- 1Laboratório de Imunomodulação e Protozoologia, Instituto Oswaldo Cruz, Fundação Oswaldo Cruz, Rio de Janeiro, Brazil
- 2Laboratório de Bioquímica de Tripanosomatídeos, Instituto Oswaldo Cruz, Fundação Oswaldo Cruz, Rio de Janeiro, Brazil
- 3Laboratório de Patologia, Instituto Oswaldo Cruz, Fundação Oswaldo Cruz, Rio de Janeiro, Brazil
- 4National Institute of Science and Technology on Neuroimmunomodulation, Oswaldo Cruz Institute, Oswaldo Cruz Foundation, Rio de Janeiro, Brazil
- 5Laboratório de Produtos Naturais e Espectrometria de Massas, Instituto de Biociências, Universidade Federal de Mato Grosso do Sul, Campo Grande, Brazil
- 6Laboratório de Parasitologia Humana, Instituto de Biociências, Universidade Federal de Mato Grosso do Sul, Campo Grande, Brazil
Treatment of leishmaniasis is a challenging subject. Although available, chemotherapy is limited, presenting toxicity and adverse effects. New drugs with antileishmanial activity are being investigated, such as antiparasitic compounds derived from plants. In this work, we investigated the antileishmanial activity of the biflavonoid amentoflavone on the protozoan Leishmania amazonensis. Although the antileishmanial activity of amentoflavone has already been reported in vitro, the mechanisms involved in the parasite death, as well as its action in vivo, remain unknown. Amentoflavone demonstrated activity on intracellular amastigotes in macrophages obtained from BALB/c mice (IC50 2.3 ± 0.93 μM). No cytotoxicity was observed and the selectivity index was estimated as greater than 10. Using BALB/c mice infected with L. amazonensis we verified the effect of an intralesional treatment with amentoflavone (0.05 mg/kg/dose, in a total of 5 doses every 4 days). Parasite quantification demonstrated that amentoflavone reduced the parasite load in treated footpads (46.3% reduction by limiting dilution assay and 56.5% reduction by Real Time Polymerase Chain Reaction). Amentoflavone decreased the nitric oxide production in peritoneal macrophages obtained from treated animals. The treatment also increased the expression of ferritin and decreased iNOS expression at the site of infection. Furthemore, it increased the production of ROS in peritoneal macrophages infected in vitro. The increase of ROS in vitro, associated with the reduction of NO and iNOS expression in vivo, points to the antioxidant/prooxidant potential of amentoflavone, which may play an important role in the balance between inflammatory and anti-inflammatory patterns at the infection site. Taken together these results suggest that amentoflavone has the potential to be used in the treatment of cutaneous leishmaniasis, working as an ally in the control and development of the lesion.
Introduction
Leishmaniases are a complex of infectious diseases caused by several species of protozoa from the genus Leishmania, which are transmitted between vertebrate and invertebrate hosts by the bite of infected female sandflies. The clinical manifestations range from more severe forms such as visceral leishmaniasis (VL) and mucous leishmaniasis (ML) to more common and benign forms as cutaneous leishmaniasis (CL). In general, the form and severity of the disease depends on the infecting species combined with the host genetics and the immune response generated by the host (Colmenares et al., 2002). With a wide global distribution, leishmaniases put at risk more than one billion people living in endemic areas. It is estimated that 30,000 new cases of visceral leishmaniasis and more than one million cases of cutaneous leishmaniasis occur annually (World Health Organization, 2020).
Pentavalent antimonials and amphotericin B are the most widely used drugs for the treatment of leishmaniases. They have important limitations such as high toxicity, adverse effects and parenteral administration. To further aggravate the current scenario of leishmaniases chemotherapy, treatment varies in effectiveness as a result of the variation in the intrinsic sensitivity of the different Leishmania species, acquired resistance and differences in the host immune response (Croft and Coombs, 2003). In 2010, the World Health Organization (WHO) promoted the use of local therapies to treat cases of uncomplicated CL (World Health Organization, 2010) and the intralesional approach was included in the CL treatment recommendations in Brazil (Brasil, 2017), after studies in the country that had already shown the benefits of CL local treatment (Oliveira-Neto et al., 1997; de Oliveira Duque et al., 2016). These studies highlighted not only the decrease in adverse effects and toxicity, but also the cost and accessibility of treatment, especially in rural areas (Añez et al., 2018; Arboleda et al., 2019; de Oliveira Duque et al., 2019). Therefore, intralesional treatment can offer advantages such as simplicity, efficiency, and safety, especially when the patient conditions do not allow systemic chemotherapy (de Oliveira Duque et al., 2019).
In the search for novel and effective treatments to combat leishmaniases, natural products have been used. In addition to exhibiting potential as therapeutic compounds, natural products may also contribute to the development of new drugs based on their chemical structures (Gervazoni et al., 2020). The biflavonoid amentoflavone (3’, 8’’ - Biapigenin) is an apigenin dimer linked by a C3’-C8’’ covalent bond. Many biological amentoflavone activities have been described, both in vitro and in vivo.
Several activities and effects have been described for this flavonoid, including a number of studies investigating its anti-inflammatory (Cao et al., 2017), antioxidant (Saroni Arwa et al., 2015; Su et al., 2019), anti-tumor (Yen et al., 2018; Hsu et al., 2019), neuroprotective (Chen et al., 2018) and, recently, anti-SARS-CoV-2 (Mishra et al., 2020) properties. Antileishmanial activity has also been investigated. The biflavonoid was active against promastigotes (Njock et al., 2017) and in a previous study, our group showed that amentoflavone has a leishmanicidal action on intracellular forms, independent of nitric oxide (NO) production (Rizk et al., 2014).
In this study, we evaluated the leishmanicidal activity of amentoflavone in vivo, using the intralesional route after BALB/c mice infection with Leishmania amazonensis promastigotes, and searched for its mechanism of action. Intralesional treatment with amentoflavone showed no toxicity and reduced the parasitic burden in infected animals, revealing its potential use in the treatment of cutaneous leishmaniasis. Furthemore, amentoflavone treatment increased reactive oxygen species (ROS) production in murine macrophages, consequently reducing the number of intracellular amastigote forms. These results points for the amentoflavone prooxidant effect as its mechanism of action against Leishmania sp infection.
Materials and Methods
Test Compounds
Amentoflavone was purchased from Sigma-Aldrich® (HPLC grade ≥ 98%). A stock solution was prepared in DMSO (Sigma-Aldrich) and then diluted in the culture medium to obtain the concentrations used in the in vitro assays. For the in vivo experiments, amentoflavone was solubilized directly in the administration vehicle, as follows: 10% Ethanol + 10% Kolliphor®EL (Sigma-Aldrich) + 1% DMSO in phosphate saline (PBS Buffer) pH 7.2. The N-methyl glucamine (Glucantime®, Sanofi-Aventis), was kindly provided by the pharmacy of the National Institute of Infectology/FIOCRUZ, Rio de Janeiro, and diluted in PBS when necessary.
Parasites
Amastigotes of L. amazonensis (IFLA/BR/1967/PH8) were isolated from cutaneous lesions of BALB/c mice and maintained as promastigotes at 26°C in liquid medium LIBHIT (Costa and Lagrande, 1981). The medium was supplemented with 10% Fetal Bovine Serum (FBS, Gibco), 10,000 U/L penicillin and 10 mg/L streptomycin (Sigma-Aldrich). Promastigote forms grown in axenic culture were used for infection. Fresh cultures were unfreezed every six passages and always used in the stationary phase (fifth day of growth) to ensure infectivity.
Intracellular Amastigote Activity
Thioglycollate-elicited peritoneal macrophages from BALB/c mice were seeded in 24-well plates containing coverslips at a concentration of 2 × 105 cells/well in complete RPMI medium - RPMI-1640 medium (Sigma-Aldrich) supplemented with 10% FBS (Gibco), 200 mM L-glutamine, 10,000 U/L penicillin and 10 mg/L streptomycin (Sigma-Aldrich). After adhesion, plates were washed with PBS to remove non-adherent cells and kept overnight at 34°C /5% CO2. Subsequently, macrophages were infected with promastigote forms of L. amazonensis 10:1 (parasite/macrophage) in the stationary growth phase for 6 h, and then the culture was washed with PBS pH 7.0 to remove promastigotes from the supernatant. After that, different concentrations of amentoflavone (0–11.14 μM) were added to wells containing infected macrophages, and plates were incubated at 37°C/5% CO2 for 72 h. After Bouin-fixing and Giemsa-staining, cells were analyzed by light microscopy. The number of intracellular amastigote forms was determined in 200 macrophages/coverslip. The concentration that inhibits 50% of growth (IC50) was calculated by dose-response and nonlinear regression analysis, using the GraphPad Prism® 7.0 software.
Cytotoxicity
Peritoneal macrophages (1 × 105 cells/well) in complete RPMI medium were seeded in 96-well plates and incubated overnight at 37°C/5% CO2. After removing non-adherent cells, macrophages were treated with different concentrations of amentoflavone (0–22.3 µM) and incubated for another 72 h. Cell viability was determined using MTT colorimetric assay, as follows. After 4 h of incubation in the dark at 37°C with MTT, the culture supernatant was completely removed and 100 µl of DMSO added per well. For the complete dissolution of formazan crystals formed in viable cells, the plates were shaken for 15 min. The absorbance was measured at 570 nm using a spectrophotometer (EZ Read 400®, Biochrom). The CC50 value was determined by logarithmic regression analysis using GraphPad Prism® 7.0. The selectivity index (SI) was calculated as macrophages CC50/intracellular amastigotes IC50.
In Vivo Experimental Protocol
Female BALB/c mice (4–6 weeks-old) ranging from 20 to 25 g were randomly distributed into five groups. Animals of the groups 1 to 3 were subcutaneously (SC) infected with 1 × 104 promastigote forms of L. amazonensis in the left hind footpad, while animals of the groups 4 and 5 were kept uninfected. As soon as the lesions appeared, on the 28th day after the inoculum, all animals were weighed and the thickness of their footpads, right and left, was measured with a caliper (0.1 mm, Schnelltäster, HC Kroplin). Then, intralesional treatment (IL) with amentoflavone (0.5 mg/kg/dose) was initiated, through subcutaneous injection in the lesion site (groups 1 and 4). N-metil glucamine (64 mg Sb5+/kg/dose) was used as a positive control, and amentoflavone vehicle (10% Ethanol/10% Cremophor/1% DMSO/PBS) as a negative control of treatments (groups 3 and 5, respectively). All treatments were performed in five doses, administered 4 days apart. The animals’ weight and footpad thickness were monitored weekly. One week after the last treatment dose, animals were weighed and the lesion was measured just before being euthanized with Xylazine Hydrochloride (30 mg/kg, Syntec) associated with Ketamine Hydrochloride (300 mg/kg, Syntec). After euthanasia, tissue and blood samples were collected for subsequent analysis.
Toxicology
Immediately after euthanasia, mice blood was collected (1 ml) via cardiac puncture and centrifuged to separate the serum. Sera were sent to the Animal Clinical Analysis Core Facilities of the Institute of Science and Technology in Biomodels (ICTB, Fiocruz RJ) to measurement of aspartate transaminase (AST), alanine transaminase (ALT), and creatinine in a Vitros 250 equipment (Ortho clinical - Jonhson & Jonhson).
Parasite Load in the Lesion Site
Parasite load was estimated by a limiting dilution assay (LDA) (Cardoso et al., 2010). In brief, footpads were aseptically removed, weighed, and macerated in LIBHIT medium. Several sequential 1:2 dilutions were plated in 96-well “U” round-bottom plates, which were incubated at 26°C. Between the 5th and 10th day, the plates were analyzed by visualization in an inverted microscope (Axiovert 25®, Zeiss) to determine the last well containing at least one parasite in its promastigote form. The parasite load of footpads was calculated from the last dilution that contained parasites, divided by the weight of the respective organ. The results were expressed as the number of parasites per gram of tissue following the method described by Taswell (1984). The percentage of reduction in the parasite load was calculated considering the group treated with vehicles as having 100% of infection.
Quantitative PCR (qPCR) was used to confirm the parasite load in the lesion. After DNA extraction by a routine phenol-chloroform technique (Sambrook and Russell, 2006), 20 ng of total DNA were amplified using a specific primer for Leishmania sp. kDNA3 (forward 5’GGGTAGGGGCGTTCTGC3’, reverse 5’CCCGGCCTATTTTACACCAACC3’) (Weirather et al., 2011), as well as for the mouse β-actin endogenous control (forward 5’CTTGGCTGAACCATCAC3’, reverse 5’GGTCCTCATCGTTTAGCA3’) (Giulietti et al., 2001). Amplification was performed in a QuantStudio 3 equipment (Applied Biosystems) using GoTaq® PCR Master Mix (Promega), with 250 nM of kDNA3 or 100 nM of β-actin primers per reaction. PCR conditions were as follows: hold at 95°C for 2 min, followed by 40 cycles of 95°C for 15 s and 62°C for 1 min followed by a dissociation curve. Standard curves were generated from 10-fold serial dilutions (100 ng–1 pg) of axenic Leishmania DNA and used to calculate parasite concentration in the samples.
Histopathological Analysis
The lesion site, draining lymph nodes, spleen and liver were routinely processed for histopathological analysis. Five micrometers thick paraffin sections were obtained in a rotating microtome (HM 360, Microm) and stained using the hematoxylin-eosin (HE) or Giemsa techniques. Photomicrographs were obtained using a microscope (Axioplan 2, Zeiss) with image capture (AxioCam ERc 5s, Zeiss).
Nitric Oxide Production by Peritoneal Macrophages
Peritoneal macrophages collected from all groups were plated in 96-well plates at a concentration of 5 × 105 cells/well in RPMI complete medium and incubated for 48 h, at 37°C/5% CO2. Cells were stimulated with total L. amazonensis antigen (1 µg/ml) or Lipopolysaccharide (LPS, 1 µg/ml) (positive control). Unstimulated cells were maintained as negative control. Cell culture supernatants were harvested and used to evaluate nitric oxide production by the Griess reaction (Rizk et al., 2014).
Local Expression of iNOS and Nrf2-Related Genes
Total RNA was extracted from mouse footpads using a standard TRI Reagent® (Sigma-Aldrich) protocol, followed by DNAse treatment. RNA concentration and quality were determined by spectrophotometry (NanoDrop One, ThermoScientific). Complementary DNA (cDNA) was synthesized using iScript™ cDNA Synthesis kit (Bio-Rad) and 1 µg of total RNA, according to the manufacturer’s recommendations. Then, qPCR was performed on QuantStudio 3 equipment (Applied Biosystems). The sequences of the specific primers targeting mouse genes were Nfe2l2 - nuclear factor, erythroid derived 2, like 2, transcript variant 1 (Nrf2) (forward 5’TCACACGAGATGAGCTTAGGGCAA3’, reverse 5’TACAGTTCTGGGCGGCGACTTTAT3’), Hmox1 - heme oxygenase 1 (HO-1) (forward 5’CCCAAAACTGGCCTGTAAAA 3’, reverse 5’CGTGGTCAGTCAACATGGAT3’), L-ferritin (forward 5’TTCCAGGATGTGCAGAAGCC3’, reverse 5’AAGAGGGCCTGATTCAGGTTC3’) and Actb - actin, beta (β-actin) (forward 5’AGCTGCGTTTTACACCCTTT3’, reverse 5’AAGCCATGCCAATGTTGTCT3’) (Tomiotto-Pellissier et al., 2018), as well as Nos2 - nitric oxide synthase 2, inducible, transcript variant 1 (iNOS) (foward 5’GGATCTTCCCAGGCAACCA3’, reverse 5’CAATCCACAACTCGCTCCAA3’) and Rplp0 - ribosomal protein, large, P0 (Rplp0) (foward 5’GCCAGCTCAGAACACTGGTCTA3’, reverse 5’ATGCCCAAAGCCTGGAAGA3’) (Cardoso et al., 2020). The reaction mixtures contained 10 µl of GoTaq® qPCR Master Mix (Promega), 20 ng of cDNA for Nrf2, HO-1 and β-actina targets, and 100 ng of cDNA for Rplp0 and iNOS targets; 100 nM of Nrf2, HO-1, ferritin and β-actin primers, 600 nM of iNOS primer and 900 nM of Rplp0 primer in a final volume of 20 µl. Cycling conditions were 2 min at 95°C, 40 cycles of 15 s at 95°C and 1 min at 60°C (HO-1, ferritin, β-actin) or 62°C (Nrf2, β-actin, Rplp0 and iNOS), followed by the dissociation curve. The results were analyzed with QuantStudio™ Design & Analysis software (Applied Biosystems). The relative quantification of mRNA of each gene was estimated by the 2-ΔΔCT method, with the β-actin and Rplp0 mouse genes as endogenous control.
ROS Measurement
The levels of intracellular ROS in macrophages infected and treated with amentoflavone were measured using the permeable dye 2’, 7’ - dichlorofluorescein diacetate (H2DCFDA). In the cell cytoplasm, this dye is deacetylated by cellular esterases and the compound formed oxidized by the ROS present, resulting in a highly fluorescent compound. Peritoneal macrophages obtained as described above were seeded in black 96-well plates at a density of 2 × 106 cells/well. After 30 min, the plates were washed with PBS to remove non-adherent cells and the adhered cells kept at 34°C/5% CO2 overnight. Promastigote forms of L. amazonensis were added (10 parasites per cell) and the cells incubated for 5 h at 34°C. The cells were washed twice with PBS and the plate was kept an additional hour at 34°C before treatment. The concentrations of 1.15 μM (1/2 IC50), 2.3 μM (IC50), 4.6 μM (2× IC50), or 9.2 μM (4× IC50) of amentoflavone were added and the plates incubated for 24, 48 or 72 h. Antimycin B (10 mM) and glucose (60 mM) + glucose oxidase (20 units/ml) (G/GO) were used as positive controls. After the incubation times, medium was discarded and the macrophages washed once with Hank’s balanced salt solution (HBSS). Then, cells were incubated with H2DCFDA (20 μM) for 30 min at 37°C. Fluorescence was measured by fluorescence spectroscopy (SpectraMax M2, Molecular Devices), at 507 nm and 530 nm (excitation/emission wavelengths).
Amentoflavone In Silico Toxicity Evaluation
To predict the toxicity of amentoflavone, the pkCSM tool was used (Pires et al., 2015). The SMILES (simplified molecular-input line-entry system) used for in silico analysis was as follows: C1=CC(=CC=C1C2=CC(=O)C3=C(O2)C(=C(C=C3O)O)C4=C(C=CC(=C4)C5=C C(=O)C6=C(C=C(C=C6O5)O)O)O)O.
Ethics Statement
Female BALB/c mice (4–6 weeks-old) were obtained from the Institute of Science and Technology in Biomodels (ICTB, Fiocruz) and kept in an experimental animal facility with controlled room temperature, water, and food ad libitum. All procedures involving the use of animals were previously evaluated and approved by the Animal Use Ethics Committee of the Oswaldo Cruz Institute (CEUA/IOC), under licenses N°. L-053/2016 and N°. L-026/2019.
Results
Amentoflavone Was Active Against L. amazonensis Intracellular Amastigotes
The treatment with amentoflavone reduced the amount of intracellular amastigotes in murine macrophages. The two highest tested concentrations (5.57 and 11.14 µM) reduced in 57% the total number of amastigotes, with no significant difference between them (t-test, p = 0.9244) (Figure 1A). The IC50 calculated from a non-linear regression curve was 2.3 ± 0.93 µM (Figure 1B).
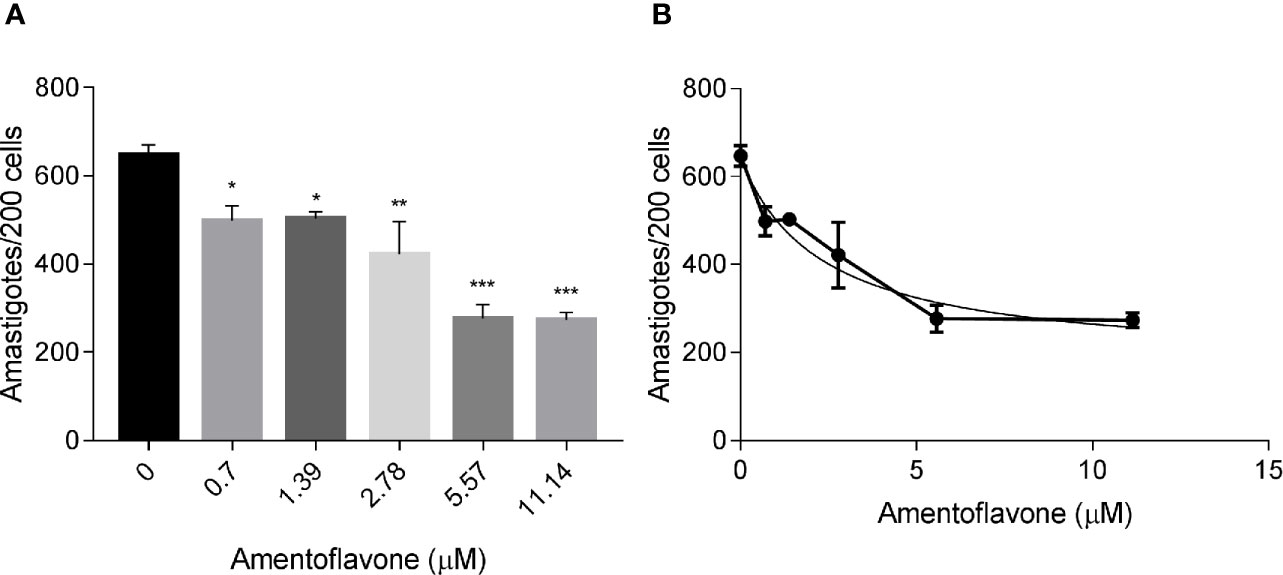
Figure 1 In vitro amentoflavone effect on macrophages infected with L. amazonensis. BALB/c mice peritoneal macrophages were infected with L. amazonensis promastigotes and treated with amentoflavone (0–11.14 μM) for 72 h. (A) Total intracellular amastigotes in 200 macrophages and (B) Dose-response curve of amentoflavone. Mock-treated infected cells were used as a control (0 μM). The columns represent the mean ± S.E.M. of quadruplicates. Significant difference (1-way ANOVA, followed by Tukey post-test) in relation to the mock-treated control *p < 0.05, **p < 0.01, ***p < 0.0001.
Amentoflavone Presented No Signs of Toxicity In Silico, In Vitro or In Vivo
To perform the in silico toxicity analysis of amentoflavone, we used the pkCSM tools (Pires et al., 2015). Amentoflavone showed absence of Ames toxicity, hepatotoxicity, skin sensitization, Minnow toxicity, and non-inhibitor of hERG I (Supplementary Table I), suggesting that amentoflavone is safe. In fact, amentoflavone was not cytotoxic to peritoneal macrophages at the tested concentrations for a period of 72 h. The viability of cells treated with the highest tested concentration (22.3 µM) was not significantly different from the mock-treated control cells. The selectivity index in vitro was estimated to be greater than 10, but it could not be calculated since the higher concentration essayed was not toxic to cells. When the drug was intralesionally injected in BALB/c infected mice, there was no weight loss and the survival rate was 100% throughout the treatment. Intralesional treatment with amentoflavone did not show hepatic or renal toxicity since no alterations were observed in the biochemical markers ALT, AST and creatinine. Similar results were observed in animals treated with the reference drug (Glucantime®) and in mock-treated controls.
Amentoflavone Reduced Parasitic Burden in L. amazonensis-Infected Mice
Intralesional treatment with amentoflavone (0.05 mg/kg/dose every 4 days in a total of 5 doses) led to a significant reduction (p = 0.0014) in the size of the lesion just after the last dose of subcutaneous treatment, 21 days after the start of treatment, in comparison to the mock-treated group. Nevertheless, after the end of treatment, lesions regained their growth, reaching the same size as the control, one week later. The group that received intralesional Glucantime® maintained the same lesion size throughout the treatment, reaching the end of it with a significantly smaller lesion than the control group treated with vehicle (p < 0.0001) (Figure 2A).
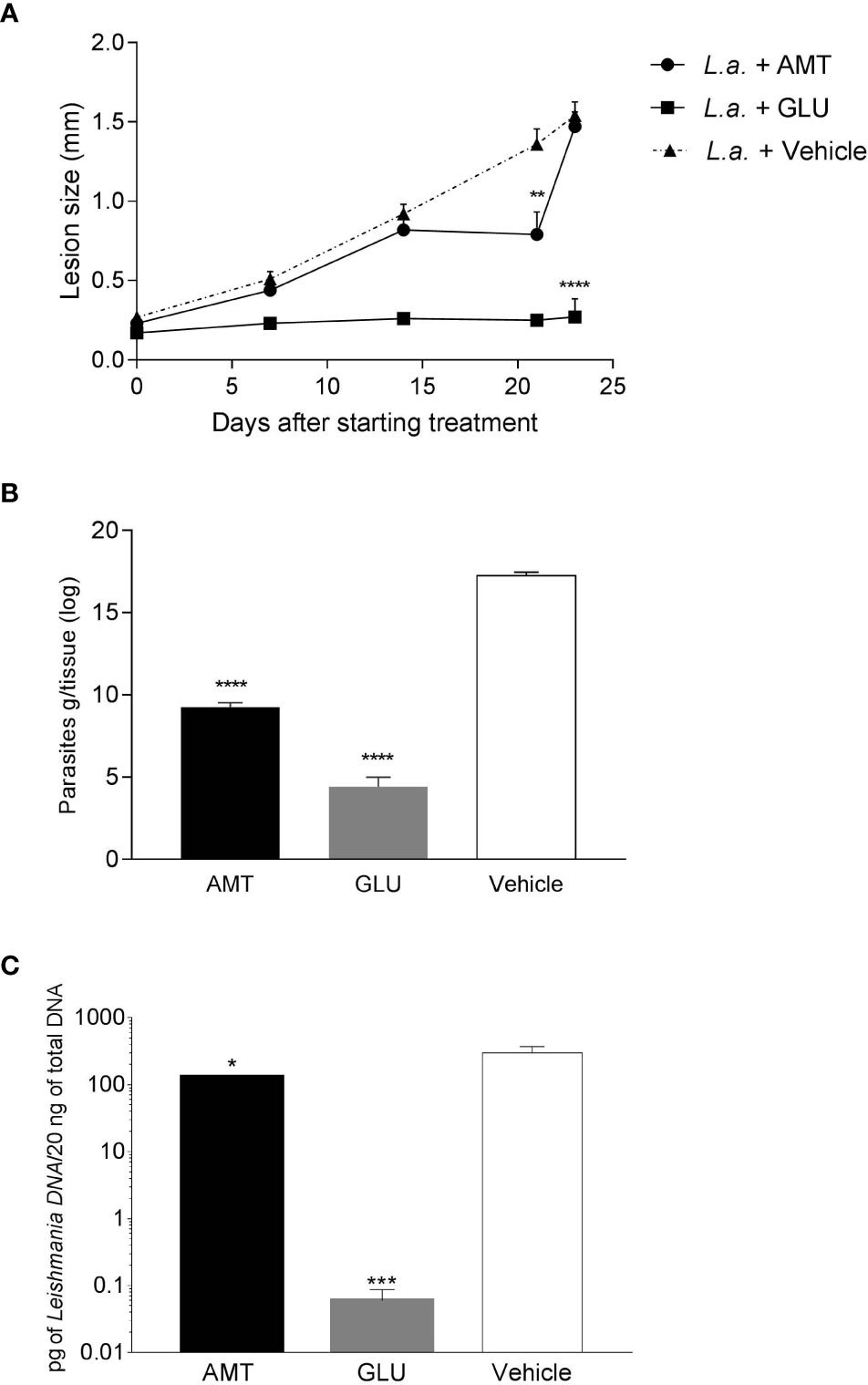
Figure 2 Effect of intralesional amentoflavone treatment on L. amazonensis-infected BALB/c mice. (A) Lesion size kinetics. The size of the lesions was monitored weekly from the beginning of treatment until 1 week after the last treatment dose by measuring the footpad with a caliper, with 0.1 mm sensitivity. The data shown represents the average of the difference between the infected footpad and the uninfected contralateral footpad ± S.E.M. of 10 animals per group. (B) Parasite load estimated by limiting dilution assay. (C) Parasite load estimated by qPCR. Points and bars represent the mean ± S.E.M. of five animals per group. *p < 0.05, **p < 0.01, ***p < 0.001, ****p < 0.0001: significant difference in relation to the mock-treated control (1-way ANOVA, followed by Tukey post-test). L.a., Animals infected with L. amazonensis; AMT, Amentoflavone; GLU, Glucantime.
Seven days after the last dose of intralesional treatment, animals were euthanized and the parasite load at the lesion site measured by a limiting dilution assay (LDA) and quantitative PCR (qPCR). Amentoflavone treatment significantly reduced the number of viable parasites by 46.3% and parasite DNA by 56.1% in the footpad, when compared to vehicle-treated animals (p < 0.0001 and p = 0.0292, for ADL and qPCR, respectively). Glucantime® induced a strong reduction in the parasite load (74.4% and 99.9%, for ADL and qPCR, respectively) as compared to mock-treated animals (Figures 2B, C and Supplementary Table II). The two techniques used to calculate parasite load presented a positive correlation (r = 0.825 on a Spearman test; p = 0.0003).
Histological Findings
Histological evaluation of footpad, draining popliteal lymph node, liver and spleen of non-infected animals did not show evident histopathological alterations. However, discrete foci of inflammatory infiltrate were observed in the footpad, with the presence of mast cells and a slight increase in collagen fibers, possibly due to the trauma of the subcutaneous injections of the vehicle. On the other hand, footpads of the infected mock-treated animals showed an intense parasitism among the muscle fibers; thinning of the conjunctiva between the superficial dermis and the epidermis; mast cells in the middle of the dermis and the first muscle layer; macrophages intensely parasitized and presence of an inflammatory infiltrate predominantly composed of polymorphonuclear cells (Figure 3A). The lymph nodes showed intense parasitism and presence of giant cells (Figure 3B). No histopathological alterations were observed in the liver and spleen of these animals. The infected animals treated with 0.05 mg/kg/dose of amentoflavone also showed moderate to intense parasitism in the footpad, with a similar inflammatory infiltrate (Figure 3C). The lymph nodes presented amastigotes and strong lymphoid activation and proliferation of germinal centers, with follicular hiperplasia (Figure 3D). The liver and spleen showed no parasitism, with the later showing a greater lymphoid activation and several of germinal centers in comparison to the infected mock-treated group. Footpad of infected animals treated with 64 mg Sb5+/kg/dose of Glucantime® showed low parasitism; fibrosis foci; presence of fibroblasts, macrophages, mast cells, and some eosinophils (Figure 3E). The lymph node showed low parasitism; presence of giant cells, as well as many polymorphonuclear cells and some mast cells (Figure 3F). The spleen, on the other hand, did not show parasitism. No changes were found in the liver of these animals.
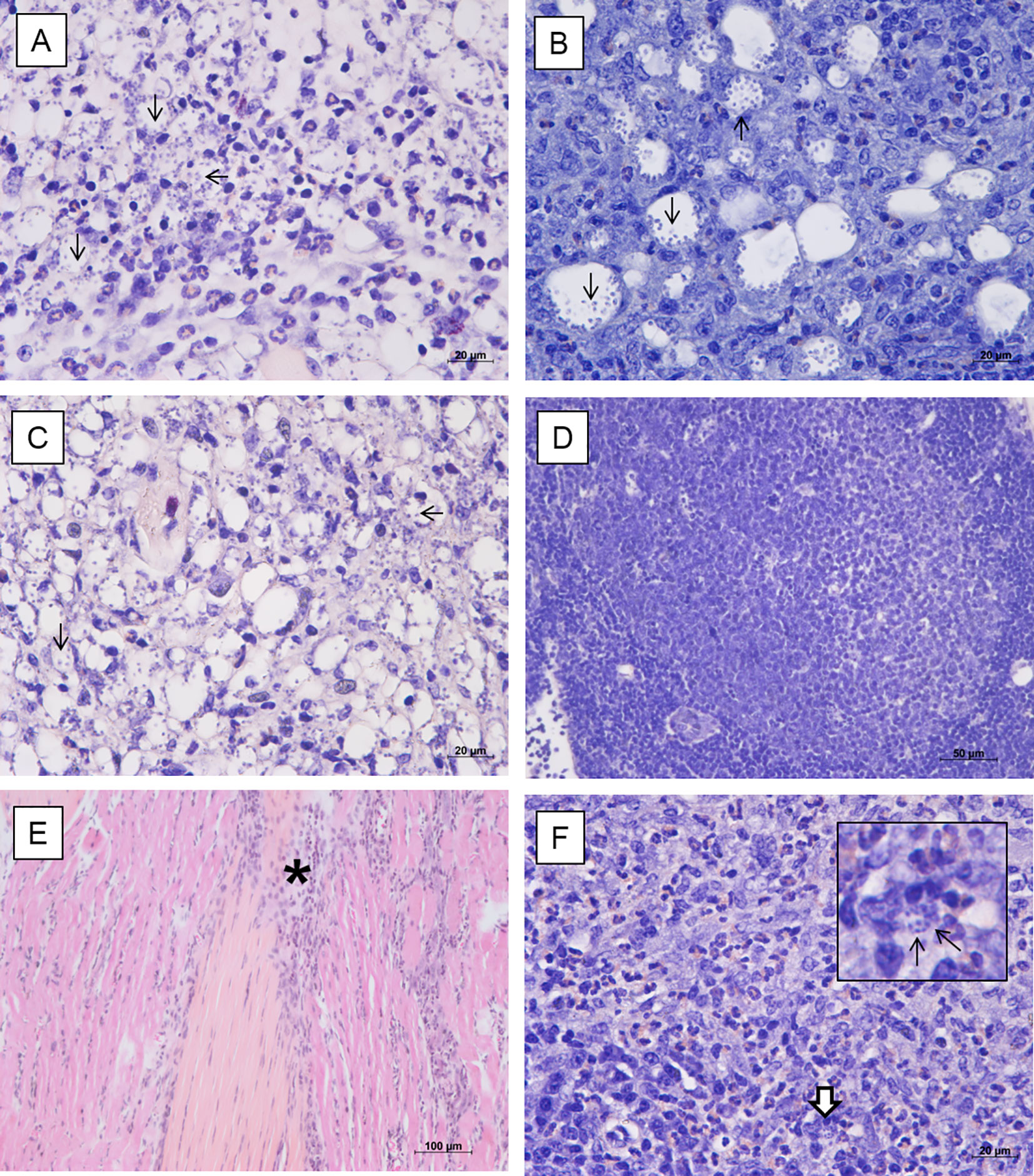
Figure 3 Histopathological analysis of BALB/c mice treated intralesionally. Photomicrographs of the footpad and lymph nodes of BALB/c mice infected with L. amazonensis and treated with vehicle (A, B), amentoflavone 0.5 mg/kg/dose (C, D) or Glucantime 64 mg Sb5+/kg/dose (E, F). (A) Inflammatory infiltrate with polymorphonuclear cells and intense parasitism (black arrows) in the footpad (hematoxylin and eosin); (B) Infected macrophages (black arrows) in the lymph node (Giemsa). (C) Amastigotes in footpad (black arrow) (hematoxylin and eosin). (D) Hyperplastic germinal center in the lymph node with follicular hyperplasia (Giemsa). (E) Connective tissue thickening among the muscles and inflammatory infiltrate (asterisk) in the footpad (hematoxylin and eosin). (F) Polymorphonuclear cells (mainly eosinophils), parasitism (detail, white arrow) in the lymph node (Giemsa). Representative images (2 animals/group).
Amentoflavone Exerts Both Antioxidative and Prooxidative Responses
To evaluate whether NO production could be associated with amentoflavone mechanism of action, peritoneal macrophages from BALB/c mice, infected or not with L. amazonensis and treated intralesionally with amentoflavone or Glucantime were stimulated in vitro with soluble antigens of L. amazonensis, LPS (positive control) or medium (negative control). As expected, LPS stimulated-cells produced NO, whereas non-stimulated cells did not. Leishmania antigens were able to stimulate NO production, but peritoneal macrophages taken from mice treated with amentoflavone produced significantly lower NO than cells from mock-treated animals. This decrease occurred in cells derived from both infected and non-infected animals (p = 0.0270 and p = 0.0035, respectively) (Figure 4A). In addition, when iNOS expression in the lesion was evaluated by RT-qPCR, although no significant difference was observed, treated animals presented slightly lower iNOS expression than infected mock-treated animals (Figure 4B).
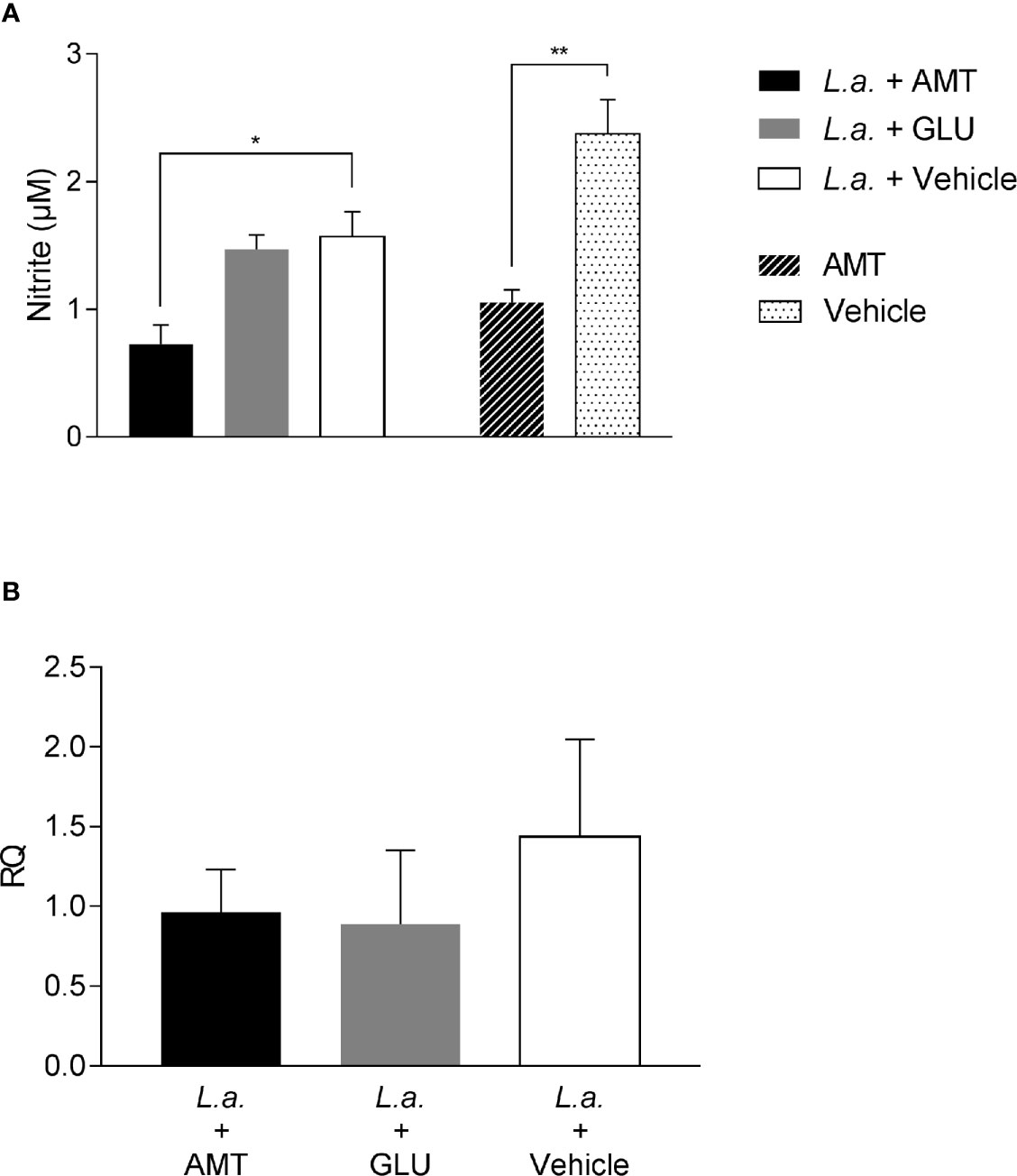
Figure 4 Evaluation of NO production and iNOS expression on amentoflavone-treated mice (A) Nitrite quantification in peritoneal macrophages isolated from L. amazonensis-infected BALB/c, treated or not with amentoflavone intralesionally. Macrophages were stimulated with total L. amazonensis antigen (1 µg/µl) and the amount of nitrite in the cell culture supernatant was measured by the Griess reaction. The bars represent the mean ± S.E.M. of five animals per group. *p < 0.05, **p < 0.01 (Test t). (B) Relative quantification of iNOS mRNA in L. amazonensis-infected BALB/c mouse footpads treated or not with amentoflavone or Glucantime, intralesionally. Expression was estimated by 2-ΔΔCT method, using Rplp0 as a reference gene. The bars represent the mean ± S.E.M. of three animals per group. L.a., Animals infected with L. amazonensis; AMT, Amentoflavone; GLU, Glucantime.
Recently, amentoflavone has been reported as a molecule capable of triggering the Nrf2 activation pathway, a gene responsible for triggering antioxidant responses in the cell (Chen et al., 2018; Wahyudi et al., 2018). Several studies point out to Nrf2 as responsible for the antioxidant effect of leishmanicidal compounds (Tomiotto-Pellissier et al., 2018; Cataneo et al., 2019; Miranda-Sapla et al., 2019). Therefore, the expression of Nrf2, as well as HO-1 and Ferritin genes, both regulated by Nrf2, were investigated in the footpad of animals infected with L. amazonensis and treated with amentoflavone intralesionally. Treatment with amentoflavone did not alter the expression of Nrf2, which was increased in Glucantime®-treated footpads (p = 0.0172). Although Nrf2 expression was not altered at that time point, HO-1 expression in the amentoflavone-treated footpad was significantly reduced (p = 0.0120), as well as Glucantime®-treated footpad (p = 0.0236). The ferritin gene expression was increased both in amentoflavone and Glucantime®-treated groups when compared to the control group (p = 0.0168 and p = 0.0042, respectively) (Figure 5).
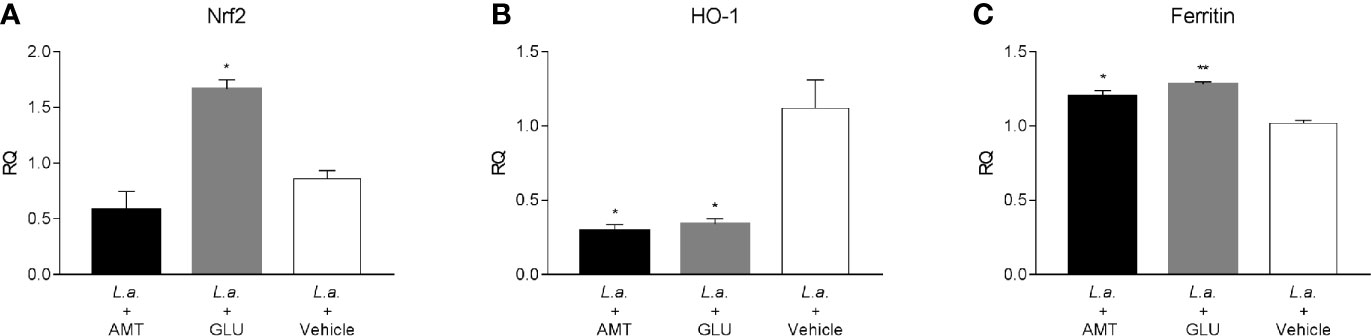
Figure 5 Relative quantification of Nrf2-related genes in L. amazonensis-infected BALB/c mice footpads treated or not with amentoflavone or Glucantime, intralesionally. RT-qPCR analyses were performed to quantify the expression of Nrf2 (A), HO-1 (B), and ferritin (C) genes. Expression was estimated by 2-ΔΔCT method, using Actb as a reference gene. The bars represent the mean ± S.E.M. of three animals per group. Significant differences (1-way ANOVA, followed by Tukey post-test); *p < 0.05; **p < 0.01 in relation to the mock-treated control. Nrf2, nuclear factor, erythroid derived 2, like 2; HO-1, heme oxygenase 1; Feritin, L-ferritin. Actb, actin beta.
Since amentoflavone’s mechanism of action could not be explained by an increase of NO production, the generation of ROS was evaluated in vitro. The ROS production was measured in amentoflavone-treated and non-infected peritoneal macrophages obtained from normal BALB/c mice (Figure 6A). After 24 h of treatment, amentoflavone did not alter the production of ROS. However, after 48 h of treatment, this production was significantly higher in cells treated with 2.3 µM (IC50) (p = 0.0009) or more of amentoflavone (4.6 µM: p = 0.0393, 9.2 µM: p = 0.0280). The increase in ROS was still noticed after 72 h of treatment with the two highest concentrations (4.6µM: p = 0.0022, 9.2 µM: p = 0.0069), but not in cells treated with 2.3 µM, which had the highest production in 48 h. Antimycin B and Glucose/Glucose oxidase, both used as positive controls, presented significantly higher production than non-treated normal cells at all times. When ROS production was evaluated in L. amazonensis-infected macrophages, amentoflavone increased ROS production at 2.3 µM or higher at 24 and 48 h, decreasing at 72 h, when the lower concentration 1.15 µM increased the ROS production in relation to mock-treat infected cells (Figure 6).
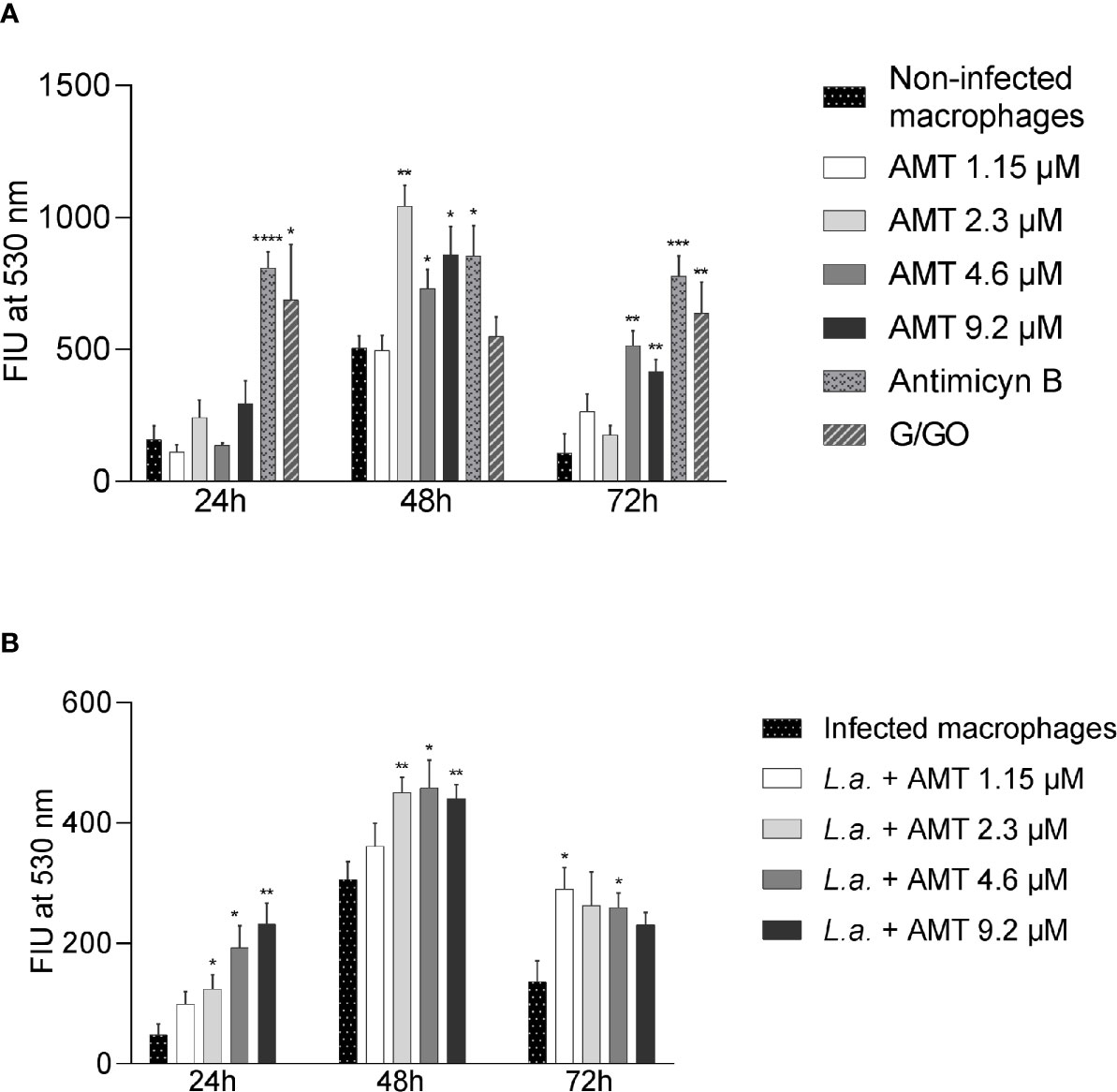
Figure 6 Amentoflavone concentration influence on the ROS production in peritoneal macrophages. Murine peritoneal macrophages (2 × 106) were treated with different concentrations of amentoflavone. (A) Uninfected macrophages treated with amentoflavone in concentrations of 1.15 μM (1/2 IC50), 2.3 μM (IC50), 4.6 μM (2× IC50) or 9.2 μM (4× IC50) for 24, 48, and 72 h. Mock-treated macrophages were used as controls. Antimycin B and Glucose/Glucose oxidase (G/GO) were used as a positive control. (B) Macrophages infected with L. amazonensis and treated with different concentrations of amentoflavone for 24, 48, or 72 h. Infected and mock-treated macrophages were used as controls. The generation of ROS was measured using the fluorescent indicator H2DCFDA. The data were expressed in units of fluorescence intensity (FIU). Significant differences in relation to the control (t test): *p < 0.05, **p < 0.01, ***p < 0.001 and ****p < 0.0001. AMT, Amentoflavone; L.a., L. amazonensis.
Discussion
Amentoflavone action on Leishmania parasites has been controversial. Weniger and collaborators (Weniger et al., 2006) found no activity against L. donovani axenic amastigotes, but our group have shown that amentoflavone can disrupt the membrane potential of mitochondria and effectively kill L. amazonensis promastigotes (manuscript in preparation). When amentoflavone was tested on intracellular amastigote forms of L. amazonensis, it showed antileishmanial activity and a good selectivity index (SI) (Oubada et al., 2014). Corroborating these data, amentoflavone isolated from S. sellowii also showed potential antileishmanial activity on this same Leishmania species and cellular form (Rizk et al., 2014). In this work, we evaluated the activity of commercially acquired amentoflavone on these intracellular amastigote forms, reaching an IC50 value of 2.3 μM, which confirms the antileishmanial activity of this biflavonoid. In addition, in silico data suggested that amentoflavone presents low toxicity. These data was corroborated by an experimental SI greater than 10, pointing out to the safe use of amentoflavone as a chemotherapeutic agent.
In order to evaluate the effect of amentoflavone treatment on cutaneous leishmaniasis in vivo, BALB/c mice were infected with promastigote forms of L. amazonensis and treated via intralesional injection. Once the oral route does not seem to be the preferred form of amentoflavone administration due to its low bioavailability (Liao et al., 2015), in this work a local application was chosen, as already used in the clinic for cutaneous leishmaniasis treatment, in order to prevent systemic metabolism and increase bioavailability at the lesion site. Animals treated either with amentoflavone or Glucantime® showed no signs of toxicity, including no alteration on ALT, AST, and creatinine levels, no decreased body weight or death, corroborating data from the literature (Lee et al., 2018; Liu and Yu, 2018; Shen et al., 2018; Tsai et al., 2018).
Throughout the intralesional treatment of L. amazonensis-infected mice, the amentoflavone-treated group showed an increase in the thickness of the lesion similar to that observed in the mock-treated group. It is interesting to note that, just after the last dose of treatment (21 days post-treatment), amentoflavone-treated mice had their lesion size reduced when compared to the mock-treated group. However, 7 days later, at the end of the experimental protocol, the increase in lesion thickness caught up to the average thickness of the mock-treated group. Although the amentoflavone treatment has not been effective in maintaining the lesions reduction, both LDA, which quantifies only viable parasites, and the real-time PCR, which quantifies parasite DNA, showed a reduction in the parasite load in the lesion of the group treated with amentoflavone (0.05 mg/kg/dose). However, as this reduction was not absolute (between 45% and 56%), the lesion remained active after the last dose and regained its growth. It is possible that the use of higher dosages and/or a longer therapeutic regimen might increase this activity in vivo.
N-metil glucamine treatment maintained the initial thickness of the lesion until the end of the experiment. In fact, the reference drug reduced the parasite load by 74% to 99% compared to mock-treated animals. Despite reducing the amount of parasitic DNA present in infected footpads by almost 100%, treatment with Glucantime did not result in a sterile cure. In fact, studies show that treatment with intralesional Glucantime does not completely eradicate the parasite in BALB/c mice (de Paladi et al., 2012; García et al., 2017; Tamargo et al., 2017). The dose of Glucantime used in our study was based on a guidance of the Ministry of Health in Brazil, which predicts the administration of intralesional non-dilluted Glucantime® through infiltration at the base of the skin lesion, until the area swells (Brasil, 2017). As a result, the volume may vary depending on the affected region and the size of the lesion. In our experimental protocol, for standardization purposes, we chose to apply 0.02 ml of Glucantime® per mouse, without previous dilution, reaching a dose of 64 mg of Sb5+/dose, which was able to reduce almost completely the parasitic burden in the footpads after five subcutaneous injections. Our results, combined with data in the literature, including studies with humans, lead us to reinforce the effectiveness of the intralesional use of Glucantime in the treatment of localized skin lesions. The intralesional use of amentoflavone has shown to have potential in the treatment of the cutaneous lesion caused by L. amazonensis in BALB/c mice. However, the use of a higher dosage may result in an increased effect in vivo. The difficulty of solubilizing higher concentrations of the compound in a vehicle that can be applied subcutaneously was a challenge. The difficulty in dissolving amentoflavone in water and organic solvents is known (Feng et al., 2020). In order to improve solubility in liquid solvents, there are several pharmacotechnical possibilities. Recently, micelles and nanomicelles have been used to improve the solubility and bioavailability of amentoflavone (Zhang et al., 2019; Feng et al., 2020). Also, the use of amorphous solid dispersion contributed to the increase in solubility, dissolution and oral bioavailability of amentoflavone, promoting the antitumor effect in rats (Chen et al., 2020). Therefore, it is suggested that the pharmacotechnical improvement of amentoflavone may increase its antileishmanial activity in vivo.
It is known that the interactions that initially occur between the Leishmania parasite and the immune cells can shape the macrophages response and the type of adaptive immune response that is being induced. The first interactions between the protozoan and eosinophils and mast cells influence the response of macrophages to infection and the development of the adaptive immune response, thus determining the final result of the infection (Rodríguez and Wilson, 2014). The histopathological analysis of our experimental protocol showed the presence of these cells in all infected groups. The decrease in parasitism with the presence of mast cells in the footpad of animals treated with Glucantime may be associated with the remodeling of the tissue injured by the parasite. In dogs naturally infected with L. infantum, the low parasitism and the presence of few clinical signs were associated with a higher density of mast cells and deposition of type III collagen in tissues of oligosymptomatic animals (Cardoso et al., 2017). The findings observed in histopathology regarding the presence of parasites were compatible with the parasite load quantification, both by LDA and by qPCR. Amentoflavone treated group presented follicular hyperplasia, which is often seen during leishmaniasis and argues for the effective activation of the immune response (Corbett et al., 1992). The group treated with Glucantime® had, in fact, lower amastigotes colonization, when compared to the other groups. It is interesting to highlight the persistence of the protozoan in the lymph nodes of this group after lesion reduction, which might serve as a reservoir of the parasite. The persistence of parasites in the lymph nodes was also shown by our group after clinical cure of the lesions in C3H/He L. amazonensis-infected mice (Cardoso et al., 2010; de Souza et al., 2018). These findings reinforce the need to consider this organ as a reservoir of the protozoan even after the clinical cure of the lesion.
Several cellular processes begin after the activation of macrophages infected by the protozoan Leishmania, including liposomal degradation of enzymes, NO and reactive oxygen species production (Van Assche et al., 2011). The oxidative attack is the main weapon used by cells against invading pathogens (Paiva and Bozza, 2014).
Bearing in mind that NO is an important inflammatory mediator in infection by Leishmania sp. (Liew et al., 1990; Stenger et al., 1994; Lima-Junior et al., 2013), the analysis of its production also becomes an interesting strategy in the investigation of the immunological factors related to the treatment of the cutaneous lesion generated by L. amazonensis. Our previous work showed that amentoflavone treatment reduces NO production in macrophages infected by L. amazonensis (Rizk et al., 2014). Similarly, the results obtained in the present work showed that amentoflavone treatment reduced the NO production in infected macrophages obtained from both infected and uninfected animals. In addition, amentoflavone treatment was also able to reduce the iNOS expression in the lesions of infected mice, reinforcing the role of amentoflavone in the reduction of nitric oxide. These results suggest that this inflammatory mediator is not the mechanism of parasitic death and that the antileishmanial activity of amentoflavone would not be directly related to the reduction of this free radical, but with its antioxidant capacity (Rizk et al., 2014). In fact, parasite death is not always linked to the production of NO. Studies show that only NO production is not enough to control the infection (Mukbel et al., 2007; Santos-Pereira et al., 2019).
The production of reactive oxygen species (ROS) is another mechanism that can interfere with the elimination of microbes (Paiva and Bozza, 2014). In the present study, amentoflavone treatment was pro-oxidant in vitro, inducing an increase of ROS in peritoneal macrophages. This increase was independent of intracellular infection, although the presence of the parasite seems to anticipate this event, as seen at 24 h after treatment. In fact, it has been reported that amentoflavone induces the formation of hydroxyl radicals and thus acts synergistically with antibiotics to exert a microbicidal effect on gram-negative bacteria (Hwang et al., 2013). The generation of these hydroxyl radicals has also been associated with mitochondrial dysfunction and apoptosis in Candida albicans cells (Hwang et al., 2012) and in breast cancer cells (Pei et al., 2012). Oxidative stress generated by the release of ROS has already been reported as a leishmanicidal mechanism in other flavonoids (Fonseca-Silva et al., 2013; Cataneo et al., 2019) and, therefore, we suggest that this is one of the means by which amentoflavone exert its antileishmanial activity.
The transcription factor Nrf2 is responsible for the balance between the antioxidant and pro-inflammatory profiles and has attracted attention in studies that investigate the immune system in the modulation of infectious diseases (Vivarini and Lopes, 2020). In vitro studies have attributed the positive regulation of Nrf2 to the activity of leishmanicidal compounds (Tomiotto-Pellissier et al., 2018; Cataneo et al., 2019; Miranda-Sapla et al., 2019). In recent years, amentoflavone has been associated with the reduction of oxidative stress in vivo through the regulation of Nrf2. In a model of Alzheimer’s disease in rats, amentoflavone exerted a neuroprotective effect, attributed to the ability to decrease oxidative stress by inducing Nrf2 (Chen et al., 2018). In our model, amentoflavone did not alter Nrf2 expression. Nonetheless, HO-1 was downregulated. The level of expression of this gene can be related to the reduction of parasite load in the lesion, since it’s known that Leishmania protozoan induces the expression of the human heme oxygenase gene (HMOX1) due to its lipophosphoglycans (LPG) (Luz et al., 2012; De Menezes et al., 2019; Saha et al., 2019). L. amazonensis induces higher amounts of this antioxidant enzyme than L. major, which may have a role in the outcome of the different clinical forms of the disease (De Menezes et al., 2019). It was expected that the decrease in HO-1 would be accompanied by a decrease in the iron-sequestering protein, given the metabolic relationship between the two. However, we found an increase in the expression of ferritin in both amentoflavone and Glucantime® treated mice. It is possible that parasite death led to an increase of free iron inside the cell, which is known to regulate ferritin synthesis without the participation of heme (Eisenstein et al., 1991).
The increase in ROS associated with high concentrations of amentoflavone in vitro together with the reduction of NO in vivo, led us to investigate the role of this biflavonoid in the balance between inflammatory and anti-inflammatory patterns at the infection site, known to be determinant in the development of cutaneous leishmaniasis. In addition, the alteration of the expression of antioxidant genes, associated with the reduction of parasites in the lesion reinforces the need for studies that deepen the role of these mechanisms in the pathogenesis of leishmaniasis. Therefore, we demonstrate that amentoflavone has an antileishmanial effect both in vitro and in vivo, acting on the balance of the inflammatory response, and may represent an ally in the control of the leishmaniotic lesion.
Data Availability Statement
The original contributions presented in the study are included in the article/Supplementary Material. Further inquiries can be directed to the corresponding author.
Ethics Statement
The animal study was reviewed and approved by Comissão de Ética no Uso de Animais do Instituto Oswaldo Cruz (CEUA-IOC).
Author Contributions
Study design: YR, CA, CC, and KC. Data collection and analysis: YR, FC, CS, LG, TZV, and MP-M. Funding acquisition: KC and EA. Investigation: YR. Methodology: FC, CS, LG, SS-P, DH, and MP-M. Project administration and supervision: KC. Writing—original draft: YR, TZV, and KC. Writing—review and editing: FC, TZV, CA, EA, and KC. All authors contributed to the article and approved the submitted version.
Funding
This work was supported by a grant from Instituto Oswaldo Cruz/Fundação Oswaldo Cruz, Fundação Carlos Chagas Filho de Amparo à Pesquisa do Estado do Rio de Janeiro (FAPERJ) [E-26/203.347/2017, E-26/010.101082/2018 and E-26/010.001759/2019]. EA and MP-M are recipients of research scholarships from Conselho Nacional de Desenvolvimento Científico e Tecnológico (CNPq) (CNPq processes 304904/2016-3 and 313520-2018-6, respectively). YR is the recipient of a research scholarship from Coordenação de Aperfeiçoamento de Pessoal de Nível Superior, Brasil (CAPES) [Finance Code 001]. The funders had no role in study design, data collection and analysis, decision to publish, or preparation of the manuscript.
Conflict of Interest
The authors declare that the research was conducted in the absence of any commercial or financial relationships that could be construed as a potential conflict of interest.
Acknowledgments
The authors wish to thank the Animal Clinical Analysis Core Facilities of the Institute of Science and Technology in Biomodels (ICTB, Fiocruz RJ) and a special thanks to João Paulo Rodrigues dos Santos for assistance in histopathology processing.
Supplementary Material
The Supplementary Material for this article can be found online at: https://www.frontiersin.org/articles/10.3389/fcimb.2021.615814/full#supplementary-material
References
Añez N., Rojas A., Scorza-Dagert J. V., Morales C. (2018). Successful treatment against American cutaneous leishmaniasis by intralesional infiltration of a generic antimonial compound-lidocaine combination. A follow up study. Acta Trop. 185, 261–266. doi: 10.1016/j.actatropica.2018.06.001
Arboleda M., Barrantes S., Úsuga L. Y., Robledo S. M. (2019). Successful treatment of cutaneous leishmaniasis with intralesional meglumine antimoniate: A case series. Rev. Soc Bras. Med. Trop. 52, e20180211. doi: 10.1590/0037-8682-0211-2018
Brasil (2017). “Ministério da Saúde. Secretaria de Vigilância em Saúde. Departamento de Vigilância das Doenças Transmissíveis,” in Manual de vigilância da leishmaniose tegumentar (Brasília: Ministério da Saúde).
Cao Q., Qin L., Huang F., Wang X., Yang L., Shi H., et al. (2017). Amentoflavone protects dopaminergic neurons in MPTP-induced Parkinson’s disease model mice through PI3K/Akt and ERK signaling pathways. Toxicol. Appl. Pharmacol. 319, 80–90. doi: 10.1016/j.taap.2017.01.019
Cardoso D. O., Freitas S., Gonc S. C., Calabrese S. (2010). Immunopathological studies of Leishmania amazonensis infection in resistant and in susceptible mice. J. Infect. Dis. 201, 1933–1940. doi: 10.1086/652870
Cardoso J. M. O., Ker H. G., Aguiar-Soares R. D. O., Moreira N. D. D., Mathias F. A. S., Reis L. E. S., et al. (2017). Association between mast cells, tissue remodelation and parasite burden in the skin of dogs with visceral leishmaniasis. Vet. Parasitol. 243, 260–266. doi: 10.1016/j.vetpar.2017.05.028
Cardoso F. O., Zaverucha-do-Valle T., Almeida-Souza F., Abreu-Silva A. L., Calabrese K. S.. (2020). Modulation of cytokines and extracellular matrix proteins expression by Leishmania amazonensis in susceptible and resistant mice. Front. Microbiol. 2020 (11), 2986. doi: 10.3389/fmicb.2020.01986
Cataneo A. H. D., Tomiotto-Pellissier F., Miranda-Sapla M. M., Assolini J. P., Panis C., Kian D., et al. (2019). Quercetin promotes antipromastigote effect by increasing the ROS production and anti-amastigote by upregulating Nrf2/HO-1 expression, affecting iron availability. Biomed. Pharmacother. 113, 108745. doi: 10.1016/j.biopha.2019.108745
Chen C., Li B., Cheng G., Yang X., Zhao N., Shi R. (2018). Amentoflavone ameliorates Aβ1–42-induced memory deficits and oxidative stress in cellular and rat model. Neurochem. Res. 43, 857–868. doi: 10.1007/s11064-018-2489-8
Chen B., Wang X., Zhang Y., Huang K., Liu H., Xu D., et al. (2020). Improved solubility, dissolution rate, and oral bioavailability of main biflavonoids from Selaginella doederleinii extract by amorphous solid dispersion. Drug Deliv. 27, 309–322. doi: 10.1080/10717544.2020.1716876
Colmenares M., Kar S., Goldsmith-Pestana K., McMahon-Pratt D. (2002). Mechanisms of pathogenesis: differences amongst Leishmania species. Trans. R. Soc Trop. Med. Hyg. 96, S3–S7. doi: 10.1016/s0035-9203(02)90044-1
Corbett C. E., Paes R. A., Laurenti M. D., Andrade Júnior H. F., Duarte M. I. (1992). Histopathology of lymphoid organs in experimental leishmaniasis. Int. J. Exp. Pathol. 73 (4), 417–433.
Costa S. C. G., Lagrande P. H. (1981). Development of cell mediated immunity to flagellar antigens and acquired resistance to infection by Trypanosoma cruzi in mice. Mem. Inst. Oswaldo Cruz 76, 367–381. doi: 10.1590/S0074-02761981000400005
Croft S. L., Coombs G. H. (2003). Leishmaniasis – current chemotherapy and recent advances in the search for novel drugs. Trends Parasitol. 19, 502–508. doi: 10.1016/j.pt.2003.09.008
De Menezes J. P. B., Khouri R., Oliveira C. V. S., Petersen A. L. O. A., De Almeida T. F., Mendes F. R. L., et al. (2019). Proteomic analysis reveals a predominant NFe2L2 (Nrf2) signature in canonical pathway and upstream regulator analysis of Leishmania-infected macrophages. Front. Immunol. 10, 1362. doi: 10.3389/fimmu.2019.01362
de Oliveira Duque M. C., Ferreira e Vasconcellos É., de C., Pimentel M. I. F., Lyra M. R., Pacheco S. J. B., et al. (2016). Standardization of intralesional meglumine antimoniate treatment for cutaneous leishmaniasis. Rev. Soc Bras. Med. Trop. 49, 774–776. doi: 10.1590/0037-8682-0213-2016
de Oliveira Duque M. C., Quintão Silva J. J., Soares P. A. O., Magalhães R. S., Horta A. P. A., Paes L. R. B., et al. (2019). Comparison between systemic and intralesional meglumine antimoniate therapy in a primary health care unit. Acta Trop. 193, 176–182. doi: 10.1016/j.actatropica.2019.03.007
de Paladi C. S., Pimentel I. A. S., Katz S., Cunha R. L. O. R., de Judice W. A. S., Caires A. C. F., et al. (2012). In vitro and in vivo activity of a palladacycle complex on Leishmania (Leishmania) amazonensis. PloS Negl. Trop. Dis. 6, 1–7. doi: 10.1371/journal.pntd.0001626
de Souza C. S. F., Calabrese K. S., Abreu-Silva A. L., Carvalho L. O. P., Cardoso F., de O., et al. (2018). Leishmania amazonensis isolated from human visceral leishmaniasis: histopathological analysis and parasitological burden in different inbred mice. Histol. Histopathol. 33, 705–716. doi: 10.14670/HH-11-965
Eisenstein R. S., Garcia-Mayol D., Pettingell W., Munro H. N. (1991). Regulation of ferritin and heme oxygenase synthesis in rat fibroblasts by different forms of iron. Proc. Natl. Acad. Sci. U. S. A. 88, 688–692. doi: 10.1073/pnas.88.3.688
Feng X., Chen Y., Li L., Zhang Y., Zhang L., Zhang Z. (2020). Preparation, evaluation and metabolites study in rats of novel amentoflavone-loaded TPGS/soluplus mixed nanomicelles. Drug Deliv. 27, 137–150. doi: 10.1080/10717544.2019.1709920
Fonseca-Silva F., Inacio J. D. F., Canto-Cavalheiro M. M., Almeida-Amaral E. E. (2013). Reactive oxygen species production by quercetin causes the death of Leishmania amazonensis intracellular amastigotes. J. Nat. Prod. 76, 1505–1508. doi: 10.1021/np400193m
García M., Scull R., Satyal P., Setzer W. N., Monzote L. (2017). Chemical characterization, antileishmanial activity, and cytotoxicity effects of the essential oil from leaves of Pluchea carolinensis (Jacq.) G. Don. (Asteraceae). Phyther. Res. 31, 1419–1426. doi: 10.1002/ptr.5869
Gervazoni L., Barcellos G., Ferreira-Paes T., Amaral E. E. A. (2020). Use of natural products in leishmaniasis chemotherapy: an overview. Front. Chem. 23 November:3389/fchem.2020.579891. doi: 10.3389/fchem.2020.579891
Giulietti A., Overbergh L., Valckx D., Decallonne B., Bouillon R., Mathieu C. (2001). An overview of real-time quantitative PCR: applications to quantify cytokine gene expression. Methods 25, 386–401. doi: 10.1006/meth.2001.1261
Hsu F. T., Chiang I. T., Kuo Y. C., Hsia T. C., Lin C. C., Liu Y. C., et al. (2019). Amentoflavone effectively blocked the tumor progression of glioblastoma via suppression of ERK/NF- κB signaling pathway. Am. J. Chin. Med. 47, 913–931. doi: 10.1142/S0192415X19500484
Hwang I., Lee J., Jin H. G., Woo E. R., Lee D. G. (2012). Amentoflavone stimulates mitochondrial dysfunction and induces apoptotic cell death in Candida albicans. Mycopathologia 173, 207–218. doi: 10.1007/s11046-011-9503-x
Hwang J. H., Choi H., Woo E. R., Lee D. G. (2013). Antibacterial effect of amentoflavone and its synergistic effect with antibiotics. J. Microbiol. Biotechnol. 23, 953–958. doi: 10.4014/jmb.1302.02045
Lee K. C., Chen W. T., Liu Y. C., Lin S. S., Hsu F. T. (2018). Amentoflavone inhibits hepatocellular carcinoma progression through blockage of ERK/NF-κB activation. In Vivo (Brooklyn) 32, 1097–1103. doi: 10.21873/invivo.11351
Liao S., Ren Q., Yang C., Zhang T., Li J., Wang X., et al. (2015). Liquid chromatography-tandem mass spectrometry determination and pharmacokinetic analysis of amentoflavone and its conjugated metabolites in rats. J. Agric. Food Chem. 63, 1957–1966. doi: 10.1021/jf5019615
Liew F. Y., Millott S., Parkinson C., Palmer R. M., Moncada S. (1990). Macrophage killing of Leishmania parasite in vivo is mediated by nitric oxide from L-arginine. J. Immunol. 144, 4794 LP–4797.
Lima-Junior D. S., Costa D. L., Carregaro V., Cunha L. D., Silva A. L. N., Mineo T. W. P., et al. (2013). Inflammasome-derived IL-1β production induces nitric oxide–mediated resistance to Leishmania. Nat. Med. 19, 909–915. doi: 10.1038/nm.3221
Liu B., Yu S. (2018). Amentoflavone suppresses hepatocellular carcinoma by repressing hexokinase 2 expression through inhibiting JAK2/STAT3 signaling. Biomed. Pharmacother. 107, 243–253. doi: 10.1016/j.biopha.2018.07.177
Luz N. F., Andrade B. B., Feijó D. F., Araújo-santos T., Graziele C., Brodyskn C. I., et al. (2012). Heme oxygenase-1 promotes the persistence of Leishmania chagasi infection. J. Immunol. 188, 55–71. doi: 10.4049/jimmunol.1103072
Miranda-Sapla M. M., Tomiotto-Pellissier F., Assolini J. P., Carloto A. C. M., Bortoleti B. T., da S., et al. (2019). trans-Chalcone modulates Leishmania amazonensis infection in vitro by Nrf2 overexpression affecting iron availability. Eur. J. Pharmacol. 853, 275–288. doi: 10.1016/j.ejphar.2019.03.049
Mishra A., Pathak Y., Choudhir G., Kumar A., Mishra S. K., Tripathi V. (2020). Natural compounds as potential inhibitors of novel coronavirus (COVID-19) main protease: An in silico study. Preprint (Version 2) available at Research Square. doi: 10.21203/rs.3.rs-22839/v2
Mukbel R. M., Patten C., Gibson K., Ghosh M., Petersen C., Jones D. E. (2007). Macrophage killing of Leishmania amazonensis amastigotes requires both nitric oxide and superoxide. Am. J. Trop. Med. Hyg. 76, 669–675. doi: 10.4269/ajtmh.2007.76.669
Njock G. B. B., Grougnet R., Efstathiou A., Smirlis D., Genta-Jouve G., Michel S., et al. (2017). A nitrile glucoside and biflavones from the leaves of Campylospermum excavatum (Ochnaceae). Chem. Biodivers. 14, e1700241. doi: 10.1002/cbdv.201700241
Oliveira-Neto M. P., Schubach A., Mattos M., Gonçalves Da Costa S. C., Pirmez C. (1997). Intralesional therapy of American cutaneous leishmaniasis with pentavalent antimony in Rio de Janeiro, Brazil - An area of Leishmania (V.) braziliensis transmission. Int. J. Dermatol. 36, 463–468. doi: 10.1046/j.1365-4362.1997.00188.x
Oubada A., García M., Bello-Alarcón A., Cuesta-Rubio O., Monzote L. (2014). Antileishmanial activity of leaf extract from Calophyllum rivulare against Leishmania amazonensis. Emirates J. Food Agric. 26, 807–812. doi: 10.9755/ejfa.v26i9.18447
Paiva C. N., Bozza M. T. (2014). Are reactive oxygen species always detrimental to pathogens? Antioxid. Redox Signal. 20 (6), 1000–1037. doi: 10.1089/ars.2013.5447
Pei J. S., Liu C. C., Hsu Y. N., Lin L. L., Wang S. C., Chung J. G., et al. (2012). Amentoflavone induces cell-cycle arrest and apoptosis in MCF-7 human breast cancer cells via mitochondria-dependent pathway. In Vivo (Brooklyn) 26, 963–970.
Pires D. E. V., Blundell T. L., Ascher D. B. (2015). pkCSM: Predicting small-molecule pharmacokinetic and toxicity properties using graph-based signatures. J. Med. Chem. 58, 4066–4072. doi: 10.1021/acs.jmedchem.5b00104
Rizk Y. S., Fischer A., Cunha M., de C., Rodrigues P. O., Marques M. C. S., et al. (2014). In vitro activity of the hydroethanolic extract and biflavonoids isolated from Selaginella sellowii on Leishmania (Leishmania) amazonensis. Mem. Inst. Oswaldo Cruz 109, 1050–1056. doi: 10.1590/0074-0276140312
Rodríguez N. E., Wilson M. E. (2014). Eosinophils and mast cells in leishmaniasis. Immunol. Res. 59, 129–141. doi: 10.1007/s12026-014-8536-x
Saha S., Basu M., Guin S., Gupta P., Mitterstiller A., Weiss G., et al. (2019). Leishmania donovani exploits macrophage Heme Oxygenase-1 to neutralize oxidative burst and TLR signaling – dependent host defense. J. Immunol. 202, 827–840. doi: 10.4049/jimmunol.1800958
Sambrook J., Russell D. W. (2006). Purification of nucleic acids by extraction with phenol:chloroform. CSH Protoc. 2006, pdb.prot4455. doi: 10.1101/pdb.prot4455
Santos-Pereira S., Cardoso F. O., Calabrese K. S., Do Valle T. Z. (2019). Leishmania amazonensis resistance in murine macrophages: Analysis of possible mechanisms. PloS One 14, 1–16. doi: 10.1371/journal.pone.0226837
Saroni Arwa P., Zeraik M. L., Farias Ximenes V., Da Fonseca L. M., Da Silva Bolzani V., Siqueira Silva D. H. (2015). Redox-active biflavonoids from Garcinia brasiliensis as inhibitors of neutrophil oxidative burst and human erythrocyte membrane damage. J. Ethnopharmacol. 174, 410–418. doi: 10.1016/j.jep.2015.08.041
Shen X., Niu X., Li G., Deng X., Wang J. (2018). Amentoflavone ameliorates Streptococcus suis-induced infection in vitro and in vivo. Appl. Environ. Microbiol. 84, e01804-18. doi: 10.1128/AEM.01804-18
Stenger S., Thüring H., Röllinghoff M., Bogdan C. (1994). Tissue expression of inducible nitric oxide synthase is closely associated with resistance to Leishmania major. J. Exp. Med. 180, 783–793. doi: 10.1084/jem.180.3.783
Su C., Yang C., Gong M., Ke Y., Yuan P., Wang X., et al. (2019). Antidiabetic activity and potential mechanism of amentoflavone in diabetic mice. Molecules 24, 1–14. doi: 10.3390/molecules24112184
Tamargo B., Monzote L., Piñón A., Machín L., García M., Scull R., et al. (2017). In vitro and in vivo evaluation of essential oil from Artemisia absinthium L. formulated in nanocochleates against Cutaneous Leishmaniasis. Medicines 4, 38. doi: 10.3390/medicines4020038
Taswell C. (1984). Limiting dilution assays for the determination of immunocompetent cell frequencies. III. Validity tests for the single-hit Poisson model. J. Immunol. Methods 72, 29–40. doi: 10.1016/0022-1759(84)90430-7
Tomiotto-Pellissier F., Alves D. R., Miranda-Sapla M. M., de Morais S. M., Assolini J. P., da Silva Bortoleti B. T., et al. (2018). Caryocar coriaceum extracts exert leishmanicidal effect acting in promastigote forms by apoptosis-like mechanism and intracellular amastigotes by Nrf2/HO-1/ferritin dependent response and iron depletion: Leishmanicidal effect of Caryocar coriaceum leaf extracts. Biomed. Pharmacother. 98, 662–672. doi: 10.1016/j.biopha.2017.12.083
Tsai J., Hsu F., Pan P., Chen C., Kuo Y. (2018). Amentoflavone enhances the therapeutic efficacy of Sorafenib by inhibiting anti-apoptotic potential and potentiating apoptosis in hepatocellular carcinoma in vivo. Anticancer Res. 2125, 2119–2125. doi: 10.21873/anticanres.12452
Van Assche T., Deschacht M., Da Luz R. A. I., Maes L., Cos P. (2011). Leishmania-macrophage interactions: Insights into the redox biology. Free Radic. Biol. Med. 51, 337–351. doi: 10.1016/j.freeradbiomed.2011.05.011
Vivarini A. D. C., Lopes U. G. (2020). The potential role of Nrf2 signaling in Leishmania infection outcomes. Front. Cell. Infect. Microbiol. 9, 453. doi: 10.3389/fcimb.2019.00453
Wahyudi L. D., Jeong J., Yang H., Kim J. H. (2018). Amentoflavone-induced oxidative stress activates NF-E2-related factor 2 via the p38 MAP kinase-AKT pathway in human keratinocytes. Int. J. Biochem. Cell Biol. 99, 100–108. doi: 10.1016/j.biocel.2018.04.006
Weirather J. L., Jeronimo S. M. B., Gautam S., Sundar S., Kang M., Kurtz M. A., et al. (2011). Serial quantitative PCR assay for detection, species discrimination, and quantification of Leishmania spp. in human samples. J. Clin. Microbiol. 49, 3892–3904. doi: 10.1128/JCM.r00764-11
Weniger B., Vonthron-Sénécheau C., Kaiser M., Brun R., Anton R. (2006). Comparative antiplasmodial, leishmanicidal and antitrypanosomal activities of several biflavonoids. Phytomedicine 13, 176–180. doi: 10.1016/j.phymed.2004.10.008
World Health Organization (2010). The control of leishmaniasis. Bull. World Health Organ 58, 807–818.
World Health Organization (2020).Leishmaniasis. Available at: https://www.who.int/health-topics/leishmaniasis#tab=tab_1 (Accessed August 17, 2020).
Yen T. H., Hsieh C. L., Liu T., Huang C. S., Chen Y. C., Chuang Y. C., et al. (2018). Amentoflavone induces apoptosis and inhibits NF-ĸB-modulated anti-apoptotic signaling in glioblastoma cells. In Vivo (Brooklyn) 32, 279–285. doi: 10.21873/invivo.11235
Keywords: amentoflavone, biflavonoid, prooxidant, antileishmanial activity, intralesional treatment, cutaneous leishmaniasis, Glucantime
Citation: Rizk YS, Santos-Pereira S, Gervazoni L, Hardoim DJ, Cardoso FO, de Souza CSF, Pelajo-Machado M, Carollo CA, de Arruda CCP, Almeida-Amaral EE, Zaverucha-do-Valle T and Calabrese KS (2021) Amentoflavone as an Ally in the Treatment of Cutaneous Leishmaniasis: Analysis of Its Antioxidant/Prooxidant Mechanisms. Front. Cell. Infect. Microbiol. 11:615814. doi: 10.3389/fcimb.2021.615814
Received: 10 October 2020; Accepted: 15 January 2021;
Published: 25 February 2021.
Edited by:
Loïc Riviere, Université de Bordeaux, FranceReviewed by:
Chiranjib Pal, West Bengal State University, IndiaCelio Geraldo Freire-de-Lima, Federal University of Rio de Janeiro, Brazil
Copyright © 2021 Rizk, Santos-Pereira, Gervazoni, Hardoim, Cardoso, de Souza, Pelajo-Machado, Carollo, de Arruda, Almeida-Amaral, Zaverucha-do-Valle and Calabrese. This is an open-access article distributed under the terms of the Creative Commons Attribution License (CC BY). The use, distribution or reproduction in other forums is permitted, provided the original author(s) and the copyright owner(s) are credited and that the original publication in this journal is cited, in accordance with accepted academic practice. No use, distribution or reproduction is permitted which does not comply with these terms.
*Correspondence: Tânia Zaverucha-do-Valle, taniazv@ioc.fiocruz.br
†These authors have contributed equally to this work