- 1The College of Life Sciences and Medicine, Northwest University, Xi’an, China
- 2Department of Neurology, Department of Medical Research Center, Xi’an Key Laboratory of Cardiovascular and Cerebrovascular Diseases, Xi’an NO.3 Hospital, The Affiliated Hospital of Northwest University, Xi’an, China
- 3Department of Spine Surgery, Honghui Hospital, The Affiliated Hospital of Xi’an Jiaotong University, Xi’an, China
Objective: Tuberculosis infection of the Central Nervous System can cause severe inflammation in microglia, and NLRP3 inflammasome is also an important source of inflammation in microglia. Therefore, in this study, we used a co-culture model of rat microglia and tuberculosis H37Ra strain to explore the influence of tuberculosis infection on the NLRP3 inflammasome in microglia and its regulation mechanism.
Methods: We cultured primary microglia from SD rats and co-cultured with tuberculosis H37Ra strain for 4 hours to establish a co-culture model. At the same time, MCC950, Z-YVAD-FMK, BAY-11-7082, Dexamethasone, RU486, BzATP, BBG and extracellular high potassium environment were used to intervene the co-cultivation process. Subsequently, western blot, real-time PCR, ELISA and other methods were used to detect the changes of NLRP3 inflammasome-related molecules in microglia.
Results: After co-cultivation, the NLRP3 inflammasomes in microglia were activated and released a large amount of IL-18 and IL-1β. By regulating NLRP3 inflammasome complex, caspase-1, NF-κB and P2X7R during the co-culture process, it could effectively reduce the release of IL-18 and IL-1β, and the mortality of microglia.
Conclusion: Our results indicate that the NLRP3 inflammasome pathway is an important part of the inflammatory response of microglia caused by tuberculosis infection. By intervening the NLRP3 inflammasome pathway, it can significantly reduce the inflammatory response and mortality of microglia during the tuberculosis H37Ra strain infection. This research can help us further understand the inflammatory response mechanism of the central nervous system during tuberculosis infection and improve its treatment.
Introduction
Central Nervous System (CNS) tuberculosis infection is a very serious disease caused by Mycobacterium tuberculosis (Mtb), with a fatality rate of 20% and a disability rate of 50% (Hernandez Pando, 2011). In the CNS, microglia are one of the immune cells against Mtb (Rock et al., 2004). In this process, microglia produce a large number of inflammatory factors (Rock et al., 2004). And excessive inflammation usually leads to a poor prognosis (Hernandez Pando, 2011). Therefore, studying the mechanism of excessive inflammation caused by microglia and its intervention factors in the process of Mtb infection in the central nervous system is of great significance for improving the treatment and prognosis of the disease.
Microglia are the most important myeloid cells in healthy brain parenchyma, and they play many important functions such as homeostatic monitoring and immune defense (Wolf et al., 2017). Microglia originate from primitive macrophages, then leave the yolk sac and colonize in the neuroepithelium, and become resident macrophages in CNS (Prinz et al., 2017). Therefore, the properties and functions of microglia and macrophages are very similar (Prinz et al., 2017). Previous studies indicate that the baseline level of NLRP3 inflammasomes in macrophages is very low, but after Mtb infection, the NLRP3 inflammasomes in macrophages are activated, meanwhile, the secretion of various inflammatory cytokines such as IL-18 and IL-1β increase significantly (Song and Li, 2018). In addition, when a variety of pathogens infect the CNS, the activation of NLRP3 inflammasomes also occurs in microglia (Dong et al., 2020; Ma et al., 2020; Wang S et al., 2020). Therefore, we speculated that when Mtb infects the CNS, the NLRP3 inflammasome in microglia might also be activated, and cause the microglia to secrete a variety of inflammatory factors.
NLRP3 inflammasome is a typical inflammasome that can respond to various danger signals inside and outside the cell, it represents a special cell death process called pyroptosis, which can occur in a variety of infections and inflammatory diseases (Wang Z et al., 2020). Similarly, the involvement of NLRP3 inflammasome can also be observed in various CNS diseases such as infection, ischemia, and degeneration (Marim et al., 2017; Heneka et al., 2018; Katuri et al., 2019; Xue et al., 2019; Cui et al., 2020; Ji et al., 2020; Ma et al., 2020; Zeng et al., 2020). The activation of NLRP3 inflammasome is a two-stage process. The first stage (signal 1) is the sensing and generation stage, which starts with the recognition of PAMP and DAMP by TLR. At this stage, TLR recognizes various stimulus signals and factors and activates the NF-κB signaling pathway, leading to an increase in the transcription and translation of a variety of precursor proteins (Shao et al., 2019). The second stage (signal 2) is the assembly and effector stage, which starts with the assembly of the NLRP3 inflammasome. NLRP3, ASC and pro-caspase-1 assemble into a mature inflammasome complex, and convert pro-caspase-1 into caspase-1, then caspase-1 convert pro-IL-1β and pro-IL-18 into their mature forms (Swanson et al., 2019). IL-1β and IL-18 can cause subsequent inflammation. The regulation of cell pyroptosis mainly focuses on signal 1 and signal 2 pathways. For example, the NF-κB in signal 1 is an important nuclear transcription factor. Interfering with the phosphorylation level of NF-κB upstream molecules can affect the transfer of NF-κB to the nucleus, thereby affecting the transcription of many molecules in the nucleus (Irrera et al., 2017). The P2X7 receptor (P2X7R) in signal 2 is a kind of non-specific positive transcription in the nucleus, it is widely distributed in microglia and other cells (Su et al., 2018), belonging to the P2X subfamily of purinergic P2 receptor ligand-gated ion channels. It can be stimulated by a large amount of extracellular ATP released by dying cells, or activated by outflow of large amounts of potassium ions, thereby triggering various signal cascades and promoting the assembly of NLRP3 inflammasome complex and the expression of related molecules (Karmakar et al., 2016). Recently, researchers have also found a protein called Gasdermin D, which is located downstream of caspase-1 and is called the executor of pyroptosis. It is necessary for the secretion of IL-1β (He et al., 2015).
Therefore, in this experiment, we used a co-culture model of rat primary microglia and Mtb H37Ra strain to simulate CNS Mtb infection. In this model, we studied whether there is activation of NLRP3 inflammasome pathways in microglia, and initially explored its possible intervention factors and mechanisms. This research can help us understand the mechanism of inflammatory response and its intervention factors after Mtb infection in the CNS, and it is of great significance to improve the treatment and prognosis of the disease.
Reagents
Pubs of SD rats were purchased from Northwestern University Animal Experiment Center. Dimethylsulfoxide (DMSO), MTT, Cytotoxicity Detection Kit-LDH, poly-L-lysine, and BCA protein quantification kit were from Beyotime, CN. P2X7R antibody, NLRP3 antibody, pro-IL-18 antibody, GSDMD antibody, CD11b antibody were from abcam, UK. Pro-IL-1β antibody, Caspase-1 (P10) antibody, pro-caspase-1(P45) antibody, and β-actin antibody, β-tubulin antibody were from Santa Cruz Biotechnology, CA. Phospho-NF-κB p65 antibody and Phospho-IκB-α were from Cell Signal Technology, USA. Serum IL-1β and IL-18 ELISA kit, BAY11-7082, RU486, Z-YVAD-FMK, TRIzol Reagent were from Sigma, USA. Revert Aid First Strand cDNA Synthesis Kit was from Thermo, USA. SYBR was from Takara, JP. GAPDH was from Sangon, CN. DEX was from Henan Runhong Pharmaceutical Co., Ltd., CN and Nicotine was from Shanghai Shuoguang Electronics Co., Ltd. Mtb H37Ra stain was from Shanghai Kanglang Biotechnology Co., Ltd., CN. Löwenstein-Jensen slope was from Solarbio, CN. Middlebrool 7H9 broth base was from BinSuiBio, CN. Nuclear and Cytoplasmic Extraction Kit was from CWBIO, CN (See the Supplementary Files for details).
Materials and Methods
Primary Microglia Culture
Primary microglia were obtained from SD rat pups within 4 hours of postnatal. After hypothermic anesthesia, the pups were decapitated and their brains were excised. Peeled off the meninges of the brain tissue, and then immersed it in 0.25% trypsin-EDTA, then mechanically separated the brain tissue with a 25-gauge needle, and homogenized it with a manual homogenizer. The cell suspension was seeded into poly-L-lysine-coated T150 tissue culture flasks and maintained in DMEM/F12 with 10% FBS and 1% penicillin-streptomycin for 10–14 days to grow a confluent mixed astrocyte/microglia population. On the 16th day, the cells were collected, positively selected by the antibody CD11b, and separated using a magnetic column combined with a magnetic ball compounded to the CD11b surface marker. Finally, the cells were transplanted into a six-well plate, adjusted the number of cells per well to about 1*105, and follow-up experiments were carried out 48 hours later. Microglial cell specific antibody CD11b was used for immunohistochemical staining. The microglial cell cultures used were >95% pure.
Cultivate Mtb H37Ra Strain
Mtb H37Ra stain was inoculated on the Löwenstein-Jensen slope for two weeks. The bacteria were then transferred to 7H9-S medium (Middlebrool 7H9 broth base, with 10% ADC-albumin glucose catalase and 0.2% glycerol), and were incubated for half an hour after shaking. Finally, the supernatant was placed in a clean test tube, and adjusted its final density to approximately 1×106 CFU/ml with 7H9-S broth (confirm the inoculum concentration by counting CFU on the agar plate).
Co-Culture of Microglia and H37Ra Strain
We co-cultured microglia with the tuberculosis H37Ra strain to establish a co-culture model. After the microglia were cultured in a six-well plate for 48 hours, 0.1ml of H37Ra strain at 1×106 CFU/ml was added to each well. In addition, different intervention factors were added to the wells, including MCC950 (10μM), Z-YVAD-FMK (50μM), BAY-11-7082 (10μM), RU486 (10μM), dexamethasone (DEX, 10μM), Potassium ion solution (K+, adjust the concentration to 150 mM), BBG (100nM), BzATP (100μM). After 4 hours of co-culture, we collected the cells and supernatant separately. Then, we washed the collected cells 3 times with PBS, and centrifuged the collected cell culture solution to remove bacteria and cells for subsequent experiments.
Western Blotting
In short, a modified RIPA buffer was used to lyse and extract cellular proteins. Nuclear and Cytoplasmic Extraction Kit was used to extract nuclear proteins. Then the extracted cell protein was quantified with BCA Protein Assay Kit. An equal amount of protein was loaded into each well of a 10% SDS-acrylamide gel, separated by gel electrophoresis, and then transferred to a nitrocellulose membrane. At room temperature, 5% skim milk blocking buffer was used to block the nitrocellulose membrane for 1 h. Then the membrane was incubated with the primary antibody overnight at 4°C. The next day, after washing three times with PBS, the membrane was incubated with a peroxidase-linked secondary antibody for 1 hour at room temperature. Subsequently, the bands were visualized by chemiluminescence (Millipore). ImageJ (NIH, USA) was used to analyze the intensity of each band. β-actin and β-tubulin were used as internal control.
ELISA to Detect Released Cytokines
After the co-cultivation, the extracellular medium was removed from each well and centrifuged at 10,000×g for 15 seconds to precipitate the cells, bacteria and other impurities. Then the concentration of IL-1β and IL-18 in the supernatant was determined according to the ELISA kit.
Real-Time PCR
After co-cultivation, the microglia cells were washed three times, and then used TRIzol Reagent to prepare total RNA according to the manufacturer’s protocol (Invitrogen Life Technologies). As RevertAidTM First Strand cDNA Synthesis Kit, one microgram of total RNA in each sample was used to generate cDNA. Amplify the resulting cDNA with SYBR. The process was detected by CFX96 Touch TM real-time PCR detection system. Finally, the 2-ΔΔCt method was used for relative quantitative analysis of mRNA. The results obtained are normalized to GAPDH. Real-time polymerase chain reaction used the following primers:
p2x7r: Forward primer 5′-CTACTCTTCGGTGGGGGCTT-3′
Reverse primer 5′-CTCTGGATCCGGGTGACTTT-3′
nlrp3: forward primer 5’-GAGCTGGACCTCAGTG ACAATGC-3’
Reverse primer 5’-ACCAATGCGAGATCCTG ACAACAC-3’
caspase-1: Forward primer 5’-AGGAGGGAATATGTGG G-3’
Reverse primer 5’-AACCTTGGGCTTGTCT T-3’
gapdh: Forward primer 5’-GACATGCCGCCTGGA GAAAC-3’
Reverse primer 5’-AGCCCAGGATGCCCTT TAGT-3’
il-1β: forward primer: 5′-CCCTGAACTCAACTGTGAAATAGCA-3′,
Reverse primer: 5′-GACTGGCTGTGACCCTATCTGTGA-3′;
il-18: Forward primer 5’-GACTGGCTGTGACCCTATCTGTGA-3’
Reverse primer 5’-TTGTGTCCTGGCACACGTTTC-3’
Cytotoxicity Determination (LDH Release)
After co-cultivation, the extracellular medium was removed from each well and centrifuged at 10,000×g for 15 seconds to pellet the cells and bacteria. Then the Cytotoxicity Detection Kit-LDH was used to determine the lactate dehydrogenase enzyme (LDH) in the supernatant.
MTT Analysis to Assess Cell Viability
After the co-cultivation was completed, discarded the original culture medium in each well and washed with PBS for three times. Then added 100μL of the prepared MTT (0.5 mg/ml) and incubate in a 5% CO2 incubator at 37°C for 4 hours. Discarded the supernatant, added 100 μL DMSO to each well, shaked well for 10 min to dissolve the crystals, and measured the absorbance at 570 nm with a microplate reader.
Statistical Analysis
All experimental results were obtained after performing at least 3 times the same experiment. All data were presented as mean ± standard deviation (SD) of independent experiments. Choose spss20.0 as the statistical analysis software. Two-tailed one-way analysis of variance (ANOVA) with multiple comparison post hoc analysis was used, P value<0.05 was considered statistically significant.
Result
The Co-Culture of H37Ra and Microglia Could Activate the NLRP3 Inflammasome in Microglia and Promote the Release of Inflammatory Factors Such as IL-18 and IL-1β
(1) NLRP3 inflammasome pathway in microglia could be activated by Mtb H37Ra, and the drugs used in this experiment had no significant effect on microglia.
As shown in Figure 1, we first applied a strong activator of the NLRP3 inflammasome pathway (nicotine (100 mM) + BzATP (100 mM)) as a control to verify whether the NLRP3 inflammasome pathway in microglia could be activated by Mtb H37Ra. At the same time, we also observed whether the relevant inhibitors and activators in this experiment could affect the state of microglia. The results showed that the NLRP3 inflammasome pathway in microglia could be activated by the Mtb H37Ra strain, and its effect was similar to nicotine +BzATP, and the effect was more obvious when the two were used in combination. After Mtb H37Ra strain or/and nicotine+BzAPT were used on microglia, the expression of NLRP3 inflammasome, pro-caspase-1, caspase-1, GSDMD, pro-IL-1β, pro-IL -18, etc. were significantly increased, and the contents of IL-1β and IL-18 in the supernate were obvious increasing, the release rate of LDH was eventually increased, and the survival rate of microglia was decreased. Subsequently, we observed the effects of related inhibitors and agonists that may be used in this experiment on microglia. The results showed that the inhibitor MCC950, Z-YVAD-FMK, BAY117802, DEX, RU486, BBG and KCl had no significant effect on the survival and activation of the NLRP3 inflammatory pathway of microglia. However, the agonist BzATP could activate the NLRP3 inflammasome pathway in microglia to a certain extent, leading to increased secretion of IL-1β and IL-18, and the increased release rate of LDH, and also decreased survival rate of microglia at the same time.
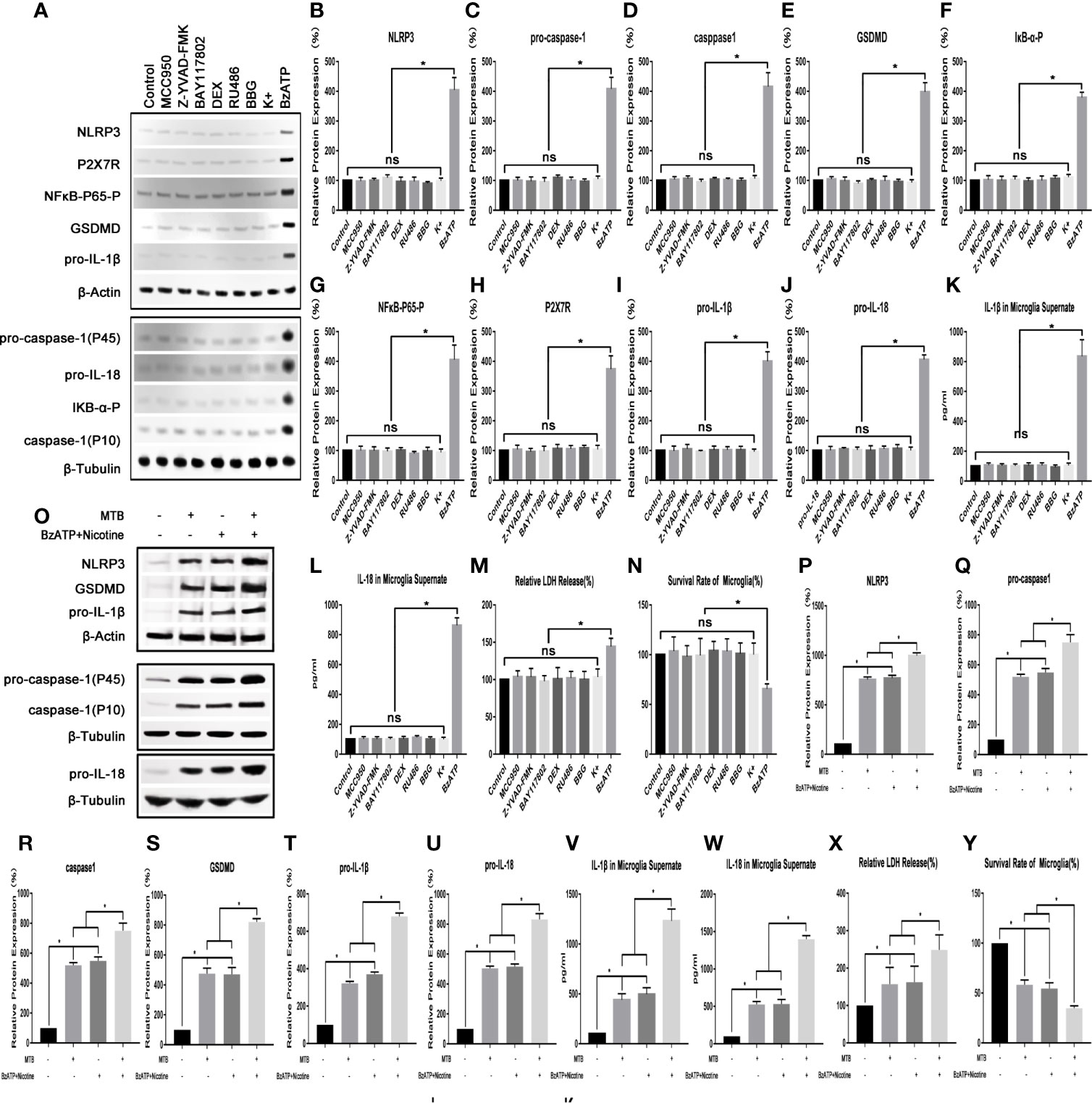
Figure 1 Observe the changes of NLRP3 inflammasome pathway and survival rate of microglia. (A–N) The influence of various inhibitors and agonists on microglia. Except for BzATP, the related drugs used in this experiment have no significant effect on the NLRP3 inflammasome pathway and survival rate of microglia under normal conditions. (O–Y) The effect of Mtb H37Ra and nicotine+BzATP on the NLRP3 inflammasome pathway in microglia. NLRP3 inflammasome pathway in microglia can be activated by the Mtb H37Ra strain, and its effect is similar to nicotine + BzATP, and the effect is more obvious when the two are used in combination. (*P < 0.05. ns, no significant difference).
(2) After co-cultivation, the expression of NLRP3 inflammasome, caspase-1, IL-18 and IL-1β in microglia were up-regulated.
As show in Figure 2, after co-cultivated microglia and Mtb H37Ra strain for 4 hours, we collected the cells and supernatant, and observed the changes of NLRP3, caspase-1, IL-18, and IL-1β in microglia in terms of transcription, protein expression, and inflammatory factor secretion. Experimental results showed that after co-culture, the NLRP3 inflammasome pathway in microglia was activated, and the secretion of LDH, IL-18 and IL-1β in the culture supernatant was also significantly increased. In addition, the survival rate of microglia decreased significantly. Compared with control group, in microglia of co-culture group, the mRNA and protein content of NLRP3 increased significantly (P<0.05). The mRNA content of caspase-1, IL-1β and IL-18, as well as the protein content of pro-caspase-1, pro-IL-18, pro-IL-1β, and activated caspase-1 were increased significantly (P <0.05). And the levels of IL-18 and IL-1β in the supernatant was also increased significantly (P<0.05). Finally, we measured the LDH content and cell survival rate in microglia after co-culture, and found that the LDH content increased significantly (P<0.05), while the cell survival rate decreased significantly (P<0.05).
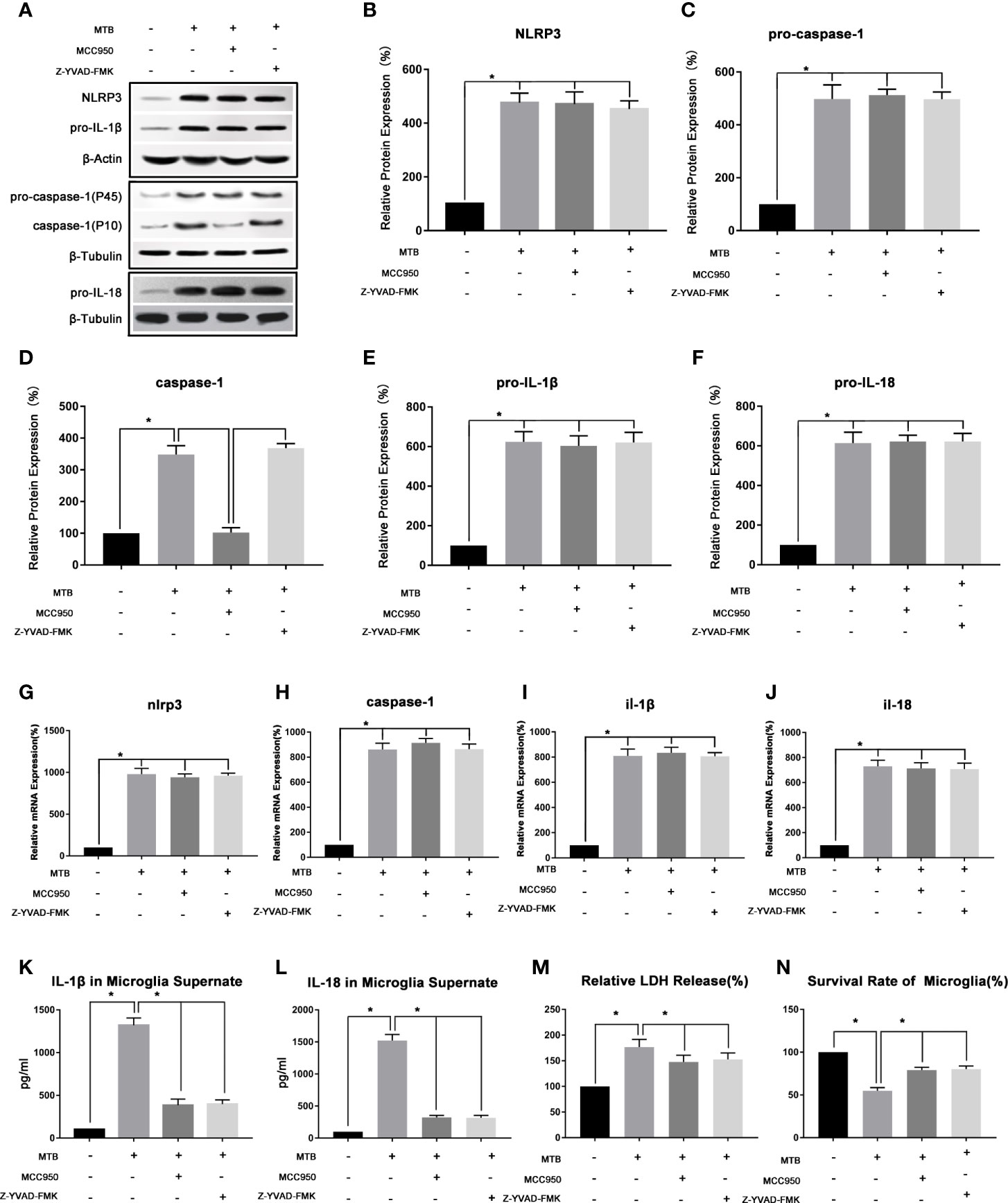
Figure 2 Observe the changes of NLRP3 inflammasome of microglia in the co-culture model. (A–F) Use Western Blot to detect the relative protein expression of NLRP3, pro-caspase-1, caspase-1, pro-IL-1β and pro-IL-18 in control group, co-culture group, MCC950 group and Z-YVAD-FMK group. (G–J) Use Real-time PCR to detect the relative mRNA expression of nlrp3, caspase-1, il-1β and il-18 in control group, co-culture group, MCC950 group and Z-YVAD-FMK group. (K, L) Use ELISA to detect the IL-1β and IL-18 secretion in supernatant of control group, co-culture group, MCC950 group and Z-YVAD-FMK group. (M) Use LDH release to measure the cytotoxicity in control group, co-culture group, MCC950 group and Z-YVAD-FMK group. (N) Use MTT analysis to detect the cell survival rate in control group, co-culture group, MCC950 group and Z-YVAD-FMK group. *P < 0.05.
(3) Intervening the co-culture process with MCC950 could inhibit the function of NLRP3 inflammasome, reduce the release of IL-18 and IL-1β, and increase the survival of microglia.
As show in Figure 2, we used the NLRP3 inhibitor MCC950 to interfere with the co-cultivation process. After 4 hours of co-cultivation, the microglia and supernatant were collected, and the NLRP3 inflammasome-related moleculars in the microglia were detected, as well as the secretion of IL-18 and IL-1β. Compared with the co-culture group, the moleculars in microglia in MCC950 intervention group changed as follow, the mRNA and protein content of NLRP3 did not change significantly (P>0.05), as well as the mRNA content of caspase-1 and the protein content of pro-caspase-1 (P>0.05). However, the protein content of activated caspase-1 was significantly reduced (P <0.05). Then we observed the mRNA content of IL-1β and IL-18, as well as the protein content of pro-IL-18 and pro-IL-1β, and found that there was also no significant change (P>0.05), but the content of IL-18 and IL-1β in the supernatant was significantly reduced (P<0.05). Finally, we measured the LDH content of microglia and the cell survival rate. Compared with the co-culture group, the LDH content of microglia in the MCC950 intervention group decreased and the cell survival rate was significantly improved (P<0.05).
(4) Z-YVAD-FMK could inhibit the function of caspase-1, thereby reducing the production of IL-18 and IL-1β in microglia.
As shown in Figure 2, we then added Z-YVAD-FMK, the inhibitor of caspase-1, during the co-cultivation process. After 4 hours of co-cultivation, the cells and supernatant were collected separately. Subsequently, the changes of NLRP3, caspase-1, IL-18, and IL-1β in microglia and supernatant were detected. The results showed that in Z-YVAD-FMK inhibitor group, compared with the co-culture group, the mRNA content of nlrp3, caspase-1, il-18, il-1β, and the protein content of NLRP3, pro-caspase-1, caspase-1, pro-IL-18 and pro-IL-1β were not significantly changed (P>0.05). However, IL-1β and IL-18 in the supernatant were significantly reduced (P<0.05), and the content of LDH in microglia decreased and the cell survival rate were increased (P<0.05).
During the Co-Cultivation, the Activation of NLRP3 Inflammasome in Microglia Involved NF-κB Pathway
(1) After co-culture, NLRP3 inflammasomes in microglia could be activated through the NF-κB pathway. By using BAY-11-7082 to inhibit IκB-α, the activation of NLRP3 could be inhibited.
As show in Figure 3, we first examined the expression levels of phosphorylated IκB-α in microglia and phosphorylated NF-κB-P65 in nucleus in microglia after co-culture. The results showed that compared with control group, the level of phosphorylated IκB-α in microglia and phosphorylated NF-κB-P65 in its nucleus of the co-culture group were significantly increased (P<0.05). After applying BAY-11-7082, the content of phosphorylated IκB-α in cell and phosphorylated NF-κB-P65 in its nucleus were significantly reduced (P<0.05), and mRNA and protein levels of NLRP3, pro-caspase-1, pro-IL-18, pro-IL-1β were also significantly reduced (P<0.05). At the same time, the protein content of activated caspase-1 and the secretion of IL-18 and IL-1β in the supernatant were also significantly reduced (P<0.05). Finally, we measured the content of LDH in microglia and their cell survival rate. Compared with the co-culture group, after applying BAY-11-7082, the content of LDH in microglia decreased and the survival rate of cells increased (P<0.05).
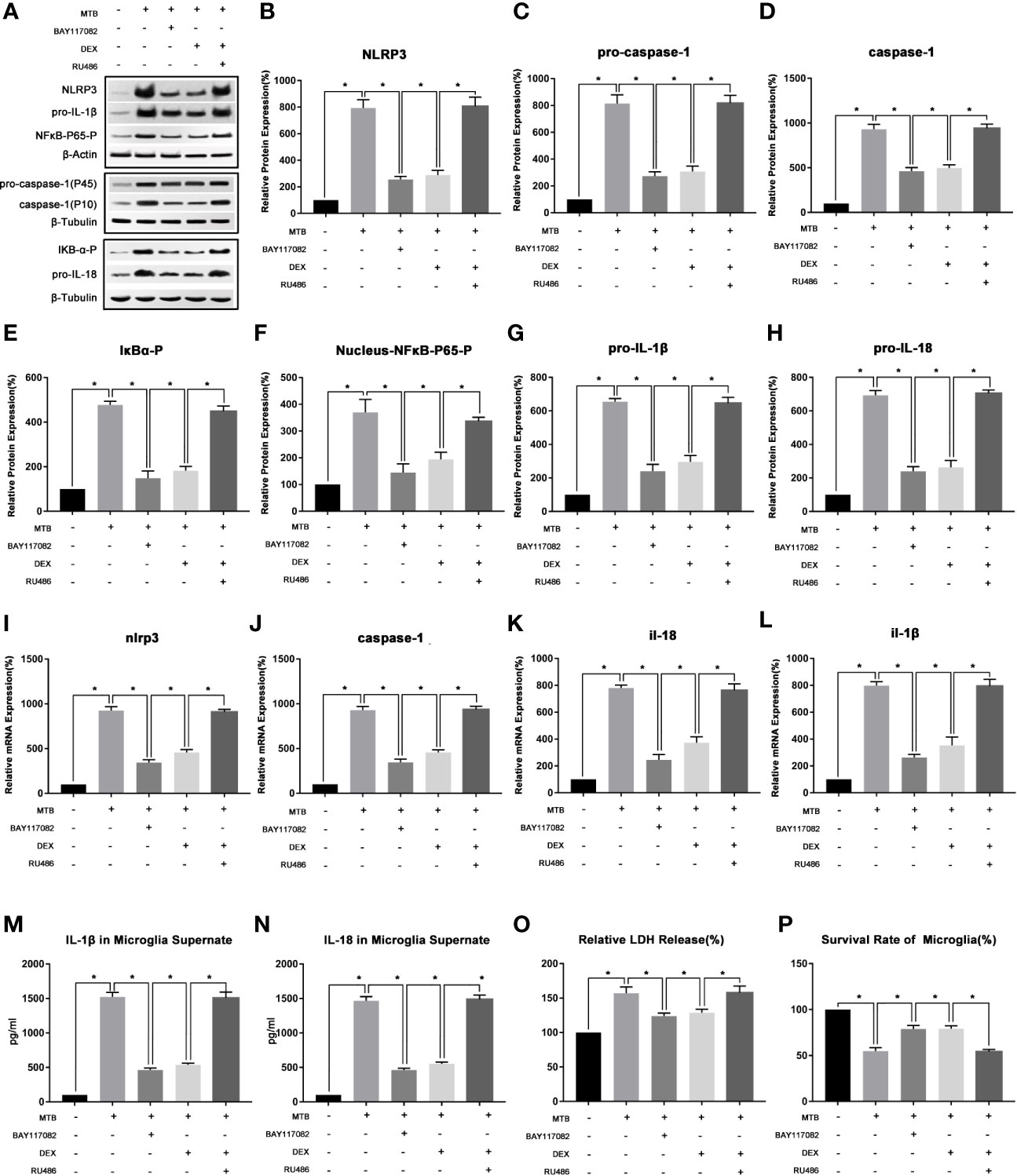
Figure 3 The changes of NF-κB pathway and its intervention factors during the activation of NLRP3 inflammasome in microglia. (A–H) Use Western Blot to detect the relative protein expression of NLRP3, pro-caspase-1, caspase-1, pro-IL-1β and pro-IL-18, phosphorylated IκB-α and phosphorylated NF-κB-P65 in control group, co-culture group, BAY-11-7082 group, DEX group and RU486+DEX group. (I–L) Use Real-time PCR to detect the relative mRNA expression of nlrp3, caspase-1, il-1β and il-18 in control group, co-culture group, BAY-11-7082 group, DEX group and RU486+DEX group. (M, N) Use ELISA to detect the IL-1β and IL-18 secretion in supernatant of control group, co-culture group, BAY-11-7082 group, DEX group and RU486+DEX group. (O) Use LDH release to measure the cytotoxicity in control group, co-culture group, BAY-11-7082 group, DEX group and RU486+DEX group. (P) Use MTT analysis to detect the cell survival rate in control group, co-culture group, BAY-11-7082 group, DEX group and RU486+DEX group. *P < 0.05.
(2) In the process of co-cultivation, DEX and RU486 could intervene the NLRP3 inflammasome in microglia by affecting the NF-κB pathway.
As show in Figure 3, we then used DEX and glucocorticoid receptor (GR) inhibitor RU486 to intervene in the co-culture process separately. After 4 hours of co-culture, cells and supernatant were collected and observed. The results showed that, DEX could play a similar effect as IκB-α inhibitor BAY117082 (P<0.05). Compared with the Co-culture group, the content of phosphorylated IκB-α in cell and phosphorylated NF-κB-P65 in the nucleus were both significantly reduced (P<0.05), and the mRNA and protein levels of NLRP3 and pro-caspase-1, pro-IL-18, pro-IL-1β were also significantly reduced (P<0.05). At the same time, the protein content of activated caspase-1 in microglia and the secretion of IL-18 and IL-1β in the supernatant were also significantly reduced (P<0.05). Finally, we measured the content of LDH in microglia and their cell survival rate. It suggested that compared with the co-culture group, after applying DEX, the content of LDH in microglia decreased and the survival rate of cells increased (P<0.05). Subsequently, we intervened the co-culture process with the RU486 and DEX at the same time, and observed the pathways of NF-κB and NLRP3 inflammasome. The results showed that after combined application, the content of phosphorylated IκB-α in cell and phosphorylated NF-κB-P65 in the nucleus, and the activation degree of NLRP3 inflammasome pathway were not significantly different from the co-culture group (P>0.05).
During the Co-Cultivation, the Activation of NLRP3 Inflammasome in Microglia Involved P2X7R Pathway
(1) After co-cultivation, the expression of P2X7R in microglia increased, and P2X7R inhibitor BBG had an inhibitory effect on NLRP3 inflammasome pathway.
As show in Figure 4, we first examined the expression level of P2X7R in microglia after co-culture. The results showed that compared with the control group, the mRNA and protein content of P2X7R in co-culture group were significantly increased (P<0.05). After applying BBG, compared with the co-culture group, the mRNA and protein content of P2X7R did not change significantly (P>0.05), but the mRNA and protein content of NLRP3 and pro-caspase-1, pro-IL-18 and pro-IL-1β were significantly reduced (P<0.05). At the same time, the protein content of activated caspase-1 and the secretion of IL-18 and IL-1β were also significantly reduced (P<0.05). Finally, we measured the content of LDH in microglia and their cell survival rate, compared with the co-culture group, after applying BBG, the content of LDH in microglia decreased and the survival rate of cells increased (P<0.05).
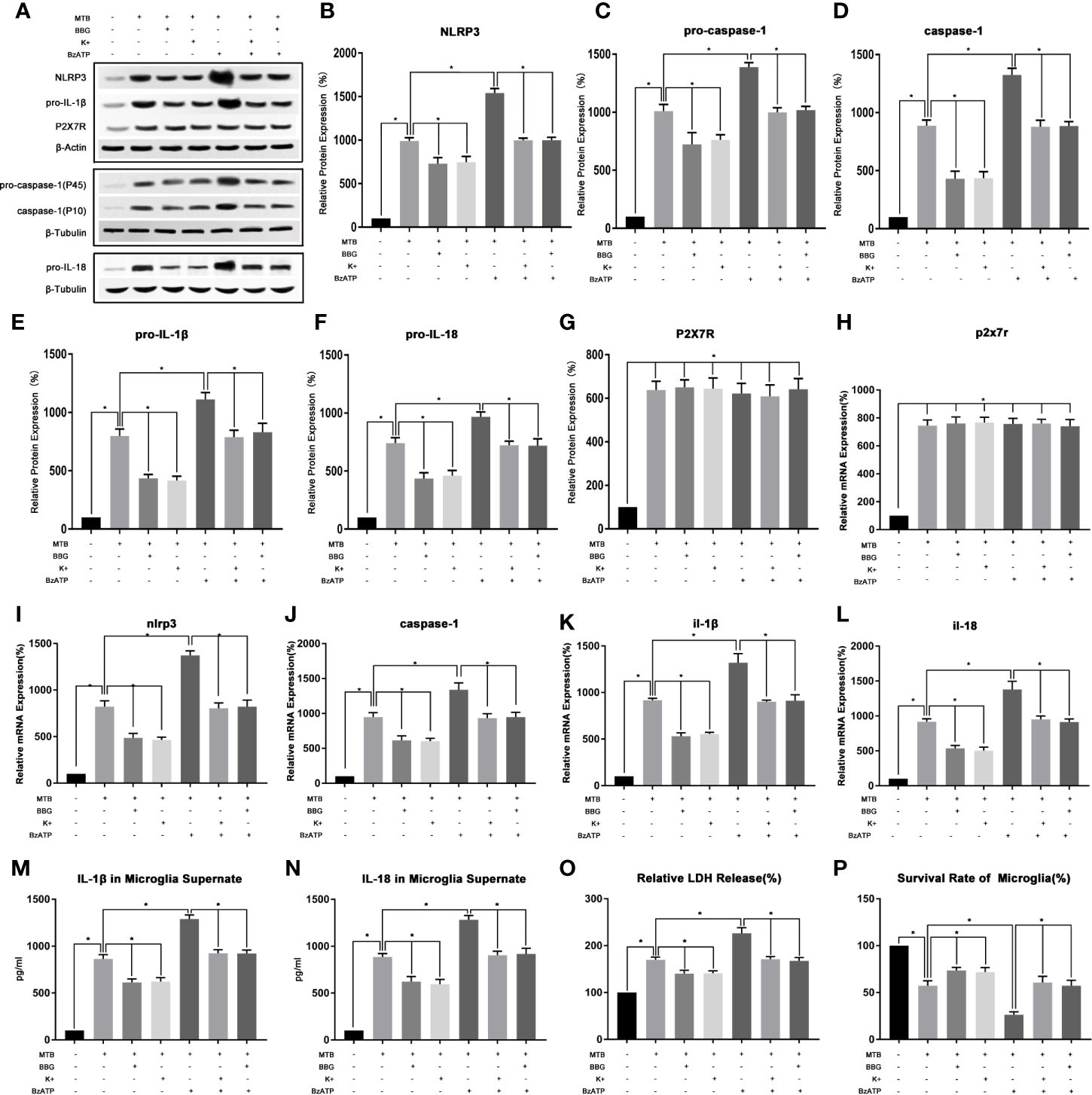
Figure 4 In the process of co-culture, the changes of P2X7R pathway and its intervention factors during the activition of NLRP3 inflammasome in microglia. (A–G) Use Western Blot to detect the relative protein expression of NLRP3, pro-caspase-1, caspase-1, pro-IL-1β and pro-IL-18, and P2X7R in control group, co-culture group, BBG group, K+ group, BzATP group, BBG+BzATP group and K++BzATP group. (H–L) Use Real-time PCR to detect the relative mRNA expression of nlrp3, caspase-1, il-1β and il-18, and p2x7r in control group, co-culture group, BBG group, K+ group, BzATP group, BBG+BzATP group and K++BzATP group. (M, N) Use ELISA to detect the IL-1β and IL-18 secretion in supernatant of control group, co-culture group, BBG group, K+ group, BzATP group, BBG+BzATP group and K++BzATP group. (O) Use LDH release to measure the cytotoxicity in control group, co-culture group, BBG group, K+ group, BzATP group, BBG+BzATP group and K++BzATP group. (P) Use MTT analysis to detect the cell survival rate in control group, co-culture group, BBG group, K+ group, BzATP group, BBG+BzATP group and K++BzATP group. *P < 0.05.
(2) Intervention with P2X7R could regulate NLRP3 inflammasome in microglia during co-culture process.
As show in Figure 4, we created an extracellular potassium environment (K+) in the co-culture medium to observe the changes in P2X7R and NLRP3 inflammatory. The results showed that the extracellular potassium environment could play a similar effect to the P2X7R inhibitor BBG (P>0.05). Compared with the co-culture group, there was no significant change in the mRNA and protein content of P2X7R in K+ group(P>0.05). However, the mRNA and protein content of NLRP3 and pro-caspase-1, pro-IL-18, and pro-IL-1β were significantly reduced (P<0.05). At the same time, the protein content of activated caspase-1 and the secretion of IL-18 and IL-1β were also significantly reduced (P<0.05). Finally, we measured the content of LDH in microglia and their cell survival rate, compared with the co-culture group, the content of LDH in microglia decreased and the survival rate of cells increased in the K+ group (P<0.05).
Then we applied the P2X7R agonist BzAPT to intervene in the co-culture process, and observe the P2X7R and NLRP3 inflammasome. The results showed that compared with the co-culture group, there was no significant change in the mRNA and protein content of P2X7R after applying BzAPT (P>0.0.5). But the mRNA and protein content of NLRP3, pro-caspase-1, pro-IL-18 and pro-IL-1β increased significantly (P <0.05). At the same time, the protein content of activated caspase-1, and the secretion of IL-18 and IL-1β were also increased significantly (P<0.05). Finally, we measured the content of LDH in microglia and their cell survival rate, compared with the co-culture group, after applying BzAPT, the content of LDH in microglia increased significantly, while the cell survival rate decreased (P<0.05).
Finally, BBG combined with BzATP, as well as extracellular high potassium combined with BzAPT were used to intervene in the co-culture process, and the changes in P2X7R and NLRP3 inflammasome was observed. The experimental results showed that after combined application, the activation of P2X7R and NLRP3 inflammasome was similar to the co-culture group (P>0.05).
Discussion
In this study, we used a co-culture model of microglia and H37Ra to simulate tuberculosis infection in the CNS, and studied whether there is activation of the NLRP3 inflammasome pathway in microglia during this process, and what are its intervention factors. The results indicated that during the co-cultivation process, the NLRP3 inflammasome and its downstream molecules in microglia activated, the gene transcription and protein expression of the involved molecules increased significantly. Finally, the secreted IL-18 and IL-1β, the LDH, and the mortality of microglia were also increased. By interfering with the functions of NLRP3 and caspase-1, or by interfering with NF-κB in signal 1, or by interfering with P2X7R in signal 2, the secretion of IL-18, IL-1β and the death of microglia could be reduced.
Mtb infection of the CNS involves a series of complex pathophysiological processes, among which excessive inflammation is an important cause of brain damage. Microglia are vital immune cells in the CNS and involved in many aspects of the inflammatory response, they play an important role in the process of tuberculosis infection in the CNS (Rock et al., 2004; Spanos et al., 2015). A number of previous studies have shown that there are many different inflammasomes in microglia. These inflammasomes are widely involved in many different types of neurological diseases, especially in neurological infectious diseases (Marim et al., 2017; Heneka et al., 2018; Katuri et al., 2019; Xue et al., 2019; Cui et al., 2020; Ji et al., 2020; Ma et al., 2020; Zeng et al., 2020). Lee et al. (2013) have found that after co-cultivation with Mtb and macrophages, the culture supernatant can activate the NLRP3 inflammasome pathway in microglia. Our experiment had similarities with it. The difference was that we used Mtb to directly interact with microglia to observe its effect on NLRP3 inflammasomes in microglia. This is because microglia and macrophages are homologous. They enter the CNS during development and eventually become macrophages that reside in the CNS. Their immune response and inflammatory response are closely related to the CNS, which is also the same in the process of CNS Mtb infection. Therefore, we made Mtb interact directly with microglia, and observed the influence of Mtb on the inflammatory response of microglia in a more intuitive way.
Our experiments suggested that after Mtb interacts with microglia, microglia produced and released a large number of inflammatory factors through the NLRP3 inflammasome pathway, which promoted cell death. By regulating this process could reduce the inflammatory response of microglia to a certain extent. Our experiment is of great significance to clarify the mechanism of inflammatory response and to regulate excessive inflammatory response in CNS tuberculosis infection.
As mentioned above, the classic activation of NLRP3 inflammasome is divided into two stages: signal 1 and signal 2. We first observed the NF-κB pathway in signal1. Previous research has shown that with the phosphorylation of IκB-α, IκB dissociates from NF-κB and moves to the proteasome and degrades, thereby translocating NF-κB to the nucleus (Kharytonchyk et al., 2018). By using BAY-11-7082 to regulate the phosphorylation of IκB-α located upstream of NF-κB, it could affect the nuclear translocation of NF-κB and reduce the phosphorylated NFκB-P65 in the nucleus, thereby reduced the gene transcription of each molecule in the NLRP3 inflammasome pathway, and ultimately reduced the secretion of IL-18 and IL-1β. This confirms that in the process of tuberculosis infection in the CNS, the activation of NLRP3 inflammasomes in microglial is mediated by NF-κB, and the regulation of NLRP3 inflammasomes can be achieved by intervening in the function of NF-κB. Subsequently, we intervened in the co-culture process with DEX, a drug that is clinically used to alleviate the inflammatory response of tuberculosis infection in the CNS (Shane and Riley, 1953; Thwaites et al., 2004), to understand whether it has a regulatory effect on NRLP3 inflammasome. Gräb et al. (Gräb et al., 2019) has found that corticosteroids such as DEX inhibit necrotic cell death of cells infected with Mtb by facilitating mitogen-activated protein kinase phosphatase 1 (MKP-1)-dependent dephosphorylation of p38 MAPK. Green et.al (Green et al., 2010) has found that Mtb can upregulates microglial matrix metalloproteinase-1 and -3 expression and secretion via NF-κB and activator Protein-1-dependent monocyte networks. And in this study, we found that DEX can affect the phosphorylation of IκB-α through GR receptors, thereby exerting a similar effect to BAY-11-7082, affecting the transcription of various molecules in the NLRP3 inflammasome pathway, and ultimately reducing the release of IL-18 and IL-1β, alleviate cytotoxicity and cell death. After applying the GR receptor inhibitor RU486, the above-mentioned effects of DEX almost disappeared. These results are consisted with previous studies (Oh et al., 2017; Seshadri et al., 2018) that GR can bind to IκB and inhibit its phosphorylation after being activated, thereby inhibiting the nuclear translocation of NF-κB and its function in the nucleus. In addition, there are also studies showed that DEX can inhibit the activation of NLRP3 inflammasomes in other different types of cells (Cao et al., 2018; Pellegrini et al., 2018; Gong et al., 2019; Yang et al., 2019). So, our experiment supports the rationality of the application of glucocorticoids in the clinical treatment of tuberculosis infection of the CNS.
In addition, the process of CNS Mtb infection is often accompanied by cell energy metabolism disorders, and the imbalance of sodium and potassium ions inside and outside the cell (Fernando et al., 2007; Davis et al., 2019). In addition, as nerve cells are continuously invaded by Mtb, the integrity of the cell membrane is destroyed, and a large number of intracellular substances including potassium ions and ATP are released (Fernando et al., 2007; Davis et al., 2019). Therefore, we chose P2X7R in signal2 for observation. P2X7R is a non-specific cation channel that can be activated by ATP released by cell death, and its function is also highly dependent on the outflow of potassium ions, its function is closely related to the assembly of NLRP3 inflammasome complex (Karmakar et al., 2016). P2X7R in microglia is widely involved in the process of many neurological diseases (Franceschini et al., 2015; Yue et al., 2017; Zhao et al., 2017), We first observed the changes of P2X7R during the co-cultivation process and found that there was indeed a significant up-regulation of P2X7R expression. Subsequently, we used BBG to inhibit the function of P2X7R and found that it could significantly reduce the expression of NLRP3 inflammasome-related molecules, and reduce the final release of IL-18 and IL-1β, suggesting that P2X7R plays an important role in the activation of NLRP3 in microglia. Subsequently, we created an extracellular high potassium environment to inhibit the outflow of potassium ions, and found that it can play a similar role to the P2X7R inhibitor BBG, and the application of BzATP could significantly enhance the effect of P2X7R and enhance the NLRP3 inflammasome pathway, and increase the release of inflammatory factors. The above experimental results indicate that in the process of tuberculosis infection in the CNS, the imbalance of intracellular and extracellular electrolytes, as well as the APT released by necrotic cells, can affect the P2X7R receptor on the microglia membrane and further lead to the NLRP3 inflammasome pathway activation and the release of inflammatory factors. These inflammatory factors can cause a subsequent cascade reaction and cause more serious nerve cell damage. This also explains from one perspective why such a severe inflammatory response occurs after the CNS is infected with Mtb.
However, this experiment also has some shortcomings. First of all, we did not clarify which bacterial components of Mtb interacted with microglia. For example, Liu et al. (2021) found that lipoprotein LpqH in Mtb can active NLRP3 inflammasome in mouse Ana-1 macrophage. Also, Beckwith et al. (2020) has found that plasma membrane damage causes NLRP3 activation and pyroptosis during Mtb infection. But as composition of Mtb is very complex, and a complex immune cascade also occurs during the infection process, we have not been able to determine the specific components that can activate microglia. In addition, there are a variety of inflammasomes in microglia, which are involved in many different kinds of neurological diseases (Marim et al., 2017; Heneka et al., 2018). However, in this study, we did not conduct further research on this, and it is not ruled out that other types of inflammasomes participate in the co-culture process. Finally, in this study, we did not study the relationship between P2X7R and NFκB, and the reasons why P2X7R caused gene transcription in changes in NLRP3 inflammasome-related moleculars. Previous studies have shown that P2X7R not only affects the assembly of NLRP3 inflammasomes, but also affects the transcription of related molecules (Ferrari et al., 2006; Potucek et al., 2006; Mingam et al., 2008; Piccini et al., 2008). Also, there is a close relationship between P2X7R and NF-κB (Yu et al., 2019; Yang et al., 2020). Therefore, the regulation of P2X7R may further affect the gene transcription process through the NF-κB pathway. This may also be one of the deeper mechanisms that P2X7R regulates NLRP3 inflammasome-related molecules. The above-mentioned problems will be further confirmed in follow-up research.
Conclusion
Through this study, we confirm for the first time that NLRP3 inflammasome and its downstream pathways are activated in the Mtb H37Ra strain and rat microglia co-culture model. The activation of NLRP3 inflammasome is closely related to the NF-κB pathway in signal1 and the P2X7R in signal2. Interventions on these can regulate the function of the NLRP3 inflammasome pathway and affect the production of inflammatory factors, cytotoxicity and cell survival in microglia. Using H37Ra and microglia co-culture model to study the Mtb infection in CNS can further understand its pathogenesis and provide a useful reference for its treatment.
Data Availability Statement
The raw data supporting the conclusions of this article will be made available by the authors, without undue reservation.
Ethics Statement
The animal study was reviewed and approved by Animal Care and Use Committee of the Northwest University.
Author Contributions
ZX is responsible for experimental design and proteomics experiments. HH is responsible for genomics experiments. QY and YD are responsible for cultivation of cells and bacteria. WL, YC, and MZ are in charge of ELIAS experiments and the collection and sorting of documents. GZ and YT are responsible for data sorting and analysis. ZX was a major contributor in writing the manuscript. All authors contributed to the article and approved the submitted version.
Funding
This work was supported by grants from: 1) Natural Science Basic Research Program of Shaanxi (Program No. 2019JQ-251) and Natural Science Basic Research Program of Shaanxi (Program No. 2019JQ-543), supported the design of the study and the collection of date. 2) National Natural Science Foundation of China (Program No. 81671185), supported in the reagent purchase and instrument usage cost. 3) Hospital-level project of Xi’an International Medical Center (Program No. 2020ZD007) and Natural Science Basic Research Program of Xi’an (Program No. 2020qn17), supported in the expenses related to laboratory animals and cell culture. 4) the Key Research and Development Program of Shaanxi (Program No.2020ZDLSF04-03), supported in the analysis, and interpretation of data. 5) Science and Technology Project of Xi’an, Shaanxi Province, China 201805104YX12SF38(2), supported in writing the manuscript.
Conflict of Interest
The authors declare that the research was conducted in the absence of any commercial or financial relationships that could be construed as a potential conflict of interest.
Acknowledgments
The authors thank all laboratory members for ongoing discussions and all the financial support of above funds.
Supplementary Material
The Supplementary Material for this article can be found online at:https://www.frontiersin.org/articles/10.3389/fcimb.2021.637769/full#supplementary-material
Abbreviations
CNS, centrol neuron system; TM, Tuberculous meningitis; Mtb, Mycobacterium tuberculosis; P2X7R, P2X7 receptor; DMSO, Dimethylsulfoxide; K+, potassium ion solution; GR, Glucocorticoid receptor; DEX, Dexamethasone; LDH, lactate dehydrogenase enzyme.
References
Beckwith, K. S., Beckwith, M. S., Ullmann, S., Sætra, R. S., Kim, H., Marstad, A., et al. (2020). Plasma membrane damage causes NLRP3 activation and pyroptosis during Mycobacterium tuberculosis infection. Nat. Commun. 11, 2270. doi: 10.1038/s41467-020-16143-6
Cao, Y., Zhou, X., Yin, Z., Yu, X., Yang, Q., Guo, Q., et al. (2018). The anti-inflammatory effect of BML-111 on COPD may be mediated by regulating NLRP3 inflammasome activation and ROS production. Prostaglandins Other Lipid Mediat. 138, 23–30. doi: 10.1016/j.prostaglandins.2018.08.001
Cui, W., Sun, C., Ma, Y., Wang, S., Wang, X., Zhang, Y. (2020). Inhibition of TLR4 Induces M2 Microglial Polarization and Provides Neuroprotection via the NLRP3 Inflammasome in Alzheimer’s Disease. Front. Neurosci. 14, 444. doi: 10.3389/fnins.2020.00444
Davis, A. G., Rohlwink, U. K., Proust, A., Figaji, A. A., Wilkinson, R. J. (2019). The pathogenesis of tuberculous meningitis. J. Leukocyte Biol. 105, 267–280. doi: 10.1002/JLB.MR0318-102R
Dong, Y., Li, S., Lu, Y., Li, X., Liao, Y., Peng, Z., et al. (2020). Stress-induced NLRP3 inflammasome activation negatively regulates fear memory in mice. J. Neuroinflamm. 17, 205. doi: 10.1186/s12974-020-01842-0
Fernando, S. L., Saunders, B. M., Sluyter, R., Skarratt, K. K., Goldberg, H., Marks, G. B., et al. (2007). A polymorphism in the P2X7 gene increases susceptibility to extrapulmonary tuberculosis. Am. J. Respir. Crit. Care Med. 175, 360–366. doi: 10.1164/rccm.200607-970OC
Ferrari, D., Pizzirani, C., Adinolfi, E., Lemoli, R. M., Curti, A., Idzko, M., et al. (2006). The P2X7 receptor: a key player in IL-1 processing and release. J. Immunol. (Baltimore Md 1950) 176, 3877–3883. doi: 10.4049/jimmunol.176.7.3877
Franceschini, A., Capece, M., Chiozzi, P., Falzoni, S., Sanz, J. M., Sarti, A. C., et al. (2015). The P2X7 receptor directly interacts with the NLRP3 inflammasome scaffold protein. FASEB J. Off. Publ. Fed. Am. Societies Exp. Biol. 29, 2450–2461. doi: 10.1096/fj.14-268714
Gong, Q., He, L., Wang, M., Zuo, S., Gao, H., Feng, Y., et al. (2019). Comparison of the TLR4/NFκB and NLRP3 signalling pathways in major organs of the mouse after intravenous injection of lipopolysaccharide. Pharm. Biol. 57, 555–563. doi: 10.1080/13880209.2019.1653326
Gräb, J., Suárez, I., van Gumpel, E., Winter, S., Schreiber, F., Esser, A., et al. (2019). Corticosteroids inhibit Mycobacterium tuberculosis-induced necrotic host cell death by abrogating mitochondrial membrane permeability transition. Nat. Commun. 10, 688. doi: 10.1038/s41467-019-08405-9
Green, J. A., Elkington, P. T., Pennington, C. J., Roncaroli, F., Dholakia, S., Moores, R. C., et al. (2010). Mycobacterium tuberculosis upregulates microglial matrix metalloproteinase-1 and -3 expression and secretion via NF-kappaB- and Activator Protein-1-dependent monocyte networks. J. Immunol. (Baltimore Md 1950) 184, 6492–6503. doi: 10.4049/jimmunol.0903811
He, W. T., Wan, H., Hu, L., Chen, P., Wang, X., Huang, Z., et al. (2015). Gasdermin D is an executor of pyroptosis and required for interleukin-1β secretion. Cell Res. 25, 1285–1298. doi: 10.1038/cr.2015.139
Heneka, M. T., McManus, R. M., Latz, E. (2018). Inflammasome signalling in brain function and neurodegenerative disease, Nature reviews. Neuroscience 19, 610–621. doi: 10.1038/s41583-018-0055-7
Hernandez Pando, R. (2011). Modelling of Cerebral Tuberculosis: Hope for Continuous Research in Solving the Enigma of the Bottom Billion’s Disease. Malaysian J. Med. Sci. MJMS 18, 12–15.
Irrera, N., Vaccaro, M., Bitto, A., Pallio, G., Pizzino, G., Lentini, M., et al. (2017). BAY 11-7082 inhibits the NF-κB and NLRP3 inflammasome pathways and protects against IMQ-induced psoriasis. Clin. Sci. 131, 487–498. doi: 10.1042/CS20160645
Ji, Y. J., Wang, H. L., Yin, B. L., Ren, X. Y. (2020). Down-regulation of DJ-1 Augments Neuroinflammation via Nrf2/Trx1/NLRP3 Axis in MPTP-induced Parkinson’s Disease Mouse Model. Neuroscience 442, 253–263. doi: 10.1016/j.neuroscience.2020.06.001
Karmakar, M., Katsnelson, M. A., Dubyak, G. R., Pearlman, E. (2016). Neutrophil P2X7 receptors mediate NLRP3 inflammasome-dependent IL-1β secretion in response to ATP. Nat. Commun. 7, 10555. doi: 10.1038/ncomms10555
Katuri, A., Bryant, J., Heredia, A., Makar, T. K. (2019). Role of the inflammasomes in HIV-associated neuroinflammation and neurocognitive disorders. Exp. Mol. Pathol. 108, 64–72. doi: 10.1016/j.yexmp.2019.03.008
Kharytonchyk, S., Brown, J. D., Stilger, K., Yasin, S., Iyer, A. S., Collins, J., et al. (2018). Influence of gag and RRE Sequences on HIV-1 RNA Packaging Signal Structure and Function. J. Mol. Biol. 430, 2066–2079. doi: 10.1016/j.jmb.2018.05.029
Lee, H. M., Kang, J., Lee, S. J., Jo, E. K. (2013). Microglial activation of the NLRP3 inflammasome by the priming signals derived from macrophages infected with mycobacteria. Glia 61, 441–452. doi: 10.1002/glia.22448
Liu, L., Zhai, K., Chen, Y., Chen, X., Wang, G., Wu, L. (2021). Effect and Mechanism of Mycobacterium tuberculosis Lipoprotein LpqH in NLRP3 Inflammasome Activation in Mouse Ana-1 Macrophage. BioMed. Res. Int. 2021, 8239135. doi: 10.1155/2021/8239135
Ma, D. C., Zhang, N. N., Zhang, Y. N., Chen, H. S. (2020). Kv1.3 channel blockade alleviates cerebral ischemia/reperfusion injury by reshaping M1/M2 phenotypes and compromising the activation of NLRP3 inflammasome in microglia. Exp. Neurol. 332, 113399. doi: 10.1016/j.expneurol.2020.113399
Marim, F. M., Franco, M. M. C., Gomes, M. T. R., Miraglia, M. C., Giambartolomei, G. H., Oliveira, S. C. (2017). The role of NLRP3 and AIM2 in inflammasome activation during Brucella abortus infection. Semin. Immunopathol. 39, 215–223. doi: 10.1007/s00281-016-0581-1
Mingam, R., De Smedt, V., Amédée, T., Bluthé, R. M., Kelley, K. W., Dantzer, R., et al. (2008). In vitro and in vivo evidence for a role of the P2X7 receptor in the release of IL-1 beta in the murine brain. Brain Behav. Immun. 22, 234–244. doi: 10.1016/j.bbi.2007.08.007
Oh, K. S., Patel, H., Gottschalk, R. A., Lee, W. S., Baek, S., Fraser, I. D. C., et al. (2017). Anti-Inflammatory Chromatinscape Suggests Alternative Mechanisms of Glucocorticoid Receptor Action. Immunity 47, 298–309.e295. doi: 10.1016/j.immuni.2017.07.012
Pellegrini, C., Fornai, M., Colucci, R., Benvenuti, L., D’Antongiovanni, V., Natale, G., et al. (2018). A Comparative Study on the Efficacy of NLRP3 Inflammasome Signaling Inhibitors in a Pre-clinical Model of Bowel Inflammation. Front. Pharmacol. 9, 1405. doi: 10.3389/fphar.2018.01405
Piccini, A., Carta, S., Tassi, S., Lasiglié, D., Fossati, G., Rubartelli, A. (2008). ATP is released by monocytes stimulated with pathogen-sensing receptor ligands and induces IL-1β and IL-18 secretion in an autocrine way. Proc. Natl. Acad. Sci. 105, 8067–8072. doi: 10.1073/pnas.0709684105
Potucek, Y., Crain, J., Watters, J. (2006). Purinergic receptors modulate MAP kinases and transcription factors that control microglial inflammatory gene expression. Neurochem. Int. 49, 204–214. doi: 10.1016/j.neuint.2006.04.005
Prinz, M., Erny, D., Hagemeyer, N. (2017). Ontogeny and homeostasis of CNS myeloid cells. Nat. Immunol. 18, 385–392. doi: 10.1038/ni.3703
Rock, R. B., Gekker, G., Hu, S., Sheng, W. S., Cheeran, M., Lokensgard, J. R., et al. (2004). Role of microglia in central nervous system infections. Clin. Microbiol. Rev. 17, 942–964, table of contents. doi: 10.1128/CMR.17.4.942-964.2004
Seshadri, S., Pope, R. L., Zenewicz, L. A. (2018). Glucocorticoids Inhibit Group 3 Innate Lymphocyte IL-22 Production. J. Immunol. 201 (4), 1267–1274. doi: 10.4049/jimmunol.1800484
Shane, S. J., Riley, C. (1953). Tuberculous meningitis: combined therapy with cortisone and antimicrobial agents. New Engl. J. Med. 249, 829–834. doi: 10.1056/NEJM195311192492101
Shao, B.-Z., Wang, S.-L., Pan, P., Yao, J., Wu, K., Li, Z.-S., et al. (2019). Targeting NLRP3 Inflammasome in Inflammatory Bowel Disease: Putting out the Fire of Inflammation. Inflammation 42, 1147–1159. doi: 10.1007/s10753-019-01008-y
Song, N., Li, T. (2018). Regulation of NLRP3 Inflammasome by Phosphorylation. Front. Immunol. 9, 2305–2305. doi: 10.3389/fimmu.2018.02305
Spanos, J. P., Hsu, N. J., Jacobs, M. (2015). Microglia are crucial regulators of neuro-immunity during central nervous system tuberculosis. Front. Cell. Neurosci. 9, 182. doi: 10.3389/fncel.2015.00182
Su, W. J., Zhang, T., Jiang, C. L., Wang, W. (2018). Clemastine Alleviates Depressive-Like Behavior Through Reversing the Imbalance of Microglia-Related Pro-inflammatory State in Mouse Hippocampus. Front. Cell. Neurosci. 12, 412. doi: 10.3389/fncel.2018.00412
Swanson, K. V., Deng, M., Ting, J. P. Y. (2019). The NLRP3 inflammasome: molecular activation and regulation to therapeutics. Nat. Rev. Immunol. 19, 477–489. doi: 10.1038/s41577-019-0165-0
Thwaites, G. E., Nguyen, D. B., Nguyen, H. D., Hoang, T. Q., Do, T. T., Nguyen, T. C., et al. (2004). Dexamethasone for the treatment of tuberculous meningitis in adolescents and adults. New Engl. J. Med. 351, 1741–1751. doi: 10.1056/NEJMoa040573
Wang, S., Wang, J., Wei, H., Gu, T., Wang, J., Wu, Z., et al. (2020). Genistein Attenuates Acute Cerebral Ischemic Damage by Inhibiting the NLRP3 Inflammasome in Reproductively Senescent Mice. Front. Aging Neurosci. 12, 153. doi: 10.3389/fnagi.2020.00153
Wang, Z., Zhang, S., Xiao, Y., Zhang, W., Wu, S., Qin, T., et al. (2020). NLRP3 Inflammasome and Inflammatory Diseases. Oxid. Med. Cell. Longevity 2020, 4063562. doi: 10.1155/2020/4063562
Wolf, S. A., Boddeke, H. W., Kettenmann, H. (2017). Microglia in Physiology and Disease. Annu. Rev. Physiol. 79, 619–643. doi: 10.1146/annurev-physiol-022516-034406
Xue, Z., Zhang, Z., Liu, H., Li, W., Guo, X., Zhang, Z., et al. (2019). lincRNA-Cox2 regulates NLRP3 inflammasome and autophagy mediated neuroinflammation. Cell Death Differ. 26, 130–145. doi: 10.1038/s41418-018-0105-8
Yang, Q., Luo, J., Lv, H., Wen, T., Shi, B., Liu, X., et al. (2019). Pulegone inhibits inflammation via suppression of NLRP3 inflammasome and reducing cytokine production in mice. Immunopharmacol. Immunotoxicol. 41, 420–427. doi: 10.1080/08923973.2019.1588292
Yang, C., Shi, S., Su, Y., Tong, J. S., Li, L. (2020). P2X7R promotes angiogenesis and tumour-associated macrophage recruitment by regulating the NF-κB signalling pathway in colorectal cancer cells. J. Cell. Mol. Med. 24, 10830–10841. doi: 10.1111/jcmm.15708
Yu, Y., Feng, S., Wei, S., Zhong, Y., Yi, G., Chen, H., et al. (2019). Extracellular ATP activates P2X7R-NF-κB (p65) pathway to promote the maturation of bone marrow-derived dendritic cells of mice. Cytokine 119, 175–181. doi: 10.1016/j.cyto.2019.03.019
Yue, N., Huang, H., Zhu, X., Han, Q., Wang, Y., Li, B., et al. (2017). Activation of P2X7 receptor and NLRP3 inflammasome assembly in hippocampal glial cells mediates chronic stress-induced depressive-like behaviors. J. Neuroinflamm. 14, 102. doi: 10.1186/s12974-017-0865-y
Zeng, X., Li, Y., Ma, S., Tang, Y., Li, H. (2020). Phoenixin-20 Ameliorates Lipopolysaccharide-Induced Activation of Microglial NLRP3 Inflammasome. Neurotoxicity Res. 38 (3), 785–792. doi: 10.1007/s12640-020-00225-w
Keywords: CNS tuberculosis infection, microglia, NLRP3 inflammasome, caspase-1, NF-κB, P2X7 receptor
Citation: Xie Z, Hui H, Yao Q, Duan Y, Li W, Cheng Y, Zhang M, Tian Y and Zhao G (2021) By Regulating the NLRP3 Inflammasome Can Reduce the Release of Inflammatory Factors in the Co-Culture Model of Tuberculosis H37Ra Strain and Rat Microglia. Front. Cell. Infect. Microbiol. 11:637769. doi: 10.3389/fcimb.2021.637769
Received: 04 December 2020; Accepted: 23 March 2021;
Published: 13 April 2021.
Edited by:
Haider H. Dar, University of Pittsburgh, United StatesReviewed by:
Jon Audia, University of South Alabama, United StatesAshu Sharma, University at Buffalo, United States
Copyright © 2021 Xie, Hui, Yao, Duan, Li, Cheng, Zhang, Tian and Zhao. This is an open-access article distributed under the terms of the Creative Commons Attribution License (CC BY). The use, distribution or reproduction in other forums is permitted, provided the original author(s) and the copyright owner(s) are credited and that the original publication in this journal is cited, in accordance with accepted academic practice. No use, distribution or reproduction is permitted which does not comply with these terms.
*Correspondence: Gang Zhao, zhaogang@nwu.edu.cn; Ye Tian, chhty@sina.com
†These authors have contributed equally to this work
‡These authors have contributed equally to this work