- 1Key Laboratory of Parasite and Vector Biology, Chinese Center for Disease Control and Prevention, National Center for International Research on Tropical Diseases, WHO Collaborating Centre for Tropical Diseases, National Institute of Parasitic Diseases, MOH, Shanghai, China
- 2Drug Discovery and Design Center, Shanghai Institute of Materia Medica, Chinese Academy of Sciences, Shanghai, China
Echinococcosis is a serious helminthic zoonosis with a great impact on human health and livestock husbandry. However, the clinically used drugs (benzimidazoles) have a low cure rate, so alternative drugs are urgently needed. Currently, drug screenings for echinococcosis are mainly phenotype-based, and the efficiency of identifying active compounds is very low. With a pharmacophore model generated from the structures of active amino alcohols, we performed a virtual screening to discover novel compounds with anti-echinococcal activity. Sixty-two compounds from the virtual screening were tested on Echinococcus multilocularis protoscoleces, and 10 of these compounds were found to be active. After further evaluation of their cytotoxicity, S6 was selected along with two active amino alcohols for in vivo pharmacodynamic and pharmacokinetic studies. At the two tested doses (50 and 25 mg/kg), S6 inhibited the growth of E. multilocularis in mice (14.43 and 9.53%), but no significant difference between the treatment groups and control group was observed. Treatment with BTB4 and HT3 was shown to be ineffective. During the 28 days of treatment, the death of mice in the mebendazole, HT3, and BTB4 groups indicated their toxicity. The plasma concentration of S6 administered by both methods was very low, with the Cmax being only 1 ng/ml after oral administration and below the detection limit after intramuscular administration. In addition, the plasma concentrations of BTB4 and HT3 in vitro did not reach high enough levels to kill the parasites. The toxicities of these two amino alcohols indicated that they are not suitable for further development as anti-echinococcal drugs. However, further attempts should be made to increase the bioavailability of S6 and modify its structure. In this study, we demonstrate that pharmacophore-based virtual screenings with high drug identification efficiency could be used to find novel drugs for treating echinococcosis.
Introduction
Echinococcus granulosus and E. multilocularis are the most important Echinococcus species currently affecting humans; in the larval stages, these species cause cystic echinococcosis (CE) and alveolar echinococcosis (AE), respectively (Eckert et al., 2001). If the eggs in the feces of definitive host (dogs or foxes) are ingested by humans, the metacestode cysts can asexually proliferate mainly in the liver and lungs (Moro and Schantz, 2009). CE occurs worldwide, while AE is confined to the Northern Hemisphere (WHO/Department of Control of Neglected Tropical Diseases, 2013). The growth of these parasites in patients is slow, and until the parasites grow to an extent that triggers clinical signs, which takes many years, their growth remains asymptomatic (Moro and Schantz, 2009). The drugs currently available in clinical settings are primarily limited to benzimidazoles (BMZ), albendazole and mebendazole, and the chemotherapy usually lasts 3 to 6 months but can last even longer (Lacey, 1990; Hemphill and Muller, 2009). Unfortunately, the efficacy of these drugs is only ~30%, with more than 40% of patients showing side effects (such as headache and abnormal liver function; Davis et al., 1986, 1989; Eckert et al., 1995). No other medications to treat echinococcosis have been approved in the last 30 years; hence, it is essential to find alternative chemotherapy strategies for treating this disease.
The development of drugs for echinococcosis, as a neglected disease, is of very limited interest to the pharmaceutical industry. Hence, none of the candidate alternative drugs or compounds tested against in vitro or in vivo models of Echinococcus spp. were first designed for the treatment of CE and AE (Siles-Lucas et al., 2018). For decades, most studies have focused on BMZ and new formulations to improve solubility or on the repurposing of clinical drugs (antitumour, antiviral, and antibiotic drugs; Siles-Lucas et al., 2018). Among the developed compounds, a limited number of drugs (isoprinosine Sarciron et al., 1992, mefloquine Rufener et al., 2018, tamoxifen Nicolao et al., 2014, etc.) have been shown to be effective in infected animal models. All the drug studies mentioned above are based on phenotypic screenings, which depend on the experience of the researchers. Hence, this screening method is essentially subjective and time consuming, resulting in low screening efficiencies. Recently, algorithms and imaging systems have been developed to measure the effects of drugs on the activities of E. multilocularis protoscoleces (PSCs). This advance has greatly increased screening efficiencies and reduced the requirement for experienced researchers; thus, it is considered an ideal screening method (Ritler et al., 2017). However, this improved test method has not substantially change the anti-echinococcal drug development pathway. The selection of candidate drugs for screening is limited and blind, and the structures of effective drugs and compounds are not fully exploited, resulting in inefficient use of research time and data.
Computational techniques have become the most effective methods in drug discovery and development. Among such methods, homology modeling, molecular docking, pharmacophore modeling and structure-based virtual screenings have been successfully applied in drug discovery. The virtual screening of a large number of compounds can identify the best molecular structure for combination with a specific biological target. In addition, analysis of structure-activity relationships (SARs) is the basis of designing new compounds, making structural modifications and predicting unknown active compounds (Shah et al., 2015; Ganesan and Barakat, 2017). For example, to find novel and potent anticancer agents, structure-based pharmacophore models based on promising cancer therapy drug targets (such as matrix metalloproteinase-2, histone deacetylase-6, protein kinase B-beta, and glycogen synthase kinase-3) have been developed and validated. Many compounds identified in virtual screenings have shown potential efficacies, and various chemical scaffolds have been proposed as potential leads for the further development of new anticancer agents (Crisan et al., 2017; Akhtar et al., 2018). Moreover, computer-aided drug design has been applied to the discovery of anti-parasitic drugs. Using pharmacophore-based virtual screening and molecular dynamics and molecular docking approaches, promising drug targets and hits are identified (Pavadai et al., 2016; Vyas et al., 2016; Kagami et al., 2017; Araujo et al., 2018). Among the predicted active compounds, some were shown to have anti-malarial activities in vitro and in vivo (Pavadai et al., 2016; Vyas et al., 2016). Leucyl-tRNA synthetase and pteridine reductase of trypanosomatid parasites were used as potential targets for screening novel anti-trypanosomatid compounds, and new potential inhibitory scaffolds were predicted; however, further validation is needed (Dube et al., 2012; Zhao et al., 2012). The development of a pharmacophore model for ligands of S. mansoni purine nucleoside phosphorylase (SmPNP) as well as a pharmacophore-based virtual screening approach led to the identification of three thioxothiazolidinones with substantial in vitro inhibitory activity against SmPNP (Postigo et al., 2010). However, there have been no reports of applying these methods in drug discovery for the treatment of echinococcosis.
In our previous study, we reported a series of amino alcohols with potential anti-echinococcal effects (Liu et al., 2018). The aim of this study was to screen potential compounds by pharmacophore models based on the structure of these active amino alcohols. The ligand- and structure-based pharmacophore models were developed to identify compounds with potential anti-echinococcal activity. HipHop-Hypo03 was selected as the most promising hypothesis, as it includes one positive ionizable (PI) moiety, one hydrophobic aromatic (HAR) moiety, one hydrogen bond donor (HC) and two hydrophobic aliphatic (HAL) moieties. Subsequently, these pharmacophore hypotheses were used in the virtual screening of ZINC databases to identify potential active compounds. The screened compounds were then tested on E. multilocularis PSCs in vitro. The in vivo efficacy and pharmacokinetics of one of the screened compounds together with two amino alcohols were determined.
Materials and Methods
Pharmacophore Model
Data Collection and Preparation
Based on our previous research results (Liu et al., 2018), six structurally diverse amino alcohols active against E. granulosus PSCs and germinal cells at 20 μg/ml were used as the training set to generate common feature-based pharmacophore models. The molecular properties of these compounds are listed in Table 1. Thirty-four amino alcohols were selected as the test set, among which 17 had anti-echinococcal effects (labeled with A+ID numbers) and 17 were inactive (labeled with ID numbers). All the molecules were prepared and optimized using Discovery Studio (DS) v4.1.
Pharmacophore Model Generation
HipHop pharmacophore models were generated from a set of six active amino alcohols with promising anti-echinococcal activities (Table 1). The principal value 2 was set for all the ligands and the maximum-omit feature was set to 0. Before building the HipHop pharmacophore model, the pharmacophore module “Feature Mapping” was used to identify the important chemical features of the training set compounds. Then, hydrogen bond acceptor (HBA), hydrogen bond donor (HBD), lipid hydrogen bond acceptor (LHBA), hydrophobic feature (HC), hydrophobic aliphatic (HAL), hydrophobic aromatic (HAR), positive ionizable (PI) and aromatic ring (AR) were selected as the building blocks for generating the pharmacophore model. To obtain diverse conformations, 255 conformations within a 20 kcal/mol energy threshold were generated using the “BEST” function. The final pharmacophore models with key common chemical features were ranked by fit value and are listed in Table 2.
Validating the Pharmacophore Model
The hypothesis of about pharmacophore is validated based on the fit results of obtained with test sets. The test sets (Supplementary Figure 1), including active compounds and inactive compounds, were prepared using the same protocol as that for the training set. Then, the “Ligand Profiler module” was used to determine whether the hypothesis was able to identifiy the active compounds according to the Fit Value.
Pharmacophore-Based Virtual Screening
The validated pharmacophore model HipHop-Hypo03 was used to screen compounds. Approximately 40,000 small molecules were obtained from the ZINC database (www.zinc.docking.org) and subjected to virtual screening. All these compounds were optimized in DS2.5, and HipHop-Hypo03 was used to screen compounds by using the Screen Library module of DS2.5. The number of conformations was set to 255, while the conformation method was set to BEST. The remaining parameters were set to the default values. Lipinski's Rule of Five and Veber's drug-likeness rule (Lipinski et al., 2001; Veber et al., 2002) were applied for the subsequent phase of screening. Then, the compounds with good Fit Value were purchased and validated by determining their in vivo and in vitro effects on E. multilocularis.
Chemicals and Reagents
The screening compounds were purchased from Enamine Ltd. (Kievska region, Ukraine) and had >90% purity. All culture media and reagents were purchased from Gibco-BRL (Zurich, Switzerland); mebendazole and other reagents were from Sigma (St. Louis, MO, USA). The CCK-8 kit was purchased from Dojindo (Tokyo, Japan). Stock solutions of all the compounds for in vitro drug screenings were prepared in DMSO at 20 mM, and on the day of treatment, the working solutions were prepared by freshly diluting the stock solution with DMSO to the appropriate concentration. For oral administration and pharmacokinetic analysis, all the compounds were suspended in 1% tragacanth solution.
Parasites and Animals
E. multilocularis-infected animals [BALB/c mice (SLAC Laboratory Animal Center)] were maintained by serial i.p. transplantation passages through for 6 months, the details can be found in our published paper (Liu et al., 2019). Eighty infected BALB/c mice were prepared for in vivo treatment experiments. Parasite materials were isolated from the peritoneal cavity of the infected animals and homogenized with Hanks' balanced salt solution (HBSS). Then, after passing the parasite homogenate through a 60-mesh sieve, the E. multilocularis PSCs were collected and rinsed 5~8 times. The viability of the PSCs was determined by the methylene blue exclusion method (Liu et al., 2018), and PSCs with > 95% viability were used in the following experiments. The PSCs were cultured in RPMI 1,640 medium supplemented with 10% CS and antibiotics (100 U/ml penicillin G and 100 μg/ml streptomycin) at 37°C in 5% CO2.
Ethics Statement
Animal care and all animal procedures were carried out in compliance with the Guidelines for the Care and Use of Laboratory Animals produced by the Shanghai Veterinary Research Institute. The study was approved by the Ethics Committee of the National Institute of Parasitic Diseases, Chinese Center for Disease Control and Prevention. The license number was IPD-2014-2.
In vitro Drug Screen With E. multilocularis Protoscoleces
In vitro treatment of PSCs was performed in 96-well microtiter plates (Costar, USA) containing 100 PSCs per 200 μl. A solution of the test compound (6.25~100 μM) was added to each well. Nitazoxanide and mebendazole (25 μM) were used as positive controls, while RPMI 1640 medium and RPMI 1640 with 0.25% DMSO were used as negative controls. The plates were incubated under the culture conditions for 72 h, and then the viability of the PSC was tested by the methylene blue exclusion method (Liu et al., 2018). All experiments were carried out in triplicate and repeated at least twice. The LC50 values were calculated by the probability unit method with SPSS version 17.0.
Cytotoxicity Test
Cytotoxicity was determined using Chang liver cells and A172 cells in DMEM with 10% FBS as previously described (Liu et al., 2018). The final concentrations of the test compounds were 3.125~50 μM for A172 cells and 6.25~100 μM for Chang liver cells. After 72 h, the cell viabilities were determined using a CCK-8 kit. The concentration that induced 50% cell toxicity (Tox50) was then calculated.
Compound Efficacy in E. multilocularis-Infected Mice
Eighty infected BALB/c mice were randomly divided into eight groups (n = 10 per group) and administered one screened compound (S6) and two amino alcohols (BTB4 and HT3) at 25 and 50 mg/kg/day (S6/BTB4/HT3-25 and S6/BTB4/HT3-50 by oral gavage, the dosage corresponded to that of mebendazole) and mebendazole (MBZ, the most effective drug for the treatment of infected animals) at 25 mg/kg/day (MBZ-25) for 28 consecutive days. The remaining mice were orally administered 1% tragacanth solution (1% TRA) as a control. All of the mice were euthanized 2 weeks after finishing the treatment, and all of the cysts were isolated and weighed. The efficacy of the treatments was assessed based on the mean cyst weight as previously described (Liu et al., 2015).
Pharmacokinetics Analysis
To study the relationship between pharmacokinetic characteristics and treatment efficacy in vivo, compounds were administered by oral (50 mg/kg) and intramuscular (12.5 mg/kg) methods to healthy BALB/c mice (22~22 g). Subgroups of 3 mice each were bled at 0.25, 0.5, 1, 2, 4, 8, 16, 24, 32, and 48 h post-administration. Then, 300 μl samples of plasma were collected and centrifuged at 2,500 g for 15 min. The compounds in the plasma were extracted on Oasis HLB cartridges (Waters, Massachusetts, USA) with praziquantel as the internal standard. The HPLC-HRMS system used for separation and analysis consisted of an Accela 1250 pump, a PAL HTC autosampler, an Accela PDA detector and an Xcalibur data system (Thermo Fisher Scientific, Waltham, MA, USA). Compounds were separated on a 3-μm Waters Atlantis 2.1 mm × 100 mm column (Massachusetts, USA) with a mobile phase containing methanol and formic acid at a flow rate of 0.35 ml/min. The data were analyzed by the non-compartmental model present in DAS 2.0 (Drug Analysis System, Shanghai University of T.C.M, China). Detailed pharmacokinetic experimental methods, including a representative HPLC-MS chromatogram, a standard curve, and a plot used to confirm precision, are shown in the Supplementary Data.
Data Analysis
The LC50 and Tox50 values were calculated by the probability unit method with SPSS version 17.0. The differences in mean cyst weight were analyzed by ANOVA in SPSS 17.0, and P < 0.05 was considered statistically significant.
Results
Pharmacophore Model Generation, Validation, and Virtual Screening
After the 10 HipHop models were generated, a test set composed of active and inactive amino alcohols was used to select the best model (Figure 1C). The pharmacophore model HipHop-Hypo03 was considered the best chemical hypothesis because the model was better at distinguishing active from inactive compounds. As shown in Figure 1B, the Fit Value of the 11 active compounds were above 3.0, and higher than the Fit Value of most of the inactive molecules, which were distributed in the blue and green areas. As shown in Figure 1B, this pharmacophore included one PI moiety, one HAR moiety, two HAL moieties and one HBD moiety (Figure 1A).
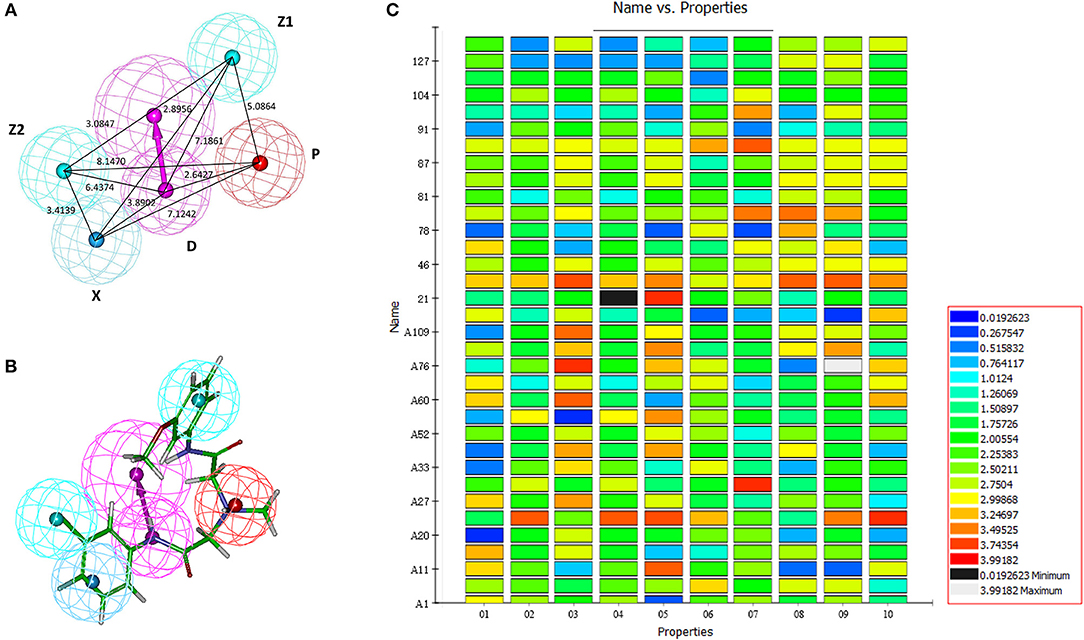
Figure 1. Generation of the HipHop pharmacophore. (A) The HipHop-Hypo03 chemical features. The color of the pharmacophore features, namely, PI, HAR, HAL and HBD, are red, blue, cyan, and magenta, respectively. (B) S6 fit to HipHop-Hypo03. (C) The heat map of the 10 hypotheses in the test.
After virtual screening based on this pharmacophore, the fitness scores of 176 compounds were >4.0. Among these compounds, 62 (Supplementary Table 1) were purchased, and their activities against parasites cultured in vitro were evaluated.
Evaluating the Activities of Candidate Compounds on E. multilocularis Protoscoleces in vitro and Their Cytotoxicities
The effects of the 62 compounds on E. multilocularis PSCs were evaluated, and at 100 μM, 10 of the 62 compounds resulted in 100% parasite death in 3 days. Next, solutions of these compounds at a series of concentrations were tested on E. multilocularis PSCs to determine their LC50 values. The LC50 values of the active compounds were 16.99~20.45 μM. As shown in Figure 2, the normal PSCs in DMSO had intact soma, and suckers and orderly arranged hooklets. Exposure to the compounds resulted in morphological damage to the parasites, including irregularities in their internal structure and the detachment of the hooklets from the rostellum. The E. multilocularis PSCs stained by methylene blue were dead (Figure 2).
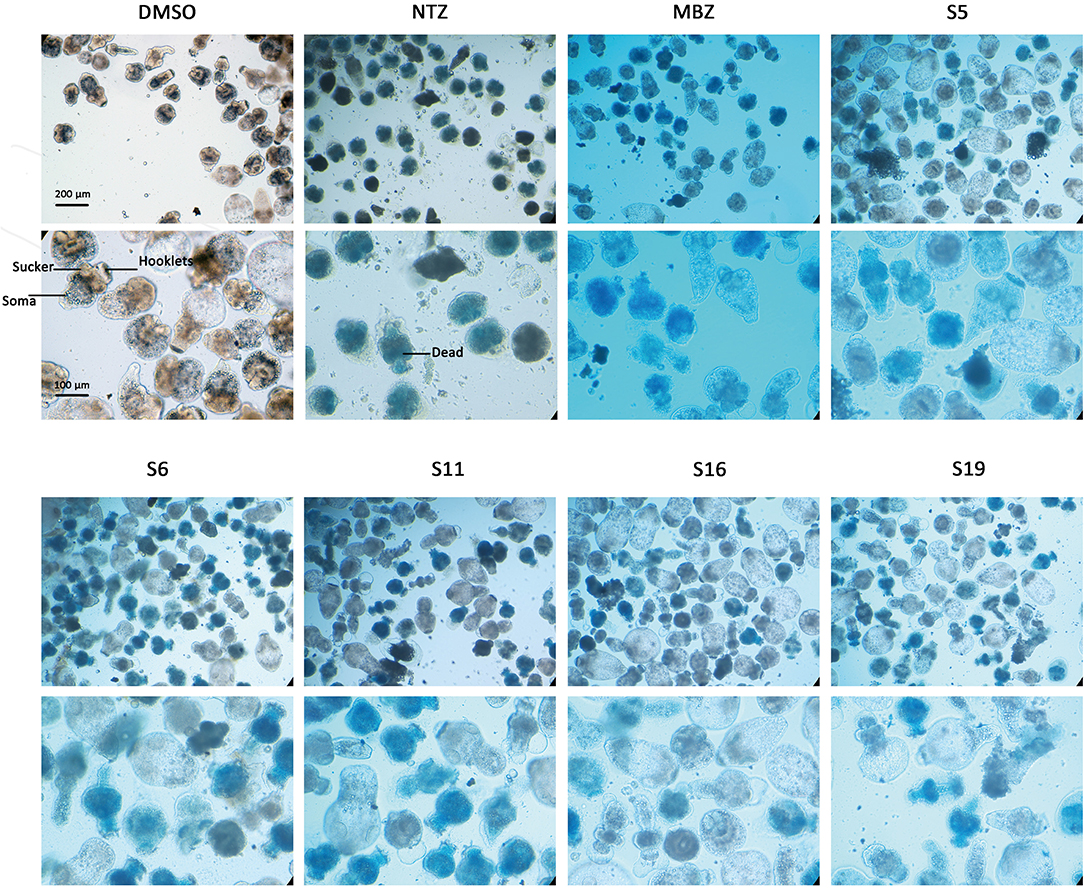
Figure 2. Morphology changes in Echinococcus multilocularis protoscoleces after incubation with the active compounds at 25 μM for 3 days. The normal protoscoleces in DMSO had intact soma, sucker and orderly arranged hooks. The drug treated parasites showed partial collapse and disruption of the inner structure, the detachment of hooks and staining with methylene blue.
The cytotoxicity of these compounds was also determined using a CCK-8 kit. The low cytotoxicities of S6, S11, S16, and S19 indicated good druggability. In addition, these active compounds (Table 3) were subjected to a cytotoxicity assay using the CCK-8 kit and A172 cells, because of the similarity of this parasite with cancer cells. The results showed that compounds S5, S6, S11, S16, S19, and S28 inhibited the proliferation of cancer cells (A172 cells).
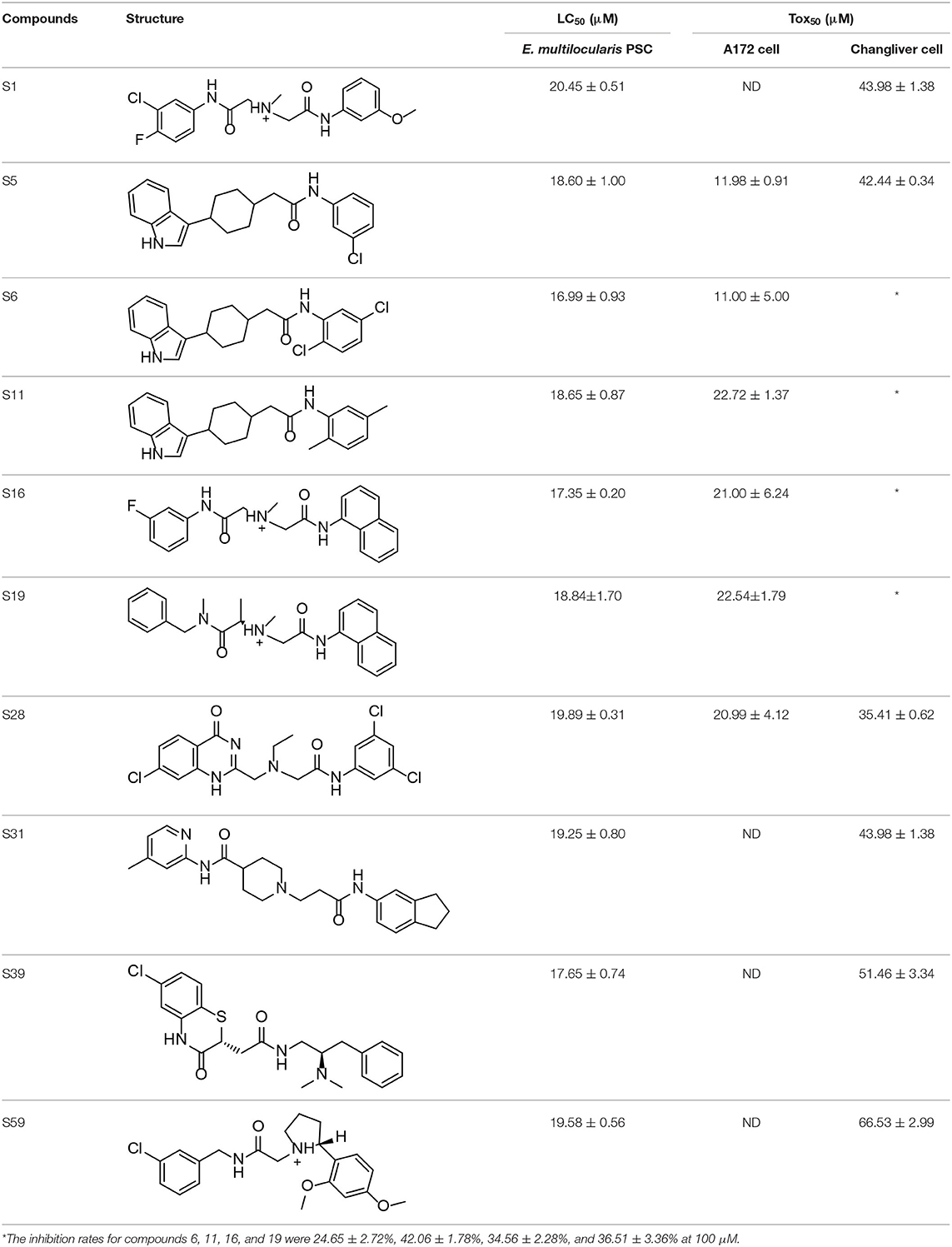
Table 3. In vitro effects of amino alcohols against E. multilocularis protoscoleces, cancer cells (A172 cells) and noncarcinogenic mammalian cells (Chang liver cells).
Considering its effect on E. multilocularis PSCs and its cytotoxicity to cancer cells and non-carcinogenic mammalian cells, compound S6 was selected for additional investigation.
In vivo Effects of the Candidate Compounds
We performed an in vivo pharmacodynamic evaluation of S6 and the previously reported active compounds, BTB4 and HT3 in mice infected with E. multilocularis. The inhibition rate of MBZ-25 was 39.66%, which was significantly lower than that of the control group (Figure 3). Although S6 at the two tested doses (50 and 25 mg/kg) inhibited the growth of E. multilocularis in mice (14.43 and 9.53%), there was no significant difference from the inhibition in the control group. Treatments with BTB4 and HT3 were confirmed to be ineffective in this study. In the 28-days treatment, MBZ-25, BTB4-50, BTB4-25, HT3-50, and HT3-25 resulted in the deaths of 1, 4, 2, 3, and 2 mice, respectively, while all the mice in the control and S6 groups remained alive during the experiment.
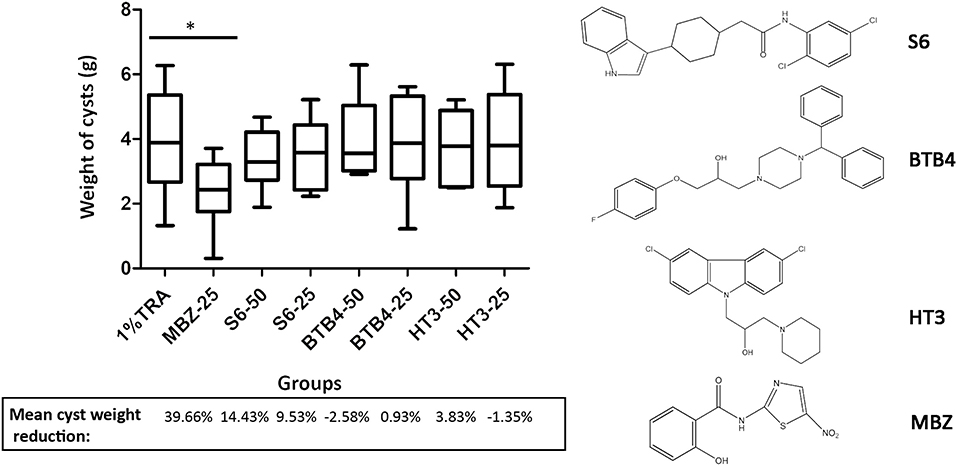
Figure 3. Treatment of mice secondarily infected with E. multilocularis. BALB/c mice were treated with S6, BTB4, and HT3 (25 mg/kg and 50 mg/kg), MBZ (25 mg/kg), or 1% TRA (control) for 28 days, n = 10. MBZ-25, BTB4-50, BTB4-25, HT3-50, and HT3-25 resulted in the deaths of 1, 4, 2, 3, and 2 mice, respectively. *p < 0.05.
Plasma Concentration and Pharmacokinetics of the Candidate Compounds in Mice
As depicted in Figure 4 and Table 4, the plasma concentrations of S6 in mice after administration by two methods were very low. The maximum concentration (Cmax) after oral administration was only 1 ng/ml, while the concentration after intramuscular administration was below the detection limit. After oral and intramuscular administration, the plasma concentration of BTB4 reached peaks of 225.13 ± 55.37 ng/ml and 86.39 ± 4.02 ng/ml (Tmax, maximum time) at 2 and 4.67 ± 3.06 h, respectively. After administration, the plasma concentration of HT3 rapidly reached its maximum. The Tmax for oral administration was 0.33 ± 0.14 (Cmax of 121.91 ± 42.63 ng/ml), and the Tmax for intramuscular administration was 1.00 ± 0.00 (Cmax of 39.59 ± 11.22 ng/ml). The area under the curve (AUC) and mean residence time (MRT), as other important pharmacokinetic parameters, for compound S6 were 5.10 ± 4.64 g/L*h and 4.34 ± 2.59 h, respectively. The AUC values for oral administration were higher than those for intramuscular administration for both BTB4 and HT3, but the intramuscular administration increased the residence time of the compound in mice.
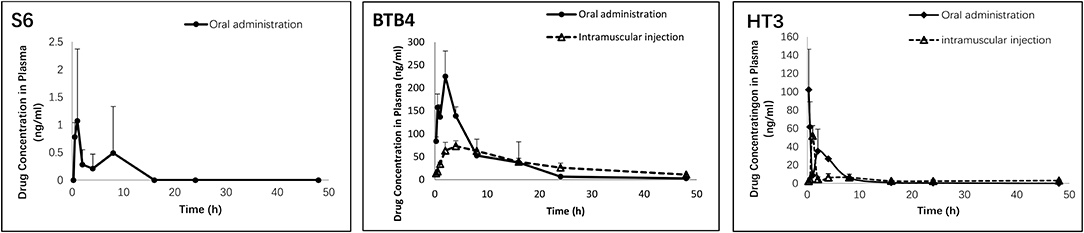
Figure 4. Concentrations of S6, HT3, and BTB4 in the plasma of mice after oral (50 mg/kg) and intramuscular (12.5 mg/kg) administration.
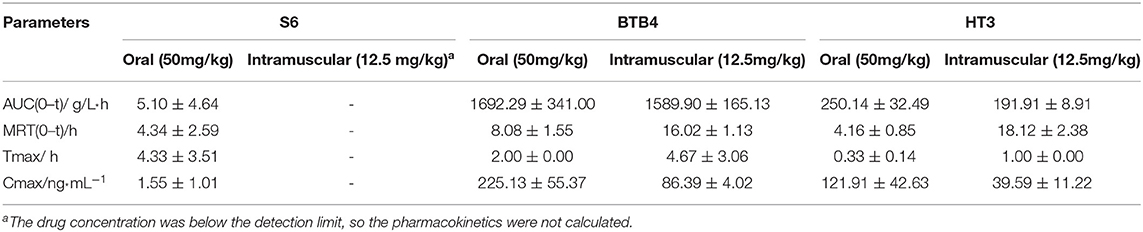
Table 4. Pharmacokinetic parameters of S6, HT3 and BTB4 in the plasma of mice after oral (50 mg/kg) and intramuscular (12.5 mg/kg) administration.
Discussion
This study was based on an analysis of the SAR of the pharmacophore model. The SAR describes the relationship between the chemical properties/three-dimensional structure of the compound and its biological activity, and researchers can identify the functional groups that play key roles in activities of small molecules (Ahamad et al., 2017). Any subsequent studies, such as the design of new compounds, structural modification of compounds, drug screenings and prediction of active compounds, are based on SARs. Quantitative structure-activity relationships (QSARs) and pharmacophore modeling are two important methods for SAR studies. QSARs are generally based on a series of compounds with the same framework and therefore can only guide the modification and activity prediction of compounds that are structurally similar (Verma et al., 2010; Yang et al., 2013). However, the pharmacophore model is derived from active compounds with different frameworks and generates the relevant pharmacophore characteristics to show the common features of active small molecules. That is, if a small molecule possesses the characteristics of a pharmacophore, then it may have a certain biological activity (Patel et al., 2002; Vlachakis et al., 2015; Gogoi et al., 2017). In our previous studies, we identified several structurally diverse active compounds out of in 130 amino alcohols, which is suitable for generating a pharmacophore model. Then we used the selected pharmacophore model to identify compounds with potential anti-echinococcal activity. Normally, the pharmacophore model can be generated by receptor-based and ligand-based methods. The former method relies on knowing the structure of the target (protein, DNA and so on) to analyse the interactions in the ligand-receptor complex (Gao et al., 2017; Kumar, 2018), while the latter method is based on the structures of the active compounds (Patel et al., 2002; Gogoi et al., 2017). Because the target of the series of amino alcohols involved in this study is unknown, a ligand-based approach was used to generate the pharmacophore model. In DS, model building includes two different pharmacophore modeling algorithms: generating common feature pharmacophore hypotheses from sets of known active ligands (HipHop) and also structure-activity relationship-based models when activity data is provided (HypoGen). The HypoGen module is for a group of compounds with different activities. With this method, the input training set compounds have activities in 4-5 orders s of magnitude, and each order has at least 3 compounds (Gupta et al., 2019). Unlike HypoGen, the HipHop algorithm does not consider the activity levels of the compounds in the training set. It only analyses only the three-dimensional spatial arrangement of the active compounds and uses an exhaustive search method to find pharmacophores that match the functional characteristics of the active compounds (Ataei et al., 2015; Fu et al., 2017; Che et al., 2018). Based on the results of in vitro drug screening of amino alcohols, we chose to establish a pharmacophore model using the HipHop module. Hence, a set of 6 training compounds was used to generate hypothetical pharmacophores using the HipHop algorithm. The structures were validated using the test set, and the best model (HipHop-Hypo03) was chosen to screen the ZINC database. Among the 176 compounds with fitness scores >4.0, 62 were tested on the parasites cultured in vitro. Ten of these compounds were identified as active, resulting in a screening hit rate of 16.13% (10/62). The virtual screening used in this study is an important example of the application of computer-aided drug design, including database searches based on pharmacophore models, QSAR models, structural similarity, molecular docking, pharmacokinetic properties and so on (Haga et al., 2016). These methods use computational methods and professional software to support hit finding and lead optimization in drug discovery. Generally, the hit rates are 5~20%, which is much higher than that of the high-throughput screening (Waszkowycz, 2008). Virtual screening has effectively shortened drug development time, reduced costs and improved efficiency, which can be very useful for the development of drugs to treat diseases that do not attract the attention of pharmaceutical companies. In recent years, computer-aided drug design has been applied to the discovery of anti-parasitic drugs (Postigo et al., 2010; Dube et al., 2012; Zhao et al., 2012; Pavadai et al., 2016; Vyas et al., 2016; Kagami et al., 2017; Araujo et al., 2018). Our study also indicated that virtual screening technology could play an important role in drug discovery for the treatment of echinococcosis.
Although the compounds in the training set used to generate pharmacophores were all amino alcohols, which are characterized by a hydroxyl group and an amino group connected by two carbon atoms, the structures of the 10 active compounds were all quite different. However, all of these compounds contained an amide bond, which is also present in BMZ and nitazoxanide, suggesting the importance of this moiety in affecting the activity against parasites. Further research on these active compounds showed that the LC50 values of PSCs were <20 μM, and 6 of them also affected cancer cells. Of these compounds, the low cytotoxicities of S5, S6, S11, and S16 against normal cells indicated good druggability. The structures of compounds S5, S6, and S11 were very similar, with the only difference being the position of the substituent on the benzene ring linked to -NH. As shown in Table 3, the substitution pattern of S5 resulted in a high toxicity, while S6 and S11, which contain two para-substituents, showed good activity and low toxicity. In addition, a –Cl substituent (S6) resulted in better efficacy than a –H substituent (S11).
Next, we selected compound S6 and two amino alcohols (HT3 and BTB4) to perform an in vivo pharmacodynamic study. Our preliminary results indicate that these two amino alcohols had better effects on cultured E. granulosus cysts in vitro and lower toxicity than the structures we reported (Liu et al., 2018). Hence, these two compounds, together with S6, were orally administered for 28 days at two doses of 50 and 25 mg/kg to mice infected with E. multilocularis. We found that although these compounds showed good effects against parasites in vitro, none were effective in mice secondarily infected with E. multilocularis. The death of experimental animals in the BTB4, HT3, and MBZ groups during the treatment period indicated that these compounds have notable toxicity.
In the development of new drugs, identifying an active compound is only the first step, and how to maintain the effective drug concentration and deliver the drugs to the target site is crucial and complicated. One difficulty in the development of anti-echinococcal drugs is that the drug must reach an effective concentration in the parasitic lesions through blood circulation, which is different from drugs for intestinal parasites that need to accumulate in only the intestine. Hence, high stability, high bioavailability, and the ability to penetrate the walls of cysts to achieve an effective concentration are requirements of good anti-echinococcal drugs. However, the existing active drugs/compounds, even the clinically used BMZ, do not have these characteristic and are limited by their poor absorption, so high-doses are still unknow, as is and long-term treatment is required in clinical use (Eckert et al., 2001). The physical and chemical properties that define good anti-echinococcal drugs are still unknown, as is how these drugs enter the cysts of parasites, whether by passive effusion or transporters. These uncertainties make screening criteria selection difficult. Hence, a pharmacokinetic study is necessary to observe how compounds behave in vivo. In this study, the concentration of S6 detected in plasma was very low. At many time points, the concentration was below the detection limit. For HT3 and BTB4, the drug concentrations did not reach the LC50. These results indicated that the poor performance in vivo may be related to the low drug concentration in plasma. In addition, the instability of S6 may also contribute to its poor drug efficacy because we found that if the drug and plasma samples were left overnight, the detectable concentration was greatly reduced. After analyzing the pharmaceutical parameters, we found that HT3 and BTB4 were not limited by absorption. Because they can rapidly enter the circulatory system and reach their peak concentration after oral administration, they are less affected by the first pass effect, suggesting that the drug's therapeutic effect can be imporved by increasing the dosage. However, their toxicities limit further studies on these two amino alcohols. For S6, the emphasis should be placed on increasing the administered dosage or structural modification after identifying the cause of its low plasma concentration, which will require additional experimental data.
Conclusion
Based on the above discussion, the efficacy of pharmacophore-based screening was higher (positive screening rate of 16.13%) than that of traditional screening, which promising for discovery of novel drugs. Especially for echinococcosis, which is one of ten neglected tropical diseases, drug discovery research is hampered by the lack of both researchers and funding. We found that the failure of S6 in the treatment of AE at a dosage of 50 mg/kg per day for 28 days was closely related to its low concentration in plasma, which is the main reason for the treatment failure of many drugs in vivo. We carried out a virtual screening of compounds with drug-like properties to avoid this problem, but unfortunately, the active compound identified still showed poor druggability. However, we believe that once the screening database for screening is modified, the application of computer-aided drug design to the development of anti-echinococcal drugs will be very useful for finding novel anti-echinococcal compounds.
Data Availability Statement
The datasets generated in this study are available by request from the corresponding author.
Ethics Statement
The animal study was reviewed and approved by Animal care and all animal procedures were carried out in compliance with the Guidelines for the Care and Use of Laboratory Animals produced by the Shanghai Veterinary Research Institute. The study was approved by the Ethics Committee of the National Institute of Parasitic Diseases, Chinese Center for Disease Control and Prevention. The license number was IPD-2014-2.
Author Contributions
HZ, YT, and CL developed the concept of the work. CL and ZX carried out the pharmacophore screening. CL, JYi, and JYa validated the effects of the compounds in vivo and in vitro. YT carried out the pharmacokinetics analysis. CL and JYi discussed and analyzed the results. CL wrote the paper.
Funding
This work was funded by grants from the National Natural Science Foundation of China (Nos. 81401691 and 81702030).
Conflict of Interest
The authors declare that the research was conducted in the absence of any commercial or financial relationships that could be construed as a potential conflict of interest.
Acknowledgments
The authors cordially acknowledge the expert help of Professor Wei-Liang Zhu from Drug Discovery and Design Center, Shanghai Institute of Materia Medica, Chinese Academy of Sciences. We are grateful to Xiumin Han from Qinghai Provincial People's Hospital for her kind provision of E. granulosus cysts.
Supplementary Material
The Supplementary Material for this article can be found online at: https://www.frontiersin.org/articles/10.3389/fcimb.2020.00118/full#supplementary-material
References
Ahamad, S., Rahman, S., Khan, F. I., Dwivedi, N., Ali, S., Kim, J., et al. (2017). QSAR based therapeutic management of M. Tuberculosis. Arch. Pharm. Res. 40, 676–694. doi: 10.1007/s12272-017-0914-1
Akhtar, N., Jabeen, I., Jalal, N., and Antilla, J. (2018). Structure-based pharmacophore models to probe anticancer activity of inhibitors of protein kinase B-beta (PKB beta). Chem. Biol. Drug Des. 93, 325–336. doi: 10.1111/cbdd.13418
Araujo, J. S. C., de Souza, B. C., Costa Junior, D. B., Oliveira, L. M., Santana, I. B., Duarte, A. A., et al. (2018). Identification of new promising Plasmodium falciparum superoxide dismutase allosteric inhibitors through hierarchical pharmacophore-based virtual screening and molecular dynamics. J. Mol. Model. 24:220. doi: 10.1007/s00894-018-3746-0
Ataei, S., Yilmaz, S., Ertan-Bolelli, T., and Yildiz, I. (2015). Generated 3D-common feature hypotheses using the HipHop method for developing new topoisomerase I inhibitors. Arch. Pharm. 348, 498–507. doi: 10.1002/ardp.201500045
Che, J., Wang, Z., Sheng, H., Huang, F., Dong, X., Hu, Y., et al. (2018). Ligand-based pharmacophore model for the discovery of novel CXCR2 antagonists as anti-cancer metastatic agents. R. Soc. Open Sci. 5:180176. doi: 10.1098/rsos.180176
Crisan, L., Avram, S., and Pacureanu, L. (2017). Pharmacophore-based screening and drug repurposing exemplified on glycogen synthase kinase-3 inhibitors. Mol. Divers. 21, 385–405. doi: 10.1007/s11030-016-9724-5
Davis, A., Dixon, H., and Pawlowski, Z. S. (1989). Multicentre clinical trials of benzimidazole-carbamates in human cystic echinococcosis (phase 2). Bull. World Health Organ. 67, 503–508.
Davis, A., Pawlowski, Z. S., and Dixon, H. (1986). Multicentre clinical trials of benzimidazolecarbamates in human echinococcosis. Bull. World Health Organ. 64, 383–388.
Dube, D., Periwal, V., Kumar, M., Sharma, S., Singh, T. P., and Kaur, P. (2012). 3D-QSAR based pharmacophore modeling and virtual screening for identification of novel pteridine reductase inhibitors. J. Mol. Model. 18, 1701–1711. doi: 10.1007/s00894-011-1187-0
Eckert, J., Gemmell, M. A., and Meslin, F. X. (2001). WHO/OIE Manual on Echinococcosis in Humans and Animals: A Public Health Problem of Global Concern. Paris: Office International des Epizooties.
Eckert, J., Pawlowski, Z., Dar, F.K., Vuitton, D.A., Kem, P., Savioli, L. (1995). Medical aspects of echinococcosis. Parasitol. Today 11, 273–276. doi: 10.1016/0169-4758(95)80036-0
Fu, Y., Sun, Y. N., Yi, K. H., Li, M. Q., Cao, H. F., Li, J. Z., et al. (2017). 3D pharmacophore-based virtual screening and docking approaches toward the discovery of novel HPPD inhibitors. Molecules 22:E959. doi: 10.3390/molecules22060959
Ganesan, A., and Barakat, K. (2017). Applications of computer-aided approaches in the development of hepatitis C antiviral agents. Expert Opin. Drug Discov. 12, 407–425. doi: 10.1080/17460441.2017.1291628
Gao, Q., Wang, Y., Hou, J., Yao, Q., and Zhang, J. (2017). Multiple receptor-ligand based pharmacophore modeling and molecular docking to screen the selective inhibitors of matrix metalloproteinase-9 from natural products. J. Comput. Aided Mol. Des. 31, 625–641. doi: 10.1007/s10822-017-0028-3
Gogoi, D., Chaliha, A. K., Sarma, D., Kakoti, B. B., and Buragohain, A. K. (2017). Novel butyrylcholinesterase inhibitors through pharmacophore modeling, virtual screening and DFT-based approaches along-with design of bioisosterism-based analogues. Biomed. Pharmacother. 85, 646–657. doi: 10.1016/j.biopha.2016.11.076
Gupta, C. L., Babu Khan, M., Ampasala, D. R., Akhtar, S., Dwivedi, U. N., and Bajpai, P. (2019). Pharmacophore-based virtual screening approach for identification of potent natural modulatory compounds of human Toll-like receptor 7. J. Biomol. Struct. Dyn. 37, 4721–4736. doi: 10.1080/07391102.2018.1559098
Haga, J. H., Ichikawa, K., and Date, S. (2016). Virtual screening techniques and current computational infrastructures. Curr. Pharm. Des. 22, 3576–3584. doi: 10.2174/1381612822666160414142530
Hemphill, A., and Muller, J. (2009). Alveolar and cystic echinococcosis: towards novel chemotherapeutical treatment options. J. Helminthol. 83, 99–111. doi: 10.1017/S0022149X0928936X
Kagami, L. P., das Neves, G. M., Rodrigues, R. P., da Silva, V. B., Eifler-Lima, V. L., and Kawano, D. F. (2017). Identification of a novel putative inhibitor of the Plasmodium falciparum purine nucleoside phosphorylase: exploring the purine salvage pathway to design new antimalarial drugs. Mol. Divers. 21, 677–695. doi: 10.1007/s11030-017-9745-8
Kumar, S. P. (2018). Receptor pharmacophore ensemble (REPHARMBLE): a probabilistic pharmacophore modeling approach using multiple protein-ligand complexes. J. Mol. Model. 24:282. doi: 10.1007/s00894-018-3820-7
Lacey, E. (1990). Mode of action of benzimidazoles. Parasitol. Today 6, 112–115. doi: 10.1016/0169-4758(90)90227-U
Lipinski, C. A., Lombardo, F., Dominy, B. W., and Feeney, P. J. (2001). Experimental and computational approaches to estimate solubility and permeability in drug discovery and development settings. Adv. Drug Deliv. Rev. 46, 3–26. doi: 10.1016/S0169-409X(00)00129-0
Liu, C., Han, X., Lei, W., Yin, J. H., Wu, S. L., and Zhang, H. B. (2019). The efficacy of an alternative mebendazole formulation in mice infected with Echinococcus multilocularis. Acta Trop. 196, 72–75. doi: 10.1016/j.actatropica.2019.05.009
Liu, C., Yin, J., Xue, J., Tao, Y., Hu, W., and Zhang, H. (2018). In vitro effects of amino alcohols on Echinococcus granulosus. Acta Trop. 182, 285–290. doi: 10.1016/j.actatropica.2017.08.031
Liu, C., Zhang, H., Yin, J., and Hu, W. (2015). In vivo and in vitro efficacies of mebendazole, mefloquine and nitazoxanide against cyst echinococcosis. Parasitol. Res. 114, 2213–2222. doi: 10.1007/s00436-015-4412-4
Moro, P., and Schantz, P. M. (2009). Echinococcosis: a review. Int. J. Infect. Dis. 13, 125–133. doi: 10.1016/j.ijid.2008.03.037
Nicolao, M. C., Elissondo, M. C., Denegri, G. M., Goya, A. B., and Cumino, A. C. (2014). In vitro and in vivo effects of tamoxifen against larval stage Echinococcus granulosus. Antimicrob. Agents Chemother. 58, 5146–5154. doi: 10.1128/AAC.02113-13
Patel, Y., Gillet, V. J., Bravi, G., and Leach, A. R. (2002). A comparison of the pharmacophore identification programs: catalyst, DISCO and GASP. J. Comput. Aided Mol. Des. 16, 653–681. doi: 10.1023/A:1021954728347
Pavadai, E., El Mazouni, F., Wittlin, S., de Kock, C., Phillips, M. A., and Chibale, K. (2016). Identification of new human malaria parasite plasmodium falciparum dihydroorotate dehydrogenase inhibitors by pharmacophore and structure-based virtual screening. J. Chem. Inf. Model. 56, 548–562. doi: 10.1021/acs.jcim.5b00680
Postigo, M. P., Guido, R. V., Oliva, G., Castilho, M. S., da, R. P. I., de Albuquerque, J.F., et al. (2010). Discovery of new inhibitors of Schistosoma mansoni PNP by pharmacophore-based virtual screening. J. Chem. Inf. Model. 50, 1693–1705. doi: 10.1021/ci100128k
Ritler, D., Rufener, R., Sager, H., Bouvier, J., Hemphill, A., and Lundstrom-Stadelmann, B. (2017). Development of a movement-based in vitro screening assay for the identification of new anti-cestodal compounds. PLoS Negl. Trop. Dis. 11:e0005618. doi: 10.1371/journal.pntd.0005618
Rufener, R., Ritler, D., Zielinski, J., Dick, L., da Silva, E. T., da Silva Araujo, A., et al. (2018). Activity of mefloquine and mefloquine derivatives against Echinococcus multilocularis. Int. J. Parasitol. Drugs Drug Resist. 8, 331–340. doi: 10.1016/j.ijpddr.2018.06.004
Sarciron, M. E., Delabre, I., Walbaum, S., Raynaud, G., and Petavy, A. F. (1992). Effects of multiple doses of isoprinosine on Echinococcus multilocularis metacestodes. Antimicrob. Agents Chemother. 36, 191–194. doi: 10.1128/AAC.36.1.191
Shah, P., Tiwari, S., and Siddiqi, M. I. (2015). Recent progress in the identification and development of anti-malarial agents using virtual screening based approaches. Comb. Chem. High Throughput Screen. 18, 257–268. doi: 10.2174/1386207318666150305154913
Siles-Lucas, M., Casulli, A., Cirilli, R., and Carmena, D. (2018). Progress in the pharmacological treatment of human cystic and alveolar echinococcosis: compounds and therapeutic targets. PLoS Negl. Trop. Dis. 12:e0006422. doi: 10.1371/journal.pntd.0006422
Veber, D. F., Johnson, S. R., Cheng, H. Y., Smith, B. R., Ward, K. W., and Kopple, K. D. (2002). Molecular properties that influence the oral bioavailability of drug candidates. J. Med. Chem. 45, 2615–2623. doi: 10.1021/jm020017n
Verma, J., Khedkar, V. M., and Coutinho, E. C. (2010). 3D-QSAR in drug design–a review. Curr. Top. Med. Chem. 10, 95–115. doi: 10.2174/156802610790232260
Vlachakis, D., Fakourelis, P., Megalooikonomou, V., Makris, C., and Kossida, S. (2015). DrugOn: a fully integrated pharmacophore modeling and structure optimization toolkit. Peer J. 3:e725. doi: 10.7717/peerj.725
Vyas, V. K., Qureshi, G., Ghate, M., Patel, H., and Dalai, S. (2016). Identification of novel PfDHODH inhibitors as antimalarial agents via pharmacophore-based virtual screening followed by molecular docking and in vivo antimalarial activity. SAR QSAR Environ. Res. 27, 427–440. doi: 10.1080/1062936X.2016.1189959
Waszkowycz, B. (2008). Towards improving compound selection in structure-based virtual screening. Drug Discov. Today 13, 219–226. doi: 10.1016/j.drudis.2007.12.002
WHO/Department of Control of Neglected Tropical Diseases (2013). Sustaning the Drive to Overcome the Global Impact of Neglected Tropical Diseases: Second WHO Report on Neglected Tropical Diseases. WHO Press.
Yang, C., Shao, Y., Zhi, X., Huan, Q., Yu, X., Yao, X., et al. (2013). Semisynthesis and quantitative structure-activity relationship (QSAR) study of some cholesterol-based hydrazone derivatives as insecticidal agents. Bioorg. Med. Chem. Lett. 23, 4806–4812. doi: 10.1016/j.bmcl.2013.06.099
Keywords: echinococcosis, Echinococcus multilocularis, pharmacophore modeling, virtual screenings, in vitro drug screen, cytotoxicity, E. multilocularis-infected mice, pharmacokinetics analysis
Citation: Liu C, Yin J, Yao J, Xu Z, Tao Y and Zhang H (2020) Pharmacophore-Based Virtual Screening Toward the Discovery of Novel Anti-echinococcal Compounds. Front. Cell. Infect. Microbiol. 10:118. doi: 10.3389/fcimb.2020.00118
Received: 01 November 2019; Accepted: 02 March 2020;
Published: 20 March 2020.
Edited by:
Ehsan Ahmadpour, Tabriz University of Medical Sciences, IranReviewed by:
Fatemeh Ghaffarifar, Tarbiat Modares University, IranHossein Mahmoudvand, Lorestan University of Medical Sciences, Iran
Copyright © 2020 Liu, Yin, Yao, Xu, Tao and Zhang. This is an open-access article distributed under the terms of the Creative Commons Attribution License (CC BY). The use, distribution or reproduction in other forums is permitted, provided the original author(s) and the copyright owner(s) are credited and that the original publication in this journal is cited, in accordance with accepted academic practice. No use, distribution or reproduction is permitted which does not comply with these terms.
*Correspondence: Yi Tao, VGFveWlfTklQREAxNjMuY29t; Haobing Zhang, emhhbmdoYW9iaW5nMkAxNjMuY29t
†These authors have contributed equally to this work