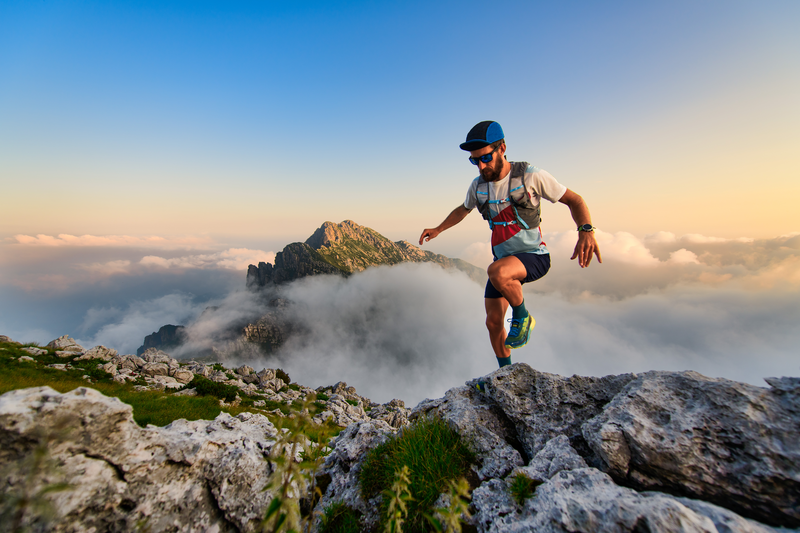
94% of researchers rate our articles as excellent or good
Learn more about the work of our research integrity team to safeguard the quality of each article we publish.
Find out more
ORIGINAL RESEARCH article
Front. Cell. Infect. Microbiol. , 04 January 2019
Sec. Clinical Microbiology
Volume 8 - 2018 | https://doi.org/10.3389/fcimb.2018.00445
Coumarin (1,2-benzopyrone), an aromatic oxygen-containing heterocyclic compound, has various biological functions. Previous studies have demonstrated that coumarin and its derivatives exhibit antifungal activity against Candida albicans. In this study, we investigated the exact mechanism by which coumarin works against this fungus using Annexin V-FITC/PI double staining, TUNEL assay, and DAPI staining, and found that it induced a series of apoptotic features, including phosphatidylserine (PS) externalization, DNA fragmentation, and nuclear condensation. Moreover, it also induced cytochrome c release from the mitochondria to the cytoplasm and metacaspase activation. Further study revealed that intracellular reactive oxygen species (ROS) levels were increased and mitochondrial functions, such as mitochondrial membrane potential and mitochondrial morphology, were altered after treatment with coumarin. Cytosolic and mitochondrial Ca2+ levels were also found to be elevated. However, pretreatment with ruthenium red (RR), a known mitochondrial Ca2+ channel inhibitor, attenuated coumarin-mediated DNA fragmentation and metacaspase activity, indicating that the coumarin-induced C. albicans apoptosis is associated with mitochondrial Ca2+ influx. Finally, coumarin was found to be low-toxic and effective in prolonging the survival of C. albicans-infected mice. This study highlights the antifungal activity and mechanism of coumarin against C. albicans and provides a potential treatment strategy for C. albicans infection.
Candida albicans is a commensal fungus that normally inhabits the human gastrointestinal tract and skin. However, in individuals who are immunocompromised due to AIDS or cancer chemotherapy, C. albicans can cause severe mucosal infections as well as fatal invasive infections (Ganguly and Mitchell, 2011). In the USA, fungal bloodstream infections caused by Candida spp. rank fourth among the nosocomial infections with considerable mortality rates (Edmond et al., 1999; Wisplinghoff et al., 2004). The presently used antifungal drugs mainly include azoles, polyenes, allylamines, echinocandins, and 5-fluorocytosine. With the large-scale application of broad-spectrum antifungal agents, the prevalence of opportunistic pathogen infections has gradually increased. Therefore, exploring novel and more effective antifungals is required to cope with lethal candidiasis.
Natural products, particularly traditional Chinese medicine, provide a rich pool for drug discovery due to the therapeutic efficacy and versatile structures of their secondary metabolites. Coumarin (1,2-benzopyrone) is an aromatic oxygen-containing heterocyclic compound containing benzopane α-pyranone structures that can be isolated from Solanaceae, Rutaceae, Umbelliferae, and other plants, or totally synthesized in the laboratory. Previous studies have demonstrated that coumarin and its derivatives have a variety of biological activities, including anticoagulant, antibacterial, and antiviral activities (Jung and Park, 2009). Additionally, coumarin complexes inhibit soil-borne fungal pathogens, plant fungal pathogens, and human fungal pathogens such as C. albicans, C. tropicalis, and Aspergillus fumigatus (Kumar et al., 2012; Hu et al., 2017). Although exposure to coumarin for 24 h reportedly alters the cell membrane and cell wall, and decreases the cytoplasmic volume with structural disorganization in C. albicans, the exact mechanism of action of coumarin (1,2-benzopyrone) has yet to be delineated (Widodo et al., 2012). Previous studies have reported that the silver (I)-coumarin complexes exert fungicidal activity against C. albicans by disrupting cytochrome synthesis, which then leads to respiration inhibition and reduced ergosterol biosynthesis. Moreover, a loss of cytochrome c synthesis (or depletion of its levels within the cells) also results in apoptosis. However, the inhibition of cytochrome synthesis by silver (I)-coumarin complexes is mainly attributed to silver addition (Thati et al., 2007). Previous studies have also demonstrated that coumarin or its derivatives exert anti-cancer activity, which is associated with apoptosis (Chuang et al., 2007; Álvarez-Delgado et al., 2009; Musa et al., 2012). However, it is still unknown whether this type of coumarin (i.e., 1,2-benzopyrone) exerts its antifungal activity against C. albicans via apoptosis.
Apoptosis is a form of programmed cell death. In addition to multicellular organisms, unicellular organisms, such as yeast, are also able to undergo programmed cell death that exhibits many hallmarks of apoptosis, including phosphatidylserine (PS) externalization, DNA fragmentation, metacaspase activation, reactive oxygen species (ROS) accumulation, loss of mitochondrial membrane potential, and elevations in cytosolic and mitochondrial Ca2+ (Leadsham et al., 2010). In this study, a variety of the aforementioned apoptosis markers were measured in C. albicans cells after treatment with this compound to investigate the antifungal mechanism of coumarin (1,2-benzopyrone) against C. albicans.
Coumarin (1,2-benzopyrone, >99%; CAS-No. 91-64-5) used in this study was purchased from Sangon Biotech. (Shanghai, China). A stock solution of coumarin (100 mg/mL) was prepared in dimethyl sulfoxide (DMSO) and then diluted in culture medium to obtain the concentrations to be tested (keeping the final concentration of DMSO at no more than 2%).
C. albicans SC5314 (ATCC MYA-2876) was obtained from the American Type Culture Collection (ATCC). The fungal strain cultures were routinely maintained in YPD medium (1% yeast extract, 2% peptone and 2% dextrose) at 30°C.
C. albicans suspensions (1 × 106 cells/mL) containing various concentrations of coumarin were dispensed into 96-well microtiter plates at a volume of 0.1 mL/well. After 48 h of incubation at 30°C, the minimal inhibitory concentration (MIC), which is the lowest concentration of the compound that prevents visible growth of fungus, was determined (Tian et al., 2017a,b; Yun and Lee, 2017). Each test was performed in triplicate.
C. albicans cells (1 × 107 cells/mL) containing three concentrations of coumarin (0.5, 1.0, and 2.0 mg/mL) were dispensed into 96-well microtiter plates at a volume of 0.1 mL/well. After 24 h and 48 h of incubation at 30°C, the optical density at 600 nm (OD600) was measured.
After incubation with coumarin (0.5, 1.0, and 2.0 mg/mL) for 4 h, the cultures were acquired and spread onto YPD agar plates. Colony-forming units (CFUs) were counted after incubation for 24 h at 30°C. The percentage survival was determined relative to the untreated cells. All experiments were performed three times independently.
Overnight cultures were refreshed in YPD medium to mid-exponential phase, then treated with 0.5, 1.0, and 2.0 mg/mL coumarin for 4 h. After that, the cells were harvested. To prepare protoplasts, C. albicans cells were incubated at 35°C for 20 min in 0.02 mg/mL Zymolyase 20T in 0.1 M potassium phosphate buffer (PPB, 50 mM K2HPO4, 5 mM EDTA, 50 mM DTT, 50 mM KH2PO4, and 40 mM β-mercaptoethanol) with 2.4 M sorbitol and at pH7.2. Thereafter, 100 μL permeabilization solution (0.1 M sodium citrate (pH6.0) with 0.1% Triton X-100) was added to the washed protoplasts, which were then plated on ice for 2 min and washed again. Next, the AnnexinV-FITC apoptosis detection kit was used according to the manufacturer's instructions. The stained cells were analyzed by flow cytometry.
DNA fragmentation and condensation were examined using TUNEL and DAPI staining (Park and Lee, 2010). The treated cells were washed twice with phosphate-buffered saline (PBS), then fixed in 3.6% paraformaldehyde for 30 min, and permeabilized on ice for 2 min. After the cells were washed again with PBS, they were stained using an in situ cell death detection kit for 1 h at 37°C and assessed by flow cytometer. Nuclear fragmentation and condensation were examined with DAPI staining. After incubation with coumarin for 4 h, the cells were harvested, washed and resuspended in PBS, stained with 1 μg/mL DAPI in the dark for 20 min, then washed three times with PBS and examined by fluorescence microscopy.
Cytochrome c levels were examined as described previously with minor modification (Choi and Lee, 2015; Yun and Lee, 2016). The treated C. albicans was harvested and prepared for protoplasts according to the abovementioned procedures. After that, the protoplasts were homogenized in buffer A (50 mM Tris, 2 mM EDTA, 1 mM phenylmethylsulfonyl fluoride, pH7.5), and the supernatant was collected. To analyze cytosolic and mitochondrial cytochrome c levels, the obtained supernatant was centrifuged at 50,000 rpm for 45 min. The released cytoplasmic cytochrome c was measured in the supernatant. To get pure mitochondria, the pellet was suspended in buffer B (50 mM Tris, 2 mM EDTA, pH 5.0). After treatment with 500 mg/mL ascorbic acid for 5 min, the cytochrome c content in the cytoplasmic or mitochondrial samples was determined by spectrophotometric analysis at 550 nm. The protein content was quantified by the Bradford method.
Metacaspase activation was examined using the CaspACE FITC-VAD-FMK in situ marker (Promega) (Tian et al., 2017b). The treated C. albicans cells were centrifuged, washed and then stained with 10 μM CaspACE FITC-VAD-FMK at 30°C for 30 min in the dark. After that, the cells were washed again and examined using flow cytometry.
To detect the production and accumulation of ROS, C. albicans cells were analyzed using 2′, 7′- dichlorofluorescein diacetate (DCFH-DA) (Jia et al., 2014). Cells exposed to coumarin for 4 h were harvested by centrifugation, washed once with YPD, and then resuspended in 0.5 mL YPD. DCFH-DA was added at a final concentration of 10 μM and incubated at 30°C for 30 min in the dark. The fluorescence intensities of resuspended cells were determined via flow cytometry.
5,5′,6,6′-Tetrachloro-1,1′,3,3′-tetraethyl-benzimidazolyl carbocyanine iodide (JC-1; Molecular Probes) was used to examine changes in mitochondrial membrane potential (Choi and Lee, 2015). C. albicans cells treated with coumarin for 4 h were washed, and stained with 2.5 μg/mL JC-1 for 20 min in the dark. After washed in PBS, the cells were analyzed by flow cytometry. The ratio of the fluorescence intensities of aggregates JC-1 (FL2) to monomer (FL1) was calculated.
The effects of coumarin on cytosolic and mitochondrial calcium were tested using Fluo-3 AM and Rhod-2 AM, respectively (Tian et al., 2017b). Briefly, the treated C. albicans cells were harvested and washed twice with HBSS buffer, and then resuspended in 500 μL HBSS buffer. For cytosolic calcium measurement, Fluo-3 AM (prepared in 0.1% Pluroinc F-127 solution in DMSO) was added to a final concentration of 2 μM. After incubation at 30°C for 40 min in the dark, the cells were washed once, resuspended in 600 μL HBSS and incubated at 30°C for further 20 min. For mitochondrial calcium measurement, Rhod-2 AM (prepared in 0.05% Pluroinc F-127 solution in DMSO) was added to a final concentration of 4 μM, and incubated at 37°C for 30 min in darkness. The fluorescence densities of Fluo-3 AM (excitation = 480 nm, emission = 526 nm), and Rhod-2 AM (excitation = 550 nm, emission = 580 nm) were immediately measured using flow cytometry.
To demonstrate whether coumarin was capable of clearing C. albicans infection, C57BL/6 mice were infected via the tail vein with 100 μL of a 5 × 106 C. albicans cells/mL normal saline suspension, and then randomly placed into four groups: normal saline group, DMSO group, 20 mg/kg coumarin group, and 40 mg/kg coumarin group. After 1 h infection, 100 μL of normal saline, DMSO, 20 mg/kg coumarin and 40 mg/kg coumarin were, respectively, administered by oral-gastric (OG) gavage, and then repeated on day 2 and day 3. Six mice were used for each group. The survival of mice was evaluated on day 10. To evaluate the toxicity of coumarin, male C57BL/6 mice aged 6–8 weeks were administrated by OG gavage with coumarin at doses of 20 and 40 mg/kg body weight once a day for 3 days. Each group included six mice. Survival was monitored for 10 days. The Kaplan–Meier log-rank test was used to determine the statistical differences between groups.
Every experiment was independently conducted at least three times, and data were presented as mean ± SD. The results were analyzed by SPSS software, using Duncan's Multiple Range test following one-way ANOVA. The statistically significant P values were noted with asterisks (* P < 0.05, ** P < 0.01, and *** P < 0.001).
The antifungal activity of coumarin against C. albicans was studied (Figure S2). The MIC for the SC5314 strain was 2.0 mg/mL. To determine the effect of coumarin on strain growth, OD600 of the strain was measured after treatment with 0.5, 1.0, and 2.0 mg/mL coumarin for 24 and 48 h. The results showed that the growth of the strains was significantly inhibited by coumarin in a dose-dependent manner (Figure 1A). To evaluate whether the decreased growth was related to cell death, the survival of the strains was examined after pretreatment with coumarin for 4 h. The CFUs assays showed that the survival rate was remarkably lowered by coumarin (Figure 1B). Taken together, these results indicated that the growth inhibition by coumarin was associated with reduced cell survival.
Figure 1. Effects of coumarin on growth and viability of Candida albicans. (A) The OD600 value was measured after treatment with coumarin for 24 h and 48 h. (B) Cell viability was assessed by counting CFUs after treatment with different concentrations of coumarin for 4 h at 30°C. Data were shown as mean ± SD. *P < 0.05 and **P < 0.01.
The inhibition of yeast proliferation and cell survival might result from induced yeast apoptosis (Almeida et al., 2008). In normal cells, phosphatidylserine (PS) exists in the inner leaflet of the plasma membrane. However, PS is exposed on the outer leaflet in apoptotic and necrotic cells, which is assumed to be an early marker of apoptosis in fungi (Choi and Lee, 2015; Tian et al., 2017a). Here, the C. albicans cells treated with coumarin were observed using AnnexinV-FITC and PI co-staining (Vermes et al., 2000). AnnexinV specifically binds to externalized PS, and PI can be used to examine cell membrane integrity. Apoptotic cells are Annexin V-FITC+/PI-, whereas necrotic cells are V-FITC+/PI+ (Choi and Lee, 2015). In this study, after treatment with different concentrations of coumarin, the number of cells in the Annexin V+/PI- quadrants increased, whereas the number of cells in the Annexin V+/PI+ quadrants remained almost unchanged (Figure 2A), revealing that coumarin induced C. albicans apoptosis.
Figure 2. Apoptosis was induced by coumarin treatment for 4 h. (A) Phosphatidylserine (PS) externalization was evaluated by Annexin V-FITC and PI double staining. The histogram was the quantitative analysis of Annexin-FITC-positive cells, and the data were shown as mean ± SD. *P < 0.05 and **P < 0.01. (B) DNA fragmentation was observed by TUNEL assay using flow cytometry. The histogram displayed the percentage of stained cells, and the values were expressed as mean ± SD, *P < 0.05 and **P < 0.01. (C) Nuclear condensation was observed by DAPI staining. BF, Bright Field. Bar = 5 μm.
As another apoptosis hallmark, DNA fragmentation can be mediated by the pro-apoptotic factors, Aif1 and Nuc1 (Madeo et al., 2009). To investigate whether coumarin could lead to DNA fragmentation, the treated cells were assessed using TUNEL assay, which could detect apoptotic DNA cleavage by labeling fluorescent dUTP at the 3'-OH ends of DNA (Phillips et al., 2003). An enhanced fluorescence intensity was observed with an increase in coumarin concentration (Figure 2B), suggesting that coumarin caused DNA fragmentation. To monitor the morphological changes in the nucleus, a DAPI assay was conducted. Fluorescence microscopy analysis revealed that cells treated with coumarin showed a more concentrated or cleaved fluorescence when compared to the control cells (Figure 2C), implying nuclear condensation and fragmentation.
Altogether, these results indicated that coumarin treatment led to PS externalization, DNA fragmentation, and nuclear condensation, which are key features of apoptosis.
Cytochrome c release from the mitochondria to the cytoplasm is a crucial event, which activates metacaspase, thereby inducing apoptosis (Wu et al., 2010). Here, the translocation of cytochrome c from the mitochondria and metacaspase activity were examined. Compared to the control cells, the relative levels of cytosolic cytochrome c were observed to have increased while mitochondrial cytochrome c contents were simultaneously observed to have decreased upon coumarin treatment (Figures 3A,B), thereby revealing that coumarin triggered the release of cytochrome c from the mitochondria to the cytosol.
Figure 3. Cytochrome c release and metacaspase activation were assayed after coumarin treatment for 4 h. (A,B) Cytosolic (A) and mitochondrial (B) cytochrome c levels were, respectively, measured. (C) Metacaspase activity was assessed by FITC-VAD-FMK assay. The percentage of stained cells was shown in the histogram, and the data were presented as mean ± SD. *P < 0.05.
Metacaspases, which are caspase-like cysteine proteases in yeast, play a central role in the early stages of apoptosis and can be detected via FITC-VAD-FMK staining (Park and Lee, 2010). Cells with activated intracellular metacaspases exhibit fluorescence, while the control cells appear unstained. Fluorescence intensity enhanced as the concentration of coumarin increased (Figure 3C), suggesting that metacaspase activity was elevated in coumarin-treated cells.
ROS play an essential role in yeast apoptosis since they induce and regulate apoptosis (Madeo et al., 1999). Thus, we used the fluorescent probe DCFH-DA, a ROS indicator, to assess intracellular ROS levels. Compared to the control cells, coumarin notably increased the fluorescence intensity in a dose-dependent manner (Figure 4A), suggesting that coumarin caused ROS accumulation in C. albicans. Elevated ROS levels can oxidize unsaturated fatty acids and trigger lipid peroxidation to form products such as malondialdehyde (MDA) (Mann et al., 2018). In our data, MDA was remarkably increased upon coumarin treatment (Figure S1), further confirming ROS accumulation in coumarin-treated cells.
Figure 4. ROS levels and mitochondrial functions were detected in C. albicans. (A) The intracellular ROS levels were analyzed using DCFH-DA. The histogram represented the percentage of stained cells, and the data were shown as mean ± SD, *P < 0.05. (B) Mitochondrial membrane potential was evaluated using JC-1 staining. The histogram was the quantitative result of fluorescence ratio (Y mean/X mean). *P < 0.05. (C) Mitochondrial morphology was observed using Mito-Tracker Green. BF, Bright Field. Bar, 5 μm.
Mitochondria also play an important role in yeast apoptosis since this organelle is the major site for ROS production and contains many proapoptotic factors such as cytochrome c, Aif1p, and Nuc1p (Pereira et al., 2008). The loss of mitochondrial membrane potential and enhancement of mitochondrial permeability are hallmarks of the early stages of apoptosis (Choi and Lee, 2015), and we assayed mitochondrial involvement in coumarin-induced apoptosis via JC-1 staining. In the mitochondrial matrix of normal cells, the lipophilic cationic JC-1 dye is observed as red aggregates. Contrastingly, in apoptotic cells with depolarized mitochondria, JC-1 remains in the cytoplasm and appears green. As shown in Figure 4B, FL2/FL1 ratio decreased in coumarin-treated cells in a concentration-dependent manner, suggesting that coumarin caused mitochondrial depolarization in C. albicans. Furthermore, mitochondrial morphology observation found that coumarin caused morphologically abnormal mitochondria that mostly had a fragmented appearance (Figure 4C). Taken together, coumarin addition affected mitochondrial functions.
Besides ROS, Ca2+ also plays an important role in initiating and executing yeast apoptosis (Carraro and Bernardi, 2016). Increased cytosolic Ca2+ triggers mitochondrial permeabilization and releases proapoptotic factors, which initiate the programmed cell death. In this study, Fluo-3 AM and Rhod-2 AM were used to measure cytosolic and mitochondrial Ca2+ levels, respectively. Data showed that the fluorescence intensities of both Fluo-3 AM and Rho-2 AM were observed to significantly increase in C. albicans cells treated with coumarin (Figures 5A,B), suggesting that coumarin triggered a movement of Ca2+ and its accumulation in the cytosol and mitochondria.
Figure 5. Ca2+ levels were, respectively, examined in the cytosol and mitochondria. (A) Cytosolic Ca2+ contents were determined using Fluo-3 AM staining. The histogram showed the percentage of stained cells, and the data were shown as mean ± SD. *P < 0.05. (B) Mitochondrial Ca2+ levels were evaluated via Rhod-2 AM staining. The histogram was the quantitative analysis of the percentage of stained cells, and the data were presented as mean ± SD. *P < 0.05.
An increase in ROS and Ca2+ is both an event of apoptosis, and plays a crucial role in the process of apoptosis (Zhang et al., 2008; Yun and Lee, 2016; Lee and Lee, 2017). To determine whether apoptosis caused by coumarin was related to increased ROS or mitochondrial Ca2+, a pretreatment with 5 mM N-acetyl cysteine (NAC), a ROS scavenger, and 0.1 mM ruthenium red (RR) (Lupetti et al., 2004; Yun and Lee, 2016), a mitochondrial Ca2+ influx inhibitor, was conducted to determine DNA fragmentation and metacaspase activity. Results showed that ROS elimination did not rescue apoptosis (data not shown). However, inhibition of the mitochondrial Ca2+ influx remarkably decreased DNA fragmentation and metacaspase activity (Figures 6A,B and Figures S3, S4). These results demonstrated that coumarin-induced C. albicans apoptosis was relevant to elevated mitochondrial Ca2+ levels.
Figure 6. Coumarin triggered apoptosis associated with mitochondrial Ca2+ influx. (A) DNA fragmentation was analyzed using TUNEL staining. The histogram displayed the percentage of stained cells, and the values were exhibited as mean ± SD, **P < 0.01 and ***P < 0.001. (B) Metacaspase activity was evaluated using FITC-VAD-FMK assay. The percentage of stained cells was shown in the histogram, and the data were displayed as mean ± SD. **P < 0.01 and ***P < 0.001.
To examine the effects of coumarin on the virulence of C. albicans in vivo, we administrated coumarin by oral-gastric (OG) gavage in a mouse model of systemic candidiasis. The results showed that mice in the normal saline, DMSO, and 20 mg/kg coumarin groups died within 3 days, while 50% of mice treated with 40 mg/kg coumarin survived the complete course of the experiment (P < 0.05) (Figure 7A). These results revealed that coumarin was able to treat C. albicans infection. To determine the toxicity of coumarin, non-infected mice were administrated with coumarin at doses of 20 and 40 mg/kg body weight by OG gavage. Data showed that all the mice survived the course of the experiment (Figure 7B), indicating that coumarin was not toxic below a dose of 40 mg/kg.
Figure 7. The effects of coumarin on the survival of mice were evaluated. (A) Survival rate of C. albicans-infected mice was assessed after exposure to coumarin for 10 days. (B) Toxicity of coumarin was observed in non-infected mice.
There is an urgent need to explore new-generation antifungal drugs due to the low efficacy, high toxicity, and drug resistance of the currently available antifungals (Carmona-Gutierrez et al., 2010). Many natural products such as lycopene, nerol, and limonene are reported to exhibit antifungal activity by inducing apoptosis. Apoptosis is a form of programmed cell death that is important for metazoan homeostasis and maintenance by eliminating unwanted, mutated, damaged, and superfluous cells (Rockenfeller and Madeo, 2008). In addition to metazoans, apoptosis has also been discovered in unicellular organisms such as C. albicans. Previous studies have demonstrated that coumarin and its derivatives display remarkable activities against fungi (Arshad et al., 2011). However, the potential mechanism explaining its antifungal action has not yet been fully elucidated.
In this study, we found that coumarin could inhibit cell growth and reduce strain viability. To study the pattern of cell death, the features of yeast apoptosis were determined, including PS externalization, DNA fragmentation, cytochrome c release, and metacaspase activation (Carmona-Gutierrez et al., 2010). The results showed that coumarin treatment led to PS exposure on the outer leaflet, DNA fragmentation and nuclear condensation, cytochrome c release from the mitochondria to the cytosol, and metacaspase activation, suggesting that coumarin induced apoptosis in C. albicans. Previous studies have demonstrated that coumarin damages C. albicans cells via pore formation in the cell wall and that cell death occurs due to the leakage of cytoplasmic contents and necrosis (Widodo et al., 2012), which is not consistent with our results. This might be due to the difference in the C. albicans strains used, distinct culture medium, incubation temperature, and coumarin treatment time.
As is known, mitochondria are both the origin and target of ROS (Terman et al., 2006), and ROS and mitochondria play an essential role in apoptotic processes (Pereira et al., 2008). During apoptosis, oxidative stress induces the mitochondrial permeability transition pore (PTP) to open, which then triggers the mitochondrial outer membrane permeabilization (MOMP) that is involved in the release of cytochrome c and other proapoptotic factors, leading to subsequent metacaspase activation (Simon et al., 2000). ROS can also directly induce apoptosis in a metacaspase-dependent manner (Wu et al., 2010). In this study, coumarin-treated C. albicans cells exhibited elevated ROS levels, decreased mitochondrial membrane potential, and abnormal mitochondrial morphology, indicating that coumarin triggered ROS accumulation and mitochondrial dysfunction.
Besides ROS, Ca2+ is also closely related to apoptosis (Carraro and Bernardi, 2016). In mammalian cells, apoptotic stimuli often cause an increase in cytosolic Ca2+ fueled via extracellular calcium influx and/or calcium release from intracellular stores (Jia et al., 2015). Among them, the release of Ca2+ from lysosomes is crucial for PS externalization (Mirnikjoo et al., 2009). Subsequently, cytosolic Ca2+ is rapidly relayed to mitochondria through MCU complex-dependent uptake (Almeida et al., 2008). Under oxidative stress, this Ca2+ signal can lead to PTP opening and release of cytochrome c and other proapoptotic factors, which ultimately results in the formation of apoptotic bodies and cell death. Although there is no MCU complex in yeast mitochondria, apoptotic stimuli could induce enough mitochondrial Ca2+ to undergo the permeability transition, which is accompanied by increased ROS and cytosolic Ca2+ levels. Once PTP opens, Ca2+ levels in the cytosol and matrix equilibrate and stabilize PTP in the open conformation, leading to matrix swelling, outer mitochondrial membrane damage, and release of proapoptotic proteins, thereby causing cell death (Carraro and Bernardi, 2016). Our study found that coumarin treatment caused Ca2+ accumulation in the cytosol and mitochondria, which might contribute to apoptosis.
Several studies reported that antioxidant or mitochondrial Ca2+ channel inhibitors could rescue cells from natural product-induced apoptosis (Zhang et al., 2008; Yun and Lee, 2016; Lee and Lee, 2017), indicating that oxidative stress or elevated mitochondrial Ca2+ might be the reasons leading to apoptosis. In our study, ROS removal did not prevent DNA fragmentation or metacaspase activation caused by coumarin, thereby demonstrating that coumarin-triggered apoptosis was independent of ROS or oxidative stress. However, the mitochondrial Ca2+ inhibitor significantly decreased coumarin-induced apoptosis-related features, suggesting that apoptosis was mediated by mitochondrial Ca2+ levels. Some coumarin derivatives have been reported to regulate mitochondrial permeability transition associated with cytosolic and mitochondrial Ca2+ equilibration (Cálgarohelena et al., 2006). When mitochondrial Ca2+ levels decreased, which was not enough to induce PTP opening, apoptosis was observed to be ameliorated. In summary, these results indicated that coumarin caused mitochondrial Ca2+-dependent apoptosis in C. albicans.
Singh et al. demonstrated that the coumarin derivative, SCD-1, could increase the survival of Aspergillus fumigatus-infected mice and decrease the colony counts in vital organs by treating with a dose of 200 mg/kg body weight orally or 100 mg/kg intraperitoneally, indicating that SCD-1 could be a potential strategy to treat aspergillosis (Singh et al., 2014). Previous studies mainly focused on the antifungal activity of coumarin or its derivatives against C. albicans in vitro (Thati et al., 2007; Creaven et al., 2009). The efficacy of these compounds in vivo is unclear. In this study, infected mice were administrated with coumarin by OG gavage to evaluate its anti-Candida activity in vivo. The results showed that the dose of 40 mg/kg coumarin prolonged the survival of the infected mice. Furthermore, toxicity assays showed that coumarin was non-toxic to the mice at the 40 mg/kg dose and low-toxic to human umbilical vein endothelial cells (HUVECs) (Figure S5). These results indicate that coumarin is effective and exhibits low toxicity in treating C. albicans infection.
Healthy C57BL/6 mice (male: 18–22 g) were provided by Wenzhou Medical University (License No. SCXK [ZJ] 2005– 0019). The Guide for the Care and Use of Laboratory Animals of the China National Institutes of Health was followed for procedures involving animals. All procedures were approved by the Animal Care and Use Committee of Wenzhou Medical University (wydw 2017-0046). All efforts were made to minimize the suffering of the mice.
CJ and JZ were responsible for designing and working in most aspects of the work, including the analysis and interpretation of data and the drafting of the manuscript. LY, CW, and YY participated in measuring ROS, Ca2+ levels, cytochrome c contents, and metacasapse activity. XR carried out the experiments about Annexin-FITC/PI co-staining and TUNEL staining. KX observed mitochondrial morphology and DNA fragmentation using Mito-Tracker and DAPI staining. MC participated in revising the manuscript and supervised the work.
This work was supported by the Natural Science Foundation of Zhejiang Province (LQ18C010003, LQ18H150003), and the Opening Project of Zhejiang Provincial Top Key Discipline of Pharmaceutical Sciences.
The authors declare that the research was conducted in the absence of any commercial or financial relationships that could be construed as a potential conflict of interest.
The Supplementary Material for this article can be found online at: https://www.frontiersin.org/articles/10.3389/fcimb.2018.00445/full#supplementary-material
Figure S1. The MDA contents were measured in C. albicans after treatment with coumarin for 4 h.
Figure S2. The MIC values of coumarin for 04311 and ATCC18804 strains were measured. Data were shown as mean ± SD. *P < 0.05 and **P < 0.01.
Figure S3. Coumarin triggered metacaspase activation related to mitochondrial Ca2+ influx in 04311 and ATCC18804 strains. The histogram displayed the percentage of stained cells, and the data were exhibited as mean ± SD, *P < 0.05, **P < 0.01 and ***P < 0.001.
Figure S4. Coumarin induced DNA fragmentation associated with mitochondrial Ca2+ influx in 04311 and ATCC18804 strains. The percentage of stained cells was shown in the histogram, and the data were displayed as mean ± SD. **P < 0.01 and ***P < 0.001.
Figure S5. Effect of coumarin on the survival of human umbilical vein endothelial cells (HUVECs). Data were shown as mean ± SD, *P < 0.05.
ROS, reactive oxygen species; DCFH-DA, 2′, 7′-dichlorodihydro-fluorescein diacetate; PI, propidium iodide; DAPI, 4′-6-diamino-2-phenylindole; CFU, colony forming units; MIC, minimal inhibitory concentration; JC-1, 5, 5′, 6, 6′-Tetrachloro-1, 1′, 3, 3′-tetraethyl-benzimidazolyl carbocyanine iodide.
Almeida, B., Silva, A., Mesquita, A., Sampaio-Marques, B., Rodrigues, F., and Ludovico, P. (2008). Drug-induced apoptosis in yeast. Biochim. Biophys. Acta 1783, 1436–1448. doi: 10.1016/j.bbamcr.2008.01.005
Álvarez-Delgado, C., Reyes-Chilpa, R., Estrada-Muñiz, E., Mendoza-Rodr'iguez, A., Quintero-Ruiz, A., Solano, J., et al. (2009). Coumarin A/AA induces apoptosis-like cell death in HeLa cells mediated by the release of apoptosis-inducing factor. J. Biochem. Mol. Toxic. 23, 263–272. doi: 10.1002/jbt.20288
Arshad, A., Osman, H., Bagley, M., Lam, C., Mohamad, S., and Zahariluddin, A. (2011). Synthesis and antimicrobial properties of some new thiazolyl coumarin derivatives. Eur. J. Med. Chem. 46, 3788–3794. doi: 10.1016/j.ejmech.2011.05.044
Cálgarohelena, A. F., Devienne, K. F., Rodrigues, T., Dorta, D. J., Raddi, M. S., Vilegas, W., et al. (2006). Effects of isocoumarins isolated from Paepalanthus bromelioides on mitochondria: uncoupling, and induction/inhibition of mitochondrial permeability transition. Chem. Biol. Interact. 161, 155–164. doi: 10.1016/j.cbi.2006.04.006
Carmona-Gutierrez, D., Eisenberg, T., Büttner, S., Meisinger, C., Kroemer, G., and Madeo, F. (2010). Apoptosis in yeast: triggers, pathways, subroutines. Cell Death Differ. 17, 763–773. doi: 10.1038/cdd.2009.219
Carraro, M., and Bernardi, P. (2016). Calcium and reactive oxygen species in regulation of the mitochondrial permeability transition and of programmed cell death in yeast. Cell Calcium 60, 102–107. doi: 10.1016/j.ceca.2016.03.005
Choi, H., and Lee, D. G. (2015). Lycopene induces apoptosis in Candida albicans through reactive oxygen species production and mitochondrial dysfunction. Biochimie 115, 108–115. doi: 10.1016/j.biochi.2015.05.009
Chuang, J. Y., Huang, Y. F., Lu, H. F., Ho, H. C., Yang, J. S., Li, T. M., et al. (2007). Coumarin induces cell cycle arrest and apoptosis in human cervical cancer HeLa cells through a mitochondria- and caspase-3 dependent mechanism and NF-kappaB down-regulation. Vivo 21, 1003–1009. doi: 10.1111/j.1471-8286.2005.01182.x
Creaven, B. S., Devereux, M., Karcz, D., Kellett, A., McCann, M., Noble, A., et al. (2009). Copper (II) complexes of coumarin-derived Schiff bases and their anti-Candida activity. J. Inorg. Biochem. 103, 1196–1203. doi: 10.1016/j.jinorgbio.2009.05.017
Edmond, M. B., Wallace, S. E., McClish, D. K., Pfaller, M. A., Jones, R. N., and Wenzel, R. P. (1999). Nosocomial bloodstream infections in United States hospitals: a three-year analysis. Clin. Infect. Dis. 29, 239–244. doi: 10.1086/520192
Ganguly, S., and Mitchell, A. (2011). Mucosal biofilms of Candida albicans. Curr. Opin. Microbiol. 14, 380–385. doi: 10.1016/j.mib.2011.06.001
Hu, X. L., Xu, Z., and Liu, M. L. (2017). Recent developments of coumarin hybrids as anti-fungal agents. Curr. Top. Med. Chem. 17, 3219–3231. doi: 10.2174/1568026618666171215100326
Jia, C., Yu, Q. L., Xu, N., Zhang, B., Dong, Y. J., Ding, X. H., et al. (2014). Role of TFP1 in vacuolar acidification, oxidative stress and filamentous development in Candida albicans. Fungal Genet. Biol. 71, 58–67. doi: 10.1016/j.fgb.2014.08.012
Jia, C., Zhang, K., Yu, Q., Zhang, B., Xiao, C., Dong, Y., et al. (2015). Tfp1 is required for ion homeostasis, fluconazole resistance and N-Acetylglucosamine utilization in Candida albicans. Biochim. Biophys. Acta 1853, 2731–2744. doi: 10.1016/j.bbamcr.2015.08.005
Jung, J. C., and Park, O. S. (2009). Synthetic approaches and biological activities of 4-Hydroxycoumarin derivatives. Molecules 14, 4790–4803. doi: 10.3390/molecules14114790
Kumar, R., Saha, A., and Saha, D. (2012). A new antifungal coumarin from Clausena excavata. Fitoterapia 83, 230–233. doi: 10.1016/j.fitote.2011.11.003
Leadsham, J., Kotiadis, V., Tarrant, D., and Gourlay, C. (2010). Apoptosis and the yeast actin cytoskeleton. Cell Death Differ. 17, 754–762. doi: 10.1038/cdd.2009.196
Lee, W., and Lee, D. G. (2017). Reactive oxygen species modulate itraconazole-induced apoptosis via mitochondrial disruption in Candida albicans. Free Radic Res. 52, 39–50. doi: 10.1080/10715762.2017.1407412
Lupetti, A., Brouwer, C. P. J. M., Dogterom-Ballering, H. E. C., Senesi, S., Campa, M., Dissel, J. T. V., et al. (2004). Release of calcium from intracellular stores and subsequent uptake by mitochondria are essential for the candidacidal activity of an N-terminal peptide of human lactoferrin. J. Antimicrob. Chemother. 54, 603–608. doi: 10.1093/jac/dkh385
Madeo, F., Carmona-Gutierrez, D., Ring, J., Büttner, S., Eisenberg, T., and Kroemer, G. (2009). Caspase-dependent and caspase-independent cell death pathways in yeast. Biochem. Biophys. Res. Commun. 382, 227–231. doi: 10.1016/j.bbrc.2009.02.117
Madeo, F., Fröhlich, E., Ligr, M., Grey, M., Sigrist, S. J., Wolf, D. H., et al. (1999). Oxygen stress: a regulator of apoptosis in yeast. J. Cell Biol. 145, 757–767. doi: 10.1083/jcb.145.4.757
Mann, J. P., Valenti, L., Scorletti, E., Byrne, C. D., and Nobili, V. (2018). Nonalcoholic fatty liver disease in children. Semin. Liver Dis. 38, 1–13. doi: 10.1055/s-0038-1627456
Mirnikjoo, B., Balasubramanian, K., and Schroit, A. (2009). Mobilization of lysosomal calcium regulates the externalization of phosphatidylserine during apoptosis. J. Biol. Chem. 284, 6918–6923. doi: 10.1074/jbc.M805288200
Musa, M. A., Badisa, V. L., Latinwo, L. M., Patterson, T. A., and Owens, M. A. (2012). Coumarin-based benzopyranone derivatives induced apoptosis in human lung (A549) cancer cells. Anticancer Res. 32, 4271–4276.
Park, C., and Lee, D. G. (2010). Melittin induces apoptotic features in Candida albicans. Biochem. Biophys. Res. Commun. 394, 170–172. doi: 10.1016/j.bbrc.2010.02.138
Pereira, C., Silva, R., Saraiva, L., Johansson, B., Sousa, M., and Côrte-Real, M. (2008). Mitochondria-dependent apoptosis in yeast. Biochim. Biophys. Acta. 1783, 1286–1302. doi: 10.1016/j.bbamcr.2008.03.010
Phillips, A. J., Sudbery, I., and Ramsdale, M. (2003). Apoptosis induced by environmental stresses and amphotericin B in Candida albicans. Proc. Natl. Acad. Sci. U.S.A. 100, 14327–14332. doi: 10.1073/pnas.2332326100
Rockenfeller, P., and Madeo, F. (2008). Apoptotic death of ageing yeast. Exp. Gerontol. 43, 876–881. doi: 10.1016/j.exger.2008.08.044
Simon, H. U., Hajyehia, A., and Levischaffer, F. (2000). Role of reactive oxygen species (ROS) in apoptosis induction. Apoptosis 5, 415–418. doi: 10.1023/A:1009616228304
Singh, S., Dabur, R., Gatne, M. M., Singh, B., Gupta, S., Pawar, S., et al. (2014). In vivo efficacy of a synthetic coumarin derivative in a murine model of aspergillosis. PLoS ONE 9:e103039. doi: 10.1371/journal.pone.0103039
Terman, A., Gustafsson, B., and Brunk, U. T. (2006). The lysosomal-mitochondrial axis theory of postmitotic aging and cell death. Chem. Biol. Interact. 163, 29–37. doi: 10.1016/j.cbi.2006.04.013
Thati, B., Noble, A., Rowan, R., Creaven, B. S., Walsh, M., McCann, M., et al. (2007). Mechanism of action of coumarin and silver(I)-coumarin complexes against the pathogenic yeast Candida albicans. Toxicol. Vitro 21, 801–818. doi: 10.1016/j.tiv.2007.01.022
Tian, H., Qu, S., Wang, Y., Lu, Z., Zhang, M., Gan, Y., et al. (2017a). Calcium and oxidative stress mediate perillaldehyde-induced apoptosis in Candida albicans. Appl. Microbiol. Biotechnol. 101, 3335–3345. doi: 10.1007/s00253-017-8146-3
Tian, J., Lu, Z., Wang, Y., Man, Z., Wang, X., Tang, X., et al. (2017b). Nerol triggers mitochondrial dysfunction and disruption via elevation of Ca2+ and ROS in Candida albicans. Int. J. Biochem. Cell Biol. 85, 114–122. doi: 10.1016/j.biocel.2017.02.006
Vermes, I., Haanen, C., and Reutelingsperger, C. (2000). Flow cytometry of apoptotic cell death. J. Immunol. Methods 243, 167–190. doi: 10.1016/S0022-1759(00)00233-7
Widodo, G. P., Sukandar, E. Y., Adnyana, I. K., and Sukrasno, S. (2012). Mechanism of action of coumarin against Candida albicans by SEM/TEM Analysis. J. Math. Fun. Sci. 44, 179–192. doi: 10.5614/itbj.sci.2012.44.2.4
Wisplinghoff, H., Bischoff, T., Tallent, S. M., Seifert, H., Wenzel, R. P., and Edmond, M. B. (2004). Nosocomial bloodstream infections in US hospitals: analysis of 24,179 cases from a prospective nationwide surveillance study. Clin. Infect. Dis. 39, 309–317. doi: 10.1086/421946
Wu, X. Z., Chang, W. Q., Cheng, A. X., Sun, L. M., and Lou, H. X. (2010). Plagiochin E, an antifungal active macrocyclic bis(bibenzyl), induced apoptosis in Candida albicans through a metacaspase-dependent apoptotic pathway. Biochim. Biophys. Acta 1800, 439–447. doi: 10.1016/j.bbagen.2010.01.001
Yun, D. G., and Lee, D. G. (2016). Silibinin triggers yeast apoptosis related to mitochondrial Ca2+ influx in Candida albicans. Int. J. Biochem. Cell Biol. 80, 1–9. doi: 10.1016/j.biocel.2016.09.008
Yun, J., and Lee, D. G. (2017). Role of potassium channels in chlorogenic acid-induced apoptotic volume decrease and cell cycle arrest in Candida albicans. Biochim. Biophys. Acta 1861, 585–592. doi: 10.1016/j.bbagen.2016.12.026
Keywords: coumarin, Candida albicans, apoptosis, ROS, Ca2+
Citation: Jia C, Zhang J, Yu L, Wang C, Yang Y, Rong X, Xu K and Chu M (2019) Antifungal Activity of Coumarin Against Candida albicans Is Related to Apoptosis. Front. Cell. Infect. Microbiol. 8:445. doi: 10.3389/fcimb.2018.00445
Received: 28 July 2018; Accepted: 13 December 2018;
Published: 04 January 2019.
Edited by:
Nahed Ismail, University of Illinois at Chicago, United StatesReviewed by:
Sadri Znaidi, Institut Pasteur de Tunis, TunisiaCopyright © 2019 Jia, Zhang, Yu, Wang, Yang, Rong, Xu and Chu. This is an open-access article distributed under the terms of the Creative Commons Attribution License (CC BY). The use, distribution or reproduction in other forums is permitted, provided the original author(s) and the copyright owner(s) are credited and that the original publication in this journal is cited, in accordance with accepted academic practice. No use, distribution or reproduction is permitted which does not comply with these terms.
*Correspondence: Maoping Chu, Y2htcGluZ0Bob3RtYWlsLmNvbQ==
†These authors have contributed equally to this work
‡Present Address: Maoping Chu, Children's Heart Center, Institute of Cardiovascular Development and Translational Medicine, and Pediatric Research Institute, The Second Affiliated Hospital and Yuying Children's Hospital, Wenzhou Medical University, Wenzhou, China
Disclaimer: All claims expressed in this article are solely those of the authors and do not necessarily represent those of their affiliated organizations, or those of the publisher, the editors and the reviewers. Any product that may be evaluated in this article or claim that may be made by its manufacturer is not guaranteed or endorsed by the publisher.
Research integrity at Frontiers
Learn more about the work of our research integrity team to safeguard the quality of each article we publish.