- Laboratory of Immunology and Cell Biology, Institute of Biotechnology, Life Sciences Center, Vilnius University, Vilnius, Lithuania
Bacterial vaginosis (BV) is a vaginal anaerobic dysbiosis that affects women of reproductive age worldwide. BV is microbiologically characterized by the depletion of vaginal lactobacilli and the overgrowth of anaerobic bacterial species. Accumulated evidence suggests that Gardnerella spp. have a pivotal role among BV-associated bacteria in the initiation and development of BV. However, Gardnerella spp. often colonize healthy women. Lactobacillus iners is considered as a prevalent constituent of healthy vaginal microbiota, and is abundant in BV. Gardnerella spp. and L. iners secrete the toxins vaginolysin (VLY) and inerolysin (INY), which have structural and activity features attributed to cholesterol-dependent cytolysins (CDCs). CDCs are produced by many pathogenic bacteria as virulence factors that participate in various stages of disease progression by forming lytic and non-lytic pores in cell membranes or via pore-independent pathways. VLY is expressed in the majority of Gardnerella spp. isolates; less is known about the prevalence of the gene that encodes INY. INY is a classical CDC; membrane cholesterol acts a receptor for INY. VLY uses human CD59 as its receptor, although cholesterol remains indispensable for VLY pore-forming activity. INY-induced damage of artificial membranes is directly dependent on cholesterol concentration in the bilayer, whereas VLY-induced damage occurs with high levels of membrane cholesterol (>40 mol%). VLY primarily forms membrane-embedded complete rings in the synthetic bilayer, whereas INY forms arciform structures with smaller pore sizes. VLY activity is high at elevated pH, which is characteristic of BV, whereas INY activity is high at more acidic pH, which is specific for a healthy vagina. Increased VLY levels in vaginal mucosa in vivo were associated with clinical indicators of BV. However, experimental evidence is lacking for the specific roles of VLY and INY in BV. The interplay between vaginal bacterial species affects the expression of the gene encoding VLY, thereby modulating the virulence of Gardnerella spp. This review discusses the current evidence for VLY and INY cytolysins, including their structures and activities, factors affecting their expression, and their potential impacts on the progression of anaerobic dysbiosis.
Introduction
Vaginal bacterial species composition and abundance were compared in reproductive-aged women (Ravel et al., 2011; Fettweis et al., 2014), and the results revealed that Lactobacillus-dominated microbial communities are the hallmark of a healthy vagina (Ma et al., 2012; Vaneechoutte, 2017a). Otherwise healthy asymptomatic women harboring a polymicrobial mixture of anaerobic bacteria with few lactic acid-producing lactobacilli also represents a healthy vagina (Ma et al., 2012; Smith and Ravel, 2017), although the lower numbers of lactobacilli reduce their protection against pathogenic microorganisms (Anahtar et al., 2015). High-throughput sequencing and microscopy studies to classify vaginal species provide a better understanding of clinical conditions associated with the disturbance of healthy, Lactobacillus-dominated microbiota, which lead to bacterial vaginosis (BV) (Nugent et al., 1991; Srinivasan et al., 2012) and the recently identified clinical condition called aerobic vaginitis (Donders et al., 2017).
BV is the vaginal condition associated with poor reproductive and obstetric sequelae (Kenyon et al., 2013; van de Wijgert and Jespers, 2017). BV is microbiologically characterized by the depletion of most vaginal Lactobacillus species and the overgrowth of diverse anaerobes (Srinivasan and Fredricks, 2008; Huang et al., 2014; Onderdonk et al., 2016). Intensive DNA-based studies on vaginal microbiota did not identify the etiology of BV and suggest that BV is a complex condition that may involve several different diseases (Cerca et al., 2017). This hypothesis was proposed because the primary causative pathogen(s) of BV have not been unambiguously determined (Muzny et al., 2019). Further, epidemiological data suggest that BV may be sexually transmitted (Fethers et al., 2008; Swidsinski et al., 2014).
Deep sequencing showed that BV is associated with an array of anaerobic bacteria (Zozaya-Hinchliffe et al., 2010; Srinivasan et al., 2012). Facultative anaerobic bacterial species of the genus Gardnerella have been recovered from vaginal samples of almost all women with BV (Fredricks et al., 2007; Srinivasan et al., 2012). Gardnerella spp. have higher virulence potential than other BV-associated bacteria, thereby supporting its possible role in BV development (Patterson et al., 2010; Muzny and Schwebke, 2013; Robinson et al., 2019). The key feature of BV is the presence of a bacterial biofilm on vaginal epithelial cells, which predominantly consists of Gardnerella spp. and other incorporated bacterial groups (Swidsinski et al., 2005, 2014). The impact of neighboring BV-associated bacteria has been analyzed for effects on BV pathogenesis (Muzny et al., 2018; Castro et al., 2019; Gilbert et al., 2019). However, the pivotal role of Gardnerella in BV has been debated because of its presence in the healthy vagina. There are likely differences in virulence among Gardnerella strains, and the expression of virulence traits may increase under certain conditions (Hickey and Forney, 2014; Janulaitiene et al., 2018; Castro et al., 2019; Muzny et al., 2019).
Lactobacillus iners is another bacterium found at high levels in healthy and BV-positive women (Srinivasan et al., 2012; Petrova et al., 2015), although the hallmark of BV is a depletion of lactobacilli. L. iners-dominated microbiota are often detected at the transitional stage between normal and BV conditions (Petrova et al., 2017; Vaneechoutte, 2017b) and during menses (Santiago Lopes dos Santos et al., 2012); therefore, these microbial populations are considered to be less stable. L. iners predominantly produces L-lactic acid, which has lower protective capacity than the D-lactic acid released by other vaginal lactobacilli (Witkin and Linhares, 2017). The adaptation of L. iners to different environmental conditions may be due to the repertoire of gene expression, which ensures competitive adaptability and survival (Macklaim et al., 2011; France et al., 2016). Different L. iners lineages or groups with different adaptive properties may have functional roles in this adaptation (Petrova et al., 2017).
Both Gardnerella spp. and L. iners are present in the healthy human vagina and during anaerobic dysbiosis, and both secrete cholesterol-dependent cytolysins (CDCs) that belong to a common family of pore-forming toxins (PFTs) (Alouf, 2003; Christie et al., 2018). CDCs are produced by many pathogenic Gram-positive bacteria, and are recognized as virulence factors that participate in various stages of disease progression (Los et al., 2013). CDCs are secreted by a few Gram-negative bacteria that inhabit anaerobic soils and do not colonize humans or animals (Hotze et al., 2013). CDCs are cytotoxic to eukaryotic cells, including erythrocytes, and are known as cytolysins or hemolysins. L. iners secretes the CDC toxin inerolysin (INY) (Rampersaud et al., 2011), whereas Gardnerella spp. secrete the CDC toxin vaginolysin (VLY) (Rottini et al., 1990; Cauci et al., 1993; Gelber et al., 2008). VLY-mediated cell lysis is visible by cultivation of Gardnerella spp. on solid agar supplemented with human blood; β-hemolysis surrounding colonies indicates complete lysis of erythrocytes in the medium. The effect of these CDC toxins could be prerequisite for the survival and adaptation of Gardnerella and L. iners under diverse environmental conditions and during the development of BV. This review discussed the current knowledge, recent evidence, and implications relating to VLY and INY.
Genetic Characteristics
The cytolysin-coding genes vly and iny are single-copy genes in the chromosomes of Gardnerella spp. and L. iners, respectively. Both genes were cloned and sequenced (Gelber et al., 2008; Rampersaud et al., 2011). Among Gardnerella spp. isolates, VLY exhibits higher amino acid sequence variations, especially in the N-terminal region, than other CDCs. However, the detected amino acid substitutions did not affect the cytolytic activity of VLY (Pleckaityte et al., 2012). The VLY protein is 56 kDa, and INY protein is 57 kDa. Both toxins contain a signal sequence at the N-terminus that ensures secretion via a type II secretion pathway (Tweten, 2005). A recent study reported that VLY was detected in extracellular vesicles (EVs) produced by the Gardnerella vaginalis ATCC 14019 strain (Shishpal et al., 2019). EV-mediated delivery of toxins and other virulence factors has been identified for many pathogenic Gram-positive bacteria (Brown et al., 2015; Liu et al., 2018). It is unclear whether the majority of VLY is secreted via EVs and how this pathway promotes Gardnerella spp. survival, colonization, and pathogenesis.
Genetic and phenotypic heterogeneity in the genus Gardnerella enabled the identification of genomic subgroups (Ahmed et al., 2012; Balashov et al., 2014; Schellenberg et al., 2016). Multiple Gardnerella subgroups were detected in non-cultured vaginal samples. Gardnerella multigroup communities were positively associated with BV (Balashov et al., 2014; Janulaitiene et al., 2017; Shipitsyna et al., 2019). A recent study identified and described 13 new species within the genus Gardnerella, including Gardnerella leopoldii, Gardnerella swidsinskii, Gardnerella piotii, and G. vaginalis (Vaneechoutte et al., 2019). It is likely that species or genomic subgroups are specifically associated with BV due to differences in their virulence potential. In our study (Janulaitiene et al., 2018), the majority of isolates of subgroup 4 that were associated with healthy microbiota produced a low amount of VLY in vitro compared to other groups. We identified isolates of subgroup 2 that were the vly-negative. Isolates with and without the vly gene colonized the same woman (Janulaitiene et al., 2018). By contrast, iny-negative isolates have not been identified, and INY was detected in all tested supernatants of cultured L. iners strains (Rampersaud et al., 2011).
Structural Features of CDC Proteins and an Overview of the Pore-Forming Mechanism
The structures of CDCs from various bacterial genera were determined and revealed a four-domain structural organization (Rossjohn et al., 1997; Polekhina et al., 2004; Lawrence et al., 2015, 2016) (Figure 1A). The key sequence motifs involved in the recognition of cholesterol and membrane binding are highly conserved across all known CDCs.
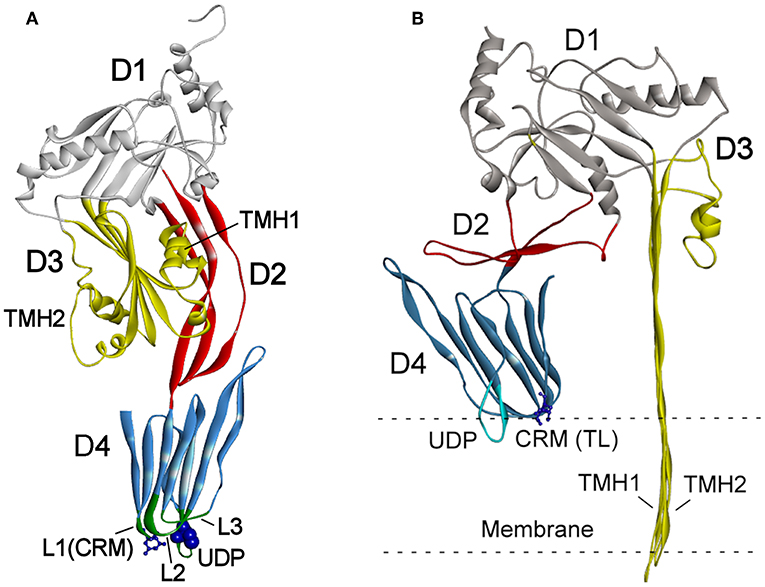
Figure 1. (A) Schematic presentation of the crystal structure of PLY (PDB ID: 4QQA; Park et al., 2016). (B) One subunit of oligomeric PLY inserted into a membrane in the cryoEM structure (PDB ID: 5LY6; van Pee et al., 2017). The features of the structure are marked as follows: domains D1–D4 (highlighted in different colors), loops L1–L3, undecapeptide (UDP) in D4, the two-residue (Thr-Leu pair shown as sticks) cholesterol recognition motive (CRM) in loop L1, and the Cys residue in UDP (shown as blue spheres). The transmembrane hairpins TMH1 and TMH2 of D3 span the membrane in (B).
The mechanisms of CDC interaction with membranes and pore formation have been extensively studied for perfringolysin (PFO) from Clostridium perfringens (Rossjohn et al., 1997; Ramachandran et al., 2002) and pneumolysin (PLY) from Streptococcus pneumoniae (Gilbert et al., 1998; Vögele et al., 2019). Cytolysins are secreted as water-soluble monomers (Tweten, 2005), which recognize membrane cholesterol via the cholesterol recognition motive (CRM) composed of the Thr-Leu pair in the L1 loop of domain D4 (Farrand et al., 2010) (Figure 1A). Recognition of cholesterol triggers the insertion of D4 structural components, an 11 amino acid undecapeptide (UDP) and the nearby L2–L3 loops, into a membrane that provides anchorage and stability of the monomer (Dowd et al., 2012; Christie et al., 2018). The conservative UDP sequence in the majority CDCs, including INY, contains a Cys residue (Figure 2) that, in the reduced state, ensures maximal activity of toxins (Tweten, 2005; Rampersaud et al., 2011). Therefore, CDCs are also known as thiol-activated cytolysins. After membrane binding, structural changes occur in the protein that enable monomer-monomer interactions. The monomers oligomerize into rings and form a prepore structure on the membrane surface. The prepore is an SDS-resistant oligomeric complex characterized by a dense structure of ordinated and correctly oriented monomers. The prepore does not perforate the membrane (Hotze et al., 2002; Ramachandran et al., 2005). The transition from prepore to pore is accompanied by dramatic structural changes in domain D3, leading to the conversion of two helix bundles of each monomer to transmembrane β-hairpins (TMH) (Hotze and Tweten, 2012). Bilayer perforation is mediated by insertion of the TMH regions, and requires the helices to closely approach a membrane (Figure 1B). This is achieved by rotation of domain D2 following disruption of the D3 interface (Czajkowsky et al., 2004; Leung et al., 2014; van Pee et al., 2017). The β-barrel of the membrane-embedded pore complex (Figure 3A) is hydrophobic on the outside and hydrophilic inside (van Pee et al., 2017; Christie et al., 2018). This structure favors the displacement of membrane lipids and stimulates water influx, which finalizes the formation of a water-filled pore (Vögele et al., 2019).
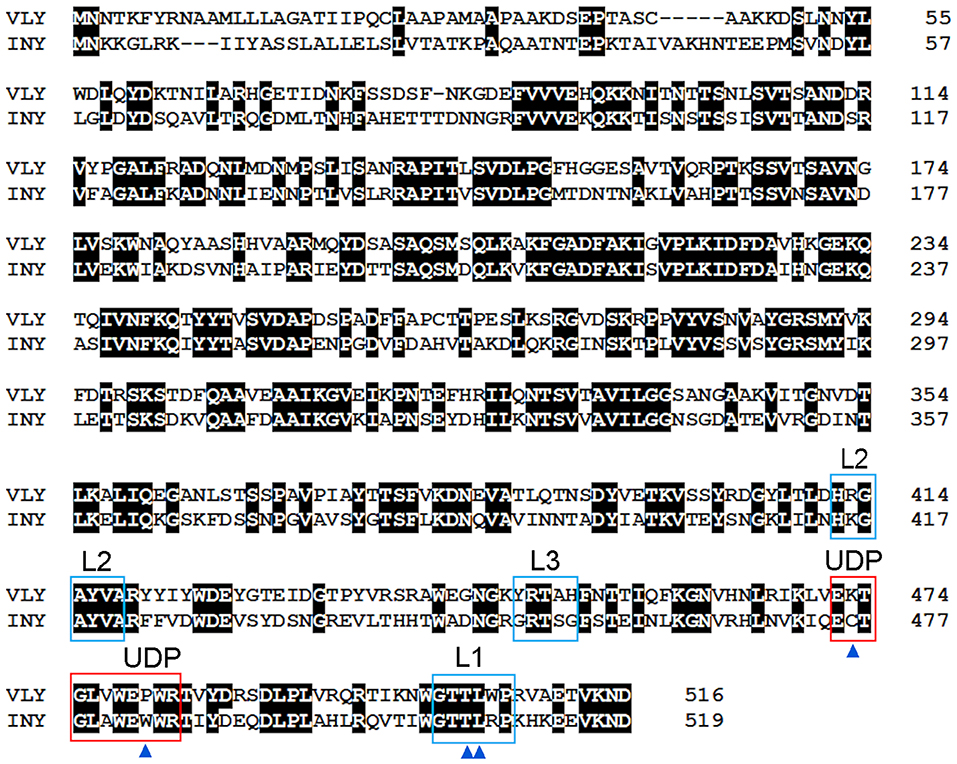
Figure 2. Amino acid sequence alignment of VLY (accession no. ACD63042) and INY (accession no. WP_006730404). The sequences were aligned using Clustal W (Aiyar, 2000). Strictly conserved residues are indicated with a black background. Loops L1–L3 and the undecapeptide (UDP) are framed in blue and red, respectively. Cys residue in UDP of INY, the Pro/Trp in UDP, and the Thr-Leu pair (CMR) in L1 are indicated by blue triangles.
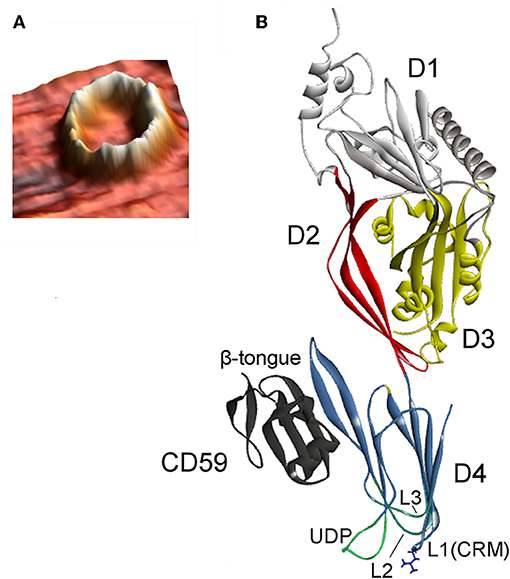
Figure 3. (A) Atomic force microscopy three-dimensional topography image of the oligomeric structure obtained after reconstitution of VLY into a cholesterol-rich artificial bilayer. Calculated protrusion of the ring-shaped defect above the membrane is consistent with the height of inserted pore (reproduced with permission from Ragaliauskas et al., 2019). (B) Crystal structure of VLY in complex with hCD59 (PDB ID: 5IMY; Lawrence et al., 2016). VLY interacts with hCD59 via the β-tongue located in D4, similarly as ILY. Loop L1 contains the Thr-Leu (TL) pair of CRM.
Characteristics of Vaginolysin and Inerolysin Activities
Cholesterol Is Not a Single CDCs' Receptor: The Vaginolysin Case
Cholesterol embedded into a cell membrane has been identified as a common and indispensable binding target for the majority CDCs (Hotze and Tweten, 2012). A distinct group of CDCs primarily bind to human complement glycoprotein CD59 (hCD59) rather than to cholesterol. Three members of this distinct group include VLY, Streptococcus intermedius intermedilysin (ILY), and Streptococcus mitis lectinolysin (Giddings et al., 2004; Gelber et al., 2008; Wickham et al., 2011). The glycosylphosphatidylinositol (GPI)-anchored human cell-surface receptor hCD59 interacts with the membrane attack complex (MAC) components C8α and C9, thereby blocking the accidental activity of MAC (Iacovache and van der Goot, 2004; Huang et al., 2007). Thus, hCD59 which ensures the unwanted lysis of host cells due to the activity of complement, serves as a receptor for some bacterial CDCs. Wickham et al. (2011) investigated ILY and MAC binding to hCD59; mutation of amino acid residues in hCD59 that modulate binding to ILY affects its protection against MAC-mediated lysis. The authors proposed that the existence of hCD59-responsive toxins may indicate microbial coevolution with humans, as the primary role of hCD59 is to block unwanted lysis of host cells.
The pore-forming mechanism of hCD59-dependent cytolysins is best-studied for ILY (LaChapelle et al., 2009; Farrand et al., 2010). When encountering host cells, ILY binds to hCD59 via the β-tongue located in D4 (Figure 3B). This event triggers the same structural changes as that of non-hCD59-dependent CDCs, but a pore does not form due to the lack of cholesterol. Contact between ILY and hCD59 allows the CRM-cholesterol interaction that triggers insertion of the L1–L3 loops into the bilayer (Farrand et al., 2010; Hotze and Tweten, 2012). After ILY is anchored to the membrane via the inserted loops, it disengages from hCD59 during the transition from a prepore to a pore. The release of hCD59 favors structural changes that are necessary for pore formation, as the increased binding affinity between ILY and hCD59 slows the rate of the prepore to pore transition and reduces cell lysis (Wickham et al., 2011). Boyd et al. (2016) recently reported that ILY interaction with hCD59, rather than with cholesterol, induced structural changes leading to collapse of the prepore structure, whereas cholesterol was required only for the final membrane perforation stage.
VLY-mediated cytotoxic activity was detected on cells that expressed hCD59 on their surface (Gelber et al., 2008; Zilnyte et al., 2015). Antibodies against VLY inhibited its cytolytic activity, demonstrating the specificity of VLY interaction with the cells (Zvirbliene et al., 2010; Pleckaityte et al., 2011). Membrane cholesterol is indispensable for the pore-forming activity of VLY and other known hCD59-dependent CDCs (Giddings et al., 2004; Gelber et al., 2008). ILY is a human-specific cytolysin (Giddings et al., 2004). By contrast, the cells lacking hCD59 were susceptible to VLY-mediated lysis, albeit at the elevated VLY concentrations (Zilnyte et al., 2015). Analysis of the crystal structure of VLY complexed to hCD59 (Figure 3B) revealed novel information regarding the dualistic activity of VLY (Lawrence et al., 2016). The stable VLY-hCD59 complex used for structural studies was obtained only with mutant VLY, which has limited capacity to oligomerize but retains its ability to interact with the receptor. The attempts of Lawrence et al. (2016) and our group (unpublished observations) to crystalize VLY alone were not successful.
The conformation of the L1 loop, which contains the CRM, is critical for cytolysin binding to cholesterol (Farrand et al., 2010; Lawrence et al., 2016). The structure of the UDP loop affects cholesterol-mediated membrane binding and D3 structural changes, which are the principal steps in the allosteric pore-formation pathway (Dowd et al., 2012). Superimposition of available CDC structures indicates that amino acids in the UPD loop of non-hCD59-dependent cytolysins interact with the L1 loop via hydrogen bonds, which leads to the proper conformation of L1 for initial binding with membrane cholesterol (Hotze and Tweten, 2012; Lawrence et al., 2016). The UDP loop in hCD59-dependent cytolysins contains Pro instead of Trp (Figure 2), which does not promote interaction of the neighboring amino acids with the L1 loop to ensure the proper orientation for cholesterol binding (Lawrence et al., 2016). Thus, hCD59-dependent cytolysins bind hCD59 instead of membrane cholesterol. Analysis of the two monomers in the VLY-hCD59 complex revealed that the UDP loop can adopt two conformations that are characteristic of either classical non-hCD59-dependent CDCs (e.g., PFO) or strictly hCD59-dependent ILY. The adopted UDP conformations predict which primary binding target, hCD59 or cholesterol, is selected in the host membrane to execute VLY pore-forming activity. It is likely that hCD59 is a more effective binding target than cholesterol for VLY interaction with the host membrane (Lawrence et al., 2016). INY is a classical non-hCD59-dependent cytolysin, although its primary amino acid sequence shows the greatest similarity to the CD59-dependent CDCs ILY (sequence identity 48.8%, Rampersaud et al., 2011) and VLY (sequence identity 50.8%, Figure 2).
Membrane Binding
CDCs bind and affect membranes with high cholesterol levels, which contain a cholesterol threshold of >30 mol% of total membrane lipid (Bavdek et al., 2007; Johnson et al., 2012; Zilnyte et al., 2015). The accessibility of membrane cholesterol to CDCs has been a subject of debate. CDCs were proposed to bind to microdomains called lipid rafts, which are enriched with sphingolipids, cholesterol, and proteins (Waheed et al., 2001). However, sphingomyelin, which is an essential component of lipid rafts, inhibited PFO binding to a membrane (Flanagan et al., 2009). Subsequent studies showed that CDC binding required membrane conditions with surface-exposed cholesterol (Nelson et al., 2008). Cholesterol accessibility is modulated by the structures of phospholipid headgroups and acyl chains. Tightly packed phospholipids reduced cholesterol exposure, whereas loosely packed phospholipids enhanced cholesterol availability to CDCs (Rojko and Anderluh, 2015; Chakrabarti et al., 2017; Morton et al., 2019).
The results of electrochemical studies revealed that VLY binds to tethered lipid bilayer membranes (tBLMs) and impacts membrane integrity when cholesterol content was 50 mol% (Ragaliauskas et al., 2019), whereas other methods detected SDS-resistant VLY oligomeric complexes on liposomes containing cholesterol contents of 40–55 mol% (Zilnyte et al., 2015). The fact that VLY did not permeabilize cells in the apical side of a three-dimensional model of vaginal epithelium can be explained by the differential abundance of surface-associated hCD59 and the diverse cholesterol contents in the cell membranes (Garcia et al., 2019). By contrast, INY was active on synthetic membranes with low cholesterol contents of 20–30 mol%. INY binding to tBLMs and the extent of INY-induced membrane damage were directly dependent on membrane cholesterol concentration (Ragaliauskas et al., 2019). INY is likely to be the first CDC with activity on synthetic membranes that does not require a certain cholesterol threshold. However, INY-induced membrane damage was measured in bilayers with specific phospholipid compositions (Ragaliauskas et al., 2019).
Measuring the effects of the lipid environment on the activity of VLY and INY requires detailed investigations. PFO and streptolysin (SLO) have different binding specificities to the same natural and synthetic membranes (Farrand et al., 2015; Johnson et al., 2017). Altering the structure of the L3 loop by mutating critical residues changed the membrane binding parameters of PFO and SLO. CDCs may have evolved the capacity to select lipid environments by manipulating the L3 structure, and thereby display individual specificities for cholesterol (Farrand et al., 2015; Christie et al., 2018).
Pore Size and Membrane Damage
CDCs belong to the β-PFT family because the β-sheet structures cross the host membrane (Figure 1). The distinguishing feature of the CDCs among β-PFTs is the large pore size; more than 30 monomers constitute the full rings, and pore diameters measure up to 30 nm (Hotze and Tweten, 2012). However, PLY (Sonnen et al., 2014), suilysin (Leung et al., 2014), ILY (Boyd et al., 2016), listeriolysin O (LLO) (Mulvihill et al., 2015), VLY, and INY (Ragaliauskas et al., 2019) also form incomplete rings, slits, and arcs that perforate synthetic membranes (Figure 4). Atomic force microscopy results indicated that arciform structures were predominate after reconstitution of INY into cholesterol-rich tBLMs (Figure 4A), whereas membrane-embedded complete rings with a diameter of 26 nm were predominate for VLY (Figure 4B) (Ragaliauskas et al., 2019). The majority of arciform structures produced by VLY and INY are inserted into the lipid bilayer and form a water-filled pore (Ragaliauskas et al., 2019). The VLY-generated pore geometries in tBLMs may differ from those in hCD59-containing membranes.
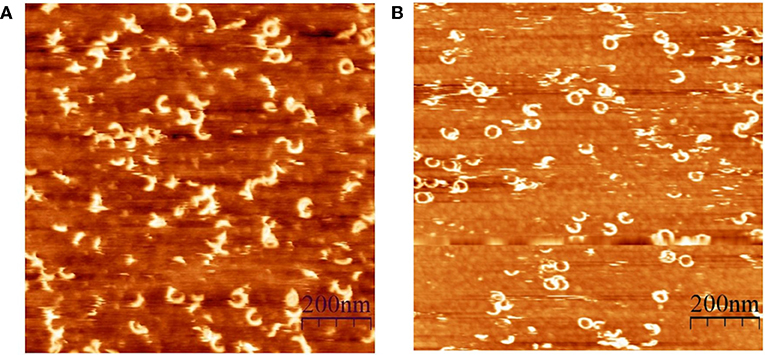
Figure 4. Atomic force microscopy topography images of the ring and arciform-shaped structures obtained after reconstitution of INY (A) and VLY (B) into tBLMs (reproduced with permission from Ragaliauskas et al., 2019).
The arcs and slits that perforate membranes generate smaller pore sizes than those of complete rings. Pore size controls the molecules that can pass through the cell membrane, including ions, small molecules, and proteins (Dal Peraro and van der Goot, 2016). Presumably, pore size depends on the CDC function in the cell. LLO exhibits diverse activities in Listeria monocytogenes infection and triggers various host cell responses (Hamon et al., 2012). LLO generated small membrane perforations that leaked Ca2+ ions and protons from a eukaryotic cell (Shaughnessy et al., 2006), whereas other toxin activities in the host may generate extensive membrane damage (Osborne and Brumell, 2017). Another study concluded that pore size is not completely correlated with PFO- and SLO-mediated membrane damage (Ray et al., 2019). Los et al. (2013) observed differential effects of CDC-induced small and large pores in vitro; however, these differences were not evident in in vivo data. Pore-mediated membrane damage depends on CDCs and host responses to seal the pores and prolong cell survival (Cassidy and O'Riordan, 2013; Brito et al., 2019).
Effect of pH
Several CDCs display pH-dependent activity, which is well-studied for LLO (Schuerch et al., 2005; Bavdek et al., 2012; Podobnik et al., 2015). LLO remains active at acidic pH, whereas it is irreversibly inactivated due to unfolding at neutral pH (Schuerch et al., 2005; Bavdek et al., 2012). The unfolding occurs in the D3 domain, and three acidic residues participate in the denaturation process (Schuerch et al., 2005). Podobnik et al. (2015) suggested that LLO forms pores irrespective of pH, but ionic conductance of the pores is regulated by a His residue that is pH-dependent. The pH-dependent activity of LLO may have biological significance. L. monocytogenes replicates within phagocytic host cells; therefore, the bacterium needs to escape from a vacuole that formed around it during entry to reach the host cell cytosol. L. monocytogenes acidifies the lumen of the vacuole, which activates LLO so that the cytolysin can destroy the vacuole membrane. LLO is not active at neutral pH, thereby preventing host cell destruction (Hamon et al., 2012; Osborne and Brumell, 2017).
INY and VLY effects on human erythrocytes and cholesterol-rich synthetic membranes is pH-sensitive. INY is active at acidic pH and inactive at neutral pH, whereas VLY activity peaks at neutral pH and is marginal at acidic pH (Rampersaud et al., 2011; Ragaliauskas et al., 2019). The pH- and temperature-dependent loss of activity is not related to protein denaturation, which is distinct from the dependence of LLO (Rampersaud et al., 2011; Ragaliauskas et al., 2019). INY regains activity after a pH shift from neutral to acidic (Rampersaud et al., 2011). Ratner et al. found that INY binding to membrane, including the oligomerization step, is pH-independent. The final step, INY membrane insertion, is impaired at neutral pH (Rampersaud et al., 2018). Ragaliauskas et al. (2019) reported that binding of INY and VLY to tBLMs is pH-sensitive. INY demonstrated weak or no binding activity to artificial lipid bilayer at neutral pH. Different INY and VLY activity profiles are consistent with vaginal pH under certain physiological conditions. INY is more active in the pH range characteristic of the healthy vagina, whereas VLY is active at higher pH specific for BV (Donders, 2007). The fact that INY is active in the pH range of 4.5–6.0 suggests that this toxin may be involved in the adaptation and survival of L. iners during transitional stages and dysbiosis.
Cytolysin Concentration in vivo
There are some limitations in interpreting the results of mechanistic and functional studies of PFTs (Los et al., 2013), and these limitations should be considered for interpreting VLY and INY data in various models. Specifically, high doses of purified toxins used in vivo might not be relevant under physiological conditions (Los et al., 2013). The concentration of CDCs may be related to the ability to form pores in host membranes. High CDC doses, which are called lytic concentrations, are linked with the formation of lytic pores that lead to cell death. CDC doses at low, sublytic levels due to expression downregulation or lower bacterial densities is likely to produce non-lytic pores that lead to the modulation of cell signaling cascades (Ratner et al., 2006; Aguilar et al., 2009). CDCs at sublytic concentrations may affect host cells indirectly through pore-independent functions (Meehl and Caparon, 2004; Osborne and Brumell, 2017). However, CDC quantities under physiological conditions are unknown, except for PLY. Unexpectedly high median concentrations of PLY up to 30 μg/mL were detected in the cerebrospinal fluid (CSF) of patients who survived pneumococcal meningitis (Wall et al., 2012). Another study reported PLY concentrations up to 0.18 μg/mL in CSF of patients with meningitis (Spreer et al., 2003). Cell lysis in vitro was not visible at 0.5 μg/mL PLY, although the in vivo lytic capacity of PLY was enhanced by other factors (Spreer et al., 2003; Wippel et al., 2011).
In previous reports, VLY content was measured solely in planktonic cultures of various Gardnerella spp. isolates (Randis et al., 2009; Pleckaityte et al., 2012; Tankovic et al., 2017; Janulaitiene et al., 2018). Recently, VLY contents were measured in vaginal samples of women with healthy vaginal microbiota or anaerobic dysbiosis (Nowak et al., 2018). The median VLY concentrations were highest (3 ng/mL) in vaginal samples with deficient Lactobacillus (Nowak et al., 2018), intermediate concentrations in the presence of primarily L. iners, and lowest concentrations in the presence of primarily L. crispatus. The authors proposed that VLY might represent a marker of BV, as other characteristics of the vaginal samples also were associated with VLY concentrations, including Gardnerella spp. abundance, pH, and Nugent score (Nowak et al., 2018). The VLY concentrations in these vaginal samples (Nowak et al., 2018) were high enough to lyse human erythrocytes in vitro. VLY-mediated cytotoxicity to vaginal epithelial cells in vitro was detected at substantially higher concentrations (Zilnyte et al., 2015).
Interpretations of the Physiological Roles of Inerolysin and Vaginolysin
There is a general concern that studies on the pathogenesis of human vaginal dysbiosis are challenging due to many factors, including the lack of a suitable animal model, vaginal microbiota are a dynamic community, and the impact of host and interplay between neighboring microorganisms. Studies on the role of cytolysins produced by vaginal bacteria have encountered similar difficulties. There are no methods for genetic manipulation of Gardnerella spp. remain a limitation. Cytolytic activity, mechanisms of pore formation, and some biological parameters have been studied using human cells, cell lines, and artificial bilayers. Recently, a three-dimensional polarized human vaginal tissue model has been used to characterize the interaction between vaginal epithelium, G. leopoldii strain (for the strain delineation see Vaneechoutte et al., 2019), and VLY (Garcia et al., 2019).
Cytolysins from Gardnerella spp. and L. iners share features that attribute them to the CDC family, and it is believed that these toxins have a similar role as their family members during infection in vivo. Studies on the functions of VLY and INY in bacterial pathogenesis are still in their infancy. This section focuses on studies that demonstrate the potential role of VLY and INY in the adaptation and survival of bacteria and the progression of vaginal anaerobic dysbiosis.
Ratner et al. reviewed the role of CDCs and other PFTs in bacterial infectious diseases (Los et al., 2013), and identified two main effects of toxins during in vivo infection. (1) The primary effect is induced barrier dysfunction of epithelial and endothelial layers via direct and indirect effects caused by cell attack and host immune response at the site of infection, respectively. Among CDCs, these effects are best-known for PLY and SLO (Meehl and Caparon, 2004; Goldmann et al., 2009; Hupp et al., 2012; Wippel et al., 2013). (2) The second effect was disruption of the host immune response by invading host factors, cytotoxicity toward immune cells, or intracellular survival. Impairment of immune defense was studied for LLO, PLY, PFO, and SLO (Marriott et al., 2008; Domon et al., 2016; Osborne and Brumell, 2017; Bhattacharjee and Keyel, 2018). The identified toxin functions involved pathological conditions associated with a bacterial infection.
The study of Los et al. (2013) could not determine whether commensal bacteria or bacteria under non-pathogenic conditions expressed CDCs in vivo. Although L. iners is not generally considered as a common commensal (Vaneechoutte, 2017b), it is still unclear whether INY is secreted in the healthy vagina (Macklaim et al., 2013) where L. iners is abundant. It is hypothesized that INY activity is utilized by L. iners to obtain necessary growth nutrients, outcompete other bacteria, and survive under various environmental conditions (France et al., 2016; Petrova et al., 2017; Vaneechoutte, 2017b).
VLY production has been detected in BV-positive women and those with healthy vaginal microbiota (Nowak et al., 2018). The median VLY concentration (1 ng/mL) in vaginal samples with predominant L. crispatus is approximately three-fold lower than that in Lactobacillus-deficient samples. Healthy microbiota characterized by low pH express minimal VLY activity in vitro (Rampersaud et al., 2011). VLY concentrations approximately six-fold higher than those detected in vivo caused membrane blebbing but not cell lysis in epithelial cell monolayers (Randis et al., 2013). The formation of membrane blebs induced by PFT-mediated membrane injury is a mechanism of cell protection that promotes the survival of affected cells (Babiychuk et al., 2010; Brito et al., 2019).
Garcia et al. (2019) found that VLY was not essential for intensive growth of the G. leopoldii strain AMD on the apical side of a three-dimensional vaginal epithelium model, as VLY does not permeabilize the apical side of vaginal tissue. LaRocca et al. (2014) reported that the hCD59-dependent cytolysins VLY and ILY induced programmed necrosis in mature human erythrocytes. Although erythrocytes are not the primary targets, they may come in contact with cytolysins during menses (Santiago Lopes dos Santos et al., 2012; Schwebke et al., 2014b) and in bacteremia (McCool and DeDonato, 2012; Tankovic et al., 2017). Bacterial growth in vitro (including Gardnerella spp.) was substantially enhanced by programmed necrosis of erythrocytes. Release of the cytosolic content of erythrocytes may provide required nutrients that lead to a burst in bacterial growth (LaRocca et al., 2014). During menses, the concentrations of Gardnerella spp. and L. iners increased in the majority of women with healthy vaginal microbiota (Santiago Lopes dos Santos et al., 2012). Menses is the most disturbing factor affecting the stability of vaginal microbial communities (Santiago Lopes dos Santos et al., 2011); therefore, cytolysin-mediated release of nutrients may allow bacteria to overcome this disturbance.
A case report described a Gardnerella spp. infection complicated with bacteremia and a toxic-type encephalopathy in a young woman (Tankovic et al., 2017). G. swidsinskii strain GV37 isolated from blood cultures produced elevated amounts of VLY in vitro compared to that of control strains. The authors hypothesized that high concentrations of VLY in vivo can break the blood-brain barrier and affect brain cells.
Treatment of cervical cells with cell-free supernatants of L. iners and G. vaginalis significantly increased permeability of the cervical epithelial barrier and induced the resulting immune response (Anton et al., 2018). VLY and INY are candidates among the secreted factors that may have induced these effects. However, the authors did not analyze the presence of these toxins in the culture supernatants. The use of Gardnerella spp. strains lacking the vly gene isolated by our group might help to clarify the effect of cytolysin on cell permeability (Janulaitiene et al., 2018).
The activity of PFTs is related to the host response that defends against bacterial infections (Huffman et al., 2004; Aguilar et al., 2009). Sublytic doses of PFTs result in sublethal numbers of pores, which are perceived by epithelial cells via osmotic stress-induced activation of p38 MAPK signaling (Ratner et al., 2006). The p38 MAPK activation is a conserved response to the disruption of cell membrane integrity caused by many PFTs (Los et al., 2013). Activation of p38 MAPK induces the expression of proinflammatory cytokines that modulate the immune response in vivo. Thus, sublytic doses of toxins due to low bacterial density during early infection may function as a signal to epithelial cells to initiate protective immune responses (Ratner et al., 2006). Sublytic doses of INY and VLY induced p38 MAPK activation through phosphorylation in epithelial cells (Gelber et al., 2008; Rampersaud et al., 2011). The mRNA for IL-8 was upregulated after treatment of HeLa cells with VLY (Gelber et al., 2008). Garcia et al. (2019) found that IL-1β was the only cytokine induced by VLY at the basolateral side of vaginal epithelium. Specific IgA antibodies against VLY were detected in 60% of women diagnosed as BV-positive (Cauci et al., 1996); this correlated with IL-8 levels and leukocyte counts in BV-positive and BV-negative women (Cauci et al., 2002).
There have been contradictory reports on cytokine profiles in cervicovaginal lavages of BV-positive women (Mitchell and Marrazzo, 2014). Some studies showed that BV-positive lavages did not have elevated levels of IL-6 and IL-8 and increased neutrophil counts (Cauci, 2004; Donders, 2007; Nowak et al., 2018). By contrast, BV-positive lavages had higher levels of IL-6, IL-8, other proinflammatory cytokines, and chemokines (Hedges et al., 2006; Mitchell and Marrazzo, 2014). The absence of leukocytes might be due to a lack of IL-8 induction in most women with BV, indicating that the host genotype conditions the immune response (Cauci et al., 2002; Mitchell and Marrazzo, 2014).
Several studies have tried to elucidate cytokine induction by individual bacterial species using monolayered or polarized human vaginal epithelial cells that were co-cultured with bacteria or exposed to cell-free supernatants. L. iners did not induce an increased production of IL-8 and IL-6 in vaginal and cervical cells (Doerflinger et al., 2014; Anton et al., 2018). However, elevated secretion of these cytokines was detected after exposure of ectocervical cells to G. vaginalis cell-free supernatants (Anton et al., 2018). Other studies confirmed that BV-associated bacteria induce cytokine upregulation and lead to the host response, whereas vaginal Lactobacillus spp. do not (Mitchell and Marrazzo, 2014). Secreted bacterial factors that modulate the immune response have not been completely determined; however, cytolysin remains as the prime suspect. The absence of inflammation in the lower genital tract is a typical clinical symptom of BV (Donders, 2007). However, BV is linked with clinical conditions that are characterized by inflammation in the upper genital tract, such as pelvic inflammatory disease and cervicitis (Mitchell and Marrazzo, 2014). Non-infectious, inflammation-related preterm delivery is associated with vaginal microbial communities that contain abundant Gardnerella spp. (DiGiulio et al., 2015).
Factors Affecting Cytolysin Expression
The expression of cytolysin genes is affected by environmental changes in oxygen concentration, the impact of other bacteria, biofilm, and planktonic growth phenotypes. Biofilm is a favorable mode of Gardnerella spp. growth that confers resistance to environmental factors and contributes to survival (Patterson et al., 2007; Verstraelen and Swidsinski, 2019). The oxygen gradient within a biofilm regulates the distribution and survival of bacterial species (Monds and Toole, 2009; Castro et al., 2019). Gardnerella spp. colonize the vagina of many women without inducing signs of BV (Ma et al., 2012; Schwebke et al., 2014a), suggesting that Gardnerella spp. may adopt the planktonic style of growth by modulating the gene expression profile (Swidsinski et al., 2014; Castro et al., 2019). Certain Gardnerella species or genomic subgroups may have a reduced capacity to adhere to epithelial cells and form a biofilm (Castro et al., 2015; Janulaitiene et al., 2018), probably due to differences in proteins that contribute to and participate in these activities (Harwich et al., 2010; Marín et al., 2018).
Comparative transcriptomic analysis of the G. leopoldii AMD strain and several other Gardnerella spp. strains demonstrated that the transcription level of the vly gene was significantly lower in biofilms than in planktonic cells (Castro et al., 2017). As expected, the transcription of genes responsible for biofilm cell metabolic activity in biofilm cells also was reduced. The authors suggested that VLY was likely not required for the persistence of mono-species biofilm, which represents a long-lasting mode of vaginal colonization. The interactions between Gardnerella spp. and other BV-associated bacteria were analyzed using dual-species biofilm assemblies (Castro et al., 2019). Expression of the vly gene was significantly upregulated in biofilms when Gardnerella was associated with Enterococcus faecalis or Actinomyces neuii. By contrast, vly expression was only slightly upregulated when Gardnerella was associated with the BV-related bacteria Prevotella bivia. Atopobium vaginae, which has most frequently been detected within the Gardnerella biofilm matrix, does not affect the vly transcription level compared to the mono-species biofilm composed solely of Gardnerella. Mobiluncus mulieris also did not affect the vly transcription level, whereas Brevibacterium ravenspurgense repressed vly expression. Castro et al. (2019) reported that urogenital bacterial species that were seldom detected in BV had a higher effect on the expression of genes related to virulence than commonly BV-associated A. vaginae and M. mulieris. One strain of Gardnerella spp. isolated from BV-positive women was used in a dual-species biofilm approach. This does not exclude that other species or genomic variants of Gardnerella may experience diverse effects. In summary, the vly transcript level is lower in Gardnerella spp. biofilm than in the planktonic mode of growth. However, certain bacterial species found in the unique dual-species biofilm morphotypes affect Gardnerella spp. cells and increase vly expression (Castro et al., 2019). The authors proposed that vaginal epithelial cell desquamation characteristic of BV was connected with the increased transcription level of vly and VLY-mediated cytotoxicity. By contrast, L. crispatus, which is distinctive to a healthy vagina, reduced the cytotoxicity of several Gardnerella spp. isolates toward HeLa vaginal epithelial cells by repressing vly expression (Castro et al., 2018).
Application of the meta-transcriptomic approach revealed that INY was upregulated six-fold and VLY was upregulated 256-fold in BV compared to a healthy state (Macklaim et al., 2013). Mitchell and Marrazzo (2014) reported that it was not clear whether these changes occurred due to strain differences or because the microbial environment reflected the gene expression levels. These studies require further investigation and analysis to detect differences in the expression profiles of cytolysins isolated from a large number of vaginal samples.
Discussion and Perspectives
CDCs have evolved a sophisticated mechanism to punch a hole in host membranes and invade the cells. Cytolysin-mediated disruption of membrane integrity of the cell barrier leads to various consequences to the host, from the direct cell death to impaired cellular functions. Research challenges still remain, such as determining the structure-function relationships in vivo, although many issues regarding the structure of CDCs and their pore-forming activity have been elucidated. Some CDC activities are accomplished by pore-independent pathways. All these activities promote growth and invasion of bacterial pathogens; therefore, CDCs are accepted as important virulence factors contributing to the pathology of infectious diseases. Bacterial species that are not regarded as traditional human pathogens also produce cytolysins, including Gardnerella spp. and L. iners. Studies to elucidate the role of Gardnerella as a pathogen have been performed for more than 60 years. In the recent past, this taxon was underrated, but has been recently revived by the definition of vaginal Gardnerella polymicrobial biofilm as a characteristic of BV, and identifying species and genomic groups with potentially diverse roles in health and disease. Future work will continue to delineate the importance of L. iners.
This review provides an overview of structural and activity characteristics of the cytolysins VLY and INY (Table 1). The environmental pH, accessibility of membrane cholesterol, and cytolysin concentration may affect the activity and function of these CDCs on eukaryotic cells. However, many issues remained unsolved. The membrane composition that is more prone to cytolysin binding remains to be determined, which may condition the specificity to the target during infection and in a commensal state. The impact of hCD59 on some characteristics of VLY activity needs to be fully elucidated because the majority of studies on synthetic membranes did not include this receptor. Knowledge of the genetic regulation of vly and iny expression is lacking. It is unclear whether non-hCD59-responsive INY is sensitive to other binding targets besides cholesterol. Gardnerella spp. and L. iners cytolysins are considered as virulence factors by analogy with other CDCs, although their particular roles in vivo are unknown. This report also provides insights into the predicted and hypothetical functions of VLY and INY in vivo (Table 1). Prospectively, the effects of cytolysins in vitro should be studied by the inclusion of different Gardnerella species and L. iners strains, and strains lacking vly and iny genes. Models that more closely resemble in vivo conditions are needed to unravel the relevance of cytolysins to the pathogenesis of BV. The polymicrobial nature of BV is technically challenging for studying the effects of cytolysins, as it is an experimentally evident influence of neighboring vaginal bacteria on the virulence genes. It is crucial to study the role of cytolysins in the context of host microbiota, its fluctuations, and disturbance that condition the virulence potential of bacterial species.
Author Contributions
The author confirms being the sole contributor of this work and has approved it for publication.
Conflict of Interest
The author declares that the research was conducted in the absence of any commercial or financial relationships that could be construed as a potential conflict of interest.
Acknowledgments
The author thanks Dr. L. Baranauskiene and Dr. A. Žvirbliene for their helpful advice.
References
Aguilar, J. L., Kulkarni, R., Randis, T. M., Soman, S., Kikuchi, A., Yin, Y., et al. (2009). Phosphatase-dependent regulation of epithelial mitogen-activated protein kinase responses to toxin-induced membrane pores. PLoS ONE 4:e8076. doi: 10.1371/journal.pone.0008076
Ahmed, A., Earl, J., Retchless, A., Hillier, S. L., Rabe, L. K., Cherpes, T. L., et al. (2012). Comparative genomic analyses of 17 clinical isolates of Gardnerella vaginalis provide evidence of multiple genetically isolated clades consistent with subspeciation into genovars. J. Bacteriol. 194, 3922–3937. doi: 10.1128/JB.00056-12
Aiyar, A. (2000). The use of CLUTAL W and CLUSTAL X for multiple sequence alignment. Methods Mol. Biol. 132, 221–241. doi: 10.1385/1-59259-192-2:221
Alouf, J. E. (2003). Molecular features of the cytolytic pore-forming bacterial protein toxins. Folia Microbiol. 48, 5–16. doi: 10.1007/BF02931271
Anahtar, M. N., Byrne, E. H., Doherty, K. E., Bowman, B. A., Yamamoto, S., Soumillon, M., et al. (2015). Cervicovaginal bacteria are a major modulator of host inflammatory responses in the female genital tract. Immunity 42, 965–976. doi: 10.1016/j.immuni.2015.04.019
Anton, L., Sierra, L., Devine, A., Barila, G., Heiser, L., Brown, A. G., et al. (2018). Common cervicovaginal microbial supernatants alter cervical epithelial function: mechanisms by which Lactobacillus crispatus contributes to cervical health. Front. Microbiol. 9:2181. doi: 10.3389/fmicb.2018.02181
Babiychuk, E. B., Monastyrskaya, K., Potez, S., and Draeger, A. (2010). Blebbing confers resistance against cell lysis. Cell Death Differ. 18, 80–89. doi: 10.1038/cdd.2010.81
Balashov, S. V., Mordechai, E., Adelson, M. E., and Gygax, S. E. (2014). Identification, quantification and subtyping of Gardnerella vaginalis in noncultured clinical vaginal samples by quantitative PCR. J. Med. Microbiol. 63, 162–175. doi: 10.1099/jmm.0.066407-0
Bavdek, A., Gekara, N. O., Priselac, D., Aguirre, I. G., Darji, A., Chakraborty, T., et al. (2007). Sterol and pH interdependence in the binding, oligomerization, and pore formation of listeriolysin O. Biochemistry 46, 4425–4437. doi: 10.1021/bi602497g
Bavdek, A., Kostanjšek, R., Antonini, V., Lakey, J. H., Dalla Serra, M., Gilbert, R. J., et al. (2012). pH dependence of listeriolysin O aggregation and pore-forming ability. FEBS J. 279, 126–141. doi: 10.1111/j.1742-4658.2011.08405.x
Bhattacharjee, P., and Keyel, P. A. (2018). Cholesterol-dependent cytolysins impair pro-inflammatory macrophage responses. Sci. Rep. 8:6458. doi: 10.1038/s41598-018-24955-2
Boyd, C. M., Parsons, E. S., Smith, R. A. G., Seddon, J. M., Ces, O., and Bubeck, D. (2016). Disentangling the roles of cholesterol and CD59 in intermedilysin pore formation. Sci. Rep. 6:38446. doi: 10.1038/srep38446
Brito, C., Cabanes, D., Sarmento Mesquita, F., and Sousa, S. (2019). Mechanisms protecting host cells against bacterial pore-forming toxins. Cell. Mol. Life Sci. 76, 1319–1339. doi: 10.1007/s00018-018-2992-8
Brown, L., Wolf, J. M., Prados-Rosales, R., and Casadevall, A. (2015). Through the wall: extracellular vesicles in gram-positive bacteria, mycobacteria and fungi. Nat. Rev. Microbiol. 13, 620–630. doi: 10.1038/nrmicro3480
Cassidy, S. K. B., and O'Riordan, M. X. D. (2013). More than a pore: The cellular response to cholesterol-dependent cytolysins. Toxins 5, 618–636. doi: 10.3390/toxins5040618
Castro, J., Alves, P., Sousa, C., Cereija, T., França, Â., Jefferson, K. K., et al. (2015). Using an in-vitro biofilm model to assess the virulence potential of bacterial vaginosis or non-bacterial vaginosis Gardnerella vaginalis isolates. Sci. Rep. 5:11640. doi: 10.1038/srep11640
Castro, J., França, A., Bradwell, K. R., Serrano, M. G., Jefferson, K. K., and Cerca, N. (2017). Comparative transcriptomic analysis of Gardnerella vaginalis biofilms vs. planktonic cultures using RNA-seq. NPJ Biofilms Microbiomes 3:3. doi: 10.1038/s41522-017-0012-7
Castro, J., Machado, D., and Cerca, N. (2019). Unveiling the role of Gardnerella vaginalis in polymicrobial bacterial vaginosis biofilms: the impact of other vaginal pathogens living as neighbors. ISME J. 13, 1306–1317. doi: 10.1038/s41396-018-0337-0
Castro, J., Martins, A. P., Rodrigues, M. E., and Cerca, N. (2018). Lactobacillus crispatus represses vaginolysin expression by BV associated Gardnerella vaginalis and reduces cell cytotoxicity. Anaerobe 50, 60–63. doi: 10.1016/j.anaerobe.2018.01.014
Cauci, S. (2004). Vaginal immunity in bacterial vaginosis. Curr. Infect. Dis. Rep. 6, 450–456. doi: 10.1007/s11908-004-0064-8
Cauci, S., Guaschino, S., Driussi, S., De Santo, D., Lanzafame, P., and Quadrifoglio, F. (2002). Correlation of local interleukin-8 with immunoglobulin A against Gardnerella vaginalis hemolysin and with prolidase and sialidase levels in women with bacterial vaginosis. J. Infect. Dis. 185, 1614–1620. doi: 10.1086/340417
Cauci, S., Monte, R., Ropele, M., Missero, C., Not, T., Quadrifoglio, F., et al. (1993). Pore-forming and haemolytic properties of the Gardnerella vaginalis cytolysin. Mol. Microbiol. 9, 1143–1155. doi: 10.1111/j.1365-2958.1993.tb01244.x
Cauci, S., Scrimin, F., Driussi, S., and Ceccone, S. (1996). Specific immune response against Gardnerella vaginalis hemolysin in patients with bacterial vaginosis. Am. J. Obs. Gynecol. 175, 1601–1605. doi: 10.1016/S0002-9378(96)70112-6
Cerca, N., Vaneechoutte, M., Guschin, A., and Swidsinski, A. (2017). Polymicrobial infections and biofilms in women's health Gahro Expert Group Meeting Report. Res. Microbiol. 168, 902–904. doi: 10.1016/j.resmic.2017.07.002
Chakrabarti, R. S., Ingham, S. A., Kozlitina, J., Gay, A., Cohen, J. C., Radhakrishnan, A., et al. (2017). Variability of cholesterol accessibility in human red blood cells measured using a bacterial cholesterol-binding toxin. Elife 6:e23355. doi: 10.7554/eLife.23355.022
Christie, M. P., Johnstone, B. A., Tweten, R. K., Parker, M. W., and Morton, C. J. (2018). Cholesterol-dependent cytolysins: from water-soluble state to membrane pore. Biophys. Rev. 10, 1337–1348. doi: 10.1007/s12551-018-0448-x
Czajkowsky, D. M., Hotze, E. M., Shao, Z., and Tweten, R. K. (2004). Vertical collapse of a cytolysin prepore moves its transmembrane β-hairpins to the membrane. EMBO J. 23, 3206–3215. doi: 10.1038/sj.emboj.7600350
Dal Peraro, M. D., and van der Goot, F. G. (2016). Pore-forming toxins: Ancient, but never really out of fashion. Nat. Rev. Microbiol. 14, 77–92. doi: 10.1038/nrmicro.2015.3
DiGiulio, D. B., Callahan, B. J., McMurdie, P. J., Costello, E. K., and Lyell, D. J. (2015). Temporal and spatial variation of the human microbiota during pregnancy. Proc. Natl. Acad. Sci. U.S.A. 112, 2–7. doi: 10.1073/pnas.1502875112
Doerflinger, S. Y., Throop, A. L., and Herbst-Kralovetz, M. M. (2014). Bacteria in the vaginal microbiome alter the innate immune response and barrier properties of the human vaginal epithelia in a species-specific manner. J. Infect. Dis. 209, 1989–1999. doi: 10.1093/infdis/jiu004
Domon, H., Oda, M., Maekawa, T., Nagai, K., Takeda, W., and Terao, Y. (2016). Streptococcus pneumoniae disrupts pulmonary immune defence via elastase release following pneumolysin-dependent neutrophil lysis. Sci. Rep. 6:38013. doi: 10.1038/srep38013
Donders, G. G. G. (2007). Definition and classification of abnormal vaginal flora. Best Pract. Res. Clin. Obstet. Gynaecol. 21, 355–373. doi: 10.1016/j.bpobgyn.2007.01.002
Donders, G. G. G., Bellen, G., Grinceviciene, S., Ruban, K., and Vieira-Baptista, P. (2017). Aerobic vaginitis : no longer a stranger. Res. Microbiol. 168, 845–858. doi: 10.1016/j.resmic.2017.04.004
Dowd, K. J., Farrand, A. J., and Tweten, R. K. (2012). The cholesterol-dependent cytolysin signature motif: A critical element in the allosteric pathway that couples membrane binding to pore assembly. PLoS Pathog. 8:e1002787. doi: 10.1371/journal.ppat.1002787
Farrand, A. J., Hotze, E. M., Sato, T. K., Wade, K. R., Wimley, W. C., Johnson, A. E., et al. (2015). The cholesterol-dependent cytolysin membrane-binding interface discriminates lipid environments of cholesterol to support β-barrel pore insertion. J. Biol. Chem. 290, 17733–17744. doi: 10.1074/jbc.M115.656769
Farrand, A. J., LaChapelle, S., Hotze, E. M., Johnson, A. E., and Tweten, R. K. (2010). Only two amino acids are essential for cytolytic toxin recognition of cholesterol at the membrane surface. Proc. Natl. Acad. Sci. U.S.A. 107, 4341–4346. doi: 10.1073/pnas.0911581107
Fethers, K. A., Fairley, C. K., Hocking, J. S., Gurrin, L. C., and Bradshaw, C. S. (2008). Sexual risk factors and bacterial vaginosis: a systematic review and meta-analysis. Clin. Infect. Dis. 47, 1426–1435. doi: 10.1086/592974
Fettweis, J. M., Brooks, J. P., Serrano, M. G., Sheth, N. U., Girerd, P. H., and Edwards, D. J. (2014). Differencies in vaginal microbiome in African American women versus women of European ancestry. Microbiology 160, 2272–2282. doi: 10.1099/mic.0.081034-0
Flanagan, J. J., Tweten, R. K., Johnson, A. E., and Heuck, A. P. (2009). Cholesterol exposure at the membrane surface is necessary and sufficient to trigger perfringolysin O binding. Biochemistry 48, 3977–3987. doi: 10.1021/bi9002309
France, M. T., Mendes-Soares, H., and Forney, L. J. (2016). Genomic comparisons of Lactobacillus crispatus and Lactobacillus iners reveal potential ecological drivers of community composition in the vagina. Appl. Env. Microbiol. 82, 7063–7073. doi: 10.1128/AEM.02385-16
Fredricks, D. N., Fiedler, T. L., Thomas, K. K., Oakley, B. B., and Marrazzo, J. M. (2007). Targeted PCR for detection of vaginal bacteria associated with bacterial vaginosis. J. Clin. Microbiol. 45, 3270–3276. doi: 10.1128/JCM.01272-07
Garcia, E. M., Kraskauskiene, V., Koblinski, J. E., and Jefferson, K. K. (2019). Interaction of Gardnerella vaginalis and vaginolysin with the apical versus basolateral face of a three-dimensional model of vaginal epithelium. Infect. Immun. 87:e00646-18. doi: 10.1128/IAI.00646-18
Gelber, S. E., Aguilar, J. L., Lewis, K. L. T., and Ratner, A. J. (2008). Functional and phylogenetic characterization of vaginolysin, the human-specific cytolysin from Gardnerella vaginalis. J. Bacteriol. 190, 3896–3903. doi: 10.1128/JB.01965-07
Giddings, K. S., Zhao, J., Sims, P. J., and Tweten, R. K. (2004). Human CD59 is a receptor for the cholesterol-dependent cytolysin intermedilysin. Nat. Struct. Mol. Biol. 11, 1173–1178. doi: 10.1038/nsmb862
Gilbert, N. M., Lewis, W. G., Li, G., Sojka, D. K., Lubin, J. B., and Lewis, A. L. (2019). Gardnerella vaginalis and Prevotella bivia trigger distinct and overlapping phenotypes in a mouse model of bacterial vaginosis. J. Infect. Dis. 220, 1099–1108. doi: 10.1093/infdis/jiy704
Gilbert, R. J. C., Rossjohn, J., Parker, M. W., Tweten, R. K., Morgan, P. J., Mitchell, T. J., et al. (1998). Self-interaction of pneumolysin, the pore-forming protein toxin of Streptococcus pneumoniae. J. Mol. Biol. 284, 1223–1237. doi: 10.1006/jmbi.1998.2258
Goldmann, O., Sastalla, I., Wos-Oxley, M., Rohde, M., and Medina, E. (2009). Streptococcus pyogenes induces oncosis in macrophages through the activation of an inflammatory programmed cell death pathway. Cell. Microbiol. 11, 138–155. doi: 10.1111/j.1462-5822.2008.01245.x
Hamon, M. A., Ribet, D., Stavru, F., and Cossart, P. (2012). Listeriolysin O: The Swiss army knife of Listeria. Trends Microbiol. 20, 360–368. doi: 10.1016/j.tim.2012.04.006
Harwich, M. D., Alves, J. M., Buck, G. A., Strauss, J. F. 3rd, Patterson, J. L., Oki, A. T., et al. (2010). Drawing the line between commensal and pathogenic Gardnerella vaginalis through genome analysis and virulence studies. BMC Genomics, 11:375. doi: 10.1186/1471-2164-11-375
Hedges, S. R., Barrientes, F., Desmond, R. A., and Schwebke, J. R. (2006). Local and systemic cytokine levels in relation to changes in vaginal flora. J. Infect Dis. 193, 552–562.9999 doi: 10.1086/499824
Hickey, R. J., and Forney, L. J. (2014). Gardnerella vaginalis does not always cause bacterial vaginosis. J. Infect. Dis. 210, 1682–1683. doi: 10.1093/infdis/jiu303
Hotze, E. M., Heuck, A. P., Czajkowsky, D. M., Shao, Z., Johnson, A. E., and Tweten, R. K. (2002). Monomer-monomer interactions drive the prepore to pore conversion of a β-barrel-forming cholesterol-dependent cytolysin. J. Biol. Chem. 277, 11597–11605. doi: 10.1074/jbc.M111039200
Hotze, E. M., Le, H. M., Sieber, J. R., Bruxvoort, C., McInerney, M. J., and Tweten, R. K. (2013). Identification and characterization of the first cholesterol-dependent cytolysins from gram-negative bacteria. Infect. Immun. 81, 216–225. doi: 10.1128/IAI.00927-12
Hotze, E. M., and Tweten, R. K. (2012). Membrane assembly of the cholesterol-dependent cytolysin pore complex. Biochim. Biophys. Acta-Biomembr. 1818, 1028–1038. doi: 10.1016/j.bbamem.2011.07.036
Huang, B., Fettweis, J. M., Brooks, J. P., Jefferson, K. K., and Buck, G. A. (2014). The changing landscape of the vaginal microbiome. Clin. Lab. Med. 34, 747–761. doi: 10.1016/j.cll.2014.08.006
Huang, Y., Fedarovich, A., Tomlinson, S., and Davies, C. (2007). Crystal structure of CD59: Implications for molecular recognition of the complement proteins C8 and C9 in the membrane-attack complex. Acta Crystallogr. Sect. D Biol. Crystallogr. 63, 714–721. doi: 10.1107/S0907444907015557
Huffman, D. L., Abrami, L., Sasik, R., Corbeil, J., van der Goot, F. G., and Aroian, R. V. (2004). Mitogen-activated protein kinase pathways defend against bacterial pore-forming toxins. Proc. Natl. Acad. Sci. U.S.A. 101, 10995–11000. doi: 10.1073/pnas.0404073101
Hupp, S., Heimeroth, V., Wippel, C., Förtsch, C., Ma, J., Mitchell, T. J., et al. (2012). Astrocytic tissue remodeling by the meningitis neurotoxin pneumolysin facilitates pathogen tissue penetration and produces interstitial brain edema. Glia 60, 137–146. doi: 10.1002/glia.21256
Iacovache, I., and van der Goot, F. G. (2004). A bacterial big-MAC attack. Nat. Struct. Mol. Biol. 11, 1163–1164. doi: 10.1038/nsmb1204-1163
Janulaitiene, M., Gegzna, V., Baranauskiene, L., Bulavaite, A., Simanavicius, M., and Pleckaityte, M. (2018). Phenotypic characterization of Gardnerella vaginalis subgroups suggests differences in their virulence potential. PLoS One 13:e020062. doi: 10.1371/journal.pone.0200625
Janulaitiene, M., Paliulyte, V., Grinceviciene, S., Zakareviciene, J., Vladisauskiene, A., Marcinkute, A., et al. (2017). Prevalence and distribution of Gardnerella vaginalis subgroups in women with and without bacterial vaginosis. BMC Infect. Dis. 17:394. doi: 10.1186/s12879-017-2501-y
Johnson, B. B., Breña, M., Anguita, J., and Heuck, A. (2017). Mechanistic insights into the cholesterol-dependent binding of perfringolysin O-based probes and cell membranes. Sci. Rep. 7:13793. doi: 10.1038/s41598-017-14002-x
Johnson, B. B., Moe, P. C., Wang, D., Rossi, K., Trigatti, B. L., and Heuck, A. P. (2012). Modifications in perfringolysin O domain 4 alter the cholesterol concentration threshold required for binding. Biochemistry 51, 3373–3382. doi: 10.1021/bi3003132
Kenyon, C., Colebunders, R., and Crucitti, T. (2013). The global epidemiology of bacterial vaginosis: a systematic review. Am. J. Obstet. Gynecol. 209, 505–523. doi: 10.1016/j.ajog.2013.05.006
LaChapelle, S., Tweten, R. K., and Hotze, E. M. (2009). Intermedilysin-receptor interactions during assembly of the pore complex. Assembly intermediates increase host cell susceptibility to complement-mediated lysis. J. Biol. Chem. 284, 12719–12726. doi: 10.1074/jbc.M900772200
LaRocca, T. J., Stivison, E. A., Hod, E. A., Spitalnik, S. L., Cowan, P. J., Randis, T. M., et al. (2014). Human-specific bacterial pore-forming toxins induce programmed necrosis in erythrocytes. MBio 5:e01251-14. doi: 10.1128/mBio.01251-14
Lawrence, S. L., Feil, S. C., Morton, C. J., Farrand, A. J., Mulhern, T. D., Gorman, M. A., et al. (2015). Crystal structure of Streptococcus pneumoniae pneumolysin provides key insights into early steps of pore formation. Sci. Rep. 5:14352. doi: 10.1038/srep14352
Lawrence, S. L., Gorman, M. A., Feil, S. C., Mulhern, T. D., Kuiper, M. J., Ratner, A. J., et al. (2016). Structural basis for receptor recognition by the human CD59-responsive cholesterol-dependent cytolysins. Structure 24, 1488–1498. doi: 10.1016/j.str.2016.06.017
Leung, C., Dudkina, N. V., Lukoyanova, N., Hodel, A. W., Farabella, I., Pandurangan, A. P., et al. (2014). Stepwise visualization of membrane pore formation by suilysin, a bacterial cholesterol-dependent cytolysin. Elife 3:e04247. doi: 10.7554/eLife.04247
Liu, Y., Defourny, K. A. Y., Smid, E. J., and Abee, T. (2018). Gram-positive bacterial extracellular vesicles and their impact on health and disease. Front. Microbiol. 9:1502. doi: 10.3389/fmicb.2018.01502
Los, F. C. O., Randis, T. M., Aroian, R. V., and Ratner, A. J. (2013). Role of pore-forming toxins in bacterial infectious diseases. Microbiol. Mol. Biol. Rev. 77, 173–207. doi: 10.1128/MMBR.00052-12
Ma, B., Forney, L. J., and Ravel, J. (2012). Vaginal microbiome: rethinking health and disease. Annu. Rev. Microbiol. 66, 371–389. doi: 10.1146/annurev-micro-092611-150157
Macklaim, J. M., Fernandes, A. D., Di Bella, J. M., Hammond, J. A., Reid, G., and Gloor, G. B. (2013). Comparative meta-RNA-seq of the vaginal microbiota and differential expression by Lactobacillus iners in health and dysbiosis. Microbiome 1:12. doi: 10.1186/2049-2618-1-12
Macklaim, J. M., Gloor, G. B., Anukam, K. C., Cribby, S., and Reid, G. (2011). At the crossroads of vaginal health and disease, the genome sequence of Lactobacillus iners AB-1. Proc. Natl. Acad. Sci. U.S.A. 108, 4688–4695. doi: 10.1073/pnas.1000086107
Marín, E., Haesaert, A., Padilla, L., Adán, J., and Hernáez, M. L. (2018). Unraveling Gardnerella vaginalis surface proteins using cell shaving proteomics. Front. Microbiol. 9:975. doi: 10.3389/fmicb.2018.00975
Marriott, H. M., Mitchell, T. J., and Dockrell, D. H. (2008). Pneumolysin: A double-edged sword during the host-pathogen interaction. Curr. Mol. Med. 8, 497–509. doi: 10.2174/156652408785747924
McCool, R. A., and DeDonato, D. M. (2012). Bacteremia of Gardnerella vaginalis after endometrial ablation. Arch. Gynecol. Obs. 286, 1337–1338. doi: 10.1007/s00404-012-2447-7
Meehl, M. A., and Caparon, M. G. (2004). Specificity of streptolysin O in cytolysin-mediated translocation. Mol. Microbiol. 52, 1665–1676. doi: 10.1111/j.1365-2958.2004.04082.x
Mitchell, C., and Marrazzo, J. (2014). Bacterial vaginosis and the cervicovaginal immune response. Am. J. Reprod. Immunol. 71, 555–563. doi: 10.1111/aji.12264
Monds, R. D., and Toole, G. A. O. (2009). The developmental model of microbial biofilms: ten years of a paradigm up for review. Trends Microbiol. 17, 73–87. doi: 10.1016/j.tim.2008.11.001
Morton, C. J., Sani, M. A., Parker, M. V., and Separovic, F. (2019). Cholesterol-dependent cytolysins: Membrane and protein structural requirements for pore formation. Chem. Rev. 119, 7771–7736. doi: 10.1021/acs.chemrev.9b00090
Mulvihill, E., van Pee, K., Mari, S. A., Müller, D. J., and Yildiz, Ö. (2015). Directly observing the lipid-dependent self-assembly and pore-forming mechanism of the cytolytic toxin listeriolysin O. Nano Lett. 15, 6965–6973. doi: 10.1021/acs.nanolett.5b02963
Muzny, C. A., Blanchard, E., Taylor, C. M., Aaron, K. J., Talluri, R., Griswold, M. E., et al. (2018). Identification of key bacteria involved in the induction of incident bacterial vaginosis: a prospective study. J. Infect. Dis. 218, 966–978. doi: 10.1093/infdis/jiy243
Muzny, C. A., and Schwebke, J. R. (2013). Gardnerella vaginalis: still a prime suspect in the pathogenesis of bacterial vaginosis. Curr. Infect. Dis. Rep. 15, 130–135. doi: 10.1007/s11908-013-0318-4
Muzny, C. A., Taylor, C. M., Swords, W. E., Tamhane, A., Chattopadhyay, D., Cerca, N., et al. (2019). An updated conceptual model on the pathogenesis of bacterial vaginosis. J. Infect. Dis. 220, 1399–1405. doi: 10.1093/infdis/jiz342
Nelson, L. D., Johnson, A. E., and London, E. (2008). How interaction of perfringolysin O with membranes is controlled by sterol structure, lipid structure, and physiological low pH: Insights into the origin of perfringolysin O-lipid raft interaction. J. Biol. Chem. 283, 4632–4642. doi: 10.1074/jbc.M709483200
Nowak, R. G., Randis, T. M., Desai, P., He, X., Robinson, C. K., Rath, J. M., et al. (2018). Higher levels of a cytotoxic protein, vaginolysin, in Lactobacillus-deficient community state types at the vaginal mucosa. Sex. Transm. Dis. 45, e14–e17. doi: 10.1097/OLQ.0000000000000774
Nugent, R. P., Krohn, M. A., and Hillier, S. L. (1991). Reliability of diagnosing bacterial vaginosis is improved by a standardized method of gram stain interpretation. J. Clin. Microbiol. 29, 297–301.
Onderdonk, A. B., Delaney, M. L., and Fichorova, R. N. (2016). The human microbiome during bacterial vaginosis. Clin. Microbiol. Rev. 29, 223–238. doi: 10.1128/CMR.00075-15
Osborne, S. E., and Brumell, J. H. (2017). Listeriolysin O: from bazooka to Swiss army knife. Philos. Trans. R. Soc. Lond. B Biol. Sci. 372. doi: 10.1098/rstb.2016.0222
Park, S. A., Park, Y. S., Bong, S. M., and Lee, K. S. (2016). Structure-based functional studies for the cellular recognition and cytolytic mechanism of pneumolysin from Streptococcus pneumoniae. J. Struct. Biol. 193, 132–140. doi: 10.1016/j.jsb.2015.12.002
Patterson, J. L., Girerd, P. H., Karjane, N. W., and Jefferson, K. K. (2007). Effect of biofilm phenotype on resistance of Gardnerella vaginalis to hydrogen peroxide and lactic acid. Am. J. Obstet. Gynecol. 192, 170.e1–e7. doi: 10.1016/j.ajog.2007.02.027
Patterson, J. L., Stull-Lane, A., Girerd, P. H., and Jefferson, K. K. (2010). Analysis of adherence, biofilm formation and cytotoxicity suggests a greater virulence potential of Gardnerella vaginalis relative to other bacterial-vaginosis-associated anaerobes. Microbiology 156, 392–399. doi: 10.1099/mic.0.034280-0
Petrova, M. I., Lievens, E., Malik, S., and Imholz, N. (2015). Lactobacillus species as biomarkers and agents that can promote various aspects of vaginal health. Front. Physiol. 6:81. doi: 10.3389/fphys.2015.00081
Petrova, M. I., Reid, G., Vaneechoutte, M., and Lebeer, S. (2017). Lactobacillus iners: friend or foe? Trends Microbiol. 25, 182–191. doi: 10.1016/j.tim.2016.11.007
Pleckaityte, M., Janulaitiene, M., Lasickiene, R., and Zvirbliene, A. (2012). Genetic and biochemical diversity of Gardnerella vaginalis strains isolated from women with bacterial vaginosis. FEMS Immunol. Med. Microbiol. 65, 69–77. doi: 10.1111/j.1574-695X.2012.00940.x
Pleckaityte, M., Mistiniene, E., Lasickiene, R., Zvirblis, G., and Zvirbliene, A. (2011). Generation of recombinant single-chain antibodies neutralizing the cytolytic activity of vaginolysin, the main virulence factor of Gardnerella vaginalis. BMC Biotechnol. 11:100. doi: 10.1186/1472-6750-11-100
Podobnik, M., Marchioretto, M., Zanetti, M., Bavdek, A., Kisovec, M., Cajnko, M. M., et al. (2015). Plasticity of listeriolysin O pores and its regulation by pH and unique histidine. Sci. Rep. 5:9623. doi: 10.1038/srep09623
Polekhina, G., Giddings, K. S., Tweten, R. K., and Parker, M. W. (2004). Crystallization and preliminary X-ray analysis of the human-specific toxin intermedilysin. Acta Crystallogr. Sect. D Biol. Crystallogr. 60, 347–349. doi: 10.1107/S0907444903027240
Ragaliauskas, T., Plečkaityte, M., Jankunec, M., Labanauskas, L., Baranauskiene, L., and Valincius, G. (2019). Inerolysin and vaginolysin, the cytolysins implicated in vaginal dysbiosis, differently impair molecular integrity of phospholipid membranes. Sci. Rep. 9:10606. doi: 10.1038/s41598-019-47043-5
Ramachandran, R., Heuck, A. P., Tweten, R. K., and Johnson, A. E. (2002). Structural insights into the membrane-anchoring mechanism of a cholesterol-dependent cytolysin. Nat. Struct. Biol. 9, 823–827. doi: 10.1038/nsb855
Ramachandran, R., Tweten, R. K., and Johnson, A. E. (2005). The domains of a cholesterol-dependent cytolysin undergo a major FRET-detected rearrangement during pore formation. Proc. Natl. Acad. Sci. U. S. A. 102, 7139–7144. doi: 10.1073/pnas.0500556102
Rampersaud, R., Lewis, E. L., LaRocca, T. J., and Ratner, A. J. (2018). Environmental pH modulates inerolysin activity via post-binding blockade. Sci. Rep. 8:1542. doi: 10.1038/s41598-018-19994-8
Rampersaud, R., Planet, P. J., Randis, T. M., Kulkarni, R., Aguilar, J. L., Lehrer, R. I., et al. (2011). Inerolysin, a cholesterol-dependent cytolysin produced by Lactobacillus iners. J. Bacteriol. 193, 1034–1041. doi: 10.1128/JB.00694-10
Randis, T. M., Kulkarni, R., Aguilar, J. L., and Ratner, A. J. (2009). Antibody-based detection and inhibition of vaginolysin, the Gardnerella vaginalis cytolysin. PLoS ONE 4:e5207. doi: 10.1371/journal.pone.0005207
Randis, T. M., Zaklama, J., LaRocca, T. J., Los, F. C. O., Lewis, E. L., Desai, P., et al. (2013). Vaginolysin drives epithelial ultrastructural responses to Gardnerella vaginalis. Infect. Immun. 81, 4544–4550. doi: 10.1128/IAI.00627-13
Ratner, A. J., Hippe, K. R., Aguilar, J. L., Bender, M. H., Nelson, A. L., and Weiser, J. N. (2006). Epithelial cells are sensitive detectors of bacterial pore-forming toxins. J. Biol. Chem. 281, 12994–12998. doi: 10.1074/jbc.M511431200
Ravel, J., Gajer, P., Abdo, Z., Schneider, G. M., Koenig, S. S. K., Mcculle, S. L., et al. (2011). Vaginal microbiome of reproductive-age women. Proc. Natl. Acad. Sci. U. S. A. 108, 4680–4687. doi: 10.1073/pnas.1002611107
Ray, S., Thapa, R., and Keyel, P. A. (2019). Multiple parameters beyond lipid binding affinity drive cytotoxicity of cholesterol-dependent cytolysins. Toxins 11, 1–19. doi: 10.3390/toxins11010001
Robinson, L. S., Schwebke, J., Lewis, W. G., and Lewis, A. L. (2019). Identification and characterization of NanH2 and NanH3, enzymes responsible for sialidase activity in the vaginal bacterium Gardnerella vaginalis. J. Biol. Chem. 294, 5230–5245. doi: 10.1074/jbc.RA118.006221
Rojko, N., and Anderluh, G. (2015). How lipid membranes affect pore forming toxin activity. Acc. Chem. Res. 48, 3073–3079. doi: 10.1021/acs.accounts.5b00403
Rossjohn, J., Feil, S. C., McKinstry, W. J., Tweten, R. K., and Parker, M. W. (1997). Structure of a cholesterol-binding, thiol-activated cytolysin and a model of its membrane form. Cell 89, 685–692. doi: 10.1016/S0092-8674(00)80251-2
Rottini, G., Dobrina, A., Forgiarini, O., Nardon, E., Amirante, G. A., and Patriarca, P. (1990). Identification and partial characterization of a cytolytic toxin produced by Gardnerella vaginalis. Infect. Immun. 58, 3751–3758.
Santiago Lopes dos Santos, G., Cools, P., Verstraelen, H., Trog, M., Missine, G., El Aila, N., et al. (2011). Longitudinal study of the dynamics of vaginal microflora during two consecutive menstrual cycles. PLoS ONE 6:e28180. doi: 10.1371/journal.pone.0028180
Santiago Lopes dos Santos, G., Tency, I., Verstraelen, H., Verhelst, R., Trog, M., Temmerman, M., et al. (2012). Longitudinal qPCR study of the dynamics of L. crispatus, L. iners, A. vaginae, (sialidase positive) G. vaginalis, and P. bivia in the vagina. PLoS ONE 7:e45281. doi: 10.1371/journal.pone.0045281
Schellenberg, J. J., Jayaprakash, T. P., Gamage, N. W., Patterson, M. H., Vaneechoutte, M., and Hill, J. E. (2016). Gardnerella vaginalis subgroups defined by cpn60 sequencing and sialidase activity in isolates from Canada, Belgium and Kenya. PLoS ONE 11:e0146510. doi: 10.1371/journal.pone.0146510
Schuerch, D. W., Wilson-Kubalek, E. M., and Tweten, R. K. (2005). Molecular basis of listeriolysin O pH dependence. Proc. Natl. Acad. Sci. U.S.A. 102, 12537–12542. doi: 10.1073/pnas.0500558102
Schwebke, J. R., Flynn, M. S., and Rivers, C. A. (2014a). Prevalence of Gardnerella vaginalis among women with lactobacillus-predominant vaginal flora. Sex. Transm. Infect. 90, 61–63. doi: 10.1136/sextrans-2013-051232
Schwebke, J. R., Muzny, C. A., and Josey, W. E. (2014b). Role of Gardnerella vaginalis in the pathogenesis of bacterial vaginosis: a conceptual model. J. Infect. Dis. 210, 338–343. doi: 10.1093/infdis/jiu089
Shaughnessy, L. M., Hoppe, A. D., Christensen, K. A., and Swanson, J. A. (2006). Membrane perforations inhibit lysosome fusion by altering pH and calcium in Listeria monocytogenes vacuoles. Cell. Microbiol. 8, 781–792. doi: 10.1111/j.1462-5822.2005.00665.x
Shipitsyna, E., Krysanova, A., Khayrullina, G., Shalepo, K., Savicheva, A., Guschin, A., et al. (2019). Quantitation of all four Gardnerella vaginalis clades detects abnormal vaginal microbiota characteristic of bacterial vaginosis more accurately than putative G. vaginalis sialidase A gene count. Mol. Diagnosis Ther. 23, 139–147. doi: 10.1007/s40291-019-00382-5
Shishpal, P., Kasarpalkar, N., Singh, D., and Bhor, V. M. (2019). Characterization of Gardnerella vaginalis membrane vesicles reveals a role in inducing cytotoxicity in vaginal epithelial cells. Anaerobe 20:102090. doi: 10.1016/j.anaerobe.2019.102090
Smith, S. B., and Ravel, J. (2017). The vaginal microbiota, host defence and reproductive physiology. J. Physiol. 595, 451–463. doi: 10.1113/JP271694
Sonnen, A. F. P., Plitzko, J. M., and Gilbert, R. J. C. (2014). Incomplete pneumolysin oligomers form membrane pores. Open Biol. 4:140044. doi: 10.1098/rsob.140044
Spreer, A., Kerstan, H., Böttcher, T., Gerber, J., Siemer, A., Zysk, G., et al. (2003). Reduced release of pneumolysin by Streptococcus pneumoniae in vitro and in vivo after treatment with nonbacteriolytic antibiotics in comparison to ceftriaxone. Antimicrob. Agents Chemother. 47, 2649–2654. doi: 10.1128/AAC.47.8.2649-2654.2003
Srinivasan, S., and Fredricks, D. N. (2008). The human vaginal bacterial biota and bacterial vaginosis. Interdiscip. Perspect. Infect. Dis. 2008:750479. doi: 10.1155/2008/750479
Srinivasan, S., Hoffman, N. G., Morgan, M. T., Matsen, F. A., Fiedler, T. L., Hall, R. W., et al. (2012). Bacterial communities in women with bacterial vaginosis: High resolution phylogenetic analyses reveal relationships of microbiota to clinical criteria. PLoS ONE 7:e37818. doi: 10.1371/journal.pone.0037818
Swidsinski, A., Loening-Baucke, V., Mendling, W., Dörffel, Y., Schilling, J., Halwani, Z., et al. (2014). Infection through structured polymicrobial Gardnerella biofilms (StPM-GB). Histol. Histophatol. 29, 567–587. doi: 10.14670/HH-29.10.567
Swidsinski, A., Mendling, W., Loening-Baucke, V., Ladhoff, A., Swidsinski, S., Hale, L. P., et al. (2005). Adherent biofilms in bacterial vaginosis. Obstet. Gynecol. 106, 1013–1023. doi: 10.1097/01.AOG.0000183594.45524.d2
Tankovic, J., Timinskas, A., Janulaitiene, M., Zilnyte, M., Baudel, J. L., Maury, E., et al. (2017). Gardnerella vaginalis bacteremia associated with severe acute encephalopathy in a young female patient. Anaerobe 47, 132–134. doi: 10.1016/j.anaerobe.2017.05.010
Tweten, R. K. (2005). Cholesterol-dependent cytolysins, a family of versatile pore-forming toxins. Infect. Immun. 73, 6199–6209. doi: 10.1128/IAI.73.10.6199-6209.2005
van de Wijgert, J. H. H. M., and Jespers, V. (2017). The global health impact of vaginal dysbiosis. Res. Microbiol. 168, 859–864. doi: 10.1016/j.resmic.2017.02.003
van Pee, K., Neuhaus, A., D'Imprima, E., Mills, D. J., Kühlbrandt, W., and Yildiz, Ö. (2017). CryoEM structures of membrane pore and prepore complex reveal cytolytic mechanism of pneumolysin. Elife 6:e23644. doi: 10.7554/eLife.23644
Vaneechoutte, M. (2017a). The human vaginal microbial community. Res. Microbiol. 168, 811–825. doi: 10.1016/j.resmic.2017.08.001
Vaneechoutte, M. (2017b). Lactobacillus iners, the unusual suspect. Res. Microbiol. 168, 826–836. doi: 10.1016/j.resmic.2017.09.003
Vaneechoutte, M., Guschin, A., Van Simaey, L., Gansemans, Y., Van Nieuwerburgh, F., and Cools, P. (2019). Emended description of Gardnerella vaginalis and description of Gardnerella leopoldii sp. nov., Gardnerella piotii sp. nov. and Gardnerella swidsinskiisp. nov., with delineation of 13 genomic species within the genus Gardnerella. Int. J. Syst. Evol. Microbiol. 69, 679–687. doi: 10.1099/ijsem.0.003200
Verstraelen, H., and Swidsinski, A. (2019). The biofilm in bacterial vaginosis: Implications for epidemiology, diagnosis and treatment: 2018 update. Curr. Opin. Infect. Dis. 32, 38–42. doi: 10.1097/QCO.0000000000000516
Vögele, M., Bhaskara, R. M., Mulvihill, E., van Pee, K., Yildiz, Ö., Kühlbrandt, W., et al. (2019). Membrane perforation by the pore-forming toxin pneumolysin. Proc. Natl. Acad. Sci. U.S.A. 116, 13352–13357. doi: 10.1073/pnas.1904304116
Waheed, A. A., Shimada, Y., Heijnen, H. F. G., Nakamura, M., Inomata, M., Hayashi, M., et al. (2001). Selective binding of perfringolysin O derivative to cholesterol-rich membrane microdomains (rafts). Proc. Natl. Acad. Sci. U.S.A. 98, 4926–4931. doi: 10.1073/pnas.091090798
Wall, E. C., Gordon, S. B., Hussain, S., Goonetilleke, U. R. S., Gritzfeld, J., Scarborough, M., et al. (2012). Persistence of pneumolysin in the cerebrospinal fluid of patients with pneumococcal meningitis is associated with mortality. Clin. Infect. Dis. 54, 701–705. doi: 10.1093/cid/cir926
Wickham, S. E., Hotze, E. M., Farrand, A. J., Polekhina, G., Nero, T. L., Tomlinson, S., et al. (2011). Mapping the intermedilysin-human CD59 receptor interface reveals a deep correspondence with the binding site on CD59 for complement binding proteins C8α and C9. J. Biol. Chem. 286, 20952–20962. doi: 10.1074/jbc.M111.237446
Wippel, C., Förtsch, C., Hupp, S., Maier, E., Benz, R., Ma, J., et al. (2011). Extracellular calcium reduction strongly increases the lytic capacity of pneumolysin from Streptococcus pneumoniae in brain tissue. J. Infect. Dis. 204, 930–936. doi: 10.1093/infdis/jir434
Wippel, C., Maurer, J., Förtsch, C., Hupp, S., Bohl, A., Ma, J., et al. (2013). Bacterial cytolysin during meningitis disrupts the regulation of glutamate in the brain, leading to synaptic damage. PLoS Pathog. 9:e1003380. doi: 10.1371/journal.ppat.1003380
Witkin, S. S., and Linhares, I. M. (2017). Why do lactobacilli dominate the human vaginal microbiota? BJOG 124, 606–611. doi: 10.1111/1471-0528.14390
Zilnyte, M., Venclovas, C., Zvirbliene, A., and Pleckaityte, M. (2015). The cytolytic activity of vaginolysin strictly depends on cholesterol and is potentiated by human CD59. Toxins 7, 110–128. doi: 10.3390/toxins7010110
Zozaya-Hinchliffe, M., Lillis, R., Martin, D. H., and Ferris, M. J. (2010). Quantitative PCR assessments of bacterial species in women with and without bacterial vaginosis. J. Clin. Microbiol. 48, 1812–1819. doi: 10.1128/JCM.00851-09
Keywords: cholesterol-dependent cytolysin, vaginolysin, inerolysin, Gardnerella spp., Lactobacillus iners, pore-forming mechanism, virulence factors, bacterial vaginosis
Citation: Pleckaityte M (2020) Cholesterol-Dependent Cytolysins Produced by Vaginal Bacteria: Certainties and Controversies. Front. Cell. Infect. Microbiol. 9:452. doi: 10.3389/fcimb.2019.00452
Received: 09 September 2019; Accepted: 12 December 2019;
Published: 10 January 2020.
Edited by:
Mario Vaneechoutte, Ghent University, BelgiumReviewed by:
Brandon Luedtke, University of Nebraska at Kearney, United StatesDerek J. Fisher, Southern Illinois University Carbondale, United States
Copyright © 2020 Pleckaityte. This is an open-access article distributed under the terms of the Creative Commons Attribution License (CC BY). The use, distribution or reproduction in other forums is permitted, provided the original author(s) and the copyright owner(s) are credited and that the original publication in this journal is cited, in accordance with accepted academic practice. No use, distribution or reproduction is permitted which does not comply with these terms.
*Correspondence: Milda Pleckaityte, milda.pleckaityte@bti.vu.lt