- 1Translational Health Science and Technology Institute, Faridabad, India
- 2Centre for Doctoral Studies, Advanced Research Centre, Manipal Academy of Higher Education, Manipal, India
Cholera is an acute secretory diarrhoeal disease caused by the bacterium Vibrio cholerae. The key determinants of cholera pathogenicity, cholera toxin (CT), and toxin co-regulated pilus (TCP) are part of the genome of two horizontally acquired Mobile Genetic Elements (MGEs), CTXΦ, and Vibrio pathogenicity island 1 (VPI-1), respectively. Besides, V. cholerae genome harbors several others MGEs that provide antimicrobial resistance, metabolic functions, and other fitness traits. VPI-1, one of the most well characterized genomic island (GI), deserved a special attention, because (i) it encodes many of the virulence factors that facilitate development of cholera (ii) it is essential for the acquisition of CTXΦ and production of CT, and (iii) it is crucial for colonization of V. cholerae in the host intestine. Nevertheless, VPI-1 is ubiquitously present in all the epidemic V. cholerae strains. Therefore, to understand the role of MGEs in the evolution of cholera pathogen from a natural aquatic habitat, it is important to understand the VPI-1 encoded functions, their acquisition and possible mode of dissemination. In this review, we have therefore discussed our present understanding of the different functions of VPI-1 those are associated with virulence, important for toxin production and essential for the disease development.
Introduction
Cholera is an acute gastrointestinal diarrheal disease that is caused by a bacterium, Vibrio cholerae (Kaper et al., 1995). The complete genome sequences of clinical and environmental strains of V. cholerae revealed that their genome consists of two circular non-homologous chromosomes that carry nearly 3,900 open reading frames (ORFs). Both the chromosomes of V. cholerae consist of core and acquired genomes. The acquired genome of V. cholerae harbor several mobile genetic elements (MGEs) and linked with DNA mobility genes and other metabolic functions (Mutreja et al., 2011). Almost all the 7th pandemic V. cholerae strains harbor four pathogenicity islands namely (i) Vibrio pathogenicity island-1 (VPI-1), ~41.3 kb in size (ii) Vibrio pathogenicity island-2 (VPI-2), ~57 kb in size, (iii) Vibrio seventh pandemic island-I (VSP-1), ~16 kb in size, and (iv) Vibrio seventh pandemic island-2 (VSP-2), ~26.9 kb in size (Heidelberg et al., 2000). Virulence functions of cholera pathogens are not endogenous, but they are part of the acquired MGEs. V. cholerae strains devoid of CTXΦ or VPI-1, two important MGEs ubiquitously present in the toxigenic strains, are non-toxigenic and can't develop cholera in animal model and human volunteer (Pang et al., 2007). Like other bacterial species MGEs present in the genome of V. cholerae have several typical characteristics of horizontally acquired elements such as (i) sporadic distribution (ii) encode DNA recombinases (iii) located in tRNA/ssrA or dif loci (iv) direct repeat sequences at the borders (v) distinct GC content and (vi) unstable.
In this review, we discuss dynamics of VPI-1; different functions encoded by the VPI-1 linked with its mobility and modulate the virulence cascades. Special focus is given to understand how VPI-1 contributed in the emergence of toxigenic pandemic strains.
The Pathogen (V. cholerae) and Integrative Mobile Genetic Elements (MGEs)
Over the period, V. cholera has evolved as one of the most successful pathogen in the history of mankind. To attain the fitness for survival, the pathogen has acquired a number of MGEs belongs to different classes such as prophages (CTXΦ, VGJΦ, RS1, TLCΦ), pathogenicity islands (VPI-1, VPI-2, VSP-1 & VSP-2) and integrative conjugative elements (ICEs) (Table 1). The key virulence factor of cholera, cholera toxin (CT) is encoded by the ctxA and ctxB genes that induces the secretion of fluid and electrolytes from the intestinal epithelial cells and causes the diarrhea. CT is acquired through irreversible integration of a single stranded DNA (+ssDNA) phage CTXΦ into the dif sites of either or both the chromosome of V. cholerae (Val et al., 2005). The second most crucial virulence factor of cholera pathogen, toxin-coregulated pilus (TCP), encoded by the genes present in the TCP locus of VPI-1, helps the pathogen in colonization in the gastrointestinal tract of the host and also act as a cell surface receptor for CTXΦ (Manning, 1997). This altogether suggests that the acquisition of the MGEs is the key for the fitness and evolution of the cholera pathogen for different pandemics. Therefore, understanding the role of MGEs acquired by pathogen over time is critical to develop strategies for managing the patient and the disease.
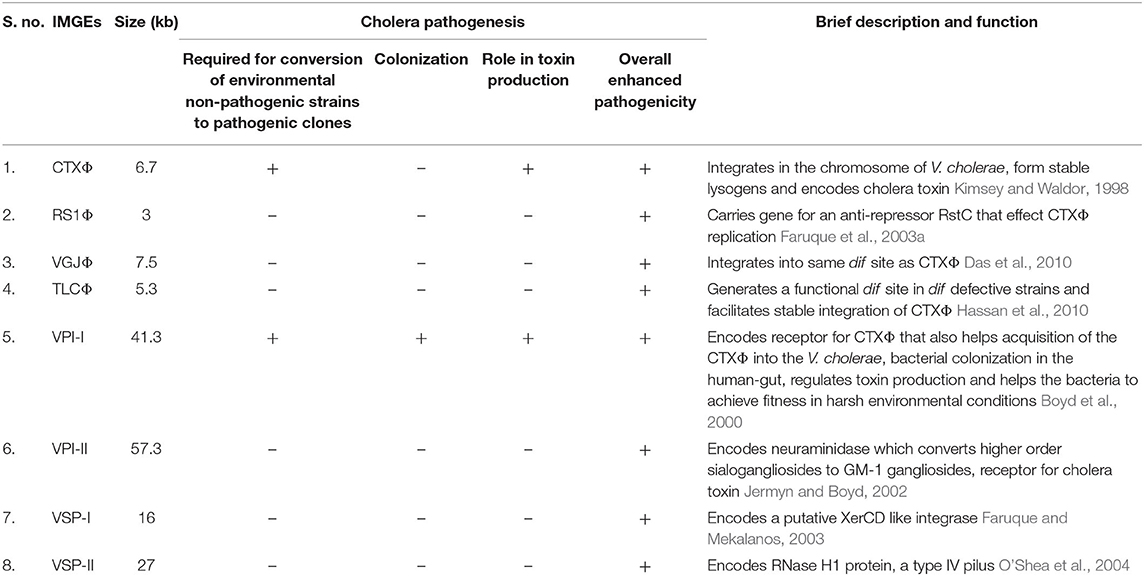
Table 1. Significance of integrative mobile genetic elements (IMGEs) in the V. cholerae pathogenesis and fitness.
Cholera and its Indispensible Association With VPI-1
The first cholera pandemic was recorded in 1817 and seven pandemics have been recorded till to date (Figure 1) (Hu et al., 2016). The ongoing 7th pandemic was first evolved on the island of Sulawesi in Indonesia in 1961 (Karaolis et al., 1995). All of the seven cholera pandemics are caused by the O1 serotype of V. cholerae, except the spatial emergences of O139 Bengal in eastern part of India and Bangladesh in 1992 (Johnson et al., 1994). Interestingly, all of these pathogenic strains harbored VPI-1 as well as CTXΦ in their chromosomes (Li et al., 2003). Since VPI-1 encoded TcpA acts as a receptor for CTXΦ, sequential acquisition of VPI-1 and CTXΦ probably convert the environmental V. cholerae strains into toxigenic strains. Although no such experimental evidences or validation or natural phenomenon has been reported yet (Singh et al., 2001). But this is most accepted hypothesis across the scientific community that environmental V. cholerae strain may become pathogenic if it acquires VPI-1 and CTXΦ. Besides, loss of VPI-1 could revert a pathogenic strain into a non-pathogenic strain. Other than VPI-1 and CTXΦ, V. cholerae O1 El Tor biotype strains have acquired RS1 element (Choi et al., 2010) and two other pathogenicity-associated islands (VSP-1 & VSP-2). However, it is believed that RS1, VSP-1 & VSP-2 are not the pre-requisite for V. cholerae pathogenesis but involved in the fitness and robustness of 7th pandemic V. cholerae strains over the classical strain of O1 serotype.
Vibrio Pathogenicity Island-1 (VPI-1)
The Vibrio pathogenicity island-1 (VPI-1) is a ~41.3 kb long DNA fragment present in all the epidemic strains including sixth-pandemic classical biotype and seventh-pandemic El Tor biotype strains. Previously, VPI-1 was experimentally demonstrated to be a filamentous bacteriophage (Karaolis et al., 1999). The authors reported that the cell-free preparations can transmit VPI-1 between V. cholerae strains by transduction. However, the protection of VPI-1 genomic DNA in a phage preparation from DNase and RNase treatment insisted the authors to conclude that the VPI-1 genes in the phage preparations are possibly wrapped with the protein coat TcpA. The authors further reported that replicative dsDNA genome of VPI-1 were detectable in toxigenic V. cholerae by DNA hybridization. However, no other laboratories were able to reproduce these findings (Faruque and Mekalanos, 2003). Nevertheless, the same group later on reported that VPI-1 is a pathogenicity island (Rajanna et al., 2003).
In the whole genome sequenced reference V. cholerae strain N16961, VPI-1 harbors 31 genes (VC0817 to VC0847) with known and unknown functions (Rajanna et al., 2003) (Table 2, Figure 2A). Like other genomic islands, VPI-1 has following typical characteristics:
(i) Sporadic distribution among environmental isolates
(ii) Distinct GC content (35% of the total in VPI-1 alone)
(iii) Flanked by direct repeat sequences (30–31 bp); one at left (attL) and another at right (attR) borders
(iv) Located downstream of a tmRNA locus
(v) Encodes two DNA mobility enzymes; one transposase [VpiT encoded by vpiT (Gene Map ID: VC0817)] and one integrase [Intvpi encoded by intV (Gene Map ID: VC0847)]
(vi) Harbors a number of virulence associated and accessory colonization factors.
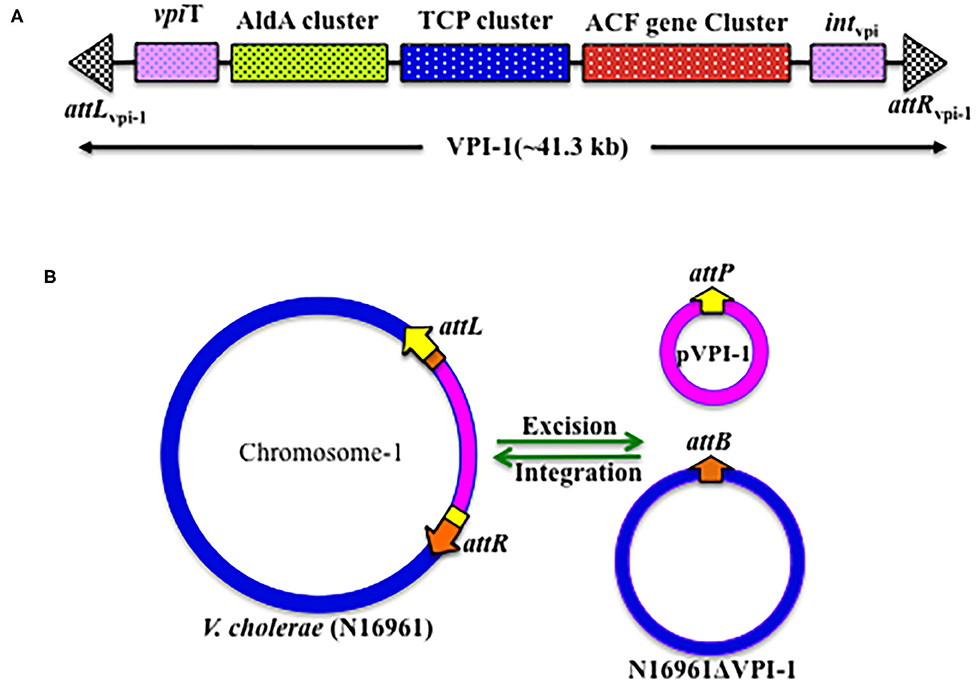
Figure 2. Genetic organization of VPI-1. (A) Schematic representation of the VPI-1 genetic element, (B) Schematic representation of the integration and excision mechanisms of VPI-1 genetic element.
The role of VPI-1 in the pathogenicity of cholera was identified while investigating the differences between pathogenic and non-pathogenic V. cholerae strains (Karaolis et al., 1998). Several proteins (ToxT, TcpA, TcpP, TcpH, ACFs gene cluster) encoded by the VPI-1 are crucial for V. cholerae pathogenesis and justified the name of the genomic island as Vibrio pathogenicity islands.
Distribution of VPI-1 in V. cholerae Isolates
Genomic analyses of thousands of clinical V. cholerae isolates revealed that VPI-1 is widely conserved in the genome of all the epidemic and pandemic cholera pathogen (Mutreja et al., 2011; Domman et al., 2017; Weill et al., 2019). However, VPI-1 distribution is sporadic among nonO1-nonO139 environmental V. cholerae strain (Chun et al., 2009). Whole genome sequence analysis of environmental strains revealed that VPI-1 is absent in several isolates belonging to serogroup O37 (MZO-3), O39 (AM-19226), O12 (1587), O14 (MZO-2), O141 (V51), and O135 (RC 385). Interestingly, VPI-1 was detected in the nonO1-nonO139 environmental V. cholerae strains belonging to serogroup O141 (V51). The same group has also reported absence of VPI-1 in the genome of environmental O1 El Tor strains 12129 and TM11079-80 (Chun et al., 2009). As expected, the genome of all the VPI-1 negative V. cholerae strains are also negative for CTX-prophage. This finding indicates that VPI-1 is absolutely important for the conversion of a non-toxigenic strain to a toxigenic variant. In addition, VPI-1 encoded functions are also essential for colonization of cholera pathogen in the host intestine. Since, colonization and toxin are the sole components for development of cholera, VPI-1 is an indispensible component for the emergence and evolution of epidemic and pandemic V. cholerae.
Mobility of VPI-1: Acquisition and Dissemination
VPI-1 has been reported to precisely excise from the V. cholerae chromosome and form an extra-chromosomal circular product (Rajanna et al., 2003) (Figure 2B). However, this study could not provide information about the loss frequency of VPI-1 from the genome of V. cholerae. To address this issue, Kumar et al. (2018) have engineered the genome of V. cholerae and developed a reporter strain to monitor the loss frequency of VPI-1 and isolated VPI-1 devoid clone from a mixed population of V. cholerae. Authors used an antibiotic resistance gene (cat) as selectable marker and a sucrose sensitive confirming counter selectable gene (sacB) to tagged the VPI-1 element. The engineered strain was used to measure loss frequency of VPI-1 in in vitro (test tube) and in vivo (rabbit ileal loop model) growth conditions. V. cholerae cell containing functional sacB in the genome is unable to grown in room temperature (~24°C) in the presence of excess sucrose (10–15%) sucrose in growth medium. Using the said vector, excision frequency of VPI-1 was investigated in the in vitro. VPI-1 was found highly stable in the animal model (excision frequency, ~10−9) compared to the in vitro laboratory growth condition (excision frequency, ~10−4) (Kumar et al., 2018).
The VPI-1 doesn't encode any known conjugative function. The pathogenicity island also has no oriT sequence. Thus, horizontal transmission of VPI-1 between different V. cholerae strains couldn't be through conjugation. Since V. cholerae is naturally competent (Meibom et al., 2005), the bacterium could uptake naked genomic DNA including VPI-1 through transformation. We believe that different V. cholerae serogroups living in the biofilms during aquatic and inflectional phases of its life cycle acquired MGEs through natural transformation. However, currently substantial experimental evidences are lacking to support this hypothesis. In another study, VPI-1 has also been shown to get transferred from V. cholerae O1 strain C6709 to VPI-negative V. cholerae isolates (468-83, GP6, V69, and 1528-79) by a generalized transducing phage CP-T1.This study proposed an alternative mode of dissemination of VPI-1 between V. cholerae strains (O'Shea and Boyd, 2002). Once inside the cell, both the tyrosine recombinases encoded by the VPI-1 helps the GI to integrate at the prfC locus by site-specific recombination. The reaction is reversible; hence the attL and attR sites generated due to integration of VPI-1 come together, possibly with the help of recombination directionality factors (RDFs), and excised from the chromosome.
Although VPI-1 has already been shown indispensable for V. cholerae pathogenicity, the molecular mechanisms and factors that modulate integration and excision of VPI-1 into chromosomes are still largely unknown.
VPI-1 and Cholera Pathogenesis
Although CT is the crucial factor for developing cholera, but it is not the only virulence factor for developing the disease. There are several other virulence-associated factors (ToxT, TCP, AphAB, ToxRS) that also contribute substantially in cholera pathogenesis. Production of CT, and intestinal colonization of V. cholerae in the host intestine are pivotal for disease development (Krukonis et al., 2000). VPI-1 plays critical role in both the processes by encoding CT expression transcriptional factor ToxT and intestinal colonization factor TCP. Nevertheless, VPI-1 also plays crucial role in the acquisition of CT encoding gene through horizontal gene transfer. The detailed role of VPI-1 in cholera pathogenesis is as given below and depicted in Figure 3.
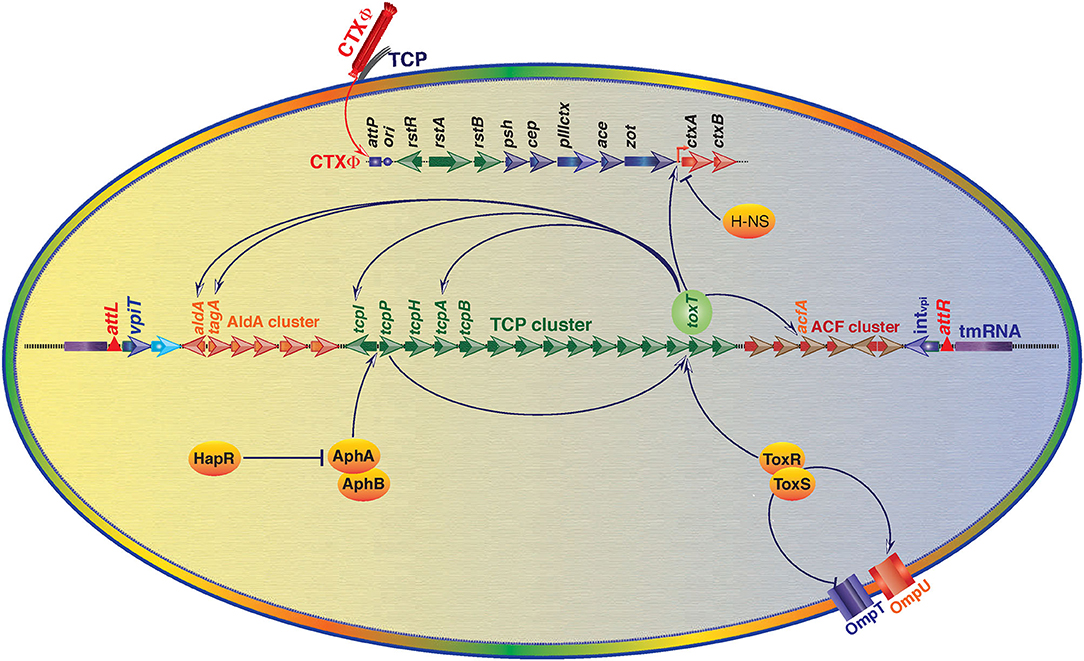
Figure 3. An overview of regulation of V. cholerae virulence gene expression through Vibrio Pathogenicity Island 1 (VPI-1) and core genome encoded functions. Transcriptional factor ToxT is the key positive regulator of cholera toxin (CT) encoding genes ctxA and ctxB. ToxT also positively modulates expression of toxin co-regulated pilus (TCP) genes and several other genes located in the VPI-1. ToxR-ToxS and AphA-AphB proteins positively regulate expression of toxT. ToxR-ToxS proteins also modulate expression of outer membrane proteins OmpU and OmpT. Histone-like nucleoid-structuring (H-NS) protein negatively regulates expression of CT. Transcriptional regulator HapR negatively regulates expression of AphA and AphB encoding gene expression. Positive regulation is depicted by arrowhead and T head depicts negative regulation.
Acquisition of CTXΦ in V. cholerae
Acquisition of CTXΦ is the first and critical step for the conversion of a non-toxigenic V. cholerae strain into toxigenic clone. The VPI-1 encoded TCP is a homopolymer of multiple subunits of major pillin protein, TcpA. After its production, TcpA trans-located to the cell surface and serve as the receptor for the CTXΦ (Lim et al., 2010). Once CTXΦ recognizes TcpA pili on the surface, it injects it's (+)ssDNA genome in to the host cytoplasm of the host cell(s) (Karaolis et al., 1999). The (+)ssDNA genome of CTXΦ either converted into replicative double stranded pCTXΦ or exploits the two tyrosine recombinases, XerC and XerD, for irreversible integration into chromosomal DNA (Boyd, 2010). XerC and XerD recombinases are conserved among the bacteria chromosomes and their native function is to resolve the chromosome dimers. XerC and XerD recognize 28 bp dif sequences as a substrate to resolve the chromosomal dimers before cell division (Das et al., 2013). The CTXΦ has two dif like attachment sequences, attP1 and attP2, on its pCTXΦ genome. In (+) ssDNA genome of CTXΦ both the attachment sites are separated by 90 bp DNA sequences. The single stranded genome of CTXΦ forms intra-strand base pairing and developed a double-forked hairpin like structure. The stem of the hairpin harbors a functional attachment site (+)attP resembles with the dif1 and dif2 site of V. cholerae chromosomes (McLeod and Waldor, 2004). Due to similar DNA sequences between dif and (+)attP, the XerC and XerD recognizes the (+)attP as their binding substrate and mediate CTXΦ integration into the dif site by site specific recombination. Once the CTXΦ gets integrated into the chromosome, the host DNA replication machinery converts (+) ssDNA CTXΦ genome into double stranded. Once integrated, the dsDNA of prophage genome is unable to excise due to lack of two functional dif like sequences. This irreversible integration of CTXΦ into the chromosomal DNA of V. cholerae is one of the important events in the V. cholerae evolution as a pathogen. Tandemly integrated CTX-prophage can initiate rolling replication for production of new virion and disseminate to other V. cholerae cells. In addition to TcpA, several other VPI-1 functions including TcpB and TcpE helps acquisition and dissemination of CTXΦ (Gutierrez-Rodarte et al., 2019).
Intestinal Colonization of V. cholerae
V. cholerae is the natural inhabitant of estuaries where it can survive as free-living cells or as biofilms. The stomach is not a suitable environment for V. cholerae to survive and multiply since, the bacterium is highly sensitive to low pH (Almagro-Moreno et al., 2015). However, on ingestion with contaminated food/water, V. cholerae strains have the ability to pass through such adverse environments and colonized in the small intestine of human gut and produce sufficient amount of cholera toxin to develop the clinical symptoms.
Historically chemotactic movement was proposed to be responsible for colonization of V. cholerae (Castro-Rosas and Escartin, 2002), however various reports later suggested that intestinal colonization of V. cholerae is the complex outcome of interplay of VPI-1, chromosomally encoded proteins and host factors that are involved in motility, chemo-taxsis, and penetration. During the initial stages of colonization that is at the proximal ends of small intestine, bacterium flagellum, and a few general (neuraminidase etc.) and V. cholerae-specific proteolytic enzymes (haemagglutinin/protease, Hap) helps in the movement and penetration of thick mucosal layer (Zhu and Mekalanos, 2003). TagA, a VPI-1 encoded metalloprotease, is known to modify the mucin glycoproteins that are attached to the host cell surface (Szabady et al., 2011). TagA is specifically expressed and secreted by the pathogen under virulence-inducing conditions. TagA has been reported to be positively coregulated along with TCP and other virulence genes and hence may potentially play critical role in colonization of the pathogen by facilitating the movement through the intestinal mucosa (Szabady et al., 2011). Along with a few pathogen-derived adhesin molecules (i.e., flagellin, Mam7, GbpA, OmpU, and FrhA etc.), TCP is also reported to help the pathogen to adhere to the epithelium and facilitate bacteria-bacteria interaction to form micro-colonies (Tam et al., 2007). The aberrant production or function of the TCP has been shown to result in reduced colonization in both humans and mice. In addition, other four VPI-1 encoded proteins called as accessory colonization factors encoded by acfA, acfB, acfC, acfD also play critical role in colonization (Everiss et al., 1994; Withey and DiRita, 2005; Valiente et al., 2018). Disruption of any of the four genes has been reported to cause ~10-fold decreases in colonization as compared to wild type.
Clinical Appearance of the Disease
Once the V. cholerae colonized inside the gut it starts producing CT and other virulence factors. CT is A1B5 multimeric protein complex, where B-subunit binds to GM1 ganglioside receptor. The receptor-toxin complex is endocytosed to endoplasmic reticulum and A1-subunit of toxin activates adenylate cyclase that ultimately opens chloride ions channels (CFTR Cystic fibrosis trans-membrane conductance regulator) and causes the secretion of fluids and ions into the lumen of gut (Vanden Broeck et al., 2007). Clinical onset of cholera may be sudden or delayed, depending upon the inoculation size. But in general after the incubation period in range of 18 h to 5 days, clinical symptoms of diseases appeared that included secretion of voluminous stools, resembling like rice-water (Due to presence of mucus in the stool), abdominal discomfort, anorexia with or without vomiting (Sack et al., 2004). Although ~2–11% of the infected person with the El Tor and Classical strain of V. cholerae, respectively showed the severe clinical manifestation (Kaper et al., 1995). In the case of severe cholera, the rate of diarrhea reaches up to 500–1,000 ml/h, which leads to decrease in the turner pressure, low blood pressure, sunken eyes and wrinkled hand, and feet skin. Although cholera patients can be easily treated with simple electrolytes replacement therapy but severe patients of cholera may die within few hours if left untreated (Bhattacharya, 2003).
VPI-1 encoded transcriptional activator ToxT activates the transcription of CT; ToxT is a 32-kDa AraC family transcriptional activator of ctxA/ctxB as well as tcpA, acfs, aldA. It has two helix-turn-helix-motifs, an N-terminal dimerization and environmental-sensing domain, along with C-terminal DNA binding domain (Lowden et al., 2010). Once induced, the ToxT directly bind via direct repeats of the sequence TTTTGAT called as Tox boxes to the promoter of ctxA, ctxB, tcpA, acfs, aldA. However, configuration of tox boxes differs at different promoters. For example, binding to the promoter of ctxA/B, ToxT required minimum three direct repeats of Tox boxes (Prouty et al., 2005). For tcpA promoter, ToxT binds to two tox boxes arranged as a direct repeat between position −44 and −67. Whereas, for acfA and acfD promoters, ToxT binds to two tox boxes organized as an inverted repeat (Krukonis et al., 2000). In contrast to tcpA, acfA, and acfD, ToxT binds to a single tox box for aldA promoter. ToxT is located downstream of tcpF and auto-regulates its own transcription. A histone-like nucleoid structural protein (H-NS) that binds to DNA in sequence-independent manner at AT-rich sequences competes with ToxT binding promoters and causes the repression of virulence protein (Ayala et al., 2017). Probably this mechanism might help in the shutdown of virulence protein expression under non-permissive conditions. Same finding was supported with mutational studies; h-ns mutants are reported to have de-repressed expression of toxT, ctxA/B, and tcpA (Nye and Taylor, 2003). As compared to tcpA promoter, H-NS strongly binds to ctxAB promoter and subsequently strongly represses the transcription of ctxAB (Nye et al., 2000).
Interaction Between VPI-1 and Chromosomally Encoded Function and Its Influence on V. cholerae Pathogenesis
The functioning of V. cholerae as a pathogen is strongly regulated by the function encoded by its core genome, genomic islands and intestinal environmental conditions in the host associated phage. In this review, we also included role of human intestinal environment in the V. cholerae pathogenesis. The human gut environment consists of a number of poorly characterized chemical components harboring thousands of host derived signaling molecules. In addition, microbiota residing in the intestine contribute in further complexity by producing and secreting several small molecules in the milieu. Auto-inducers that triggers the expression of number of virulence factors in V. cholerae are one of the important bacterial component that modulate disease severity and dissemination of bacteria from host to the environment (Higgins et al., 2007). A number of host and microbial origin molecule have been discovered to-date and majority of which have integrated molecular circuit with VPI-1 encoded transcriptional regulators that play role at various stages of cholera pathogenesis.
Core Genome Encoded HapR Repressed VPI-1 Encoded ToxT
The aphA/hapR mediated quorum sensing is the central mechanism that regulates the virulence-associated cascade in toxigenic V. cholerae. The low cell density of the pathogen in the gut is typically sensed by a membrane bound sensor kinase (the CqsS), based on the concentration of a cholera auto-inducer 1 (CAI-1). CAI-1 triggers the expression of aphA, which induces the expression of tcpP and tcpH (Haycocks et al., 2019; Herzog et al., 2019). This quorum sensing of V. cholerae modulates the virulence gene expression cascades for development of the disease and its severity. Besides at low cell density, CqsS activates LuxO (a sigma-54 dependent protein) that represses the expression of hapR which favors the bacterial growth and pathogenesis (Zhu et al., 2002). Whereas, at high cell density, LuxO is unable to activate the repressor protein of HapR, and hence it remains available to aphA promoter between −85 and −58 and repress its expression, which leads to deficiency of TcpP and TcpH. Ultimately because of this, ToxR/ToxS regulon becomes unable to activate the toxT; the master regulator of VPI-1 encoded factors and cholera toxin production (Kovacikova and Skorupski, 2002). Interestingly in V. cholerae strain N16961, hapR is not active due to a frame-shift mutation (Joelsson et al., 2006). Since, N16961 is a clinical isolate and it can develop typical disease phenotype in animal model indicating HapR is dispensable for disease development. For a clear understanding of the HapR mediated regulation, the interactions of HapR with the transcriptional factors AphAB has been depicted in the Figure 3. Some additional modulators like ToxT, H-NS, ToxR/S involved in the regulation of toxin production, colonization and disease development can compensate the absence of HapR in N16961.
ToxR and TcpP Mediated Activation of ToxT
ToxR is a 32.5-kDa inner trans-membrane transcriptional activator consisting of 294 amino acids. It has three functional domains: a 180 amino acids long cytoplasmic domain, 16 amino acids long trans-membrane domain, and 100 amino acids long peri-plasmic domain (Miller et al., 1987). ToxR helps V. cholerae in sensing external intestinal environmental conditions (like pH, bile, temperature) and facilitates bacterial adaptation (Childers and Klose, 2007). ToxR modulates the expression of CT, OmpU, OmpT, and ToxT by binding to their promoters and modulating their transcription (Provenzano and Klose, 2000). However, experimental data indicates that ToxR alone is unable to activate the ToxT expression and need another membrane bound protein TcpP. Possibly, ToxR act as an enhancer for TcpP at the toxT promoter. ToxR also interact with another co-transcribed protein, ToxS (Bina et al., 2003), which is required for the maximal transcriptional activation of ToxT regulated genes. Like ToxR, TcpP interacts with another protein, TcpH that is required for TcpP stability. Experimental data showed that when V. cholerae cells shifted from permissive to non-permissive growth conditions, TcpP gets degraded even in presence of TcpH suggesting the role of TcpP at the initial stage of virulence cascade as a switch of ToxT dependent virulence associated genes in permissive and non-permissive growth conditions (Raskin et al., 2020). Once the bacteria sense the gut environment through its quorum sensing, ToxR and TcpP binds to toxT promoter at −100 to −69 and at −51 to −32 region with respect to transcription start site, respectively and subsequently activates the expression toxT, the master regulator of V. cholerae pathogenesis and hence the disease.
VarS/VarA Mediated Activation of Virulence-Associated Genes
It is reported that VarS/VarA, two-component system acting downstream of ToxR/S signaling cascade, also independently controls the expression of ToxT and other virulence proteins in response to environmental signals. V. cholerae O395 varA mutant are reported to have reduced expression of tcpA and ctxA and ctxB (Tsou et al., 2011). VarS mutant of classical O395 and El Tor C6706 strains exhibit the decreased production of TcpA (134-fold), CT (2.5-fold), ctxA (1.75-fold) and ctxB (1.72-fold) as compared to wild types (Jang et al., 2011). Recently, downstream target of VarS/VarA system, PckA which is a key player in central carbon metabolism and affects the levels of many metabolic intermediates, has been shown to modulate HapR activity (Jang et al., 2010). Although more evidences are required, this supported to hypothesize that VarS/VarA system to be a way for V. cholerae to combine information about surrounding cell density and nutrient availability to fine-tune its gene expression profile precisely to the surrounding microenvironmental conditions.
Genetic Variation in VPI-1, Among Various Clinical Isolates of V. cholerae
Classical strains of V. cholerae represent the remnant of the sixth-pandemics toxigenic clones, while El Tor and O139 Bengal represent the current seventh-pandemic toxigenic clones (Karaolis et al., 1995). Comparative studies of VPI-1 of sixth and seventh pandemics strains found that the GI integrate in the same locus of chromosome 1 of both the biotypes of O1 isolates. VPI-1 is very similar in both the biotypes and has similar numbers of ORFs. However, ~483 polymorphic nucleotides were reported between both the biotypes. The central region of VPI-1 that harbors gene for TcpI, TcpP, TcpH, and TcpA was found to have interestingly highest level of polymorphic nucleotides (Karaolis et al., 2001). Highest variation was observed for tcpA, gene encoding the type IV pilus that works as a receptor for CTXΦ, around 22.5% variation at nucleotide level and 16.9% at the protein level. The accessory colonization factor, AcfD that help in the intestinal colonization contained longer open reading frame in El Tor strain (Table 3). Despite the huge importance of VPI-1 encoded genes in the pathogenesis of V. cholerae, yet a detail comparative study of their contents including the characterization of genetic, proteomic, and phenotypic variations among the various pathogenic clones of V. cholerae is not available. Therefore, in order to understand the reason behind the disappearance of O1 classical strains and emergences of O1 El Tor strain and the temporal emergences of O139 Bengal in 1992, further investigations are of much demand.
Conclusion
Cholera, an acute gastrointestinal diarrheal disease caused by the V. cholerae, is still a major public health concern to many developing countries including India. Improved understanding of the cholera pathogenesis at molecular level is a pre-requisite for the development of appropriate strategies for disease management.
Acquisition of MGEs through HGT is among the most common approaches of the pathogens to achieve fitness and survival traits in hostile and/or changing environments. Two important virulence factors for cholera pathogenesis, CT and TCP, are part of two MGEs, CTXΦ and VPI-1, respectively. This indicates that understanding the MGEs biology, their integration mechanisms, stable inheritance and dissemination between bacterial species, may help in reducing the disease burden and development of novel therapeutics for treating the disease. To date, V. cholerae have acquired a number of MGEs (CTXΦ, VPI-1, VPI-2, RS1, VSP-1, & VSP-2) that help the pathogen survival under the changing environmental conditions and contribute in causing the pathogenesis. The VPI-1 is unique, as it has been involved in regulation of almost all the stages of cholera pathogenesis. First, the VPI-1 encoded TCP helps the CTXΦ to recognize the host bacterium and introduce their (+) ssDNA inside the host cell. The V. cholerae strains that lack CTXΦ are typically non-pathogenic. Then, VPI-1 encoded factors, including TCP, TagA, AcfA, AcfB, AcfC, and AcfD, facilitate the pathogenic bacterium to colonize in the human gut. The VPI-1 encoded ToxT induces the expression of CT, the most critical virulence factor of cholera pathogenesis and helps the pathogen to cause the disease. TCP, Tpx, and ToxT are also integral part of quorum sensing mechanisms that protects the pathogen from harsh micro-environmental conditions in the gut. Deregulation of VPI-1 or its encoded factor(s) have already been reported to negatively impact the ability of the pathogen to cause the disease. This altogether supports the role of VPI-1 as the master regulator of cholera pathogenesis and hence suggesting it as potential therapeutic target for disease control and/or management.
Author Contributions
AK provided the general concept. AK and NK drafted the initial concept of manuscript. AK, NK, and BD wrote the manuscript. All the authors have seen and approved the final manuscript.
Funding
The work has been supported by funding from Department of Biotechnology, India and Indian Council of Medical Research.
Conflict of Interest
The authors declare that the research was conducted in the absence of any commercial or financial relationships that could be construed as a potential conflict of interest.
Acknowledgments
We are grateful to Professor Gagandeep Kang (Translational Health Science and Technology Institute Faridabad), for her support and Indian council of Medical Research for providing the Junior/Senior Research Fellowship to pursue AK for perusal of Doctoral degree.
References
Almagro-Moreno, S., Napolitano, M. G., and Boyd, E. F. (2010). Excision dynamics of Vibrio pathogenicity island-2 from Vibrio cholerae: role of a recombination directionality factor VefA. BMC Microbiol. 10:306. doi: 10.1186/1471-2180-10-306
Almagro-Moreno, S., Pruss, K., and Taylor, R. K. (2015). Intestinal colonization dynamics of Vibrio cholerae. PLoS Pathog. 11:e1004787. doi: 10.1371/journal.ppat.1004787
Ayala, J. C., Silva, A. J., and Benitez, J. A. (2017). H-NS: an overarching regulator of the Vibrio cholerae life cycle. Res. Microbiol. 168, 16–25. doi: 10.1016/j.resmic.2016.07.007
Bhattacharya, S. K. (2003). An evaluation of current cholera treatment. Expert Opin. Pharmacother. 4, 141–146. doi: 10.1517/14656566.4.2.141
Bina, J., Zhu, J., Dziejman, M., Faruque, S., Calderwood, S., and Mekalanos, J. (2003). ToxR regulon of Vibrio cholerae and its expression in vibrios shed by cholera patients. Proc. Natl. Acad. Sci. U.S.A. 100, 2801–2806. doi: 10.1073/pnas.2628026100
Bose, N., and Taylor, R. K. (2005). Identification of a TcpC-TcpQ outer membrane complex involved in the biogenesis of the toxin-coregulated pilus of Vibrio cholerae. J. Bacteriol. 187, 2225–2232. doi: 10.1128/JB.187.7.2225-2232.2005
Boyd, E. F. (2010). Efficiency and specificity of CTXphi chromosomal integration: dif makes all the difference. Proc. Natl. Acad. Sci. U.S.A. 107, 3951–3952. doi: 10.1073/pnas.1000310107
Boyd, E. F., Moyer, K. E., Shi, L., and Waldor, M. K. (2000). Infectious CTXPhi and the vibrio pathogenicity island prophage in Vibrio mimicus: evidence for recent horizontal transfer between V. mimicus and V. cholerae. Infect. Immun. 68, 1507–1513. doi: 10.1128/IAI.68.3.1507-1513.2000
Carroll, P. A., Tashima, K. T., Rogers, M. B., DiRita, V. J., and Calderwood, S. B. (1997). Phase variation in tcpH modulates expression of the ToxR regulon in Vibrio cholerae. Mol. Microbiol. 25, 1099–1111. doi: 10.1046/j.1365-2958.1997.5371901.x
Castro-Rosas, J., and Escartin, E. F. (2002). Adhesion and colonization of Vibrio cholerae O1 on shrimp and crab carapaces. J. Food Prot. 65, 492–498. doi: 10.4315/0362-028X-65.3.492
Cha, M. K., Hong, S. K., Lee, D. S., and Kim, I. H. (2004). Vibrio cholerae thiol peroxidase-glutaredoxin fusion is a 2-Cys TSA/AhpC subfamily acting as a lipid hydroperoxide reductase. J. Biol. Chem. 279, 11035–11041. doi: 10.1074/jbc.M312657200
Chang, Y. W., Kjaer, A., Ortega, D. R., Kovacikova, G., Sutherland, J. A., Rettberg, L. A., et al. (2017). Architecture of the Vibrio cholerae toxin-coregulated pilus machine revealed by electron cryotomography. Nat. Microbiol. 2:16269. doi: 10.1038/nmicrobiol.2016.269
Childers, B. M., and Klose, K. E. (2007). Regulation of virulence in Vibrio cholerae: the ToxR regulon. Future Microbiol. 2, 335–344. doi: 10.2217/17460913.2.3.335
Choi, S. Y., Lee, J. H., Kim, E. J., Lee, H. R., Jeon, Y. S., von Seidlein, L., et al. (2010). Classical RS1 and environmental RS1 elements in Vibrio cholerae O1 El Tor strains harbouring a tandem repeat of CTX prophage: revisiting Mozambique in 2005. J. Med. Microbiol. 59(Pt 3), 302–308. doi: 10.1099/jmm.0.017053-0
Chun, J., Grim, C. J., Hasan, N. A., Lee, J. H., Choi, S. Y., Haley, B. J., et al. (2009). Comparative genomics reveals mechanism for short-term and long-term clonal transitions in pandemic Vibrio cholerae. Proc. Natl. Acad. Sci. U.S.A. 106, 15442–15447. doi: 10.1073/pnas.0907787106
Das, B., Bischerour, J., Val, M. E., and Barre, F. X. (2010). Molecular keys of the tropism of integration of the cholera toxin phage. Proc. Natl. Acad. Sci. U.S.A. 107, 4377–4382. doi: 10.1073/pnas.0910212107
Das, B., Martinez, E., Midonet, C., and Barre, F. X. (2013). Integrative mobile elements exploiting Xer recombination. Trends Microbiol. 21, 23–30. doi: 10.1016/j.tim.2012.10.003
Davies, B. W., Bogard, R. W., Young, T. S., and Mekalanos, J. J. (2012). Coordinated regulation of accessory genetic elements produces cyclic di-nucleotides for V. cholerae virulence. Cell 149, 358–370. doi: 10.1016/j.cell.2012.01.053
Domman, D., Quilici, M. L., Dorman, M. J., Njamkepo, E., Mutreja, A., Mather, A. E., et al. (2017). Integrated view of Vibrio cholerae in the Americas. Science 358, 789–793. doi: 10.1126/science.aao2136
Everiss, K. D., Hughes, K. J., Kovach, M. E., and Peterson, K. M. (1994). The Vibrio cholerae acfB colonization determinant encodes an inner membrane protein that is related to a family of signal-transducing proteins. Infect. Immun. 62, 3289–3298. doi: 10.1128/IAI.62.8.3289-3298.1994
Faruque, S. M., Kamruzzaman, M., Asadulghani Sack, D. A., Mekalanos, J. J., and Nair, G. B. (2003a). CTXphi-independent production of the RS1 satellite phage by Vibrio cholerae. Proc. Natl. Acad. Sci. U.S.A. 100, 1280–1285. doi: 10.1073/pnas.0237385100
Faruque, S. M., and Mekalanos, J. J. (2003). Pathogenicity islands and phages in Vibrio cholerae evolution. Trends Microbiol. 11, 505–510. doi: 10.1016/j.tim.2003.09.003
Faruque, S. M., Zhu, J., Asadulghani Kamruzzaman, M., and Mekalanos, J. J. (2003b). Examination of diverse toxin-coregulated pilus-positive Vibrio cholerae strains fails to demonstrate evidence for Vibrio pathogenicity island phage. Infect Immun. 71, 2993–2999. doi: 10.1128/IAI.71.6.2993-2999.2003
Gao, Y., Hauke, C. A., Marles, J. M., and Taylor, R. K. (2016). Effects of tcpB mutations on biogenesis and function of the toxin-coregulated pilus, the type IVb pilus of Vibrio cholerae. J. Bacteriol. 198, 2818–2828. doi: 10.1128/JB.00309-16
Gutierrez-Rodarte, M., Kolappan, S., Burrell, B. A., and Craig, L. (2019). The Vibrio cholerae minor pilin TcpB mediates uptake of the cholera toxin phage CTXphi. J. Biol. Chem. 294, 15698–15710. doi: 10.1074/jbc.RA119.009980
Hammer, B. K., and Bassler, B. L. (2003). Quorum sensing controls biofilm formation in Vibrio cholerae. Mol. Microbiol. 50, 101–104. doi: 10.1046/j.1365-2958.2003.03688.x
Harkey, C. W., Everiss, K. D., and Peterson, K. M. (1994). The Vibrio cholerae toxin-coregulated-pilus gene tcpI encodes a homolog of methyl-accepting chemotaxis proteins. Infect. Immun. 62, 2669–2678. doi: 10.1128/IAI.62.7.2669-2678.1994
Hase, C. C., and Mekalanos, J. J. (1998). TcpP protein is a positive regulator of virulence gene expression in Vibrio cholerae. Proc. Natl. Acad. Sci. U.S.A. 95, 730–734. doi: 10.1073/pnas.95.2.730
Hassan, F., Kamruzzaman, M., Mekalanos, J. J., and Faruque, S. M. (2010). Satellite phage TLCphi enables toxigenic conversion by CTX phage through dif site alteration. Nature 467, 982–985. doi: 10.1038/nature09469
Haycocks, J. R. J., Warren, G. Z. L., Walker, L. M., Chlebek, J. L., Dalia, T. N., Dalia, A. B., et al. (2019). The quorum sensing transcription factor AphA directly regulates natural competence in Vibrio cholerae. PLoS Genet. 15:e1008362. doi: 10.1371/journal.pgen.1008362
Heidelberg, J. F., Eisen, J. A., Nelson, W. C., Clayton, R. A., Gwinn, M. L., Dodson, R. J., et al. (2000). DNA sequence of both chromosomes of the cholera pathogen Vibrio cholerae. Nature 406, 477–483. doi: 10.1038/35020000
Herzog, R., Peschek, N., Frohlich, K. S., Schumacher, K., and Papenfort, K. (2019). Three autoinducer molecules act in concert to control virulence gene expression in Vibrio cholerae. Nucleic Acids Res. 47, 3171–3183. doi: 10.1093/nar/gky1320
Higgins, D. A., Pomianek, M. E., Kraml, C. M., Taylor, R. K., Semmelhack, M. F., and Bassler, B. L. (2007). The major Vibrio cholerae autoinducer and its role in virulence factor production. Nature 450, 883–886. doi: 10.1038/nature06284
Hu, D., Liu, B., Feng, L., Ding, P., Guo, X., Wang, M., et al. (2016). Origins of the current seventh cholera pandemic. Proc. Natl. Acad. Sci. U.S.A. 113, E7730–E7739. doi: 10.1073/pnas.1608732113
Hughes, K. J., Everiss, K. D., Kovach, M. E., and Peterson, K. M. (1995). Isolation and characterization of the Vibrio cholerae acfA gene, required for efficient intestinal colonization. Gene 156, 59–61. doi: 10.1016/0378-1119(95)00054-A
Jang, J., Jung, K.-T., Yoo, C.-K., and Rhie, G.-E. (2010). Regulation of hemagglutinin/protease expression by the VarS/VarA–CsrA/B/C/D system in Vibrio cholerae. Microb. Pathog. 48, 245–250. doi: 10.1016/j.micpath.2010.03.003
Jang, J., Jung, K. T., Park, J., Yoo, C. K., and Rhie, G. E. (2011). The Vibrio cholerae VarS/VarA two-component system controls the expression of virulence proteins through ToxT regulation. Microbiology 157(Pt 5), 1466–1473. doi: 10.1099/mic.0.043737-0
Jermyn, W. S., and Boyd, E. F. (2002). Characterization of a novel Vibrio pathogenicity island (VPI-2) encoding neuraminidase (nanH) among toxigenic Vibrio cholerae isolates. Microbiology 148(Pt 11), 3681–3693. doi: 10.1099/00221287-148-11-3681
Joelsson, A., Liu, Z., and Zhu, J. (2006). Genetic and phenotypic diversity of quorum-sensing systems in clinical and environmental isolates of Vibrio cholerae. Infect. Immun. 74, 1141–1147. doi: 10.1128/IAI.74.2.1141-1147.2006
Johnson, J. A., Salles, C. A., Panigrahi, P., Albert, M. J., Wright, A. C., Johnson, R. J., et al. (1994). Vibrio cholerae O139 synonym bengal is closely related to Vibrio cholerae El Tor but has important differences. Infect. Immun. 62, 2108–2110. doi: 10.1128/IAI.62.5.2108-2110.1994
Kaper, J. B., Morris, J. G. Jr., and Levine, M. M. (1995). Cholera. Clin. Microbiol. Rev. 8, 48–86. doi: 10.1128/CMR.8.1.48
Karaolis, D. K., Johnson, J. A., Bailey, C. C., Boedeker, E. C., Kaper, J. B., and Reeves, P. R. (1998). A Vibrio cholerae pathogenicity island associated with epidemic and pandemic strains. Proc. Natl. Acad. Sci. U.S.A. 95, 3134–3139. doi: 10.1073/pnas.95.6.3134
Karaolis, D. K., Lan, R., Kaper, J. B., and Reeves, P. R. (2001). Comparison of Vibrio cholerae pathogenicity islands in sixth and seventh pandemic strains. Infect. Immun. 69, 1947–1952. doi: 10.1128/IAI.69.3.1947-1952.2001
Karaolis, D. K., Lan, R., and Reeves, P. R. (1995). The sixth and seventh cholera pandemics are due to independent clones separately derived from environmental, nontoxigenic, non-O1 Vibrio cholerae. J. Bacteriol. 177, 3191–3198. doi: 10.1128/JB.177.11.3191-3198.1995
Karaolis, D. K., Somara, S., Maneval, D. R. Jr., Johnson, J. A., and Kaper, J. B. (1999). A bacteriophage encoding a pathogenicity island, a type-IV pilus and a phage receptor in cholera bacteria. Nature 399, 375–379. doi: 10.1038/20715
Kaufman, M. R., Seyer, J. M., and Taylor, R. K. (1991). Processing of TCP pilin by TcpJ typifies a common step intrinsic to a newly recognized pathway of extracellular protein secretion by gram-negative bacteria. Genes Dev. 5, 1834–1846. doi: 10.1101/gad.5.10.1834
Kimsey, H. H., and Waldor, M. K. (1998). CTXphi immunity: application in the development of cholera vaccines. Proc. Natl. Acad. Sci. U.S.A. 95, 7035–7039. doi: 10.1073/pnas.95.12.7035
Klose, K. E. (2000). The suckling mouse model of cholera. Trends Microbiol. 8, 189–191. doi: 10.1016/S0966-842X(00)01721-2
Kolappan, S., and Craig, L. (2013). Structure of the cytoplasmic domain of TcpE, the inner membrane core protein required for assembly of the Vibrio cholerae toxin-coregulated pilus. Acta Crystallogr. D Biol. Crystallogr. 69(Pt 4), 513–519. doi: 10.1107/S0907444912050330
Kovacikova, G., and Skorupski, K. (2002). Regulation of virulence gene expression in Vibrio cholerae by quorum sensing: HapR functions at the aphA promoter. Mol. Microbiol. 46, 1135–1147. doi: 10.1046/j.1365-2958.2002.03229.x
Krukonis, E. S., Yu, R. R., and Dirita, V. J. (2000). The Vibrio cholerae ToxR/TcpP/ToxT virulence cascade: distinct roles for two membrane-localized transcriptional activators on a single promoter. Mol. Microbiol. 38, 67–84. doi: 10.1046/j.1365-2958.2000.02111.x
Kumar, A., Bag, S., and Das, B. (2018). Novel genetic tool to study the stability of genomic islands. Recent Pat. Biotechnol. 12, 200–207. doi: 10.2174/1872208312666180223113618
Li, M., Kotetishvili, M., Chen, Y., and Sozhamannan, S. (2003). Comparative genomic analyses of the vibrio pathogenicity island and cholera toxin prophage regions in nonepidemic serogroup strains of Vibrio cholerae. Appl. Environ. Microbiol. 69, 1728–1738. doi: 10.1128/AEM.69.3.1728-1738.2003
Lim, M. S., Ng, D., Zong, Z., Arvai, A. S., Taylor, R. K., Tainer, J. A., et al. (2010). Vibrio cholerae El Tor TcpA crystal structure and mechanism for pilus-mediated microcolony formation. Mol. Microbiol. 77, 755–770. doi: 10.1111/j.1365-2958.2010.07244.x
Lowden, M. J., Skorupski, K., Pellegrini, M., Chiorazzo, M. G., Taylor, R. K., and Kull, F. J. (2010). Structure of Vibrio cholerae ToxT reveals a mechanism for fatty acid regulation of virulence genes. Proc. Natl. Acad. Sci. U.S.A. 107, 2860–2865. doi: 10.1073/pnas.0915021107
Manning, P. A. (1997). The tcp gene cluster of Vibrio cholerae. Gene 192, 63–70. doi: 10.1016/S0378-1119(97)00036-X
McLeod, S. M., and Waldor, M. K. (2004). Characterization of XerC- and XerD-dependent CTX phage integration in Vibrio cholerae. Mol. Microbiol. 54, 935–947. doi: 10.1111/j.1365-2958.2004.04309.x
Megli, C. J., Yuen, A. S., Kolappan, S., Richardson, M. R., Dharmasena, M. N., Krebs, S. J., et al. (2011). Crystal structure of the Vibrio cholerae colonization factor TcpF and identification of a functional immunogenic site. J. Mol. Biol. 409, 146–158. doi: 10.1016/j.jmb.2011.03.027
Meibom, K. L., Blokesch, M., Dolganov, N. A., Wu, C. Y., and Schoolnik, G. K. (2005). Chitin induces natural competence in Vibrio cholerae. Science 310, 1824–1827. doi: 10.1126/science.1120096
Miller, V. L., Taylor, R. K., and Mekalanos, J. J. (1987). Cholera toxin transcriptional activator toxR is a transmembrane DNA binding protein. Cell 48, 271–279. doi: 10.1016/0092-8674(87)90430-2
Mishra, A., Srivastava, R., Pruzzo, C., and Srivastava, B. S. (2003). Mutation in tcpR gene (VC0832) of Vibrio cholerae O1 causes loss of tolerance to high osmolarity and affects colonization and virulence in infant mice. J. Med. Microbiol. 52(Pt 11), 933–939. doi: 10.1099/jmm.0.05171-0
Mutreja, A., Kim, D. W., Thomson, N. R., Connor, T. R., Lee, J. H., Kariuki, S., et al. (2011). Evidence for several waves of global transmission in the seventh cholera pandemic. Nature 477, 462–465. doi: 10.1038/nature10392
Nye, M. B., Pfau, J. D., Skorupski, K., and Taylor, R. K. (2000). Vibrio cholerae H-NS silences virulence gene expression at multiple steps in the ToxR regulatory cascade. J. Bacteriol. 182, 4295–4303. doi: 10.1128/JB.182.15.4295-4303.2000
Nye, M. B., and Taylor, R. K. (2003). Vibrio cholerae H-NS domain structure and function with respect to transcriptional repression of ToxR regulon genes reveals differences among H-NS family members. Mol. Microbiol. 50, 427–444. doi: 10.1046/j.1365-2958.2003.03701.x
O'Shea, Y. A., and Boyd, E. F. (2002). Mobilization of the Vibrio pathogenicity island between Vibrio cholerae isolates mediated by CP-T1 generalized transduction. FEMS Microbiol. Lett. 214, 153–157. doi: 10.1111/j.1574-6968.2002.tb11339.x
O'Shea, Y. A., Finnan, S., Reen, F. J., Morrissey, J. P., O'Gara, F., and Boyd, E. F. (2004). The Vibrio seventh pandemic island-II is a 26.9 kb genomic island present in Vibrio cholerae El Tor and O139 serogroup isolates that shows homology to a 43.4 kb genomic island in V. vulnificus. Microbiology 150(Pt 12), 4053–4063. doi: 10.1099/mic.0.27172-0
Pang, B., Yan, M., Cui, Z., Ye, X., Diao, B., Ren, Y., et al. (2007). Genetic diversity of toxigenic and nontoxigenic Vibrio cholerae serogroups O1 and O139 revealed by array-based comparative genomic hybridization. J. Bacteriol. 189, 4837–4849. doi: 10.1128/JB.01959-06
Parsot, C., Taxman, E., and Mekalanos, J. J. (1991). ToxR regulates the production of lipoproteins and the expression of serum resistance in Vibrio cholerae. Proc. Natl. Acad. Sci. U.S.A. 88, 1641–1645. doi: 10.1073/pnas.88.5.1641
Prouty, M. G., Osorio, C. R., and Klose, K. E. (2005). Characterization of functional domains of the Vibrio cholerae virulence regulator ToxT. Mol. Microbiol. 58, 1143–1156. doi: 10.1111/j.1365-2958.2005.04897.x
Provenzano, D., and Klose, K. E. (2000). Altered expression of the ToxR-regulated porins OmpU and OmpT diminishes Vibrio cholerae bile resistance, virulence factor expression, and intestinal colonization. Proc. Natl. Acad. Sci. U.S.A. 97:10220. doi: 10.1073/pnas.170219997
Rajanna, C., Wang, J., Zhang, D., Xu, Z., Ali, A., Hou, Y. M., et al. (2003). The vibrio pathogenicity island of epidemic Vibrio cholerae forms precise extrachromosomal circular excision products. J. Bacteriol. 185, 6893–6901. doi: 10.1128/JB.185.23.6893-6901.2003
Raskin, D. M., Mishra, A., He, H., and Lundy, Z. (2020). Stringent response interacts with the ToxR regulon to regulate Vibrio cholerae virulence factor expression. Arch. Microbiol. 202, 1359–1368. doi: 10.1007/s00203-020-01847-6
Rhine, J. A., and Taylor, R. K. (1994). TcpA pilin sequences and colonization requirements for O1 and O139 Vibrio cholerae. Mol. Microbiol. 13, 1013–1020. doi: 10.1111/j.1365-2958.1994.tb00492.x
Sack, D. A., Sack, R. B., Nair, G. B., and Siddique, A. K. (2004). Cholera. Lancet 363, 223–233. doi: 10.1016/S0140-6736(03)15328-7
Schuhmacher, D. A., and Klose, K. E. (1999). Environmental signals modulate ToxT-dependent virulence factor expression in Vibrio cholerae. J. Bacteriol. 181, 1508–1514. doi: 10.1128/JB.181.5.1508-1514.1999
Singh, D. V., Matte, M. H., Matte, G. R., Jiang, S., Sabeena, F., Shukla, B. N., et al. (2001). Molecular analysis of Vibrio cholerae O1, O139, non-O1, and non-O139 strains: clonal relationships between clinical and environmental isolates. Appl. Environ. Microbiol. 67, 910–921. doi: 10.1128/AEM.67.2.910-921.2001
Szabady, R. L., Yanta, J. H., Halladin, D. K., Schofield, M. J., and Welch, R. A. (2011). TagA is a secreted protease of Vibrio cholerae that specifically cleaves mucin glycoproteins. Microbiology 157(Pt 2), 516–525. doi: 10.1099/mic.0.044529-0
Tam, V. C., Serruto, D., Dziejman, M., Brieher, W., and Mekalanos, J. J. (2007). A type III secretion system in Vibrio cholerae translocates a formin/spire hybrid-like actin nucleator to promote intestinal colonization. Cell Host Microbe 1, 95–107. doi: 10.1016/j.chom.2007.03.005
Tripathi, S. A., and Taylor, R. K. (2007). Membrane association and multimerization of TcpT, the cognate ATPase ortholog of the Vibrio cholerae toxin-coregulated-pilus biogenesis apparatus. J. Bacteriol. 189, 4401–4409. doi: 10.1128/JB.00008-07
Tsou, A. M., Liu, Z., Cai, T., and Zhu, J. (2011). The VarS/VarA two-component system modulates the activity of the Vibrio cholerae quorum-sensing transcriptional regulator HapR. Microbiology 157(Pt 6), 1620–1628. doi: 10.1099/mic.0.046235-0
Val, M. E., Bouvier, M., Campos, J., Sherratt, D., Cornet, F., Mazel, D., et al. (2005). The single-stranded genome of phage CTX is the form used for integration into the genome of Vibrio cholerae. Mol. Cell 19, 559–566. doi: 10.1016/j.molcel.2005.07.002
Valiente, E., Davies, C., Mills, D. C., Getino, M., Ritchie, J. M., and Wren, B. W. (2018). Vibrio cholerae accessory colonisation factor AcfC: a chemotactic protein with a role in hyperinfectivity. Sci. Rep. 8:8390. doi: 10.1038/s41598-018-26570-7
Vanden Broeck, D., Horvath, C., and De Wolf, M. J. (2007). Vibrio cholerae: cholera toxin. Int. J. Biochem. Cell Biol. 39, 1771–1775. doi: 10.1016/j.biocel.2007.07.005
Weill, F. X., Domman, D., Njamkepo, E., Almesbahi, A. A., Naji, M., Nasher, S. S., et al. (2019). Genomic insights into the 2016-2017 cholera epidemic in Yemen. Nature 565, 230–233. doi: 10.1038/s41586-018-0818-3
Withey, J. H., and DiRita, V. J. (2005). Activation of both acfA and acfD transcription by Vibrio cholerae ToxT requires binding to two centrally located DNA sites in an inverted repeat conformation. Mol. Microbiol. 56, 1062–1077. doi: 10.1111/j.1365-2958.2005.04589.x
Zhu, J., and Mekalanos, J. J. (2003). Quorum sensing-dependent biofilms enhance colonization in Vibrio cholerae. Dev. Cell 5, 647–656. doi: 10.1016/S1534-5807(03)00295-8
Keywords: cholera pathogenesis, mobile genetic elements (MGEs), VPI-1, quorum sensing, toxin co-regulated pilus
Citation: Kumar A, Das B and Kumar N (2020) Vibrio Pathogenicity Island-1: The Master Determinant of Cholera Pathogenesis. Front. Cell. Infect. Microbiol. 10:561296. doi: 10.3389/fcimb.2020.561296
Received: 12 May 2020; Accepted: 11 September 2020;
Published: 06 October 2020.
Edited by:
Yang Fu, Southern University of Science and Technology, ChinaReviewed by:
Jiandong Chen, University of Pennsylvania, United StatesZhi Liu, Huazhong University of Science and Technology, China
Copyright © 2020 Kumar, Das and Kumar. This is an open-access article distributed under the terms of the Creative Commons Attribution License (CC BY). The use, distribution or reproduction in other forums is permitted, provided the original author(s) and the copyright owner(s) are credited and that the original publication in this journal is cited, in accordance with accepted academic practice. No use, distribution or reproduction is permitted which does not comply with these terms.
*Correspondence: Niraj Kumar, nkumar@thsti.res.in; Bhabatosh Das, bhabatosh@thsti.res.in