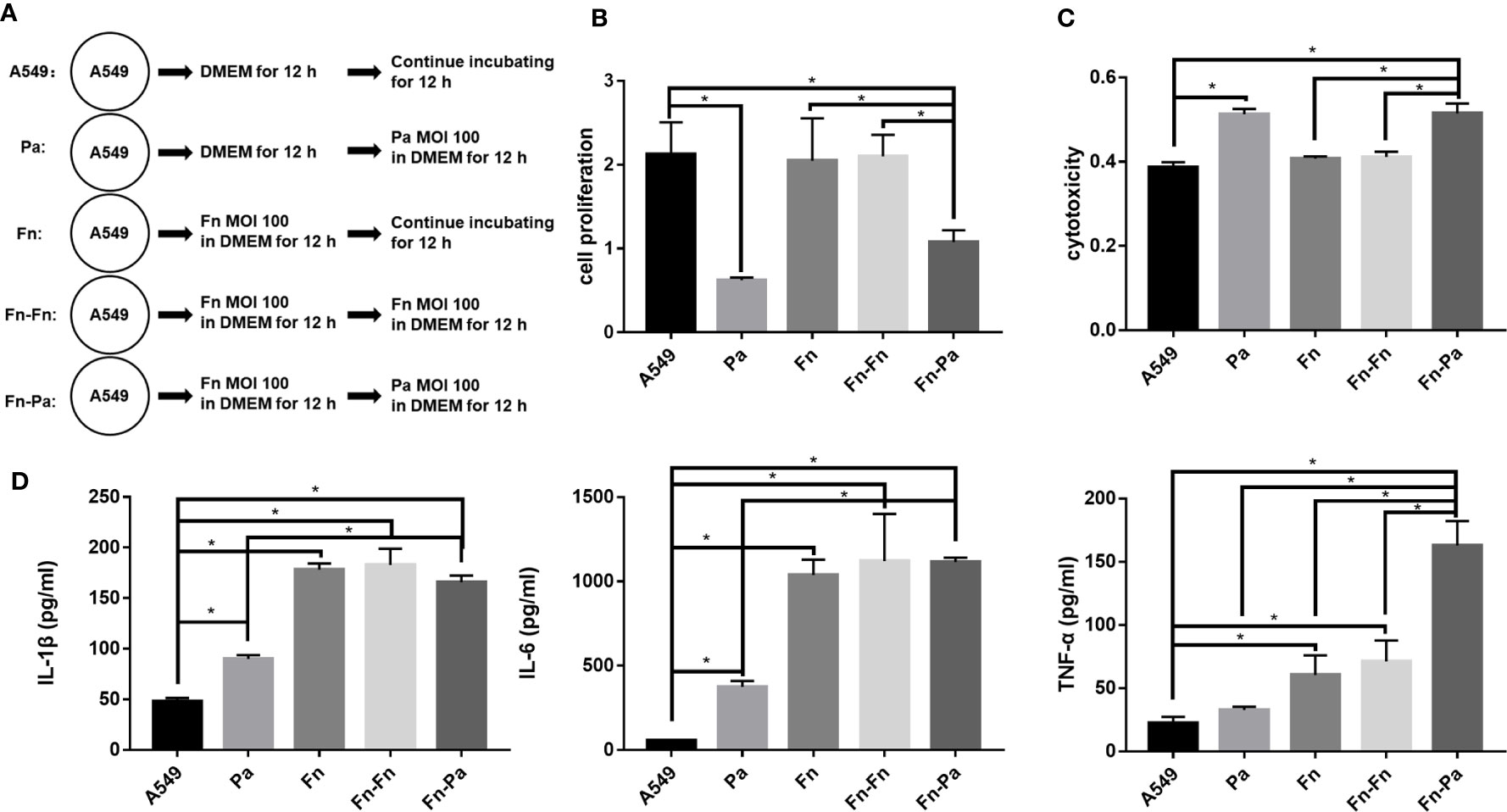
Figure 6 The effect of F. nucleatum pretreatment on cellular proliferation, cytotoxicity and inflammation. (A) Schematic representation of cell assay. A549 cells cultured in DMEM for 24 h were the blank control. Pa represented A549 cells precultured in DMEM for 12 h followed by P. aeruginosa (MOI 100) infection for other 12 h. Fn represented A549 cells treated with F. nucleatum (MOI 100) for 24 h. Fn-Fn means that A549 cells pretreated with F. nucleatum (MOI 100) for 12 h continue to be infected with another F. nucleatum (MOI 100) for 12 h. Fn-Pa means that A549 cells pretreated with F. nucleatum (MOI 100) for 12 h continue to be infected with P. aeruginosa (MOI 100) for 12 h. (B) The effect of F. nucleatum pretreatment on cellular proliferation of pulmonary epithelial cells. (C) The effect of F. nucleatum pretreatment on cytotoxicity of pulmonary epithelial cells. (D) The effect of F. nucleatum pretreatment on IL-1β, IL-6 and TNF-α productions of pulmonary epithelial cells. *P < 0.05, statistics were achieved by analysis of variance followed by Sidak’s multiple comparisons test.
Discussion
Several studies have analyzed oral microbiome and lung microbiome of patients with respiratory infection, and demonstrate that the bacterial composition of dental plaque and lung samples is highly similar, and pathogens isolated from the lung are genetically indistinguishable from strains of the same species isolated from the oral cavity (Heo et al., 2008; Tan et al., 2014). In addition, some studies analyzing the microbiome associated with respiratory infection indicate that F. nucleatum widely exists in the respiratory tract of patients with respiratory diseases such as COPD, empyema and lung abscess (Nagaoka et al., 2017; Dyrhovden et al., 2019; Li et al., 2020), and F. nucleatum is considered as a biomarker of lung function deterioration in COPD patients coinfected with P. aeruginosa (Li et al., 2020). In polymicrobial diseases, there are complex interactions among different species, leading to synergisms in creating clinical symptoms and pathologies. Therefore, this study plans to explore the potential mechanism of F. nucleatum cooperating with P. aeruginosa to weaken lung function of COPD patients from two aspects: interspecies interaction between P. aeruginosa and F. nucleatum, and the interaction of P. aeruginosa and F. nucleatum with pulmonary epithelial cells.
Bacterial autoaggregation and coaggregation were defined as the adherence of bacteria belonging to the same strain and different bacterial strains, respectively, which are essential for the development of multispecies biofilm communities and the infection of host cell (Polak et al., 2012; Karched et al., 2015). This study demonstrated that F. nucleatum, a Gram-negative obligate anaerobe in the oral cavity, was a strong autoaggregator, which is consistent with Karched’s study (Karched et al., 2015). F. nucleatum expresses a variety of surface adhesins, allowing coaggregation with most oral bacteria (Kinder and Holt, 1993; Kaplan et al., 2009; Coppenhagen-Glazer et al., 2015). That may be the reason that P. aeruginosa coaggregates with F. nucleatum moderately, although P. aeruginosa is a poor autoaggregator. On the other hand, the adhesion and invasion of bacteria to host cells is a prerequisite for their virulence and infection. This study found that both P. aeruginosa and F. nucleatum were able to adhere to and invade into pulmonary epithelial cells, which was consistent with the previous studies (Fleiszig et al., 1994; Fleiszig et al., 1995; Han et al., 2000), and their infection number increased as the MOI of bacteria increased. Interestingly, the number of P. aerugionosa attaching to pulmonary epithelial cells at a MOI of 50 was similar to that at a MOI of 100, but the number of P. aerugionosa invading pulmonary epithelial cells at a MOI of 50 was significantly lower than that at a MOI of 100. Pan et al. have explained this phenomenon as “adhesion may not be the sole prerequisite for invasion” (Pan et al., 2009), which may also be the reason why coinfection with P. aeruginosa and F. nucleatum did not affect the adhesion of each other to pulmonary epithelial cells but enhanced each bacterial invasion. In addition, when pulmonary epithelial cells were coinfected with P. aeruginosa and F. nucleatum, the number of P. aeruginosa or F. nucleatum adhering to pulmonary epithelial cells is equal to that of the single P. aeruginosa or F. nucleatum group, respectively. That means that the total number of bacteria attached to the cell surface in the combined infection group is equivalent to the sum of the number of the single species infection groups, which is also the reason that more bacteria to coaggregate and adhere to the cell surface in the combined infection group compared with the single species groups. It has been reported that F. nucleatum not only enhances the adhesion and invasion of invasive bacteria such as P. gingivalis and A. actinomycetemcomitans (Saito et al., 2012; Li et al., 2015), but also transports bacteria with limited adhesion abilities such as S. cristatus and S. sanguis into the oral epithelium via its coaggregation function (Edwards et al., 2006). This study also demonstrated that when the MOI of bacteria was increased from 100 to 200, it did not significantly change the number of bacterial attachment and invasion. Therefore, these results suggest that coinfection with P. aeruginosa and F. nucleatum promotes each bacterial invasion into pulmonary epithelial cells due to coaggregation between two bacterial species, which may allow bacteria to evade host immune defenses, but also make host cells suffer huge damage.
After bacteria entry into host cells, some bacteria can inhibit the apoptosis of host cells to replicate and survive within the cells in order to avoid host immune defense, but certain bacteria directly induce cell death to aggravate tissue damage. Previous studies show that P. aeruginosa can directly act on tissue cells through its type III secretion system or toxic factors such as pyocyanin to induce apoptosis (Broquet and Asehnoune, 2015; Ranjani et al., 2015). This study demonstrated that P. aeruginosa attached to and destroyed the cell junction of pulmonary epithelial cells, significantly inhibited cell proliferation, and induced cytotoxic death of pulmonary epithelial cells. As distinct from P. aeruginosa, F. nucleatum promoted the proliferation of pulmonary epithelial cells without inducing cytotoxicity in the initial stage of infection (8 h). However, the presence of F. nucleatum transiently alleviated the inhibitory effect of P. aeruginosa on cell proliferation and weaken P. aeruginosa-induced cytotoxicity of pulmonary epithelial cells that simultaneously infected with P. aeruginosa and F. nucleatum, but simply increasing the MOI of the same bacteria could not change the effects of this bacteria on cell proliferation and cytotoxicity of pulmonary epithelial cells. Our previous study also demonstrated that periodontal pathogen P. gingivalis could transiently inhibit P. aeruginosa-induced apoptosis of pulmonary epithelial cells though the STAT3 signaling pathway (Li et al., 2014). These results indicate that F. nucleatum promotes P. aeruginosa invasion and transiently resists P. aeruginosa-induced damage of pulmonary epithelial cells, which may be a common characteristic of periodontal anaerobic pathogen to cause chronic persistent infection of P. aeruginosa in the lung of COPD patients.
It is well-known that the acute exacerbation of COPD is closely related to the persistent airway inflammation induced by bacterial infection. The expression of IL-1β in patients with COPD is upregulated, and IL-1β level is correlated with the increase of airway neutrophils and the decline of lung function (Sapey et al., 2009). Airway (sputum) IL-1β is considered to be a biomarker for acute exacerbation of COPD associated with bacteria (Bafadhel et al., 2011). Demera et al. found that the content of P. aeruginosa in the sputum was positively corelated with IL-1β level, and TNF-α and IL-6 are IL-1β signature and associated with IL-1β-mediated COPD exacerbation (Damera et al., 2016). This study showed that both P. aeruginosa and F. nucleatum could significantly induce IL-1β and IL-6 secretion, F. nucleatum also induce TNF-α secretion in pulmonary epithelial cells. In addition, the simultaneous infection with P. aeruginosa and F. nucleatum significantly enhance IL-6 and TNF-α expressions in pulmonary epithelial cells compared with single P. aeruginosa infection group, which were similar to respiratory syncytial virus that enhanced the release of inflammatory factors IL-6 and IL-8 in bronchial epithelial cells coinfected with P. aeruginosa (Bellinghausen et al., 2016). However, there was no statistical difference in the expressions of IL-1β, IL-6 and TNF-α between F. nucleatum group and the combined infection group, which may be due to the fact that coinfection with P. aeruginosa and F. nucleatum significantly induced cytotoxicity death of pulmonary epithelial cells at 24 h. In addition, this study also demonstrated that simply increasing the MOI of the same bacteria could not change the effects of this bacteria on the secretions of IL-1β, IL-6 and TNF-α in pulmonary epithelial cells. Fu et al. pointed out that sputum IL-1β and serum IL-6 were higher in the frequent exacerbators with COPD, which activated the IL-1β-system inflammation axis to increase the risk of frequent exacerbations of COPD patients (Fu et al., 2015). Therefore, these results suggest that the simultaneous infection with F. nucleatum and P. aeruginosa induces over-release of pro-inflammatory cytokines IL-6 and TNF-α in pulmonary epithelial cells, which may contribute to increase the susceptibility of COPD exacerbation.
It is a widely recognized clinical phenomenon that patients with more severe COPD are more likely to have severe periodontal disease (Si et al., 2012; Tan et al., 2019), and a significantly increased risk of COPD occurs among severe periodontitis subjects (Takeuchi et al., 2019). Our previous study finds that 45.3% patients with COPD exacerbation are associated with respiratory coinfection with F. nucleatum and P. aeruginosa (Li et al., 2020), two types of sequential infection model with P. aeruginosa and F. nucleatum are established to fully elaborate the role of mixed infection in COPD. The current study demonstrated that no matter with F. nucleatum pretreatment or subsequential infection, P. aeruginosa significantly induced cytotoxic damage of pulmonary epithelial cells. F. nucleatum pretreatment or subsequential infection kept or even amplified P. aeruginosa-induced cytotoxicity of pulmonary epithelial cells, which may explain why F. nucleatum reduced lung function of COPD patients accompanied with P. aeruginosa infection (Li et al., 2020). On the other hand, F. nucleatum subsequential infection maintained the secretion levels of IL-1β, IL-6 and TNF-α in pulmonary epithelial cells compared to the single P. aeruginosa group, which may be due to the low cellular viability caused by P. aeruginosa pretreatment. Nevertheless, F. nucleatum pretreatment amplified the secretions of IL-1β, IL-6 and TNF-α of pulmonary epithelial cells induced by P. aeruginosa, and only TNF-α secretion of F. nucleatum and P. aeruginosa sequential infection group showed a significant synergistic increase effect compared with the single species groups. In addition, sequential inoculation of the same bacteria did not significant affect the effects of this bacteria on cell proliferation, cytotoxicity and inflammation of pulmonary epithelial cells. These results suggest that F. nucleatum can maintain or even aggravate P. aeruginosa-induced inflammatory cytotoxicity of pulmonary epithelial cells in the sequential infection models, which may be the reason for F. nucleatum cooperating with P. aeruginosa to exacerbate lung injury and induce acute exacerbation of COPD.
In conclusion, considerable research has focused on the interaction between single bacterium and host cells, while this study highlights the effect of bacterial mixed infection on cellular biological function through establishing bacterial simultaneous and sequential infection models, which is summarized in Figure 7. It demonstrated that P. aeruginosa mainly induces IL-1β and IL-6 associated inflammatory cytotoxicity of pulmonary epithelial cells, F. nucleatum mainly initiates high inflammatory proliferation of pulmonary epithelial cells. When pulmonary epithelial cells are simultaneously infected with P. aeruginosa and F. nucleatum, F. nucleatum coaggregates with P. aeruginosa to synergistically invade into pulmonary epithelial cells and transiently resists P. aeruginosa-induced cytotoxic damage, and induces over-release of IL-6 and TNF-α, which may cause a chronic persistent pulmonary infection and increase the susceptibility of COPD exacerbation. In addition, the presence of F. nucleatum maintains or even aggravates P. aeruginosa-induced inflammatory cytotoxicity of pulmonary epithelial cells in the sequential infection models, which may be the reason for F. nucleatum reducing lung function of COPD patients accompanied with P. aeruginosa infection and may contribute to persistent and/or frequent exacerbation of COPD patients accompanied with P. aeruginosa and F. nucleatum coinfection. Therefore, oral anaerobic pathogens, especially F. nucleatum, should never be neglected in the treatment of COPD.
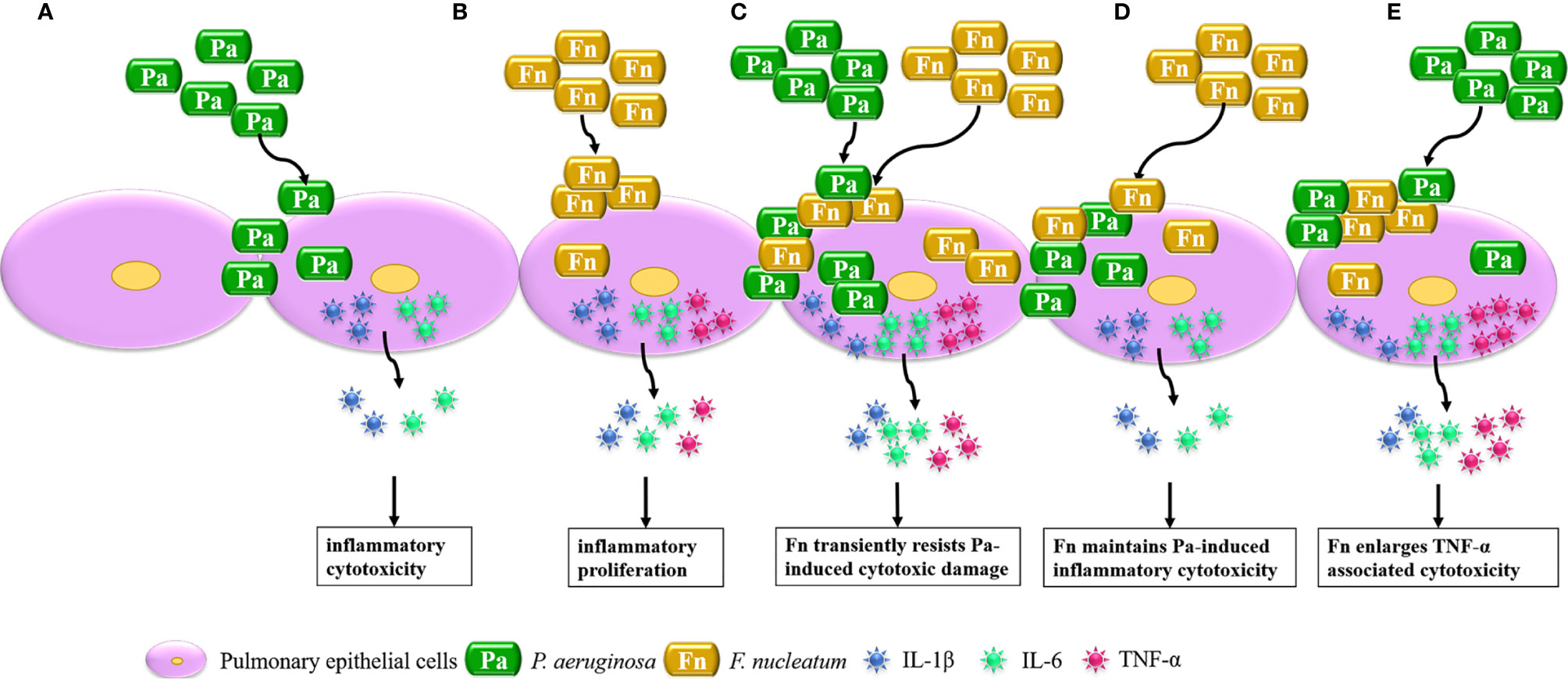
Figure 7 Schematic diagram of the effect of mixed infection with P. aeruginosa and F. nucleatum on pulmonary epithelial cells based on the present model. (A) When pulmonary epithelial cells are infected with P. aeruginosa alone, P. aeruginosa induces IL-1β and IL-6 associated inflammatory cytotoxicity of pulmonary epithelial cells. (B) When pulmonary epithelial cells are infected with F. nucleatum alone, F. nucleatum initiates high inflammatory proliferation of pulmonary epithelial cells. (C) When pulmonary epithelial cells are simultaneously infected with P. aeruginosa and F. nucleatum, F. nucleatum coaggregates with P. aeruginosa to synergistically invade into pulmonary epithelial cells and transiently resists P. aeruginosa-induced cytotoxic damage to induce over-release of IL-6 and TNF-α. (D) When pulmonary epithelial cells are pretreated with P. aeruginosa, F. nucleatum subsequential infection maintains P. aeruginosa-induced inflammatory cytotoxicity. (E) When pulmonary epithelial cells are pretreated with F. nucleatum, F. nucleatum pretreatment enlarged TNF-α associated cytotoxicity of pulmonary epithelial cells subsequentially infected with P. aeruginosa.
Data Availability Statement
The original contributions presented in the study are included in the article/supplementary material. Further inquiries can be directed to the corresponding author.
Author Contributions
All authors contributed to the article and approved the submitted version. QL designed and performed the experiments, analyzed the data, wrote and revised the draft of the paper. HW, LT, SZ, LL and XT participated in the experiments, analyzed the data and revised the article. YP developed the idea for this study and revised the manuscript.
Funding
This work was supported by the National Natural Science Foundation of China (NO. 81670997 to YP), the Plan of the talents for Liaoning Development (NO. XLYC1802129 to YP) and the Higher Education Fundamental Research Project of Liaoning Province (NO. LQNK201724 to QL).
Conflict of Interest
The authors declare that the research was conducted in the absence of any commercial or financial relationships that could be construed as a potential conflict of interest.
References
Almagro P., Salvado M., Garcia-Vidal C., Rodriguez-Carballeira M., Cuchi E., Torres J., et al. (2012). Pseudomonas aeruginosa and mortality after hospital admission for chronic obstructive pulmonary disease. Respiration 84 (1), 36–43. doi: 10.1159/000331224
Angus A. A., Lee A. A., Augustin D. K., Lee E. J., Evans D. J., Fleiszig S. M. (2008). Pseudomonas aeruginosa induces membrane blebs in epithelial cells, which are utilized as a niche for intracellular replication and motility. Infect. Immun. 76 (5), 1992–2001. doi: 10.1128/IAI.01221-07
Bafadhel M., McKenna S., Terry S., Mistry V., Reid C., Haldar P., et al. (2011). Acute exacerbations of chronic obstructive pulmonary disease: identification of biologic clusters and their biomarkers. Am. J. Respir. Crit. Care Med. 184 (6), 662–671. doi: 10.1164/rccm.201104-0597OC
Bailhache M., Mariani-Kurkdjian P., Lehours P., Sarlangue J., Pillet P., Bingen E., et al. (2013). Fusobacterium invasive infections in children: a retrospective study in two French tertiary care centres. Eur. J. Clin. Microbiol. Infect. Dis. 32 (8), 1041–1047. doi: 10.1007/s10096-013-1848-2
Bellinghausen C., Gulraiz F., Heinzmann A. C., Dentener M. A., Savelkoul P. H., Wouters E. F., et al. (2016). Exposure to common respiratory bacteria alters the airway epithelial response to subsequent viral infection. Respir. Res. 17 (1), 68. doi: 10.1186/s12931-016-0382-z
Broquet A., Asehnoune K. (2015). Apoptosis induced by Pseudomonas aeruginosa: a lonely killer? Microb. Biotechnol. 8 (1), 49–51. doi: 10.1111/1751-7915.12144
Choi J., Oh J. Y., Lee Y. S., Hur G. Y., Lee S. Y., Shim J. J., et al. (2019). Bacterial and viral identification rate in acute exacerbation of chronic obstructive pulmonary disease in Korea. Yonsei Med. J. 60 (2), 216–222. doi: 10.3349/ymj.2019.60.2.216
Cisar J. O., Kolenbrander P. E., McIntire F. C. (1979). Specificity of coaggregation reactions between human oral streptococci and strains of Actinomyces viscosus or Actinomyces naeslundii. Infect. Immun. 24 (3), 742–752. doi: 10.1128/IAI.24.3.742-752.1979
Coppenhagen-Glazer S., Sol A., Abed J., Naor R., Zhang X., Han Y. W., et al. (2015). Fap2 of Fusobacterium nucleatum is a galactose-inhibitable adhesin involved in coaggregation, cell adhesion, and preterm birth. Infect. Immun. 83 (3), 1104–1113. doi: 10.1128/IAI.02838-14
Damera G., Pham T. H., Zhang J., Ward C. K., Newbold P., Ranade K., et al. (2016). A sputum proteomic signature that associates with increased IL-1beta levels and bacterial exacerbations of COPD. Lung 194 (3), 363–369. doi: 10.1007/s00408-016-9877-0
Dyrhovden R., Nygaard R. M., Patel R., Ulvestad E., Kommedal O. (2019). The bacterial aetiology of pleural empyema. A descriptive and comparative metagenomic study. Clin. Microbiol. Infect. 25 (8), 981–986. doi: 10.1016/j.cmi.2018.11.030
Edwards A. M., Grossman T. J., Rudney J. D. (2006). Fusobacterium nucleatum transports noninvasive Streptococcus cristatus into human epithelial cells. Infect. Immun. 74 (1), 654–662. doi: 10.1128/IAI.74.1.654-662.2006
Eklof J., Sorensen R., Ingebrigtsen T. S., Sivapalan P., Achir I., Boel J. B., et al. (2020). Pseudomonas aeruginosa and risk of death and exacerbations in patients with chronic obstructive pulmonary disease: an observational cohort study of 22 053 patients. Clin. Microbiol. Infect. 26 (2), 227–234. doi: 10.1016/j.cmi.2019.06.011
Fleiszig S. M., Zaidi T. S., Fletcher E. L., Preston M. J., Pier G. B. (1994). Pseudomonas aeruginosa invades corneal epithelial cells during experimental infection. Infect. Immun. 62 (8), 3485–3493. doi: 10.1128/IAI.62.8.3485-3493.1994
Fleiszig S. M., Zaidi T. S., Pier G. B. (1995). Pseudomonas aeruginosa invasion of and multiplication within corneal epithelial cells in vitro. Infect. Immun. 63 (10), 4072–4077. doi: 10.1128/IAI.63.10.4072-4077.1995
Fu J. J., McDonald V. M., Baines K. J., Gibson P. G. (2015). Airway IL-1beta and systemic inflammation as predictors of future exacerbation risk in asthma and COPD. Chest 148 (3), 618–629. doi: 10.1378/chest.14-2337
Garcia-Vidal C., Almagro P., Romani V., Rodriguez-Carballeira M., Cuchi E., Canales L., et al. (2009). Pseudomonas aeruginosa in patients hospitalised for COPD exacerbation: a prospective study. Eur. Respir. J. 34 (5), 1072–1078. doi: 10.1183/09031936.00003309
Gedik A. H., Cakir E., Soysal O., Umutoglu T. (2014). Endobronchial lesion due to pulmonary Fusobacterium nucleatum infection in a child. Pediatr. Pulmonol. 49 (3), E63–E65. doi: 10.1002/ppul.22834
Han Y. W., Shi W., Huang G. T., Kinder Haake S., Park N. H., Kuramitsu H., et al. (2000). Interactions between periodontal bacteria and human oral epithelial cells: Fusobacterium nucleatum adheres to and invades epithelial cells. Infect. Immun. 68 (6), 3140–3146. doi: 10.1128/iai.68.6.3140-3146.2000
Hayata M., Watanabe N., Tamura M., Kamio N., Tanaka H., Nodomi K., et al. (2019). The periodontopathic bacterium Fusobacterium nucleatum induced proinflammatory cytokine production by human respiratory epithelial cell lines and in the lower respiratory organs in mice. Cell Physiol. Biochem. 53 (1), 49–61. doi: 10.33594/000000120
Heo S. M., Haase E. M., Lesse A. J., Gill S. R., Scannapieco F. A. (2008). Genetic relationships between respiratory pathogens isolated from dental plaque and bronchoalveolar lavage fluid from patients in the intensive care unit undergoing mechanical ventilation. Clin. Infect. Dis. 47 (12), 1562–1570. doi: 10.1086/593193
Kaplan C. W., Lux R., Haake S. K., Shi W. (2009). The Fusobacterium nucleatum outer membrane protein RadD is an arginine-inhibitable adhesin required for inter-species adherence and the structured architecture of multispecies biofilm. Mol. Microbiol. 71 (1), 35–47. doi: 10.1111/j.1365-2958.2008.06503.x
Karched M., Bhardwaj R. G., Asikainen S. E. (2015). Coaggregation and biofilm growth of Granulicatella spp. with Fusobacterium nucleatum and Aggregatibacter actinomycetemcomitans. BMC Microbiol. 15, 114. doi: 10.1186/s12866-015-0439-z
Kinder S. A., Holt S. C. (1993). Localization of the Fusobacterium nucleatum T18 adhesin activity mediating coaggregation with Porphyromonas gingivalis T22. J. Bacteriol. 175 (3), 840–850. doi: 10.1128/JB.175.3.840-850.1993
Li Q., Pan C., Teng D., Lin L., Kou Y., Haase E. M., et al. (2014). Porphyromonas gingivalis modulates Pseudomonas aeruginosa-induced apoptosis of respiratory epithelial cells through the STAT3 signaling pathway. Microbes Infect. 16 (1), 17–27. doi: 10.1016/j.micinf.2013.10.006
Li Y., Guo H., Wang X., Lu Y., Yang C., Yang P. (2015). Coinfection with Fusobacterium nucleatum can enhance the attachment and invasion of Porphyromonas gingivalis or Aggregatibacter actinomycetemcomitans to human gingival epithelial cells. Arch. Oral. Biol. 60 (9), 1387–1393. doi: 10.1016/j.archoralbio.2015.06.017
Li Q., Zhou J., Lin L., Zhao H., Miao L., Pan Y. (2019). Porphyromonas gingivalis degrades integrin beta1 and induces AIF-mediated apoptosis of epithelial cells. Infect. Dis. (Lond) 51 (11-12), 793–801. doi: 10.1080/23744235.2019.1653490
Li Q., Tan L., Wang H., Kou Y., Shi X., Zhang S., et al. (2020). Fusobacterium nucleatum interaction with Pseudomonas aeruginosa induces biofilm-associated antibiotic tolerance via Fusobacterium adhesin A. ACS Infect. Dis. 6 (7), 1686–1696. doi: 10.1021/acsinfecdis.9b00402
Loozen G., Ozcelik O., Boon N., De Mol A., Schoen C., Quirynen M., et al. (2014). Inter-bacterial correlations in subgingival biofilms: a large-scale survey. J. Clin. Periodontol. 41 (1), 1–10. doi: 10.1111/jcpe.12167
Nagaoka K., Yanagihara K., Morinaga Y., Kohno S. (2017). Detection of Fusobacterium nucleatum in two cases of empyema and lung abscess using paromomycin-vancomycin supplemented Brucella HK agar. Anaerobe 43, 99–101. doi: 10.1016/j.anaerobe.2016.12.011
Nakamoto K., Watanabe M., Sada M., Inui T., Nakamura M., Honda K., et al. (2019). Pseudomonas aeruginosa-derived flagellin stimulates IL-6 and IL-8 production in human bronchial epithelial cells: A potential mechanism for progression and exacerbation of COPD. Exp. Lung Res. 45 (8), 255–266. doi: 10.1080/01902148.2019.1665147
Pan Y., Teng D., Burke A. C., Haase E. M., Scannapieco F. A. (2009). Oral bacteria modulate invasion and induction of apoptosis in HEp-2 cells by Pseudomonas aeruginosa. Microb. Pathog. 46 (2), 73–79. doi: 10.1016/j.micpath.2008.10.012
Papi A., Bellettato C. M., Braccioni F., Romagnoli M., Casolari P., Caramori G., et al. (2006). Infections and airway inflammation in chronic obstructive pulmonary disease severe exacerbations. Am. J. Respir. Crit. Care Med. 173 (10), 1114–1121. doi: 10.1164/rccm.200506-859OC
Polak D., Shapira L., Weiss E. I., Houri-Haddad Y. (2012). The role of coaggregation between Porphyromonas gingivalis and Fusobacterium nucleatum on the host response to mixed infection. J. Clin. Periodontol. 39 (7), 617–625. doi: 10.1111/j.1600-051X.2012.01889.x
Proud D., Leigh R. (2011). Epithelial cells and airway diseases. Immunol. Rev. 242 (1), 186–204. doi: 10.1111/j.1600-065X.2011.01033.x
Ranjani J., Pushpanathan M., Mahesh A., Niraimathi M., Gunasekaran P., Rajendhran J. (2015). Pseudomonas aeruginosa PAO1 induces distinct cell death mechanisms in H9C2 cells and its differentiated form. J. Basic Microbiol. 55 (10), 1191–1202. doi: 10.1002/jobm.201500037
Saito A., Kokubu E., Inagaki S., Imamura K., Kita D., Lamont R. J., et al. (2012). Porphyromonas gingivalis entry into gingival epithelial cells modulated by Fusobacterium nucleatum is dependent on lipid rafts. Microb. Pathog. 53 (5-6), 234–242. doi: 10.1016/j.micpath.2012.08.005
Sands K. M., Wilson M. J., Lewis M. A. O., Wise M. P., Palmer N., Hayes A. J., et al. (2017). Respiratory pathogen colonization of dental plaque, the lower airways, and endotracheal tube biofilms during mechanical ventilation. J. Crit. Care 37, 30–37. doi: 10.1016/j.jcrc.2016.07.019
Sapey E., Ahmad A., Bayley D., Newbold P., Snell N., Rugman P., et al. (2009). Imbalances between interleukin-1 and tumor necrosis factor agonists and antagonists in stable COPD. J. Clin. Immunol. 29 (4), 508–516. doi: 10.1007/s10875-009-9286-8
Scannapieco F. A. (1999). Role of oral bacteria in respiratory infection. J. Periodontol. 70 (7), 793–802. doi: 10.1902/jop.1999.70.7.793
Shamriz O., Engelhard D., Temper V., Revel-Vilk S., Benenson S., Brooks R., et al. (2015). Infections caused by Fusobacterium in children: a 14-year single-center experience. Infection 43 (6), 663–670. doi: 10.1007/s15010-015-0782-x
Shimazu K., Oguchi R., Takahashi Y., Konishi K., Karibe H. (2016). Effects of surface reaction-type pre-reacted glass ionomer on oral biofilm formation of Streptococcus gordonii. Odontology 104 (3), 310–317. doi: 10.1007/s10266-015-0217-2
Si Y., Fan H., Song Y., Zhou X., Zhang J., Wang Z. (2012). Association between periodontitis and chronic obstructive pulmonary disease in a Chinese population. J. Periodontol. 83 (10), 1288–1296. doi: 10.1902/jop.2012.110472
Singh D., Agusti A., Anzueto A., Barnes P. J., Bourbeau J., Celli B. R., et al. (2019). Global Strategy for the diagnosis, management, and prevention of chronic obstructive lung disease: the GOLD science committee report 2019. Eur. Respir. J. 53 (5), 1900164. doi: 10.1183/13993003.00164-2019
Takeuchi K., Matsumoto K., Furuta M., Fukuyama S., Takeshita T., Ogata H., et al. (2019). Periodontitis is associated with chronic obstructive pulmonary disease. J. Dent. Res. 98 (5), 534–540. doi: 10.1177/0022034519833630
Tan L., Wang H., Li C., Pan Y. (2014). 16S rDNA-based metagenomic analysis of dental plaque and lung bacteria in patients with severe acute exacerbations of chronic obstructive pulmonary disease. J. Periodontal Res. 49 (6), 760–769. doi: 10.1111/jre.12159
Tan L., Tang X., Pan C., Wang H., Pan Y. (2019). Relationship among clinical periodontal, microbiologic parameters and lung function in participants with chronic obstructive pulmonary disease. J. Periodontol. 90 (2), 134–140. doi: 10.1002/JPER.17-0705
Thurnheer T., Karygianni L., Flury M., Belibasakis G. N. (2019). Fusobacterium Species and Subspecies Differentially Affect the Composition and Architecture of Supra- and Subgingival Biofilms Models. Front. Microbiol. 10:1716:1716. doi: 10.3389/fmicb.2019.01716
Vos J. B., van Sterkenburg M. A., Rabe K. F., Schalkwijk J., Hiemstra P. S., Datson N. A. (2005). Transcriptional response of bronchial epithelial cells to Pseudomonas aeruginosa: identification of early mediators of host defense. Physiol. Genomics 21 (3), 324–336. doi: 10.1152/physiolgenomics.00289.2004
Wu C., Al Mamun A. A. M., Luong T. T., Hu B., Gu J., Lee J. H., et al. (2018). Forward Genetic Dissection of Biofilm Development by Fusobacterium nucleatum: Novel Functions of Cell Division Proteins FtsX and EnvC. MBio 9 (2), e00360–18. doi: 10.1128/mBio.00360-18
Yang C. C., Ye J. J., Hsu P. C., Chang H. J., Cheng C. W., Leu H. S., et al. (2011). Characteristics and outcomes of Fusobacterium nucleatum bacteremia–a 6-year experience at a tertiary care hospital in northern Taiwan. Diagn. Microbiol. Infect. Dis. 70 (2), 167–174. doi: 10.1016/j.diagmicrobio.2010.12.017
Keywords: Pseudomonas aeruginosa, Fusobacterium nucleatum, chronic obstructive pulmonary disease, bacterial coaggregation, pulmonary epithelial cells, inflammatory cytotoxicity
Citation: Li Q, Wang H, Tan L, Zhang S, Lin L, Tang X and Pan Y (2021) Oral Pathogen Fusobacterium nucleatum Coaggregates With Pseudomonas aeruginosa to Modulate the Inflammatory Cytotoxicity of Pulmonary Epithelial Cells. Front. Cell. Infect. Microbiol. 11:643913. doi: 10.3389/fcimb.2021.643913
Received: 19 December 2020; Accepted: 02 March 2021;
Published: 19 March 2021.
Edited by:
Loreto Abusleme, University of Chile, ChileCopyright © 2021 Li, Wang, Tan, Zhang, Lin, Tang and Pan. This is an open-access article distributed under the terms of the Creative Commons Attribution License (CC BY). The use, distribution or reproduction in other forums is permitted, provided the original author(s) and the copyright owner(s) are credited and that the original publication in this journal is cited, in accordance with accepted academic practice. No use, distribution or reproduction is permitted which does not comply with these terms.
*Correspondence: Yaping Pan, eXBwYW5AY211LmVkdS5jbg==