- 1Center of Clinical Laboratory, Zhongshan Hospital, School of Medical, Xiamen University, Xiamen, China
- 2Department of Laboratory Medicine, Sichuan Provincial People’s Hospital, University of Electronic Science and Technology of China, Chengdu, China
- 3Department of Dermatology, Zhongshan Hospital, School of Medical, Xiamen University, Xiamen, China
Monocytes are widely involved in the body’s defense response, and abnormally regulated monocyte subsets are closely related to the pathogenesis of various diseases. It is unclear whether Treponema pallidum (Tp) dysregulates monocyte subsets and impacts the functions of monocytes. This study aims to analyze the distribution of monocyte subsets in syphilis patients and the effect of Tp on monocyte functions to explore the pathogenesis of syphilis. Flow cytometry was employed to detect monocyte subsets. With or without pre-treatment with rapamycin, THP-1 cell migration stimulated by Tp was investigated by a Transwell migration assay, and THP-1 cell phagocytosis was studied using fluorescent microspheres. IL-1β and TNF-α expression was quantified by PCR and flow cytometry, while LC3 and mTOR were investigated in Tp-exposed THP-1 cells using western blotting. Tp infection led to an increase in the proportion of CD14++CD16+ monocytes and a decrease in the proportion of CD14++CD16- monocytes. In addition, Tp promoted monocyte (THP-1) CD14 and CD16 expression in vitro, induced the expression of IL-1β and TNF-α in a dose-dependent manner and promoted the migration and autophagy of monocytes. Furthermore, mTOR phosphorylation on monocytes was stimulated by Tp, and the levels peaked at 30 min. Pre-treatment with rapamycin (mTOR inhibitor) attenuated the expression of IL-1β and migration in Tp-exposed THP-1 cells. Tp abnormally regulates monocyte subsets and promotes migration, autophagy, and the expression of IL-1β and TNF-α in THP-1 cells. Meanwhile, the mTOR affected the expression of IL-1β and migration in Tp-exposed THP-1 cells. This study is important as it sheds light on the mechanism by which monocytes interact with Tp during infection.
Introduction
Syphilis caused by Treponema pallidum (Tp) continues to be a prevalent disease, which raises public health concerns. The number of new syphilis cases reported in China increased to 587,464 in 2019 (https://www.cdc.gov/). Although the advent of penicillin is crucial to the treatment of syphilis, syphilis remains a worldwide public health problem. Tp infection triggers a complex host response that involves both innate and adaptive immunity (Rekart et al., 2017). Recently, increasing evidence has shown that host innate immune responses play critical roles in the containment of Tp infection (Salazar et al., 2007).
Monocytes are essential components of the innate immune system, accounting for approximately 10% of circulating leukocytes in humans and are responsible for phagocytosis of pathogens and dead cells, as well as antitumor activities (Parihar et al., 2010). A recent study suggests that circulating monocytes are important participants in the defense against a wide range of microbial pathogens, and monocyte polarization may differ in the development of different diseases (Serbina et al., 2008; Andrade et al., 2014). Based on the expression patterns of FcγRIII, also known as CD16, and the LPS receptor CD14, circulating human monocytes are classified into three subsets: classical monocytes (CD14++CD16− monocytes), non-classical monocytes (CD14+CD16++ monocytes) and intermediate monocytes (CD14++CD16+ monocytes). These different monocyte subpopulations can exhibit some distinct functional roles in a range of homeostatic and pathological conditions. It was reported that the heterogeneous monocyte population exerts its immune function through migration, phagocytosis, secretion of cytokines (Kapellos et al., 2019). In healthy donors, classical monocytes (CD14++CD16− monocytes) were primed for phagocytosis, innate sensing/immune responses and migration using a novel single-cell PCR gene expression analysis tool (Gren et al., 2015); however, Brucella infection led to an increase in the proportion of classical monocytes, which showed downregulation of immune responses (Wang Y. et al., 2017). Meanwhile, Nabatanzi et al. (2019) observed that the IL-1β production of classical monocytes among human immunodeficiency virus (HIV)-positive patients who received antiretroviral therapy was lower than that of those among healthy HIV-negative adults. Some studies have found that intermediate monocytes were well-suited for antigen presentation and cytokine secretion, while Szaflarska and colleagues (Szaflarska et al., 2004) described an antitumoral phenotype of these cells. Zawada et al. (2016) discovered that the distinct functional properties of monocyte subsets rely on the differential methylation status of immune-related genes. However, the exact role of monocyte subsets in immunity remains elusive, as there are different viewpoints toward this matter. To summarize, each monocyte subset has different features in different homeostasis and disease states. To the best of our knowledge, no detailed analysis of the different monocyte subsets in Tp infection and the effects of Tp on monocyte function has been reported up to date.
The goal of the present study was to analyze the peripheral blood mononuclear cell subset distribution of syphilis patients, meanwhile, to explore the pathogenic effects of Tp on monocyte polarization, inflammatory cytokine expression and migration in vitro.
Methods
Study Participants
This study was conducted between March 2019 and October 2019 at Zhongshan Hospital, School of Medicine, Xiamen University, China. During the study period, 55 syphilis patients diagnosed at Zhongshan Hospital, Xiamen University, were included in the study, and the same number of healthy controls were recruited. The selection criteria adhered to the United States Centers for Disease Control and Prevention guidelines (Workowski and Bolan, 2015), the European Centre for Disease Prevention and Control guidelines, and our previous report (Janier et al., 2014). Specimens from all participants were collected as previously published (Liu et al., 2019); whole blood was collected in 2-ml tube that contained EDTA-K2 for the flow cytometry assays, and subjects who had co-infection with human immunodeficiency virus (HIV), hepatitis B virus (HBV), and hepatitis C virus (HCV) were excluded. This study was approved by the Ethics Committee of Zhongshan Hospital, Xiamen University and was in accordance with the Declaration of Helsinki.
Flow Cytometry Assays
Flow cytometry was performed on a Mindray BriCyte E6 flow cytometer (Mindray, Shenzhen, China) as described in our previous paper (Liu et al., 2019). Briefly, participants’ peripheral blood samples and monocytes (THP-1 cells) were stained with the following antibodies: mouse anti-human CD14-PE and mouse anti-human CD16-PE-Cy7, anti-human gamma 1-PE and anti-human gamma 1-PE-Cy7 for non-fluorescent and non-specific fluorescence controls. In vitro experiments, intracellular staining was performed using the following mouse anti-human mAbs: TNF-α-PE-Cy7 and IL-1β-FITC. Antibodies were purchased from BioLegend (San Diego, CA, USA), except the anti-CD14-PE antibody (BD Biosciences, San Jose, CA, USA). Data were analyzed with FlowJo (TreeStar Software, Ashland, OR, USA).
Cell Culture
THP-1 cells (purchased from the American Type Culture Collection, Manassas, VA, USA) were incubated in RPMI-1640 medium (HyClone, Logan, USA) supplemented with 10% heat-inactivated fetal bovine serum (Biological Industries Ltd., Kibbutz Beit HaEmek, Israel), 100 U/ml penicillin, and 100 g/ml streptomycin (Invitrogen/Life Technologies, Carlsbad, CA, USA) at 37°C in 5% CO2. For stimulation experiments, THP-1 cells incubated were with Tp at different multiplicities of infection (MOIs) (Tp: cells 1:1, 10:1 and 20:1) at 37°C in 5% CO2 for 12 h in 12-well culture plates. Concurrently, the autophagy and mTOR were assessed by adding the inhibitors, which are 3-methyladenine (3-MA) (1 mM) and rapamycin (Rapa) (50 nM), respectively, dissolved in PBS to pre-treat the cells for 30 min. 3-MA and Rapa were purchased from Sigma-Aldrich (St. Louis, MO, USA).
Propagation of Tp
The Nichols strain was kindly donated by Lorenzo Giacani, PhD (University of Washington, Seattle, USA) and was maintained as previously described (Gao et al., 2018). The strain was propagated intratesticularly in adult male New Zealand white rabbits.
Real-Time PCR Assay
In order to evaluate the mRNA expression, total RNA was extracted from the cultured cells using an RNeasy kit (Tiangen Biotech Co., Ltd., Beijing, China) and then reverse transcripted with a high-capacity cDNA reverse transcription kit (Takara, Shiga, Japan) and sequenced using the Illumina HiSeq2500 platform by Gene Denovo Biotechnology Co (Guangzhou, China). DESeq2 software was performed. RNA differential expression analysis and principal component analysis were performed with R package gmodels (http://www.rproject.org/). Gene Ontology (GO) enrichment analysis provides all GO terms that are significantly enriched in differentially expressed genes (DEGs) comparing to the genome background, and filter the DEGs that correspond to biological functions. RT-PCR was carried out using a QuantiFast SYBR One-Step RT-PCR kit (Qiagen, Shanghai, China) and the LightCycler R96 instrument (Roche Diagnostics, Roche Instrument Center AG, Rotkreuz, Switzerland). The expression level was normalized with reference to the GAPDH housekeeping gene, and the relative gene expression was calculated using the 2−ΔΔCT method. The primers are listed in Supplementary Table 1.
Western Blotting Assays
Western blotting analysis was performed according to the method described in the literature (Deng et al., 2016). The primary (rabbit anti-human LC3, rabbit anti-human mTOR and mouse anti-human GAPDH antibodies) and secondary (anti-rabbit horseradish peroxidase-labeled antibody and anti-mouse horseradish peroxidase-labeled antibody) antibodies were purchased from Cell Signaling Technology (Danvers, MA, USA) and used at a dilution of 1:1,000 and 1:10,000, respectively. The results were quantified using ImageJ software.
Cell Migration Assays
For cell migration experiments, cells cocultured with Tp (MOI = 10) for 12 h were collected in 24-well Transwell plates (Corning Incorporation, NY, USA) Approximately 5 × 105 cells in a volume of 200 µl (serum free) were seeded in the upper chamber, and then 400 µl of RPMI 1640 medium with 10% FBS was added to the lower chambers of Transwell plates. The Transwell plates were incubated at 37°C with 5% CO2 for 2, 4, and 6 h. Cells that migrated to the lower chamber were counted by flow cytometry, in which flow cytometry absorbed 30 µl of cell suspension and calculated the cell concentration of each group.
Phagocytosis Assays
Phagocytic capacity was assayed by flow cytometry using FITC-dextran. Tp-treated cells, and an equal amount of PBS-treated cells as a control group was collected, and the removed cells (5 × 105) suspended in RPMI 1640 medium with 10% FBS were incubated together with FITC-dextran (0.1 mg/ml) at 37°C for 2, 4, and 6 h as described previously (Aryanpur et al., 2016). The cells were washed with PBS three times, and flow cytometry was used to detect the phagocytic rate of each group of cells.
Statistical Analysis
SPSS version 19.0 (SPSS Inc., Chicago, IL, USA) was used for statistical analysis of the experimental data. To be more specific, Shapiro–Wilk test was used to examine the normal distribution of continuous variables. Two-tailed Student’s t test and one-way analysis of variance (ANOVA) were used to compare means. A 2-sided P value less than 0.05 was considered statistically significant.
Results
Monocyte Subsets Associated With Syphilis
Among the 55 syphilis patients, 32 were male, and 23 were female. The median age of syphilis patients was 56 years (range: 38 to 74). All syphilis patients were subjected to reactive baseline serum RPR and serum TPPA tests. Among the 55 subjects in the control group, 23 were male, and 32 were female. The median age of the participants was 52 years (range: 39 to 65), and serum RPR and serum TPPA tests were negative. The characteristics of the study participants are summarized in Supplementary Table 2. To determine whether Tp affected the frequency and phenotype of monocyte subsets, cells were analyzed by flow cytometry, and the gating strategy is shown in Figure 1A. Based on CD14 and CD16 expression, the monocytes were divided into three subsets. The proportion of the intermediate monocyte (CD14++CD16+ monocytes) subsets was significantly elevated in syphilis patients compared to healthy controls (15.06 ± 10.75 vs. 5.52 ± 2.89%, P < 0.001), whereas the proportion of classical monocytes (CD14++CD16– monocytes) was significantly lower in syphilis patients than in healthy controls (68.97 ± 14.45% vs. 82.52 ± 4.56%, P < 0.001) (Figure 1B).
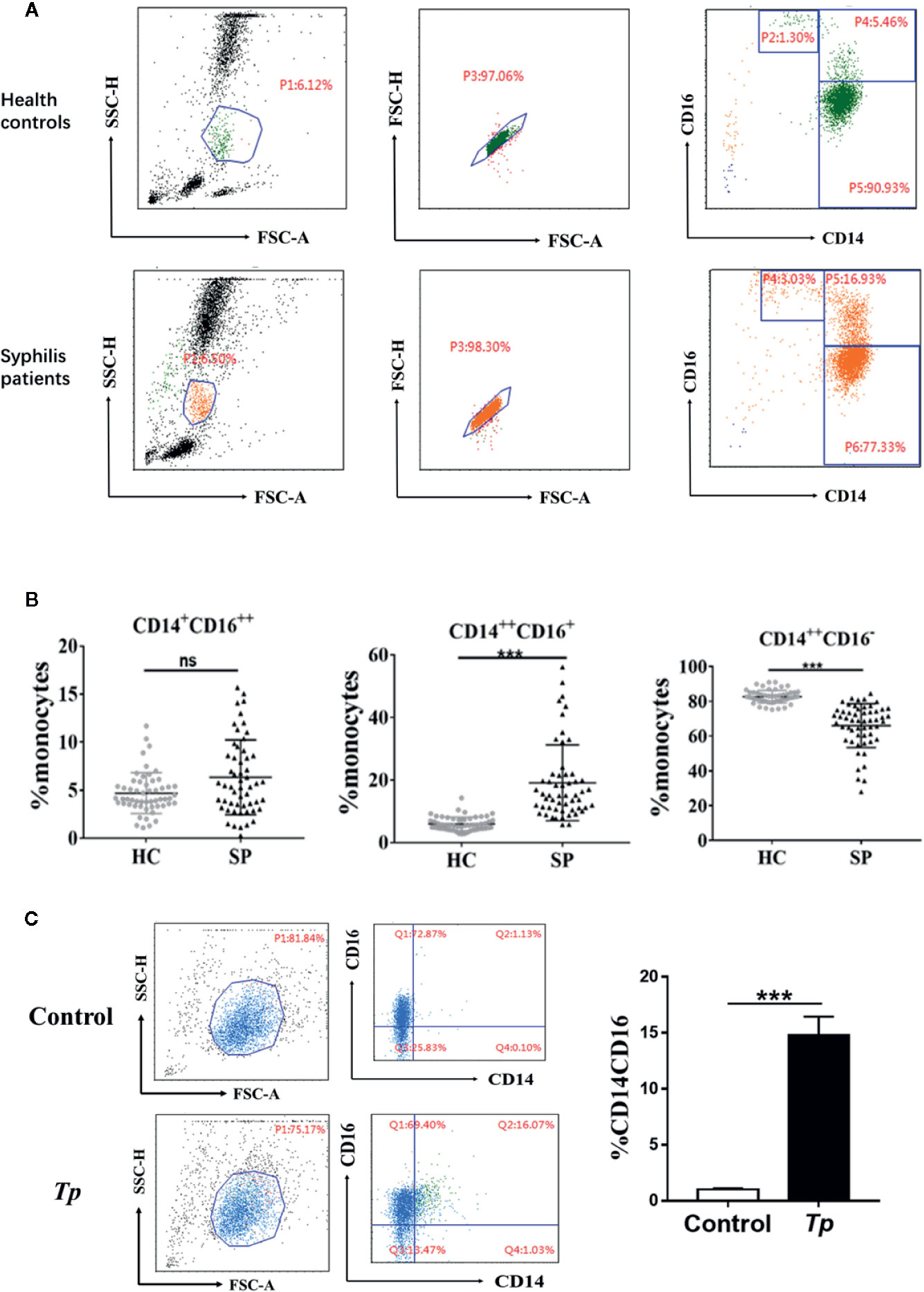
Figure 1 Characteristics of monocyte subsets in syphilis patients and healthy controls. (A) Gating strategy for monocyte subsets. (B) The percentage of each monocyte subset in syphilis patients (n = 55) and healthy controls (n = 55). (C) Tp promoted the expression of CD14 and CD16 in THP-1 cells. The data in the bar graphs are the means ± SEMs of three separate experiments. ***P < 0.001. FSC, forward scatter; SSC, side scatter; HC, healthy controls; SP, syphilis patient.
The effect of Tp on monocytes was further examined in vitro. When Tp was co-cultured with THP-1 cells, the proportion of the CD14+CD16+ monocyte subset significantly increased, compared with that of the control group (14.81 ± 0.9% vs. 1.03 ± 0.05%, P < 0.001, Figure 1C).
Differential Expression of mRNAs in Cells Between Tp-Exposed Monocytes THP-1 and Control Groups
To further elucidate the effects of Tp on monocytes, Tp (MOI = 10) was co-cultured with THP-1 cells for 12 h and transcriptome profiling by RNA-Seq was conducted. In total, 2,614 mRNAs were differentially expressed between the monocytes of three Tp-exposed monocyte groups and three PBS-treated controls, and those with a fold change greater than two were considered over-expressed or under-expressed (Figure 2A). After applying the criteria, specifically, 1,477 mRNAs were significantly upregulated, whereas 1,137 mRNAs were significantly downregulated in the Tp-exposed monocytes compared to PBS-treated monocytes (Figure 2B). There were 47 differentially expressed cytokines, of which three were downregulated, and 44 were upregulated. CXCL1, IL-1β, and TNF-α were the differentially regulated in Tp infected monocytes among the top 50 differential genes. In gene ontology (GO) analysis, which evaluates the enrichment of dysregulated mRNAs in cellular components, biological processes, and molecular functions, the most significant associations were observed with the following terms: immune system process, cell migration, and cell motility (Figure 2C).
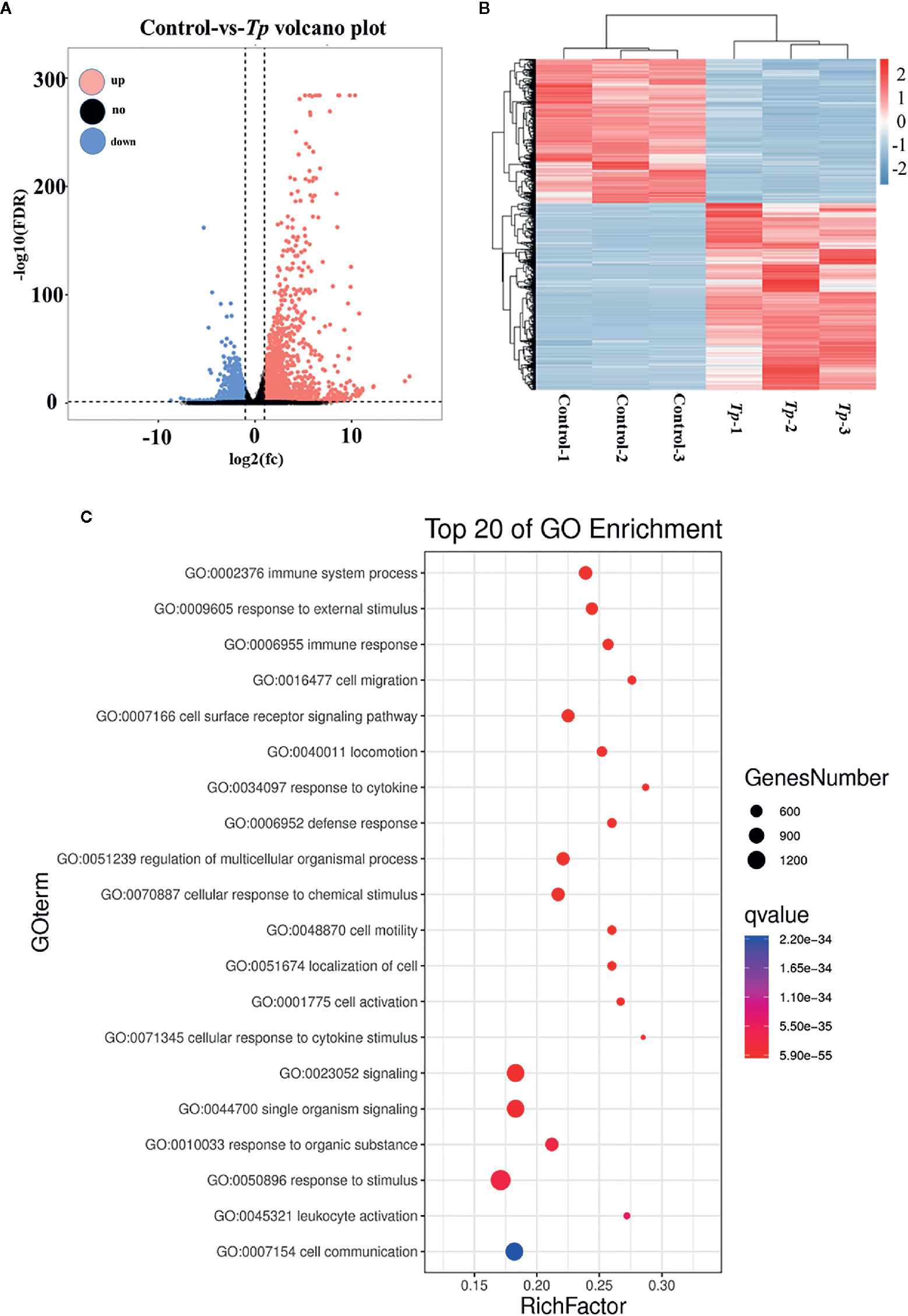
Figure 2 Differential expression of mRNAs in cells between the Tp and control groups. The volcano plots (A) and heatmap (B) demonstrate the differentially expressed mRNAs between the Tp-exposed THP-1 cells and PBS-treated control cells. In total, 1,477 mRNAs were significantly upregulated, and 1,137 mRNAs were significantly downregulated in the Tp-exposed THP-1 cells compared with control cells. The red dots indicate the mRNAs with upregulated expression, the blue dots indicate the mRNAs with downregulated expression, and the black dots indicate the mRNAs with no significant differences between groups. mRNAs with an expression fold change >2 and with an FDR-adjusted P < 0.05 were considered statistically significant. (C) GO analysis evaluated the pathways enriched by the top 20 dysregulated mRNAs.
Tp Changes the Function of Monocytes THP-1
Tp Promoted Immune System Process Changes in Monocytes THP-1
According to the GO analysis results of transcriptome profiling, the differentially expressed genes in Tp-exposed THP-1 cells were mostly involved in immune system processes. Therefore, the changes in immune function in THP-1 cells caused by Tp were analyzed.
Tp Induced the Expression of IL-1β and TNF-α in THP-1 Cells
To verify the effects of Tp on monocyte function, we firstly detected the expression levels of IL-1β mRNA and TNF-α mRNA in THP-1 cells incubated with Tp at different MOIs. As shown in Figures 3A, B, the expression of IL-1β mRNA in Tp-treated monocytes at different MOIs (Tp: cells 1:1, 10:1 and 20:1) was significantly increased by 401.8 ± 6.01, 1,929 ± 60.47, and 3,909 ± 137.5 times compared to the control group, respectively. Meanwhile, the expression of TNF-α mRNA in monocytes increased significantly compared with the control group by 14.67 ± 2.07, 19.09 ± 0.85, and 24.77 ± 1.83 times (P < 0.001), respectively. Next, the expression levels of IL-1β protein and TNF-α protein were detected by flow cytometry. As shown in the Figures 3C, D, the average fluorescence intensities of IL-1β and TNF-α increased with increasing MOIs of Tp. This demonstrated a dose-dependent effect (P < 0.05).
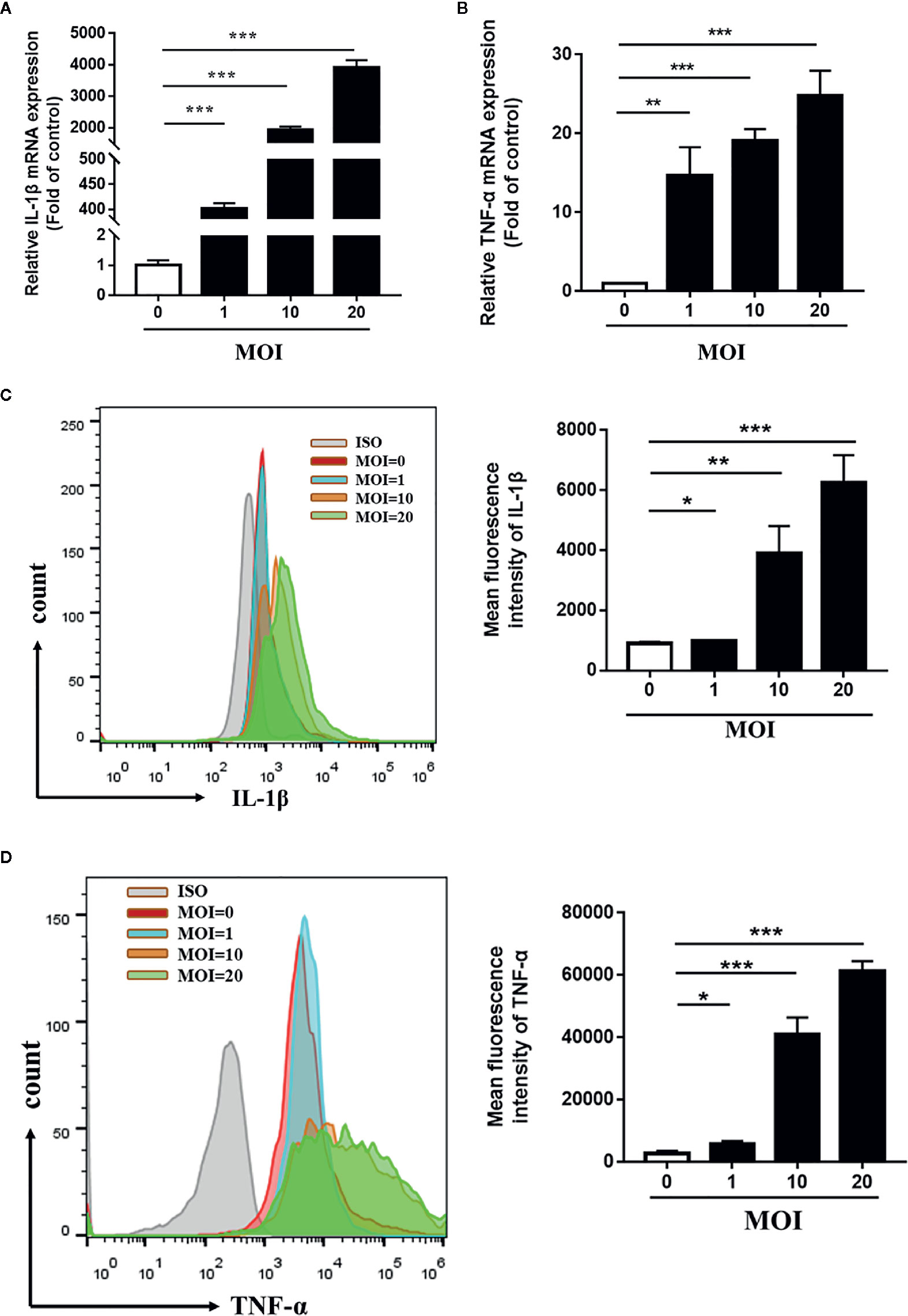
Figure 3 Tp-induced expression of IL-1β and TNF-α in THP-1 cells. THP-1 cells were incubated with Tp at different MOIs for 12 h. The mRNA expression of IL-1β and TNF-α was evaluated by RT-PCR (A, B). The protein expression of IL-1β and TNF-α was evaluated by flow cytometry (C, D). (*P < 0.05; **P < 0.01; ***P < 0.001). MOI, multiplicity of infection.
mTOR Was Essential for the Expression of IL-1β on Tp-Treated THP-1 Cells
To explore the mechanism of Tp’s effect on monocyte function, we first evaluated the role of mTOR on monocyte function with Tp-treated cells by western blotting. The phosphorylation of mTOR (P-mTOR), a well-defined indicator of mTOR protein activation, was stimulated on monocytes by Tp in a time-dependent manner, and the concentration peak appeared at 30 min and then declined over time (Figure 4A). Next, THP-1 cells were pre-treated with Rapa (mTOR inhibitor), which inhibited the phosphorylation of mTOR (Figure 4B), and then attenuated the expression of IL-1β mRNA and IL-1β protein induced by Tp (Figures 4C, E). The mRNA expression and protein expression of TNF-α were also downregulated, but the differences were not significant (Figures 4D, F).
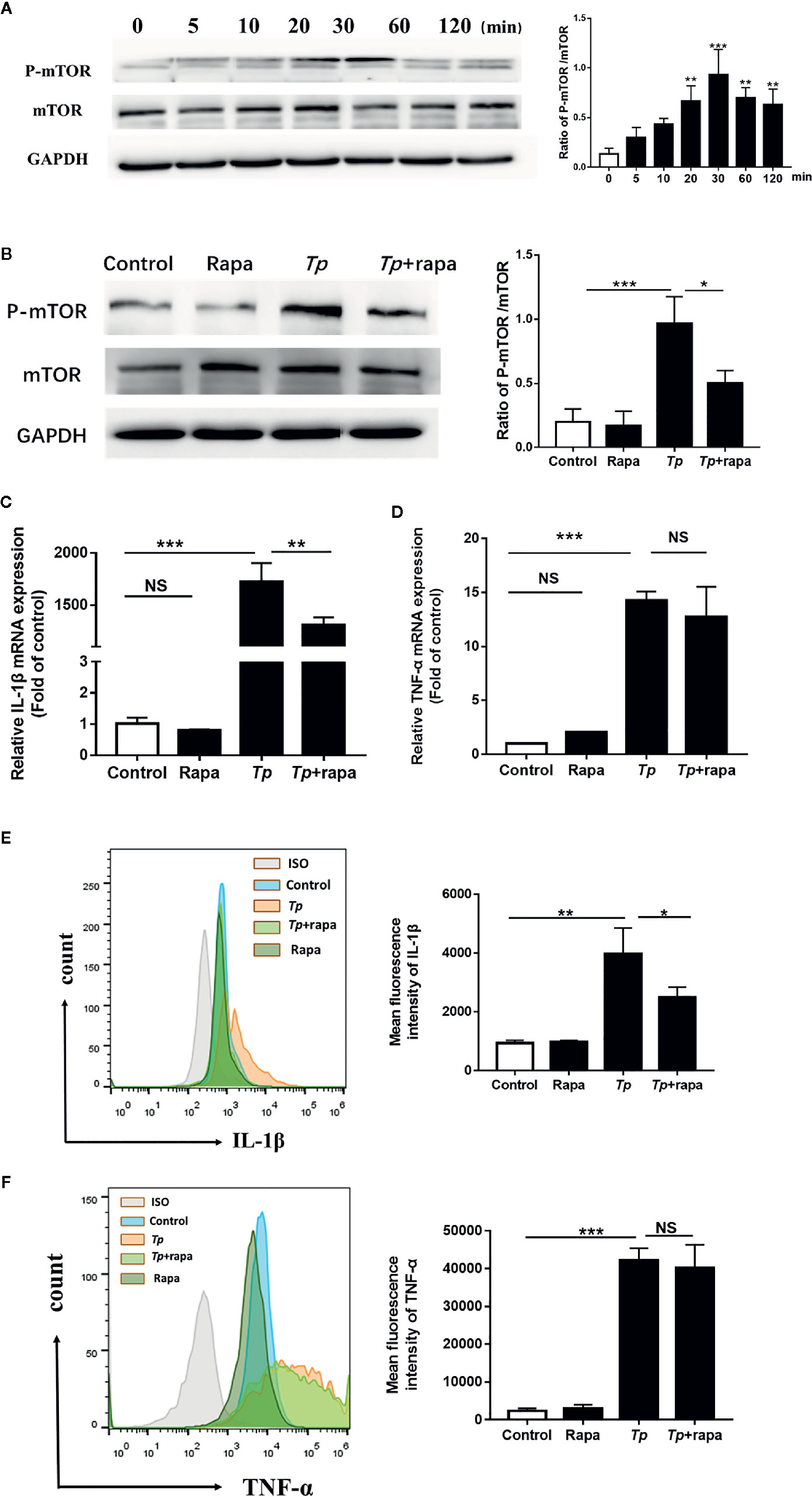
Figure 4 mTOR, but not autophagy, was essential for the expression of IL-1β induced by Tp. (A) THP-1 cells were incubated with Tp at an MOI of 10 for different amounts of time, and the levels of phosphorylated and total mTOR protein were detected by Western blotting. (B) THP-1 cells were pre-treated with Rapa (50 nM) for 30 min and then incubated with Tp at an MOI of 10 for 30 min. The levels of phosphorylated and total mTOR protein were detected by Western blotting. (C, D) THP-1 cells were pre-treated with Rapa (50 nM) for 30 min and then incubated with Tp at an MOI of 10 for 12 h. The levels of IL-1β and TNF-α were evaluated by RT-PCR. (E, F) The protein expression of IL-1β and TNF-α was detected by flow cytometry. (*P < 0.05; **P < 0.01; ***P < 0.001).
Autophagy is closely related to the secretion of inflammatory cytokines (Cadwell, 2016). In the present study, the autophagy level of Tp-exposed monocytes was significantly increased compared to that in the control group (Supplementary Figure 1A). However, when the autophagy was suppressed by pre-treatment with 3-MA (Supplementary Figure 1B), IL-1β and TNF-α expression showed no significant changes (Supplementary Figures 1C–F).
Effect of TP on Phagocytosis of Monocytes THP-1
The phagocytosis of innate immune cells is essential for the clearance of pathogens invading the body. We used fluorescent microspheres to detect the phagocytic capacity of monocytes after Tp stimulation. After THP-1 cells were incubated with FITC-dextran for 2, 4, and 6 h; the phagocytic rates of the monocytes in the experimental group and the control group showed no significant differences (P > 0.05, Figures 5A, B and Supplementary Figure 2).
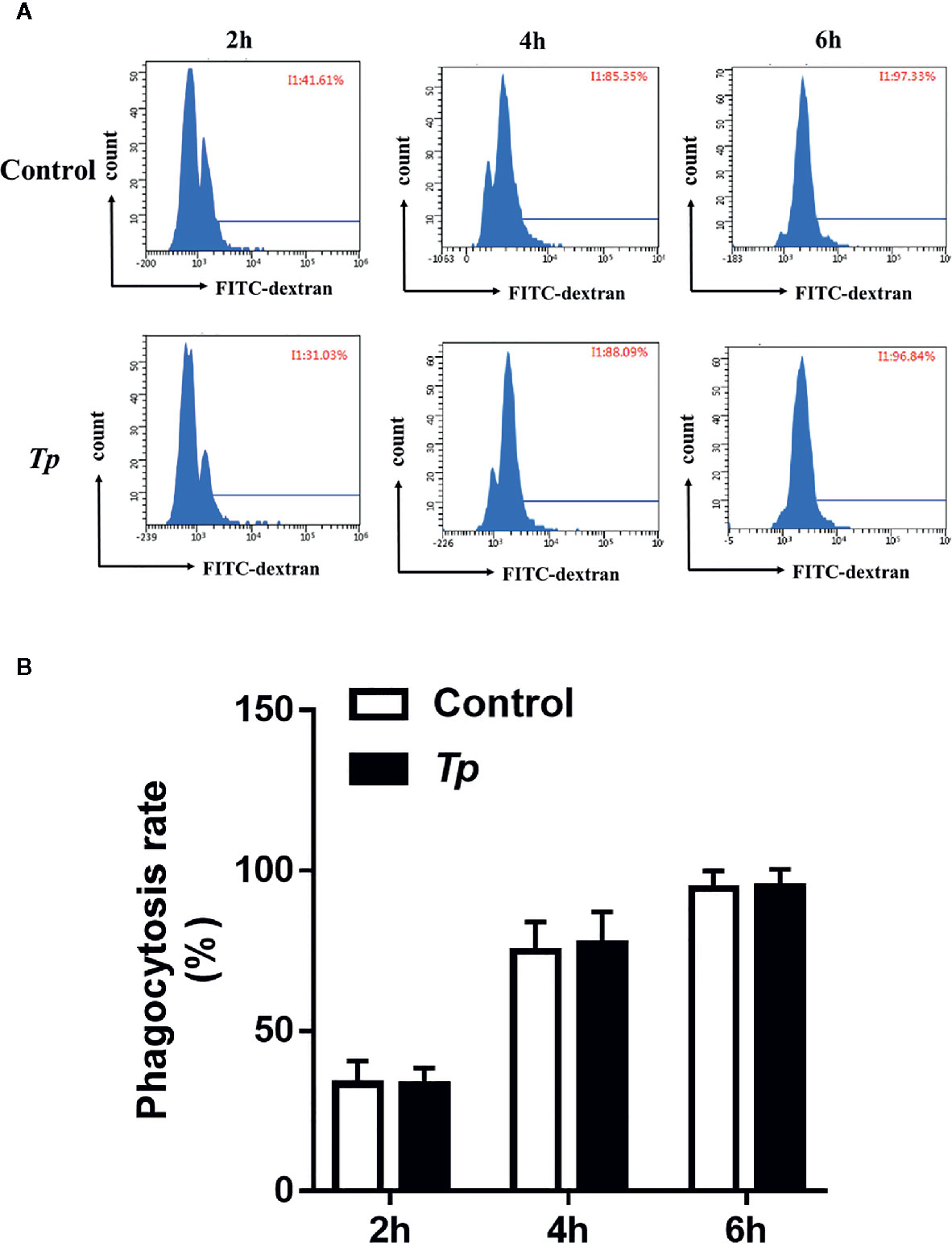
Figure 5 Tp had no effect on the phagocytosis of monocytes. (A) THP-1 cells were incubated with Tp at an MOI of 10 for 12 h, and then, the phagocytosis ability of monocytes was tested by flow cytometry. (B) The data in the bar graphs are the means ± SEMs of three separate experiments.
Tp Promoted Monocyte THP-1 Migration
According to the GO analysis results of transcriptome profiling, the differentially expressed genes in Tp-exposed THP-1 cells were enriched in the primary function of cell migration. Therefore, we analyzed changes in Tp-induced THP-1 cell migration. To examine the effects of Tp on monocyte migration ability, we added RPMI 1640 medium with 10% FBS to the lower chambers of a Transwell system containing monocytes pre-exposed to Tp in the upper chambers. The number of cells pre-exposed to Tp that migrated to the lower chambers at the 4 and 6 h was significantly higher than that of the cells not pre-exposed to Tp that migrated to the lower chambers. There were no significant differences in the number of cells during the 2 h migration. The results are shown in Figures 6A, B.
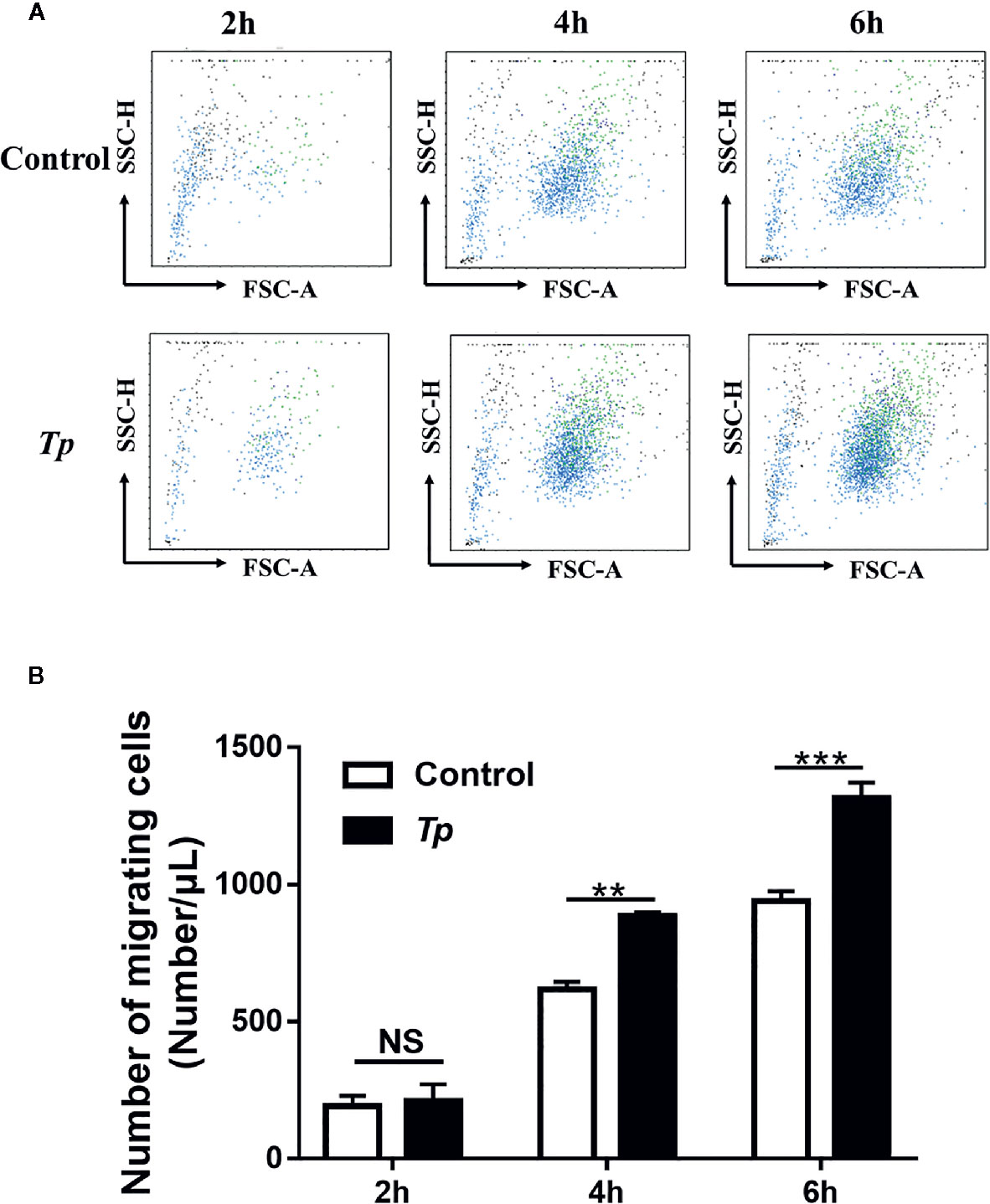
Figure 6 Tp promoted monocyte migration. (A) THP-1 cells were incubated with Tp at an MOI of 10 for 12 h. Then, the number of migrated cells was detected by using flow cytometry at different time points (2, 4, and 6 h). (B) The data in the bar graphs are the means ± SEMs of three separate experiments. (**P < 0.01; ***P < 0.001).
Tp Altered Monocyte THP-1 Migration via mTOR
After Rapa pre-treatment, THP-1 cells were cocultured with Tp for 12 h. The migrated monocytes in the lower chamber were collected at 6 h and counted by flow cytometry. Compared with that in the inhibitor-free group, the number of cells migrating to the lower chamber in the Rapa pre-treatment group was significantly reduced (P < 0.01, Figures 7A, B).
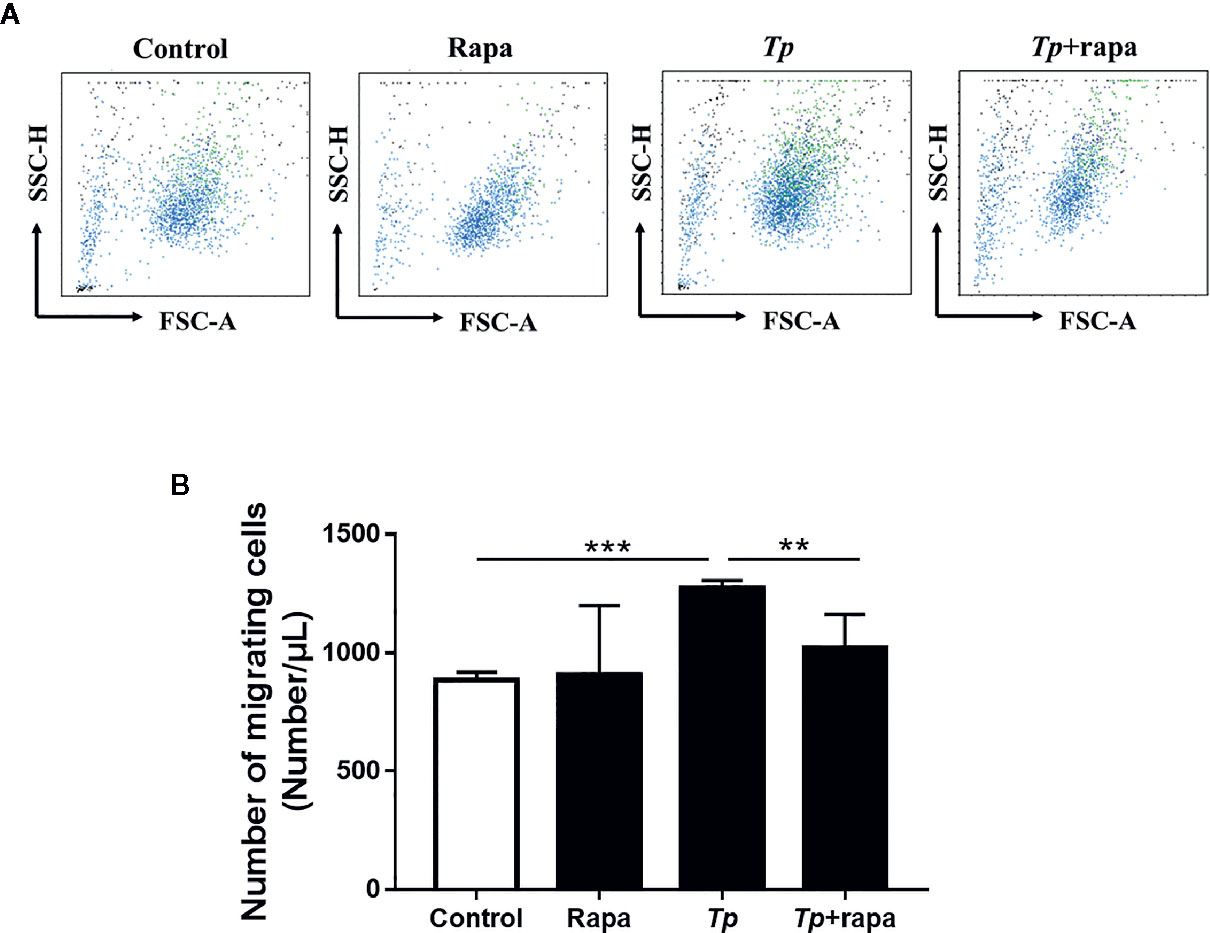
Figure 7 Tp altered monocyte migration via mTOR. (A) THP-1 cells were pre-treated with Rapa (50 nM) for 30 min and then incubated with Tp at an MOI of 10 for 12 h. Then, the number of cells that migrated to the lower chamber at 6 h was detected by using flow cytometry. (B) The data in the bar graphs are the means ± SEMs of three separate experiments. (**P < 0.01; ***P < 0.001).
Discussion
Abnormalities of monocyte subsets have been reported in many studies and was associated with a wide range of diseases and related to disease prognosis (Hirose et al., 2019; Nabatanzi et al., 2019). Our study found that syphilis patients demonstrate changes in monocyte subpopulations, of which intermediate monocytes were significantly increased. In addition, Tp could increase the expression of CD14 and CD16 in a human monocyte cell line (THP-1) in vitro and promote the transformation of monocytes into intermediate monocytes (CD14++CD16+ monocytes). Because intermediate monocytes controlled the differentiation of Treg subsets in HIV-1 coinfections (Guo et al., 2019), we speculated that the increase in intermediate monocytes in syphilis patients may be important for the differentiation of T lymphocyte subsets and the immune evasion of Tp. Studies have also found a significant increase in Treg cells in syphilis patients with sero-resistance (Zhao et al., 2016) and abnormal T lymphocyte subsets in neurosyphilis (Liu et al., 2019). In addition, upon viral infection, non-classical monocytes exhibited strong pro-inflammatory properties that skewed the immune response towards a Th2 profile (Kang et al., 2020). In agreement with our findings, the monocyte subpopulation in patients with brucellosis was also abnormal; what was different from our study was that the number of CD14++CD16− monocytes was significantly higher in patients with brucellosis than the numbers of other monocyte subsets. This suggests that each monocyte subset plays divergent roles in different diseases, as well as differing in the ability to secrete cytokines.
Intermediate monocytes (CD14++CD16+ monocytes), compared with the classical monocyte subsets (CD14++CD16− monocytes), showed a higher phagocytosis rate and secreted higher levels of IL-1β and TNF-α (Andrade et al., 2014). This study showed that Tp promoted IL-1β and TNF-α expression in monocytes THP-1, which was in agreement with a previous study (Babolin et al., 2011). IL-1β and TNF-α are pro-inflammatory cytokines that can cause inflammatory responses and tissue destruction. Through these inflammatory factors, immune cells such as neutrophils, macrophages, T lymphocytes, and B lymphocytes can be recruited, which leads to the inflammation of the skin and blood vessels (Gao et al., 2019). Inflammation in tissues is accompanied by the continued presence of Tp to maintain a strong immune response (Hawley et al., 2017). A study found that the levels of IL-1β, IL-6, and TNF-α were elevated in serum collected from syphilis patients (Peng et al., 2019). Although studies have shown the expression of Tp-induced pro-inflammatory cytokines (Zhu et al., 2017; Lin et al., 2018a; Lin et al., 2018b), very little is known about the Tp-mediated intracellular signaling pathway activation that leads to cytokine expression in monocytes.
To uncover the potential roles of monocytes in the occurrence of syphilis, a GO analysis was performed with the differentially expressed mRNAs in the Tp-exposed monocytes THP-1 and control cells. The differentially expressed mRNAs were mainly involved in biological processes. Among them, the most significant associations were observed with the following terms: immune system process, immune response, cell migration, response to cytokine, and cell activation, and these results are consistent with our results that Tp mainly affects monocyte migration and secretion of IL-1β and TNF-α; however, no significant effect on the phagocytic function of monocytes was observed. Radolf et al. (Moore et al., 2007) found that Tp had the ability to avoid detection and uptake by virtue of its denuded outer membrane. Cruz et al. (2012) observed that while human syphilitic sera promoted uptake of Tp in conjunction with monocyte activation, most Tp bacteria were not internalized. These results indicate that Tp does not affect the phagocytic system of monocytes, thus achieving the purpose of being able to escape immune clearance.
A subsequent KEGG pathway analysis also found the abnormal mTOR and autophagy signaling pathways in the Tp-induced monocytes. Mammalian target of rapamycin (mTOR) is a central regulator of growth and host immunity cells. Our previous research showed that the activation of the mTOR pathway in the pathological process of Tp infection caused the differentiation of macrophages (Zeng et al., 2016; Lin et al., 2018a). In this study, we further found that mTOR activated by Tp was involved in IL-1β secretion but not in TNF-α expression. It proved that Tp regulated IL-1β secretion through the mTOR pathway. In addition to the mTOR signaling pathway, recent studies have found that autophagy is closely related to inflammation (Matsuzawa et al., 2012; Bah and Vergne, 2017; Wang XL et al., 2017), which represents a major adaptive response for maintaining cellular and tissue homeostasis. Our research found that Tp also significantly increased the level of autophagy in monocytes THP-1, but autophagy had no effect on the secretion of cytokines IL-1β and TNF-α stimulated by Tp. Autophagy regulates cytokine secretion depending on the cellular context. Pun et al. (2015) found that autophagy decreased TNF-α expression in primary macrophages. However, in human peripheral blood mononuclear cells stimulated with LPS, 3-MA decreased the transcription of TNF-α while upregulated the transcription of IL-1β (Crisan et al., 2011). The effect of autophagy on the inflammatory status was different in cells and pathogens. Based on our current results, Tp-induced autophagy had no effect on the inflammatory response. The role of Tp-induced autophagy needs to be further studied.
Inflammatory responses following exposure to a stimulator are highly dependent on the migration of monocytes. We found that Tp increased the migration ability of monocytes and promoted the expression of monocyte migration-related genes through GO analysis. Enhanced monocyte migration exacerbates the formation of tissue inflammation injury. A large number of monocytes, T lymphocytes, and other immune cells accumulate in syphilis skin lesions (Cruz et al., 2012). Tp promoted the expression of intercellular adhesion molecules in endothelial cells, the overexpression of matrix metalloproteinases, and the recruitment of monocytes to endothelial cells (Gao et al., 2019; Zang et al., 2019). However, Zhang et al. (Hu et al., 2020) found that Tp stimulated macrophages to produce exosomes containing miR-146a-5p, which reduced the expression of adhesion-reducing molecules in endothelial cells, as well as endothelial migration of monocytes. The effects of Tp on the migration of monocytes were varied. Our experiments started with Tp-stimulated monocytes and revealed a novel fact in syphilis pathophysiology. Our results showed that mTOR activated by Tp was involved in monocyte migration. After adding rapamycin, an inhibitor of mTOR protein, the Tp-induced monocyte migration ability was significantly inhibited.
In this study, we investigated the effect of Tp on peripheral monocyte subsets and functions in vitro. Tp induces the transition of monocytes into intermediate subpopulations (CD14++CD16+ monocytes), promotes monocyte secretion of IL-1β, and increases monocyte migration capacity through the mTOR pathway. These data may be useful for understanding the pathogen–host relationship and the pathogenesis of syphilis. Further studies are required to understand the role of monocytes in Tp-infected animals. In addition, the analysis of monocyte subsets in syphilis at different clinical stages would better elucidate the pathogenesis of Tp. Meanwhile, Tp might also affect other pathways in THP-1 cells, such as pathways involving phosphatases, and further studies are needed for related mechanisms.
Data Availability Statement
The original contributions presented in the study are included in the article/Supplementary Materials. Further inquiries can be directed to the corresponding author.
Ethics Statement
The studies involving human participants were reviewed and approved by the ethics committee of Zhongshan Hospital, Xiamen University. The patients/participants provided their written informed consent to participate in this study.
Author Contributions
W-NL and L-LL conceived and designed the experiments. W-NL performed the experiments. W-NL, K-XW, and X-HS analyzed the data. X-YJ, Y-ZX, X-LH, YL, L-RL, and M-LT contributed reagents, materials, and analysis tools. W-NL, YL, and L-LL wrote the paper. All authors contributed to the article and approved the submitted version.
Funding
This work was supported by the National Natural Science Foundation of China (grant numbers 81971147, 81771312, 81471231, 81101324, 81672094, 81871729, 81972028). The funders played no role in the study design, data collection, or analyses, the decision to publish, or manuscript preparation.
Conflict of Interest
The authors declare that the research was conducted in the absence of any commercial or financial relationships that could be construed as a potential conflict of interest.
Supplementary Material
The Supplementary Material for this article can be found online at: https://www.frontiersin.org/articles/10.3389/fcimb.2020.592864/full#supplementary-material
Supplementary Figure 1 | Autophagy was not involved in the expression of IL-1β induced by Tp. (A, B) THP-1 cells were incubated with Tp at an MOI of 10 for 12 h with or without pre-treatment with 3-MA (1 mM) for 30 min, and the levels of LC3 were detected by western blotting. (C, D) THP-1 cells were pre-treated with 3-MA (1 mM) for 30 min and then incubated with Tp at an MOI of 10 for 12 h. The levels of IL-1β and TNF-α were evaluated by RT-PCR and (E, F). The protein expression of IL-1β and TNF-α was detected by flow cytometry.
Supplementary Figure 2 | Tp had no effect on the phagocytosis of monocytes. THP-1 cells were incubated with Tp at an MOI of 10 for 12 h, and then, the phagocytosis ability of monocytes was tested by flow cytometry showed in one histogram from different time points.
Abbreviations
FSC, forward scatter; SSC, side scatter; HC, healthy controls; SP, syphilis patients; Tp, Treponema pallidum; HIV, human immunodeficiency virus; HBV, hepatitis B virus; HCV, hepatitis C virus; RPR, rapid plasma regain; TPPA, treponema pallidum gelatin; agglutination; ANOVA, one-way analysis of variance; MOI, multiplicity of infection; Rapa, rapamycin; mTOR, mammalian target of rapamycin.
References
Andrade B. B., Singh A., Narendran G., Schechter M. E., Nayak K., Subramanian S., et al. (2014). Mycobacterial antigen driven activation of CD14++CD16- monocytes is a predictor of tuberculosis-associated immune reconstitution inflammatory syndrome. PloS Pathog. 10 (10), e1004433. doi: 10.1371/journal.ppat.1004433
Aryanpur M., Mortaz E., Masjedi M. R., Tabarsi P., Garssen J., Adcock I. M., et al. (2016). Reduced Phagocytic Capacity of Blood Monocyte/Macrophages in Tuberculosis Patients Is Further Reduced by Smoking. Iran. J. Allergy Asthma Immunol. 15 (3), 174–182. doi: 10.1183/13993003.congress-2016.OA1480
Babolin C., Amedei A., Ozolins D., Zilevica A., D’Elios M. M., de Bernard M. (2011). TpF1 from Treponema pallidum activates inflammasome and promotes the development of regulatory T cells. J. Immunol. (Baltimore Md 1950) 187 (3), 1377–1384. doi: 10.4049/jimmunol.1100615
Bah A., Vergne I. (2017). Macrophage Autophagy and Bacterial Infections. Front. Immunol. 8, 1483. doi: 10.3389/fimmu.2017.01483
Cadwell K. (2016). Crosstalk between autophagy and inflammatory signalling pathways: balancing defence and homeostasis. Nat. Rev. Immunol. 16 (11), 661–675. doi: 10.1038/nri.2016.100
Crisan T. O., Plantinga T. S., van de Veerdonk F. L., Farcaş M. F., Stoffels M., Kullberg B-J., et al. (2011). Inflammasome-independent modulation of cytokine response by autophagy in human cells. PloS One 6 (4), e18666. doi: 10.1371/journal.pone.0018666
Cruz A. R., Ramirez L. G., Zuluaga A. V., Pillay A., Abreu C., Valencia C. A., et al. (2012). Immune evasion and recognition of the syphilis spirochete in blood and skin of secondary syphilis patients: two immunologically distinct compartments. PLoS Negl. Trop. Dis. 6 (7), e1717. doi: 10.1371/journal.pntd.0001717
Deng Q. C., Wang Y., Zhang Y. Q., Li M., Li D., Huang X., et al. (2016). Pseudomonas aeruginosa Triggers Macrophage Autophagy To Escape Intracellular Killing by Activation of the NLRP3 Inflammasome [Article]. Infect. Immun. 84 (1), 56–66. doi: 10.1128/IAI.00945-15
Gao K., Shen X., Lin Y., Zhu X-Z., Lin L-R., Tong M-L., et al. (2018). Origin of Nontreponemal Antibodies During Treponema pallidum Infection: Evidence From a Rabbit Model. J. Infect. Dis. 218 (5), 835–843. doi: 10.1093/infdis/jiy241
Gao Z. X., Liu L. L., Lin L. R., Tong M.-L., Liu F., Yang T-C. (2019). Treponema pallidum Induces the Secretion of HDVSMC Inflammatory Cytokines to Promote the Migration and Adhesion of THP-1 Cells. Front. Cell. Infect. Microbiol. 9, 220. doi: 10.3389/fcimb.2019.00220
Gren S. T., Rasmussen T. B., Janciauskiene S., Håkansson K., Gerwien J. G., Grip O. (2015). A Single-Cell Gene-Expression Profile Reveals Inter-Cellular Heterogeneity within Human Monocyte Subsets. PloS One 10 (12), e0144351. doi: 10.1371/journal.pone.0144351
Guo N., Liu L., Yang X., Song T., Li G., Li L., et al. (2019). Immunological Changes in Monocyte Subsets and Their Association With Foxp3 Regulatory T Cells in HIV-1-Infected Individuals With Syphilis: A Brief Research Report. Front. Immunol. 10, 714. doi: 10.3389/fimmu.2019.00714
Hawley K. L., Cruz A. R., Benjamin S. J., La Vake C. J., Cervantes J. L., LeDoyt M., et al. (2017). Treponema pallidumIFNγ Enhances CD64-Potentiated Phagocytosis of Opsonized with Human Syphilitic Serum by Human Macrophages. Front. Immunol. 8, 1227. doi: 10.3389/fimmu.2017.01227
Hirose S., Lin Q., Ohtsuji M., Nishimura H., Sjef Verbeek J. (2019). Monocyte subsets involved in the development of systemic lupus erythematosus and rheumatoid arthritis. Int. Immunol. 31 (11), 687–696. doi: 10.1093/intimm/dxz036
Hu W., Xu B., Zhang J., Kou C., Liu J., Wang Q., et al. (2020). Exosomal miR-146a-5p from Treponema pallidum-stimulated macrophages reduces endothelial cells permeability and monocyte transendothelial migration by targeting JAM-C. Exp. Cell Res. 388 (1), 111823. doi: 10.1016/j.yexcr.2020.111823
Janier M., Hegyi V., Dupin N., Unemo M., Tiplica G. S., Potočnik M., et al. (2014). European guideline on the management of syphilis. J. Eur. Acad. Dermatol. Venereol. 28 (12), 1581–1593. doi: 10.1111/jdv.12734
Kang S.-Y., Kim J., Ham J., Cho S.-H., Kang H.-R., Kim H. Y.. (2020). Altered T cell and monocyte subsets in prolonged immune reconstitution inflammatory syndrome related with DRESS (drug reaction with eosinophilia and systemic symptoms). Asia Pac. Allergy 10 (1), e2. doi: 10.5415/apallergy.2020.10.e2
Kapellos T. S., Bonaguro L., Gemund I., Reusch N., Saglam A., Hinkley E. R., et al. (2019). Human Monocyte Subsets and Phenotypes in Major Chronic Inflammatory Diseases. Front. Immunol. 10, 2035. doi: 10.3389/fimmu.2019.02035
Lin L. R., Gao Z. X., Lin Y., Zhu X.-Z., Liu W., Liu D., et al. (2018a). Akt, mTOR and NF-kappaB pathway activation in Treponema pallidum stimulates M1 macrophages. Int. Immunopharmacol. 59, 181–186. doi: 10.1016/j.intimp.2018.03.040
Lin L. R., Xiao Y., Liu W., Chen Y.-Y., Zhu X.-Z., Gao Z.-X, et al. (2018b). Development of tissue inflammation accompanied by NLRP3 inflammasome activation in rabbits infected with Treponema pallidum strain Nichols. BMC Infect. Dis. 18 (1), 101. doi: 10.1186/s12879-018-2993-0
Liu L. L., Liu W. N., Jiang X. Y., Jun-Ren, Chen M. H., Liu Z.-J., et al. (2019). Changes of T lymphocyte subsets in patients with HIV-negative symptomatic neurosyphilis. Microb. Pathog. 130, 213–218. doi: 10.1016/j.micpath.2019.03.008
Matsuzawa T., Kim B. H., Shenoy A. R., Kamitani S., Miyake M., Macmicking M. D. (2012). IFN-gamma Elicits Macrophage Autophagy via the p38 MAPK Signaling Pathway. J. Immunol. 189 (2), 813–818. doi: 10.4049/jimmunol.1102041
Moore M. W., Cruz A. R., LaVake C. J., Marzo A. L., Eggers C. H., Salazar J. C., et al. (2007). Phagocytosis of Borrelia burgdorferi and Treponema pallidum potentiates innate immune activation and induces gamma interferon production. Infect. Immun. 75 (4), 2046–2062. doi: 10.1128/IAI.01666-06
Nabatanzi R., Bayigga L., Cose S., Jones S. R., Joloba M., Canderan G., et al. (2019). Monocyte Dysfunction, Activation, and Inflammation After Long-Term Antiretroviral Therapy in an African Cohort. J. Infect. Dis 220 (9), 1414–1419. doi: 10.1093/infdis/jiz320
Parihar A., Eubank T. D., Doseff A. I. (2010). Monocytes and macrophages regulate immunity through dynamic networks of survival and cell death. J. Innate Immun. 2 (3), 204–215. doi: 10.1159/000296507
Peng R. R., Shang S. X., Zhao L. S., Long F. Q. (2019). MiR-216a-5p-containing exosomes suppress rTp17-induced inflammatory response by targeting TLR4. Biosci. Rep. 39 (8), undefined. doi: 10.1042/BSR20190686
Pun N. T., Subedi A., Kim M. J., Park P.-H. (2015). Globular Adiponectin Causes Tolerance to LPS-Induced TNF-α Expression via Autophagy Induction in RAW 264.7 Macrophages: Involvement of SIRT1/FoxO3A Axis. PLoS One. 10 (5), e0124636. doi: 10.1371/journal.pone.0124636
Rekart M. L., Ndifon W., Brunham R. C., Dushoff J., Park S. W., Rawat S., et al. (2017). A double-edged sword: does highly active antiretroviral therapy contribute to syphilis incidence by impairing immunity to Treponema pallidum? Sex. Transm. Infect. 93 (5), 374–378. doi: 10.1136/sextrans-2016-052870
Salazar J. C., Cruz A. R., Pope C. D., Valderrama L., Trujillo R., Saravia N. G., et al. (2007). Treponema pallidum elicits innate and adaptive cellular immune responses in skin and blood during secondary syphilis: a flow-cytometric analysis. J. Infect. Dis. 195, 879–887. doi: 10.1086/511822
Serbina N. V., Jia T., Hohl T. M., Pamer E. G. (2008). Monocyte-mediated defense against microbial pathogens. Annu. Rev. Immunol. 26, 421–452. doi: 10.1146/annurev.immunol.26.021607.090326
Szaflarska A., Baj-Krzyworzeka M., Siedlar M., Weglarczyk K., Ruggiero I., Hajto B., et al. (2004). Antitumor response of CD14(+)/CD16(+) monocyte subpopulation. Exp. Hematol. 32 (8), 748–755. doi: 10.1016/j.exphem.2004.05.027
Wang X. L., Xu Y. J., Zhang Y., Si Y., Jing L., Bao H. (2017). The effect of adiponectin on LPS-induced inflammation via autophagy in RAW 264.7 macrophages. Eur. J. Inflamm. 15 (3), 160–167. doi: 10.1177/1721727X17737208
Wang Y., Li Y. X., Li H. J., Song H., Zhai N., Lou L., et al. (2017). Brucella Dysregulates Monocytes and inhibits Macrophage Polarization through LC3-Dependent Autophagy. Front. Immunol. 8, 691. doi: 10.3389/fimmu.2017.00691
Workowski K. A., Bolan G. A. (2015). Sexually transmitted diseases treatment guidelines, 2015. Practice Guideline MMWR Recomm. Rep. 64 (Rr-03), 1–137.
Zang L. H., Wang J., Ren Y. L., Liu W., Yu Y., Zhao S., et al. (2019). Activated toll-like receptor 4 is involved in oridonin-induced phagocytosis via promotion of migration and autophagy-lysosome pathway in RAW264.7 macrophages. Int. Immunopharmacol. 66, 99–108. doi: 10.1016/j.intimp.2018.11.014
Zawada A. M., Schneider J. S., Michel A. I., Rogacev K. S., Hummel B., Krezdorn N., et al. (2016). DNA methylation profiling reveals differences in the 3 human monocyte subsets and identifies uremia to induce DNA methylation changes during differentiation. Epigenetics 11 (4), 259–272. doi: 10.1080/15592294.2016.1158363
Zeng H., Cohen S., Guy C., Shrestha S., Neale G., Brown S. A., et al. (2016). mTORC1 and mTORC2 Kinase Signaling and Glucose Metabolism Drive Follicular Helper T Cell Differentiation. Immunity 45 (3), 540–554. doi: 10.1016/j.immuni.2016.08.017
Zhao J., Ma J., Zhang X., Li Q., Yang X. (2016). Equilibrium of Treg/Th17 cells of peripheral blood in syphilitic patients with sero-resistance. Exp. Ther. Med. 11 (6), 2300–2304. doi: 10.3892/etm.2016.3178
Keywords: mTOR, IL-1β, migration, monocytes, Treponema pallidum
Citation: Liu W-N, Jiang X-Y, Xu Y-Z, Sun X-H, Wu K-X, Hu X-L, Lin Y, Lin L-R, Tong M-L and Liu L-L (2020) Treponemapallidum Dysregulates Monocytes and Promotes the Expression of IL-1β and Migration in Monocytes Through the mTOR Signaling Pathway. Front. Cell. Infect. Microbiol. 10:592864. doi: 10.3389/fcimb.2020.592864
Received: 08 August 2020; Accepted: 09 October 2020;
Published: 13 November 2020.
Edited by:
Hridayesh Prakash, Amity University, IndiaReviewed by:
Surya Prakash Pandey, University of Pittsburgh, United StatesWalace Gomes-Leal, Federal University of Pará, Brazil
Copyright © 2020 Liu, Jiang, Xu, Sun, Wu, Hu, Lin, Lin, Tong and Liu. This is an open-access article distributed under the terms of the Creative Commons Attribution License (CC BY). The use, distribution or reproduction in other forums is permitted, provided the original author(s) and the copyright owner(s) are credited and that the original publication in this journal is cited, in accordance with accepted academic practice. No use, distribution or reproduction is permitted which does not comply with these terms.
*Correspondence: Li-Li Liu, liulili@xmu.edu.cn
†These authors have contributed equally to this work