- 1Department of Parasitology, Harbin Medical University, Harbin, China
- 2National Institute of Parasitic Diseases, Chinese Center for Disease Control and Prevention, Chinese Center for Tropical Diseases Research, WHO Collaborating Centre for Tropical Diseases, National Center for International Research on Tropical Diseases, Ministry of Science and Technology, Key Laboratory of Parasite and Vector Biology, MOH, Shanghai, China
Cryptosporidium and Giardia are two important zoonotic intestinal protozoa responsible for diarrheal diseases in humans and animals worldwide. Feces from infected hosts, water and food contaminated by Cryptosporidium oocysts and Giardia cysts as well as predictors such as poverty have been involved in their transmission. Myanmar is one of the world’s most impoverished countries. To date, there are few epidemiological studies of Cryptosporidium and Giardia in humans. To understand the prevalence and genetic characterization of Cryptosporidium spp. and Giardia duodenalis in humans in Myanmar, a molecular epidemiological investigation of the two protozoa was conducted in four villages of Shan State. 172 fecal specimens were collected from Wa people (one each) and identified for the presence of Cryptosporidium spp. and G. duodenalis by sequence analysis of their respective small subunit ribosomal RNA genes. 1.74% of investigated people were infected with Cryptosporidium spp.—C. andersoni (n = 2) and C. viatorum (n = 1) while 11.05% infected with G. duodenalis—assemblages A (n = 6) and B (n = 13). By sequence analysis of 60-kDa glycoprotein gene, the C. viatorum isolate belonged to a novel subtype XVcA2G1c. DNA preparations positive for G. duodenalis were further subtyped. Five of them were amplified and sequenced successfully: different assemblage B sequences (n = 2) at the triosephosphate isomerase (tpi) locus; sub-assemblage AII sequence (n = 1) and identical assemblage B sequences (n = 2) at the β-giardin (bg) locus. This is the first molecular epidemiological study of Cryptosporidium spp. and G. duodenalis in humans in Myanmar at both genotype and subtype levels. Due to unclear transmission patterns and dynamics of Cryptosporidium spp. and G. duodenalis, future research effort should focus on molecular epidemiological investigations of the two parasites in humans and animals living in close contact in the investigated areas, even in whole Myanmar. These data will aid in making efficient control strategies to intervene with and prevent occurrence of both diseases.
Introduction
Cryptosporidium and Giardia are two ubiquitous intestinal protozoan parasites in humans and numerous animals. Both cryptosporidiosis and giardiasis are clinically characterized by diarrhea, and the severity of diarrhea is closely related to the age and health status of the infected hosts as well as the genetic background and infective dose of the parasites (Xiao and Fayer, 2008). Immunocompetent individuals typically experience self-limiting diarrhea and are often asymptomatic while chronic diarrhea in immunocompromised individuals (Plutzer et al., 2018). Severe life-threatening diarrhea has been reported in cryptosporidiosis patients infected with human immunodeficiency virus (HIV) (Ryan et al., 2016). The infective dose of the two parasites are low: <10 Cryptosporidium (Cryptosporidium hominis or Cryptosporidium parvum) oocysts and 10–100 Giardia duodenalis (syn. Giardia intestinalis, Giardia lamblia) cysts can cause infection in immunocompetent persons (Rendtorff, 1954; Okhuysen et al., 1999; Chappell et al., 2006). More seriously, a single C. parvum oocyst has been reported to initiate infection in immunosuppressed persons (Zhao et al., 2014). In general, the pathogenicity of Cryptosporidium is considered to be more severe in humans than that of Giardia (Prado et al., 2005). Humans can acquire Cryptosporidium and Giardia infections through the fecal-oral route, either directly (via human-to-human/animal contact) or indirectly (via ingestion of contaminated water or food) (Osman et al., 2016). The role of water and food in the epidemiology of the two parasitic diseases is now well recognized. To date, waterborne and foodborne outbreaks of cryptosporidiosis (>524 and >26) (Liu et al., 2020) and giardiasis (>344 and >38) had been reported worldwide (Karanis et al., 2007; Baldursson and Karanis, 2011; Efstratiou et al., 2017; Ryan et al., 2019). Based on clinical and public health importance, Cryptosporidium and Giardia are listed on the Environmental Protection Agency (EPA) microbial contaminant candidate list of concern for waterborne transmission (https://www.epa.gov/ground-water-and-drinking-water/national-primary-drinking-water-regulations). The two pathogens have also been ranked as the 5th and 11th most important foodborne parasites worldwide by a joint Food and Agriculture Organization (FAO)/World Health Organization (WHO) in 2014, respectively (Plutzer et al., 2018).
Both Cryptosporidium and Giardia are complicated genera. To date, 41 Cryptosporidium species and over 40 genotypes have been recognized (Feng et al., 2018; Holubová et al., 2019; Bolland et al., 2020; Holubová et al., 2020). Among them, 22 Cryptosporidium species/genotypes have been identified in humans (Xiao and Feng, 2017; Kváč et al., 2018), and C. hominis and C. parvum are the two most common species, reported in > 90% of human cryptosporidiosis cases (Squire and Ryan, 2017). However, in some countries, especially in developing countries, some unusual species have a high occurrence in human cryptosporididosis cases, such as Cryptosporidium meleagridis (10–20%) in Thailand and in Peru (Gatei et al., 2002; Cama et al., 2007; Cama et al., 2008); Cryptosporidium andersoni (79.59%) in India (Hussain et al., 2017); Cryptosporidium viatorum (7.14–11.11%) in Ethiopia (Adamu et al., 2014; de Lucio et al., 2016). Among the eight recognized species, and only G. duodenalis has been found to infect humans with eight assemblages (A to H) being identified (Ryan et al., 2019). Assemblages A and B are responsible for the vast majority (99%) of human giardiasis cases, and both of them have also been found in a variety of mammal species (Sprong et al., 2009). Assemblages C to H are specific to some animal species, but assemblages C to F are occasionally found in humans (Cacciò et al., 2018).
Cryptosporidiosis and giardiasis cause considerable human disease burdens worldwide. Like other infectious diseases, these two parasitic diseases also usually affect people living in poverty, and may further promote poverty. Developing countries usually have higher prevalence than developed countries: 5–10% versus 1% for cryptosporidiosis (Checkley et al., 2015); 0.9–40.7% versus 0.4–7.0% for giardiasis (Feng and Xiao, 2011; Ryan and Cacciò, 2013). In fact, early in 2004, Cryptosporidium spp. and G. duodenalis were included in the WHO’s “Neglected Disease Initiative” due to their link with poverty (Savioli et al., 2006). Myanmar is one of the world’s most impoverished countries. As of 2019, Myanmar ranks 145 out of 189 countries according to the Human Development Index (http://hdr.undp.org/en/content/2019-human-development-index-ranking). However, it is unclear on epidemiological status of human cryptosporidiosis and giardiasis and genetic characterization of Cryptosporidium spp. and G. duodenalis. To date, only two studies reported the prevalence by microscopy in Myanmar: Cryptosporidium spp. (3.4%, 7/203) in infants with diarrhea (Aye et al., 1994) and G. duodenalis (3.4%, 28/821) in schoolchildren and guardians (Kim et al., 2016). The present study was conducted to understand the prevalence and genetic characterization of Cryptosporidium spp. and G. duodenalis in humans in Myanmar at the genotype and subtype levels.
Materials and Methods
Ethics Statement
Scientific approval and ethical clearance for this study was given by the Ethics Committee of the National Institute of Parasitic Diseases, Chinese Center for Disease Control and Prevention, China, and the Myanmar Eastern Shan State Special Region 2 Ethic Health Organization. All study participants were informed of the aims and the procedures of this study at enrollment. Before collection of fecal specimens, written informed consents for all study participants were obtained from each adult individual or legal guardian of enrolled children.
Study Site and Collection of Fecal Specimens
In October, 2018, a total of 172 fecal specimens (approximately 5–10 g each) were collected from Wa people (one specimen each) in four villages of Pangsang Township (22˚10′N, 99˚11′E) of Matman District of Shan State, which is located in the east of Myanmar, bordering with China’s Yunnan Province in about 133-kilometer-long border line (Figure 1). The villages investigated have poor sanitation and garbage collection. Domestic pigs and chickens are the most common animals, which are kept by almost every household. The villagers are poorly educated and have weak hygiene awareness. The participants were composed of children (n = 97), teenagers (n = 41) and adults (n = 34), with their ages ranging from seven to 53 years. At the time of sampling, we only recorded the presence or absence of diarrhea. All fecal specimens were delivered to the laboratory in a cooler with ice packs within 24 h after collection and stored in a refrigerator at −20°C for future analysis.
DNA Extraction
Genomic DNA was extracted directly from approximately 180 to 200 mg of each fecal specimen using a QIAamp DNA Mini Stool Kit (Qiagen, Hilden, Germany) according to the manufacturer-recommended procedures. To obtain a high yield of DNA, the lysis temperature was increased to 95°C. DNA was eluted in 200 μl of AE elution buffer and stored at −20°C. Extracted DNA preparations were analyzed by nested polymerase chain reaction (PCR) amplification.
Genotyping and Subtyping of Cryptosporidium spp. and G. duodenalis
Cryptosporidium species were identified by nested PCR amplification of the partial small subunit ribosomal RNA (SSU rRNA) gene (approximately 830 bp) (Huang et al., 2016). Subtyping of DNA preparations positive for Cryptosporidium at the SSU rRNA locus was performed by nested PCR amplification of the partial 60 kDa glycoprotein (gp60) gene (approximately 950 bp) (Stensvold et al., 2015). Meanwhile, all DNA preparations were screened for the presence of G. duodenalis by nested PCR amplification of the partial SSU rRNA gene (approximately 290 bp) and were identified to the assemblage level as previously described by Appelbee et al. (2003). DNA preparations positive for G. duodenalis at the SSU rRNA locus were further analyzed to determine sub-assemblages by nested PCR amplification of the triose phosphate isomerase (tpi) and β-giardin (bg) genes. Assemblage A/B-specific nested PCRs were performed to amplify approximately 330 and 460 bp nucleotide fragments of the tpi gene, respectively (Geurden et al., 2008; Levecke et al., 2009). Approximately 510 bp fragment of bg gene was amplified (Lalle et al., 2005).
Each DNA preparation was performed two times and TaKaRa Taq DNA polymerase (TaKaRa Bio Inc., Tokyo, Japan) was used for all PCR reactions. A negative control (DNase-free water) and a positive control (C. baileyi or G. duodenalis assemblage E) were included in all PCR tests. All secondary PCR products were subjected to electrophoresis in a 1.5% agarose gel and visualized by staining the gel with GelStrain (TransGen Biotech., Beijing, China) before sequencing.
Sequence Analysis
Positive secondary PCR products of expected size were sent to Comate Bioscience Company Limited (Jilin, China) for sequencing using their respective secondary PCR primers on an ABI PRISM 3730 XL DNA Analyzer using the BigDye Terminator v3.1Cycle Sequencing Kit (Applied Biosystems, Carlsbad, CA, USA). The accuracy of the sequencing data was confirmed by sequencing in both directions. Species/genotypes and subtypes of Cryptosporidium, and assemblages and sub-assemblages of G. duodenalis were identified by comparing the nucleotide sequences obtained in the present study with reference sequences downloaded from GenBank using the Basic Local Alignment Search Tool (BLAST) (http://www.ncbi.nlm.nih.gov/blast/) and Clustal X 1.83 (http://www.clustal.org/).
Phylogenetic Analysis
To assess phylogenetic relationships among C. viatorum subtypes obtained in the present study and those published in GenBank databases, all gp60 gene sequences of C. viatorum subtypes were implemented in the software Mega 5 (http://www.megasoftware.net/). A neighbor-joining tree was constructed based on the evolutionary distances calculated by the Kimura 2-parameter model. The reliability of the trees was assessed using the bootstrap analysis with 1,000 replicates.
Statistical Analysis
All statistical analyses were performed with Statistical Package for the Social Sciences (SPSS) 19.0. Pearson chi-square (χ2) and Fisher’s exact tests were used to determine statistical significance in the present study. All results were interpreted using odds ratios, 95% confidence intervals and significance level (P-values < 0.05).
Nucleotide Sequence Accession Numbers
The representative nucleotide sequences obtained in this study were deposited in the GenBank database under the following accession numbers: MW014313 to MW014315 (SSU rRNA) and MW014316 (gp60) for Cryptosporidium; MW011715 and MW011716 (tpi), MW011717 and MW011718 (bg) for G. duodenalis.
Results
Prevalence of Cryptosporidium spp. and G. duodenalis
All 172 fecal specimens were screened for the presence of Cryptosporidium spp. and G. duodenalis by PCR amplification and sequence analysis of their respective partial SSU rRNA gene. G. duodenalis (11.05%, 19/172) was observed to be more prevalent than Cryptosporidium spp. (1.74%, 3/172) in the investigated people. Cryptosporidium spp. was found only in children (1.03%, 1/97) and adults (5.88%, 2/34) while G. duodenalis only in children (12.37%, 12/97) and teenagers (17.07%, 7/41) (Table 1).
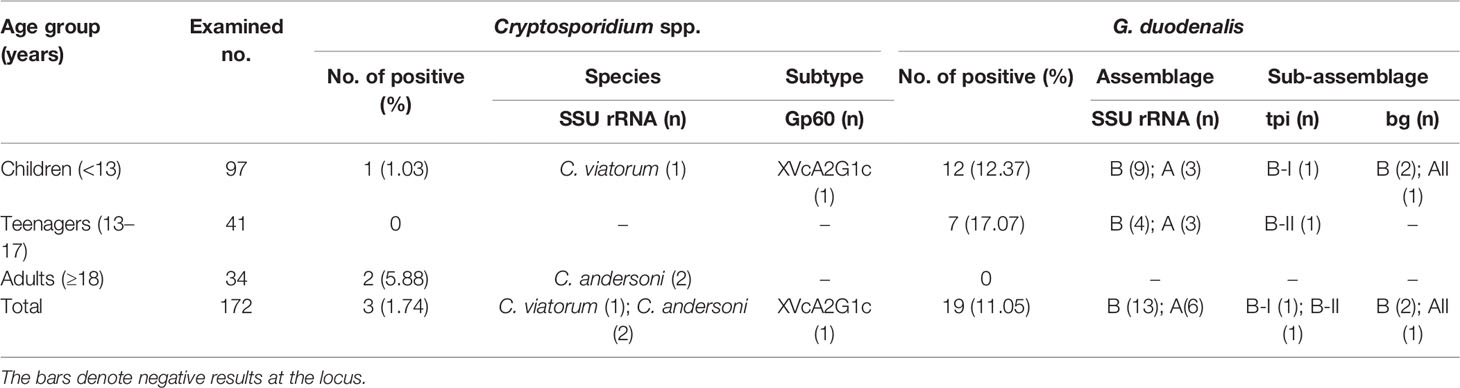
Table 1 Prevalence and genetic characterization of Cryptosporidium spp. and G. duodenalis in humans.
By χ2 tests, only a statistically higher prevalence of G. duodenalis was observed in children than in adults (P = 0.04). Both prevalences of Cryptosporidium spp. and G. duodenalis were higher in people without diarrhea than those with diarrhea (Table 2). Furthermore, there were no relationships between Cryptosporidium spp. or G. duodenalis infection and diarrhea in each age group (P > 0.05) (Table 3).
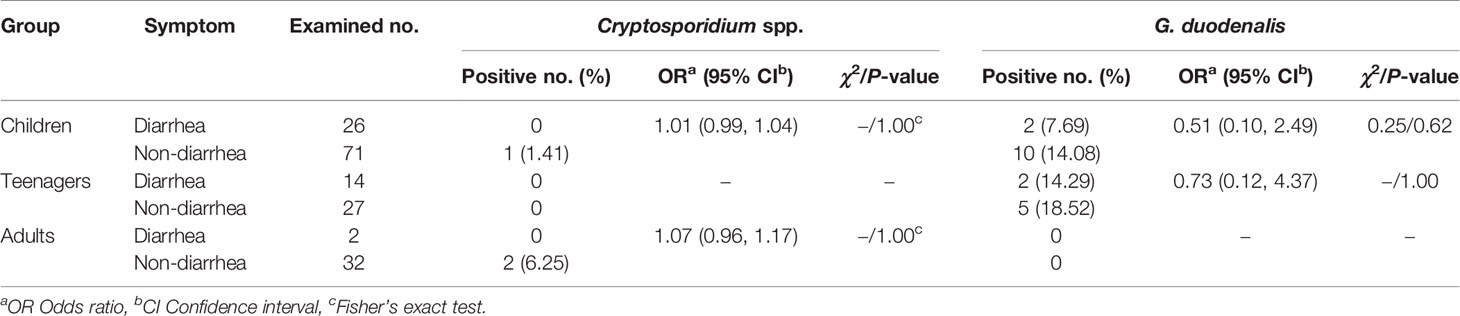
Table 3 Relationships between Cryptosporidium spp. or G. duodenalis infection and diarrhea in each age group.
Genotyping and Subtyping of Cryptosporidium
Sequence analysis of the SSU rRNA gene identified two Cryptosporidium species: C. andersoni (n = 2) and C. viatorum (n = 1). Two C. andersoni isolates (MW014313 and MW014314) had 99.73% (two-base difference) and 99.62% (three-base difference) similarity with C. andersoni isolates from a dairy calf (JX515549), respectively. C. viatorum isolate was identical to a wild rat-derived C. viatorum isolate (MK522269).
In phylogenetic analysis of the gp60 gene sequences, the C. viatorum isolate obtained in the present study was grouped with C. viatorum subtypes XVcA2G1b, XVcA2G1a, and XVcA2G1 (Figure 2). Meanwhile, this result was also supported by evolutionary analysis at the nucleotide and amino acid levels: low genetic variations (0.12–0.99% and 0.39–1.96%) compared to the other three subtypes in XVc subtype family; high genetic variations (2.13–21.47% and 3.59–35.60%) compared to all 11 subtypes in subtype families (XVa, XVb, XVd) (Table 4). According to the terminology of C. viatorum subtypes established by Stensvold et al. (2015), a novel subtype XVcA2G1c was identified, which had the largest similarity (99.88%) with subtype XVcA2G1 (MK796005) from a Bower’s white-toothed rat in China.
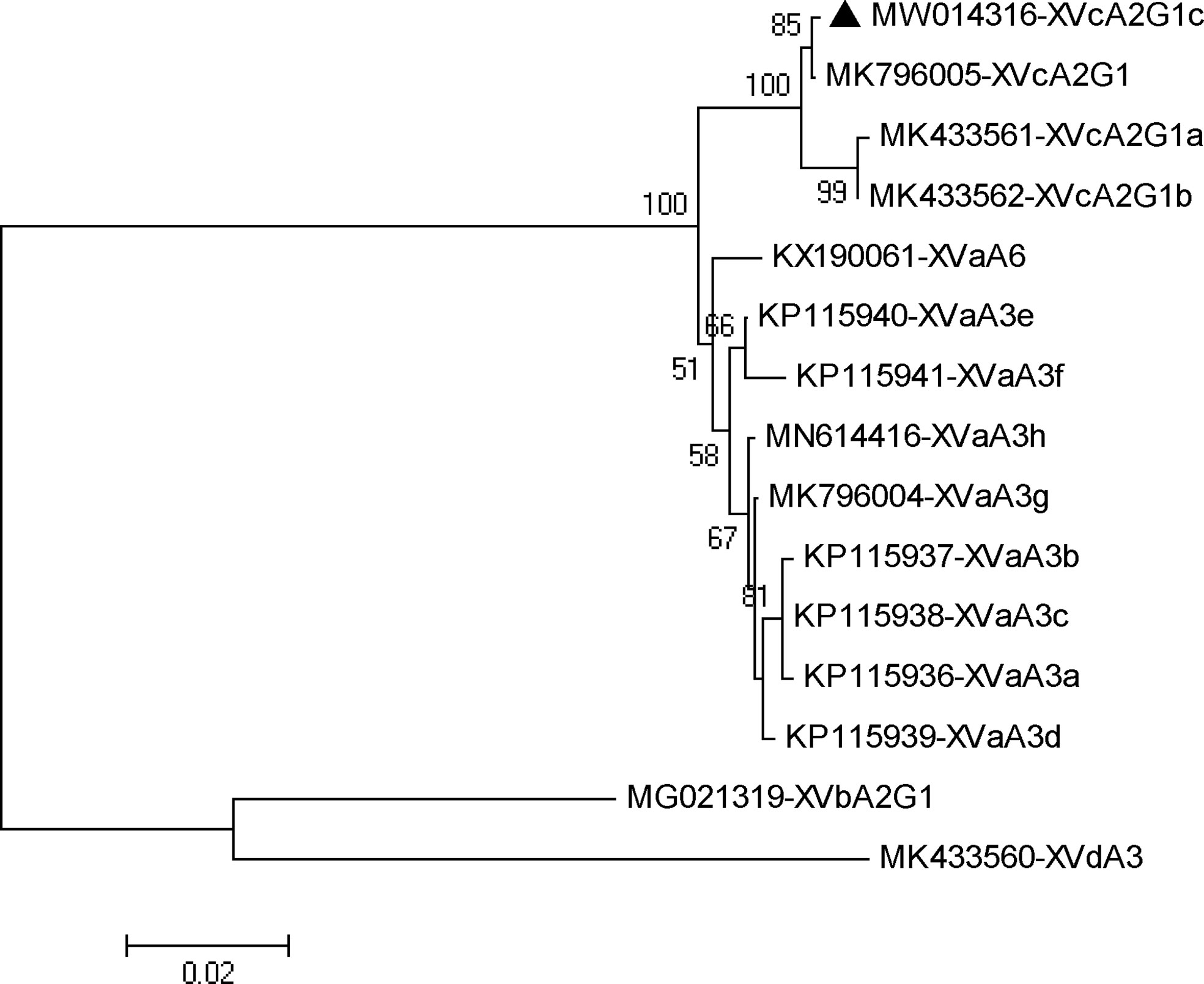
Figure 2 Phylogenetic relationship of gp60 subtypes of Cryptosporidium viatorum. The relationships among C. viatorum subtypes identified in the present study and those deposited in the GenBank were inferred by a neighbor-joining analysis of gp60 gene sequences based on genetic distance by the Kimura 2-parameter model. The numbers on the branches are percent bootstrapping values from 1000 replicates. Each sequence is identified by its accession number and subtype designation. The triangle filled in black indicates the subtype identified in this study.
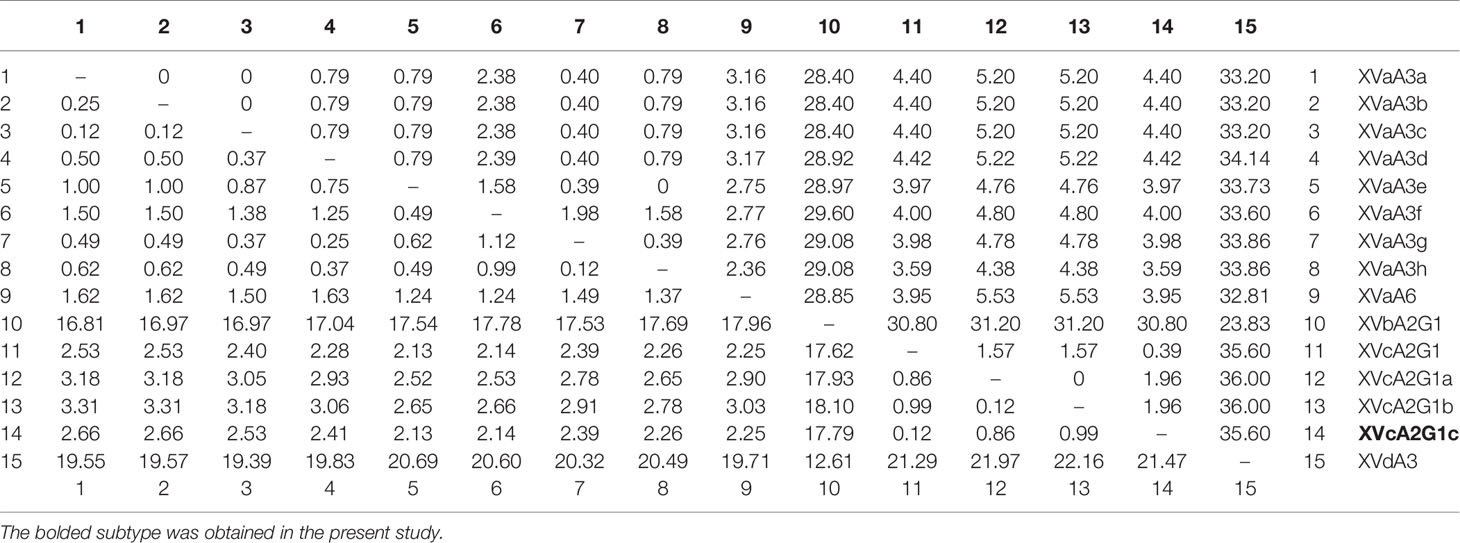
Table 4 Pairwise differences (percentage) among gp60 subtypes of C. viatorum for nucleotide (below the diagonal) and amino acid sequences (above the diagonal).
Genotyping and Subtyping of G. duodenalis
Sequence analysis of the SSU rRNA gene identified two G. duodenalis assemblages: A (n = 6) and B (n = 13). All assemblage A isolates were identical to each other, and so were assemblage B isolates, which had 100% similarity with two horse-derived isolates (MN174121 and MN174122), respectively.
At the tpi locus, only two isolates of assemblage B were successfully amplified and sequenced, with two different tpi gene sequences being obtained. Due to no clear subgrouping within assemblage B, both of them were named as sub-assemblage B-I (MW011715) and B-II (MW011716) for convenient description, respectively (Table 1). Sub-assemblage B-I had one-base difference compared to the JX994251 and KM977638 sequences from a human and a chinchilla, respectively. Sub-assemblage B-II had 100% similarity with those isolates from a human (JX994245) and animals—non-human primates (MK982533), a bos indicus (MF459680), a dog (LC437486), a cat (LC341576), a goat (MF095053), a pig (MH644772), a rabbit (MH475909), a fox (KY304077), an orangutan (KR011753), a chinchilla (KF843914), and an anteater (GU797247).
At the bg locus, only three G. duodenalis isolates were successfully amplified and sequenced. The assemblage A isolate was identified as sub-assemblage AII, which was identical to those G. duodenalis isolates from a human (MN844143), a sheep (MK452883), and a cattle (MK452836). Two assemblage B isolates were identical to each other, and shared 100% similarity with a human-derived G. duodenalis isolate (MK982542).
Discussion
To the best of our knowledge, the present study is the first report of the prevalence and genetic characterization of Cryptosporidium spp. and G. duodenalis by molecular techniques in Wa people in Myanmar. Cryptosporidium spp. was detected in children (1.03%, 1/97) and adults (5.88%, 2/34), while G. duodenalis in children (12.37%, 12/97) and teenagers (17.07%, 7/41). However, in two studies conducted in Myanmar, a prevalence of 3.4% was found in detection of either Cryptosporidium spp. in infants or G. duodenalis in schoolchildren and guardians by microscopy (Aye et al., 1994; Kim et al., 2016). The difference in prevalence may be related to detection methods employed. PCR-based molecular techniques are demonstrated to be more sensitive than conventional microscopy, such as Cryptosporidium spp. prevalence in sheep in Australia (26.25% versus 2.6%) (Ryan et al., 2005) and in the US (50.8% versus 20.6%) (Santín et al., 2007); G. duodenalis prevalence in dogs in India (20.0% versus 3.0%) (Traub et al., 2004) and in Italy (20.5% versus 11.0%) (Scaramozzino et al., 2009). Besides that, the prevalence may be related to population specimens collected and their clinical features. Children are reported to have a statistically significantly higher prevalence than adults: such as 2.56% versus 1.89% for Cryptosporidium spp. in China (Liu et al., 2020); 53.2% versus 22.2% for G. duodenalis in Uganda (Johnston et al., 2010). Meanwhile, some studies reported significant difference in prevalence of both parasites between diarrheal and non-diarrheal children, such as 16.3% versus 3.1% for Cryptosporidium spp. in Tanzania (Tellevik et al., 2015); 20.5% versus 8.0% for G. dudenalis in Ethiopia (Feleke et al., 2018). However, in the present study, G. duodenalis was more prevalent in non-diarrheal cases than in diarrheal cases (14.08% versus 7.69% for children; 18.52% versus 14.29% for teenagers), and all three Cryptosporidium-positive cases were from non-diarrheal individuals. Similar results are also reported in some previous studies, such as Cryptosporidium spp. in Peru and G. duodenalis in Ethiopia (Cama et al., 2008; Tellevik et al., 2015). Even so, the two parasites can also lead to growth and development retardation of asymptomatic children (Prado et al., 2005; Checkley et al., 2015), thus constituting a serious public health problem in this population. The prevalences are complicated and difficult to compare due to differences in the detection methods employed, the size of specimens analyzed, the populations investigated, and the health status of individuals.
C. parvum and C. hominis are recognized as the main causative agents (> 90%) in reported human cases of Cryptosporidium infection (Squire and Ryan, 2017; Liu et al., 2020). However, in the present study, two unusual Cryptosporidium species were identified: C. andersoni (n = 2) and C. viatorum (n = 1). Since the first report of C. andersoni in humans in 2001, there have been 144 human cases of cryptosporidiosis attributed to C. andersoni, composed of 141 diarrheal cases from India (n = 78) (Hussain et al., 2017), China (n = 59) (Liu et al., 2014; Jiang et al., 2014; Su et al., 2017), the UK (n = 3) (Leoni et al., 2006) and Malawi (n = 1) (Morse et al., 2007), and three cases having no information on clinical symptoms from Australia (Waldron et al., 2011), Iran (Agholi et al., 2013), and France (Guyot et al., 2001). C. viatorum was first identified in 10 travellers with gastrointestinal symptoms returning to the UK from the Indian subcontinent in 2012 (Elwin et al., 2012). The name was chosen to underscore its link to foreign travel. To date, 37 human cases of cryptosporidiosis including the present case caused by C. viatorum have been identified in nine countries: Australia (n = 1) (Braima et al., 2019), China (n = 1) (Xu et al., 2020), Colombia (n = 1) (Sánchez et al., 2017), Ethiopia (n = 12) (Adamu et al., 2014; Stensvold et al., 2015; de Lucio et al., 2016), India (n = 2) (Khalil et al., 2017; Khalil et al., 2018), Myanmar (n = 1), Nigeria (n = 2) (Ayinmode et al., 2014; Ukwah et al., 2017), Sweden (n = 3) (Insulander et al., 2013; Stensvold et al., 2015), and the UK (n = 14) (Elwin et al., 2012; Stensvold et al., 2015). 45.95% (17/37) individuals had a history of travel abroad (Kenya, Guatemala, India, Barbados, Pakistan, Nepal and Bangladesh) (Table 5). Travel abroad was considered to be significantly associated with an increased risk of Cryptosporidium infections in the US and UK studies (Hunter et al., 2004; Roy et al., 2004). Meanwhile, it was observed that 75.68% (28/37) of human cases experienced diarrhea while 13.51% (5/37) had no diarrhea (Table 5). Occurrence of diarrhea and the severity of cryptosporidiosis in humans are complicated, often involving the immune status of the infected hosts, the virulence of Cryptosporidium species/genotypes, the infective dose of oocysts and other intestinal pathogens.
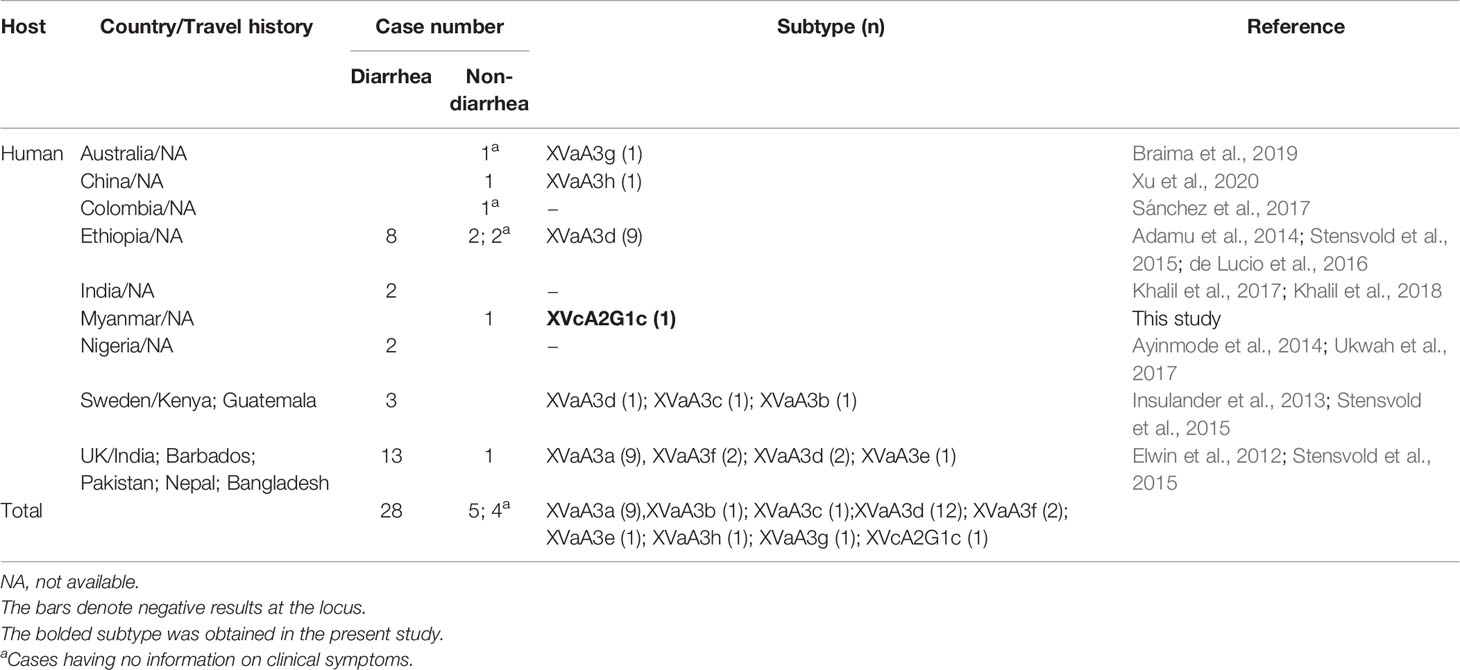
Table 5 Geographical distribution of C. viatorum subtypes identified in humans and their travel history.
Currently, it is unclear on the source of infection/contamination of C. andersoni and C. viatorum in the investigated areas. C. andersoni is actually the major species causing cattle cryptosporidiosis, especially in yearlings and adults (Wang K. et al., 2019). With the accumulation of molecular epidemiological data of Cryptosporidium, C. andersoni has also been found occasionally in other animal species, such as sheep, horses, camels, golden takins, monkeys, hamsters, and ostriches (Liu et al., 2020). In a previous molecular epidemiological study of Cryptosporidium in diarrheal outpatients conducted in China, 21 C. andersoni isolates were identical to cattle/goat-derived isolates at the SSU rRNA locus (Jiang et al., 2014). In the present study, we observed high homology (99.73% and 99.62%) of the SSU rRNA gene of two C. andersoni isolates with a cattle-derived isolate. C. viatorum was initially thought to occur exclusively in humans. However, it has also been detected in some rat species in Australia (Koehler et al., 2018) and China (Chen et al., 2019; Zhao et al., 2019). The same subtypes (XVaA3h and XVaA3g) have been identified in humans (Braima et al., 2019; Xu et al., 2020) and rats (Chen et al., 2019) (Tables 5, 6). In the present study, a novel subtype (XVcA2G1c) was identified in humans for the first time, which had the largest similarity of 99.88% (one-base difference) with that (XVcA2G1) from a Bower’s white-toothed rat in China (Chen et al., 2019). These results above indicated the large potential of zoonotic transmission of C. andersoni and C. viatorum. Therefore, the true burden of human cryptosporidiosis caused by C. andersoni and C. viatorum attributed to humans and animals as well as the transmission dynamic of this disease needs to be assessed in the investigated areas by systematic molecular epidemiological surveys of humans and animals in the future.
The genotyping results showed all G. duodenalis-positive individuals in the investigated areas were infected with assemblages A (6/19, 31.58%) and B (13/19, 68.42%). Molecular epidemiological data indicated that assemblage B commonly had higher prevalence than assemblage A worldwide (Ryan and Cacciò, 2013), such as 68.0% (66/97) versus 29.9% (29/97) in Spain (Wang Y. et al., 2019); 66.7% (8/12) versus 33.3% (4/12) in China (Yu et al., 2019). However, an oppositive pattern of distribution of assemblages A and B has also been noticed in humans. Assemblage A showed a predominance compared to assemblage B in some studies, such as 35.9% (33/92) versus 21.7% (20/92) in Ethiopia (Damitie et al., 2018); 52.5% (31/59) versus 22.0% (13/59) in Ethiopia (Gelanew et al., 2007). Although epidemiological investigations have been conducted worldwide, the number of molecular epidemiological studies of giardiasis in humans is relatively small. To date, there is a lack of geographical structuring of the G. duodenalis assemblages across the globe. Difference in geographical distribution of assemblages might be associated to the socioeco-epidemiological factors of the population investigated (Sánchez et al., 2017) and methodological aspects, such as targeted genes, number of loci, primers, downstream procedures etc (Oliveira-Arbex et al., 2016).
One of six assemblage A isolates was successfully amplified and identified as sub-assemblage AII based on sequence analysis of the bg gene. Currently, there are three sub-assemblages (AI, AII and AIII) identified within assemblage A. Sub-assemblages AI and AII are commonly found in animals and humans, respectively; however, so far sub-assemblage AIII has been found only in animals, mostly in wildlife (Ryan et al., 2019). Although some studies indicated that contact with farm animals was associated with an increased risk of G. duodenalis infection for adults (Hoque et al., 2002; Hoque et al., 2003), genotyping and subtyping data point only to the potential role for zoonotic transmission with little epidemiological support (Xiao and Fayer, 2008). Sub-assemblage AII has ever been found in both pets (dogs) and their owners in Belgium (Claerebout et al., 2009). In India, genetically similar sub-assemblage AII isolates have been found in dogs and humans living within the same household (Traub et al., 2004). The same bg gene sequences of sub-assemblage AII were observed in human (here) and in sheep and cattle (previously). Meanwhile, at the tpi locus, one assemblage B isolate had 100% similarity with those from various animals (seen in Results). The finding of the same gene sequences of G. duodenalis isolates derived from humans and animals indicated the possibility of zoonotic transmission in the investigated areas. Due to the lack of data of G. duodenalis in local animals, the epidemiologic role of animals in the spread of giardiasis will be assessed.
Conclusion
This is the first molecular epidemiological investigation of Cryptosporidium spp. and G. duodenalis in humans in Myanmar. In general, G. duodenalis was more prevalent than Cryptosporidium spp. in the investigated areas. High percentage of non-diarrheal individuals infected with Cryptosporidium spp. and G. duodenalis should be made aware of the importance and epidemiological significance. Two unusual Cryptosporidium species (C. andersoni and C. viatorum) were identified, with a novel C. viatorum subtype XVcA2G1c being found for the first time. DNA sequences of Cryptosporidium spp. and G. duodenalis isolates from investigated people had high similarity or even identity of animal-derived isolates, implying the potential of zoonotic transmission. Due to unclear transmission patterns and dynamics of Cryptosporidium spp. and G. duodenalis, future research effort should focus on molecular epidemiological investigations of the two parasites in humans and animals living in close contact in the investigated areas, even in whole Myanmar. These data will aid in making efficient control strategies to intervene with and prevent occurrence of both diseases.
Data Availability Statement
The representative nucleotide sequences obtained in this study were deposited in the GenBank database under the following accession numbers: MW014313 to MW014315 (SSU rRNA) and MW014316 (gp60) for Cryptosporidium; MW011715 and MW011716 (tpi), MW011717 and MW011718 (bg) for G. duodenalis.
Ethics Statement
The studies involving human participants were reviewed and approved by The Ethics Committee of the National Institute of Parasitic Diseases, Chinese Center for Disease Control and Prevention, China, and the Myanmar Eastern Shan State Special Region 2 Ethic Health Organization. Written informed consent to participate in this study was provided by the participants’ legal guardian/next of kin.
Author Contributions
AL and YS designed this study. YW, BG, and HL performed the experiments. YW, BG, XL, YJ, and LY analyzed the data. JC contributed reagents/materials. YW and BG wrote the first draft of the manuscript and prepared the tables and the figures. AL and YS made the final revision. All authors contributed to the article and approved the submitted version.
Funding
This work was supported partially by the National Science and Technology Major Program of China (No. 2018ZX10713001-004 to YS), the National Key Research and Development Program of China (No. 2017YFD0501300 to YJ), the Fifth Round of Three-Year Public Health Action Plan of Shanghai (No. GWV-10.1-XK13 to JC).
Conflict of Interest
The authors declare that the research was conducted in the absence of any commercial or financial relationships that could be construed as a potential conflict of interest.
Acknowledgments
We thank the staff at the department of helminth, Yunnan Institute of Parasitic Diseases and the Centre for Disease Control and Prevention, and Zhiliang Zheng at Health Without Borders, for their assistance with sample collection and case investigation.
References
Adamu H., Petros B., Zhang G., Kassa H., Amer S., Ye J., et al. (2014). Distribution and clinical manifestations of Cryptosporidium species and subtypes in HIV/AIDS patients in Ethiopia. PLoS Negl. Trop. Dis. 8, e2831. doi: 10.1371/journal.pntd.0002831
Agholi M., Hatam G. R., Motazedian M. H. (2013). HIV/AIDS-associated opportunistic protozoal diarrhea. AIDS Res. Hum. Retroviruses 29, 35–41. doi: 10.1089/AID.2012.0119
Appelbee A. J., Frederick L. M., Heitman T. L., Olson M. E. (2003). Prevalence and genotyping of Giardia duodenalis from beef calves in Alberta, Canada. Vet. Parasitol. 112, 289–294. doi: 10.1016/s0304-4017(02)00422-3
Aye T., Moe K., Nyein M. M., Swe T. (1994). Cryptosporidiosis in Myanmar infants with acute diarrhea. Southeast Asian J. Trop. Med. Public Health 25, 654–656.
Ayinmode A. B., Zhang H., Dada-Adegbola H. O., Xiao L. (2014). Cryptosporidium hominis subtypes and Enterocytozoon bieneusi genotypes in HIV-infected persons in Ibadan, Nigeria. Zoonoses Public Health 61, 297–303. doi: 10.1111/zph.12072
Baldursson S., Karanis P. (2011). Waterborne transmission of protozoan parasites: review of worldwide outbreaks-an update 2004–2010. Water Res. 45, 6603–6614. doi: 10.1016/j.watres.2011.10.013
Bolland S. J., Zahedi A., Oskam C., Murphy B., Ryan U. (2020). Cryptosporidium bollandi n. sp. (Apicomplexa: Cryptosporidiiae) from angelfish (Pterophyllum scalare) and Oscar fish (Astronotus ocellatus). Exp. Parasitol. 217, 107956. doi: 10.1016/j.exppara.2020.107956
Braima K., Zahedi A., Oskam C., Reid S., Pingault N., Xiao L., et al. (2019). Retrospective analysis of Cryptosporidium species in Western Australian human populations, (2015–2018), and emergence of the C. hominis IfA12G1R5 subtype. Infect. Genet. Evol. 73, 306–313. doi: 10.1016/j.meegid.2019.05.018
Cacciò S. M., Lalle M., Svärd S. G. (2018). Host specificity in the Giardia duodenalis species complex. Infect. Genet. Evol. 66, 335–345. doi: 10.1016/j.meegid.2017.12.001
Cama V. A., Ross J. M., Crawford S., Kawai V., Chavez-Valdez R., Vargas D., et al. (2007). Differences in clinical manifestations among Cryptosporidium species and subtypes in HIV-infected persons. J. Infect. Dis. 196, 684–691. doi: 10.1086/519842
Cama V. A., Bern C., Roberts J., Cabrera L., Sterling C. R., Ortega Y., et al. (2008). Cryptosporidium species and subtypes and clinical manifestations in children, Peru. Emerg. Infect. Dis. 14, 1567–1574. doi: 10.3201/eid1410.071273
Chappell C. L., Okhuysen P. C., Langer-Curry R., Widmer G., Akiyoshi D. E., Tanriverdi S., et al. (2006). Cryptosporidium hominis: experimental challenge of healthy adults. Am. J. Trop. Med. Hyg. 75, 851–857.
Checkley W., White A. C., Jaganath D., Arrowood M. J., Chalmers R. M., Chen X.-M., et al. (2015). A review of the global burden, novel diagnostics, therapeutics, and vaccine targets for Cryptosporidium. The Lancet Infect. Dis. 15, 85–94. doi: 10.1016/s1473-3099(14)70772-8
Chen Y., Zheng W., Zhang N., Gui B., Lv Q., Yan J., et al. (2019). Identification of Cryptosporidium viatorum XVa subtype family in two wild rat species in China. Parasitol. Vectors 12, 502. doi: 10.1186/s13071-019-3763-6
Claerebout E., Casaert S., Dalemans A. C., De Wilde N., Levecke B., Vercruysse J., et al. (2009). Giardia and other intestinal parasites in different dog populations in Northern Belgium. Vet. Parasitol. 161, 41–46. doi: 10.1016/j.vetpar.2008.11.024
Damitie M., Mekonnen Z., Getahun T., Santiago D., Leyns L. (2018). Molecular epidemiology of Giardia duodenalis infection in humans in Southern Ethiopia: a triosephosphate isomerase gene-targeted analysis. Infect. Dis. Poverty 7, 17. doi: 10.1186/s40249-018-0397-4
de Lucio A., Amor-Aramendía A., Bailo B., Saugar J. M., Anegagrie M., Arroyo A., et al. (2016). Prevalence and Genetic Diversity of Giardia duodenalis and Cryptosporidium spp. among School Children in a Rural Area of the Amhara Region, North-West Ethiopia. PLoS One 11, e0159992. doi: 10.1371/journal.pone.0159992
Efstratiou A., Ongerth J. E., Karanis P. (2017). Waterborne transmission of protozoan parasites: Review of worldwide outbreaks-An update 2011–2016. Water Res. 114, 14–22. doi: 10.1016/j.watres.2017.01.036
Elwin K., Hadfield S. J., Robinson G., Crouch N. D., Chalmers R. M. (2012). Cryptosporidium viatorum n. sp. (Apicomplexa: Cryptosporidiidae) among travellers returning to Great Britain from the Indian subcontinent 2007–2011. Int. J. Parasitol. 42, 675–682. doi: 10.1016/j.ijpara.2012.04.016
Feleke H., Medhin G., Abebe A., Beyene B., Kloos H., Asrat D. (2018). Enteric pathogens and associated risk factors among under-five children with and without diarrhea in Wegera District, Northwestern Ethiopia. Pan. Afr. Med. J. 29, 72. doi: 10.11604/pamj.2018.29.72.13973
Feng Y., Ryan U. M., Xiao L. (2018). Genetic Diversity and Population Structure of Cryptosporidium. Trends Parasitol. 34, 997–1011. doi: 10.1016/j.pt.2018.07.009
Feng Y., Xiao L. (2011). Zoonotic potential and molecular epidemiologyof Giardia species and giardiasis. Clin. Microbiol. Rev. 24, 110–140. doi: 10.1128/CMR.00033-10
Gatei W., Suputtamongkol Y., Waywa D., Ashford R. W., Bailey J. W., Greensill J., et al. (2002). Zoonotic species of Cryptosporidium are as prevalent as the anthroponotic in HIV-infected patients in Thailand. Ann. Trop. Med. Parasitol. 96, 797–802. doi: 10.1179/000349802125002202
Gelanew T., Lalle M., Hailu A., Pozio E., Cacciò S. M. (2007). Molecular characterization of human isolates of Giardia duodenalis from Ethiopia. Acta Tropica 102, 92–99. doi: 10.1016/j.actatropica.2007.04.003
Geurden T., Geldhof P., Levecke B., Martens C., Berkvens D., Casaert S., et al. (2008). Mixed Giardia duodenalis assemblage A and E infections in calves. Int. J. Parasitol. 38, 259–264. doi: 10.1016/j.ijpara.2007.07.016
Guyot K., Follet-Dumoulin A., Lelièvre E., Sarfati C., Rabodonirina M., Nevez G., et al. (2001). Molecular characterization of Cryptosporidium isolates obtained from humans in France. J. Clin. Microbiol. 39, 3472–3480. doi: 10.1128/JCM.39.10.3472-3480.2001
Holubová N., Zikmundová V., Limpouchová Z., Sak B., Konečný R., Hlásková L., et al. (2019). Cryptosporidium proventriculi sp. n. (Apicomplexa: Cryptosporidiidae) in Psittaciformes birds. Eur. J. Protistol. 69, 70–87. doi: 10.1016/j.ejop.2019.03.001
Holubová N., Tůmová L., Sak B., Hejzlarová A., Konečný R., McEvoy J., et al. (2020). Description of Cryptosporidium ornithophilus n. sp. (Apicomplexa: Cryptosporidiidae) in farmed ostriches. Parasitol. Vectors 13, 340. doi: 10.1186/s13071-020-04191-2
Hoque M. E., Hope V. T., Kjellström T., Scragg R., Lay-Yee R. (2002). Risk of giardiasis in Aucklanders: a case-control study. Int. J. Infect. Dis. 6, 191–197. doi: 10.1016/s1201-9712(02)90110-4
Hoque M. E., Hope V. T., Scragg R., Kjellström T. (2003). Children at risk of giardiasis in Auckland: a case-control analysis. Epidemiol. Infect. 131, 655–662. doi: 10.1017/s0950268803008598
Huang D., Zhang R., Tang Y., Li X., Yang F., Wu C., et al. (2016). Establishment and application of PCR method for detection of Cryptosporidium. China. Tropical. Med. 16, 8. doi: 10.13604/j.cnki.46-1064/r.2016.08.07. (in Chinese).
Hunter P. R., Hughes S., Woodhouse S., Raj N., Syed Q., Chalmers R. M., et al. (2004). Health sequelae of human cryptosporidiosis in immunocompetent patients. Clin. Infect. Dis. 39, 504–510. doi: 10.1086/422649
Hussain G., Roychoudhury S., Singha B., Paul J. (2017). Incidence of Cryptosporidium andersoni in diarrheal patients from southern Assam, India: a molecular approach. Eur. J. Clin. Microbiol. Infect. Dis. 36, 1023–1032. doi: 10.1007/s10096-016-2887-2
Insulander M., Silverlås C., Lebbad M., Karlsson L., Mattsson J. G., Svenungsson B. (2013). Molecular epidemiology and clinical manifestations of human cryptosporidiosis in Sweden. Epidemiol. Infect. 141, 1009–1020. doi: 10.1017/S0950268812001665
Jiang Y., Ren J., Yuan Z., Liu A., Zhao H., Liu H., et al. (2014). Cryptosporidium andersoni as a novel predominant Cryptosporidium species in outpatients with diarrhea in Jiangsu Province, China. BMC Infect. Dis. 14, 555. doi: 10.1186/s12879-014-0555-7
Johnston A. R., Gillespie T. R., Rwego I. B., McLachlan T. L., Kent A. D., Goldberg T. L. (2010). Molecular epidemiology of cross-species Giardia duodenalis transmission in western Uganda. PLoS Negl. Trop. Dis. 4, e683. doi: 10.1371/journal.pntd.0000683
Karanis P., Kourenti C., Smith H. (2007). Waterborne transmission of protozoan parasites: a worldwide review of outbreaks and lessons learnt. J. Water Health 5, 1–38. doi: 10.2166/wh.2006.002
Khalil S., Mirdha B. R., Panda A., Singh Y., Makharia G., Paul J. (2017). Cryptosporidium species subtypes and associated clinical manifestations in Indian patients. Gastroenterol. Hepatol. Bed. Bench. 10, 311–318.
Khalil S., Mirdha B. R., Paul J., Panda A., Singh Y. (2018). Molecular Detection and Identification of Cryptosporidium viatorum in a Human Immunodeficiency Virus-seropositive Patient. J. Glob. Infect. Dis. 10, 28–29. doi: 10.4103/jgid.jgid_26_17
Kim M. J., Jung B. K., Cho J., Kim D. G., Song H., Lee K. H., et al. (2016). Prevalence of Intestinal Protozoans among Schoolchildren in Suburban Areas near Yangon, Myanmar. Korean J. Parasitol. 54, 345–348. doi: 10.3347/kjp.2016.54.3.345
Koehler A. V., Wang T., Haydon S. R., Gasser R. B. (2018). Cryptosporidium viatorum from the native Australian swamp rat Rattus lutreolus-An emerging zoonotic pathogen? Int. J. Parasitol. Parasites Wildl. 7, 18–26. doi: 10.1016/j.ijppaw.2018.01.004
Kváč M., Vlnatá G., Ježková J., Horčičková M., Konečný R., Hlásková L., et al. (2018). Cryptosporidium occultus sp. n. (Apicomplexa: Cryptosporidiidae) in rats. Eur. J. Protistol. 63, 96–104. doi: 10.1016/j.ejop.2018.02.001
Lalle M., Pozio E., Capelli G., Bruschi F., Crotti D., Cacciò S. M. (2005). Genetic heterogeneity at the β-giardin locus among human and animal isolates of Giardia duodenalis and identification of potentially zoonotic subgenotypes. Int. J. Parasitol. 35, 207–213. doi: 10.1016/j.ijpara.2004.10.022
Leoni F., Amar C., Nichols G., Pedraza-Díaz S., McLauchlin J. (2006). Genetic analysis of Cryptosporidium from 2414 humans with diarrhoea in England between 1985 and 2000. J. Med. Microbiol. 55, 703–707. doi: 10.1099/jmm.0.46251-0
Levecke B., Geldhof P., Claerebout E., Dorny P., Vercammen F., Cacciò S. M., et al. (2009). Molecular characterisation of Giardiaduodenalis in captive non–human primates reveals mixed assemblage A and B infections and novel polymorphisms. Int. J. Parasitol. 39, 1595–1601. doi: 10.1016/j.ijpara.2009.05.013
Liu H., Shen Y., Yin J., Yuan Z., Jiang Y., Xu Y., et al. (2014). Prevalence and genetic characterization of Cryptosporidium, Enterocytozoon, Giardia and Cyclospora in diarrheal outpatients in China. BMC Infect. Dis. 14, 25. doi: 10.1186/1471-2334-14-25
Liu A., Gong B., Liu X., Shen Y., Wu Y., Zhang W., et al. (2020). A retrospective epidemiological analysis of human Cryptosporidium infection in China during the past three decades, (1987–2018). PLoS Negl. Trop. Dis. 14, e0008146. doi: 10.1371/journal.pntd.0008146
Morse T. D., Nichols R. A., Grimason A. M., Campbell B. M., Tembo K. C., Smith H. V. (2007). Incidence of cryptosporidiosis species in paediatric patients in Malawi. Epidemiol. Infect. 135, 1307–1315. doi: 10.1017/S0950268806007758
Okhuysen P. C., Chappell C. L., Crabb J. H., Sterling C. R., DuPont H. L. (1999). Virulence of three distinct Cryptosporidium parvum isolates for healthy adults. J. Infect. Dis. 180, 1275–1281. doi: 10.1086/315033
Oliveira-Arbex A. P., David E. B., Oliveira-Sequeira T. C., Bittencourt G. N., Guimarães S. (2016). Genotyping of Giardia duodenalis isolates in asymptomatic children attending daycare centre: evidence of high risk for anthroponotic transmission. Epidemiol. Infect. 144, 1418–1428. doi: 10.1017/S0950268815002514
Osman M., El Safadi D., Cian A., Benamrouz S., Nourrisson C., Poirier P., et al. (2016). Prevalence and Risk Factors for Intestinal Protozoan Infections with Cryptosporidium, Giardia, Blastocystis and Dientamoeba among Schoolchildren in Tripoli, Lebanon. PLoS Negl. Trop. Dis. 10, e0004496. doi: 10.1371/journal.pntd.0004496
Plutzer J., Lassen B., Jokelainen P., Djurković-Djaković O., Kucsera I., Dorbek-Kolin E., et al. (2018). Review of Cryptosporidium and Giardia in the eastern part of Europe 2016. Euro. Surveill. 23, 16–00825. doi: 10.2807/1560-7917.ES.2018.23.4.16-00825
Prado M. S., Cairncross S., Strina A., Barreto M. L., Oliveira-Assis A. M., Rego S. (2005). Asymptomatic giardiasis and growth in young children; a longitudinal study in Salvador, Brazil. Parasitology 131, 51–56. doi: 10.1017/s0031182005007353
Rendtorff R. C. (1954). The experimental transmission of human intestinal protozoan parasites. II. Giardia lamblia cysts given in capsules. Am. J. Hygiene 59, 209–220. doi: 10.1093/oxfordjournals.aje.a119634
Roy S. L., DeLong S. M., Stenzel S. A., Shiferaw B., Roberts J. M., Khalakdina A., et al. (2004). Risk factors for sporadic cryptosporidiosis among immunocompetent persons in the United States from 1999 to 2001. J. Clin. Microbiol. 42, 2944–2951. doi: 10.1128/JCM.42.7.2944-2951.2004
Ryan U. M., Bath C., Robertson I., Read C., Elliot A., McInnes L., et al. (2005). Sheep may not be an important zoonotic reservoir for Cryptosporidium and Giardia parasites. Appl. Environ. Microbiol. 71, 4992–4997. doi: 10.1128/AEM.71.9.4992-4997.2005
Ryan U., Zahedi A., Paparini A. (2016). Cryptosporidium in humans and animals-a one health approach to prophylaxis. Parasite Immunol. 38, 535–547. doi: 10.1111/pim.12350
Ryan U., Hijjawi N., Feng Y., Xiao L. (2019). Giardia: an under–reported foodborne parasite. Int. J. Parasitol. 49, 1–11. doi: 10.1016/j.ijpara.2018.07.003
Ryan U., Cacciò S. M. (2013). Zoonotic potential of Giardia. Int. J. Parasitol. 43, 943–956. doi: 10.1016/j.ijpara.2013.06.001
Sánchez A., Munoz M., Gómez N., Tabares J., Segura L., Salazar Á, et al. (2017). Molecular Epidemiology of Giardia, Blastocystis and Cryptosporidium among Indigenous Children from the Colombian Amazon Basin. Front. Microbiol. 8, 248. doi: 10.3389/fmicb.2017.00248
Santín M., Trout J. M., Fayer R. (2007). Prevalence and molecular characterization of Cryptosporidium and Giardia species and genotypes in sheep in Maryland. Vet. Parasitol. 146, 17–24. doi: 10.1016/j.vetpar.2007.01.010
Savioli L., Smith H., Thompson A. (2006). Giardia and Cryptosporidium join the ‘Neglected Diseases Initiative’. Trends Parasitol. 22, 203–208. doi: 10.1016/j.pt.2006.02.015
Scaramozzino P., Di Cave D., Berrilli F., D’Orazi C., Spaziani A., Mazzanti S., et al. (2009). A study of the prevalence and genotypes of Giardia duodenalis infecting kennelled dogs. Vet. J. 182, 231–234. doi: 10.1016/j.tvjl.2008.07.003
Sprong H., Cacciò S. M., van der Giessen J. W., ZOOPNET network and partners (2009). Identification of zoonotic genotypes of Giardia duodenalis. PLoS Negl. Trop. Dis. 3, e558. doi: 10.1371/journal.pntd.0000558
Squire S. A., Ryan U. (2017). Cryptosporidium and Giardia in Africa: current and future challenges. Parasitol. Vectors 10, 195. doi: 10.1186/s13071-017-2111-y
Stensvold C. R., Elwin K., Winiecka-Krusnell J., Chalmers R. M., Xiao L., Lebbad M. (2015). Development and Application of a gp60-Based Typing Assay for Cryptosporidium viatorum. J. Clin. Microbiol. 53, 1891–1897. doi: 10.1128/JCM.00313-15
Su H., Huang X., Qin Y., Qin S., Hung Z., Wu N., et al. (2017). An investigation on the gene types of Cryptosporidium among HIV/AIDS patients. J. Med. Pest Control 33, 2. doi: 10.7629/yxdwfz201702011. (in Chinese).
Tellevik M. G., Moyo S. J., Blomberg B., Hjøllo T., Maselle S. Y., Langeland N., et al. (2015). Prevalence of Cryptosporidium parvum/hominis, Entamoeba histolytica and Giardia lamblia among Young Children with and without Diarrhea in Dar es Salaam, Tanzania. PLoS Negl. Trop. Dis. 9, e0004125. doi: 10.1371/journal.pntd.0004125
Traub R. J., Monis P. T., Robertson I., Irwin P., Mencke N., Thompson R. C. A. (2004). Epidemiological and molecular evidence supports the zoonotic transmission of Giardia among humans and dogs living in the same community. Parasitology 128, 253–262. doi: 10.1017/s0031182003004505
Ukwah B. N., Ezeonu I. M., Ezeonu C. T., Roellig D., Xiao L. (2017). Cryptosporidium species and subtypes in diarrheal children and HIV-infected persons in Ebonyi and Nsukka, Nigeria. J. Infect. Dev. Ctries. 11, 173–179. doi: 10.3855/jidc.8034
Waldron L. S., Dimeski B., Beggs P. J., Ferrari B. C., Power M. L. (2011). Molecular epidemiology, spatiotemporal analysis, and ecology of sporadic human cryptosporidiosis in Australia. Appl. Environ. Microbiol. 77, 7757–7765. doi: 10.1128/AEM.00615-11
Wang K., Gazizova A., Wang Y., Zhang K., Zhang Y., Chang Y., et al. (2019). First Detection of Cryptosporidium spp. in Migratory Whooper Swans (Cygnus cygnus) in China. Microorganisms 8, 6. doi: 10.3390/microorganisms8010006
Wang Y., Gonzalez-Moreno O., Roellig D. M., Oliver L., Huguet J., Guo Y., et al. (2019). Epidemiological distribution of genotypes of Giardia duodenalis in humans in Spain. Parasitol. Vectors 12, 432. doi: 10.1186/s13071-019-3692-4
Xiao L., Fayer R. (2008). Molecular characterisation of species and genotypes of Cryptosporidium and Giardia and assessment of zoonotic transmission. Int. J. Parasitol. 38, 1239–1255. doi: 10.1016/j.ijpara.2008.03.006
Xiao L., Feng Y. (2017). Molecular epidemiologic tools for waterborne pathogens Cryptosporidium spp. and Giardia duodenalis. Food. Waterborne Parasitol. 8–9, 14–32. doi: 10.1016/j.fawpar.2017.09.002
Xu N., Liu H., Jiang Y., Yin J., Yuan Z., Shen Y., et al. (2020). First report of Cryptosporidium viatorum and Cryptosporidium occultus in humans in China, and of the unique novel C. viatorum subtype XVaA3h. BMC Infect. Dis. 20, 16. doi: 10.1186/s12879-019-4693-9
Yu F., Li D., Chang Y., Wu Y., Guo Z., Jia L., et al. (2019). Molecular characterization of three intestinal protozoans in hospitalized children with different disease backgrounds in Zhengzhou, central China. Parasitol. Vectors 12, 543. doi: 10.1186/s13071-019-3800-5
Zhao Z., Dong H., Wang R., Zhao W., Chen G., Li S., et al. (2014). Genotyping and subtyping Cryptosporidium parvum and Giardia duodenalis carried by flies on dairy farms in Henan, China. Parasitol. Vectors 7, 190. doi: 10.1186/1756-3305-7-190
Zhao W., Zhou H., Huang Y., Xu L., Rao L., Wang S., et al. (2019). Cryptosporidium spp. in wild rats (Rattus spp.) from the Hainan Province, China: Molecular detection, species/genotype identification and implications for public health. Int. J. Parasitol. Parasites Wildl. 9, 317–321. doi: 10.1016/j.ijppaw.2019.03.017
Keywords: Cryptosporidium, Giardia, humans, genotyping, subtyping
Citation: Wu Y, Gong B, Liu X, Jiang Y, Cao J, Yao L, Li H, Liu A and Shen Y (2020) Identification of Uncommon Cryptosporidiumviatorum (a Novel Subtype XVcA2G1c) and Cryptosporidium andersoni as Well as Common Giardia duodenalis Assemblages A and B in Humans in Myanmar. Front. Cell. Infect. Microbiol. 10:614053. doi: 10.3389/fcimb.2020.614053
Received: 05 October 2020; Accepted: 30 October 2020;
Published: 25 November 2020.
Edited by:
Zhan Zhou, Zhejiang University, ChinaReviewed by:
Junqiang Li, Henan University of Traditional Chinese Medicine, ChinaMeng Qi, Tarim University, China
Copyright © 2020 Wu, Gong, Liu, Jiang, Cao, Yao, Li, Liu and Shen. This is an open-access article distributed under the terms of the Creative Commons Attribution License (CC BY). The use, distribution or reproduction in other forums is permitted, provided the original author(s) and the copyright owner(s) are credited and that the original publication in this journal is cited, in accordance with accepted academic practice. No use, distribution or reproduction is permitted which does not comply with these terms.
*Correspondence: Aiqin Liu, bGl1YWlxaW4xMTI4QDEyNi5jb20=; Yujuan Shen, YW15c2h5ajEyQDE2My5jb20=
†These authors have contributed equally to this work