- 1Department of Otolaryngology, The First Affiliated Hospital, Sun Yat-sen University, Guangzhou, China
- 2Guangzhou Key Laboratory of Otorhinolaryngology, Guangzhou, China
- 3Department of Surgery-Otolaryngology Head and Neck Surgery, University of Adelaide, Adelaide, SA, Australia
- 4Department of Otolaryngology-Head and Neck Surgery, Hokkaido University Graduate School of Medicine, Sapporo, Japan
Background: Pseudomonas aeruginosa causes severe chronic respiratory diseases and is associated with recalcitrant chronic rhinosinusitis (CRS). P. aeruginosa exoproteins contain virulence factors and play important roles in the pathogenicity of P. aeruginosa, however their role in CRS pathophysiology remains unknown.
Methods: We isolated P. aeruginosa clinical isolates (CIs) and obtained clinical information from 21 CRS patients. Elastase activity of the CIs was measured at different phases of growth. Primary human nasal epithelial cells (HNECs) were cultured at air-liquid interface (ALI) and challenged with P. aeruginosa exoproteins or purified elastase, followed by measuring Transepithelial Electrical Resistance (TEER), permeability of FITC-dextrans, western blot, and immunofluorescence.
Results: 14/21 CIs had a significant increase in elastase activity in stationary phase of growth. There was a highly significant strong correlation between the in vitro elastase activity of P. aeruginosa CIs with mucosal barrier disruption evidenced by increased permeability of FITC-dextrans (r = 0.95, p = 0.0004) and decreased TEER (r = −0.9333, P < 0.01) after 4 h of challenge. Western blot showed a significant degradation of ZO-1, Occludin and β-actin in relation to the elastase activity of the exoproteins. There was a highly significant correlation between the in vitro elastase activity of P. aeruginosa CIs and CRS disease severity (for log phase, r = 0.5631, p = 0.0097; for stationary phase, r = 0.66, p = 0.0013) assessed by CT imaging of the paranasal sinuses.
Conclusion: Our results implicate P. aeruginosa exoproteins as playing a major role in the pathophysiology of P. aeruginosa associated CRS by severely compromising mucosal barrier structure and function.
Introduction
Pseudomonas aeruginosa is an opportunistic pathogen causing a wide range of community and nosocomial infections of the airway, urinary tract, and skin such as chronic wounds and burns. It can cause aggressive infections particularly in patients with compromised host defense mechanisms or those affected by pre-existing conditions, such as cystic fibrosis (CF) (Kobayashi et al., 2009; Marvig et al., 2015). Microbiome studies indicate that P. aeruginosa dominates the lower airway niche in > 50% of adult CF patients (Filkins et al., 2012). P. aeruginosa secretes a number of virulence factors including proteases, toxins, phenazines, and pyocyanin that play crucial roles in infection (Coggan and Wolfgang, 2012). Secreted proteases include elastase A (LasA), elastase B (LasB), alkaline protease (AP), protease IV (PIV), and P. aeruginosa aminopeptidase (PAAP). They interact with the host during pathogenic infections and have a critical role in invasiveness (Janda and Bottone, 1981; Bleves et al., 2010). The expression of these virulence factors is coordinated and regulated by the las and rhl quorum sensing (QS) systems (Nouwens et al., 2003). Mutations in these systems significantly inhibit overall virulence factor production reducing the severity of both acute and chronic P. aeruginosa infections (Smith and Iglewski, 2003). P. aeruginosa elastase (LasB) is a major exoprotein and the primary elastolytic enzyme (Peters and Galloway, 1990). It plays an important role in the pathogenesis of P. aeruginosa infection and has been shown to have a highly efficient proteolytic activity on a range of host proteins. These include structural proteins such as elastin, critically important for maintaining the integrity of blood vessels as well as the elasticity of the lung, and collagen type III and IV, important for maintaining basement membrane integrity. P. aeruginosa elastase-induced disintegration of those proteins is thought to contribute to lung fibrosis and vasculitis in CF patients (Schultz and Miller, 1974; Bruce et al., 1985). LasB also degrades proteins critical in the human immune defense system such as IgA (Heck et al., 1990), IgG (Bainbridge and Fick, 1989), key components of the complement system (Schultz and Miller, 1974), and Surfactant Protein A and D (Mariencheck et al., 2003; Alcorn and Wright, 2004). Purified P. aeruginosa elastase has been reported to cause a transient disruption of tight junctions of airway epithelial cells and is thought to enhance the permeability of mucosal membranes facilitating tissue invasion (Azghani et al., 2000; Cowell et al., 2003; Nomura et al., 2014). Increased mucosal permeability is associated with airway inflammation and is a major contributor to respiratory infection (Coyne et al., 2002).
P. aeruginosa is frequently isolated from the sinonasal cavities of chronic rhinosinusitis (CRS) patients and is associated with severe recalcitrant CRS (Cleland et al., 2016). CRS patients with diabetes have been shown to be significantly more likely to have P. aeruginosa infections, and the infection rate of P. aeruginosa is correlated with the presence of nasal polyps (Zhang et al., 2014). Nasal polyps have decreased trans-epithelial electrical resistance and an irregular, decreased expression of the tight junction proteins occludin and zonulaoccludens-1 (ZO-1), critical for the normal functioning of the mucosal barrier (Soyka et al., 2012). Despite substantial evidence of the role of extracellular virulence factors and proteases including P. aeruginosa elastase in airway pathologies, the correlation between P. aeruginosa elastase activity of CRS clinical isolates and disease severity in the context of CRS remains unknown.
This study determined the P. aeruginosa elastase activity in clinical isolates (CIs) harvested from the sinonasal cavities of CRS patients, and determined the relationship between elastase activity, effect on the mucosal barrier structure and function, and its relation to objective CRS disease severity scores.
Materials and Methods
Study Participants and Disease Characteristics
This study was carried out in accordance with the recommendations of The Queen Elizabeth Hospital Human Research Ethics Committee with written informed consent from all subjects in accordance with the Declaration of Helsinki. HNECs for in vitro experiments were harvested from 4 independent control patients without CRS or cystic fibrosis (CF) (two males, two females, aged 32–71 years). Demographics, diagnoses, Lund-Mackay Computed Tomography (CT) scores, Lund-Kennedy endoscopic scores, Sino-nasal Outcome Test 22 (SNOT-22) scores (within 12 months of the swab being taken) and other clinical information of CRS patients with P. aeruginosa infection were collected.
Human Nasal Epithelial Cell Culture
HNECs were harvested from the inferior turbinate using a nasal brush and cultured as described (Ramezanpour et al., 2016, 2017). Briefly, extracted HNECs were suspended in Bronchial Epithelial Growth Media (BEGM, CC-3170, Lonza, Walkersville, MD, USA), supplemented with 2% Ultroser G (Pall Corporation, Port Washington, NY, USA). The cell suspension was depleted of monocytes using anti-CD68 (Dako, Glostrup, Denmark) coated culture dishes, and HNECs expanded in routine cell culture conditions of 37°C humidified air with 5% CO2 in collagen coated flasks (Thermo Scientific, Walthman, MA, USA). HNECs were tested at passage two and confirmed to be of epithelial lineage via reactivity to pan-Cytokeratin and CD45 antibodies (both from Abcam, Cambridge, MA, USA), and a Diff-Quick staining method used in the assessment of cell morphology by professional cytologists (IMVS, The Queen Elizabeth Hospital, Woodville, Australia).
Air Liquid Interface Culture
HNECs were maintained at an Air-Liquid Interface (ALI), following the Lonza ALI culture method (Lonza, Walkersville, USA). Briefly, Transwells (BD Biosciences, San Jose, California, USA) were coated with collagen (Stem cell Technologies, Australia). 0.7 × 106 HNECs were seeded in 100 μL B-ALI growth medium into the apical chamber of the Transwell plate and 500 μL of B-ALI growth medium was added to the basal chamber in all wells containing the inserts. Cells were incubated at 37°C. On day 3 after seeding, B-ALI growth medium was removed from the apical and basal chambers and 500 μL B-ALI differentiation medium was added to the basal chamber only, exposing the apical cell surface to the atmosphere.
Preparation of P. aeruginosa Exoproteins
P. aeruginosa PAO1 reference strain was obtained from American Type Culture Collection (ATCC) and in total 21 P. aeruginosa clinical isolates were harvested from the sinonasal cavities of CRS patients using endoscopically-guided sinus swabs. P. aeruginosa CIs were isolated by an independent pathology laboratory (Adelaide Pathology Partners, Adelaide, South Australia) and multi-locus sequence typing (MLST) was used to confirm taxonomic classification of P. aeruginosa (Fong et al., 2017). Growth curves were established for each strain to ascertain the log and stationary phases of planktonic bacterial growth in Luria Broth (LB) medium, grown shaking at 180 rpm at 37°C as described (Balda et al., 1993). Exoproteins of all strains were collected at log phase and stationary phase, sterilized by 0.22 μm filters and concentrated using Vivaspin protein concentrator spin columns with 3000 molecular weight cut-off (MWCO) (GE Healthcare Bio-Sciences, Pittsburgh, USA). Concentration of exoproteins was measured using NanoOrange™ Protein Quantitation Kit (Thermo Scientific, Walthman, MA, USA) according to manufacturer's instructions.
Trans Epithelial Electrical Resistance (TEER) Analysis
An EVOM voltohmmeter (World Precision Instruments, Sarasota, FL, USA) was used to measure TEER as described (Ramezanpour et al., 2016, 2017). Briefly, 100 μL of B-ALI growth medium was added to the apical chamber of ALI cultures to form an electrical circuit across the cell monolayer and into the basal chamber. Only wells displaying baseline resistance readings greater than 500 Ω/cm2 were used for the experiments. Exoproteins (50 μL exoproteins in LB medium and 50 μL B-ALI growth medium), purified P. aeruginosa elastase (0.5, 5, 25, and 50 μg/mL in B-ALI growth medium) (Sigma-Aldrich, St. Louis, MO, USA) and negative control (50 μL B-ALI growth medium/50 μL LB medium for exoprotein experiments and B-ALI growth medium for experiments with purified elastase) were added to the apical chamber of each well, and TEER measurements were obtained at time 0, 1, 2, 3, and 4 h for exoproteins and at time 0 and 24 h for the purified P. aeruginosa elastase. TEER values were normalized to the average TEER measurement prior to challenge. Between samples the probe was washed stepwise in 70% ethanol, PBS, then B-ALI medium to minimize cross-contamination between samples. Each well was measured 3 times via two ports on either side of the insert.
Dextran-FITC Permeability Assay
Paracellular permeability was studied by measuring the apical-to-basolateral flux of Dextran-FITC-4 kDa (Sigma-Aldrich). Briefly, after treating the cells for 4 h (exoproteins) or 24 h (purified P. aeruginosa elastase), the upper chambers were filled with 3 mg/mL of Dextran-FITC and incubated at 37°C for 2 h. Forty Microliter samples were recovered from the bottom chamber and serially diluted in a 96-well plate (Sigma-Aldrich), and the fluorescence was measured with a microplate fluorometer (FLUOstar Optima, BMG Labtech, Ortenberg, Germany).
Cell Cytotoxicity Assay
Exoproteins were collected from basal chambers of each sample following TEER measurements and the release of lactate dehydrogenase (LDH) was measured by using Cytotox Homogeneous Membrane Integrity Assay (Promega, Australia) as described (Ramezanpour et al., 2016).
Pseudomonas aeruginosa Elastase Activity Analysis
The elastase activity of all P. aeruginosa strains was determined using Elastin-Congo red (ECR; Sigma-Aldrich). Briefly, for each sample, 100 μL concentrated filter-sterilized bacterial exoprotein was added to 900 μL of ECR buffer (100 mM Tris-HCl, pH 7.5, 1 mM CaCl2, 20 mg ECR) and incubated for 18 h at 37°C with agitation at 200 rpm. Then insoluble ECR was removed by centrifugation at 16,000 g for 15 min at room temperature, and the absorbance was measured at 495 nm. Fresh LB medium was used as the blank control, and LB with ECR as the negative control. Secreted elastase activity was expressed as the ratio of the OD495 and OD600 absorbance value.
Immunofluorescence Staining
HNEC monolayers were fixed with 2.5% formalin in phosphate-buffered saline (PBS) for 15 min, washed three times with cold PBS and permeabilized in 100 μL 0.1% Triton X-100 in PBS. Cells were rinsed with Tris-buffered saline-0.5% Tween (TBST) four times and blocked with serum free blocker (SFB; Dako, Glostrup, Denmark) for 60 min, at room temperature. Mouse monoclonal anti-human ZO-1, diluted to 10 μg/mL in TBST-10% SFB, or Rabbit polyclonal anti-human Occludin, diluted to 2 μg/mL in TBST-10% SFB (both Invitrogen, Carlsbad, CA, USA), was added to the monolayers and allowed to incubate overnight at 4°C. Cells were washed 3 times with TBST followed by incubation with 2 μg/mL anti-mouse Cy3 or anti-rabbit Alexa-488 conjugated secondary antibody (Jackson ImmunoResearchLabs Inc., West Grove, PA, USA) for 1 h at room temperature. Cells were rinsed in TBST followed by adding 200 ng/mL of 4′,6-diamidino-2-phenylindole (DAPI, Sigma-Aldrich). Membranes were rinsed once with ultrapure water and 95% ice-cold ethanol was added for 1 h at 4°C. Membranes were transferred to a glass slide and a drop of anti-fade mounting medium (Dako) was added before cover-slipping. Samples were visualized using a LSM700 confocal laser scanning microscope (Zeiss Microscopy, Germany).
Western Blot of Tight Junction and Cytoskeleton Proteins
HNECs in 6-well plates were treated for 24 h with 5% (v/v) exoproteins from 2 strains at log phase (log) and stationary phase (sta). Luria-Bertani (LB) medium 5% (v/v) and B-ALI growth medium were used as negative controls. Western analysis was performed using the X Cell Sure Lock Mini Cell System M (Invitrogen) as previously described (Clark et al., 2011). Membranes were probed with Mouse monoclonal anti-human ZO-1 (1:125 dilution, Invitrogen), Rabbit polyclonal anti-human Occludin (3 μg/mL, Invitrogen) and mouse monoclonal anti-human β-actin antibodies (1:1,000; Sigma–Aldrich). Equal protein loading (10 μg) was assessed using the rabbit polyclonal anti-human Profilin-1 antibody (1:5,000 dilution; Invitrogen). Goat anti-rabbit IgG horseradish peroxidase (HRP) (Abcam) and Goat anti-mouse IgG HRP (Abnova, Taipei, Taiwan, China) were used as secondary antibodies. Luminescence was detected using the ImageQuant LAS-4000 biomolecular imager (Tokyo, Japan), and densitometry analyses performed using Multi-Gauge software (V3.0, Fugifilm Science Lab, Tokyo, Japan). Log-transformed density scores were normalized to both profilin-1 and the biological control.
Statistical Analysis
Data are presented as mean ± Standard Error of Mean (SEM). The TEER, permeability assay, cell cytotoxicity assay experiments were performed using 4 biological replicates from 3 independent donors. Elastase activity was measured 3 times in 3 independent experiments. Statistical analysis of all data was carried out using ANOVA, followed by Tukey honest significant difference (HSD) post-hoc test. The Spearman correlation analysis was performed between two variables. Spearman's rank correlation coefficient and P-value were calculated to describe the correlation result. These tests were performed using SPSS software (version 18) and GraphPad Prism 5 (version 5.01).
Results
Pseudomonas aeruginosa Exoproteins Reduce the Transepithelial Electrical Resistance (TEER) of HNEC-ALI Cultures
P. aeruginosa exoproteins contain virulence factors including secreted proteases that interact with the host during pathogenic infections and have a critical role in invasiveness (Janda and Bottone, 1981; Bleves et al., 2010). To test the effect of exoproteins on the mucosal barrier in vitro, primary HNEC-ALI cultures were established from 4 independent control patients without CRS or CF (two males, two females, aged 32–71 years) followed by measuring Trans Epithelial Electrical Resistance (TEER) and passage of Dextran-FITC. Exoproteins were collected from log and stationary growth phases of 4 P. aeruginosa strains [PAO1 reference strain and three CIs collected from the sinus cavities of CRS patients—one with CF (S.M.), two without], filtered and protein concentration measured (Supplementary Figure 1 and Supplementary Table 1). TEER was examined after application of exoproteins at different time points. Whilst only some exoproteins from log phase cultures affected TEER values after 2 h (one isolate) and 4 h (two isolates) of challenge, stationary phase exoproteins of all 4 strains significantly reduced the TEER values at 1 to 2 h after application. The stationary phase exoproteins of the G.R strain had the largest effect on TEER values, with a highly significant reduction of TEER after 1 h of application (2.73-fold reduction compared to the medium control, P = 0.0292). This further decreased to a 12.04-fold reduction after 4 h of application (P < 0.0001). Also, exponential phase exoproteins of the G.R strain significantly reduced TEER within 2 h of application (Figure 1A).
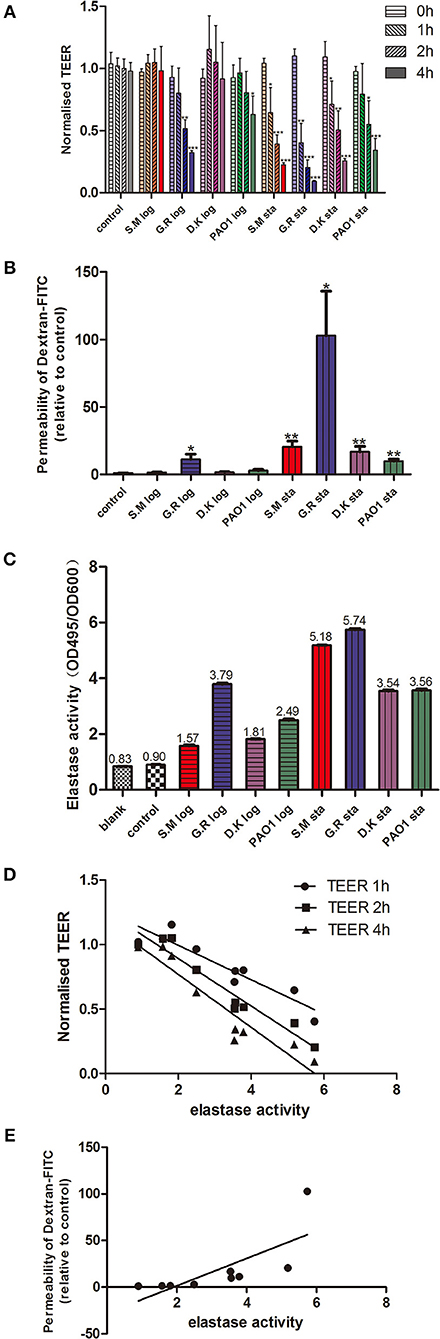
Figure 1. P. aeruginosa exoproteins cause mucosal barrier dysfunction in correlation with elastase activity. P. aeruginosa exoproteins collected from 3 clinical isolates (S.M.-red color in Figures 1A–C; G.R.-blue color in Figures 1A–C; D.K.-purple color in Figures 1A–C) and 1 reference strain (PAO1, green color in Figures 1A–C), at exponential phase (log) and stationary phase (sta) of growth were applied to HNEC-ALI cultures, followed by measuring Transepithelial Electrical Resistance (TEER) hourly for 4 h (A) and passage of Dextran-FITC 4 h after application (B). The elastase activity was measured by Elastin-Congo red (ECR) reaction and shown as OD495/OD600 ratio. Fresh LB medium was used as blank control (blank), and LB with ECR as a negative control (control) (C). The Spearman's rank correlation coefficient was determined between the elastase activity and TEER at different time points (D) or Dextran-FITC permeability (E). The values are shown as mean±SEM for n = 4. *p < 0.05, **p < 0.01, ***p < 0.001. ANOVA, followed by Tukey HSD post-hoc test.
Pseudomonas aeruginosa Exoproteins Increase the Paracellular Permeability of HNEC-ALI Cultures
TEER measures the flux of all ions across the epithelium and cannot be used to measure tight junction size (Shen et al., 2011). To assess this, we measured the flux of Dextran-FITC tracers across HNEC-ALI cultures. Exoproteins from all stationary phase cultures as well as from the CI G.R log phase caused significant enhancement of paracellular permeability within 4 h after application. Stationary phase exoproteins from the G.R strain had the strongest effect on permeability with a >100-fold increase of Dextran-FITC crossing the HNECs monolayer 6 h after application (Figure 1B).
Nasal Epithelial Layer Disruption Does Not Correlate With Cytotoxicity
To assess the contribution of cellular toxicity on the increased permeability of the HNEC-ALI cultures, the effect of P. aeruginosa exoproteins on cellular toxicity was determined by measuring LDH release from HNECs 4 h after application. There was no statistically significant change in LDH release after stimulation with P. aeruginosa exoproteins compared to control (Supplementary Figure 1B).
Purified P. aeruginosa Elastase Decreases HNEC Barrier Function in a Dose-Dependent Way
P. aeruginosa elastase plays an important role in the pathogenesis and invasion of P. aeruginosa infection and can cause a transient disruption of tight junctions of airway epithelial cells (Azghani et al., 2000; Cowell et al., 2003; Nomura et al., 2014). However, the effect of P. aeruginosa elastase on primary HNECs is not known. Application of purified P. aeruginosa elastase to HNEC-ALI cultures had a dose-dependent effect on mucosal barrier function with a significant reduction in TEER observed for 25 μg/mL (P = 0.0149) and 50 μg/mL (P = 0.0004). An increased paracellular permeability of Dextran-FITC was seen after application of 50 μg/mL purified P. aeruginosa elastase 24 h after application (P < 0.0001) (Supplementary Figure 2).
Elastase Activity Differs Amongst Clinical Pseudomonas aeruginosa Isolates
Given that clinical isolates might differ in their virulence factor production and activation, we next wanted to determine the elastase activity of different strains at different phases of growth. The P. aeruginosa elastase activity was measured by ECR reaction and expressed as the ratio of the OD495 and OD600 absorbance values. Different strains had different elastase activities for both exponential and stationary phase of growth. For all strains tested, the elastase activity from stationary phase was higher than from exponential phase (p < 0.0001). The highest elastase activity was from the G.R strain (Figure 1C).
Elastase Activity Significantly Correlates With Monolayer Permeability and TEER Values of Pseudomonas aeruginosa Clinical Isolates in vitro
To assess a potential relationship between the mucosal barrier function and elastase activity, the Spearman's rank correlation coefficient was determined between the elastase activity and TEER at different time points or Dextran-FITC permeability, respectively. There was a significant very strong positive correlation between elastase activity and Dextran-FITC permeability (r = 0.9500, p < 0.01) and a significant strong negative correlation between elastase activity and TEER after 1 h (r = −0.8667, P < 0.01), 2 h (r = -0.8833, P < 0.01) and 4 h (r = −0.9333, P < 0.01) challenge with P. aeruginosa exoproteins (Figures 1D,E and Table 1).
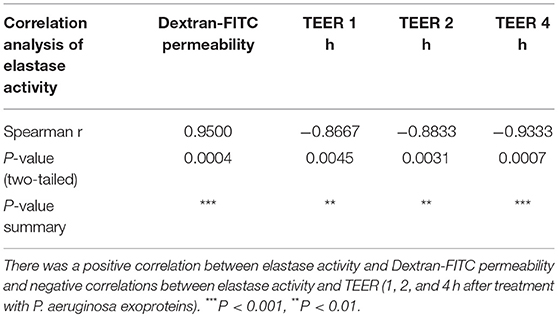
Table 1. Spearman correlation analysis of elastase activity with Dextran-FITC permeability and TEER at different time-points.
P. aeruginosa Exoproteins Cause Disruption and Degradation of Tight Junction Proteins and Cytoskeleton
The Apical Junctional Complex (AJC) contains tight and adherens junctions and maintains mucosal barrier integrity. To get insights into the potential mechanism of increased mucosal barrier permeability induced by application of the exoproteins, the localization of Zona Occludens-1 (ZO-1) and Occludin was examined by immunofluorescence staining and confocal laser scanning microscopy 6 h after application of P. aeruginosa exoproteins. In control cells, ZO-1 and Occludin were consistently located at the periphery of epithelial cells. ZO-1 and Occludin discontinuous immunolocalization was seen after challenge with P. aeruginosa exoproteins, particularly those from stationary phase cultures (Figure 2A). To investigate whether P. aeruginosa exoproteins affected ZO-1, Occludin and β-actin protein levels, western blots were performed after application of exoproteins from 2 CIs at log and stationary growth phase, and in relation to the elastase activity of those isolates. The exoproteins of the B.V strain had high elastase activity (mean elastase activity, 5.86 of B.V stationary phase, 2.93 of B.V log phase) and caused a complete degradation of ZO-1 (compared to control, P < 0.0001 for B.V log and B.V sta), a significant degradation of Occludin (compared to control, P = 0.0187 for B.V sta, P = 0.0713 for B.V log) and significant reduction in β-actin protein levels (compared to control, P = 0.0103 for B.V log, P = 0.0003 for B.V sta). The exoprotein of the M.P strain had a mean elastase activity of 2.17 for the stationary phase and 0.92 for the log phase, and also caused significant degradation of ZO-1 (compared to control, P < 0.0001 for M.P log, P = 0.0003 for M.P sta) and significantly decreased the protein level of β-actin (compared to control, P = 0.0338 for M.P log, P = 0.1427 for M.P sta) (Figure 2B).
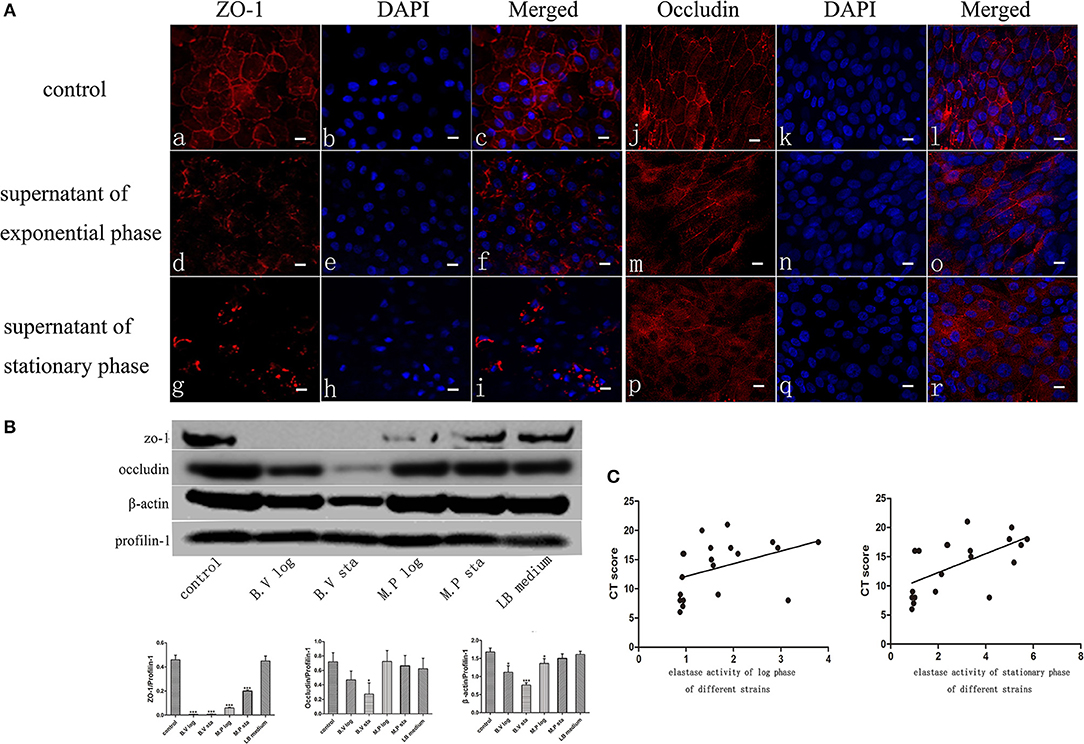
Figure 2. P. aeruginosa exoproteins cause disruption of ZO-1, Occludin and Actin of primary human nasal epithelial cells (HNECs). (A) The effect of P. aeruginosa B.V strain exoproteins at Log phase (d-f and m-o) and Stationary phase (g-i and p-r) on the localization of Zona Occludens-1 (ZO-1) (red staining with DAPI stain in blue in a-i) and Occludin (red staining with DAPI stain in blue in j-r) using immunofluorescence confocal laser scanning microscopy 6 h after application of the exoproteins. (B) Western blot analysis and relative densitometric quantification of Zona Occludens-1 (ZO-1), Occludin and β-actin after application of 5% (v/v) exoproteins of P. aeruginosa strains B.V and M.P for 24 h. HNEC monolayers without treatment were control group. Luria-Bertani (LB) medium was used as the negative control. Profilin-1 was used as the loading control. Densitometric quantification relative to Profilin-1. (C) Spearman correlation analysis of Lund-Mackay Computed Tomography (CT) scores with elastase activity of 20 P. aeruginosa clinical isolates at log phase or stationary phase of growth. Results are representative of three independent experiments. *p < 0.05, ***p < 0.001. ANOVA, followed by Tukey HSD post-hoc test.
Elastase Activity of P. aeruginosa Clinical Isolates Significantly Correlates With Disease Severity CT Scores of CRS Patients
To elucidate if elastase activity of CIs collected from the nasal cavity of CRS patients correlates with CRS disease severity, we randomly selected a further 18 P. aeruginosa clinical isolates. Phylogenetic analysis using MLST analysis was performed on 12 CIs and showed no significant relationship between the isolates (Supplementary Table 2) (Fong et al., 2017). Growth curves were established (Supplementary Figure 3A), exoproteins collected at log phase (12–13 h) and stationary phase (24–25 h) (see Supplementary Table 2 for OD values), filtered with Vivaspin 2 (3000 MWCO), followed by determination of protein concentration and elastase activity by ECR reaction. There was no significant increase in protein concentration between log phase and stationary phase of the same isolates. 10/18 and 11/18 isolates significantly increased their elastase activity compared to control in the Log and Stationary phase, respectively (Supplementary Figures 3B,C). There was a significant negative correlation between the elastase activity and exoprotein concentration of log phase and stationary phase cultures (r = –0.66, p < 0.0008 and r = –0.57, p < 0.005) (Supplementary Table 3, Supplementary Figure 4). Lund-Mackay Computed Tomography (CT) scores, Lund-Kennedy endoscopic scores, the 22-item Sinonasal Outcome Test (SNOT-22) score (all within 12 months of the swab being taken), and number of previous sinus surgeries were used to estimate CRS disease severity. Demographics and disease severity scores are shown in Supplementary Table 2. Spearman correlation analysis showed there was no correlation between elastase activity and subjective evaluation scores (VAS and SNOT-22 scores), Lund-Kennedy endoscopic score, or number of previous sinus surgeries (data not shown). However, there was a strong significant correlation between Lund-Mackay CT scores and elastase activity of the isolates at log phase (r = 0.5631, P < 0.01) and stationary phase (r = 0.6689, P < 0.01) (Figure 2C, Table 2).
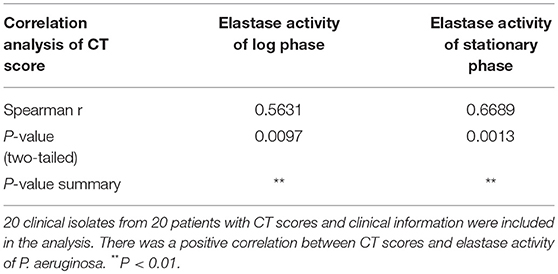
Table 2. Spearman correlation analysis of Lund-Mackay Computed Tomography (CT) scores with elastase activity at log phase or stationary phase.
Discussion
Our study showed a differential ability of P. aeruginosa clinical isolates exoproteins to disrupt mucosal barrier structure and function in a manner that directly correlated with the elastase activity of those isolates. Strikingly, in vitro P. aeruginosa elastase activity strongly correlated with CT-scan scores of disease severity in patients with CRS. In addition, dose-dependent detrimental effects on the HNEC-ALI mucosal barrier function were observed after application of purified P. aeruginosa elastase. Our findings contribute significant insights into the pathophysiology of CRS and implicate P. aeruginosa elastase or exoproteins secreted in conjunction with elastase as correlating with P. aeruginosa-associated CRS disease severity. Not only does it support the notion that barrier dysfunction may play a key role in chronic inflammation and polyp formation in CRS patients, it implicates P. aeruginosa exoproteins as major contributing factors in that process implicating these exoproteins as potentially important therapeutic targets.
Whilst our results indicate elastase as potentially directly contributing to the mucosal barrier disruption observed, it is known that the secretion of P. aeruginosa elastase is a highly coordinated process regulated by the las and rhl quorum sensing (QS) systems regulating the expression of over 50 genes (Whiteley et al., 1999; Nouwens et al., 2003). Further studies are required to elucidate the exact role and contribution of elastase in this process, by specifically blocking the P. aeruginosa elastase activity and/or constructing and characterizing P. aeruginosa LasB and LasA mutants.
In this study, 61% (14/20) of the P. aeruginosa clinical isolates harvested from within the sinonasal cavities demonstrated significant elastase activity. This is low given that 100% of clinical P. aeruginosa isolates are reported to carry the LasB gene and can produce elastase (Finnan et al., 2004). Whilst the molecular basis for this finding remains to be defined, mutations in LasR, the transcriptional regulator of the LasB gene, have been shown to reduce P. aeruginosa elastase production (Pearson et al., 1997) and are associated with lung function decline and altered sensitivity to antibiotics in patients with CF (Hoffman et al., 2009).
Nomura et al has previously shown that purified P. aeruginosa elastase could disrupt the epithelial barrier in a transient manner (Nomura et al., 2014), however the effect was slower and less profound compared to the effects observed in this study. This could be due to reduced functional properties of the recombinant purified elastase and/or differences in concentration used. Also, Nomura et al. used HNECs transfected with the human catalytic subunit of the telomerase reverse transcriptase (hTERT) and it is unclear how that might affect the elastase-induced effect on the barrier.
Details of the mechanistic effects of P. aeruginosa exoproteins against the epithelial barrier remain to be explored but this study indicates an extensive disruption of both cytoskeleton and apical junctional complex (AJC) proteins. This might indicate a disruption of common gene regulatory elements and/or signal transduction pathways as has previously been demonstrated for P. aeruginosa elastase (Nomura et al., 2014). The AJC comprises tight and adherens junctions and maintains epithelial cell polarity and mucosal barrier integrity. The AJC includes the transmembrane proteins ZO-1, occludin, Junction Adhesion Molecule 1 (JAM-A), claudin family members, and linker proteins that bind the underlying actin cytoskeleton. Disruption and re-organization of AJC proteins is a hallmark of mucosal barrier dysfunction. It is frequently found in severe chronic inflammatory diseases of the gut, skin, and airway such as inflammatory bowel disease, allergic dermatitis, and asthma (Weidinger et al., 2008; Turner, 2009). In the context of CRS, bacterial secreted products as well as different cytokines have been reported to disrupt the mucosal barrier in vitro, and barrier dysfunction has been proposed to have a critical role in the pathogenesis of this disease (Tieu et al., 2009; Soyka et al., 2012; Malik et al., 2015; Ramezanpour et al., 2016; Murphy et al., 2017). Results of previous studies with primary alveolar pneumocytes indicated that P. aeruginosa elastase enhanced epithelial permeability by redistribution and disruption of tight junction proteins (Azghani et al., 2000). Likewise, using HNECs, our results indicated that P. aeruginosa secreted products induced a severe disruption of the barrier function and degraded the TJ and cytoskeleton proteins ZO-1, occludin and β-actin. Strikingly, the extent of barrier dysfunction strongly correlated with the elastase activity of those products. P. aeruginosa elastase has been shown to have a highly efficient proteolytic activity on a range of host proteins, facilitating tissue invasion and contributing to airway pathology such as lung fibrosis and vasculitis in cystic fibrosis patients (Schultz and Miller, 1974; Bruce et al., 1985). P. aeruginosa elastase also contributes to P. aeruginosa's ability to evade and disarm the host immune system by degrading proteins of the human immune defense system (Schultz and Miller, 1974; Bainbridge and Fick, 1989; Heck et al., 1990; Mariencheck et al., 2003; Alcorn and Wright, 2004). Taken together, the concerted action of exoproteins such as P. aeruginosa elastase in increasing mucosal permeability and degrading innate immune defense mechanisms could lead to an ongoing, but ineffective, immune response, which contributes to the severe inflammation and polyp formation in P. aeruginosa-associated CRS patients.
Author Contributions
All authors agree to be accountable for all aspects of the work and gave their final approval of the version to be published. In addition, JL, MR, SF, and CC had substantial contributions to the acquisition and analysis of data and drafting the work. JM, MS, AP, and PW had substantial contributions to the design of the work and critical revision. SV had substantial contribution to the conception and design of the work, drafting of the work and critical revision.
Conflict of Interest Statement
The authors declare that the research was conducted in the absence of any commercial or financial relationships that could be construed as a potential conflict of interest.
Supplementary Material
The Supplementary Material for this article can be found online at: https://www.frontiersin.org/articles/10.3389/fcimb.2019.00038/full#supplementary-material
Supplementary Figure 1. Growth curves of P. aeruginosa and Lactate Dehyrogenase (LDH) assays. Growth curves from 3 P. aeruginosa clinical isolates (S.M., G.R., D.K.) and from PAO1 were established and exoproteins collected at 11–12 h (exponential phase, log) and 22–23 h (stationary phase, sta) of growth (A) followed by application to HNEC-ALI cultures and measuring cell viability by LDH assay 4 h after stimulation, calculated relative to control HNECs (B). The values are shown as mean ± SEM for n = 4. ANOVA, followed by Tukey HSD post hoc test.
Supplementary Figure 2. Purified P. aeruginosa elastase disrupts the barrier function of HNECs. Different concentrations of purified P. aeruginosa elastase (0.5, 5, 25, and 50 μg/mL in B-ALI medium) were added onto HNEC-ALI cultures for 24 h followed by measuring TEER (A) and paracellular permeability (B). B-ALI medium was used as control. The values are shown as mean ± SEM for n = 4. *p < 0.05, **p < 0.01, ***p < 0.001. ANOVA, followed by Tukey HSD post hoc test.
Supplementary Figure 3. Growth curves and elastase activity of 18 P. aeruginosa CRS clinical isolates. Cell number was estimated by measuring OD600 and exoproteins collected at 12–13 h as exponential phase and 24–25 h as stationary phase (A). Elastase activity of log phase (B) and stationary phase (C) was shown as OD495/OD600 ratio. The values are shown as mean ± SEM for n = 3. Acronyms refer to different strains of clinical isolates. Fresh LB medium was used as the blank control, and LB with ECR as the negative control.
Supplementary Figure 4. Spearman correlation analysis of elastase activity with exoprotein concentration of log phase and stationary phase cultures of 22 strains. Twenty-one clinical isolates from 21 patients and 1 PAO1 reference strain was included in the analysis. There were negative correlations between elastase activity and exoprotein concentration of log phase and stationary phase cultures.
Supplementary Table 1. Elastase activity and protein concentrations for PAO1 and clinical isolates.
Supplementary Table 2. Demographics, disease severity scores, elastase activity and multi-locus sequence typing (MLST) analysis of P. aeruginosa clinical isolates.
Supplementary Table 3. Spearman correlation analysis of elastase activity with exoprotein concentration of log phase and stationary phase of 22 strains. There were negative correlations between elastase activity and exoprotein concentration of log phase and stationary phase. ***P < 0.001, **P < 0.01.
References
Alcorn, J. F., and Wright, J. R. (2004). Degradation of pulmonary surfactant protein D by Pseudomonas aeruginosa elastase abrogates innate immune function. J. Biol. Chem. 279, 30871–30879. doi: 10.1074/jbc.M400796200
Azghani, A. O., Miller, E. J., and Peterson, B. T. (2000). Virulence factors from Pseudomonas aeruginosa increase lung epithelial permeability. Lung 178, 261–269. doi: 10.1007/s004080000031
Bainbridge, T., and Fick, R. B. Jr. (1989). Functional importance of cystic fibrosis immunoglobulin G fragments generated by Pseudomonas aeruginosa elastase. J. Lab. Clin. Med. 114, 728–733.
Balda, M. S., Gonzalez-Mariscal, L., Matter, K., Cereijido, M., and Anderson, J. M. (1993). Assembly of the tight junction: the role of diacylglycerol. J. Cell Biol. 123, 293–302. doi: 10.1083/jcb.123.2.293
Bleves, S., Viarre, V., Salacha, R., Michel, G. P., Filloux, A., and Voulhoux, R. (2010). Protein secretion systems in Pseudomonas aeruginosa: a wealth of pathogenic weapons. Int. J. Med. Microbiol. 300, 534–543. doi: 10.1016/j.ijmm.2010.08.005
Bruce, M. C., Poncz, L., Klinger, J. D., Stern, R. C., Tomashefski, J. F. Jr., and Dearborn, D. G. (1985). Biochemical and pathologic evidence for proteolytic destruction of lung connective tissue in cystic fibrosis. Am. Rev. Respir. Dis. 132, 529–535.
Clark, C. A., Thomas, L. K., and Azghani, A. O. (2011). Inhibition of protein kinase C attenuates Pseudomonas aeruginosa elastase-induced epithelial barrier disruption. Am. J. Respir. Cell Mol. Biol. 45, 1263–1271. doi: 10.1165/rcmb.2010-0459OC
Cleland, E. J., Bassiouni, A., Vreugde, S., and Wormald, P. J. (2016). The bacterial microbiome in chronic rhinosinusitis: richness, diversity, postoperative changes, and patient outcomes. Am. J. Rhinol. Allergy 30, 37–43. doi: 10.2500/ajra.2016.30.4261
Coggan, K. A., and Wolfgang, M. C. (2012). Global regulatory pathways and cross-talk control Pseudomonas aeruginosa environmental lifestyle and virulence phenotype. Curr. Issues Mol. Biol. 14, 47–70. doi: 10.21775/cimb.014.047
Cowell, B. A., Twining, S. S., Hobden, J. A., Kwong, M. S., and Fleiszig, S. M. (2003). Mutation of lasA and lasB reduces Pseudomonas aeruginosa invasion of epithelial cells. Microbiology 149, 2291–2299. doi: 10.1099/mic.0.26280-0
Coyne, C. B., Vanhook, M. K., Gambling, T. M., Carson, J. L., Boucher, R. C., and Johnson, L. G. (2002). Regulation of airway tight junctions by proinflammatory cytokines. Mol. Biol. Cell 13, 3218–3234. doi: 10.1091/mbc.e02-03-0134
Filkins, L. M., Hampton, T. H., Gifford, A. H., Gross, M. J., Hogan, D. A., Sogin, M. L., et al. (2012). Prevalence of streptococci and increased polymicrobial diversity associated with cystic fibrosis patient stability. J. Bacteriol. 194, 4709–4717. doi: 10.1128/JB.00566-12
Finnan, S., Morrissey, J. P., O'Gara, F., and Boyd, E. F. (2004). Genome diversity of Pseudomonas aeruginosa isolates from cystic fibrosis patients and the hospital environment. J. Clin. Microbiol. 42, 5783–5792. doi: 10.1128/JCM.42.12.5783-5792.2004
Fong, S. A., Drilling, A., Morales, S., Cornet, M. E., Woodworth, B. A., Fokkens, W. J., et al. (2017). Activity of bacteriophages in removing biofilms of pseudomonas aeruginosa isolates from chronic rhinosinusitis patients. Front. Cell. Infect. Microbiol. 7:418. doi: 10.3389/fcimb.2017.00418
Heck, L. W., Alarcon, P. G., Kulhavy, R. M., Morihara, K., Russell, M. W., and Mestecky, J. F. (1990). Degradation of IgA proteins by Pseudomonas aeruginosa elastase. J. Immunol. 144, 2253–2257.
Hoffman, L. R., Kulasekara, H. D., Emerson, J., Houston, L. S., Burns, J. L., Ramsey, B. W., et al. (2009). Pseudomonas aeruginosa lasR mutants are associated with cystic fibrosis lung disease progression. J. Cyst Fibros. 8, 66–70. doi: 10.1016/j.jcf.2008.09.006
Janda, J. M., and Bottone, E. J. (1981). Pseudomonas aeruginosa enzyme profiling: predictor of potential invasiveness and use as an epidemiological tool. J. Clin. Microbiol. 14, 55–60.
Kobayashi, H., Kobayashi, O., and Kawai, S. (2009). Pathogenesis and clinical manifestations of chronic colonization by Pseudomonas aeruginosa and its biofilms in the airway tract. J. Infect. Chemother. 15, 125–142. doi: 10.1007/s10156-008-0691-3
Malik, Z., Roscioli, E., Murphy, J., Ou, J., Bassiouni, A., Wormald, P. J., et al. (2015). Staphylococcus aureus impairs the airway epithelial barrier in vitro. Int. Forum Allergy Rhinol. 5, 551–556. doi: 10.1002/alr.21517
Mariencheck, W. I., Alcorn, J. F., Palmer, S. M., and Wright, J. R. (2003). Pseudomonas aeruginosa elastase degrades surfactant proteins A and D. Am. J. Respir. Cell Mol. Biol. 28, 528–537. doi: 10.1165/rcmb.2002-0141OC
Marvig, R. L., Sommer, L. M., Molin, S., and Johansen, H. K. (2015). Convergent evolution and adaptation of Pseudomonas aeruginosa within patients with cystic fibrosis. Nat. Genet. 47, 57–64. doi: 10.1038/ng.3148
Murphy, J., Ramezanpour, M., Stach, N., Dubin, G., Psaltis, A. J., Wormald, P. J., et al. (2017). Staphylococcus aureus V8 protease disrupts the integrity of the airway epithelial barrier and impairs IL-6 production in vitro. Laryngoscope 128, E8–E15. doi: 10.1002/lary.26949
Nomura, K., Obata, K., Keira, T., Miyata, R., Hirakawa, S., Takano, K., et al. (2014). Pseudomonas aeruginosa elastase causes transient disruption of tight junctions and downregulation of PAR-2 in human nasal epithelial cells. Respir. Res. 15:21. doi: 10.1186/1465-9921-15-21
Nouwens, A. S., Beatson, S. A., Whitchurch, C. B., Walsh, B. J., Schweizer, H. P., Mattick, J. S., et al. (2003). Proteome analysis of extracellular proteins regulated by the las and rhl quorum sensing systems in Pseudomonas aeruginosa PAO1. Microbiology 149, 1311–1322. doi: 10.1099/mic.0.25967-0
Pearson, J. P., Pesci, E. C., and Iglewski, B. H. (1997). Roles of Pseudomonas aeruginosa las and rhl quorum-sensing systems in controlof elastase and rhamnolipid biosynthesis genes. J. Bacteriol. 179, 5756–5767. doi: 10.1128/jb.179.18.5756-5767.1997
Peters, J. E., and Galloway, D. R. (1990). Purification and characterization of an active fragment of the LasA protein from Pseudomonas aeruginosa: enhancement of elastase activity. J. Bacteriol. 172, 2236–2240. doi: 10.1128/jb.172.5.2236-2240.1990
Ramezanpour, M., Moraitis, S., Smith, J. L., Wormald, P. J., and Vreugde, S. (2016). Th17 cytokines disrupt the airway mucosal barrier in chronic rhinosinusitis. Mediators Inflamm. 2016:9798206. doi: 10.1155/2016/9798206
Ramezanpour, M., Rayan, A., Smith, J. L. P., and Vreugde, S. (2017). The effect of topical treatments for CRS on the sinonasal epithelial barrier. Rhinology 55, 161–169. doi: 10.4193/Rhin16.284
Schultz, D. R., and Miller, K. D. (1974). Elastase of Pseudomonas aeruginosa: inactivation of complement components and complement-derived chemotactic and phagocytic factors. Infect. Immun. 10, 128–135.
Shen, L., Weber, C. R., Raleigh, D. R., Yu, D., and Turner, J. R. (2011). Tight junction pore and leak pathways: a dynamic duo. Annu. Rev. Physiol. 73, 283–309. doi: 10.1146/annurev-physiol-012110-142150
Smith, R. S., and Iglewski, B. H. (2003). P. aeruginosa quorum-sensing systems and virulence. Curr. Opin. Microbiol. 6, 56–60. doi: 10.1016/S1369-5274(03)00008-0
Soyka, M. B., Wawrzyniak, P., Eiwegger, T., Holzmann, D., Treis, A., Wanke, K., et al. (2012). Defective epithelial barrier in chronic rhinosinusitis: the regulation of tight junctions by IFN-gamma and IL-4. J. Allergy Clin. Immunol. 130, 1087–1096e1010. doi: 10.1016/j.jaci.2012.05.052
Tieu, D. D., Kern, R. C., and Schleimer, R. P. (2009). Alterations in epithelial barrier function and host defense responses in chronic rhinosinusitis. J. Allergy Clin. Immunol. 124, 37–42. doi: 10.1016/j.jaci.2009.04.045
Turner, J. R. (2009). Intestinal mucosal barrier function in health and disease. Nat. Rev. Immunol. 9, 799–809. doi: 10.1038/nri2653
Weidinger, S., O'Sullivan, M., Illig, T., Baurecht, H., Depner, M., Rodriguez, E., et al. (2008). Filaggrin mutations, atopic eczema, hay fever, and asthma in children. J. Allergy Clin. Immunol. 121, 1203–1209e1201. doi: 10.1016/j.jaci.2008.02.014
Whiteley, M., Lee, K. M., and Greenberg, E. P. (1999). Identification of genes controlled by quorum sensing in Pseudomonas aeruginosa. Proc. Natl. Acad. Sci. U.S.A. 96, 13904–13909. doi: 10.1073/pnas.96.24.13904
Keywords: chronic rhinosinusitis, CT-score, elastase, mucosal barrier, Pseudomonas aeruginosa
Citation: Li J, Ramezanpour M, Fong SA, Cooksley C, Murphy J, Suzuki M, Psaltis AJ, Wormald PJ and Vreugde S (2019) Pseudomonas aeruginosa Exoprotein-Induced Barrier Disruption Correlates With Elastase Activity and Marks Chronic Rhinosinusitis Severity. Front. Cell. Infect. Microbiol. 9:38. doi: 10.3389/fcimb.2019.00038
Received: 25 September 2018; Accepted: 07 February 2019;
Published: 27 February 2019.
Edited by:
Rong Fang, The University of Texas Medical Branch at Galveston, United StatesReviewed by:
Carlos Juan, Instituto de Investigación Sanitaria de Palma (IdISPa), SpainGyanendra Prakash Dubey, Institut Pasteur, France
Copyright © 2019 Li, Ramezanpour, Fong, Cooksley, Murphy, Suzuki, Psaltis, Wormald and Vreugde. This is an open-access article distributed under the terms of the Creative Commons Attribution License (CC BY). The use, distribution or reproduction in other forums is permitted, provided the original author(s) and the copyright owner(s) are credited and that the original publication in this journal is cited, in accordance with accepted academic practice. No use, distribution or reproduction is permitted which does not comply with these terms.
*Correspondence: Sarah Vreugde, sarah.vreugde@adelaide.edu.au