- Molecular Medicine, Research Institute, Hospital for Sick Children, Toronto, ON, Canada
Verotoxin, VT (aka Shiga toxin,Stx) is produced by enterohemorrhagic E. coli (EHEC) and is the key pathogenic factor in EHEC-induced hemolytic uremic syndrome (eHUS-hemolytic anemia/thrombocytopenia/glomerular infarct) which can follow gastrointestinal EHEC infection, particularly in children. This AB5 subunit toxin family bind target cell globotriaosyl ceramide (Gb3), a glycosphingolipid (GSL) (aka CD77, pk blood group antigen) of the globoseries of neutral GSLs, initiating lipid raft-dependent plasma membrane Gb3 clustering, membrane curvature, invagination, scission, endosomal trafficking, and retrograde traffic via the TGN to the Golgi, and ER. In the ER, A/B subunits separate and the A subunit hijacks the ER reverse translocon (dislocon-used to eliminate misfolded proteins-ER associated degradation-ERAD) for cytosolic access. This property has been used to devise toxoid-based therapy to temporarily block ERAD and rescue the mutant phenotype of several genetic protein misfolding diseases. The A subunit avoids cytosolic proteosomal degradation, to block protein synthesis via its RNA glycanase activity. In humans, Gb3 is primarily expressed in the kidney, particularly in the glomerular endothelial cells. Here, Gb3 is in lipid rafts (more ordered membrane domains which accumulate GSLs/cholesterol) whereas renal tubular Gb3 is in the non-raft membrane fraction, explaining the basic pathology of eHUS (glomerular endothelial infarct). Females are more susceptible and this correlates with higher renal Gb3 expression. HUS can be associated with encephalopathy, more commonly following verotoxin 2 exposure. Gb3 is expressed in the microvasculature of the brain. All members of the VT family bind Gb3, but with varying affinity. VT2e (pig edema toxin) binds Gb4 preferentially. Verotoxin-specific therapeutics based on chemical analogs of Gb3, though effective in vitro, have failed in vivo. While some analogs are effective in animal models, there are no good rodent models of eHUS since Gb3 is not expressed in rodent glomeruli. However, the mouse mimics the neurological symptoms more closely and provides an excellent tool to assess therapeutics. In addition to direct cytotoxicity, other factors including VT–induced cytokine release and aberrant complement cascade, are now appreciated as important in eHUS. Based on atypical HUS therapy, treatment of eHUS patients with anticomplement antibodies has proven effective in some cases. A recent switch using stem cells to try to reverse, rather than prevent VT induced pathology may prove a more effective methodology.
Introduction
Originally termed Verotoxin (VT), because of its discovery as a novel enterohemorrhagic E coli (O157:H7) derived toxin effective against the vero African green monkey kidney cell line (Konowalchuk et al., 1977), but currently more frequently termed Shiga toxin or Shiga-like toxin due to its single amino acid difference with Shiga toxin (Stx) from Shigella dysenteriae (DeGrandis et al., 1987).
EHEC produced Verotoxin 1 was shown, in Karmali's landmark studies (Karmali et al., 1985), to be responsible for the hemolytic uremic syndrome, a renal glomerular pathology with a triad of symptoms: thrombocytopenia, hemolytic anemia, and glomerular endothelial infarct, with no previously defined cause. Unfortunately, antibiotic treatment increases rather than reduces pathology (Zhang et al., 2000). Females are more susceptible and this correlates with increased renal Gb3 (Fujii et al., 2016). With a fatality rate of ~10% and highest incidence in the pediatric and elderly population, it is of concern that since the infectious cause was defined 35 years ago, no specific therapeutic approach has been achieved.
EHEC Toxins
The Shiga (vero) toxins are a family of AB5 bacterial subunit toxins, primarily comprising VT1 and VT2 (Nakao and Takeda, 2000) [though many other minor variants of VT2 are known (Zhang et al., 2008)]. VT2 is 60% identical but significantly less toxic than VT1 in cell culture (Fuller et al., 2011). VT2 binds Gb3 with lower affinity (Nakajima et al., 2001) but VT2 causes increased toxicity in mice (Conrady et al., 2010; Fuller et al., 2011) and other animal models (Mizuguchi et al., 2001; Takahashi et al., 2008) with increased association with disease (Kawano et al., 2008), particularly neurological sequelae following EHEC infection (Trachtman et al., 2012; Kramer et al., 2015). The different toxicity potency is due to B subunit differences (Head et al., 1991).
VT Receptor
The receptor binding pentameric B subunit of Shiga toxin binds the neutral glycosphingolipid (GSL), globotriaosyl ceramide (Gb3, aka CD77 and the pk blood group antigen) (Lingwood et al., 1987), which is highly expressed in the human kidney (Boyd and Lingwood, 1989; Lingwood, 1994). GSLs are sugar-ceramide conjugates, for the most part, based on the ceramide monohexoside, glucosyl ceramide. Single sugar units are added in α or β anomeric linkage to form linear or branch chain GSLs in the Golgi membrane, which are then transported to the plasma membrane by vesicular traffic. Over 400 carbohydrate structures have been defined (Stults et al., 1989). The structure of Gb3 is galactose α 1-4 galactose β 1-4 glucosyl ceramide. The terminal gal α 1-4 gal is bound by the VT B subunit pentamer, but the lipid moiety is necessary for high affinity binding. While Gb3 is the receptor for VTs from human EHEC pathogens, VT2e from the pig EHEC binds Gb4, the next member of the globoseries GSLs which contains an additional β 1-3 galNAc (DeGrandis et al., 1989). Interestingly, although VT1 and VT2 do not bind Gb4, they bind (non-physiological) deacetylGb4 (terminal free amino sugar) in preference to Gb3 (Nyholm et al., 1996). Differential chemical substitution of this free amine has a remarkable and varied effect on the binding of different VTs (Mylvaganam et al., 2015). The binding affinity for the lipid-free gal α 1-4 gal disaccharide is many orders less than for Gb3 (St. Hilaire et al., 1994). The ceramide lipid component of Gb3 (and all GSLs) varies greatly and this has a major effect on VTB:Gb3 binding (Pellizzari et al., 1992; Kiarash et al., 1994). The orientation of the carbohydrate of membrane GSLs is dependent on both the lipid composition of the GSL itself and the membrane in which it is embedded (Nyholm et al., 1989; Nyholm and Pascher, 1993). Moreover, GSLs accumulate in (detergent resistant) cholesterol enriched lipid rafts (Hooper, 1999; Legros et al., 2017).
Retrograde Transport
The presence of Gb3 in lipid plasma membrane rafts is required for the retrograde transport of internalized VT to the ER (Falguieres et al., 2001). Non-raft Gb3 targets internalized VT to lysosomes for degradation. Receptor mediated VT cell internalization is via clathrin dependent and independent mechanisms (Khine et al., 2004). The multivalent B subunit pentamer binding to cell surface raft and model membrane Gb3 has been shown to cluster Gb3 (Khine and Lingwood, 1994; Windschiegl et al., 2009; Pezeshkian et al., 2017) which causes subsequent energy and clathrin independent tubular invaginations (negative membrane curvature) (Römer et al., 2007; Bosse et al., 2019) (Figure 1). This effect is Gb3 unsaturated fatty acid dependent and does not involve cytoskeletal components (Römer et al., 2007). Pinching off of the vesicles occurs after actin mediated, cholesterol dependent membrane domain reorganization (Römer et al., 2010). Membrane incorporation of lysophospholipids with large head groups blocks VT membrane Gb3 binding and can reverse Gb3 bound VT (Ailte et al., 2016). These phospholipids induce a positive membrane curvature which may prevent the negative membrane curvature induced by VT-Gb3 binding.
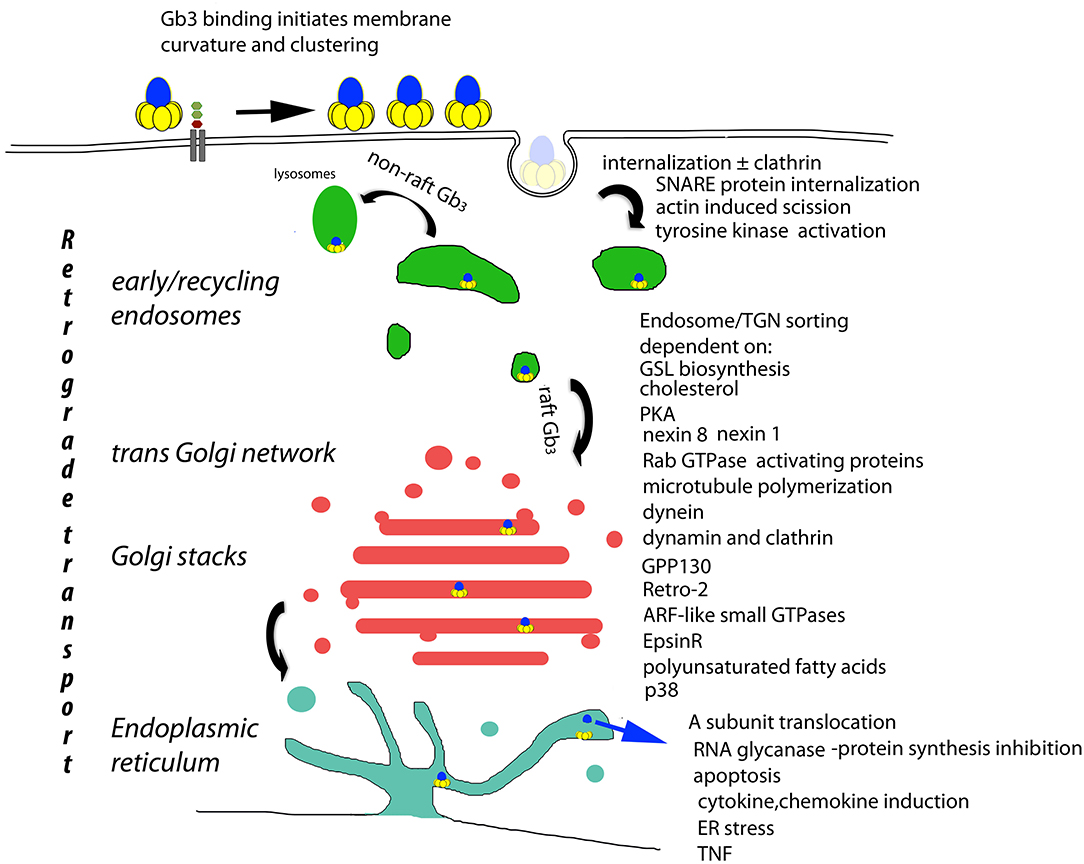
Figure 1. Basis of cellular VT cytopathology. The retrograde transport of plasma membrane bound VT (Römer et al., 2007) is the central determinant of cytopathology. Receptor mediated endocytosis (Sandvig et al., 1989; Khine et al., 2004; Bujny et al., 2007; Rydell et al., 2014; Renard et al., 2015), tyrosine kinase activation (Katagiri et al., 1999; Mori et al., 2000; Lauvrak et al., 2005; Utskarpen et al., 2010), non-raft Gb3 bound VT lysosomal transit (Falguieres et al., 2001) raft Gb3 bound VT endosomal/TGN transit [and A subunit ER/cytosolic transport] (Lord et al., 2003; Spooner and Lord, 2012) are all essential to end stage cytopathology (Brigotti et al., 1997; Foster et al., 2000; Fujii et al., 2003; Yamasaki et al., 2004; Jandhyala et al., 2008; Stearns-Kurosawa et al., 2010; Leyva-Illades et al., 2012; Debernardi et al., 2018). These control points provide candidates for the prevention of VT cytopathology. However, they also control retrograde transport in general, and although no physiological substrate for retrograde transport has yet been described, the potential for side effects persists.
Control of endosomal VT/Gb3 sorting to the transGolgi network for retrograde transport to the Golgi and ER, is achieved by a large array of trafficking regulators (Figure 1). However, cell bound VT1 and VT2 reach this sorting pathway by different routes (Tam et al., 2008). The A subunit is proteolytically clipped by furin (Lea et al., 1999) in the Golgi (Voorhees et al., 1995), but the C terminal disulfide bond holding the A1 and A2 peptides together is only cleaved in the ER where the A1 subunit separates from the B pentamer, and, via its newly exposed C terminal misfolded protein-mimic sequence (Hazes and Read, 1997), hijacks the ER chaperone quality control system to be unfolded and transported via the ER dislocon into the cytosol (Yu and Haslam, 2005; Tam and Lingwood, 2007) for inhibition of protein synthesis/cell killing via RNA glycanase activity (Endo and Tsurugi, 1987). Since this dislocon is key in ER associated degradation of misfolded proteins (Hebert et al., 2010), this hijacking can be used therapeutically in protein misfolding diseases (Adnan et al., 2016).
GB3 Binding
In the human renal glomerulus, Gb3 is in lipid rafts whereas in tubular cells, Gb3 is in the non-raft fraction (Khan et al., 2009). This correlates with the glomerular site of primary pathology in eHUS. However, the interaction of cholesterol with GSLs can markedly restrict the orientation of the membrane GSL carbohydrate moiety for ligand binding (Mahfoud et al., 2010; Yahi et al., 2010; Lingwood et al., 2011).
Site specific mutagenesis and molecular modeling from the B subunit crystal structure (Stein et al., 1992) identified 3 potential Gb3 binding sites (Nyholm et al., 1989; Ling et al., 1998; Bast et al., 1999; Soltyk et al., 2002). Site 1 is in the interface between B subunits, site 2 is a shallow groove on the membrane apposed B subunit surface and site 3 is the single central tryptophan34. Thermodynamically site 1 is the high affinity site (Nyholm et al., 1996) but cocrystalization with a gal α 1-4 gal carbohydrate showed site 2 was most frequently occupied (Ling et al., 1998). It remains likely that site 1 is the Gb3 glycolipid binding site but site 2 has been targeted using gal α 1-4 gal β 1-4 glc sugar derivatives as a potential prophylactic approach (Ling et al., 1998).
Receptor Analogs
Synsorb pk was the first wherein gal α 1-4 gal β 1-4 glc oligosaccharide was coupled to an inert silica matrix (Armstrong et al., 1991). This was effective to block cytotoxicity in cell culture but not in a randomized clinical trial (Armstrong et al., 1995; Trachtman et al., 2003). Multimeric derivatives (Watanabe et al., 2004; Nishikawa et al., 2005; Jacobson et al., 2014; Matsuoka et al., 2018) [particularly the pentameric “starfish” array (Kitov et al., 2000; Mulvey et al., 2003)] have been used to increase binding avidity generating a “sandwich” whereby the polymeric galactose α 1-4 galactose disaccharide binds to two B subunit pentameric arrays (Kitov et al., 2000). This sandwich structure prevents cell surface Gb3 binding to block toxicity. Although effective in cell culture and the mouse to prevent VT cytotoxicity (Kitov et al., 2008a), the compound has yet to be tested clinically as a prophylactic for HUS.
Gb3 carbohydrate multimerically coupled to a series of acrylamide polymers also proved effective inhibitors of VT cytopathology in cell culture and in mice (Nishikawa et al., 2005; Watanabe et al., 2006; Watanabe-Takahashi et al., 2010). A Gb3-trehalose acrylamide copolymer was also effective against VT in cell culture and the mouse model (Neri et al., 2007a). However acrylamide is toxic in vivo (Tareke et al., 2002). In optimization studies of these complexes, it was found the VT 1 and VT2 bind to completely different regions of the Gb3 oligosaccharide (Watanabe et al., 2006), a result consistent with our earlier observation that VT1 and VT2 bind preferentially to different conformers of Gb3 (Nyholm et al., 1996). A tetravalent peptide was developed (Nishikawa et al., 2006) which prevented the traffic of VT to the ER in cell culture and prevented VT2-induced fluid accumulation in rabbit ileal loops (Watanabe-Takahashi et al., 2010). Gb3 oligosaccharides complexed with cyclodextrin proved high affinity receptors for both VT1 and VT2 (Zhang et al., 2017) but not as yet reported for neutralization. Other Gb3 mimics have been selected from peptide libraries (Miura et al., 2004, 2006) which are bound by VTs (and antiGb3). These were protective in cell culture. However, peptides can be immunogenic and were not tested clinically. Gb3 sugar coupled to phosphatidylethanolamine in a liposomal format also proved effective against VT in cell culture (Neri et al., 2007b; Detzner et al., 2019). A unique Gb3 sugar based heterobifunctional crosslinker was made to decorate the pentameric serum amyloid P to block VT1/VT2 in vitro but rapid clearance prevented in vivo efficacy (Kitov et al., 2008b). A similar construct using a GalNAc instead of a Gal Gb3 trisaccharide selectively blocked VT2 in vivo (Jacobson et al., 2014).
The sugar sequence of Gb3 is mimicked by the carbohydrate moiety of some bacterial lipooligosaccharides (Mandrell and Apicella, 1993). The bacterial β-galactosyl and α-galactosyl transferases responsible from Neiseria were cloned into a commensal E.coli expressing glucose terminating LPS (Paton et al., 2000). This E.coli proved effective to protect cells and animal models against VT pathology (Paton A. W. et al., 2001). A similar Gb4-LPS E.coli was protective in pig edema disease (Paton A. W. et al., 2001; Hostetter et al., 2014). These constructs were further modified to be suitable for clinical trial (Paton J. C. et al., 2001) and non-genetically modified “Gb3” expressing bacterial “ghosts” also were effective (Paton et al., 2015), but as yet no clinical trials have been reported.
Our approach was to target the B subunit Gb3 glycolipid binding site, site1 (Nyholm et al., 1996). Since the binding affinity for the lipid-free trisaccharide of Gb3 is so low, we attempted to generate a “water soluble” Gb3 analog (Mylvaganam and Lingwood, 1999). We found that substituting the fatty acid of GSLs with an adamantane frame generated species which partitioned into water yet retained similar hydrophobicity as monitored by thin layer chromatogram mobility (Lingwood and Mylvaganam, 2003). Although the fatty acid is important in VT-Gb3 binding (Kiarash et al., 1994; Pezeshkian et al., 2015), these species (unlike the free sugar) retained the biological activity of the membrane embedded GSL in solution (Lingwood et al., 2006). AdaGlcCer and adaGalCer proved effective inhibitors of cellular GSL biosynthesis (Kamani et al., 2011), while adaSGC was an effective mimic of SGC (3-sulfogalactosyl ceramide), binding to Hsp70 (Mamelak and Lingwood, 2001), thereby inhibiting its ATPase activity (Whetstone and Lingwood, 2003) and chaperone action in cells (Park et al., 2009). Hsp70-SGC binding promotes aggregation of Hsp70 for high affinity peptide binding and blocks ATP-mediated peptide release (Harada et al., 2015).
AdamantylGb3 is a highly effective receptor for the VTB subunit (Mylvaganam and Lingwood, 1999; Lingwood et al., 2006). AdaGb3 is bound by VT2 more effectively than Gb3 (Saito et al., 2012). AdaGb3 blocks VT –Gb3 and cell binding in vitro (Mylvaganam and Lingwood, 1999). We made several lipid derivatives of adaGb3. However, adaGb3 and derivatives can incorporate into Gb3 negative cell lines and induce VT1/2 sensitivity (Saito et al., 2012). This is interesting, since the different analogs can differentially subvert the intracellular traffic of VT (Saito et al., 2012), but is not a feature consistent with a therapeutic. We therefore made an adamantyl bis Gb3 which has two deacylGb3s linked to a single central adamantane frame. This protected cells from VT more effectively than adaGb3 and was not incorporated into receptor negative cells (Saito et al., 2012). Despite protection against VT in cell culture, subsequent in vivo mouse susceptibility studies with adabisGb3 showed that treated mice showed a more rapid VT-induced pathology than control mice. A similar effect has been reported for lysoGb3 containing liposomes in vivo (Takenaga et al., 2000). While the basis of this effect in not clear, it may relate to the potential of multivalent VT to show cooperative receptor binding (Peter and Lingwood, 2000).
VT and eHUS
The pathology of eHUS is complex. In the baboon model, sterile VT was clearly shown to induce HUS following intravenous administration (Siegler et al., 2003). The question of how VT enters the blood stream following gastrointestinal EHEC infection, is still unclear. VT is difficult to detect in patient blood after EHEC infection but can be detected in the acute phase (He et al., 2015; Yamada et al., 2019). However, these blood concentrations are far lower than needed to cause HUS in the baboon. Moreover, if the VT dose inducing HUS in baboon was divided into 4 (still much higher than ever detected in eHUS patients) and given every 12 h, no HUS pathology was observed (Siegler R. et al., 2001).
Significantly, Gb3 is upregulated in many human cancers (LaCasse et al., 1999; Kovbasnjuk et al., 2005; Distler et al., 2009; Stimmer et al., 2014). In light of the baboon data indicating there could be a “safe” dose for sterile VT, we (Farkas-Himsley et al., 1995; Arab et al., 1999; Arbus et al., 2000) and later others (Gariepy, 2001; Falguieres et al., 2008; Devenica et al., 2011; Engedal et al., 2011), have proposed VT could be used as the basis of an antineoplastic.
Other Factors in eHUS
It is clear that other systemic factors are at play in eHUS. One of these is bacterial LPS, which augments baboon VT renal toxicity (Siegler R. L., et al., 2001) and renal Gb3 (Clayton et al., 2005). LPS can induce proinflammatory cytokines TNF and IL1 β (Eggesbo et al., 1996) which in turn, can increase cell Gb3 synthesis (Warnier et al., 2006) and VT sensitivity (Louise and Obrig, 1991). VT itself can induce TNF production (van Setten et al., 1996) by monocytes. These cells are not sensitive to VT toxicity but the A subunit is required (Foster et al., 2000) (Figure 1). This cytokine has been recently shown to play a role to set VT sensitivity in eHUS (Brigotti et al., 2018; Lafalla Manzano et al., 2019).
VT interaction with neutrophils has also been suggested to be important. Gb3 is not expressed in the human colonic epithelium (Miyamoto et al., 2006) and so the mechanism by which gastrointestinal VT gains systemic access remains to be answered. Several studies suggest a VT-neutrophil transport function (Hurley et al., 2001; Griener et al., 2007; Brigotti et al., 2008, 2010). Other work, however, concluded VT does not bind human neutrophils (Flagler et al., 2007; Geelen et al., 2007), although mouse neutrophils were bound (Fernandez et al., 2000, 2006) and can target mouse kidney (Roche et al., 2007). Nevertheless, recent studies show neutrophil released traps [NETs -anti infectious/inflammatory response (Boeltz et al., 2019)] are increased (Ramos et al., 2016) and their degradation reduced (Leffler et al., 2017) in eHUS.
Since complement insufficiency plays a central role in idiopathic thrombocytopenia and atypical HUS (Nielsen et al., 1994), VT activation of the alternative complement pathway (Morigi et al., 2011) has become considered of potential importance in eHUS (Karpman and Tati, 2016; Fakhouri and Loirat, 2018). However, these interactions of VT with different components of the blood system should be reviewed in light of the differences showed for the binding of native vs. proteolytically clipped VT recently reported (Brigotti et al., 2019).
New Therapeutics
Complement
The treatment of acquired or congenital thrombocytopenia with Eculizumab, an anti-C5 monoclonal antibody which blocks complement activation, has proven successful (Pecoraro et al., 2015; Bitzan et al., 2018). Since VT can activate complement, this approach has been tested for efficacy in eHUS (Rasa et al., 2017; Walsh and Johnson, 2019). Therapeutic effect has been shown, particularly for patients with neurological symptoms (Percheron et al., 2018) but results overall are, as yet, highly variable (Loos et al., 2018; Monet-Didailler et al., 2019).
Stem Cells
Advantage has recently been taken of the huge effort to develop stem cells as disease therapeutics. Muse (multilineage differentiating stress enduring) cells are a small non-tumorigenic, non-immunogenic component of the mesenchymal stem cell fraction (Kuroda et al., 2010) which can be isolated from many sources (Leng et al., 2019), including bone marrow, adipose tissue and fibroblasts. Muse cells home to sites of tissue damage and there differentiate to regenerate the damaged tissue type (Young, 2018). This includes neuronal cells (Nitobe et al., 2019). There are many current clinical trials based on these cells (Dezawa, 2018; Kuroda et al., 2018).
Human Muse cells were used to rescue a NOD-SCID mouse model of EHEC (VT2) induced neurological disease (Ozuru et al., 2019). Forty eight hour after gastrointestinal EHEC treatment Muse cell i.v. injection resulted in complete survival and lack of weight loss, whereas >50% of untreated mice died of encephalopathy. Splitting the Muse cell dosage and treating at 24 and 48 h post-infection did not give protection. Human Muse cells were found in the mouse brain and the level of VT2 activated astrocytes significantly reduced. Knockdown of G-CSF largely ablated the protection.
These studies bode well for the development of an effective means to prevent/treat VT-based disease in the future.
Author Contributions
The author confirms being the sole contributor of this work and has approved it for publication.
Conflict of Interest
CL is a founder of ERAD Therapeutic which is developing bacterial toxoids as treatment for protein misfolding diseases.
References
Adnan, H., Zhang, Z., Park, H. J., Tailor, C., Che, C., Kamani, M., et al. (2016). Endoplasmic reticulum-targeted subunit toxins provide a new approach to rescue misfolded mutant proteins and revert cell models of genetic diseases. PLoS ONE 11:e0166948. doi: 10.1371/journal.pone.0166948
Ailte, I., Lingelem, A. B., Kavaliauskiene, S., Bergan, J., Kvalvaag, A. S., Myrann, A. G., et al. (2016). Addition of lysophospholipids with large head groups to cells inhibits Shiga toxin binding. Sci. Rep. 6:30336. doi: 10.1038/srep30336
Arab, S., Rutka, J., and Lingwood, C. (1999). Verotoxin induces apoptosis and the complete, rapid, long-term elimination of human astrocytoma xenografts in nude mice. Oncol. Res. 11, 33–39.
Arbus, G. S., Grisaru, S., Segal, O., Dosch, M., Pop, M., Lala, P., et al. (2000). Verotoxin targets lymphoma infiltrates of patients with post-transplant lymphoproliferative disease. Leukemia Res. 24, 857–864. doi: 10.1016/S0145-2126(00)00060-6
Armstrong, G. D., Fodor, E., and Vanmaele, R. (1991). Investigation of Shiga-like toxin binding to chemically synthesized oligosaccharide sequences. J. Infect. Dis. 164, 1160–1167. doi: 10.1093/infdis/164.6.1160
Armstrong, G. D., Rowe, P. C., Goodyer, P., Orrbine, E., Klassen, T. P., Wells, G., et al. (1995). A phase 1 study of chemically synthesized verotoxin (shiga-like toxin) Pk-trisaccharide receptors attached to chromosorb for preventing Hemolytic Uremic Syndrome (HUS). J. Infect. Dis. 171, 1042–1045. doi: 10.1093/infdis/171.4.1042
Bast, D. J., Banerjee, L., Clark, C., Read, R. J., and Brunton, J. L. (1999). The identification of three biologically relevant globotriaosyl ceramide receptor binding sites on the verotoxin 1 B subunit. Mol. Microbiol. 32, 953–960. doi: 10.1046/j.1365-2958.1999.01405.x
Bitzan, M., Hammad, R. M., Bonnefoy, A., Al Dhaheri, W. S., Vezina, C., and Rivard, G. E. (2018). Acquired thrombotic thrombocytopenic purpura with isolated CFHR3/1 deletion-rapid remission following complement blockade. Pediatr. Nephrol. 33, 1437–1442. doi: 10.1007/s00467-018-3957-8
Boeltz, S., Amini, P., Anders, H. J., Andrade, F., Bilyy, R., Chatfield, S., et al. (2019). To NET or not to NET: current opinions and state of the science regarding the formation of neutrophil extracellular traps. Cell Death Differ. 26, 395–408. doi: 10.1038/s41418-018-0261-x
Bosse, M., Sibold, J., Scheidt, H. A., Patalag, L. J., Kettelhoit, K., Ries, A., et al. (2019). Shiga toxin binding alters lipid packing and the domain structure of Gb3-containing membranes: a solid-state NMR study. Phys. Chem. Chem. Phys. 21, 15630–15638. doi: 10.1039/C9CP02501D
Boyd, B., and Lingwood, C. A. (1989). Verotoxin receptor glycolipid in human renal tissue. Nephron 51, 207–210. doi: 10.1159/000185286
Brigotti, M., Carnicelli, D., Alvergna, P., Mazzaracchio, R., Sperti, S., and Montanaro, L. (1997). The RNA-N-glycosidase activity of Shiga-like toxin I: kinetic parameters of the native and activated toxin. Toxicon 35, 1431–1437. doi: 10.1016/S0041-0101(96)00225-5
Brigotti, M., Carnicelli, D., Arfilli, V., Porcellini, E., Galassi, E., Valerii, M. C., et al. (2018). Human monocytes stimulated by Shiga toxin 1a via globotriaosylceramide release proinflammatory molecules associated with hemolytic uremic syndrome. Int. J. Med. Microbiol. 308, 940–946. doi: 10.1016/j.ijmm.2018.06.013
Brigotti, M., Carnicelli, D., Ravanelli, E., Barbieri, S., Ricci, F., Bontadini, A., et al. (2008). Interactions between Shiga toxins and human polymorphonuclear leukocytes. J. Leukoc. Biol. 84, 1019–1027. doi: 10.1189/jlb.0308157
Brigotti, M., Orth-Holler, D., Carnicelli, D., Porcellini, E., Galassi, E., Tazzari, P. L., et al. (2019). The structure of the Shiga toxin 2a A-subunit dictates the interactions of the toxin with blood components. Cell Microbiol. 21:e13000. doi: 10.1111/cmi.13000
Brigotti, M., Tazzari, P. L., Ravanelli, E., Carnicelli, D., Barbieri, S., Rocchi, L., et al. (2010). Endothelial damage induced by Shiga toxins delivered by neutrophils during transmigration. J. Leukoc. Biol. 88, 201–210. doi: 10.1189/jlb.0709475
Bujny, M. V., Popoff, V., Johannes, L., and Cullen, P. J. (2007). The retromer component sorting nexin-1 is required for efficient retrograde transport of Shiga toxin from early endosome to the trans Golgi network. J. Cell Sci. 120, 2010–2021. doi: 10.1242/jcs.003111
Clayton, F., Pysher, T. J., Lou, R., Kohan, D. E., Denkers, N. D., Tesh, V. L., et al. (2005). Lipopolysaccharide upregulates renal shiga toxin receptors in a primate model of hemolytic uremic syndrome. Am. J. Nephrol. 25, 536–540. doi: 10.1159/000088523
Conrady, D. G., Flagler, M. J., Friedmann, D. R., Vander Wielen, B. D., Kovall, R. A., Weiss, A. A., et al. (2010). Molecular basis of differential B-pentamer stability of Shiga toxins 1 and 2. PLoS ONE 5:e15153. doi: 10.1371/journal.pone.0015153
Debernardi, J., Hollville, E., Lipinski, M., Wiels, J., and Robert, A. (2018). Differential role of FL-BID and t-BID during verotoxin-1-induced apoptosis in Burkitt's lymphoma cells. Oncogene 37, 2410–2421. doi: 10.1038/s41388-018-0123-5
DeGrandis, S., Law, H., Brunton, J., Gyles, C., and Lingwood, C. A. (1989). Globotetraosyl ceramide is recognized by the pig edema disease toxin. J. Biol. Chem. 264, 12520–12525.
DeGrandis, S. A., Ginsberg, J., Toone, M., Climie, S., Friesen, J., and Brunton, J. (1987). Nucleotide sequence and promoter mapping of the Escherichia coli Shiga-like toxin operation of bacteriophage H-19B. J. Bacteriol. 169, 4313–4319. doi: 10.1128/JB.169.9.4313-4319.1987
Detzner, J., Gloerfeld, C., Pohlentz, G., Legros, N., Humpf, H. U., Mellmann, A., et al. (2019). Structural insights into Escherichia coli Shiga Toxin (Stx) glycosphingolipid receptors of porcine renal epithelial cells and inhibition of stx-mediated cellular injury using neoglycolipid-spiked glycovesicles. Microorganisms 7:E582. doi: 10.3390/microorganisms7110582
Devenica, D., Cikes Culic, V., Vuica, A., and Markotic, A. (2011). Biochemical, pathological and oncological relevance of Gb3Cer receptor. Med. Oncol. 28(Suppl. 1):S675–S684. doi: 10.1007/s12032-010-9732-8
Dezawa, M. (2018). Clinical trials of muse cells. Adv. Exp. Med. Biol. 1103, 305–307. doi: 10.1007/978-4-431-56847-6_17
Distler, U., Souady, J., Hulsewig, M., Drmic-Hofman, I., Haier, J., Friedrich, A. W., et al. (2009). Shiga toxin receptor Gb3Cer/CD77: tumor-association and promising therapeutic target in pancreas and colon cancer. PLoS ONE 4:e6813. doi: 10.1371/journal.pone.0006813
Eggesbo, J. B., Hjermann, I., Hostmark, A. T., and Kierulf, P. (1996). LPS induced release of IL-1 beta, IL-6, IL-8 and TNF-alpha in EDTA or heparin anticoagulated whole blood from persons with high or low levels of serum HDL. Cytokine 8, 152–160. doi: 10.1006/cyto.1996.0022
Endo, Y., and Tsurugi, K. (1987). RNA N-glycosidase activity of ricin A-chain. Mechanism of action of the toxic lectin ricin on eukaryotic ribosomes. J. Biol. Chem. 262, 8128–8130.
Engedal, N., Skotland, T., Torgersen, M. L., and Sandvig, K. (2011). Shiga toxin and its use in targeted cancer therapy and imaging. Microb. Biotechnol. 4, 32–46. doi: 10.1111/j.1751-7915.2010.00180.x
Fakhouri, F., and Loirat, C. (2018). Anticomplement treatment in atypical and typical hemolytic uremic syndrome. Semin. Hematol. 55, 150–158. doi: 10.1053/j.seminhematol.2018.04.009
Falguieres, T., Maak, M., von Weyhern, C., Sarr, M., Sastre, X., Poupon, M. F., et al. (2008). Human colorectal tumors and metastases express Gb3 and can be targeted by an intestinal pathogen-based delivery tool. Mol. Cancer Ther. 7, 2498–2508. doi: 10.1158/1535-7163.MCT-08-0430
Falguieres, T., Mallard, F., Baron, C., Hanau, D., Lingwood, C., Goud, B., et al. (2001). Targeting of Shiga toxin b-subunit to retrograde transport route in association with detergent-resistant membranes. Mol. Biol. Cell. 12, 2453–2468. doi: 10.1091/mbc.12.8.2453
Farkas-Himsley, H., Rosen, B., Hill, R., Arab, S., and Lingwood, C. A. (1995). Bacterial colicin active against tumour cells in vitro and in vivo is verotoxin 1. Proc. Natl. Acad. Sci. U.S.A. 92, 6996–7000. doi: 10.1073/pnas.92.15.6996
Fernandez, G. C., Lopez, M. F., Gomez, S. A., Ramos, M. V., Bentancor, L. V., Fernandez-Brando, R. J., et al. (2006). Relevance of neutrophils in the murine model of haemolytic uraemic syndrome: mechanisms involved in Shiga toxin type 2-induced neutrophilia. Clin. Exp. Immunol. 146, 76–84. doi: 10.1111/j.1365-2249.2006.03155.x
Fernandez, G. C., Rubel, C., Dran, G., Gomez, S., Isturiz, M. A., and Palermo, M. S. (2000). Shiga toxin-2 induces neutrophilia and neutrophil activation in a murine model of hemolytic uremic syndrome. Clin. Immunol. 95, 227–234. doi: 10.1006/clim.2000.4862
Flagler, M. J., Strasser, J. E., Chalk, C. L., and Weiss, A. A. (2007). Comparative analysis of the abilities of Shiga toxins 1 and 2 to bind to and influence neutrophil apoptosis. Infect. Immun. 75, 760–765. doi: 10.1128/IAI.01594-06
Foster, G. H., Armstrong, C. S., Sakiri, R., and Tesh, V. L. (2000). Shiga toxin-induced tumor necrosis factor alpha expression: requirement for toxin enzymatic activity and monocyte protein kinase C and protein tyrosine kinases. Infect. Immun. 68, 5183–5189. doi: 10.1128/IAI.68.9.5183-5189.2000
Fujii, J., Matsui, T., Heatherly, D. P., Schlegel, K. H., Lobo, P. I., Yutsudo, T., et al. (2003). Rapid apoptosis induced by Shiga toxin in HeLa cells. Infect. Immun. 71, 2724–2735. doi: 10.1128/IAI.71.5.2724-2735.2003
Fujii, J., Mizoue, T., Kita, T., Kishimoto, H., Joh, K., Nakada, Y., et al. (2016). Risk of haemolytic uraemic syndrome caused by shiga-toxin-producing Escherichia coli infection in adult women in Japan. Epidemiol. Infect. 144, 952–961. doi: 10.1017/S0950268815002289
Fuller, C. A., Pellino, C. A., Flagler, M. J., Strasser, J. E., and Weiss, A. A. (2011). Shiga toxin subtypes display dramatic differences in potency. Infect. Immun. 79, 1329–1337. doi: 10.1128/IAI.01182-10
Gariepy, J. (2001). The use of Shiga-like toxin 1 in cancer therapy. Crit. Rev. Oncol. Hematol. 39, 99–106. doi: 10.1016/S1040-8428(01)00126-3
Geelen, J. M., van der Velden, T. J., Te Loo, D. M., Boerman, O. C., van den Heuvel, L. P., and Monnens, L. A. (2007). Lack of specific binding of Shiga-like toxin (verocytotoxin) and non-specific interaction of Shiga-like toxin 2 antibody with human polymorphonuclear leucocytes. Nephrol. Dial. Transplant. 22, 749–755. doi: 10.1093/ndt/gfl688
Griener, T. P., Mulvey, G. L., Marcato, P., and Armstrong, G. D. (2007). Differential binding of Shiga toxin 2 to human and murine neutrophils. J. Med. Microbiol. 56, 1423–1430. doi: 10.1099/jmm.0.47282-0
Harada, Y., Sato, C., and Kitajima, K. (2015). Sulfatide-Hsp70 interaction promotes Hsp70 clustering and stabilizes binding to unfolded protein. Biomolecules 5, 958–973. doi: 10.3390/biom5020958
Hazes, B., and Read, R. J. (1997). Accumulating evidence suggests that several AB-toxins subvert the endoplasmic reticulum-associated protein degradation pathway to enter target cells. Biochemistry 36, 11051–11054. doi: 10.1021/bi971383p
He, X., Quinones, B., Loo, M. T., Loos, S., Scavia, G., Brigotti, M., et al. (2015). Serum Shiga toxin 2 values in patients during acute phase of diarrhoea-associated haemolytic uraemic syndrome. Acta Paediatr. 104, e564–e568. doi: 10.1111/apa.13211
Head, S., Karmali, M., and Lingwood, C. A. (1991). Preparation of VT1 and VT2 hybrid toxins from their purified dissociated subunits: Evidence for B subunit modulation of A subunit function. J. Biol. Chem. 266, 3617–3621.
Hebert, D. N., Bernasconi, R., and Molinari, M. (2010). ERAD substrates: which way out? Semin. Cell Dev. Biol. 21, 526–532. doi: 10.1016/j.semcdb.2009.12.007
Hooper, N. (1999). Detergent-insoluble glycosphingolipid/cholesterol-rich membrane domains, lipid rafts and caveolae. Mol. Membr. Biol. 16, 145–156. doi: 10.1080/096876899294607
Hostetter, S. J., Helgerson, A. F., Paton, J. C., Paton, A. W., and Cornick, N. A. (2014). Therapeutic use of a receptor mimic probiotic reduces intestinal Shiga toxin levels in a piglet model of hemolytic uremic syndrome. BMC Res. Notes. 7:331. doi: 10.1186/1756-0500-7-331
Hurley, B. P., Thorpe, C. M., and Acheson, D. W. (2001). Shiga Toxin Translocation across intestinal epithelial cells is enhanced by neutrophil transmigration. Infect. Immun. 69, 6148–6155. doi: 10.1128/IAI.69.10.6148-6155.2001
Jacobson, J. M., Yin, J., Kitov, P. I., Mulvey, G., Griener, T. P., James, M. N., et al. (2014). The crystal structure of shiga toxin type 2 with bound disaccharide guides the design of a heterobifunctional toxin inhibitor. J. Biol. Chem. 289, 885–894. doi: 10.1074/jbc.M113.518886
Jandhyala, D. M., Ahluwalia, A., Obrig, T., and Thorpe, C. M. (2008). ZAK: a MAP3Kinase that transduces Shiga toxin and ricin induced proinflammatory cytokine expression. Cell. Microbiol. 10, 1468–1477. doi: 10.1111/j.1462-5822.2008.01139.x
Kamani, M., Mylvaganam, M., Tian, R., Binnington, B., and Lingwood, C. (2011). Adamantyl glycosphingolipids provide a new approach to the selective regulation of cellular glycosphingolipid metabolism. J. Biol. Chem. 286, 21413–21426. doi: 10.1074/jbc.M110.207670
Karmali, M. A., Petric, M., Lim, C., Fleming, P. C., Arbus, G. S., and Lior, H. (1985). The association between idiopathic hemolytic uremic syndrome and infection by verotoxin-producing Escherichia coli. J. Infect. Dis. 151, 775–782. doi: 10.1093/infdis/151.5.775
Karpman, D., and Tati, R. (2016). Complement contributes to the pathogenesis of Shiga toxin-associated hemolytic uremic syndrome. Kidney Int. 90, 726–729. doi: 10.1016/j.kint.2016.07.002
Katagiri, Y., Mori, T., Nakajima, H., Katagiri, C., Taguchi, T., Takeda, T., et al. (1999). Activation of Src family kinase induced by Shiga toxin binding to globotriaosyl ceramide (Gb3/CD77) in low density, detergent-insoluble microdomains. J. Biol. Chem. 274, 35278–35282. doi: 10.1074/jbc.274.49.35278
Kawano, K., Okada, M., Haga, T., Maeda, K., and Goto, Y. (2008). Relationship between pathogenicity for humans and stx genotype in Shiga toxin-producing Escherichia coli serotype O157. Eur. J. Clin. Microbiol. Infect. Dis. 27, 227–232. doi: 10.1007/s10096-007-0420-3
Khan, F., Proulx, F., and Lingwood, C. A. (2009). Detergent-resistant globotriaosyl ceramide may define verotoxin/glomeruli-restricted hemolytic uremic syndrome pathology. Kidney Int. 75, 1209–1216. doi: 10.1038/ki.2009.7
Khine, A. A., and Lingwood, C. A. (1994). Capping and receptor mediated endocytosis of cell bound verotoxin(Shiga-like toxin) 1; chemical identification of an amino acid in the B subunit necessary for efficient receptor glycolipid binding and cellular internalization. J. Cell Physiol. 161, 319–332. doi: 10.1002/jcp.1041610217
Khine, A. A., Tam, P., Nutikka, A., and Lingwood, C. A. (2004). Brefeldin A and filipin distinguish two Globotriaosyl ceramide/ Verotoxin-1 intracellular trafficking pathways involved in Vero cell cytotoxicity. Glycobiology 14, 701–712. doi: 10.1093/glycob/cwh085
Kiarash, A., Boyd, B., and Lingwood, C. A. (1994). Glycosphingolipid receptor function is modified by fatty acid content: verotoxin 1 and verotoxin 2c preferentially recognize different globotriaosyl ceramide fatty acid homologues. J. Biol. Chem. 269, 11138–11146.
Kitov, P. I., Lipinski, T., Paszkiewicz, E., Solomon, D., Sadowska, J. M., Grant, G. A., et al (2008b) An entropically efficient supramolecular inhibition strategy for Shiga toxins. Angew. Chem. Int. Ed. Engl. 47, 672–676. doi: 10.1002/anie.200704064.
Kitov, P. I., Mulvey, G. L., Griener, T. P., Lipinski, T., Solomon, D., Paszkiewicz, E., et al. (2008a). In vivo supramolecular templating enhances the activity of multivalent ligands: a potential therapeutic against the Escherichia coli O157 AB5 toxins. Proc. Natl. Acad. Sci. U.S.A. 105, 16837–16842. doi: 10.1073/pnas.0804919105
Kitov, P. I., Sadowska, J. M., Mulvey, G., Armstrong, G. D., Lingaw, H., Pannu, N. S., et al. (2000). Shiga-like toxins are neutralized by tailored multivalent carbohydrate ligands. Nature 403, 669–672. doi: 10.1038/35001095
Konowalchuk, J., Speirs, J. I., and Stavric, S. (1977). Vero response to a cytotoxin of Escherichia coli. Infect. Immun. 18, 775–779. doi: 10.1128/IAI.18.3.775-779.1977
Kovbasnjuk, O., Mourtazina, R., Baibakov, B., Wang, T., Elowsky, C., Choti, M. A., et al. (2005). The glycosphingolipid globotriaosylceramide in the metastatic transformation of colon cancer. Proc. Natl. Acad. Sci. U.S.A. 102, 19087–19092. doi: 10.1073/pnas.0506474102
Kramer, J., Deppe, M., Gobel, K., Tabelow, K., Wiendl, H., and Meuth, S. G. (2015). Recovery of thalamic microstructural damage after Shiga toxin 2-associated hemolytic-uremic syndrome. J. Neurol. Sci. 356, 75–83. doi: 10.1016/j.jns.2015.06.045
Kuroda, S., Koh, M., Hori, E., Hayakawa, Y., and Akai, T. (2018). Muse cell: a new paradigm for cell therapy and regenerative homeostasis in ischemic stroke. Adv. Exp. Med. Biol. 1103, 187–198. doi: 10.1007/978-4-431-56847-6_10
Kuroda, Y., Kitada, M., Wakao, S., Nishikawa, K., Tanimura, Y., Makinoshima, H., et al. (2010). Unique multipotent cells in adult human mesenchymal cell populations. Proc. Natl. Acad. Sci. U.S.A. 107, 8639–8643. doi: 10.1073/pnas.0911647107
LaCasse, E. C., Bray, M. R., Patterson, B., Lim, W. M., Perampalam, S., Radvanyi, L. G., et al. (1999). Shiga-like toxin I receptor on human breast cancer, lymphoma, and myeloma and absence from CD34+ hematopoietic stem cells: implications for ex vivo tumor purging and autologous stem cell transplantation. Blood 94, 2901–2910.
Lafalla Manzano, A. F., Gil Lorenzo, A. F., Bocanegra, V., Costantino, V. V., Cacciamani, V., Benardon, M. E., et al. (2019). Rab7b participation on the TLR4 (Toll-like receptor) endocytic pathway in Shiga toxin-associated Hemolytic Uremic Syndrome (HUS). Cytokine 121:154732. doi: 10.1016/j.cyto.2019.05.019
Lauvrak, S. U., Walchli, S., Iversen, T. G., Slagsvold, H. H., Torgersen, M. L., Spilsberg, B., et al. (2005). Shiga toxin regulates its entry in a syk-dependent manner. Mol. Biol. Cell. 17, 1096–1109. doi: 10.1091/mbc.e05-08-0766
Lea, N., Lord, J. M., and Roberts, L. M. (1999). Proteolytic cleavage of the A subunit is essential for maximal cytotoxicity of Escherichia coli O157:H7 Shiga-like toxin-1. Microbiology 145, 999–1004. doi: 10.1099/13500872-145-5-999
Leffler, J., Prohaszka, Z., Mikes, B., Sinkovits, G., Ciacma, K., Farkas, P., et al. (2017). Decreased neutrophil extracellular trap degradation in shiga toxin-associated haemolytic uraemic syndrome. J. Innate. Immun. 9, 12–21. doi: 10.1159/000450609
Legros, N., Pohlentz, G., Runde, J., Dusny, S., Humpf, H. U., Karch, H., et al. (2017). Colocalization of receptors for Shiga toxins with lipid rafts in primary human renal glomerular endothelial cells and influence of D-PDMP on synthesis and distribution of glycosphingolipid receptors. Glycobiology 27, 947–965. doi: 10.1093/glycob/cwx048
Leng, Z., Sun, D., Huang, Z., Tadmori, I., Chiang, N., Kethidi, N., et al. (2019). Quantitative analysis of SSEA3+ cells from human umbilical cord after magnetic sorting. Cell Transplant. 28, 907–923. doi: 10.1177/0963689719844260
Leyva-Illades, D., Cherla, R. P., Lee, M. S., and Tesh, V. L. (2012). Regulation of cytokine and chemokine expression by the ribotoxic stress response elicited by Shiga toxin type 1 in human macrophage-like THP-1 cells. Infect. Immun. 80, 2109–2120. doi: 10.1128/IAI.06025-11
Ling, H., Boodhoo, A., Hazes, B., Cummings, M., Armstrong, G., Brunton, J., et al. (1998). Structure of the Shiga toxin B-pentamer complexed with an analogue of its receptor Gb3. Biochem 37, 1777–1788. doi: 10.1021/bi971806n
Lingwood, C., and Mylvaganam, M. (2003). “Lipid Modulation of Glycosphingolipid (GSL) receptors: novel soluble GSL mimics provide new probes of GSL receptor function,” in Meth Enzymol, Vol. 363, eds Y. C. Lee and R. T. Lee (San Diego, CA: Academic Press), 264–283. doi: 10.1016/S0076-6879(03)01057-7
Lingwood, C., Sadacharan, S., Abul-Milh, M., Mylvaganum, M., and Peter, M. (2006). Soluble adamantyl glycosphingolipid analogs as probes of glycosphingolipid function. Methods Mol. Biol. 347, 305–320. doi: 10.1385/1-59745-167-3:305
Lingwood, C. A. (1994). Verotoxin-binding in human renal sections. Nephron 66, 21–28. doi: 10.1159/000187761
Lingwood, C. A., Law, H., Richardson, S., Petric, M., Brunton, J. L., DeGrandis, S., et al. (1987). Glycolipid binding of purified and recombinant Escherichia coli-produced verotoxin in vitro. J. Biol. Chem. 262, 8834–8839.
Lingwood, D., Binnington, B., Róg, T., Vattulainen, I., Grzybek, M., Coskun, U., et al. (2011). Cholesterol modulates glycolipid conformation and receptor activity. Nat. Chem. Biol. 7, 260–262. doi: 10.1038/nchembio.551
Loos, S., Oh, J., and Kemper, M. J. (2018). Eculizumab in STEC-HUS: need for a proper randomized controlled trial. Pediatr. Nephrol. 33, 1277–1281. doi: 10.1007/s00467-018-3972-9
Lord, J. M., Deeks, E., Marsden, C. J., Moore, K., Pateman, C., Smith, D. C., et al. (2003). Retrograde transport of toxins across the endoplasmic reticulum membrane. Biochem. Soc. Trans. 31, 1260–1262. doi: 10.1042/bst0311260
Louise, C. B., and Obrig, T. G. (1991). Shiga toxin-associated hemolytic-uremic syndrome: combined cytotoxic effects of Shiga toxin, interleukin-1ß, and tumor necrosis factor alpha on human vascular endothelial cells in vitro. Infect. Immun. 59, 4173–4179. doi: 10.1128/IAI.59.11.4173-4179.1991
Mahfoud, R., Manis, A., Binnington, B., Ackerley, C., and Lingwood, C. A. (2010). A major fraction of glycosphingolipids in model and cellular cholesterol containing membranes are undetectable by their binding proteins. J. Biol. Chem. 285, 36049–36059. doi: 10.1074/jbc.M110.110189
Mamelak, D., and Lingwood, C. (2001). The ATPase domain of Hsp70 possesses a unique binding specificity for 3'Sulfogalactolipids. J. Biol. Chem. 276, 449–456. doi: 10.1074/jbc.M006732200
Mandrell, R. E., and Apicella, M. A. (1993). Lipo-oligosaccharides (LOS) of mucosal pathogens: molecular mimicry and host-modification of LOS. Immunobiology 187, 382–402. doi: 10.1016/S0171-2985(11)80352-9
Matsuoka, K., Nishikawa, K., Goshu, Y., Koyama, T., Hatano, K., Matsushita, T., et al. (2018). Synthetic construction of sugar-amino acid hybrid polymers involving globotriaose or lactose and evaluation of their biological activities against Shiga toxins produced by Escherichia coli O157:H7. Bioorg. Med. Chem. 26, 5792–5803. doi: 10.1016/j.bmc.2018.10.023
Miura, Y., Sakaki, A., Kamihira, M., Iijima, S., and Kobayashi, K. (2006). A globotriaosylceramide (Gb3Cer) mimic peptide isolated from phage display library expressed strong neutralization to Shiga toxins. Biochim. Biophys. Acta 1760, 883–889. doi: 10.1016/j.bbagen.2006.03.018
Miura, Y., Sasao, Y., Kamihira, M., Sakaki, A., Iijima, S., and Kobayashi, K. (2004). Peptides binding to a Gb3 mimic selected from a phage library. Biochim. Biophys. Acta 1673, 131–138. doi: 10.1016/j.bbagen.2004.04.009
Miyamoto, Y., Iimura, M., Kaper, J. B., Torres, A. G., and Kagnoff, M. F. (2006). Role of Shiga toxin versus H7 flagellin in enterohaemorrhagic Escherichia coli signalling of human colon epithelium in vivo. Cell. Microbiol. 8, 869–879. doi: 10.1111/j.1462-5822.2005.00673.x
Mizuguchi, M., Sugatani, J., Maeda, T., Momoi, T., Arima, K., Takashima, S., et al. (2001). Cerebrovascular damage in young rabbits after intravenous administration of Shiga toxin 2. Acta Neuropathol. 102, 306–312. doi: 10.1007/s004010100384
Monet-Didailler, C., Chevallier, A., Godron-Dubrasquet, A., Allard, L., Delmas, Y., Contin-Bordes, C., et al. (2019). Outcome of children with Shiga toxin-associated haemolytic uraemic syndrome treated with eculizumab: a matched cohort study. Nephrol. Dial Transplant. doi: 10.1093/ndt/gfz158. [Epub ahead of print].
Mori, T., Kiyokawa, N., Katagiri, Y. U., Taguchi, T., Suzuki, T., Sekino, T., et al. (2000). Globotriaosyl ceramide (CD77/Gb3) in the glycolipid-enriched membrane domain participates in B-cell receptor-mediated apoptosis by regulating lyn kinase activity in human B cells. Exp. Hematol. 28, 1260–1268. doi: 10.1016/S0301-472X(00)00538-5
Morigi, M., Galbusera, M., Gastoldi, S., Locatelli, M., Buelli, S., Pezzotta, A., et al. (2011). Alternative pathway activation of complement by Shiga toxin promotes exuberant C3a formation that triggers microvascular thrombosis. J. Immunol. 187, 172–180. doi: 10.4049/jimmunol.1100491
Mulvey, G. L., Marcato, P., Kitov, P. I., Sadowska, J., Bundle, D. R., and Armstrong, G. D. (2003). Assessment in mice of the therapeutic potential of tailored, multivalent Shiga toxin carbohydrate ligands. J. Infect. Dis. 187, 640–649. doi: 10.1086/373996
Mylvaganam, M., Binnington, B., Budani, M., Soltyk, A. M., and Lingwood, C. A. (2015). The dramatic modulatory role of the 2'N substitution of the terminal amino hexose of globotetraosylceramide in determining binding by members of the verotoxin family. Chromatography 2, 529–544. doi: 10.3390/chromatography2030529
Mylvaganam, M., and Lingwood, C. (1999). Adamantyl globotriaosyl ceramide- a monovalent soluble glycolipid mimic which inhibits verotoxin binding to its glycolipid receptor. Biochem. Biophys. Res. Commun. 257, 391–394. doi: 10.1006/bbrc.1999.0474
Nakajima, H., Kiyokawa, N., Katagiri, Y. U., Taguchi, T., Suzuki, T., Sekino, T., et al. (2001). Kinetic analysis of binding between Shiga toxin and receptor glycolipid Gb3Cer by surface plasmon resonance. J. Biol. Chem. 276, 42915–42922. doi: 10.1074/jbc.M106015200
Neri, P., Nagano, S. I., Yokoyama, S., Dohi, H., Kobayashi, K., Miura, T., et al. (2007a). Neutralizing activity of polyvalent Gb3, Gb2 and galacto-trehalose models against Shiga toxins. Microbiol. Immunol. 51, 581–592. doi: 10.1111/j.1348-0421.2007.tb03944.x
Neri, P., Tokoro, S., Yokoyama, S., Miura, T., Murata, T., Nishida, Y., et al. (2007b). Monovalent Gb3-/Gb2-derivatives conjugated with a phosphatidyl residue: a novel class of Shiga toxin-neutralizing agent. Biol. Pharm. Bull. 30, 1697–1701. doi: 10.1248/bpb.30.1697
Nielsen, H. E., Truedsson, L., and Donner, M. (1994). Partial complement deficiencies in idiopathic thrombocytopenia of childhood. Acta Paediatr. 83, 749–753. doi: 10.1111/j.1651-2227.1994.tb13132.x
Nishikawa, K., Matsuoka, K., Watanabe, M., Igai, K., Hino, K., Hatano, K., et al. (2005). Identification of the optimal structure required for a shiga toxin neutralizer with oriented carbohydrates to function in the circulation. J. Infect. Dis. 191, 2097–2105. doi: 10.1086/430388
Nishikawa, K., Watanabe, M., Kita, E., Igai, K., Omata, K., Yaffe, M. B., et al. (2006). A multivalent peptide library approach identifies a novel Shiga toxin inhibitor that induces aberrant cellular transport of the toxin. FASEB. J. 20, 2597–2599. doi: 10.1096/fj.06-6572fje
Nitobe, Y., Nagaoki, T., Kumagai, G., Sasaki, A., Liu, X., Fujita, T., et al. (2019). Neurotrophic factor secretion and neural differentiation potential of multilineage-differentiating stress-enduring (muse) cells derived from mouse adipose tissue. Cell Transplant. 28, 1132–1139. doi: 10.1177/0963689719863809
Nyholm, P.-G., and Pascher, I. (1993). Orientation of the saccharide chains of glycolipids at the membrane surface: conformational analysis of the glucose-ceramide and the glucose-glyceride linkages using molecular mechanics (MM3). Biochemistry 32, 1225–1234. doi: 10.1021/bi00056a005
Nyholm, P.-G., Samuelsson, B. E., Breimer, M., and Pascher, I. (1989). Conformational analysis of blood group A-active glycosphingolipids using HSEA-calculations. The possible significance of the core oligosaccharide chain for the presentation and recognition of the A-determinant. J. Mol. Recognit. 2, 103–113. doi: 10.1002/jmr.300020302
Nyholm, P. G., Magnusson, G., Zheng, Z., Norel, R., Binnington-Boyd, B., and Lingwood, C. A. (1996). Two distinct binding sites for globotriaosyl ceramide on verotoxins: molecular modelling and confirmation by analogue studies and a new glycolipid receptor for all verotoxins. Chem. Biol. 3, 263–275. doi: 10.1016/S1074-5521(96)90106-4
Ozuru, R., Wakao, S., Tsuji, T., Ohara, N., Matsuba, T., Amuran, M. Y., et al. (2019). Rescue from Stx2-Producing E. coli-Associated encephalopathy by intravenous injection of muse cells in NOD-SCID mice. Mol. Ther. 28, 100–118. doi: 10.1016/j.ymthe.2019.09.023
Park, H. J., McPherson, A., Fewell, S., Brodsky, J., and Lingwood, C. A. (2009). A soluble sulfogalactosyl ceramide mimic promotes ΔF508 CFTR escape from endoplasmic reticulum associated degradation (ERAD). Chem. Biol. 16, 461–470. doi: 10.1016/j.chembiol.2009.02.014
Paton, A. W., Chen, A. Y., Wang, H., McAllister, L. J., Hoggerl, F., Mayr, U. B., et al. (2015). Protection against shiga-toxigenic escherichia coli by non-genetically modified organism receptor mimic bacterial ghosts. Infect. Immun. 83, 3526–3533. doi: 10.1128/IAI.00669-15
Paton, A. W., Morona, R., and Paton, J. C. (2000). A new biological agent for treatment of Shiga toxigenic Escherichia coli infections and dysentery in humans. Nat. Med. 6, 265–270. doi: 10.1038/73111
Paton, A. W., Morona, R., and Paton, J. C. (2001). Neutralization of Shiga toxins stx1, stx2c, and stx2e by recombinant bacteria expressing mimics of globotriose and globotetraose. Infect. Immun. 69, 1967–1970. doi: 10.1128/IAI.69.3.1967-1970.2001
Paton, J. C., Rogers, T. J., Morona, R., and Paton, A. W. (2001). Oral administration of formaldehyde-killed recombinant bacteria expressing a mimic of the Shiga toxin receptor protects mice from fatal challenge with Shiga-toxigenic Escherichia coli. Infect. Immun. 69, 1389–1393. doi: 10.1128/IAI.69.3.1389-1393.2001
Pecoraro, C., Ferretti, A. V., Rurali, E., Galbusera, M., Noris, M., and Remuzzi, G. (2015). Treatment of congenital thrombotic thrombocytopenic purpura with eculizumab. Am. J. Kidney Dis. 66, 1067–1070. doi: 10.1053/j.ajkd.2015.06.032
Pellizzari, A., Pang, H., and Lingwood, C. A. (1992). Binding of verocytotoxin 1 to its receptor is influenced by differences in receptor fatty acid content. Biochemistry 31, 1363–1370. doi: 10.1021/bi00120a011
Percheron, L., Gramada, R., Tellier, S., Salomon, R., Harambat, J., Llanas, B., et al. (2018). Eculizumab treatment in severe pediatric STEC-HUS: a multicenter retrospective study. Pediatr. Nephrol. 33, 1385–1394. doi: 10.1007/s00467-018-3903-9
Peter, M., and Lingwood, C. (2000). Apparent cooperativity in multivalent verotoxin globotriaosyl ceramide binding: kinetic and saturation binding experiments with radiolabelled verotoxin [125I]-VT1. Biochim. Biophys Acta. 1501, 116–124. doi: 10.1016/S0925-4439(00)00011-9
Pezeshkian, W., Chaban, V. V., Johannes, L., Shillcock, J., Ipsen, J. H., and Khandelia, H. (2015). The effects of globotriaosylceramide tail saturation level on bilayer phases. Soft. Matter. 11, 1352–1361. doi: 10.1039/C4SM02456G
Pezeshkian, W., Gao, H., Arumugam, S., Becken, U., Bassereau, P., Florent, J. C., et al. (2017). Mechanism of shiga toxin clustering on membranes. ACS Nano 11, 314–324. doi: 10.1021/acsnano.6b05706
Ramos, M. V., Mejias, M. P., Sabbione, F., Fernandez-Brando, R. J., Santiago, A. P., Amaral, M. M., et al. (2016). Induction of neutrophil extracellular traps in shiga toxin-associated hemolytic uremic syndrome. J. Innate. Immun. 8, 400–411. doi: 10.1159/000445770
Rasa, M., Musgrave, J., Abe, K., Tanaka, L., Xoinis, K., Shiramizu, B., et al. (2017). A case of escherichia coli hemolytic uremic syndrome in a 10-year-old male with severe neurologic involvement successfully treated with eculizumab. J. Investig. Med. High Impact. Case Rep. 5:2324709617741144. doi: 10.1177/2324709617741144
Renard, H. F., Garcia-Castillo, M. D., Chambon, V., Lamaze, C., and Johannes, L. (2015). Shiga toxin stimulates clathrin-independent endocytosis of VAMP2/3/8 SNARE proteins. J. Cell Sci. 128, 2891–2902. doi: 10.1242/jcs.171116
Roche, J. K., Keepers, T. R., Gross, L. K., Seaner, R. M., and Obrig, T. G. (2007). CXCL1/KC and CXCL2/MIP-2 are critical effectors and potential targets for therapy of Escherichia coli O157:H7-associated renal inflammation. Am. J. pathol. 170, 526–537. doi: 10.2353/ajpath.2007.060366
Römer, W., Berland, L., Chambon, V., Gaus, K., Windschiegl, B., Tenza, D., et al. (2007). Shiga toxin induces tubular membrane invaginations for its uptake into cells. Nature 450, 670–675. doi: 10.1038/nature05996
Römer, W., Pontani, L. L., Sorre, B., Rentero, C., Berland, L., Chambon, V., et al. (2010). Actin dynamics drive membrane reorganization and scission in clathrin-independent endocytosis. Cell 140, 540–553. doi: 10.1016/j.cell.2010.01.010
Rydell, G. E., Renard, H. F., Garcia-Castillo, M. D., Dingli, F., Loew, D., Lamaze, C., et al. (2014). Rab12 localizes to Shiga toxin-induced plasma membrane invaginations and controls toxin transport. Traffic 15, 772–787. doi: 10.1111/tra.12173
Saito, M., Mylvaganam, M., Tam, P., Binnington, B., and Lingwood, C. (2012). Structure-dependent pseudo-receptor intracellular traffic of adamantyl globotriaosyl ceramide mimics. J. Biol. Chem. 287, 16073–16087. doi: 10.1074/jbc.M111.318196
Sandvig, K., Olnes, S., Brown, J., Peterson, O., and van Deurs, B. (1989). Endocytosis from coated pits of Shiga toxin: a glycolipid-binding protein from Shigella dysenteriae 1. J. Cell Biol. 108, 1331–1343. doi: 10.1083/jcb.108.4.1331
Siegler, R., Pysher, T., Tesh, V., and Taylor, F. (2001). Response to single and divided doses of Shiga toxin-1 in a primate model of hemolytic uremic syndrome. J. Am. Soc. Nephrol. 12, 1458–1467.
Siegler, R. L., Obrig, T. G., Pysher, T. J., Tesh, V. L., Denkers, N. D., and Taylor, F. B. (2003). Response to Shiga toxin 1 and 2 in a baboon model of hemolytic uremic syndrome. Pediatr. Nephrol. 18, 92–96. doi: 10.1007/s00467-002-1035-7
Siegler, R. L., Pysher, T. J., Lou, R., Tesh, V. L., and Taylor, F. B. Jr. (2001). Response to Shiga toxin-1, with and without lipopolysaccharide, in a primate model of hemolytic uremic syndrome. Am. J. Nephrol. 21, 420–425. doi: 10.1159/000046288
Soltyk, A. M., MacKenzie, C. R., Wolski, V. M., Hirama, T., Kitov, P. I., Bundle, D. R., et al. (2002). A mutational analysis of the Globotriaosylceramide binding sites of Verotoxin VT1. J. Biol. Chem. 277, 5351–5359. doi: 10.1074/jbc.M107472200
Spooner, R. A., and Lord, J. M. (2012). How ricin and Shiga toxin reach the cytosol of target cells: retrotranslocation from the endoplasmic reticulum. Curr. Top. Microbiol. Immunol. 357, 19–40. doi: 10.1007/82_2011_154
Stearns-Kurosawa, D. J., Collins, V., Freeman, S., Tesh, V. L., and Kurosawa, S. (2010). Distinct physiologic and inflammatory responses elicited in baboons after challenge with Shiga toxin type 1 or 2 from enterohemorrhagic Escherichia coli. Infect. Immun. 78, 2497–2504. doi: 10.1128/IAI.01435-09
Stein, P. E., Boodhoo, A., Tyrrell, G. J., Brunton, J. L., and Read, R. J. (1992). Crystal structure of the cell-binding B oligomer of verotoxin-1 from E. coli. Nature 355, 748–750. doi: 10.1038/355748a0
St. Hilaire, P. M., Boyd, M. K., and Toone, E. J. (1994). Interaction of the Shiga-like toxin type 1 B-subunit with its carbohydrate receptor. Biochemistry 33, 14452–14463. doi: 10.1021/bi00252a011
Stimmer, L., Dehay, S., Nemati, F., Massonnet, G., Richon, S., Decaudin, D., et al. (2014). Human breast cancer and lymph node metastases express Gb3 and can be targeted by STxB-vectorized chemotherapeutic compounds. BMC Cancer 14:916. doi: 10.1186/1471-2407-14-916
Stults, C. L. M., Sweeley, C. H., and Macher, B. A. (1989). Glycosphingolipids: structure biological source and properties. Methods Enzymol. 179, 167–214. doi: 10.1016/0076-6879(89)79122-9
Takahashi, K., Funata, N., Ikuta, F., and Sato, S. (2008). Neuronal apoptosis and inflammatory responses in the central nervous system of a rabbit treated with Shiga toxin-2. J. Neuroinflammation 5:11. doi: 10.1186/1742-2094-5-11
Takenaga, M., Igarashi, R., Higaki, M., Nakayama, T., Yuki, K., and Mizushima, Y. (2000). Effect of a soluble pseudo-receptor on verotoxin2-induced toxicity. J. Infect. Chemother. 6, 21–25. doi: 10.1007/s101560050044
Tam, P., and Lingwood, C. (2007). Membrane-cytosolic translocation of Verotoxin A1-subunit in target cells. Microbiology 153, 2700–2710. doi: 10.1099/mic.0.2007/006858-0
Tam, P., Mahfoud, R., Nutikka, A., Khine, A., Binnington, B., Paroutis, P., et al. (2008). Differential intracellular trafficking and binding of verotoxin 1 and verotoxin 2 to globotriaosylceramide-containing lipid assemblies. J. Cell Physiol. 216, 750–763. doi: 10.1002/jcp.21456
Tareke, E., Rydberg, P., Karlsson, P., Eriksson, S., and Tornqvist, M. (2002). Analysis of acrylamide, a carcinogen formed in heated foodstuffs. J. Agric. Food Chem. 50, 4998–5006. doi: 10.1021/jf020302f
Trachtman, H., Austin, C., Lewinski, M., and Stahl, R. A. (2012). Renal and neurological involvement in typical Shiga toxin-associated HUS. Nat. Rev. Nephrol. 8, 658–669. doi: 10.1038/nrneph.2012.196
Trachtman, H., Cnaan, A., Christen, E., Gibbs, K., Zhao, S., Acheson, D. W., et al. (2003). Effect of an oral Shiga toxin-binding agent on diarrhea-associated hemolytic uremic syndrome in children: a randomized controlled trial. JAMA 290, 1337–1344. doi: 10.1001/jama.290.10.1337
Utskarpen, A., Massol, R., van Deurs, B., Lauvrak, S. U., Kirchhausen, T., and Sandvig, K. (2010). Shiga toxin increases formation of clathrin-coated pits through Syk kinase. PLoS ONE 5:e10944. doi: 10.1371/journal.pone.0010944
van Setten, P. A., Monnens, L. A., Verstraten, R. G., van den Heuvel, L. P., and van Hinsbergh, V. W. (1996). Effects of verocytotoxin-1 on nonadherent human monocytes: binding characteristics, protein synthesis, and induction of cytokine release. Blood 88, 174–183. doi: 10.1182/blood.V88.1.174.174
Voorhees, P., Deignan, E., van Donselaar, E., Humphrey, J., Marks, M. S., Peters, P. J., et al. (1995). An acidic sequence within the cytoplasmic domain of furin functions as a determinant of trans-Golgi network localization and internalization from the cell surface. EMBO J. 14, 4961–4975. doi: 10.1002/j.1460-2075.1995.tb00179.x
Walsh, P. R., and Johnson, S. (2019). Eculizumab in the treatment of Shiga toxin haemolytic uraemic syndrome. Pediatr. Nephrol. 34, 1485–1492. doi: 10.1007/s00467-018-4025-0
Warnier, M., Römer, W., Geelen, J., Lesieur, J., Amessou, M., van den Heuvel, L., et al. (2006). Trafficking of Shiga toxin/Shiga-like toxin-1 in human glomerular microvascular endothelial cells and human mesangial cells. Kidney Int. 70, 2085–2091. doi: 10.1038/sj.ki.5001989
Watanabe, M., Igai, K., Matsuoka, K., Miyagawa, A., Watanabe, T., Yanoshita, R., et al. (2006). Structural analysis of the interaction between Shiga toxin B subunits and linear polymers bearing clustered globotriose residues. Infect. Immun. 74, 1984–1988. doi: 10.1128/IAI.74.3.1984-1988.2006
Watanabe, M., Matsuoka, K., Kita, E., Igai, K., Higashi, N., Miyagawa, A., et al. (2004). Oral therapeutic agents with highly clustered globotriose for treatment of Shiga toxigenic Escherichia coli infections. J. Infect. Dis. 189, 360–368. doi: 10.1086/381124
Watanabe-Takahashi, M., Sato, T., Dohi, T., Noguchi, N., Kano, F., Murata, M., et al. (2010). An orally applicable Shiga toxin neutralizer functions in the intestine to inhibit the intracellular transport of the toxin. Infect. Immun. 78, 177–183. doi: 10.1128/IAI.01022-09
Whetstone, D., and Lingwood, C. (2003). 3'Sulfogalactolipid binding specifically inhibits Hsp70 ATPase activity in vitro. Biochemistry 42, 1611–1617. doi: 10.1021/bi026735t
Windschiegl, B., Orth, A., Römer, W., Berland, L., Stechmann, B., Bassereau, P., et al. (2009). Lipid reorganization induced by Shiga toxin clustering on planar membranes. PLoS ONE 4:ee6238. doi: 10.1371/journal.pone.0006238
Yahi, N., Aulas, A., and Fantini, J. (2010). How cholesterol constrains glycolipid conformation for optimal recognition of Alzheimer's beta amyloid peptide (Abeta1–40). PLoS ONE 5:e9079. doi: 10.1371/journal.pone.0009079
Yamada, S., Shimizu, M., Kuroda, M., Inoue, N., Sugimoto, N., and Yachie, A. (2019). Interleukin-33/ST2 signaling contributes to the severity of hemolytic uremic syndrome induced by enterohemorrhagic Escherichia coli. Clin. Exp. Nephrol. 23, 544–550. doi: 10.1007/s10157-018-1675-y
Yamasaki, C., Nishikawa, K., Zeng, X. T., Katayama, Y., Natori, Y., Komatsu, N., et al. (2004). Induction of cytokines by toxins that have an identical RNA N-glycosidase activity: shiga toxin, ricin, and modeccin. Biochim. Biophys. Acta 1671, 44–50. doi: 10.1016/j.bbagen.2004.01.002
Young, W. (2018). Future of Muse Cells. Adv. Exp. Med. Biol. 1103, 309–315. doi: 10.1007/978-4-431-56847-6_18
Yu, M., and Haslam, D. B. (2005). Shiga toxin is transported from the endoplasmic reticulum following interaction with the luminal chaperone HEDJ/ERdj3. Infect. Immun. 73, 2524–2532. doi: 10.1128/IAI.73.4.2524-2532.2005
Zhang, P., Paszkiewicz, E., Wangm, Q., Sadowska, J. M., Kitov, P. I., Bundle, D. R., et al. (2017). Clustering of P(K)-trisaccharides on amphiphilic cyclodextrin reveals unprecedented affinity for the Shiga-like toxin Stx2. Chem. Commun (Camb). 53, 10528–10531. doi: 10.1039/c7cc06299k
Zhang, W., Bielaszewska, M., Pulz, M., Becker, K., Friedrich, A. W., Karch, H., et al. (2008). New immuno-PCR assay for detection of low concentrations of shiga toxin 2 and its variants. J. Clin. Microbiol. 46, 1292–1297. doi: 10.1128/JCM.02271-07
Keywords: lipid raft, retrograde transport, endothelial cell, astrocyte, hemolytic uremic syndrome
Citation: Lingwood C (2020) Verotoxin Receptor-Based Pathology and Therapies. Front. Cell. Infect. Microbiol. 10:123. doi: 10.3389/fcimb.2020.00123
Received: 27 November 2019; Accepted: 05 March 2020;
Published: 31 March 2020.
Edited by:
Vernon L. Tesh, Texas A&M University, United StatesReviewed by:
Ludger Johannes, Maria Sklodowska Curie Institute of Oncology, PolandAngela Melton-Celsa, Uniformed Services University, United States
Copyright © 2020 Lingwood. This is an open-access article distributed under the terms of the Creative Commons Attribution License (CC BY). The use, distribution or reproduction in other forums is permitted, provided the original author(s) and the copyright owner(s) are credited and that the original publication in this journal is cited, in accordance with accepted academic practice. No use, distribution or reproduction is permitted which does not comply with these terms.
*Correspondence: Clifford Lingwood, Y2xpbmcmI3gwMDA0MDtzaWNra2lkcy5jYQ==