- State Key Laboratory of Natural Medicines, Department of Natural Medicinal Chemistry, China Pharmaceutical University, Nanjing, China
The frequent emergence of azole-resistant strains has increasingly led azoles to fail in treating candidiasis. Combination with other drugs is a good option to effectively reduce or retard its incidence of resistance. Natural products are a promising synergist source to assist azoles in treating resistant candidiasis. Eucalyptal D (ED), a formyl-phloroglucinol meroterpenoid, is one of the natural synergists, which could significantly enhance the anticandidal activity of fluconazole (FLC) in treating FLC resistant C. albicans. The checkerboard microdilution assay showed their synergistic effect. The agar disk diffusion test illustrated the key role of ED in synergy. The rhodamine 6G (R6G) efflux assay reflected ED could reduce drug efflux, but quantitative reverse transcription PCR analysis revealed the upregulation of CDR1 and CDR2 genes in ED treating group. Efflux pump-deficient strains were hyper-susceptible to ED, thus ED was speculated to be the substrate of efflux pump Cdr1p and Cdr2p to competitively inhibit the excretion of FLC or R6G, which mainly contributed to its synergistic effect.
Introduction
Candidiasis is the most frequently encountered fungal infection. Recent years have seen its steadily increasing incidence and mortality with risk factors continually emerging (Gamarra et al., 2010; Ruhnke, 2014; Tsai et al., 2015; Li et al., 2016). The azoles such as fluconazole (FLC), itraconazole (ICZ) and ketoconazole (KCZ) are widely and frequently used in clinic to fight candidiasis due to their high efficiency and low toxicity (Marchetti et al., 2003; Prasad and Rawal, 2014; Shrestha et al., 2015; Lu et al., 2017). However, it should be of concern that the continuing emergence of azole-resistant candidiasis leads to frequent failures in clinical treatment (Ahmad et al., 2013; Eddouzi et al., 2013; Liu et al., 2014; Shrestha et al., 2017).
The reason for azoles resistance could be multi-aspect. One of them is the increase of membrane transporters, such as Cdr1p, Cdr2p, and Mdr1p, which can significantly increase drug efflux to allow tolerable intracellular drug concentration for Candida spp. Another important factor is the overexpression or point mutation of ERG11 gene, which can alter the affinity of azoles with target enzyme lanosterol 14-demethylase (Luna-Tapia et al., 2015). Clearly, the current new drugs discovery and development is not efficient enough to combat the rising azole-resistant problems (Harvey et al., 2015). Combination therapy might be an effective way to treat severe fungal infections and to reduce or retard the inducing incidence of resistant strains (Ghannoum and Elewski, 1999; Pinavaz et al., 2005; Holmes et al., 2016). Thus, the combined use of drugs or adjuncts with azoles is now increasingly popular in academic research for the treatment of azole-resistant candidiasis.
The structural variety of natural products makes them a great resource for azole synergists, some of which were already found to be promising. For instance, the fungal metabolized immunosuppressive agents, cyclosporine A (CsA) and tacrolimus (FK506) were found to have synergistic effect with FLC in treating FLC resistant strains (Marchetti et al., 2000; Sun et al., 2008; Uppuluri et al., 2008; Cui et al., 2015). Several other natural products such as diorcinol D, osthole, and garlic also showed significant synergistic activity with FLC, although they were all weak alone (Li et al., 2015a, 2016; Li D. D. et al., 2017). In addition, Formyl-phloroglucinol meroterpenoids (FPMs), which are unique secondary metabolites of Eucalyptus and Psidium genera, have been recognized for their antifungal activities against pathomycetes like Candida and Trichophyton spp (Musyimi and Ogur, 2008; Wong et al., 2015). Our previous research also demonstrated that several novel FPMs exerted antifungal and antibiofilm activities against Candida spp. (Liu R. H. et al., 2017). Further investigations on FPMs showed that those FPMs with weak or without antifungal effects demonstrated enhanced activities when combined with other antifungal FPMs or azoles (data not shown). In this study, eucalyptal D (ED, Figure 1), an FPM, was revealed to have a strong efficacy when in synergy with azoles to reverse the resistance of C. albicans in vitro. However, ED upregulated the expression of efflux pump genes and could not synergistically inhibit CDR-deficient strains with azoles, which along with other experiments, indicated that ED functioned as a more competitive substrate of the Cdr1p and Cdr2p than azoles, and thus inhibited the excretion of azoles.
Materials and Methods
Strains
One reference strain (SC5314), seven clinical isolates including five FLC resistant isolates (24D, 28I, CA102, CA901, and CA112869) and two FLC susceptible strains (CA13, CA21), four transporter-deletion mutant strains (DSY448, DSY653, DSY465, and DSY659) and their parent strain CAF2-1, were used in our study. C. albicans SC5314 was purchased from ATCC, USA. The transporter-deletion mutant strains, and clinical strains 24D, 28I were kindly provided by Prof. Hongxiang Lou from the department of Natural Product Chemistry in Shandong University. The clinical strains CA13, CA21, CA102, CA901, and CA112869 were kindly offered by Prof. Yuanying Jiang from the School of Pharmacy, Second Military Medical University. Strains employed in this study are listed in Table 1. All strains were routinely stored at −80°C in yeast-peptone-dextrose medium (YPD; 1% yeast extract, 2% peptone, and 2% dextrose), supplemented with 20% glycerol (vol/vol) and were subcultured on YPD plates twice at 35°C before each experiment.
Chemicals
Fluconazole (Gibco, USA), ketoconazole (TargetMol, USA), and itraconazole (Gibco, USA) were obtained commercially. ED was previously isolated from the leaves of E. robusta in our laboratory, and its purity (>99.80%) was analyzed by high performance liquid chromatography (HPLC). ED was prepared in dimethyl sulfoxide to achieve stock solutions of 12,800 μg/ml, and FLC was prepared in sterile distilled water to a concentration of 5,120 μg/ml. Ketoconazole and itraconazole were dissolved in dimethyl sulfoxide to form stock solutions of 3,000 and 1,000 μg/ml, respectively. These stock solutions were all stored at −20°C.
Antifungal Susceptibility Test
The minimum inhibitory concentrations (MIC) of ED and FLC against C. albicans strains were determined by the broth microdilution method based in the Clinical and Laboratory Standards Institute (CLSI) standard M27-A3 (CLSI, 2008). One hundred microliter serially diluted drug and 100 μl cells suspension with a final concentration of 0.5–2.5 × 103 cells/ml were added into 96-well plates, then the plates were incubated at 35°C for 24 h. Optical densities at 540 nm (OD540) were measured by microplate reader (Tecan SUNRISE) and the MIC was defined as the concentration of drugs that inhibited 80% of cell growth. For the broth microdilution checkerboard assays, each drug was serially diluted 2-fold in RPMI 1640 as previously described (Tabbene et al., 2015). Briefly, the final ED concentrations ranged from 1 to 64 μg/ml and the final FLC concentrations ranged from 1 to 32 μg/ml for resistant isolates and from 0.0625 to 4 μg/ml for susceptible isolates. A 50-μl aliquot of each ED dilution and FLC dilution was added to the wells in the 2nd to 9th columns and the B to H lines, respectively. Row A and column 1 contained the ED and FLC alone respectively, and the well at the intersection of row A and column 1 was the drug-free one that served as the growth control. The 12th columns were performed in 200 μl RPMI1640 to act as negative controls. One hundred microliter aliquots cells to a final concentration of 0.5–2.5 × 103 cells/ml were added to each well mentioned above. All the wells on the plate were filled with RPMI 1640 to a final volume of 200 μl. The plate was incubated for 24 h at 35°C. Drug interactions were analyzed using the following two different models: the fractional inhibitory concentration index (FICI) model and the percentage of growth difference (ΔE) model (Meletiadis et al., 2003; Sun et al., 2017). FICI model expressed as FICI = FIC of A + FIC of B = MICA comb /MICA alone + MICB comb /MICB alone, where MICA alone and MICB alone are the MICs of drugs A and B acting alone and MICA comb and MICB comb are the concentrations of drugs A and B at the effective combinations, respectively. The FICI was interpreted as synergy when it was ≤ 0.5, as antagonism when it was >4.0, and any value in between was considered indifferent (Li D. D. et al., 2017). ΔE model was calculated as ΔE = E predicted-E measured, where E predicted and E measured are the predicted and measured percentages of growth with drugs A and B at diverse concentrations, respectively. When the ΔE value was positive, synergy was concluded, and higher ΔE values suggested stronger synergistic interactions. Conversely, negative ΔE values represent antagonism (Meletiadis et al., 2003). The ΔE values of each combination were used to depict three-dimensional plot and contour plot by OriginPro 7.5.
Agar Disk Diffusion Test
Disk diffusion testing was done by following the previously described method with a few modifications (Quan et al., 2006; Li et al., 2015b). The C. albicans 24D was used in this test. Briefly, 3 ml of aliquot of 5 × 105 cells/ml suspension was spread uniformly onto a series of yeast peptone dextrose (YPD) agar plates containing ED in different concentrations. A plate with 1% DMSO was used as the vehicle control. The 6-mm-diameter filter disks impregnated with FLC were placed onto the agar surface. The growth inhibition zones were measured after the plates were incubated at 35°C for 24 h. Images were collected using ChemiDOCTM XRS + system with Image Lab™ software.
Quantitative Reverse Transcription PCR
Quantitative reverse transcription PCR (qRT-PCR) was performed to detect the expression levels of efflux pump-related genes (CDR1, CDR2, and MDR1) affected by ED. C. albicans 24D cells were grown overnight in YPD medium and diluted to a cell density of 1.0 × 106 cells/ml in RPMI 1640 medium with treatment at final concentrations of FLC 2 and ED 32 μg/ml. Cultures without drugs served as the control. After the incubation at 35°C for 12 h, cells were harvested for RNA extraction. Total RNAs were isolated using a Yeast RNAiso kit (Takara Bio, China), and OD260/280 was between 1.8-2.2. cDNA was synthesized using the kit HiScript II Q RT SuperMix for qPCR (+gDNA wiper) (Vazyme, Biotech) and diluted five times. qRT-PCR was mixed with cDNA (2 μl), and then fast started with SYBR Green Master Mix kit (Vazyme, Biotech) and gene primers in a final volume of 20 μl. The ACT1 gene was used as the endogenous control. qRT-PCR was carried out on the QuantStudio® 3 real-time PCR System (Applied Biosystems). Primers used in this study referred to Yi et al. (2017) and were listed in Supplementary Table 1. The relative expression levels of target genes were calculated by the 2−ΔΔCT method (Livak and Schmittgen, 2001; Rybak et al., 2017). All experiments were performed three times independently from each other.
R6G EffluxAssay
Rhodamine 6G (R6G) efflux was assessed to determine whether transport proteins activity could be affected by ED as a previously described protocol (Liu X. et al., 2017). Briefly, single colonies of C. albicans 24D were inoculated into liquid YPD medium and grown overnight at 35°C. The cells were resuspended in glucose-free PBS (pH = 7.0) to a final concentration of 1 × 108 cells/ml to de-energized for 2 h after being centrifuged and washed three times. Then, a final R6G concentration of 10 μM was first added to the above-mentioned cells and incubated at 35°C for 55 min. After the R6G uptake into cells was terminated with an ice-water bath for 10 min, the cells were harvested and washed three times with glucose-free PBS to remove the extracellular R6G, and ED was added to the suspension at the concentration of 32 μg/ml. A drug-free sample with only R6G was used as the control group. Then, each 100 μl sample of the extracellular remaining R6G was detected at specific time intervals after the addition of 0.1 M glucose (5, 10, 20, 30, 60 min) and centrifuged at 12,000 g for 30 s. The fluorescence of the supernatant was recorded with Thermo Scientific Varioskan Flash using the SkanIt software (excitation and emission wavelengths of 529 nm and an emission wavelength of 553 nm). All experiments were performed three times.
Spot Assay
Spot assays were performed to measure susceptibilities of transporter-deletion mutant strains to ED and FLC as described elsewhere (Kumar et al., 2014). Briefly, cells were grown overnight in YPD medium at 30°C and adjusted to an initial cell density of 1.0 × 106 cells/ml and were 5-fold serially diluted. Then 5 μl aliquots of serial dilution cells were spotted onto YPD medium containing 128 μg/ml ED. Cultures without drugs served as the controls. The differences in plates after incubation at 30°C for 48 h were visualized and pictures were taken with ChemiDOCTM XRS + system with Image Lab™ software.
Cell Toxicity Assay
The MTT [3(4,5-dimethylthiazol-2yl) 2,5-diphenyltetrazolium bromide] method was used to evaluate its cytotoxicity in vitro Jeon et al., 2014; Liu et al., 2016. Briefly, the LO2 (normal human hepatic cell line) and MCF 10A (normal breast cell line) cell lines were seeded into 96-well plates (5 × 103 cells/well) and then incubated overnight, followed by treatment with various concentrations of ED. After 48 h for LO2 and 24 h for MCF 10A, respectively, the MTT solution was added to each well. After further incubation for 4 h, DMSO was added to dissolve the dark formazan crystals. Optical densities at 570 nm (OD570) were measured by microplate reader (Tecan SUNRISE).
Statistical Analysis
Group data are presented as mean ± SD. Two-way RM ANOVA was used to determine differences between means. Differences were considered significant at P < 0.05. The GraphPad Prism (version 5.0) statistical package was used.
Results
Susceptibilities and Interactions of Drugs Against C. albicans
Five FLC resistant strains, two FLC susceptible isolates and a reference strain were used to evaluate the anti-Candida actions of ED and its combination with FLC. Although ED was active against FLC susceptible strains with MIC value lower than 64 μg/ml, it didn't exhibit evident effects against FLC resistant isolates under the concentration of 128 μg/ml. However, when ED was simultaneously incubated with FLC, the combination showed strong synergistic effects (Table 2). With the help of ED, the concentrations of FLC exerted the same MIC80 values decreased 8-fold and more than 64-fold when treating against susceptible and resistant strains, respectively. The FICI values below 0.5 revealed the synergy between two drugs. The three-dimensional (3D) and contour plot constructed with ΔE values further demonstrated the synergy of most combinations, while the treatment with concentration lower than 2 μg/ml of ED showed almost all peaks below the zero planes, indicating their antagonistic effects (Figure 2A). The succedent evaluation of ED with KCZ and ITZ, respectively, resulted in a similar synergetic effect. ED remarkably reduced their doses in killing all five tested FLC resistant isolates with 4-fold to more than 256-fold potentiation effects (Table 3). Similarly, the 3D plot and contour plot also confirmed their synergies and showed antagonism at the low concentration of ED (Figures 2B,C). It was clear that ED could significantly improve the effect of azoles, especially on FLC resistant strains.
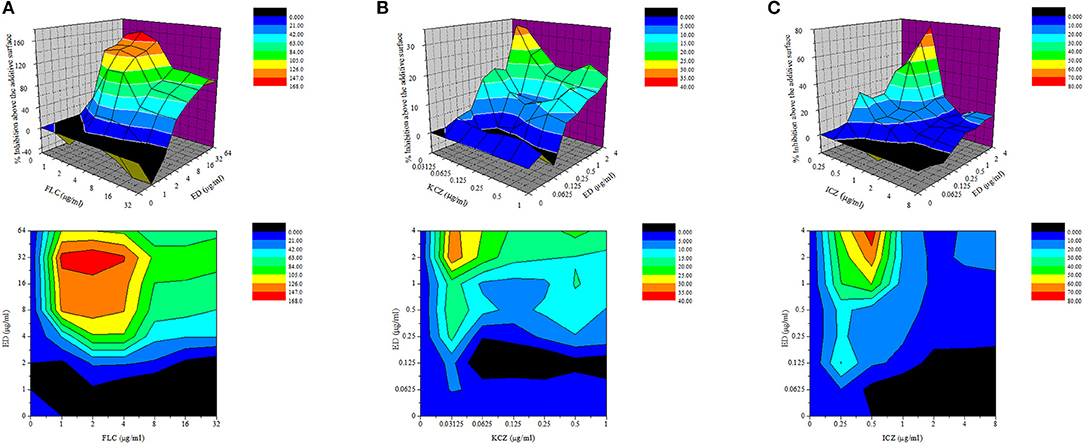
Figure 2. The drug interactions of ED and FLC (A), KCZ (B), and ICZ (C) against resistant C. albicans 24D are interpreted by the ΔE model. The 3D and contour plot were constructed by using OriginPro 7.5. The concentrations of FLC and ED are depicted on the x axis and y axis, respectively, and the ΔE values obtained for each combination is depicted on the z axis. Positive ΔE values represent synergy, and higher ΔE values suggested stronger synergistic interactions. Negative ΔE values represent antagonism.
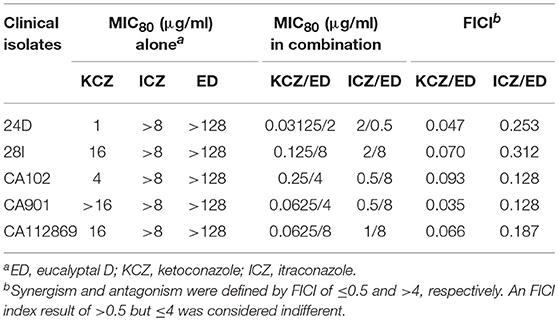
Table 3. Interactions of other azoles and ED against five FLC resistant isolates by microdilution assay.
Interactions of ED and FLC by Agar Disk Diffusion Test
The agar diffusion assay allowed an intuitionistic observation of the combination effects. A dozen combinations with various concentrations of ED and FLC were applied as shown in Figure 3. When using FLC alone, all the three concentration groups did not show any distinct inhibition zone. By contrast, FLC in different concentrations yielded positive inhibition zones with the help of ED, even when the FLC concentration was a quarter of the optimal combination concentration (0.5 μg/ml). And the inhibition zones produced by the same quality of FLC were more distinct and clearer when concentrations of ED increased. Thus, there was no doubt about the important role of ED in synergistic antifungal action.
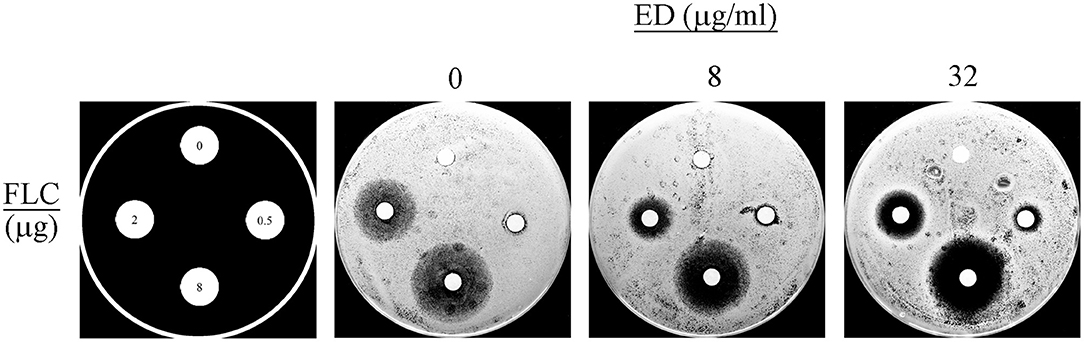
Figure 3. The disk diffusion assay results. The assays were performed by plating albicans 24D (5 × 105 cells/ml) on the agar plates in the absence (DMSO vehicle alone) or presence of ED at the concentration of 8 and 32 μg/ml. FLC was used at 0, 0.5, 2, and 8 μg per disc, respectively. Each plate was incubated at 35°C for 24 h. Large and clear inhibition zones represent better antifungal effect.
Effect of ED on the Expression Levels of Efflux Pump and Other Genes
The expression levels of efflux pump genes were quantified by qRT-PCR, along with those of ergosterol biosynthesis genes related to FLC resistance. Interestingly, the results showed that ED alone could upregulate expression level of CDR1 and CDR2, while the expression level of MDR1 remained nearly unchanged. The expression level of CDR2 was the most upregulated, which was 3.40 (alone) and 1.72 (with FLC) times higher than that of the control (Figure 4). All the three gene levels of combination groups were the compromise of ED and FLC effect (see details in Supplementary Table 2). In the meantime, the relative expression levels of ergosterol biosynthesis genes were evaluated to see if there was any other synergetic pathway (Supplementary Figure 4). Although the combination led to the overexpression of several ergosterol biosynthesis genes, these results were mostly due to FLC which alone could also induce similar expression levels. Thus, ED mainly affected CDR1 and CDR2 expressions in tested strains.
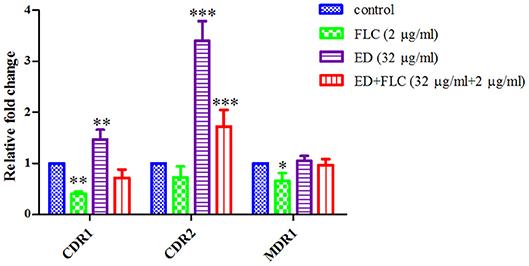
Figure 4. The relative expression levels of efflux genes CDR1, CDR2, and MDR1 in FLC resistant strain 24D. Cells were treated with FLC (2 μg/ml), ED (32 μg/ml), and their combination, respectively. Data are normalized for control group. ACT1 was used as an expression control. Relative fold change above one represents gene upregulation, while below one represents gene downregulation. Data are means ± SD from three experiments. *p < 0.05, **p < 0.01, ***p < 0.001.
Effect of ED on Inhibiting Drug Efflux of Resistant C. albicans
The R6G efflux assay was further used to verify whether ED could influence drug efflux. As shown in Figure 5, the extracellular concentration of R6G in two groups steadily increased at first, while the graph of ED treating group flattened out gradually after 20 min incubation. After 60 min, the R6G concentration of ED group (1.05 ± 0.15 nmol/ml) was about 43.0% of the control (2.44 ± 0.10 nmol/ml). These data obviously revealed that ED could inhibit the efflux of intracellular R6G, and that the predictable same inhibitory effect on azoles efflux would be responsible for the interaction between ED and azoles (see details in Supplementary Table 3).
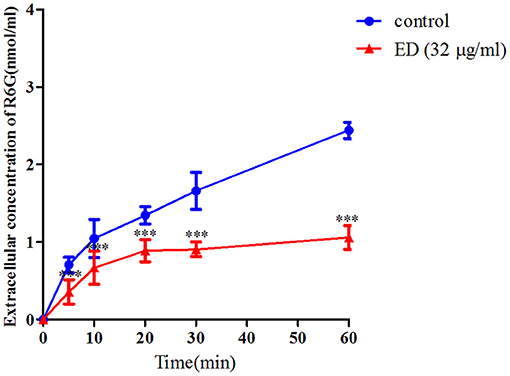
Figure 5. The effect of ED on the efflux of R6G in FLC resistant strain 24D. The R6G fluorescence of supernatant in the absence and presence of ED were determined with a Varioskan Flash (Thermo Scientific) using the SkanIt software. The extracellular concentration of R6G was calculated by using the R6G standard curve. Data are means ± SD from three experiments, ***p < 0.001, compared with the control.
Susceptibilities of ED Against Transporter-Deletion Mutants
The upregulation of pump genes seemed inconsistent with the result of R6G efflux assay, since the overproduction of efflux pumps caused by their upregulated genes should have afforded a higher extracellular concentration of R6G. To further verify the results of R6G efflux assay, susceptibility test, and spot assay were carried out on transporter-deletion mutants. In the drug susceptibility test (Table 4), MIC80 values of ED in CDR1 deficient strain were reduced by more than 50% (from more than 128 μg/ml to lower than 64 μg/ml). Although no activity change was found in the CDR2 deficient strain, inhibition in double mutant strain DSY659 was two times stronger when compared with that of CDR1 deficient strain. Susceptibility was unchanged in MDR1 deficient strain. Similar results were revealed in spot assay. The ABC transporter-deletion mutants DSY448 (cdr1Δ/Δ), DSY653 (cdr2Δ/Δ), and DSY659 (cdr1Δ/cdr2Δ) were hypersensitive to ED. The strain DSY659 (cdr1Δ/cdr2Δ) was especially more sensitive to ED than the parent strain CAF2-1 (Figure 6). More significantly, ED failed to enhance FLC activity when the combinations were facing the double mutant DSY659 (cdr1Δ/cdr2Δ) in checkerboard microdilution assay (Supplementary Figure 1).
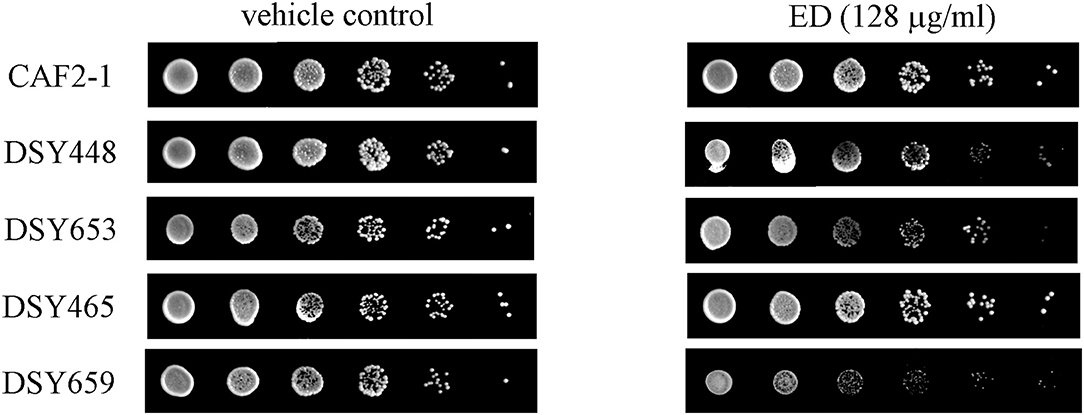
Figure 6. The susceptibility of ED and FLC against transporter-deletion mutants by spot assay. Cells were adjusted to 1 × 107 cells/ml, 5-fold serially diluted, and spotted onto YPD medium containing 128 μg/ml ED or 1% DMSO (vehicle control). The plates were incubated at 30°C for 48 h. less colony means more sensitive to drug.
Cytotoxicity of ED on Human Normal Cell Lines
The in vitro cytotoxic effect was tested on LO2 and MCF 10A cell lines. As showed in Supplementary Figure 2, ED at the concentration of 32 μg/ml had a slight inhibition on LO2 cell lines and no cytotoxicity on MCF 10A cell lines.
Discussion
Drug combination is commonly used to treat various illnesses. It helps to increase drug potency and to decrease toxicity, meanwhile it allows to retard the potential drug resistance and to recover the potency of resistant drugs (Cui et al., 2015; Li et al., 2015a). Drug combination is also a valid and pragmatic strategy to overcome resistance in C. albicans infections (Gu et al., 2016; Sun et al., 2016). There were many antifungal researches on drug combination, which investigated not only clinical drugs but also their combination with natural products. Some non-antifungal agents such as Hsp90 inhibitor geldanamycin and calcium homeostasis regulator amiodarone were found to be useful in improving activities of antifungal drugs (Gamarra et al., 2010; Hill et al., 2013; Liu et al., 2014). Some natural products did display prominent synergistic effects when used in combination with azoles (Li et al., 2016; Li D. D. et al., 2017; Sun et al., 2017). Yet to our knowledge, most of the combinations were to increase the intercellular concentration by reducing the overexpressed efflux pump genes (Eddouzi et al., 2013; Sarah et al., 2016).
Although ED showed weak antifungal activity, it was found to exert outstanding synergistic effect with FLC, KCZ, and ICZ on FLC resistant C. albicans strains (Tables 2, 3). Reconfirmation was conducted by the non-parametric ΔE model based on BI theory (Meletiadis et al., 2003; Sun et al., 2008), which verified the synergy effect of ED with azoles at different concentrations. Meanwhile, it was interesting to find that ED below specific low concentrations yielded antagonistic effects in all the combinations with tested azoles (Figures 2A–C). The antagonism was rarely observed in previous combination studies using dexamethasone, thymol, and carvacrol which were able to downregulate the expression of drug efflux pump genes (Ahmad et al., 2013; Sun et al., 2017). Thus, a different action of ED could be responsible for its synergetic effect. Subsequently, we tried to figure out the role played by ED in the synergy effect. As was shown by its inhibition zones in the agar disk diffusion test, the inhibition of FLC against C. albicans was dose dependent. However, complete inhibition could only be achieved by the participation of ED (Figure 3). Obviously, ED could assist FLC in killing resistant C. albicans when applying them together.
By quantifying the gene expression level, we knew that the resistance of tested strain 24D was mainly due to the overexpression in genes of ABC, MFS (major facilitator superfamily) plasma membrane transporter, and ERG11 key gene encoding FLC target enzyme lanosterol 14-demethylase (Supplementary Table 4). This clue gave priority to find out if there would be any differences on gene expression levels after treating with ED. It was unforeseen that ED led to the upregulation of CDR1 and CDR2 genes (Figure 4) which was always correlated with the increase of drug efflux, resulting in the resistance to FLC (Eddouzi et al., 2013; Bhattacharya et al., 2016; Sun et al., 2017). However, ED not only induced the overexpression of efflux pump genes but also simultaneously helped FLC to reverse the resistance. At the same time, it was a little unusual that FLC slightly downregulated the level of CDR1 and CDR2. Investigations on references indicated that this downregulation could be due to the strain's peculiarity (Guo et al., 2014; Wei et al., 2016), the treatment concentration or time of FLC (De Backer et al., 2001; Lepak et al., 2006). An additional test was carried out on other drug-resistant and susceptible strains (28I and CA21). Both of them overexpressed the CDR genes after treatment with either FLC or ED (Supplementary Figure 5). Therefore, ED could consistently upregulate the expression of efflux pump genes in different C. albicans strains. The accumulation of fluorescent dyes at different time intervals showed the ability of efflux pump (Kolaczkowski et al., 2009). The dye R6G can be used to identify the CDR pump-mediated azole-resistant strains (Maesaki et al., 1999; Holmes et al., 2016), and R6G assay is also widely applied in evidencing whether synergists could reduce the extrusion of drugs (Ahmad et al., 2013; Sun et al., 2017). In our test, the R6G assay undoubtfully confirmed that ED could reduce the efflux of FLC. This inhibition obviously surpassed ED's simultaneous effect on the upregulation of efflux pump genes, otherwise ED could not reverse the resistance to FLC. Further literature investigations revealed that fluphenazine, a known substrate of Cdr1p and Cdr2p, also had similar implications (Henry et al., 1999). It could competitively inhibit the efflux of FLC at high concentrations, and meanwhile could upregulate the expression of efflux pump genes. Judging from the similar behavior, we thought that ED might have played the same role as a substrate of Cdr1p and Cdr2p. One of the strategies used to screen for substrates of efflux pumps is to test drugs on the mutants of efflux pump genes (Sanglard et al., 1996; Tsao et al., 2009; Szczepaniak et al., 2015; Li S. et al., 2017; Chang et al., 2018). A series of efflux pump deletion strains were thus used to confirm whether and which efflux transporters were affected by ED. Compared with the parental strain C. albicans CAF2-1, the CDR1 deficient strain was more susceptible to ED (Table 4 and Figure 6), which meant that ED was pumped out mainly by Cdr1p in wild strains. Considering the synergistic effect with azoles, ED was most likely a more competitive substrate for Cdr1p than azoles. In line with this, ED could not assist FLC anymore when used against double mutant strain DSY659 (cdr1Δ/cdr2Δ) (Supplementary Figure 1). We tried to directly quantify the variation of extracellular FLC concentrations in vain due to the poor quantification limit of microspectrophotometer and HPLC detector. Nevertheless, the competitive inhibition on FLC efflux is the most likely mechanism for the synergistic effect of ED according to this study. In addition, since ED alone did show weak inhibition on susceptible strains, its inhibiting effect could be another synergistic factor when combined with azoles. Yet it's not the key factor as no synergy was found when the combination facing CDR double mutants.
Conclusions
In this study, azoles were found to significantly restore their antifungal activities against azole-resistant C. albicans when they were applied in combination with ED, a formyl-phloroglucinol meroterpenoid. Differently from the other synergists that normally reduce the expression level of efflux pump genes, ED was found to competitively inhibit FLC from being excreted. It suggests that FPMs like ED are hopeful to be developed as an antifungal adjunct of azoles.
Author Contributions
JX, FS, LA, and ZS performed the experiments. JX and RL designed the research. JX and MY analyzed the data and wrote the paper. LK reviewed the manuscript and the experiments. All authors read and approved the final manuscript.
Funding
This research work was funded by the National Natural Science Foundation of China (81503218, 81773901), the Program for Changjiang Scholars and Innovative Research Team in University (IRT_15R63), the Priority Academic Program Development of Jiangsu Higher Education Institutions (PAPD) and the Program of Introducing Talents of Discipline to Universities (B18056).
Conflict of Interest Statement
The authors declare that the research was conducted in the absence of any commercial or financial relationships that could be construed as a potential conflict of interest.
Acknowledgments
We thank Professor Hongxiang Lou from Department of Natural Product Chemistry in Shandong University for offering two clinical strains and mutant strains. We also thank Prof. Yuanying Jiang from College of Pharmacy in Second Military Medical University for providing five clinical strains.
Supplementary Material
The Supplementary Material for this article can be found online at: https://www.frontiersin.org/articles/10.3389/fcimb.2019.00211/full#supplementary-material
References
Ahmad, A., Khan, A., and Manzoor, N. (2013). Reversal of efflux mediated antifungal resistance underlies synergistic activity of two monoterpenes with fluconazole. Eur. J. Pharm. Sci. 48, 80–86. doi: 10.1016/j.ejps.2012.09.016
Bhattacharya, S., Sobel, J. D., and White, T. C. (2016). A combination fluorescence assay demonstrates increased efflux pump activity as a resistance mechanism in azole-resistant vaginal Candida albicans isolates. Antimicrob. Agents Chemother. 60, 5858–5866. doi: 10.1128/AAC.01252-16
Chang, W., Liu, J., Zhang, M., Shi, H., Zheng, S., Jin, X., et al. (2018). Efflux pump-mediated resistance to antifungal compounds can be prevented by conjugation with triphenylphosphonium cation. Nat. Commun. 9:5102. doi: 10.1038/s41467-018-07633-9
CLSI (2008). Reference Methods for Broth Dilution Antifungal susceptibility testing of yeast; Approved Standard-third edition, CLSI Document M27-A3. Wayne, PA: CLSI.
Cui, J., Ren, B., Tong, Y., Dai, H., and Zhang, L. (2015). Synergistic combinations of antifungals and anti-virulence agents to fight against Candida albicans. Virulence. 6, 362–371. doi: 10.1080/21505594.2015.1039885
De Backer, M. D., Ilyina, T., Ma, X. J., Vandoninck, S., Luyten, W. H., and Vanden, B. H. (2001). Genomic profiling of the response of Candida albicans to itraconazole treatment using a dna microarray. Antimicrob. Agents Chemother. 45, 1660–1670. doi: 10.1128/AAC.45.6.1660-1670.2001
Eddouzi, J., Parker, J. E., Valesilva, L. A., Coste, A., Ischer, F., Kelly, S., et al. (2013). Molecular mechanisms of drug resistance in clinical Candida species isolated from tunisian hospitals. Antimicrob. Agents Chemother. 57, 3182–3193. doi: 10.1128/AAC.00555-13
Fonzi, W. A., and Irwin, M. Y. (1993). Isogenic strain construction and gene mapping in Candida albicans. Genetics. 134, 717–728.
Gamarra, S., Rocha, E. M., Zhang, Y. Q., Park, S., Rao, R., and Perlin, D. S. (2010). Mechanism of the synergistic effect of amiodarone and fluconazole in Candida albicans. Antimicrob. Agents Chemother. 54, 1753–1761. doi: 10.1128/AAC.01728-09
Ghannoum, M. A., and Elewski, B. (1999). Successful treatment of fluconazole-resistant oropharyngeal candidiasis by a combination of fluconazole and terbinafine. Clin. Diagn. Lab. Immunol. 6, 921–923. doi: 10.1006/cimm.1999.1566
Gu, W., Guo, D., Zhang, L., Xu, D., and Sun, S. (2016). The synergistic effect of azoles and fluoxetine against resistant Candida albicans strains is attributed to attenuating fungal virulence. Antimicrob. Agents Chemother. 60, 6179–6188. doi: 10.1128/AAC.03046-15
Guo, H., Xie, S. M., Li, S. X., Song, Y. J., Lv, X. L., and Zhang, H. (2014). Synergistic mechanism for tetrandrine on fluconazole against Candida albicans through the mitochondrial aerobic respiratory metabolism pathway. J. Med. Microbiol. 63, 988–996. doi: 10.1099/jmm.0.073890-0
Harvey, A. L., Edrada-Ebel, R. A., and Quinn, R. J. (2015). The re-emergence of natural products for drug discovery in the genomics era. Nat. Rev. Drug. Discov. 14, 111–129. doi: 10.1038/nrd4510
Henry, K. W., Cruz, M. C., Katiyar, S. K., and Edlind, T. D. (1999). Antagonism of azole activity against Candida albicans following induction of multidrug resistance genes by selected antimicrobial agents. Antimicrob. Agents Chemother. 43, 1968–1974. doi: 10.1128/AAC.43.8.1968
Hill, J. A., Ammar, R., Torti, D., Nislow, C., and Cowen, L. E. (2013). Genetic and genomic architecture of the evolution of resistance to antifungal drug combinations. PLoS Genet. 9:e1003390. doi: 10.1371/journal.pgen.1003390
Holmes, A. R., Cardno, T. S., Strouse, J. J., Ivnitski-Steele, I., Keniya, M. V., Lackovic, K., et al. (2016). Targeting efflux pumps to overcome antifungal drug resistance. Future. Med. Chem. 8, 1485–1501. doi: 10.4155/fmc-2016-0050
Jeon, H., Kim, J. H., Lee, E., Jang, Y. J., and Lee, K. W. (2014). Methionine deprivation suppresses triple-negative breast cancer metastasis in vitro and in vivo. Oncotarget. 7, 67223–67234. doi: 10.18632/oncotarget.11615
Kolaczkowski, M., Kolaczkowska, A., Motohashi, N., and Michalak, K. (2009). New high-throughput screening assay to reveal similarities and differences in inhibitory sensitivities of multidrug ATP-binding cassette transporters. Antimicrob. Agents Chemother. 53, 1516–1527. doi: 10.1128/AAC.00956-08
Kumar, A., Dhamgaye, S., Maurya, I. K., Singh, A., Sharma, M., and Prasad, R. (2014). Curcumin targets cell wall integrity via calcineurin-mediated signaling in Candida albicans. Antimicrob. Agents Chemother. 58, 167–175. doi: 10.1128/AAC.01385-13
Lepak, A., Nett, J., Lincoln, L., Marchillo, K., and Andes, D. (2006). Time course of microbiologic outcome and gene expression in Candida albicans during and following in vitro and in vivo exposure to fluconazole. Antimicrob Agents Chemother. 50, 1311–13119. doi: 10.1128/AAC.50.4.1311-1319.2006
Li, D. D., Chai, D., Huang, X. W., Guan, S. X., Du, J., Zhang, H. Y., et al. (2017). Potent in vitro synergism of fluconazole and osthole against fluconazole-resistant Candida albicans. Antimicrob. Agents Chemother. 61:e00436–17. doi: 10.1128/AAC.00436-17
Li, S., Shi, H., Chang, W., Li, Y., Zhang, M., Qiao, Y., et al. (2017). Eudesmane sesquiterpenes from chinese liverwort are substrates of CDRs and display antifungal activity by targeting ERG6 and ERG11 of Candida albicans. Bioorg Med Chem. 25, 5764–5771. doi: 10.1016/j.bmc.2017.09.001
Li, W. R., Shi, Q. S., Dai, H. Q., Liang, Q., Xie, X. B., Huang, X. M., et al. (2016). Antifungal activity, kinetics and molecular mechanism of action of garlic oil against Candida albicans. Sci. Rep. 6:22805. doi: 10.1038/srep22805
Li, Y., Chang, W., Zhang, M., Li, X., Jiao, Y., and Lou, H. (2015a). Synergistic and drug-resistant reversing effects of diorcinol d combined with fluconazole against Candida albicans. FEMS Yeast Res. 15:fov001. doi: 10.1093/femsyr/fov001
Li, Y., Wan, Z., Liu, W., and Li, R. (2015b). Synergistic activity of chloroquine with fluconazole against fluconazole-resistant isolates of Candida species. Antimicrob. Agents Chemother. 59, 1365–1369. doi: 10.1128/AAC.04417-14
Liu, L. J., Wang, W., Kang, T. S., Liang, J. X., Liu, C., Kwong, D. W. J., et al. (2016). Antagonizing stat5b dimerization with an osmium complex. Sci. Rep. 6:36044. doi: 10.1038/srep36044
Liu, R. H., Shang, Z. C., Li, T. X., Yang, M. H., and Kong, L. Y. (2017). In vitro antibiofilm activity of eucarobustol E against Candida albicans. Antimicrob. Agents Chemother. 61:e02707–16. doi: 10.1128/AAC.02707-16
Liu, S., Hou, Y., Chen, X., Gao, Y., Li, H., and Sun, S. (2014). Combination of fluconazole with non-antifungal agents: a promising approach to cope with resistant Candida albicans infections and insight into new antifungal agent discovery. Int. J. Antimicrob. Agents. 43, 395–402. doi: 10.1016/j.ijantimicag.2013.12.009
Liu, X., Li, T., Wang, D., Yang, Y., Sun, W., Liu, J., et al. (2017). Synergistic antifungal effect of fluconazole combined with licofelone against resistant Candida albicans. Front. Microbiol. 8:2101. doi: 10.3389/fmicb.2017.02101
Livak, K. J., and Schmittgen, T. D. (2001). Analysis of relative gene expression data using real-time quantitative PCR and the 2(-delta delta c(t)) method. Methods.25, 402–408 doi: 10.1006/meth.2001.1262
Lu, M., Yu, C., Cui, X., Shi, J., Yuan, L., and Sun, S. (2017). Gentamicin synergizes with azoles against resistant Candida albicans. Int. J. Antimicrob. Agents. 51, 107–114. doi: 10.1016/j.ijantimicag.2017.09.012
Luna-Tapia, A., Kerns, M. E., Eberle, K. E., Jursic, B. S., and Palmer, G. E. (2015). Trafficking through the late endosome significantly impacts Candida albicans tolerance of the azole antifungals. Antimicrob. Agents Chemother. 59, 2410–2420. doi: 10.1128/AAC.04239-14
Maesaki, S., Marichal, P., Vanden, B. H., Sanglard, D., and Kohno, S. (1999). Rhodamine 6G efflux for the detection of CDR1-overexpressing azole-resistant Candida albicans strains. J. Antimicrob Chemother. 44, 27–31. doi: 10.1093/jac/44.1.27
Marchetti, O., Moreillon, P., Glauser, M. P., Bille, J., and Sanglard, D. (2000). Potent synergism of the combination of fluconazole and cyclosporine in Candida albicans. Antimicrob. Agents Chemother. 44, 2373–81. doi: 10.1128/AAC.44.9.2373-2381.2000
Marchetti, O., Moreillon, P., Josè, M, Entenza, V. J., Glauser, M. P., Bille, J., et al. (2003). Fungicidal synergism of fluconazole and cyclosporine in Candida albicans is not dependent on multidrug efflux transporters encoded by the CDR1, CDR2, CaMDR1, and FLU1 genes. Antimicrob. Agents Chemother. 47, 1565–1570. doi: 10.1128/AAC.47.5.1565-1570.2003
Meletiadis, J., Mouton, J. W., Meis, J. F. G. M., and Verweij, P. E. (2003). In vitro drug interaction modeling of combinations of azoles with terbinafine against clinical scedosporium prolificans isolates. Antimicrob. Agents Chemother. 47, 106–117. doi: 10.1128/AAC.47.1.106-117.2003
Musyimi, D., and Ogur, J. (2008). Comparative assessment of antifungal activity of extracts from eucalyptus globulus and eucalyptus citriodora. Res. J. Phytochem. 2, 35–43. doi: 10.3923/rjphyto.2008.35.43
Pinavaz, C., Rodrigues, A., Costadeoliveira, S., Ricardo, E., and Mardh, PerAnders. (2005). Potent synergic effect between ibuprofen and azoles on Candida resulting from blockade of efflux pumps as determined by fun-1 staining and flow cytometry. J. Antimicrob Chemother. 56, 678–685. doi: 10.1093/jac/dki264
Prasad, R., and Rawal, M. K. (2014). Efflux pump proteins in antifungal resistance. Front. Pharmacol. 5:202. doi: 10.3389/fphar.2014.00202
Quan, H., Cao, Y. Y., Xu, Z., Zhao, J. X., Gao, P. H., Qin, X. F., et al. (2006). Potent in vitro synergism of fluconazole and berberine chloride against clinical isolates of Candida albicans resistant to fluconazole. Antimicrob. Agents Chemother. 50, 1096–1099. doi: 10.1128/AAC.50.3.1096-1099.2006
Ruhnke, M. (2014). Antifungal stewardship in invasive Candida infections. Clin. Microbiol. Infect. 20, 11–18. doi: 10.1111/1469-0691.12622
Rybak, J. M., Dickens, C. M., Parker, J. E., Caudle, K., Manigaba, K., Whaley, S. G., et al. (2017). Loss of c-5 sterol desaturase activity results in increased resistance to azole and echinocandin antifungals in a clinical isolate of candida parapsilosis. Antimicrob Agents Chemother. 61:AAC.00651–17. doi: 10.1128/AAC.00651-17
Sanglard, D., and Coste, A. T. (2015). Activity of isavuconazole and other azoles against candida clinical isolates and yeast model systems with known azole resistance mechanisms. Antimicrob Agents Chemother. 60:AAC.02157–15. doi: 10.1128/AAC.02157-15
Sanglard, D., Ischer, F., Monod, M., and Bille, J. (1996). Susceptibilities of Candida albicans multidrug transporter mutants to various antifungal agents and other metabolic inhibitors. Antimicrob Agents Chemother. 40, 2300–2305. doi: 10.1128/AAC.40.10.2300
Sanglard, D., Ischer, F., Monod, M., and Bille, J. (1997). Cloning of Candida albicans genes conferring resistance to azole antifungal agents: characterization of CDR2, a new multidrug ABC transporter gene. Microbiology. 143, 405–416. doi: 10.1099/00221287-143-2-405
Sarah, T.S., Sandra, W., Christine, C., Dominic, N., Elaheh, A., and Martine, R. (2016). Positive regulation of the Candida albicans multidrug efflux pump Cdr1p function by phosphorylation of its n-terminal extension. J. Antimicrob. Chemother. 71, 3125–3134. doi: 10.1093/jac/dkw252
Shrestha, S. K., Fosso, M. Y., and Garneautsodikova, S. (2015). A combination approach to treating fungal infections. Sci. Rep. 5:17070. doi: 10.1038/srep17070
Shrestha, S. K., Garzan, A., and Garneautsodikova, S. (2017). Novel alkylated azoles as potent antifungals. Eur. J. Med. Chem. 133, 309–318. doi: 10.1016/j.ejmech.2017.03.075
Sun, S., Li, Y., Guo, Q., Shi, C., Yu, J., and Ma, L. (2008). In vitro interactions between tacrolimus and azoles against Candida albicans determined by different methods. Antimicrob. Agents Chemother. 52, 409–417. doi: 10.1128/AAC.01070-07
Sun, W., Sanderson, P., and Zheng, W. (2016). Drug combination therapy increases successful drug repositioning. Drug. Discov. Today. 21, 1189–1195. doi: 10.1016/j.drudis.2016.05.015
Sun, W., Wang, D., Yu, C., Huang, X., Li, X., and Sun, S. (2017). Strong synergism of dexamethasone in combination with fluconazole against resistant Candida albicans mediated by inhibiting drug efflux and reducing virulence. Int J. Antimicrob. Agents. 50, 399–405. doi: 10.1016/j.ijantimicag.2017.03.015
Szczepaniak, J., Łukaszewicz, M., and Krasowska, A. (2015). Detection of inhibitors of Candida albicans CDR transporters using a dis-c3(3) fluorescence. Front. Microbiol. 6:176. doi: 10.3389/fmicb.2015.00176
Tabbene, O., Di, G. A., Azaiez, S., Ben, S. I., Elkahoui, S., Alfeddy, M. N., et al. (2015). Synergistic fungicidal activity of the lipopeptide bacillomycin d with amphotericin b against pathogenic Candida species. FEMS Yeast Res. 15:fov022. doi: 10.1093/femsyr/fov022
Tsai, M. H., Wang, S. H., Hsu, J. F., Lin, L. C., Chu, S. M., Huang, H. R., et al. (2015). Clinical and molecular characteristics of bloodstream infections caused by Candida albicans in children from 2003 to 2011. Clin. Microbiol. Infect. 21:1018.e1–8. doi: 10.1016/j.cmi.2015.06.024
Tsao, S., Rahkhoodaee, F., and Raymond, M. (2009). Relative contributions of the Candida albicans ABC transporters Cdr1p and Cdr2p to clinical azole resistance. Antimicrob. Agents Chemother. 53, 1344–1352. doi: 10.1128/AAC.00926-08
Uppuluri, P., Nett, J., Heitman, J., and Andes, D. (2008). Synergistic effect of calcineurin inhibitors and fluconazole against Candida albicans biofilms. Antimicrob. Agents Chemother. 52, 1127–1132. doi: 10.1128/AAC.01397-07
Wei, J., Zhang, H., Li, C., Gang, L., Liu, X., and Wei, J. (2016). The calcineruin inhibitor cyclosporine a synergistically enhances the susceptibility of Candida albicans biofilms to fluconazole by multiple mechanisms. BMC Microbiol. 16. 113. doi: 10.1186/s12866-016-0728-1
Wong, J. H., Lau, K. M., Wu, Y. O., Ling, C., Wong, C. W., Yew, D. T., et al. (2015). Antifungal mode of action of macrocarpal c extracted from eucalyptus globulus labill (lan an) towards the dermatophyte trichophyton mentagrophytes. Chin. Med. 10, 34. doi: 10.1186/s13020-015-0068-3
Keywords: fluconazole resistance, Candida albicans, eucalyptal D, efflux pump substrate, synergism, formyl-phloroglucinol meroterpenoids
Citation: Xu J, Liu R, Sun F, An L, Shang Z, Kong L and Yang M (2019) Eucalyptal D Enhances the Antifungal Effect of Fluconazole on Fluconazole-Resistant Candida albicans by Competitively Inhibiting Efflux Pump. Front. Cell. Infect. Microbiol. 9:211. doi: 10.3389/fcimb.2019.00211
Received: 08 January 2019; Accepted: 31 May 2019;
Published: 20 June 2019.
Edited by:
Guilhem Janbon, Institut Pasteur, FranceReviewed by:
Vishukumar Aimanianda, Institut Pasteur, FranceSlavena Vylkova, Friedrich Schiller University Jena, Germany
Copyright © 2019 Xu, Liu, Sun, An, Shang, Kong and Yang. This is an open-access article distributed under the terms of the Creative Commons Attribution License (CC BY). The use, distribution or reproduction in other forums is permitted, provided the original author(s) and the copyright owner(s) are credited and that the original publication in this journal is cited, in accordance with accepted academic practice. No use, distribution or reproduction is permitted which does not comply with these terms.
*Correspondence: Lingyi Kong, Y3B1X2x5a29uZ0AxMjYuY29t; Minghua Yang, c2tlZXBqYWNrQDE2My5jb20=