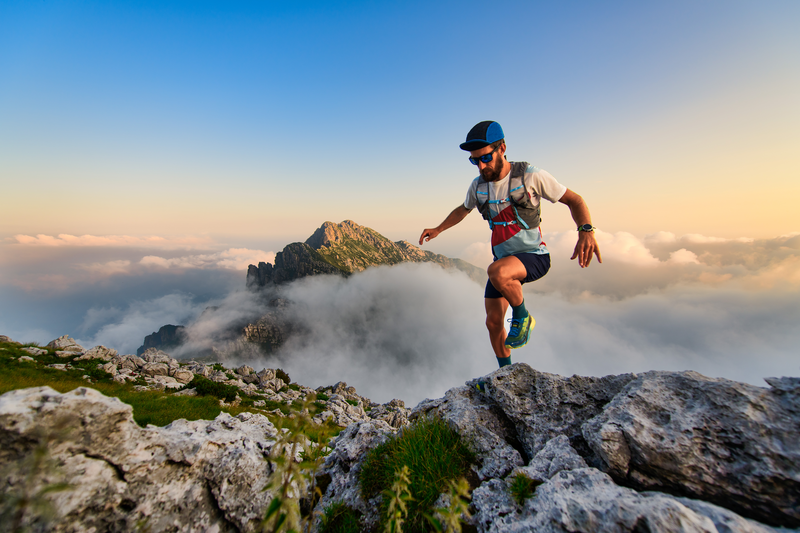
95% of researchers rate our articles as excellent or good
Learn more about the work of our research integrity team to safeguard the quality of each article we publish.
Find out more
REVIEW article
Front. Cell. Infect. Microbiol. , 28 February 2020
Sec. Fungal Pathogenesis
Volume 10 - 2020 | https://doi.org/10.3389/fcimb.2020.00069
This article is part of the Research Topic Advances in Understanding Innate Immunity to Fungi View all 7 articles
Fungi are ubiquitous. Yet, despite our frequent exposure to commensal fungi of the normal mammalian microbiota and environmental fungi, serious, systemic fungal infections are rare in the general population. Few, if any, fungi are obligate pathogens that rely on infection of mammalian hosts to complete their lifecycle; however, many fungal species are able to cause disease under select conditions. The distinction between fungal saprophyte, commensal, and pathogen is artificial and heavily determined by the ability of an individual host's immune system to limit infection. Dramatic examples of commensal fungi acting as opportunistic pathogens are seen in hosts that are immune compromised due to congenital or acquired immune deficiency. Genetic variants that lead to immunological susceptibility to fungi have long been sought and recognized. Decreased myeloperoxidase activity in neutrophils was first reported as a mechanism for susceptibility to Candida infection in 1969. The ability to detect genetic variants and mutations that lead to rare or subtle susceptibilities has improved with techniques such as single nucleotide polymorphism (SNP) microarrays, whole exome sequencing (WES), and whole genome sequencing (WGS). Still, these approaches have been limited by logistical considerations and cost, and they have been applied primarily to Mendelian impairments in anti-fungal responses. For example, loss-of-function mutations in CARD9 were discovered by studying an extended family with a history of fungal infection. While discovery of such mutations furthers the understanding of human antifungal immunity, major Mendelian susceptibility loci are unlikely to explain genetic disparities in the rate or severity of fungal infection on the population level. Recent work using unbiased techniques has revealed, for example, polygenic mechanisms contributing to candidiasis. Understanding the genetic underpinnings of susceptibility to fungal infections will be a powerful tool in the age of personalized medicine. Future application of this knowledge may enable targeted health interventions for susceptible individuals, and guide clinical decision making based on a patient's individual susceptibility profile.
We are constantly exposed to fungi capable of causing disease. Every human is colonized with a commensal fungal mycobiome (Huffnagle and Noverr, 2013), and it is nearly impossible to eliminate the spores of saprophytic environmental fungi even from the cleanest settings (Oberle et al., 2015). It should not be surprising, then, that superficial fungal infections are among the most common infectious diseases worldwide (White et al., 2014). Yet, remarkably, most people do not regularly get fungal infections, and invasive fungal infections are rare in the general population (Bongomin et al., 2017; Li et al., 2017).
One population at high risk of fungal illness is those who are immunocompromised. This has long been recognized; for example, Acquired Immunodeficiency Syndrome (AIDS) was first reported as a case series of opportunistic fungal infections in homosexual men (Centers for Disease Control, 1981). The number of “at risk” immunocompromised people is growing largely due to secondary causes, e.g., immunosuppressive drugs used in the setting of malignancy, organ transplantation, or autoimmune disease (Yapar, 2014; Pana et al., 2017).
Primary immunodeficiencies (PIDs) continues to be a threat. PIDs are inherited defects in the immune response; depending on the affected pathway(s), PIDs have different severity, onset, and risks of infection by certain groups of organisms. Perhaps the most dramatic PID is Severe Combined Immunodeficiency (SCID), a condition where the absence of an adaptive immune response uniformly leads to overwhelming infections and death in the first few years of life in the absence of stem cell transplantation. SCID was first described in 1950 and soon recognized as heritable (Buckley, 2004).
A number of monogenic deficits in immunity engender fungal illness. For example, multiple different loss-of-function mutations in CARD9 have been associated with autosomal recessive inheritance of susceptibility to invasive infections by fungi, including Candida spp., dermatophytes, and fungal plant pathogens (Vaezi et al., 2018). Understanding the immune deficits that underlie “idiopathic” fungal disease in otherwise healthy people has provided valuable insight into human antifungal immunity, and there is clinical utility to the diagnosis of these conditions (e.g., genetic counseling, antifungal prophylaxis) (Li et al., 2017). Attribution of these PIDs to biological processes has historically relied on a “candidate gene” approach, where animal models of disease and in vitro assays with patient cells have guided the search for “lesions” in select genes.
While monogenic, “Mendelian” susceptibility variants have revealed the pathways that are critical for control of different pathogens, they are unlikely to explain population-level patterns in risk of fungal disease. Such monogenic “lesions” in non-redundant immune pathways are overwhelmingly deleterious; they confer a substantial risk of illness, and as a result there is strong purifying selection that favors recognition and control of infection (Netea et al., 2012).
Fine variations in the immune responses that do not result in susceptibility to overwhelming infection are more likely to explain population-level trends in fungal illness. Susceptibility to mild infections may be maintained in a population because overly exuberant antimicrobial immune responses can be disadvantageous; they may cause undue tissue pathology in response to infection (Jaeger et al., 2016), or engender autoimmunity (Zhernakova et al., 2010; Ramos, 2017). Thus, a person's genetic susceptibility is the net result of many variants that each alter immune function in nearly imperceptible ways. Such variants are under substantially less selective pressure than those underlying PIDs and Mendelian susceptibility variants, and are more prone to changes in frequency due to stochastic effects like genetic drift (Netea et al., 2012).
Advances in bioinformatic approaches have made it more practical to screen a large number of donors, and to search widely in the genome for rare variants or mutations. This has facilitated the discovery of many common variants that confer a small risk of select infections. High-quality studies seeking such risk variants will involve a large number of participants that share a well-defined set of clinical parameters, plus a large number of well-matched participants serving as controls, and efforts will be made to validate functional impacts of risk variants. Validation may not always be possible due to the limitations of experimental systems, in which case animal models of illness may provide corroborating evidence to demonstrate biological feasibility. Consideration must be given to confounding factors that could introduce error. One notable confounder in the search for risk variants is ethnic/racial differences between study group and control. Thus, even a well-conducted study may have limited generalizability as it only involves participants of a given genetic background. Replicating studies with cohorts from different genetic backgrounds is valuable in beginning to understand how given risk variants may be masked by epistatic effects. Throughout this review, the ethnic/racial background of participants in a given study is reported using the demographic terms used by the authors of the study.
This review addresses genetic susceptibility to fungal infection, with an emphasis on the growing understanding of common, subtle variants that underlie population-level patterns of disease. We present “case studies” of illness caused by four groups of fungal pathogens: dermatophytes, Candida spp., Aspergillus spp., and dimorphic fungi endemic to the United States. Each section begins with an overview of relevant ecological, evolutionary, and epidemiologic features, then proceeds to human susceptibility to infection. Fungal diseases are discussed in order of global prevalence.
Dermatophytic fungal diseases are among the most common infectious diseases worldwide (White et al., 2014; Bongomin et al., 2017). This group includes fungi with diverse natural histories and clinical courses. Dermatophytes are a monophyletic group of ascomycetes of the genera Trichophyton, Microsporum, and Epidermophyton (White et al., 2014). Anthropophilic species are found exclusively on humans, zoophilic species are found on a number of different animal hosts, and geophilic species are found in the environment (White et al., 2014). Anthropophilic species are capable of a commensal lifestyle on human hosts, zoophilic species generally cause chronic or mild illness in humans, and geophilic species that are not adapted to live hosts are a rare cause of acute illness (White et al., 2014). Studies have identified a number of virulence factors that determine pathogenicity of dermatophytes (Gnat et al., 2019).
Dermatophyte infection can lead to illness characterized by scaly, pruritic rashes. These illnesses are often named by the location; e.g., tinea pedis for infections affecting the feet, and tinea capitis for infections affecting the scalp. Local prevalence of dermatophytic illness is reported to be over 70% in some populations, and up to one billion people are affected globally (Bongomin et al., 2017). The development of illness is dependent on environmental factors like hygiene and humidity; thus, prevalence of illness is lower in developed areas (Bongomin et al., 2017). Though dermatophytes are primary pathogens in that they cause illness in otherwise healthy people, disease is often mild and self-limiting, and exposure to and colonization with these fungi without associated disease is well-described (Abdel-Rahman et al., 2006; Gnat et al., 2019).
Dermatophyte infection was the subject of the first genome wide association study (GWAS) of fungal infection. Abdel-Rahman et al. (2006) studied 446 predominantly African-American children over 2 years in an urban daycare center, documenting symptoms of tinea capitis and carriage of the most commonly associated dermatophyte (T. tonsurans). They found that donors were either exclusive carriers of one strain, predominant carriers of one strain with occasional other strains recorded, or “random” carriers who did not appear to have a predilection for one strain (Abdel-Rahman et al., 2006). Intriguingly, “random” carriers had the fewest symptomatic infections (Abdel-Rahman et al., 2006). Abdel-Rahman and Preuett (2012) then did whole genome genotyping on 20 children who carried T. tonsurans >90% of the time, and 20 children who carried the fungus <10% of the time. The authors identified 21 genes whose genotype was associated with carriage of the fungus, though they did not study whether this was correlated with symptoms of tinea capitis. Genes uncovered in this GWAS were associated with various different functions, including leukocyte function, remodeling of extracellular matrix, wound repair, and cutaneous permeability (Abdel-Rahman and Preuett, 2012). Genotyping an additional 115 children, the authors found that genotype at just 8 of these genes accounted for about 60% of the variance in carriage rate (Abdel-Rahman and Preuett, 2012).
Few candidate gene studies have been carried out with patients suffering from superficial dermatophytosis. CLEC7A-Y238X, an early stop codon variant that impacts recognition of fungal β-glucan by the receptor Dectin-1, was reported in a Dutch family where all were affected by onychomycosis (Ferwerda et al., 2009). Subsequent studies of CLEC7A-Y238X have described more subtle clinical phenotypes (discussed below), and have not discussed an association with superficial dermatophytosis. Other studies of onychomycosis propose a role for variation at the Human Leukocyte Antigen (HLA) complex, which is involved with presentation of extracellular antigens to T cells and initiation of adaptive responses (Sadahiro et al., 2004; Asz-Sigall et al., 2010; Garcia-Romero et al., 2012; Carrillo-Melendrez et al., 2016). The keratinized nail is sometimes thought to be out of reach of the immune system (Gnat et al., 2019), but these studies suggest a role for both innate and adaptive immune responses to onychomycosis.
Early observations of disparities between those with dermatophyte illness and those at risk led to the first studies proposing a genetic contribution to dermatophytosis. Tinea imbricata, chronic dermatophytosis with a characteristic pattern of skin lesions caused by infection with T. concentricum, is common in some tropical areas (Ravine et al., 1980). The rate of tinea imbricata differs between people of different racial backgrounds despite similar exposure risk (Ravine et al., 1980). Illness begins early in life, and either resolves spontaneously or becomes chronic (Ravine et al., 1980). One study of 228 families in Papua New Guinea concluded that risk of persistent tinea imbricata is likely autosomal recessive (Ravine et al., 1980). A smaller study of a polygamous Mexican family found an autosomal dominant pattern of infection (Bonifaz et al., 2004). The differences between these reports may be due to the small number of people studied, or reflect a different underlying genetic determinant of susceptibility.
Immune function in patients with chronic widespread dermatophytosis (CWD) due to T. rubrum is characterized by selective functional deficits. Phagocytes from immunocompetent patients with T. rubrum CWD were less effective at killing T. rubrum, and produced less hydrogen peroxide and pro-inflammatory cytokines in response to the fungus, compared to cells from healthy donors (de Sousa Mda et al., 2015). The same deficits were not noted when comparing cells stimulated with maximal stimuli (e.g., LPS), or in a cohort of patients with acute tinea pedis (de Sousa Mda et al., 2015). Although genetic studies have not been done on patients with CWD, it is noteworthy that several of these functional deficits are also seen in patients with CARD9 deficiency (Drummond et al., 2015; Liang et al., 2015). CARD9 is the intracellular adaptor for several pattern recognition receptors (PRRs), including several C-type lectin receptors (CLRs) that are critical for recognition of fungi (Zhong et al., 2018). Loss-of-function mutations in CARD9 are the only known genetic etiology underlying deep dermatophytosis, where dermatophytes invade through skin and often disseminate (Lanternier et al., 2013; Gnat et al., 2019). The immune deficits that render patients with CARD9 deficiency susceptible to fungal infection are discussed at length below, in the section on “idiopathic” infections by Candida spp.
Dermatophytosis is one of the most common infectious diseases globally, often presenting as self-limiting superficial infections. Environmental factors strongly influence the development of disease. Still, carriage of dermatophytic fungi is correlated with illness, and this is influenced strongly by a small number of genes involved in leukocyte function and other processes (Abdel-Rahman and Preuett, 2012). Multiple studies have proposed a role for host genetics in the development of illness, including observations of possible Mendelian inheritance patterns for chronic dermatophytosis (Ravine et al., 1980; Bonifaz et al., 2004). Candidate gene studies implicate fungal recognition, especially signaling through the adaptor CARD9, as a critical component in controlling dermatophytosis (Lanternier et al., 2013).
Candida spp. are responsible for an estimated 138 million cases per year of recurrent vulvovaginal candidiasis (RVVC), in excess of 3 million cases per year of mucosal candidiasis (including oropharyngeal and esophageal), and about 750 thousand cases per year of invasive candidiasis and candidemia (Bongomin et al., 2017; Denning et al., 2018). Candida spp. are the fourth leading pathogen, and most common fungal pathogen causing healthcare-associated infections (Magill et al., 2018). Candidemia is a feared complication of immunosuppression, with case mortality rates of over 20% (Pagano et al., 2017). Still, the most prevalent clinical illness caused by Candida spp. is, by far, primary infections in immunocompetent women (Sobel, 2007; Bongomin et al., 2017; Denning et al., 2018).
Colonization with Candida spp. occurs within the first month of life (Ward et al., 2018), and Candida spp. are known to be commensal with humans (Soll et al., 1991; Huffnagle and Noverr, 2013; Neville et al., 2015; Nash et al., 2017). There is evidence that colonization with Candida spp. is important in the development of antifungal immunity, conferring protection against future fungal illness (Shao et al., 2019). Yet genetic typing has found that the Candida strains that cause disease originate from the healthy mycobiome (Odds et al., 1989; Gouba and Drancourt, 2015). Fungal biology plays a role in the disease process; the transition from the “commensal” yeast morphology to a “pathogenic” filamentous morphology is a well-studied virulence factor for C. albicans (Cheng et al., 2011; Cassone and Cauda, 2012; Pais et al., 2019).
Though C. albicans has long been the most common agent of candidiasis, the number of infections attributed to non-albicans Candida spp. (NAC) is increasing (Bongomin et al., 2017). This is concerning since the NAC generally have a higher level of resistance to clinically important antifungals (Bongomin et al., 2017). Candida is not a monophyletic genus; C. glabrata, the most common etiological agent of NAC infections, is more closely related to Saccharomyces cerevisiae than C. albicans (Fitzpatrick et al., 2006). One notable difference is that C. glabrata does not undergo the yeast-to-pseudohyphae transition that is important for pathogenicity of C. albicans (Pais et al., 2019).
VVC affects about 75% of women at least once in their life (Sobel, 2007; Denning et al., 2018). Estimating the prevalence of VVC is complicated by a lack of diagnostic workup and reporting. Relying on self-reported data carries a risk of including myriad other conditions that may be mistaken for VVC. After taking this into account, Denning et al. estimated that about 138 million women per year are affected by RVVC (defined as four or more cases of VVC in 1 year) (Denning et al., 2018).
While most cases of RVVC are not attributable to a known comorbidity, many conditions are known to increase risk (Sobel, 2007; Denning et al., 2018). These include diabetes, cystic fibrosis, antibiotic use, pregnancy, and hormone replacement therapy (Sobel, 2007; Denning et al., 2018). Intriguingly, HIV infection and immunodeficiency do not confer risk of VVC; this, along with corroborating studies of a murine model of VVC, suggests that adaptive immunity is dispensable for prevention of VVC (Verma et al., 2017; Peters et al., 2019). Some features of the vaginal microbiome may confer resistance to VVC (Zangl et al., 2019). There are reported differences in the vaginal microbiomes of women of different ethnicities, even when donors live in the same area (Ravel et al., 2011). This may account for some of the inconsistencies in reported associations between select variants and risk of VVC when studies are carried out in genetically distinct populations.
An increasing number of genetic studies of susceptibility to RVVC support a critical role for innate immunity. Mannose-binding lectin (MBL, coded by the gene MBL2) is a soluble CLR that activates the complement cascade, facilitating opsonophagocytosis of fungi (Brouwer et al., 2008). A meta-analysis of five candidate gene studies found that a common loss-of-function variant MBL2 allele B confers risk of RVVC when compared to the wild-type MBL2 allele A (Nedovic et al., 2014). The risk of RVVC with MBL2 genotype B,B was substantial (MBL2 genotype B,B vs. A,A; odds ratio = 12.68, 95% confidence interval = 3.74–42.92) (Nedovic et al., 2014). This meta-analysis included studies in European and East Asian (Chinese) donors, and findings have since been replicated by studying MBL2 promoter variants in South Asian (Indian) donors (Kalia et al., 2017). Interestingly, studies with European (Italian) (Milanese et al., 2008) and Mexican (Velazquez-Hernandez et al., 2017) donors have failed to find an association between variation at MBL2 and risk of RVVC. This could reflect either slight differences in donor characteristics, or unappreciated epistatic effects masking the role of MBL2 in these populations.
Risk variants have been identified in other receptors involved in the innate recognition of Candida spp. Recently, a WES approach in two independent cohorts of Northern and Southern Europeans identified variants in SIGLEC15 associated with RVVC (Jaeger et al., 2019). The authors go on to validate that SIGLEC15, a lectin that binds sialic acid-containing structures, binds Candida spp. and is upregulated by immune cells upon exposure to Candida (Jaeger et al., 2019). They further demonstrate that risk variants lead to an altered cytokine profile after stimulation of immune cells with heat-killed C. albicans yeast, and that SIGLEC15 silencing leads to an increased inflammatory response and fungal burden in a murine model of vaginal candidiasis (Jaeger et al., 2019). Recognition of β glucans in the yeast cell wall may be important in RVVC. A coding variant in TLR2 is associated with RVVC (Rosentul et al., 2014a), and multiple studies have proposed a role for Dectin-1. CLEC7A-Y238X, a variant that decreases the ability of Dectin-1 to recognize to β glucan, was found to confer risk in studies of Dutch (Ferwerda et al., 2009) and Caucasian (De Luca et al., 2013) women, but not in Western-European (Rosentul et al., 2014a), Turkish (Usluogullari et al., 2014), or Iranian (Zahedi et al., 2016) women. A study of Indian women found other CLEC7A variants to be associated with risk of RVVC (Kalia et al., 2018). Studies have failed to find associations between RVVC and polymorphisms in other PRRs (TLR1, TLR4, NOD2) or CARD9 (Morre et al., 2002; van der Graaf et al., 2006a; Rosentul et al., 2014a).
There are conflicting reports on the role of the inflammasome and IL-1β signaling in the risk of RVVC, perhaps suggesting alternative mechanisms for susceptibility to RVVC. NLRP3 encodes one component of the inflammasome, which cleaves and activates the pro-inflammatory cytokines IL-1β and IL-18. Intron 4 of NLRP3 contains a variable number tandem repeats (VNTR). Decreased activity of NLRP3, associated with NLRP3 allele 7, is associated with RVVC (Lev-Sagie et al., 2009). Donor cells stimulated with C. albicans produce less IL-1β when carrying NLRP3 genotype 7,7 vs. 12,12 (Lev-Sagie et al., 2009). Decreased NLRP3 activity also confers susceptibility to VVC in a murine model (Bruno et al., 2015). Interestingly, another study found the opposite association between NLRP3 activity and RVVC. Jaeger et al. (2016) reported that the hyper-active NLRP3 genotype 12,9 is associated with RVVC and the NLRP3 genotype 7,7 is not associated (p = 0.06). In support of their reported association, the authors noted increased IL-1β production in response to C. albicans for NLRP3 genotype 12,9 vs. 12,12 (Jaeger et al., 2016). Both genetic studies of NLRP3 involved several hundred participants of white and Western-European background, respectively (Lev-Sagie et al., 2009; Jaeger et al., 2016). Indeed, both models of susceptibility are compatible; it may be that both over- and under-activity of NLRP3 engender symptomatic infection depending on the activity of other components of the immune response. Interestingly, infection is not a prominent feature of the spectrum of conditions caused by gain-of-function variants in NLRP3 (Gupta et al., 2019), suggesting that overactivity of NLRP3 is likely not sufficient to predispose an individual to RVVC. While properly calibrated host responses limit pathogenesis, both too much and too little inflammation can lead to disease (Robinson and Huppler, 2017).
A third avenue of research has implicated variants affecting cellular immunity in risk of RVVC. De Luca et al. described variants in IL22A and IDO1 significantly associated with RVVC (and not VVC) in Caucasian women (De Luca et al., 2013). They demonstrated that risk variants were associated with decreased production of IL-22 and IDO1, and downstream deficits in calprotectin and kynurenine levels, respectively (De Luca et al., 2013). A study of Latvian women found that a variant in the IL4 promoter was associated with higher levels of IL-4 in vaginal lavage and elevated risk of RVVC (Babula et al., 2005). Considered together, these reports suggest that IL-22-producing cells, including Th17 cells, are important in RVVC and that responses nurtured by IL-4, including Th2 cells, confer risk. Interestingly, though IL17A and IL22 are highly up-regulated during infection in a murine model of VVC, there was no difference in fungal burden or neutrophil responses between IL22−/− mice and wildtype (Peters et al., 2019). Likewise, lack of CD4+ T cells in humans, for example during AIDS, does not confer risk of RVVC.
A final mechanism that may confer risk of RVVC involves glycosylation of the vaginal mucosa. Three small studies have addressed the relationship between Lewis antigen secretor status and risk of RVVC in humans (Hilton et al., 1995; Chaim et al., 1997; Kulkarni and Venkatesh, 2004). Results from the earliest study are difficult to interpret in light of potential error due to a small number of African American donors that could account for observed differences (Hilton et al., 1995). The other two studies, however, found that non-secretor status was a risk factor for RVVC in white women (Chaim et al., 1997) and VVC in Indian women (Kulkarni and Venkatesh, 2004). Compellingly, FUT2−/− mice, a model for non-secretors, are more susceptible than wild type controls to C. albicans vaginitis (Hurd and Domino, 2004; Domino et al., 2009). Underlying mechanisms for this susceptibility have not been studied. However, a growing body of evidence has related FUT2 activity and downstream mucosal fucosylation to altered composition of the gut microbiome, risk of bacterial and viral infections, and several autoimmune conditions (Kononova, 2017). In addition to differences that exist at steady state, there is evidence linking IL-22 and FUT2 during infection. In a mouse model of opportunistic bacterial infection, signaling through IL-22RA1 was required for Fut2 enzyme activity and the resultant fucosylation (Pham et al., 2014). Direct replacement of 2′-fucosyllactose, the product of Fut2, restrained the intestinal infection phenotype in IL22RA1−/− mice (Pham et al., 2014).
Oropharyngeal candidiasis (OPC) and esophageal candidiasis (OEC) are closely associated with the immunocompromised state. Together, these conditions affect about 5% of HIV-positive patients on antiretroviral therapy and 20% of those with low T cell counts (Bongomin et al., 2017). Infection with HIV may engender candidiasis by targeting and depleting Candida-specific CD4+ T cells (Liu et al., 2016) and otherwise impacting host responses in such a way that selects for fungal virulence (Cassone and Cauda, 2012). Another risk factor for oral candidiasis is IL-17 blockade used to treat inflammatory diseases (Nash et al., 2018); this is an interesting corollary to the pathways implicated in candidate gene studies of chronic mucocutaneous candidiasis (CMC), as discussed in the section on “idiopathic” infections by Candida spp. Yet, the population “at risk” is much greater than the number affected, implying a possible role for genetic susceptibility.
Studies in animal models of OPC have demonstrated that CD4+ Th17 cells are involved in the control of infection (Hernandez-Santos et al., 2013). Compensatory CD8+ Tc17 cells and innate IL-17-producing cells confer protection in the context of CD4-deficiency (Hernandez-Santos et al., 2013) or loss of pathways required for Th17 responses (e.g., CARD9−/− mice) (Bishu et al., 2014). Type 3 innate lymphoid cells cells (ILC3), natural Th17 cells, and γδT cells are innate sources of IL-17 in this OPC model. Other models suggest a central role for IL-22 signaling in protection against OPC (Goupil et al., 2014), an interesting corollary to observed susceptibilities to RVVC in humans (discussed above). Still, multiple studies have demonstrated that immune responses to Candida spp. at the oral and vaginal mucosas are fundamentally different (Verma et al., 2017; Gao et al., 2019), which may account for some of the differences in the epidemiology of OPC and RVVC.
Attempts to identify human genetic variants associated with opportunistic mucosal candidiasis have yet to report significant risk variants. Candidate gene studies comparing HIV-positive patients who did and did not develop OPC have failed to find significant risks of variants in genes involved in fungal recognition [e.g., CLEC7A (Dectin-1), TLR2, TLR4, TIRAP, CARD9], cytokine production (CASP12), and autophagy (ATG16L1 and IRGM) (Plantinga et al., 2010; Rosentul et al., 2011b, 2014b).
Bloodstream infection with Candida spp. (candidemia) and disseminated candidiasis are feared complications of immunosuppression. Mortality rates of over 20% have been reported (Pagano et al., 2017), despite improvements in prevention and treatment (Yapar, 2014). While several non-genetic risk factors are known (Yapar, 2014), extensive research has addressed the possibility of genetic susceptibility to candidemia and disseminated candidiasis.
Multiple studies have addressed the potential for recognition of Candida ligands to impact risk of invasive disease. Recent reports have detailed a mechanism whereby decreased Dectin-1 signaling confers risk of candidemia. CD82 is involved in the clustering of Dectin-1 receptors, and the efficient activation of intracellular signaling upon ligand binding (Tam et al., 2019). Variants in CD82 are associated with both candidemia risk and decreased cytokine production upon stimulation with fungal ligands (Tam et al., 2019). It is less clear if there is a role for variants in CLEC7A, the gene encoding Dectin-1. A role for CLEC7A-Y238X was proposed after reports that this variant is associated with increased colonization by Candida spp. (Plantinga et al., 2009). However, CLEC7A-Y238X is not associated with risk of invasive infection in studies of patients with candidemia (Plantinga et al., 2009; Rosentul et al., 2011a). Vav proteins are involved in signal transduction following CLR recognition of fungal PAMPs. Roth et al. (2016) report an association between rate of candidemia in a European cohort and a block of variants near Vav proteins, which they further support by demonstrating a critical role for Vav1/2/3 in the murine response to C. albicans and experimental candidemia.
TLRs are also involved in recognition of Candida spp., and variants may confer risk of candidemia. A large study identified three risk variants in TLR1, although these variants were only significant in white donors (Plantinga et al., 2012). The authors validated the functional impact of these variants, and proposed a mechanism for susceptibility involving recognition of Candida by TLR1/TLR2 heterodimers (Plantinga et al., 2012). This study did not identify risk variants in TLR2 or TLR4 (Plantinga et al., 2012), however, earlier studies did report risk variants in these genes (van der Graaf et al., 2006b; Woehrle et al., 2008); all three studies were carried out in European-ancestry donors. Studies have failed to find associations with variants in other PRRs (TLR6, TLR9, MBL2, NOD2), TLR adaptors (MyD88, TIRAP), and FCγRs (FCGR2A, FCGR3A, FCGR3B) (Choi et al., 2005; van der Graaf et al., 2006a; Aydemir et al., 2011; Plantinga et al., 2012). Studies in the same cohorts have failed to find risk or protective variants in genes associated with autophagy (ATG16L1, IRGM), generation of reactive oxygen species (CYBA), or chitin breakdown (CHIT1) (Choi et al., 2005; Rosentul et al., 2014b).
While risk variants impacting recognition of fungi are broadly associated with candidemia, variants impacting phagocyte function have been more closely related to disseminated candidiasis following candidemia. Susceptibility variants have been identified in CXCR1, which is involved with neutrophil killing of yeast (Swamydas et al., 2016), and CX3CR1, which plays a role in macrophage chemotaxis and survival (McDermott et al., 2003; Lionakis et al., 2013; Collar et al., 2018). These studies have been done in European-ancestry populations; CX3CR1-M280, the risk variant, is not associated with invasive candidiasis in African American donors (Lionakis et al., 2013), or RVVC in European donors (Break et al., 2015). A mouse model recapitulated the role of CX3CR1 in decreasing macrophage survival, thereby engendering susceptibility to invasive infection but not mucosal candidiasis (Lionakis et al., 2013; Break et al., 2015). Interestingly, CX3CR1-M280 was first recognized as a protective variant for cardiovascular disease (McDermott et al., 2003). The diverse findings concerning CX3CR1-M280 provide a good example of how susceptibility variants may be highly context specific.
Cell-mediated immunity may play a role in chronic disseminated candidiasis (CDC) in the context of neutropenia. Both a protective haplotype and a risk haplotype were identified in IL4 in neutropenic patients (Choi et al., 2003). The protective haplotype was associated with decreased IL-4 production, suggesting that Th2 responses are deleterious in CDC (Choi et al., 2003). Other polymorphisms that impact cytokine production are associated with persistent candidemia, although not studied in the context of neutropenia. Two polymorphisms, one in IL10 and one in IL12B, are associated with persistent candidemia, but not candidemia in general (Johnson et al., 2012). The authors report functional effects from these risk alleles: increased IL-10 production and decreased IFN-γ production, respectively (Johnson et al., 2012). This suggests a detrimental role of Treg cells and a beneficial role of type 1 T cell responses in limiting the duration of candidemia. Interestingly, there was no significant association between genotype at IL10 and CDC in neutropenic patients, although both studies were carried out in European ancestry populations (Choi et al., 2003; Johnson et al., 2012). Studies have failed to find significant associations with CDC or candidemia for several other cytokines (IFNG, IL1B, IL8, IL12A, IL18, TGFB1, TNF), a cytokine receptor (IL12RB1), and a gene involved in cytokine processing (CASP12) (Choi et al., 2003; Johnson et al., 2012; Rosentul et al., 2012).
Unbiased studies that sought to overcome the limitations of candidate gene studies have implicated viral recognition and response pathways in candidemia. One unbiased approach is to identify novel candidate genes by using a hypothesis-generating technique such as RNAseq, then applying these findings to the analysis of a patient cohort. This workflow was used to identify a linked block of variants at the gene encoding RIG-I-like receptor (RLR) MDA5 in association with candidemia (Jaeger et al., 2015). Another study using a similar approach implicated type I interferon signaling in the response to Candida spp. (Smeekens et al., 2013). The authors then studied signaling downstream of type I interferon, and reported that STAT1 polymorphisms are associated with candidemia (Smeekens et al., 2013).
Another unbiased strategy is to study patient genomes directly with a GWAS. A GWAS with 217 European-ancestry candidemia patients reported candidate risk variants near the genes encoding CD58, TAGAP, and the LCD4A-C1orf68 locus (Kumar et al., 2014). The authors note that linked variants at the CD58 locus fall near several non-coding RNAs, and found that genotype at CD58 correlated with cytokine production by macrophages in response to Candida but not LPS (Kumar et al., 2014). TAGAP contributes to T cell trafficking in the thymus and negative selection (Duke-Cohan et al., 2018). All three candidate loci have been associated with autoimmunity (Kumar et al., 2014). This GWAS dataset has been used in two further studies. Using a new approach to analyze the data, Matzaraki et al. (2017) identified 18 candidate risk variants and 31 candidate susceptibility genes. The authors noted that 9 of 31 candidate genes are involved with the complement system or coagulation, and validated a functional variant in MAP3K8 (Matzaraki et al., 2017). Finally, Li and Oosting et al. found that variants at Golgi membrane protein 1 (GOLM1) are cytokine quantitative trait loci (cQTL) for IL-6 (Li et al., 2016); these cQTL are associated with risk of candidemia and lower levels of IL-6 in candidemia patient serum (Li et al., 2016).
Candida spp. are a rare cause of serious or invasive fungal infections in otherwise healthy people. People suffering from such “idiopathic” infections have been the subject of extensive research revealing genetic deficits in immunity (Li et al., 2017). As reviewed above, these deficits are understood to be the result of rare, monogenic polymorphisms or mutations that are overwhelmingly deleterious, and thus under strong negative selection (Netea et al., 2012; Li et al., 2017). These conditions provide an invaluable glimpse into mechanisms of antifungal immunity. The clinical manifestation of a given immune deficit illustrates areas of antifungal immunity that are non-redundant (Netea et al., 2012). Many monogenic conditions result in Chronic Mucocutaneous Candidiasis (CMC), which involves recurrent and persistent Candida spp. infections of the mucosa and skin (Li et al., 2017). Others result in invasive candidiasis. This topic has been the subject of recent excellent reviews, and the treatment here will thus be brief (Li et al., 2017; Lionakis and Levitz, 2018).
SCID patients, who lack an adaptive immune system, develop mucosal infection and diarrhea from Candida spp., in addition to multiple other infections. SCID occurs in about 1/65,000 live births (Amatuni et al., 2019), and can result from loss-of-function mutations and variants that affect the development, proliferation, or survival of T cells and/or B cells (Buckley, 2004; Heimall et al., 2017; Lionakis and Levitz, 2018; Aluri et al., 2019). Increasingly, SCID patients are being recognized by newborn screening (before infection) and are treated with stem cell transplant, which will likely improve outcomes (Heimall et al., 2017). Even with prompt diagnosis from newborn screening or family history, Candida spp. cause infection in 8% of patients with SCID in the first few months of life, prior to transplant (Heimall et al., 2017). Untreated, SCID is associated with OPC in ~20% of patients and chronic diarrhea, which may be caused by C. albicans (Aluri et al., 2019; Parvaneh et al., 2019). Interestingly, patients with untreated SCID are reported to have C. parapsilosis as a commensal without apparent symptoms, and to have a higher frequency than healthy controls of C. parapsilosis culturable from feces (Taylor et al., 1985).
Patients with severely decreased Th17 cell counts suffer from CMC, even in the absence of more global T cell or B cell deficits (Li et al., 2017; Lionakis and Levitz, 2018). Interestingly, PIDs that selectively impact Th17 cells and IL-17 signaling are associated with fewer opportunistic bacterial infections (Li et al., 2017; Lionakis and Levitz, 2018). Patients with Hyper-IgE Syndrome (HIES), also known as Job's syndrome, have impaired STAT3 signaling and diminished Th17 cell responses, and are vulnerable to CMC (Ma et al., 2008; Milner et al., 2008; Zhang et al., 2018). While STAT3 is critical in signal transduction for several cytokines, it appears that the critical deficit that confers susceptibility to CMC is lack of IL-17-producing cells per se (Ma et al., 2008; Hillmer et al., 2016; Zhang et al., 2018). This is illustrated by the clinical presentations of patients with genetic deficiency in other cytokine-signaling components. Loss-of-function mutations in IL6ST, which codes a critical subunit of the receptors for IL-6 and multiple other cytokines that signal through STAT3, result in elevated IgE and susceptibility to infections, but IL-17 responses are relatively intact and CMC is absent (Schwerd et al., 2017; Shahin et al., 2019). Deficiency in TYK2, an intracellular adaptor for multiple cytokine receptors that signal via STAT3, is associated with multiple susceptibilities, but normal Th17 responses and, in most cases, no apparent increased risk of candidiasis (Kreins et al., 2015). However, mutations that lead to decreased numbers of IL-17-producing cells are also associated with CMC susceptibility; these include gain-of-function mutations affecting STAT1 (Zheng et al., 2015; Eren Akarcan et al., 2017; Mogensen, 2018) and loss-of-function mutations affecting RORγ/RORγT (Okada et al., 2015).
Impaired IL-17 signaling due to mutations in cytokines and cytokine receptors is also associated with susceptibility to CMC. The six members of the IL-17 cytokine family (IL-17A through IL-17F) are recognized by dimeric receptors composed of the five receptor subunits in the IL-17R family (IL-17RA through IL-17RE), with an intracellular adaptor (ACT1) (Monin and Gaffen, 2018). Loss-of-function mutations conferring susceptibility to CMC have been reported in IL17F, IL17RA, IL17RC, and ACT1 (Li et al., 2017; Monin and Gaffen, 2018). An interesting corollary is the high degree of CMC seen patients with Autoimmune Polyendocrine Syndrome Type 1 (APS-1), who produce autoantibodies that neutralize IL-17 cytokines due to mutations in the autoimmune regulator gene (AIRE) (Humbert et al., 2018).
Mutations affecting neutrophil function confer susceptibility to invasive candidiasis, in contrast to the selective susceptibility to mucosal candidiasis associated with loss of adaptive immunity and IL-17 signaling. Patients with Chronic Granulomatous Disease (CGD) suffer from invasive fungal disease due primarily to filamentous fungi, although invasive candidiasis is also reported (Wolach et al., 2017; Kanariou et al., 2018; Lionakis and Levitz, 2018). CGD results from mutations causing defective NADPH oxidase, which abrogate the oxidative burst required for phagocytes to kill microorganisms (Wolach et al., 2017; Lionakis and Levitz, 2018).
A relatively common genetic condition that predisposes patients to invasive candidiasis is myeloperoxidase (MPO) deficiency. MPO deficient cells are unable to generate HOCl, although generation of an oxidative burst is unaffected (Klebanoff et al., 2013). Neutrophils from patients with MPO deficiency display delayed killing, unlike those from patients with CGD, which fail to kill microbes at all (Klebanoff et al., 2013). Patients with MPO deficiency are narrowly susceptible to Candida spp.; still, only a small fraction of patients ever develop invasive candidiasis (Klebanoff et al., 2013; Lionakis and Levitz, 2018). This may indicate that early killing of phagocytosed pathogens is critical for control of Candida spp., but only after other defenses have been overcome (e.g., barrier integrity) (Klebanoff et al., 2013).
Loss-of-function mutations affecting CARD9 are unique in their association with susceptibility to both CMC and invasive candidiasis (Lionakis and Levitz, 2018). CARD9 deficiency abrogates fungal recognition by CLRs and downstream neutrophil responses, with little or no impact on bacterial recognition (Drewniak et al., 2013). There are conflicting reports on whether Th17 responses are impacted by loss of CARD9 in humans (Drewniak et al., 2013; Drummond et al., 2015; Vaezi et al., 2018). Over 40% of patients with CARD9 deficiency present with infections by Candida spp. (Vaezi et al., 2018); a large portion of those patients present with Candida spp. infecting their central nervous system (CNS) (Lanternier et al., 2015; Vaezi et al., 2018). Information from case reports of CARD9 deficient patients, plus studies of CNS C. albicans infection in CARD9−/− mice, suggests that the predilection for CNS infections is due to impaired recognition of fungi by microglia that in turn leads to diminished recruitment of neutrophils (Drummond et al., 2018, 2019).
CARD9 has two binding partners, MALT1 and BCL10, that are required for signal transduction (Zhong et al., 2018). The few patients reported with loss-of-function mutations in these proteins presented with combined immunodeficiency, with the implication that MALT1 and BCL10 are indispensable in the proper development of memory T cells (Torres et al., 2014; Punwani et al., 2015). These patients also had histories of mucosal candidiasis (Torres et al., 2014; Punwani et al., 2015); it is difficult to distinguish whether fungal susceptibility was due to impaired CARD9 signaling or secondary to deficits in adaptive immunity in these patients.
Candida spp. cause a broad spectrum of infectious diseases, and a growing body of evidence reveals differences in host response and susceptibility across this spectrum. RVVC is a primary illness, largely due to C. albicans. Perturbations of innate immunity, especially involving events at the mucosa, appear to confer susceptibility to RVVC. This includes fungal recognition, IL-22 signaling, and possibly, mucosal fucosylation. Factors that influence susceptibility to candidiasis at the oral mucosa are different; in the absence of T cells, patients are highly vulnerable to OPC and OEC. There is not yet a clear role for genetic susceptibility in modifying risk of mucosal candidiasis in the setting of immunosuppression and immunodeficiency.
A number of immune processes have been implicated in genetic susceptibility to invasive candidiasis. These again include recognition of fungal ligands, although by different receptors than those implicated in RVVC. Mild impairment of neutrophil recruitment may predispose patients to disseminated candidiasis, and in the absence of neutrophils, defects in the development of adaptive immunity may also be a risk factor. Recently, unbiased approaches have uncovered diverse processes that could contribute to genetic susceptibility, such as antiviral responses and trans-regulation of cytokine production.
Rare, “idiopathic” infections by Candida spp. have been extensively studied. Deficits in cellular immunity predispose patients to CMC, while deficits in innate responses, especially in the ability of neutrophils to kill yeast, predispose to invasive disease. CARD9 deficiency is notable in causing both.
Aspergillus spp. are filamentous fungi that are associated with the ecological niche of saprophyte. They spread by spores, which reach great density in association with soils or decomposition and readily become airborne (Williams et al., 2019). In indoor environments, Aspergillus spores are associated with water-damaged structures and potted plants (Mousavi et al., 2016). Even with efforts to limit airborne contaminants, it is difficult to exclude Aspergillus spores from healthcare environments (Oberle et al., 2015).
Aspergillus spp. are associated with a number of different disease states. There are more than 11 million people who suffer from fungal allergic asthma, associated with Aspergillus (Bongomin et al., 2017). Genetic predisposition to allergic asthma will not be discussed here; we instead focus on diseases caused by Aspergillus infection per se. There are an estimated 3 million cases of chronic pulmonary aspergillosis (CPA) worldwide (Bongomin et al., 2017). CPA is often comorbid with chronic respiratory conditions that affect the structure or function of the lungs (Bongomin et al., 2017). Invasive aspergillosis (IA) affects some 300,000 people annually (Bongomin et al., 2017). IA is typically associated with profoundly immune compromised states; still, there is growing appreciation that patients with multiple comorbid conditions, such as chronic obstructive pulmonary disease (COPD) plus diabetes, are predisposed to IA and undercounted in estimates of prevalence of this infection (Bao et al., 2017; Bongomin et al., 2017).
Further complicating an understanding of these infections is the fact that multiple Aspergillus species are capable of causing illness. Biological differences between these species, plus subtle differences in the immunosuppressed states of different patient populations may contribute to the relative likelihood that IA is caused by A. fumigatus vs. other Aspergillus spp.; for example, IA in neutropenic patients is predominantly caused by non-fumigatus Aspergillus spp., but in bone marrow transplant patients, A. fumigatus was the most common species (Torres et al., 2003). This distinction has clinical relevance, as the species causing non-fumigatus aspergillosis tend to have higher minimum inhibitory concentration and minimum fungicidal concentration for clinically important antifungals (Torres et al., 2003).
Studying genetic predisposition to CPA is complicated by the heterogeneity of the patient populations and presentation of illness. The clinical spectrum of CPA includes aspergilloma and chronic cavitary pulmonary aspergillosis (CCPA), and at-risk patients include those with COPD, sarcoidosis, and chronic pulmonary infections that cavitate such as tuberculosis (Bongomin et al., 2017). While these conditions may have a component of immune dysregulation, patients with these conditions are usually not immune compromised in the classic sense.
Studies in a cohort of patients in Manchester, UK, suggest that CCPA is associated with an overly-active immune response to fungi. Macrophages from CCPA patients showed greater up-regulation of cytokines at the experiment's endpoint as compared to healthy donor cells, though CCPA patient cells also had greater lag time in their response to fungal stimuli (Smith et al., 2014a). CCPA patient cells had different PRR expression profiles as compared to healthy donor cells (Smith et al., 2014a). These authors identified risk variants in genes encoding cytokines (IL1B, IL1RN, IL15), PRRs [TLR1, CLEC7A (Dectin-1)], and the genes DENND1B, PLAT (plasminogen activator), and VEGFA (Smith et al., 2014a,b). The association between CPA and hyper-inflammatory states is supported by a study of CPA patients in Shanghai, China, which found that greater levels of IL-1β are correlated with markers of advanced illness such as aspergilloma size (Zhan et al., 2018).
A study of COPD patients with CPA suggests that infection in this context may be associated with decreased recognition of fungi. A variant in the gene encoding the PRR pentraxin 3 (PTX3) is associated with the development of CPA in a cohort of Chinese COPD patients (He et al., 2018). This risk variant is associated with decreased pentraxin 3 in patient plasma, but this association was not found in the group with CPA alone (He et al., 2018). Variants in PTX3 have been extensively studied in relation to invasive aspergillosis and are discussed further below.
Immune compromise is almost essential for IA. IA is most often associated with allogeneic hematopoietic stem cell transplant (HSCT), and almost a quarter of HSCT patients develop IA in some studies (Robin et al., 2019). Patients undergoing allo-HSCT experience an initial risk at transplant, as well as a late risk associated with milder immunosuppression from graft-versus-host disease (GVHD) (Robin et al., 2019). In all cases, studying genetic risk in allo-HSCT patient populations is complicated by the presence of both donor and recipient genetics.
Mounting evidence suggests that variants associated with weaker recognition of fungi by the innate immune system are associated with greater risk of IA. Perhaps the best studied gene in this regard is pentraxin 3, a soluble PRR that recognizes Aspergillus conidia (Garlanda et al., 2002). PTX3−/− mice are unable to control Aspergillus infection, with deficits noted in phagocyte function, as compared to wildtype mice (Garlanda et al., 2002). Multiple studies have associated PTX3 variants that result in decreased expression with risk of IA in HSCT patients (Cunha et al., 2014; Fisher et al., 2017; Herrero-Sanchez et al., 2018). Donor PTX3 genotype determined risk, and recipient PTX3 genotype had no significant effect (Cunha et al., 2014; Fisher et al., 2017).
Linked TLR4 coding variants D299G-T399I, which result in decreased signaling by TLR4 (Arbour et al., 2000), have also been implicated in IA risk following allo-HSCT. Risk variants are either significant only considering donor TLR4 genotype (Bochud et al., 2008; de Boer et al., 2011), or, in one study, considering either donor or recipient TLR4 genotype (Koldehoff et al., 2013). Several studies, including the largest study of genetic risk of IA in allo-HSCT patients, have failed to detect a significant risk of the TLR4-D299G-T399I genotype (Kesh et al., 2005; Grube et al., 2013; Fisher et al., 2017); at least in some studies, this may be attributable to low frequency of the risk genotype (Fisher et al., 2017). HSCT recipients with the TLR5-Stop variant, missense variant TLR1-R80T, or both missense variants TLR1-N248S and TLR6-S249P together are at increased risk of IA (Kesh et al., 2005; Grube et al., 2013). These associations seem to arise from fungal recognition by non-hematopoietic-lineage cells, as there is no significant effect on risk of IA by HSCT donor genotype at variants in TLR1, TLR5, and TLR6 (Kesh et al., 2005; Grube et al., 2013). Variants in the genes S100B and RAGE also predispose allo-HSCT patients to IA by a mechanism thought to involve hyperactivity of this innate recognition pathway, which results in TLR2 blockade and TLR3 and TLR9 activation (Cunha et al., 2011; Sorci et al., 2011). Variants associated with risk were found either in HSCT donors (S100B and RAGE), or recipients (S100B) (Cunha et al., 2011). There are conflicting reports as to the association of CLEC7A-Y238X with IA risk in HSCT; two studies have correlated risk of IA with either recipient or donor carrying this variant (Cunha et al., 2010; Fisher et al., 2017), while others have failed to detect an effect (Chai et al., 2011; Herrero-Sanchez et al., 2018).
Several other innate receptor variants increase the risk of IA in the setting of HSCT. The CLR MelLec, which recognizes fungal DHN-melanin, has been implicated in IA risk upon HSCT. HSCT donor genotype at MelLec is associated with risk, while recipient genotype is not (Stappers et al., 2018). Macrophages of the risk MelLec genotype produce less pro-inflammatory cytokine in response to A. fumigatus conidia than do cells of the alternate genotype (Stappers et al., 2018). In contrast, IA rates are higher in HSCT donors that harbor a pro-inflammatory variant of NOD2, an intracellular PRR, whereas genetic deficiency of NOD2 confers resistance to IA (Gresnigt et al., 2018). While not innate fungal recognition per se, a coding variant in plasminogen has been implicated in IA risk; the risk is significant when considering HSCT recipient genotype and not donor genotype, which is consistent with the hepatic origin of plasminogen (Zaas et al., 2008). The authors suggest that differences in interaction between plasminogen and Aspergillus conidia could lead to differences in local inflammation and pathogen entry (Zaas et al., 2008). Considered intracellular signaling downstream of PRRs, one study of variants in multiple NF-κB-related genes failed to uncover significant associations with risk of IA in HSCT patients, although there may have been a weak association with a haplotype at IRF4 (Lupianez et al., 2016).
Multiple variants associated with risk of IA following HSCT have been identified in genes involved in the development of appropriate T cell responses. A haplotype in CXCL10 is associated with risk of IA following HSCT (Mezger et al., 2008). Cells with the risk haplotype were found to produce less CXCL10 upon stimulation with A. fumigatus germlings than cells with the alternate haplotype (Mezger et al., 2008). One role of CXCL10 is recruitment of Th1 cells, thus the risk haplotype may be associated with a relatively weaker Th1 response. The AspBIOmics Consortium uncovered several IA risk variants that could be associated with altered T cell responses; these associations were stronger in HSCT patients than in the larger pool of at-risk hematology patients (Lupianez et al., 2016). Risk variants were identified in IL4R, IL8, IL12B, and IFNG, with experiments demonstrating functional effects of the IFNG variant on IFNγ production and related endpoints (Lupianez et al., 2016). A risk variant that increases IL-10 production would suppress T cell responses. Donor genotype at a variant upstream of IL10 is associated with development of IA, and the risk genotype leads to increased production of the anti-inflammatory cytokine IL-10 (Cunha et al., 2017). Mouse models of Aspergillus infection support this finding; IL10−/− animals are more resistant to experimental aspergillosis (Clemons et al., 2000). Taken together, these findings suggest that variants that lead to relatively weaker Th1 responses may predispose HSCT patients to IA.
Much of what is known about genetic predisposition to IA following HSCT has been reiterated in studies of other vulnerable populations. PTX3 variants are associated with risk of IA in patients with COPD (He et al., 2018), or following solid organ transplant (Wojtowicz et al., 2015b). A small study of patients with hematologic malignancies found that expression of S100B was significantly associated with IA (Dix et al., 2016). Three studies have demonstrated that CLEC7A (Dectin-1) or CD209 (DC-SIGN) variants are associated with the development of IA in patients with hematologic malignancies (Oberhofer, 1979; Sainz et al., 2010b, 2012), and this is further supported by a study finding decreased CLEC7A expression in hematologic malignancy patients with IA (Camargo et al., 2015). While genetic studies have not been done, MBL levels are lower in immunocompromised patients with IA vs. febrile immunocompromised controls (Lambourne et al., 2009). As discussed in the section on candidiasis, loss-of-function MBL variants predispose patients to RVVC, but no association has been found for candidemia. The risk associated with relatively greater production of IL-10 was reiterated in a study of patients with hematologic malignancies, including some that had undergone HSCT (Sainz et al., 2007a).
Recent work has also addressed the role of early responses, especially cytokine signaling, in predisposition to IA in high-risk populations aside from HSCT patients. Variants impacting IL-1β are reported to influence risk of IA in patients with hematologic malignancies, and following solid organ transplant; the genes harboring risk variants include IL1A, IL1B, IL1RA, and IL1RN (Sainz et al., 2008; Wojtowicz et al., 2015a). When stimulated with A. fumigatus conidia, cells harboring these variants produce less of several cytokines, including IL-1β and TNF-α, than do control cells (Wojtowicz et al., 2015a). Signaling through TNF has also been implicated in two studies of patients with hematologic malignancies, some of whom underwent HSCT; these studies report significantly higher rates of IA in patients with genetic variants that decrease expression of TNFR1 and TNFR2 (Sainz et al., 2007b, 2010a; Li and Anderson, 2018). One report also identified a variant in the promoter of DEFB1, encoding β-defensin 1, associated with IA in solid organ transplant patients (Wojtowicz et al., 2015a). As with IL-1β and TNF-α, β-defensin 1 helps coordinate the early immune response by acting as a chemotactic signal. Direct anti-Aspergillus activity of β-defensin 1 has not been investigated.
Aspergillus infection in otherwise healthy people is rare, and generally associated with monogenic susceptibility. The best example of this is Aspergillus infections in patients with ineffective neutrophil oxidative bursts due to CGD; these patients are at risk for both pulmonary and disseminated aspergillosis (Wolach et al., 2017). CARD9 deficiency has also been identified in patients who present with extrapulmonary Aspergillus infection without evidence of pulmonary aspergillosis (Rieber et al., 2016). HIES, which is STAT3 deficiency, is associated with susceptibility to invasive aspergillosis (Vinh et al., 2010; Dureault et al., 2019). Interestingly, CARD9- and STAT3-deficient patients are similar to control donors in terms of neutrophil responses to A. fumigatus (Vinh et al., 2010; Rieber et al., 2016).
It is not clear whether STAT3 deficiency alone is sufficient to predispose patients to aspergillosis. HIES patients develop aspergillosis later in life, after suffering other structural insults to the lung (Dureault et al., 2019). Extrapulmonary aspergillosis is reported, however, in association with STAT3 haploinsufficiency that did not appear to impact antifungal Th17 responses (Natarajan et al., 2018). STAT3 is pleiotropic, and dozens of mutations in STAT3 are associated with varied impacts on the final gene product (Vogel et al., 2015); future reports may clarify the spectrum of disease that results from select mutations.
Aspergillosis encompasses varied clinical manifestations, and the differences are manifest in the genetic predisposition to different forms of Aspergillus infection; from hyper-inflammatory responses that predispose to CPA to ineffective fungal recognition that predispose to IA. Despite the difficulty of studying these varied illnesses, progress has been made toward identifying and validating risk variants, especially in the context of HSCT. In the coming years, this avenue of research holds great promise for improving HSCT outcomes, both by informing donor selection and by proactively identifying those recipients at the highest risk of IA.
Dimorphic fungal pathogens that are native to North America, including the genera Histoplasma, Coccidioides, and Blastomyces, infect hundreds of thousands of people per year (Bongomin et al., 2017). Yet, the majority of these infections are asymptomatic. Puzzlingly, many of those who develop symptoms do not appear to be at increased risk for fungal infection; they are young and seemingly healthy. Additionally, each of these fungal infections has a higher incidence in select groups, especially people of African, Native American, or Asian ancestry. On the basis of these two observations, a significant role for host genetics has long been supposed for these infections.
Histoplasmosis is the most prevalent of the dimorphic fungal infections, with an estimated 500,000 infections per year (Bongomin et al., 2017). As with the other dimorphic fungi, Histoplasma capsulatum grows in organic soils; outbreaks have been associated with disturbances that lead to aerosolization of infectious spores, e.g., construction in both rural and urban settings (Deepe, 2018). H. capsulatum is also associated with bird and bat droppings; thus, outbreaks have been associated with clearing bird waste and disturbing bat guano in caves and tunnels (Deepe, 2018). Although the geographic distribution of H. capsulatum is often quoted to be the Ohio River Valley, suitable environmental conditions exist to support the fungus throughout the upper Midwest (Maiga et al., 2018).
The vast majority of exposures to H. capsulatum do not result in clinical illness. In fact, virtually the entire population in some highly endemic areas may be repeatedly exposed (Goodwin et al., 1981). Pulmonary nodules are a common incidental finding on medical imaging of patients living in endemic areas; in highly endemic areas, up to 12% of these nodules may be associated with infection with H. capsulatum (Benedict et al., 2019; Deppen et al., 2019). Such latent infections are usually asymptomatic, although they may present as invasive disease decades later upon immunosuppression (Bourgeois et al., 2011). In immunocompetent individuals, histoplasmosis most commonly manifests as isolated fungal pneumonia (Ouellette et al., 2018), and acute illness is often associated with high levels of exposure to infectious spores (Deepe, 2018). There is a noted racial health disparity, with historical reports of higher incidences of histoplasmosis amongst black people in outbreaks (Wheat et al., 1981); however, these reports may be confounded by geographical factors.
Histoplasmosis poses a threat to organ transplant recipients (Tanveer et al., 2019) and patients with AIDS (Assi et al., 2006; Wheat et al., 2018). Disseminated histoplasmosis is most commonly associated with the immunocompromised patient, and rare in immunocompetent patients (Ouellette et al., 2018). Sites of dissemination include the central nervous system (Wheat et al., 2018) and gastrointestinal tract (Assi et al., 2006). Another recognized risk of disseminated histoplasmosis is TNF-α blockade, for example in the treatment of inflammatory bowel disease (Smith and Kauffman, 2009; Vergidis et al., 2015). As with overall risk of infection, black race has been associated with increased risk of severe histoplasmosis in AIDS patients (Wheat et al., 2000). There are currently no reports addressing genetic determinants of racial disparities in histoplasmosis pneumonia or disseminated histoplasmosis.
Mouse models of histoplasmosis have recapitulated many of the features of human susceptibility. TNF-α is required for the establishment of memory and control of infection (Zhou et al., 1998; Deepe, 2006). Type 1 cytokines are indispensable to control of infection (Allendoerfer and Deepe, 1997), and Th17 cells contribute to protective immunity (Deepe et al., 2018). However, IL-17 responses are ultimately not required for survival of infection in the mouse model (Deepe and Gibbons, 2009). Th2 responses prolong illness, perhaps by delaying the development protective Th1 cells (Gildea et al., 2003).
Case reports concerning patients with mutations that affect immunity further elucidate the immune responses that are required for control of H. capsulatum. Patients with deficient type 1 cytokine responses are at risk for disseminated histoplasmosis; such deficiencies include loss-of-function mutations impacting IFNγR1 (Zerbe and Holland, 2005) and IL12Rβ1 (Rosain et al., 2018). Histoplasmosis is reported in patients with HIES resulting from loss-of-function mutations in STAT3 (Robinson et al., 2011; Odio et al., 2015), as well as gain-of-function mutations in STAT1 (Sampaio et al., 2013); this could be related to impaired Th17 responses, other perterbations resulting from loss of these transcription factors, or a combination of Th17-dependent and Th17-independent factors. Patients lacking NEMO, a component of NF-κB signaling (Lovell et al., 2016), or GATA2, a transcription factor involved in the differentiation of myeloid cells (Collin et al., 2015) are also very susceptible to disseminated histoplasmosis. This could be due in part to impaired macrophage and monocyte responses, including signaling downstream of TNF-α; however, loss of NEMO or GATA2 result in very complicated immune disturbances, so it is not possible to attribute this susceptibility to any one mechanism.
While coccidioidomycosis is a clinical entity similar to histoplasmosis and blastomycosis, the genus Coccidioides is more closely related to the causative agents of dermatophytosis than to other dimorphic fungal pathogens (White et al., 2014; Whiston and Taylor, 2015). This may account for subtle differences in environmental niche and antifungal immunity against Coccidioides spp., compared to Histoplasma spp., and Blastomyces spp. Coccidioidomycosis is mainly reported in the southwestern United States, and is associated with a complex set of climactic conditions including alternating wet and dry periods (Shriber et al., 2017).
There are an estimated 25,000 cases of coccidioidomycosis that require medical treatment per year (Bongomin et al., 2017), with an incidence of more than 40 cases per year per 100,000 population in endemic states (Centers for Disease Control Prevention, 2013). Up to 29% of community-acquired pneumonia may be due to Coccidioides spp. in highly endemic areas (Valdivia et al., 2006). Still, a minority of those who are exposed to Coccidioides spp. develop acute illness; the seropositivity rate to the fungus is about 30% in endemic areas (Dodge et al., 1985), with reports of over half of the population being reactive to Coccidioides antigen in some areas (Nguyen et al., 2013). Chronic infection is common, with up to 29% of lung nodules being attributable to Coccidioides spp. in endemic areas (Forseth et al., 1986).
Progression to disseminated coccidioidomycosis is rare, occurring in fewer than 1% of infections (Stevens, 1995). Immunosuppression increases risk of dissemination, as does use of TNF-α inhibitors (Bergstrom et al., 2004; Blair et al., 2019). It has long been recognized that the risk of dissemination is not equal across people of different races and ethnicities. Native American (McCotter et al., 2019), African American (Ruddy et al., 2011), and Pacific Islander (Drake and Adam, 2009) ancestry all confer increased risk of dissemination.
In mouse models of coccidioidomycosis, recognition of the fungus by Dectin-1 is required to control infection (Viriyakosol et al., 2013; Feriotti et al., 2015), as is downstream signaling by CARD9 (Hung et al., 2014). MyD88 is also required for the induction of protective T cell responses (Hung et al., 2014; Viriyakosol et al., 2018). Considering the critical role of MyD88, it is surprising that TLR recognition of fungal ligands is largely dispensable; instead, the role of MyD88 may reflect signaling downstream of the IL-1β receptor (Hung et al., 2014; Viriyakosol et al., 2018). Th1 and Th17 responses contribute to control of infection (Hung et al., 2011). While Th1 responses are dispensable, Th17 responses are not (Hung et al., 2011).
Many of the same genetic deficits in immunity that predispose patients to disseminated histoplasmosis also confer risk of disseminated coccidioidomycosis. There are case reports to this effect for loss-of-function mutations impacting IFNγR1 (Vinh et al., 2009), IL12Rβ1 (Vinh et al., 2011), STAT3 (Odio et al., 2015), and gain-of-function in STAT1 (Sampaio et al., 2013). Interestingly, based on a small number of cases reported to date, it appears that the fungus may disseminate preferentially to distinct sites depending on which component of immunity is impaired. STAT3 mutations are associated with dissemination to the CNS, while mutations that more directly impact Th1 responses are associated with dissemination to bone and lymph node (Odio et al., 2015).
A few reports have addressed population-level patterns of susceptibility to disseminated coccidioidomycosis. Some of the risk may be attributable to differences in antigen presentation, as certain HLA alleles are associated with dissemination in specific populations (Louie et al., 1999). More recently, a preliminary description of a genomic study of 58 patients with disseminated coccidioidomycosis identified 103 rare variants in 21 genes associated with antifungal immunity (Hung et al., 2019). Affected pathways included IL-17 signaling, IL-12-IFN-γ signaling, and NF-κB signaling (Hung et al., 2019). Two thirds of patients had functionally relevant variants with a population frequency of <0.1%, including several patients with biallelic deleterious variants in key genes (Hung et al., 2019).
The ecology of Blastomyces spp. is somewhat enigmatic, due largely to difficulty in isolating the fungus from the environment (Reed et al., 2008). The microbial physiology of Blastomyces spp. suggests they are adapted for woody substrates and possibly animal waste (Baumgardner and Laundre, 2001). Blastomyces dermatitidis and the closely related B. gilchristii are classically associated with waterways (Reed et al., 2008; McTaggart et al., 2016). The two species have somewhat different distributions, with B. dermatitidis found throughout eastern North America and B. gilchristii largely restricted to Canada (McTaggart et al., 2016). Blastomyces strains isolated from patients cluster by clinical presentation, suggesting a contribution of fungal genetics to clinical outcomes (Meece et al., 2013).
Most cases of blastomycosis are associated with outdoor activities involving exposure to soil (Klein et al., 1986; Choptiany et al., 2009). While outdoor recreation or such activities as forestry are a common source of exposure, blastomycosis is also reported from the urban setting (Pfister et al., 2011). Periodic outbreaks of blastomycosis are associated with a point source where multiple people are exposed to the infectious spores (Pfister et al., 2011); multifocal outbreaks have also been described, and may be associated with environmental factors that produce ideal conditions for sporulation or aerosolization of spores (Roy et al., 2013). As with the other dimorphic fungal infections, evidence suggests that exposure to Blastomyces spp. is more common than appreciated in endemic areas, and that the majority of these exposures do not lead to clinical presentation (Vaaler et al., 1990).
The majority of blastomycosis cases present as fungal pneumonia (Baumgardner et al., 1992; Castillo et al., 2016). Though blastomycosis is comparatively uncommon, with perhaps only 3000 cases reported annually (Bongomin et al., 2017), in highly endemic areas, the incidence may reach 40 cases per year per 100,000 population at risk (Baumgardner and Brockman, 1998). The majority of cases are in people under the age of 50 who are immunocompetent (Baumgardner et al., 1992). Blastomycosis is more common in people of African American, Native American, and Asian, especially Hmong, ancestry (Howard, 1984; Baumgardner et al., 2002; Roy et al., 2013; Khuu et al., 2014). During outbreaks, the incidence of blastomycosis in these select populations is reported to be as high as 277 cases per year per 100,000 populaton at risk (Baumgardner et al., 2002). Some of these associations may be due to a combination of genetic and non-genetic factors. However, comorbidity and exposure risk were recently excluded as contributors to a greatly increased incidence rate of blastomycosis during investigation of a large Wisconsin outbreak that included Asian people, strongly implying a genetic risk (Roy et al., 2013).
There is substantial heterogeneity in the clinical presentation of blastomycosis. A minority of patients present with severe pneumonia, even Acute Respiratory Distress Syndrome (ARDS); this is thought to be a function of exposure to an especially large inoculum in many of the cases (Castillo et al., 2016). From 15 to 40% of patients develop blastomycosis outside of the lung, with the most common site of dissemination being the skin (Baumgardner et al., 1992; Castillo et al., 2016). Immunosuppression is a risk factor for both ARDS and dissemination, although there is not an apparent, overall increased risk of infection (Choptiany et al., 2009; Castillo et al., 2016). Limited data suggest that patients on TNF-α inhibitors may be more susceptible to infection (Castillo et al., 2016).
Studies in a mouse model of blastomycosis have revealed a critical role for early responses by lung epithelial cells (Hernandez-Santos et al., 2018). MyD88 is indispensable in the response to B. dermatitidis; MyD88−/− mice succumb to lethal infection even by an attenuated vaccine strain that is non-lethal in wild-type mice (Wang et al., 2016). Signaling through IL-1R1 drives protective Th17 immunity (Wuthrich et al., 2013; Wang et al., 2014; Hernandez-Santos et al., 2018). Interestingly, Tc17 cells confer durable protection in CD4-deficient animals (Nanjappa et al., 2012). Innate, IL-17-producing lymphocytes also play an important role (Hernandez-Santos et al., 2018). As with the other dimorphic fungal infections, TNF-α coordinates antifungal immunity to blastomycosis (Finkel-Jimenez et al., 2001).
Recently, work has addressed the genetic underpinning of health disparities in rates of blastomycosis in otherwise healthy individuals. A homozygosity mapping approach was used to study 9 Hmong blastomycosis patient genomes, and candidate susceptibility variants were identified on the basis of their rarity in European populations and other features that may indicate that a given variant influences susceptibility (Merkhofer et al., 2019). This approach revealed 113 candidate susceptibility variants. The authors validated the impact of a block of variants near IL6 that is nearly fixed in Wisconsin Hmong at large but rare in European populations (Merkhofer et al., 2019). Among other readouts, the authors found that healthy Wisconsin Hmong donors had relatively hypoactive IL-6 and antifungal Th17 responses compared to healthy European donors (Merkhofer et al., 2019). The latter finding is consistent with differences in IL-6 responses, which could also explain several as-yet unstudied population differences that impact T cell development. To date, the only reported monogenic condition associated with blastomycosis is GATA2 deficiency (Spinner et al., 2016).
A genetic basis for susceptibility to endemic dimorphic fungi has long been hypothesized, due to their propensity for causing disease in otherwise healthy people, and to their predilection for people of certain genetic backgrounds. Extensive experiments in mouse models have highlighted similarities and differences in the immune responses to this diverse group of pathogens. Only in the past few years have studies in patients begun to reveal those processes that are critical to human immunity. The role of Th1 and Th17 responses is evinced by the severe infections seen in patients with monogenic deficits in those pathways. More recently, studies of disseminated coccidioidomycosis and pulmonary blastomycosis have revealed subtle variants in these pathways. The identification of these variants represents progress toward understanding the genetic underpinnings of observed health disparities in these pathogens.
While an increasing number of mutations and variants are reported to confer risk of fungal diseases, only PIDs and Mendelian susceptibility variants are currently clinically actionable. This is partially because there are substantial challenges in translating these findings to clinical applications. By necessity, most studies of susceptibility to fungal diseases have limited their donor population to one ethnic/racial background, which may limit generalizability of findings or, at the very least, necessitate extensive validation of variants in diverse patient populations. Still, the availability of next-gen sequencing technologies may soon make it feasible to incorporate testing for subtle risk variants into clinical practice, and guidelines will be developed for how to incorporate an individual's susceptibility profile into patient care. Future efforts may seek to develop tools to stratify patients by risk based on susceptibility variants; for example, IA susceptibility variants could be incorporated into a tool to stratifying potential HSCT donors by risk of invasive molds.
RM and BK conceived the content of the manuscript together. RM drafted much of the manuscript. BK contributed to portions, and reviewed and edited the entire manuscript. RM and BK jointly finalized the manuscript.
Support was provided by the National Institutes of Health (R01 AI035681, AI130411, AI040996 to BK); and by the Ruth L. Kirschstein National Research Service Award, through the National Institute for Minority Health and Health Disparities (F30 MD011547 to RM).
The authors declare that the research was conducted in the absence of any commercial or financial relationships that could be construed as a potential conflict of interest.
Abdel-Rahman, S. M., and Preuett, B. L. (2012). Genetic predictors of susceptibility to cutaneous fungal infections: a pilot genome wide association study to refine a candidate gene search. J. Dermatol. Sci. 67, 147–152. doi: 10.1016/j.jdermsci.2012.05.003
Abdel-Rahman, S. M., Simon, S., Wright, K. J., Ndjountche, L., and Gaedigk, A. (2006). Tracking Trichophyton tonsurans through a large urban child care center: defining infection prevalence and transmission patterns by molecular strain typing. Pediatrics 118, 2365–2373. doi: 10.1542/peds.2006-2065
Allendoerfer, R., and Deepe, G. S. Jr. (1997). Intrapulmonary response to Histoplasma capsulatum in gamma interferon knockout mice. Infect. Immun. 65, 2564–2569. doi: 10.1128/IAI.65.7.2564-2569.1997
Aluri, J., Desai, M., Gupta, M., Dalvi, A., Terance, A., Rosenzweig, S. D., et al. (2019). Clinical, immunological, and molecular findings in 57 patients with severe combined immunodeficiency (SCID) from India. Front. Immunol. 10:23. doi: 10.3389/fimmu.2019.00023
Amatuni, G. S., Currier, R. J., Church, J. A., Bishop, T., Grimbacher, E., Nguyen, A. A., et al. (2019). Newborn screening for severe combined immunodeficiency and T-cell Lymphopenia in California, 2010–2017. Pediatrics 143. doi: 10.1542/peds.2018-2300
Arbour, N. C., Lorenz, E., Schutte, B. C., Zabner, J., Kline, J. N., Jones, M., et al. (2000). TLR4 mutations are associated with endotoxin hyporesponsiveness in humans. Nat. Genet. 25, 187–191. doi: 10.1038/76048
Assi, M., McKinsey, D. S., Driks, M. R., O'Connor, M. C., Bonacini, M., Graham, B., et al. (2006). Gastrointestinal histoplasmosis in the acquired immunodeficiency syndrome: report of 18 cases and literature review. Diagn. Microbiol. Infect. Dis. 55, 195–201. doi: 10.1016/j.diagmicrobio.2006.01.015
Asz-Sigall, D., Lopez-Garcia, L., Vega-Memije, M. E., Lacy-Niebla, R. M., Garcia-Corona, C., Ramirez-Renteria, C., et al. (2010). HLA-DR6 association confers increased resistance to T. rubrum onychomycosis in mexican mestizos. Int. J. Dermatol. 49, 1406–1409. doi: 10.1111/j.1365-4632.2010.04550.x
Aydemir, C., Onay, H., Oguz, S. S., Ozdemir, T. R., Erdeve, O., Ozkinay, F., et al. (2011). Mannose-binding lectin codon 54 gene polymorphism in relation to risk of nosocomial invasive fungal infection in preterm neonates in the neonatal intensive care unit. J. Matern. Fetal Neonatal Med. 24, 1124–1127. doi: 10.3109/14767058.2010.536865
Babula, O., Lazdane, G., Kroica, J., Linhares, I. M., Ledger, W. J., and Witkin, S. S. (2005). Frequency of interleukin-4 (IL-4)−589 gene polymorphism and vaginal concentrations of IL-4, nitric oxide, and mannose-binding lectin in women with recurrent vulvovaginal candidiasis. Clin. Infect. Dis. 40, 1258–1262. doi: 10.1086/429246
Bao, Z., Chen, H., Zhou, M., Shi, G., Li, Q., and Wan, H. (2017). Invasive pulmonary aspergillosis in patients with chronic obstructive pulmonary disease: a case report and review of the literature. Oncotarget 8, 38069–38074. doi: 10.18632/oncotarget.16971
Baumgardner, D. J., and Brockman, K. (1998). Epidemiology of human blastomycosis in Vilas County, Wisconsin. II: 1991–1996. WMJ 97, 44–47.
Baumgardner, D. J., Buggy, B. P., Mattson, B. J., Burdick, J. S., and Ludwig, D. (1992). Epidemiology of blastomycosis in a region of high endemicity in north central Wisconsin. Clin. Infect. Dis. 15, 629–635. doi: 10.1093/clind/15.4.629
Baumgardner, D. J., Egan, G., Giles, S., and Laundre, B. (2002). An outbreak of blastomycosis on a United States Indian reservation. Wilderness Environ. Med. 13, 250–252. doi: 10.1580/1080-6032(2002)013[0250:aooboa]2.0.co;2
Baumgardner, D. J., and Laundre, B. (2001). Studies on the molecular ecology of Blastomyces dermatitidis. Mycopathologia 152, 51–58. doi: 10.1023/A:1012438029997
Benedict, K., Beer, K. D., and Jackson, B. R. (2019). Histoplasmosis-related healthcare use, diagnosis, and treatment in a commercially insured population, United States. Clin. Infect. Dis. doi: 10.1093/cid/ciz324
Bergstrom, L., Yocum, D. E., Ampel, N. M., Villanueva, I., Lisse, J., Gluck, O., et al. (2004). Increased risk of coccidioidomycosis in patients treated with tumor necrosis factor alpha antagonists. Arthritis Rheum. 50, 1959–1966. doi: 10.1002/art.20454
Bishu, S., Hernandez-Santos, N., Simpson-Abelson, M. R., Huppler, A. R., Conti, H. R., Ghilardi, N., et al. (2014). The adaptor CARD9 is required for adaptive but not innate immunity to oral mucosal Candida albicans infections. Infect. Immun. 82, 1173–1180. doi: 10.1128/IAI.01335-13
Blair, J. E., Ampel, N. M., and Hoover, S. E. (2019). Coccidioidomycosis in selected immunosuppressed hosts. Med. Mycol. 57, S56–S63. doi: 10.1093/mmy/myy019
Bochud, P. Y., Chien, J. W., Marr, K. A., Leisenring, W. M., Upton, A., Janer, M., et al. (2008). Toll-like receptor 4 polymorphisms and aspergillosis in stem-cell transplantation. N. Engl. J. Med. 359, 1766–1777. doi: 10.1056/NEJMoa0802629
Bongomin, F., Gago, S., Oladele, R. O., and Denning, D. W. (2017). Global and multi-national prevalence of fungal diseases-estimate precision. J. Fungi 3:E57. doi: 10.3390/jof3040057
Bonifaz, A., Araiza, J., Koffman-Alfaro, S., Paredes-Solis, V., Cuevas-Covarrubias, S., and Rivera, M. R. (2004). Tinea imbricata: autosomal dominant pattern of susceptibility in a polygamous indigenous family of the Nahuatl zone in Mexico. Mycoses 47, 288–291. doi: 10.1111/j.1439-0507.2004.00989.x
Bourgeois, N., Douard-Enault, C., Reynes, J., Lechiche, C., Basset, D., Rispail, P., et al. (2011). Seven imported histoplasmosis cases due to Histoplasma capsulatum var. capsulatum: from few weeks to more than three decades asymptomatic period. J. Mycol. Med. 21, 19–23. doi: 10.1016/j.mycmed.2010.11.009
Break, T. J., Jaeger, M., Solis, N. V., Filler, S. G., Rodriguez, C. A., Lim, J. K., et al. (2015). CX3CR1 is dispensable for control of mucosal Candida albicans infections in mice and humans. Infect. Immun. 83, 958–965. doi: 10.1128/IAI.02604-14
Brouwer, N., Dolman, K. M., van Houdt, M., Sta, M., Roos, D., and Kuijpers, T. W. (2008). Mannose-binding lectin (MBL) facilitates opsonophagocytosis of yeasts but not of bacteria despite MBL binding. J. Immunol. 180, 4124–4132. doi: 10.4049/jimmunol.180.6.4124
Bruno, V. M., Shetty, A. C., Yano, J., Fidel, P. L. Jr., Noverr, M. C., and Peters, B. M. (2015). Transcriptomic analysis of vulvovaginal candidiasis identifies a role for the NLRP3 inflammasome. mBio 6, e00182–e001815. doi: 10.1128/mBio.00182-15
Buckley, R. H. (2004). The multiple causes of human SCID. J. Clin. Invest. 114, 1409–1411. doi: 10.1172/JCI200423571
Camargo, J. F., Bhimji, A., Kumar, D., Kaul, R., Pavan, R., Schuh, A., et al. (2015). Impaired T cell responsiveness to interleukin-6 in hematological patients with invasive aspergillosis. PLoS ONE 10:e0123171. doi: 10.1371/journal.pone.0123171
Carrillo-Melendrez, H., Ortega-Hernandez, E., Granados, J., Arroyo, S., Barquera, R., and Arenas, R. (2016). Role of HLA-DR alleles to increase genetic susceptibility to onychomycosis in nail psoriasis. Skin Appendage Disord. 2, 22–25. doi: 10.1159/000446444
Cassone, A., and Cauda, R. (2012). Candida and candidiasis in HIV-infected patients: where commensalism, opportunistic behavior and frank pathogenicity lose their borders. AIDS 26, 1457–1472. doi: 10.1097/QAD.0b013e3283536ba8
Castillo, C. G., Kauffman, C. A., and Miceli, M. H. (2016). Blastomycosis. Infect. Dis. Clin. North Am. 30, 247–264. doi: 10.1016/j.idc.2015.10.002
Centers for Disease Control (1981). Pneumocystis pneumonia—Los Angeles. MMWR Morb. Mortal. Wkly. Rep. 30, 250–252.
Centers for Disease Control and Prevention (2013). Increase in reported coccidioidomycosis—United States, 1998–2011. MMWR Morb. Mortal. Wkly. Rep. 62, 217–221.
Chai, L. Y., de Boer, M. G., van der Velden, W. J., Plantinga, T. S., van Spriel, A. B., Jacobs, C., et al. (2011). The Y238X stop codon polymorphism in the human beta-glucan receptor dectin-1 and susceptibility to invasive aspergillosis. J. Infect. Dis. 203, 736–743. doi: 10.1093/infdis/jiq102
Chaim, W., Foxman, B., and Sobel, J. D. (1997). Association of recurrent vaginal candidiasis and secretory ABO and Lewis phenotype. J. Infect. Dis. 176, 828–830. doi: 10.1086/517314
Cheng, S. C., van de Veerdonk, F. L., Lenardon, M., Stoffels, M., Plantinga, T., Smeekens, S., et al. (2011). The dectin-1/inflammasome pathway is responsible for the induction of protective T-helper 17 responses that discriminate between yeasts and hyphae of Candida albicans. J. Leukoc. Biol. 90, 357–366. doi: 10.1189/jlb.1210702
Choi, E. H., Foster, C. B., Taylor, J. G., Erichsen, H. C., Chen, R. A., Walsh, T. J., et al. (2003). Association between chronic disseminated candidiasis in adult acute leukemia and common IL4 promoter haplotypes. J. Infect. Dis. 187, 1153–1156. doi: 10.1086/368345
Choi, E. H., Taylor, J. G., Foster, C. B., Walsh, T. J., Anttila, V. J., Ruutu, T., et al. (2005). Common polymorphisms in critical genes of innate immunity do not contribute to the risk for chronic disseminated candidiasis in adult leukemia patients. Med. Mycol. 43, 349–353. doi: 10.1080/13693780412331282322
Choptiany, M., Wiebe, L., Limerick, B., Sarsfield, P., Cheang, M., Light, B., et al. (2009). Risk factors for acquisition of endemic blastomycosis. Can. J. Infect. Dis. Med. Microbiol. 20, 117–121. doi: 10.1155/2009/824101
Clemons, K. V., Grunig, G., Sobel, R. A., Mirels, L. F., Rennick, D. M., and Stevens, D. A. (2000). Role of IL-10 in invasive aspergillosis: increased resistance of IL-10 gene knockout mice to lethal systemic aspergillosis. Clin. Exp. Immunol. 122, 186–191. doi: 10.1046/j.1365-2249.2000.01382.x
Collar, A. L., Swamydas, M., O'Hayre, M., Sajib, M. S., Hoffman, K. W., Singh, S. P., et al. (2018). The homozygous CX3CR1-M280 mutation impairs human monocyte survival. JCI Insight 3:e95417. doi: 10.1172/jci.insight.95417
Collin, M., Dickinson, R., and Bigley, V. (2015). Haematopoietic and immune defects associated with GATA2 mutation. Br. J. Haematol. 169, 173–187. doi: 10.1111/bjh.13317
Cunha, C., Aversa, F., Lacerda, J. F., Busca, A., Kurzai, O., Grube, M., et al. (2014). Genetic PTX3 deficiency and aspergillosis in stem-cell transplantation. N. Engl. J. Med. 370, 421–432. doi: 10.1056/NEJMoa1211161
Cunha, C., Di Ianni, M., Bozza, S., Giovannini, G., Zagarella, S., Zelante, T., et al. (2010). Dectin-1 Y238X polymorphism associates with susceptibility to invasive aspergillosis in hematopoietic transplantation through impairment of both recipient- and donor-dependent mechanisms of antifungal immunity. Blood 116, 5394–5402. doi: 10.1182/blood-2010-04-279307
Cunha, C., Giovannini, G., Pierini, A., Bell, A. S., Sorci, G., Riuzzi, F., et al. (2011). Genetically-determined hyperfunction of the S100B/RAGE axis is a risk factor for aspergillosis in stem cell transplant recipients. PLoS ONE 6:e27962. doi: 10.1371/journal.pone.0027962
Cunha, C., Goncalves, S. M., Duarte-Oliveira, C., Leite, L., Lagrou, K., Marques, A., et al. (2017). IL-10 overexpression predisposes to invasive aspergillosis by suppressing antifungal immunity. J. Allergy Clin. Immunol. 140, 867–870. doi: 10.1016/j.jaci.2017.02.034
de Boer, M. G., Jolink, H., Halkes, C. J., van der Heiden, P. L., Kremer, D., Falkenburg, J. H., et al. (2011). Influence of polymorphisms in innate immunity genes on susceptibility to invasive aspergillosis after stem cell transplantation. PLoS ONE 6:e18403. doi: 10.1371/journal.pone.0018403
De Luca, A., Carvalho, A., Cunha, C., Iannitti, R. G., Pitzurra, L., Giovannini, G., et al. (2013). IL-22 and IDO1 affect immunity and tolerance to murine and human vaginal candidiasis. PLoS Pathog. 9:e1003486. doi: 10.1371/journal.ppat.1003486
de Sousa Mda, G., Santana, G. B., Criado, P. R., and Benard, G. (2015). Chronic widespread dermatophytosis due to Trichophyton rubrum: a syndrome associated with a Trichophyton-specific functional defect of phagocytes. Front. Microbiol. 6:801. doi: 10.3389/fmicb.2015.00801
Deepe, G. S. Jr. (2018). Outbreaks of histoplasmosis: the spores set sail. PLoS Pathog. 14:e1007213. doi: 10.1371/journal.ppat.1007213
Deepe, G. S. Jr., Buesing, W. R., Ostroff, G. R., Abraham, A., Specht, C. A., Huang, H., et al. (2018). Vaccination with an alkaline extract of Histoplasma capsulatum packaged in glucan particles confers protective immunity in mice. Vaccine 36, 3359–3367. doi: 10.1016/j.vaccine.2018.04.047
Deepe, G. S. Jr., and Gibbons, R. S. (2006). T cells require tumor necrosis factor-alpha to provide protective immunity in mice infected with Histoplasma capsulatum. J. Infect. Dis. 193, 322–330. doi: 10.1086/498981
Deepe, G. S. Jr., and Gibbons, R. S. (2009). Interleukins 17 and 23 influence the host response to Histoplasma capsulatum. J. Infect. Dis. 200, 142–151. doi: 10.1086/599333
Denning, D. W., Kneale, M., Sobel, J. D., and Rautemaa-Richardson, R. (2018). Global burden of recurrent vulvovaginal candidiasis: a systematic review. Lancet Infect. Dis. 18, e339–e347. doi: 10.1016/S1473-3099(18)30103-8
Deppen, S. A., Massion, P. P., Blume, J., Walker, R. C., Antic, S., Chen, H., et al. (2019). Accuracy of a novel histoplasmosis enzyme immunoassay to evaluate suspicious lung nodules. Cancer Epidemiol. Biomarkers Prev. 28, 321–326. doi: 10.1158/1055-9965.EPI-18-0169
Dix, A., Czakai, K., Springer, J., Fliesser, M., Bonin, M., Guthke, R., et al. (2016). Genome-wide expression profiling reveals S100B as biomarker for invasive aspergillosis. Front. Microbiol. 7:320. doi: 10.3389/fmicb.2016.00320
Dodge, R. R., Lebowitz, M. D., Barbee, R., and Burrows, B. (1985). Estimates of C. immitis infection by skin test reactivity in an endemic community. Am. J. Public Health 75, 863–865. doi: 10.2105/AJPH.75.8.863
Domino, S. E., Hurd, E. A., Thomsson, K. A., Karnak, D. M., Holmen Larsson, J. M., Thomsson, E., et al. (2009). Cervical mucins carry alpha(1,2)fucosylated glycans that partly protect from experimental vaginal candidiasis. Glycoconj. J. 26, 1125–1134. doi: 10.1007/s10719-009-9234-0
Drake, K. W., and Adam, R. D. (2009). Coccidioidal meningitis and brain abscesses: analysis of 71 cases at a referral center. Neurology 73, 1780–1786. doi: 10.1212/WNL.0b013e3181c34b69
Drewniak, A., Gazendam, R. P., Tool, A. T., van Houdt, M., Jansen, M. H., van Hamme, J. L., et al. (2013). Invasive fungal infection and impaired neutrophil killing in human CARD9 deficiency. Blood 121, 2385–2392. doi: 10.1182/blood-2012-08-450551
Drummond, R. A., Collar, A. L., Swamydas, M., Rodriguez, C. A., Lim, J. K., Mendez, L. M., et al. (2015). CARD9-dependent neutrophil recruitment protects against fungal invasion of the central nervous system. PLoS Pathog. 11:e1005293. doi: 10.1371/journal.ppat.1005293
Drummond, R. A., Franco, L. M., and Lionakis, M. S. (2018). Human CARD9: a critical molecule of fungal immune surveillance. Front. Immunol. 9:1836. doi: 10.3389/fimmu.2018.01836
Drummond, R. A., Swamydas, M., Oikonomou, V., Zhai, B., Dambuza, I. M., Schaefer, B. C., et al. (2019). CARD9(+) microglia promote antifungal immunity via IL-1beta- and CXCL1-mediated neutrophil recruitment. Nat. Immunol. 20, 559–570. doi: 10.1038/s41590-019-0377-2
Duke-Cohan, J. S., Ishikawa, Y., Yoshizawa, A., Choi, Y. I., Lee, C. N., Acuto, O., et al. (2018). Regulation of thymocyte trafficking by Tagap, a GAP domain protein linked to human autoimmunity. Sci. Signal. 11:eaan8799. doi: 10.1126/scisignal.aan8799
Dureault, A., Tcherakian, C., Poiree, S., Catherinot, E., Danion, F., Jouvion, G., et al. (2019). Spectrum of pulmonary aspergillosis in hyper-ige syndrome with autosomal-dominant STAT3 deficiency. J. Allergy Clin. Immunol. Pract. 7, 1986–1995. doi: 10.1016/j.jaip.2019.02.041
Eren Akarcan, S., Ulusoy Severcan, E., Edeer Karaca, N., Isik, E., Aksu, G., Migaud, M., et al. (2017). Gain-of-function mutations in STAT1: a recently defined cause for chronic mucocutaneous candidiasis disease mimicking combined immunodeficiencies. Case Rep. Immunol. 2017:2846928. doi: 10.1155/2017/2846928
Feriotti, C., Bazan, S. B., Loures, F. V., Araujo, E. F., Costa, T. A., and Calich, V. L. (2015). Expression of dectin-1 and enhanced activation of NALP3 inflammasome are associated with resistance to paracoccidioidomycosis. Front. Microbiol. 6:913. doi: 10.3389/fmicb.2015.00913
Ferwerda, B., Ferwerda, G., Plantinga, T. S., Willment, J. A., van Spriel, A. B., Venselaar, H., et al. (2009). Human dectin-1 deficiency and mucocutaneous fungal infections. N. Engl. J. Med. 361, 1760–1767. doi: 10.1056/NEJMoa0901053
Finkel-Jimenez, B., Wuthrich, M., Brandhorst, T., and Klein, B. S. (2001). The WI-1 adhesin blocks phagocyte TNF-alpha production, imparting pathogenicity on Blastomyces dermatitidis. J. Immunol. 166, 2665–2673. doi: 10.4049/jimmunol.166.4.2665
Fisher, C. E., Hohl, T. M., Fan, W., Storer, B. E., Levine, D. M., Zhao, L. P., et al. (2017). Validation of single nucleotide polymorphisms in invasive aspergillosis following hematopoietic cell transplantation. Blood 129, 2693–2701. doi: 10.1182/blood-2016-10-743294
Fitzpatrick, D. A., Logue, M. E., Stajich, J. E., and Butler, G. (2006). A fungal phylogeny based on 42 complete genomes derived from supertree and combined gene analysis. BMC Evol. Biol. 6:99. doi: 10.1186/1471-2148-6-99
Forseth, J., Rohwedder, J. J., Levine, B. E., and Saubolle, M. A. (1986). Experience with needle biopsy for coccidioidal lung nodules. Arch. Intern. Med. 146, 319–320. doi: 10.1001/archinte.1986.00360140141020
Gao, Y., Liang, G., Wang, Q., She, X., Shi, D., Shen, Y., et al. (2019). Different host immunological response to C. albicans by human oral and vaginal epithelial cells. Mycopathologia 184, 1–12. doi: 10.1007/s11046-018-0301-6
Garcia-Romero, M. T., Granados, J., Vega-Memije, M. E., and Arenas, R. (2012). Analysis of genetic polymorphism of the HLA-B and HLA-DR loci in patients with dermatophytic onychomycosis and in their first-degree relatives. Actas Dermosifiliogr. 103, 59–62. doi: 10.1016/j.adengl.2011.03.017
Garlanda, C., Hirsch, E., Bozza, S., Salustri, A., De Acetis, M., Nota, R., et al. (2002). Non-redundant role of the long pentraxin PTX3 in anti-fungal innate immune response. Nature 420, 182–186. doi: 10.1038/nature01195
Gildea, L. A., Gibbons, R., Finkelman, F. D., and Deepe, G. S. Jr. (2003). Overexpression of interleukin-4 in lungs of mice impairs elimination of Histoplasma capsulatum. Infect. Immun. 71, 3787–3793. doi: 10.1128/IAI.71.7.3787-3793.2003
Gnat, S., Nowakiewicz, A., Lagowski, D., and Zieba, P. (2019). Host- and pathogen-dependent susceptibility and predisposition to dermatophytosis. J. Med. Microbiol. 68, 823–836 doi: 10.1099/jmm.0.000982
Goodwin, R. A., Loyd, J. E., and Des Prez, R. M. (1981). Histoplasmosis in normal hosts. Medicine 60, 231–266. doi: 10.1097/00005792-198107000-00001
Gouba, N., and Drancourt, M. (2015). Digestive tract mycobiota: a source of infection. Med. Mal. Infect. 45, 9–16. doi: 10.1016/j.medmal.2015.01.007
Goupil, M., Cousineau-Cote, V., Aumont, F., Senechal, S., Gaboury, L., Hanna, Z., et al. (2014). Defective IL-17- and IL-22-dependent mucosal host response to Candida albicans determines susceptibility to oral candidiasis in mice expressing the HIV-1 transgene. BMC Immunol. 15:49. doi: 10.1186/s12865-014-0049-9
Gresnigt, M. S., Cunha, C., Jaeger, M., Goncalves, S. M., Malireddi, R. K. S., Ammerdorffer, A., et al. (2018). Genetic deficiency of NOD2 confers resistance to invasive aspergillosis. Nat. Commun. 9:2636. doi: 10.1038/s41467-018-04912-3
Grube, M., Loeffler, J., Mezger, M., Kruger, B., Echtenacher, B., Hoffmann, P., et al. (2013). TLR5 stop codon polymorphism is associated with invasive aspergillosis after allogeneic stem cell transplantation. Med. Mycol. 51, 818–825. doi: 10.3109/13693786.2013.809630
Gupta, A., Tripathy, S. K., Phulware, R. H., Arava, S., and Bagri, N. K. (2019). Cryopyrin-associated periodic fever syndrome in children: a case-based review. Int. J. Rheum. Dis. 23, 262–270. doi: 10.1111/1756-185X.13772
He, Q., Li, H., Rui, Y., Liu, L., He, B., Shi, Y., et al. (2018). Pentraxin 3 gene polymorphisms and pulmonary aspergillosis in chronic obstructive pulmonary disease patients. Clin. Infect. Dis. 66, 261–267. doi: 10.1093/cid/cix749
Heimall, J., Logan, B. R., Cowan, M. J., Notarangelo, L. D., Griffith, L. M., Puck, J. M., et al. (2017). Immune reconstitution and survival of 100 SCID patients post-hematopoietic cell transplant: a PIDTC natural history study. Blood 130, 2718–2727. doi: 10.1182/blood-2017-05-781849
Hernandez-Santos, N., Huppler, A. R., Peterson, A. C., Khader, S. A., McKenna, K. C., and Gaffen, S. L. (2013). Th17 cells confer long-term adaptive immunity to oral mucosal Candida albicans infections. Mucosal Immunol. 6, 900–910. doi: 10.1038/mi.2012.128
Hernandez-Santos, N., Wiesner, D. L., Fites, J. S., McDermott, A. J., Warner, T., Wuthrich, M., et al. (2018). Lung epithelial cells coordinate innate lymphocytes and immunity against pulmonary fungal infection. Cell Host Microbe 23, 511–522. doi: 10.1016/j.chom.2018.02.011
Herrero-Sanchez, M. C., Angomas, E. B., de Ramon, C., Telleria, J. J., Corchete, L. A., Alonso, S., et al. (2018). Polymorphisms in receptors involved in opsonic and nonopsonic phagocytosis, and correlation with risk of infection in oncohematology patients. Infect. Immun. 86, e00709–e00718. doi: 10.1128/IAI.00709-18
Hillmer, E. J., Zhang, H., Li, H. S., and Watowich, S. S. (2016). STAT3 signaling in immunity. Cytokine Growth Factor Rev. 31, 1–15. doi: 10.1016/j.cytogfr.2016.05.001
Hilton, E., Chandrasekaran, V., Rindos, P., and Isenberg, H. D. (1995). Association of recurrent candidal vaginitis with inheritance of Lewis blood group antigens. J. Infect. Dis. 172, 1616–1619. doi: 10.1093/infdis/172.6.1616
Howard, D. H. (1984). The epidemiology and ecology of blastomycosis, coccidioidomycosis and histoplasmosis. Zentralbl. Bakteriol. Mikrobiol. Hyg. A. 257, 219–227. doi: 10.1016/S0174-3031(84)80075-X
Huffnagle, G. B., and Noverr, M. C. (2013). The emerging world of the fungal microbiome. Trends Microbiol. 21, 334–341. doi: 10.1016/j.tim.2013.04.002
Humbert, L., Cornu, M., Proust-Lemoine, E., Bayry, J., Wemeau, J. L., Vantyghem, M. C., et al. (2018). Chronic mucocutaneous candidiasis in autoimmune polyendocrine syndrome type 1. Front. Immunol. 9:2570. doi: 10.3389/fimmu.2018.02570
Hung, C. Y., Gonzalez, A., Wuthrich, M., Klein, B. S., and Cole, G. T. (2011). Vaccine immunity to coccidioidomycosis occurs by early activation of three signal pathways of T helper cell response (Th1, Th2, and Th17). Infect. Immun. 79, 4511–4522. doi: 10.1128/IAI.05726-11
Hung, C. Y., Hsu, A. P., Holland, S. M., and Fierer, J. (2019). A review of innate and adaptive immunity to coccidioidomycosis. Med. Mycol. 57, S85–S92. doi: 10.1093/mmy/myy146
Hung, C. Y., Jimenez-Alzate Mdel, P., Gonzalez, A., Wuthrich, M., Klein, B. S., and Cole, G. T. (2014). Interleukin-1 receptor but not Toll-like receptor 2 is essential for MyD88-dependent Th17 immunity to Coccidioides infection. Infect. Immun. 82, 2106–2114. doi: 10.1128/IAI.01579-13
Hurd, E. A., and Domino, S. E. (2004). Increased susceptibility of secretor factor gene Fut2-null mice to experimental vaginal candidiasis. Infect. Immun. 72, 4279–4281. doi: 10.1128/IAI.72.7.4279-4281.2004
Jaeger, M., Carvalho, A., Cunha, C., Plantinga, T. S., van de Veerdonk, F., Puccetti, M., et al. (2016). Association of a variable number tandem repeat in the NLRP3 gene in women with susceptibility to RVVC. Eur. J. Clin. Microbiol. Infect. Dis. 35, 797–801. doi: 10.1007/s10096-016-2600-5
Jaeger, M., Pinelli, M., Borghi, M., Constantini, C., Dindo, M., van Emst, L., et al. (2019). A systems genomics approach identifies. Sci. Transl. Med. 11:eaar3558. doi: 10.1126/scitranslmed.aar3558
Jaeger, M., van der Lee, R., Cheng, S. C., Johnson, M. D., Kumar, V., Ng, A., et al. (2015). The RIG-I-like helicase receptor MDA5 (IFIH1) is involved in the host defense against Candida infections. Eur. J. Clin. Microbiol. Infect. Dis. 34, 963–974. doi: 10.1007/s10096-014-2309-2
Johnson, M. D., Plantinga, T. S., van de Vosse, E., Velez Edwards, D. R., Smith, P. B., Alexander, B. D., et al. (2012). Cytokine gene polymorphisms and the outcome of invasive candidiasis: a prospective cohort study. Clin. Infect. Dis. 54, 502–510. doi: 10.1093/cid/cir827
Kalia, N., Kaur, M., Sharma, S., and Singh, J. (2018). A comprehensive in silico analysis of regulatory snps of human clec7a gene and its validation as genotypic and phenotypic disease marker in recurrent vulvovaginal infections. Front. Cell. Infect. Microbiol. 8:65. doi: 10.3389/fcimb.2018.00065
Kalia, N., Singh, J., Sharma, S., Arora, H., and Kaur, M. (2017). Genetic and phenotypic screening of mannose-binding lectin in relation to risk of recurrent vulvovaginal infections in women of north india: a prospective cohort study. Front. Microbiol. 8:75. doi: 10.3389/fmicb.2017.00075
Kanariou, M., Spanou, K., and Tantou, S. (2018). Long-term observational studies of chronic granulomatous disease. Curr. Opin. Hematol. 25, 7–12. doi: 10.1097/MOH.0000000000000396
Kesh, S., Mensah, N. Y., Peterlongo, P., Jaffe, D., Hsu, K., M, V. D. B., et al. (2005). TLR1 and TLR6 polymorphisms are associated with susceptibility to invasive aspergillosis after allogeneic stem cell transplantation. Ann. N. Y. Acad. Sci. 1062, 95–103. doi: 10.1196/annals.1358.012
Khuu, D., Shafir, S., Bristow, B., and Sorvillo, F. (2014). Blastomycosis mortality rates, United States, 1990–2010. Emerging Infect. Dis. 20, 1789–1794. doi: 10.3201/eid2011.131175
Klebanoff, S. J., Kettle, A. J., Rosen, H., Winterbourn, C. C., and Nauseef, W. M. (2013). Myeloperoxidase: a front-line defender against phagocytosed microorganisms. J. Leukoc. Biol. 93, 185–198. doi: 10.1189/jlb.0712349
Klein, B. S., Vergeront, J. M., and Davis, J. P. (1986). Epidemiologic aspects of blastomycosis, the enigmatic systemic mycosis. Semin. Respir. Infect. 1, 29–39.
Koldehoff, M., Beelen, D. W., and Elmaagacli, A. H. (2013). Increased susceptibility for aspergillosis and post-transplant immune deficiency in patients with gene variants of TLR4 after stem cell transplantation. Transpl. Infect. Dis. 15, 533–539. doi: 10.1111/tid.12115
Kononova, S. V. (2017). How fucose of blood group glycotopes programs human gut microbiota. Biochemistry 82, 973–989. doi: 10.1134/S0006297917090012
Kreins, A. Y., Ciancanelli, M. J., Okada, S., Kong, X. F., Ramirez-Alejo, N., Kilic, S. S., et al. (2015). Human TYK2 deficiency: mycobacterial and viral infections without hyper-IgE syndrome. J. Exp. Med. 212, 1641–1662. doi: 10.1084/jem.20140280
Kulkarni, D. G., and Venkatesh, D. (2004). Non-secretor status; a predisposing factor for vaginal candidiasis. Indian J. Physiol. Pharmacol. 48, 225–229.
Kumar, V., Cheng, S. C., Johnson, M. D., Smeekens, S. P., Wojtowicz, A., Giamarellos-Bourboulis, E., et al. (2014). Immunochip SNP array identifies novel genetic variants conferring susceptibility to candidaemia. Nat. Commun. 5:4675. doi: 10.1038/ncomms5675
Lambourne, J., Agranoff, D., Herbrecht, R., Troke, P. F., Buchbinder, A., Willis, F., et al. (2009). Association of mannose-binding lectin deficiency with acute invasive aspergillosis in immunocompromised patients. Clin. Infect. Dis. 49, 1486–1491. doi: 10.1086/644619
Lanternier, F., Mahdaviani, S. A., Barbati, E., Chaussade, H., Koumar, Y., Levy, R., et al. (2015). Inherited CARD9 deficiency in otherwise healthy children and adults with Candida species-induced meningoencephalitis, colitis, or both. J. Allergy Clin. Immunol. 135, 1558–1568. doi: 10.1016/j.jaci.2014.12.1930
Lanternier, F., Pathan, S., Vincent, Q. B., Liu, L., Cypowyj, S., Prando, C., et al. (2013). Deep dermatophytosis and inherited CARD9 deficiency. N. Engl. J. Med. 369, 1704–1714. doi: 10.1056/NEJMoa1208487
Lev-Sagie, A., Prus, D., Linhares, I. M., Lavy, Y., Ledger, W. J., and Witkin, S. S. (2009). Polymorphism in a gene coding for the inflammasome component NALP3 and recurrent vulvovaginal candidiasis in women with vulvar vestibulitis syndrome. Am. J. Obstet. Gynecol. 200, e301–e306. doi: 10.1016/j.ajog.2008.10.039
Li, H., and Anderson, S. K. (2018). Association of TNFRSF1B promoter polymorphisms with human disease: further studies examining t-regulatory cells are required. Front. Immunol. 9:443. doi: 10.3389/fimmu.2018.00443
Li, J., Vinh, D. C., Casanova, J. L., and Puel, A. (2017). Inborn errors of immunity underlying fungal diseases in otherwise healthy individuals. Curr. Opin. Microbiol. 40, 46–57. doi: 10.1016/j.mib.2017.10.016
Li, Y., Oosting, M., Deelen, P., Ricano-Ponce, I., Smeekens, S., Jaeger, M., et al. (2016). Inter-individual variability and genetic influences on cytokine responses to bacteria and fungi. Nat. Med. 22, 952–960. doi: 10.1038/nm.4139
Liang, P., Wang, X., Wang, R., Wan, Z., Han, W., and Li, R. (2015). CARD9 deficiencies linked to impaired neutrophil functions against Phialophora verrucosa. Mycopathologia 179, 347–357. doi: 10.1007/s11046-015-9877-2
Lionakis, M. S., and Levitz, S. M. (2018). Host control of fungal infections: lessons from basic studies and human cohorts. Annu. Rev. Immunol. 36, 157–191. doi: 10.1146/annurev-immunol-042617-053318
Lionakis, M. S., Swamydas, M., Fischer, B. G., Plantinga, T. S., Johnson, M. D., Jaeger, M., et al. (2013). CX3CR1-dependent renal macrophage survival promotes Candida control and host survival. J. Clin. Invest. 123, 5035–5051. doi: 10.1172/JCI71307
Liu, F., Fan, X., Auclair, S., Ferguson, M., Sun, J., Soong, L., et al. (2016). Sequential dysfunction and progressive depletion of Candida albicans-specific CD4 T cell response in HIV-1 infection. PLoS Pathog. 12:e1005663. doi: 10.1371/journal.ppat.1005663
Louie, L., Ng, S., Hajjeh, R., Johnson, R., Vugia, D., Werner, S. B., et al. (1999). Influence of host genetics on the severity of coccidioidomycosis. Emerging Infect. Dis. 5, 672–680. doi: 10.3201/eid0505.990508
Lovell, J. P., Foruraghi, L., Freeman, A. F., Uzel, G., Zerbe, C. S., Su, H., et al. (2016). Persistent nodal histoplasmosis in nuclear factor kappa B essential modulator deficiency: report of a case and review of infection in primary immunodeficiencies. J. Allergy Clin. Immunol. 138, 903–905. doi: 10.1016/j.jaci.2016.02.040
Lupianez, C. B., Villaescusa, M. T., Carvalho, A., Springer, J., Lackner, M., Sanchez-Maldonado, J. M., et al. (2016). Common genetic polymorphisms within NFkappaB-related genes and the risk of developing invasive aspergillosis. Front. Microbiol. 7:1243. doi: 10.3389/fmicb.2016.01243
Ma, C. S., Chew, G. Y., Simpson, N., Priyadarshi, A., Wong, M., Grimbacher, B., et al. (2008). Deficiency of Th17 cells in hyper IgE syndrome due to mutations in STAT3. J. Exp. Med. 205, 1551–1557. doi: 10.1084/jem.20080218
Magill, S. S., O'Leary, E., Janelle, S. J., Thompson, D. L., Dumyati, G., Nadle, J., et al. (2018). Changes in prevalence of health care-associated infections in U.S. Hospitals. N. Engl. J. Med. 379, 1732–1744. doi: 10.1056/NEJMoa1801550
Maiga, A. W., Deppen, S., Scaffidi, B. K., Baddley, J., Aldrich, M. C., Dittus, R. S., et al. (2018). Mapping histoplasma capsulatum exposure, United States. Emerging Infect. Dis. 24, 1835–1839. doi: 10.3201/eid2410.180032
Matzaraki, V., Gresnigt, M. S., Jaeger, M., Ricano-Ponce, I., Johnson, M. D., Oosting, M., et al. (2017). An integrative genomics approach identifies novel pathways that influence candidaemia susceptibility. PLoS ONE 12:e0180824. doi: 10.1371/journal.pone.0180824
McCotter, O., Kennedy, J., McCollum, J., Bartholomew, M., Iralu, J., Jackson, B. R., et al. (2019). Coccidioidomycosis among American Indians and Alaska Natives, 2001–2014. Open Forum Infect. Dis. 6:ofz052. doi: 10.1093/ofid/ofz052
McDermott, D. H., Fong, A. M., Yang, Q., Sechler, J. M., Cupples, L. A., Merrell, M. N., et al. (2003). Chemokine receptor mutant CX3CR1-M280 has impaired adhesive function and correlates with protection from cardiovascular disease in humans. J. Clin. Invest. 111, 1241–1250. doi: 10.1172/JCI16790
McTaggart, L. R., Brown, E. M., and Richardson, S. E. (2016). Phylogeographic analysis of blastomyces dermatitidis and blastomyces gilchristii reveals an association with North American freshwater drainage basins. PLoS ONE 11:e0159396. doi: 10.1371/journal.pone.0159396
Meece, J. K., Anderson, J. L., Gruszka, S., Sloss, B. L., Sullivan, B., and Reed, K. D. (2013). Variation in clinical phenotype of human infection among genetic groups of Blastomyces dermatitidis. J. Infect. Dis. 207, 814–822. doi: 10.1093/infdis/jis756
Merkhofer, R. M. Jr., O'Neill, M. B., Xiong, D., Hernandez-Santos, N., Dobson, H., Fites, J. S., et al. (2019). Investigation of genetic susceptibility to Blastomycosis reveals interleukin-6 as a potential susceptibility locus. MBio 10, e01224–e01219. doi: 10.1128/mBio.01224-19
Mezger, M., Steffens, M., Beyer, M., Manger, C., Eberle, J., Toliat, M. R., et al. (2008). Polymorphisms in the chemokine (C-X-C motif) ligand 10 are associated with invasive aspergillosis after allogeneic stem-cell transplantation and influence CXCL10 expression in monocyte-derived dendritic cells. Blood 111, 534–536. doi: 10.1182/blood-2007-05-090928
Milanese, M., Segat, L., De Seta, F., Pirulli, D., Fabris, A., Morgutti, M., et al. (2008). MBL2 genetic screening in patients with recurrent vaginal infections. Am. J. Reprod. Immunol. 59, 146–151. doi: 10.1111/j.1600-0897.2007.00549.x
Milner, J. D., Brenchley, J. M., Laurence, A., Freeman, A. F., Hill, B. J., Elias, K. M., et al. (2008). Impaired T(H)17 cell differentiation in subjects with autosomal dominant hyper-IgE syndrome. Nature 452, 773–776. doi: 10.1038/nature06764
Mogensen, T. H. (2018). IRF and STAT transcription factors - from basic biology to roles in infection, protective immunity, and primary immunodeficiencies. Front. Immunol. 9:3047. doi: 10.3389/fimmu.2018.03047
Monin, L., and Gaffen, S. L. (2018). Interleukin 17 family cytokines: signaling mechanisms, biological activities, and therapeutic implications. Cold Spring Harb. Perspect. Biol. 10:a028522. doi: 10.1101/cshperspect.a028522
Morre, S. A., Murillo, L. S., Spaargaren, J., Fennema, H. S., and Pena, A. S. (2002). Role of the toll-like receptor 4 Asp299Gly polymorphism in susceptibility to Candida albicans infection. J. Infect. Dis. 186, 1377–1379. doi: 10.1086/344328
Mousavi, B., Hedayati, M. T., Hedayati, N., Ilkit, M., and Syedmousavi, S. (2016). Aspergillus species in indoor environments and their possible occupational and public health hazards. Curr. Med. Mycol. 2, 36–42. doi: 10.18869/acadpub.cmm.2.1.36
Nanjappa, S. G., Heninger, E., Wuthrich, M., Sullivan, T., and Klein, B. (2012). Protective antifungal memory CD8(+) T cells are maintained in the absence of CD4(+) T cell help and cognate antigen in mice. J. Clin. Invest. 122, 987–999. doi: 10.1172/JCI58762
Nash, A. K., Auchtung, T. A., Wong, M. C., Smith, D. P., Gesell, J. R., Ross, M. C., et al. (2017). The gut mycobiome of the Human Microbiome Project healthy cohort. Microbiome 5:153. doi: 10.1186/s40168-017-0373-4
Nash, P., Mease, P. J., McInnes, I. B., Rahman, P., Ritchlin, C. T., Blanco, R., et al. (2018). Efficacy and safety of secukinumab administration by autoinjector in patients with psoriatic arthritis: results from a randomized, placebo-controlled trial (FUTURE 3). Arthritis Res. Ther. 20:47. doi: 10.1186/s13075-018-1551-x
Natarajan, M., Hsu, A. P., Weinreich, M. A., Zhang, Y., Niemela, J. E., Butman, J. A., et al. (2018). Aspergillosis, eosinophilic esophagitis, and allergic rhinitis in signal transducer and activator of transcription 3 haploinsufficiency. J. Allergy Clin. Immunol. 142, 993–997. doi: 10.1016/j.jaci.2018.05.009
Nedovic, B., Posteraro, B., Leoncini, E., Ruggeri, A., Amore, R., Sanguinetti, M., et al. (2014). Mannose-binding lectin codon 54 gene polymorphism and vulvovaginal candidiasis: a systematic review and meta-analysis. Biomed. Res. Int. 2014:738298. doi: 10.1155/2014/738298
Netea, M. G., Wijmenga, C., and O'Neill, L. A. (2012). Genetic variation in Toll-like receptors and disease susceptibility. Nat. Immunol. 13, 535–542. doi: 10.1038/ni.2284
Neville, B. A., d'Enfert, C., and Bougnoux, M. E. (2015). Candida albicans commensalism in the gastrointestinal tract. FEMS Yeast Res. 15:fov081. doi: 10.1093/femsyr/fov081
Nguyen, C., Barker, B. M., Hoover, S., Nix, D. E., Ampel, N. M., Frelinger, J. A., et al. (2013). Recent advances in our understanding of the environmental, epidemiological, immunological, and clinical dimensions of coccidioidomycosis. Clin. Microbiol. Rev. 26, 505–525. doi: 10.1128/CMR.00005-13
Oberhofer, T. R. (1979). Growth of nonfermentative bacteria at 42 degrees C. J. Clin. Microbiol. 10, 800–804. doi: 10.1128/JCM.10.6.800-804.1979
Oberle, M., Reichmuth, M., Laffer, R., Ottiger, C., Fankhauser, H., and Bregenzer, T. (2015). Non-seasonal variation of airborne Aspergillus spore concentration in a hospital building. Int. J. Environ. Res. Public Health 12, 13730–13738. doi: 10.3390/ijerph121113730
Odds, F. C., Webster, C. E., Fisk, P. G., Riley, V. C., Mayuranathan, P., and Simmons, P. D. (1989). Candida species and C. albicans biotypes in women attending clinics in genitourinary medicine. J. Med. Microbiol. 29, 51–54. doi: 10.1099/00222615-29-1-51
Odio, C. D., Milligan, K. L., McGowan, K., Rudman Spergel, A. K., Bishop, R., Boris, L., et al. (2015). Endemic mycoses in patients with STAT3-mutated hyper-IgE (Job) syndrome. J. Allergy Clin. Immunol. 136, 1411–1413. doi: 10.1016/j.jaci.2015.07.003
Okada, S., Markle, J. G., Deenick, E. K., Mele, F., Averbuch, D., Lagos, M., et al. (2015). Immunodeficiencies. Impairment of immunity to Candida and Mycobacterium in humans with bi-allelic RORC mutations. Science 349, 606–613. doi: 10.1126/science.aaa4282
Ouellette, C. P., Stanek, J. R., Leber, A., and Ardura, M. I. (2018). Pediatric histoplasmosis in an area of endemicity: a contemporary analysis. J. Pediatric Infect. Dis. Soc. 8, 400–407. doi: 10.1093/jpids/piy073
Pagano, L., Dragonetti, G., Cattaneo, C., Marchesi, F., Veggia, B., Busca, A., et al. (2017). Changes in the incidence of candidemia and related mortality in patients with hematologic malignancies in the last ten years. A SEIFEM 2015-B report. Haematologica 102, e407–e410. doi: 10.3324/haematol.2017.172536
Pais, P., Galocha, M., Viana, R., Cavalheiro, M., Pereira, D., and Teixeira, M. C. (2019). Microevolution of the pathogenic yeasts Candida albicans and Candida glabrata during antifungal therapy and host infection. Microb. Cell 6, 142–159. doi: 10.15698/mic2019.03.670
Pana, Z. D., Roilides, E., Warris, A., Groll, A. H., and Zaoutis, T. (2017). Epidemiology of invasive fungal disease in children. J. Pediatric Infect. Dis. Soc. 6, S3–S11. doi: 10.1093/jpids/pix046
Parvaneh, L., Sharifi, N., Azizi, G., Abolhassani, H., Sharifi, L., Mohebbi, A., et al. (2019). Infectious etiology of chronic diarrhea in patients with primary immunodeficiency diseases. Eur. Ann. Allergy Clin. Immunol. 51, 32–37. doi: 10.23822/EurAnnACI.1764-1489.77
Peters, B. M., Coleman, B. M., Willems, H. M. E., Barker, K. S., Aggor, F. E. Y., Cipolla, E., et al. (2019). The IL-17R/IL-22R signaling axis is dispensable for vulvovaginal candidiasis regardless of estrogen status. J. Infect. Dis. doi: 10.1093/infdis/jiz649
Pfister, J. R., Archer, J. R., Hersil, S., Boers, T., Reed, K. D., Meece, J. K., et al. (2011). Non-rural point source blastomycosis outbreak near a yard waste collection site. Clin. Med. Res. 9, 57–65. doi: 10.3121/cmr.2010.958
Pham, T. A., Clare, S., Goulding, D., Arasteh, J. M., Stares, M. D., Browne, H. P., et al. (2014). Epithelial IL-22RA1-mediated fucosylation promotes intestinal colonization resistance to an opportunistic pathogen. Cell Host Microbe 16, 504–516. doi: 10.1016/j.chom.2014.08.017
Plantinga, T. S., Hamza, O. J., Willment, J. A., Ferwerda, B., van de Geer, N. M., Verweij, P. E., et al. (2010). Genetic variation of innate immune genes in HIV-infected african patients with or without oropharyngeal candidiasis. J. Acquir. Immune Defic. Syndr. 55, 87–94. doi: 10.1097/QAI.0b013e3181e53c64
Plantinga, T. S., Johnson, M. D., Scott, W. K., van de Vosse, E., Velez Edwards, D. R., Smith, P. B., et al. (2012). Toll-like receptor 1 polymorphisms increase susceptibility to candidemia. J. Infect. Dis. 205, 934–943. doi: 10.1093/infdis/jir867
Plantinga, T. S., van der Velden, W. J., Ferwerda, B., van Spriel, A. B., Adema, G., Feuth, T., et al. (2009). Early stop polymorphism in human DECTIN-1 is associated with increased Candida colonization in hematopoietic stem cell transplant recipients. Clin. Infect. Dis. 49, 724–732. doi: 10.1086/604714
Punwani, D., Wang, H., Chan, A. Y., Cowan, M. J., Mallott, J., Sunderam, U., et al. (2015). Combined immunodeficiency due to MALT1 mutations, treated by hematopoietic cell transplantation. J. Clin. Immunol. 35, 135–146. doi: 10.1007/s10875-014-0125-1
Ramos, P. S. (2017). Population genetics and natural selection in rheumatic disease. Rheum. Dis. Clin. North Am. 43, 313–326. doi: 10.1016/j.rdc.2017.04.001
Ravel, J., Gajer, P., Abdo, Z., Schneider, G. M., Koenig, S. S., McCulle, S. L., et al. (2011). Vaginal microbiome of reproductive-age women. Proc. Natl. Acad. Sci. U.S.A. 108(Suppl. 1), 4680–4687. doi: 10.1073/pnas.1002611107
Ravine, D., Turner, K. J., and Alpers, M. P. (1980). Genetic inheritance of susceptibility to tinea imbricata. J. Med. Genet. 17, 342–348. doi: 10.1136/jmg.17.5.342
Reed, K. D., Meece, J. K., Archer, J. R., and Peterson, A. T. (2008). Ecologic niche modeling of Blastomyces dermatitidis in Wisconsin. PLoS ONE 3:e2034. doi: 10.1371/journal.pone.0002034
Rieber, N., Gazendam, R. P., Freeman, A. F., Hsu, A. P., Collar, A. L., Sugui, J. A., et al. (2016). Extrapulmonary Aspergillus infection in patients with CARD9 deficiency. JCI Insight 1:e89890. doi: 10.1172/jci.insight.89890
Robin, C., Cordonnier, C., Sitbon, K., Raus, N., Lortholary, O., Maury, S., et al. (2019). Mainly post-transplant factors are associated with invasive Aspergillosis after allogeneic stem cell transplantation: a study from the surveillance des Aspergilloses invasives en france and societe francophone de greffe de moelle et de therapie cellulaire. Biol. Blood Marrow Transplant. 25, 354–361. doi: 10.1016/j.bbmt.2018.09.028
Robinson, R. T., and Huppler, A. R. (2017). The Goldilocks model of immune symbiosis with Mycobacteria and Candida colonizers. Cytokine 97, 49–65. doi: 10.1016/j.cyto.2017.05.015
Robinson, W. S., Arnold, S. R., Michael, C. F., Vickery, J. D., Schoumacher, R. A., Pivnick, E. K., et al. (2011). Case report of a young child with disseminated histoplasmosis and review of hyper immunoglobulin e syndrome (HIES). Clin. Mol. Allergy 9:14. doi: 10.1186/1476-7961-9-14
Rosain, J., Oleaga-Quintas, C., Deswarte, C., Verdin, H., Marot, S., Syridou, G., et al. (2018). A variety of alu-mediated copy number variations can underlie IL-12Rbeta1 deficiency. J. Clin. Immunol. 38, 617–627. doi: 10.1007/s10875-018-0527-6
Rosentul, D. C., Delsing, C. E., Jaeger, M., Plantinga, T. S., Oosting, M., Costantini, I., et al. (2014a). Gene polymorphisms in pattern recognition receptors and susceptibility to idiopathic recurrent vulvovaginal candidiasis. Front. Microbiol. 5:483. doi: 10.3389/fmicb.2014.00483
Rosentul, D. C., Plantinga, T. S., Farcas, M., Oosting, M., Hamza, O. J., Scott, W. K., et al. (2014b). Role of autophagy genetic variants for the risk of Candida infections. Med. Mycol. 52, 333–341. doi: 10.1093/mmy/myt035
Rosentul, D. C., Plantinga, T. S., Oosting, M., Scott, W. K., Velez Edwards, D. R., Smith, P. B., et al. (2011a). Genetic variation in the dectin-1/CARD9 recognition pathway and susceptibility to candidemia. J. Infect. Dis. 204, 1138–1145. doi: 10.1093/infdis/jir458
Rosentul, D. C., Plantinga, T. S., Papadopoulos, A., Joosten, L. A., Antoniadou, A., Venselaar, H., et al. (2011b). Variation in genes of beta-glucan recognition pathway and susceptibility to opportunistic infections in HIV-positive patients. Immunol. Invest. 40, 735–750. doi: 10.3109/08820139.2011.599088
Rosentul, D. C., Plantinga, T. S., Scott, W. K., Alexander, B. D., van de Geer, N. M., Perfect, J. R., et al. (2012). The impact of caspase-12 on susceptibility to candidemia. Eur. J. Clin. Microbiol. Infect. Dis. 31, 277–280. doi: 10.1007/s10096-011-1307-x
Roth, S., Bergmann, H., Jaeger, M., Yeroslaviz, A., Neumann, K., Koenig, P. A., et al. (2016). Vav proteins are key regulators of card9 signaling for innate antifungal immunity. Cell Rep. 17, 2572–2583. doi: 10.1016/j.celrep.2016.11.018
Roy, M., Benedict, K., Deak, E., Kirby, M. A., McNiel, J. T., Sickler, C. J., et al. (2013). A large community outbreak of blastomycosis in Wisconsin with geographic and ethnic clustering. Clin. Infect. Dis. 57, 655–662. doi: 10.1093/cid/cit366
Ruddy, B. E., Mayer, A. P., Ko, M. G., Labonte, H. R., Borovansky, J. A., Boroff, E. S., et al. (2011). Coccidioidomycosis in African Americans. Mayo Clin. Proc. 86, 63–69. doi: 10.4065/mcp.2010.0423
Sadahiro, A., Moraes, J. R. F., Moraes, M. E. H., Romero, M., Gouvea, N. A. D., Gouvea, C. J., et al. (2004). HLA in Brazilian Ashkenazic Jews with chronic dermatophytosis caused by Trichophyton rubrum. Braz. J. Microbiol. 35, 69–73. doi: 10.1590/S1517-83822004000100011
Sainz, J., Hassan, L., Perez, E., Romero, A., Moratalla, A., Lopez-Fernandez, E., et al. (2007a). Interleukin-10 promoter polymorphism as risk factor to develop invasive pulmonary aspergillosis. Immunol. Lett. 109, 76–82. doi: 10.1016/j.imlet.2007.01.005
Sainz, J., Lupianez, C. B., Segura-Catena, J., Vazquez, L., Rios, R., Oyonarte, S., et al. (2012). Dectin-1 and DC-SIGN polymorphisms associated with invasive pulmonary Aspergillosis infection. PLoS ONE 7:e32273. doi: 10.1371/journal.pone.0032273
Sainz, J., Perez, E., Gomez-Lopera, S., and Jurado, M. (2008). IL1 gene cluster polymorphisms and its haplotypes may predict the risk to develop invasive pulmonary aspergillosis and modulate C-reactive protein level. J. Clin. Immunol. 28, 473–485. doi: 10.1007/s10875-008-9197-0
Sainz, J., Perez, E., Hassan, L., Moratalla, A., Romero, A., Collado, M. D., et al. (2007b). Variable number of tandem repeats of TNF receptor type 2 promoter as genetic biomarker of susceptibility to develop invasive pulmonary aspergillosis. Hum. Immunol. 68, 41–50. doi: 10.1016/j.humimm.2006.10.011
Sainz, J., Salas-Alvarado, I., Lopez-Fernandez, E., Olmedo, C., Comino, A., Garcia, F., et al. (2010a). TNFR1 mRNA expression level and TNFR1 gene polymorphisms are predictive markers for susceptibility to develop invasive pulmonary aspergillosis. Int. J. Immunopathol. Pharmacol. 23, 423–436. doi: 10.1177/039463201002300205
Sainz, J., Segura-Catena, J., and Jurado, M. (2010b). Association between genetic polymorphism in the promotor region of CD209 and propensity to develop invasive pulmonary aspergillosis. Methods Find. Exp. Clin. Pharmacol. 32(Suppl. A), 9–13.
Sampaio, E. P., Hsu, A. P., Pechacek, J., Bax, H. I., Dias, D. L., Paulson, M. L., et al. (2013). Signal transducer and activator of transcription 1 (STAT1) gain-of-function mutations and disseminated coccidioidomycosis and histoplasmosis. J. Allergy Clin. Immunol. 131, 1624–1634. doi: 10.1016/j.jaci.2013.01.052
Schwerd, T., Twigg, S. R. F., Aschenbrenner, D., Manrique, S., Miller, K. A., Taylor, I. B., et al. (2017). A biallelic mutation in IL6ST encoding the GP130 co-receptor causes immunodeficiency and craniosynostosis. J. Exp. Med. 214, 2547–2562. doi: 10.1084/jem.20161810
Shahin, T., Aschenbrenner, D., Cagdas, D., Bal, S. K., Conde, C. D., Garncarz, W., et al. (2019). Selective loss of function variants in IL6ST cause Hyper-IgE syndrome with distinct impairments of T-cell phenotype and function. Haematologica 104, 609–621. doi: 10.3324/haematol.2018.194233
Shao, T. Y., Ang, W. X. G., Jiang, T. T., Huang, F. S., Andersen, H., Kinder, J. M., et al. (2019). Commensal Candida albicans positively calibrates systemic Th17 immunological responses. Cell Host Microbe 25, 404–417. doi: 10.1016/j.chom.2019.02.004
Shriber, J., Conlon, K. C., Benedict, K., McCotter, O. Z., and Bell, J. E. (2017). Assessment of vulnerability to coccidioidomycosis in Arizona and California. Int. J. Environ. Res. Public Health 14:E680. doi: 10.3390/ijerph14070680
Smeekens, S. P., Ng, A., Kumar, V., Johnson, M. D., Plantinga, T. S., van Diemen, C., et al. (2013). Functional genomics identifies type I interferon pathway as central for host defense against Candida albicans. Nat. Commun. 4:1342. doi: 10.1038/ncomms2343
Smith, J. A., and Kauffman, C. A. (2009). Endemic fungal infections in patients receiving tumour necrosis factor-alpha inhibitor therapy. Drugs 69, 1403–1415. doi: 10.2165/00003495-200969110-00002
Smith, N. L., Hankinson, J., Simpson, A., Bowyer, P., and Denning, D. W. (2014a). A prominent role for the IL1 pathway and IL15 in susceptibility to chronic cavitary pulmonary aspergillosis. Clin. Microbiol. Infect. 20, O480–O488. doi: 10.1111/1469-0691.12473
Smith, N. L., Hankinson, J., Simpson, A., Denning, D. W., and Bowyer, P. (2014b). Reduced expression of TLR3, TLR10 and TREM1 by human macrophages in Chronic cavitary pulmonary aspergillosis, and novel associations of VEGFA, DENND1B and PLAT. Clin. Microbiol. Infect. 20, O960–O968. doi: 10.1111/1469-0691.12643
Sobel, J. D. (2007). Vulvovaginal candidosis. Lancet 369, 1961–1971. doi: 10.1016/S0140-6736(07)60917-9
Soll, D. R., Galask, R., Schmid, J., Hanna, C., Mac, K., and Morrow, B. (1991). Genetic dissimilarity of commensal strains of Candida spp. carried in different anatomical locations of the same healthy women. J. Clin. Microbiol. 29, 1702–1710. doi: 10.1128/JCM.29.8.1702-1710.1991
Sorci, G., Giovannini, G., Riuzzi, F., Bonifazi, P., Zelante, T., Zagarella, S., et al. (2011). The danger signal S100B integrates pathogen- and danger-sensing pathways to restrain inflammation. PLoS Pathog. 7:e1001315. doi: 10.1371/journal.ppat.1001315
Spinner, M. A., Ker, J. P., Stoudenmire, C. J., Fadare, O., Mace, E. M., Orange, J. S., et al. (2016). GATA2 deficiency underlying severe blastomycosis and fatal herpes simplex virus-associated hemophagocytic lymphohistiocytosis. J. Allergy Clin. Immunol. 137, 638–640. doi: 10.1016/j.jaci.2015.07.043
Stappers, M. H. T., Clark, A. E., Aimanianda, V., Bidula, S., Reid, D. M., Asamaphan, P., et al. (2018). Recognition of DHN-melanin by a C-type lectin receptor is required for immunity to Aspergillus. Nature 555, 382–386. doi: 10.1038/nature25974
Stevens, D. A. (1995). Coccidioidomycosis. N. Engl. J. Med. 332, 1077–1082. doi: 10.1056/NEJM199504203321607
Swamydas, M., Gao, J. L., Break, T. J., Johnson, M. D., Jaeger, M., Rodriguez, C. A., et al. (2016). CXCR1-mediated neutrophil degranulation and fungal killing promote Candida clearance and host survival. Sci. Transl. Med. 8:322ra310. doi: 10.1126/scitranslmed.aac7718
Tam, J. M., Reedy, J. L., Lukason, D. P., Kuna, S. G., Acharya, M., Khan, N. S., et al. (2019). Tetraspanin CD82 organizes dectin-1 into signaling domains to mediate cellular responses to Candida albicans. J. Immunol. 202, 3256–3266. doi: 10.4049/jimmunol.1801384
Tanveer, F., Younas, M., and Johnson, L. B. (2019). Chronic progressive disseminated histoplasmosis in a renal transplant recipient. Med. Mycol. Case Rep. 23, 53–54. doi: 10.1016/j.mmcr.2018.12.006
Taylor, G. R., Kropp, K. D., and Molina, T. C. (1985). Nine-year microflora study of an isolator-maintained immunodeficient child. Appl. Environ. Microbiol. 50, 1349–1356. doi: 10.1128/AEM.50.6.1349-1356.1985
Torres, H. A., Rivero, G. A., Lewis, R. E., Hachem, R., Raad, I. I., and Kontoyiannis, D. P. (2003). Aspergillosis caused by non-fumigatus Aspergillus species: risk factors and in vitro susceptibility compared with Aspergillus fumigatus. Diagn. Microbiol. Infect. Dis. 46, 25–28. doi: 10.1016/S0732-8893(03)00013-0
Torres, J. M., Martinez-Barricarte, R., Garcia-Gomez, S., Mazariegos, M. S., Itan, Y., Boisson, B., et al. (2014). Inherited BCL10 deficiency impairs hematopoietic and nonhematopoietic immunity. J. Clin. Invest. 124, 5239–5248. doi: 10.1172/JCI77493
Usluogullari, B., Gumus, I., Gunduz, E., Kaygusuz, I., Simavli, S., Acar, M., et al. (2014). The role of human Dectin-1 Y238X gene polymorphism in recurrent vulvovaginal candidiasis infections. Mol. Biol. Rep. 41, 6763–6768. doi: 10.1007/s11033-014-3562-2
Vaaler, A. K., Bradsher, R. W., and Davies, S. F. (1990). Evidence of subclinical blastomycosis in forestry workers in northern Minnesota and northern Wisconsin. Am. J. Med. 89, 470–476. doi: 10.1016/0002-9343(90)90378-Q
Vaezi, A., Fakhim, H., Abtahian, Z., Khodavaisy, S., Geramishoar, M., Alizadeh, A., et al. (2018). Frequency and geographic distribution of CARD9 mutations in patients with severe fungal infections. Front. Microbiol. 9:2434. doi: 10.3389/fmicb.2018.02434
Valdivia, L., Nix, D., Wright, M., Lindberg, E., Fagan, T., Lieberman, D., et al. (2006). Coccidioidomycosis as a common cause of community-acquired pneumonia. Emerging Infect. Dis. 12, 958–962. doi: 10.3201/eid1206.060028
van der Graaf, C. A., Netea, M. G., Franke, B., Girardin, S. E., van der Meer, J. W., and Kullberg, B. J. (2006a). Nucleotide oligomerization domain 2 (Nod2) is not involved in the pattern recognition of Candida albicans. Clin. Vaccine Immunol. 13, 423–425. doi: 10.1128/CVI.13.3.423-425.2006
van der Graaf, C. A., Netea, M. G., Morre, S. A., Den Heijer, M., Verweij, P. E., Van der Meer, J. W., et al. (2006b). Toll-like receptor 4 Asp299Gly/Thr399Ile polymorphisms are a risk factor for Candida bloodstream infection. Eur. Cytokine Netw. 17, 29–34.
Velazquez-Hernandez, N., Aguilar-Duran, M., Perez-Alamos, A. R., Estrada-Martinez, S., Salas-Pacheco, J. M., Sanchez-Anguiano, L. F., et al. (2017). Lack of association between mannose-binding lectin 2 codons 54 and 57 gene polymorphisms and cervicovaginal infections in Mexican women. Int. J. Biomed. Sci. 13, 79–83.
Vergidis, P., Avery, R. K., Wheat, L. J., Dotson, J. L., Assi, M. A., Antoun, S. A., et al. (2015). Histoplasmosis complicating tumor necrosis factor-alpha blocker therapy: a retrospective analysis of 98 cases. Clin. Infect. Dis. 61, 409–417. doi: 10.1093/cid/civ299
Verma, A., Gaffen, S. L., and Swidergall, M. (2017). Innate immunity to Mucosal Candida infections. J. Fungi 3:60. doi: 10.3390/jof3040060
Vinh, D. C., Masannat, F., Dzioba, R. B., Galgiani, J. N., and Holland, S. M. (2009). Refractory disseminated coccidioidomycosis and mycobacteriosis in interferon-gamma receptor 1 deficiency. Clin. Infect. Dis. 49, e62–e65. doi: 10.1086/605532
Vinh, D. C., Schwartz, B., Hsu, A. P., Miranda, D. J., Valdez, P. A., Fink, D., et al. (2011). Interleukin-12 receptor beta1 deficiency predisposing to disseminated Coccidioidomycosis. Clin. Infect. Dis. 52, e99–e102. doi: 10.1093/cid/ciq215
Vinh, D. C., Sugui, J. A., Hsu, A. P., Freeman, A. F., and Holland, S. M. (2010). Invasive fungal disease in autosomal-dominant hyper-IgE syndrome. J. Allergy Clin. Immunol. 125, 1389–1390. doi: 10.1016/j.jaci.2010.01.047
Viriyakosol, S., Jimenez Mdel, P., Gurney, M. A., Ashbaugh, M. E., and Fierer, J. (2013). Dectin-1 is required for resistance to coccidioidomycosis in mice. MBio 4, e00597–e00512. doi: 10.1128/mBio.00597-12
Viriyakosol, S., Walls, L., Okamoto, S., Raz, E., Williams, D. L., and Fierer, J. (2018). Myeloid differentiation factor 88 and interleukin-1R1 signaling contribute to resistance to coccidioides immitis. Infect. Immun. 86, e00028–e00018. doi: 10.1128/IAI.00028-18
Vogel, T. P., Milner, J. D., and Cooper, M. A. (2015). The ying and yang of STAT3 in human disease. J. Clin. Immunol. 35, 615–623. doi: 10.1007/s10875-015-0187-8
Wang, H., LeBert, V., Hung, C. Y., Galles, K., Saijo, S., Lin, X., et al. (2014). C-type lectin receptors differentially induce th17 cells and vaccine immunity to the endemic mycosis of North America. J. Immunol. 192, 1107–1119. doi: 10.4049/jimmunol.1302314
Wang, H., Li, M., Hung, C. Y., Sinha, M., Lee, L. M., Wiesner, D. L., et al. (2016). MyD88 shapes vaccine immunity by extrinsically regulating survival of CD4+ T cells during the contraction phase. PLoS Pathog. 12:e1005787. doi: 10.1371/journal.ppat.1005787
Ward, T. L., Dominguez-Bello, M. G., Heisel, T., Al-Ghalith, G., Knights, D., and Gale, C. A. (2018). Development of the human Mycobiome over the first month of life and across body sites. mSystems 3, e00140–e00117. doi: 10.1128/mSystems.00140-17
Wheat, J., Myint, T., Guo, Y., Kemmer, P., Hage, C., Terry, C., et al. (2018). Central nervous system histoplasmosis: multicenter retrospective study on clinical features, diagnostic approach and outcome of treatment. Medicine 97:e0245. doi: 10.1097/MD.0000000000010245
Wheat, L. J., Chetchotisakd, P., Williams, B., Connolly, P., Shutt, K., and Hajjeh, R. (2000). Factors associated with severe manifestations of histoplasmosis in AIDS. Clin. Infect. Dis. 30, 877–881. doi: 10.1086/313824
Wheat, L. J., Slama, T. G., Eitzen, H. E., Kohler, R. B., French, M. L., and Biesecker, J. L. (1981). A large urban outbreak of histoplasmosis: clinical features. Ann. Intern. Med. 94, 331–337. doi: 10.7326/0003-4819-94-3-331
Whiston, E., and Taylor, J. W. (2015). Comparative phylogenomics of pathogenic and nonpathogenic species. G3 6, 235–244. doi: 10.1534/g3.115.022806
White, T. C., Findley, K., Dawson, T. L. Jr., Scheynius, A., Boekhout, T., Cuomo, C. A., et al. (2014). Fungi on the skin: dermatophytes and Malassezia. Cold Spring Harb. Perspect. Med. 4:a019802. doi: 10.1101/cshperspect.a019802
Williams, B., Douglas, P., Roca Barcelo, A., Hansell, A. L., and Hayes, E. (2019). Estimating Aspergillus fumigatus exposure from outdoor composting activities in England between 2005 and 14. Waste Manag. 84, 235–244. doi: 10.1016/j.wasman.2018.11.044
Woehrle, T., Du, W., Goetz, A., Hsu, H. Y., Joos, T. O., Weiss, M., et al. (2008). Pathogen specific cytokine release reveals an effect of TLR2 Arg753Gln during Candida sepsis in humans. Cytokine 41, 322–329. doi: 10.1016/j.cyto.2007.12.006
Wojtowicz, A., Lecompte, T. D., Bibert, S., Manuel, O., Rueger, S., Berger, C., et al. (2015b). PTX3 polymorphisms and invasive mold infections after solid organ transplant. Clin. Infect. Dis. 61, 619–622. doi: 10.1093/cid/civ386
Wojtowicz, A., Gresnigt, M. S., Lecompte, T., Bibert, S., Manuel, O., Joosten, L. A., et al. (2015a). IL1B and DEFB1 polymorphisms increase susceptibility to invasive mold infection after solid-organ transplantation. J. Infect. Dis. 211, 1646–1657. doi: 10.1093/infdis/jiu636
Wolach, B., Gavrieli, R., de Boer, M., van Leeuwen, K., Berger-Achituv, S., Stauber, T., et al. (2017). Chronic granulomatous disease: clinical, functional, molecular, and genetic studies. The Israeli experience with 84 patients. Am. J. Hematol. 92, 28–36. doi: 10.1002/ajh.24573
Wuthrich, M., LeBert, V., Galles, K., Hu-Li, J., Ben-Sasson, S. Z., Paul, W. E., et al. (2013). Interleukin 1 enhances vaccine-induced antifungal T-helper 17 cells and resistance against Blastomyces dermatitidis infection. J. Infect. Dis. 208, 1175–1182. doi: 10.1093/infdis/jit283
Yapar, N. (2014). Epidemiology and risk factors for invasive candidiasis. Ther. Clin. Risk Manag. 10, 95–105. doi: 10.2147/TCRM.S40160
Zaas, A. K., Liao, G., Chien, J. W., Weinberg, C., Shore, D., Giles, S. S., et al. (2008). Plasminogen alleles influence susceptibility to invasive aspergillosis. PLoS Genet. 4:e1000101. doi: 10.1371/journal.pgen.1000101
Zahedi, N., Abedian Kenari, S., Mohseni, S., Aslani, N., Ansari, S., and Badali, H. (2016). Is human Dectin-1 Y238X gene polymorphism related to susceptibility to recurrent vulvovaginal candidiasis? Curr. Med. Mycol. 2, 15–19. doi: 10.18869/acadpub.cmm.2.3.15
Zangl, I., Pap, I. J., Aspöck, C., and Schüller, C. (2019). The role of Lactobacillus species in the control of Candida via biotrophic interactions. Microb. Cell 7, 1–14. doi: 10.15698/mic2020.01.702
Zerbe, C. S., and Holland, S. M. (2005). Disseminated histoplasmosis in persons with interferon-gamma receptor 1 deficiency. Clin. Infect. Dis. 41, e38–e41. doi: 10.1086/432120
Zhan, M., Xu, B., Zhao, L., Li, B., Xu, L., Sun, Q., et al. (2018). The serum level of IL-1B correlates with the activity of chronic pulmonary Aspergillosis. Can. Respir. J. 2018:8740491. doi: 10.1155/2018/8740491
Zhang, Q., Boisson, B., Beziat, V., Puel, A., and Casanova, J. L. (2018). Human hyper-IgE syndrome: singular or plural? Mamm. Genome 29, 603–617. doi: 10.1007/s00335-018-9767-2
Zheng, J., van de Veerdonk, F. L., Crossland, K. L., Smeekens, S. P., Chan, C. M., Al Shehri, T., et al. (2015). Gain-of-function STAT1 mutations impair STAT3 activity in patients with chronic mucocutaneous candidiasis (CMC). Eur. J. Immunol. 45, 2834–2846. doi: 10.1002/eji.201445344
Zhernakova, A., Elbers, C. C., Ferwerda, B., Romanos, J., Trynka, G., Dubois, P. C., et al. (2010). Evolutionary and functional analysis of celiac risk loci reveals SH2B3 as a protective factor against bacterial infection. Am. J. Hum. Genet. 86, 970–977. doi: 10.1016/j.ajhg.2010.05.004
Zhong, X., Chen, B., Yang, L., and Yang, Z. (2018). Molecular and physiological roles of the adaptor protein CARD9 in immunity. Cell Death Dis. 9:52. doi: 10.1038/s41419-017-0084-6
Keywords: genes, fungi, immunity, innate, polymorphism
Citation: Merkhofer RM and Klein BS (2020) Advances in Understanding Human Genetic Variations That Influence Innate Immunity to Fungi. Front. Cell. Infect. Microbiol. 10:69. doi: 10.3389/fcimb.2020.00069
Received: 03 December 2019; Accepted: 12 February 2020;
Published: 28 February 2020.
Edited by:
Rebecca Drummond, University of Birmingham, United KingdomReviewed by:
Michail Lionakis, National Institute of Allergy and Infectious Diseases (NIAID), United StatesCopyright © 2020 Merkhofer and Klein. This is an open-access article distributed under the terms of the Creative Commons Attribution License (CC BY). The use, distribution or reproduction in other forums is permitted, provided the original author(s) and the copyright owner(s) are credited and that the original publication in this journal is cited, in accordance with accepted academic practice. No use, distribution or reproduction is permitted which does not comply with these terms.
*Correspondence: Bruce S. Klein, YnNrbGVpbkB3aXNjLmVkdQ==
Disclaimer: All claims expressed in this article are solely those of the authors and do not necessarily represent those of their affiliated organizations, or those of the publisher, the editors and the reviewers. Any product that may be evaluated in this article or claim that may be made by its manufacturer is not guaranteed or endorsed by the publisher.
Research integrity at Frontiers
Learn more about the work of our research integrity team to safeguard the quality of each article we publish.