- 1Division of Obstetrics and Gynecology, School of Medicine, The University of Western Australia, Crawley, WA, Australia
- 2The Marshall Centre for Infectious Diseases Research and Training, School of Biomedical Sciences, The University of Western Australia, Crawley, WA, Australia
Increasing reports of antimicrobial resistance and limited new antibiotic discoveries and development have fuelled innovation in other research fields and led to a revitalization of bacteriophage (phage) studies in the Western world. Phage therapy mainly utilizes obligately lytic phages to kill their respective bacterial hosts, while leaving human cells intact and reducing the broader impact on commensal bacteria that often results from antibiotic use. Phage therapy is rapidly evolving and has resulted in cases of life-saving therapeutic use and multiple clinical trials. However, one of the biggest challenges this antibiotic alternative faces relates to regulations and policy surrounding clinical use and implementation beyond compassionate cases. This review discusses the multi-drug resistant Gram-negative pathogens of highest critical priority and summarizes the current state-of-the-art in phage therapy targeting these organisms. It also examines phage therapy in humans in general and the approaches different countries have taken to introduce it into clinical practice and policy. We aim to highlight the rapidly advancing field of phage therapy and the challenges that lie ahead as the world shifts away from complete reliance on antibiotics.
The Challenge of Multi-Drug Resistant Bacteria
In 2017 the World Health Organization published a list of global priority pathogens comprising 12 species of bacteria categorized into critical, high and medium priority based on their level of resistance and available therapeutics (Tacconelli et al., 2018). The current rate of resistance development far exceeds the level of antibiotic discovery and development and represents a global public health challenge. Estimates have suggested that upwards of 10 million people could die each year due to antimicrobial resistance by 2050 (O'neill, 2014). While this is a contentious figure (De Kraker et al., 2016), it nonetheless highlights the serious problem we face regarding therapeutic options for multi-drug resistant (MDR) bacterial infections (Bassetti et al., 2017). The natural predators of bacteria are the bacterial viruses known as bacteriophages or phages. Found ubiquitously, these organisms are estimated to be present at numbers equivalent to a trillion per grain of sand on Earth (Keen, 2015). Evolving in parallel with bacteria, phages are potential antibacterial therapeutic agents against such MDR pathogens (Burrowes et al., 2011). Here we focus on three critical priority pathogens, Acinetobacter baumannii, Pseudomonas aeruginosa, and members of the Enterobacteriaceae (Tacconelli et al., 2018) and the current advances in phage therapy research to target these organisms, as well as exploring more general issues of clinical trials and regulatory complexities of phage therapy.
Acinetobacter Baumannii
A. baumannii is recognized as a critical priority pathogen due to the increasing incidence of antimicrobial resistance and significant role in nosocomial infections (Mcconnell et al., 2013). Around 20 years after an early trial of anti-A. baumannii phage therapy in mice (Soothill, 1992), a surge in reports of A. baumannii lytic phage isolation and their in vitro activity occurred, as reviewed by Garcia-Quintanilla et al. (2013). Since this time, significant advances have been made with further in vitro studies (Liu et al., 2016; Ghajavand et al., 2017) and numerous in vivo animal studies (Kusradze et al., 2016; Regeimbal et al., 2016; Yin et al., 2017; Zhou et al., 2018). Phage therapy evaluation in a mouse model of A. baumannii infection resulted in 2.3-fold increased survival in the phage-treated group compared to control groups (Cha et al., 2018).
A novel lysin from A. baumannii prophages with the capacity to kill clinical MDR isolates and rescue mice from lethal infections has also been characterized (Lood et al., 2015). The use of these enzymatic compounds is not a new concept; and although lysin use has been restricted in Gram-negative bacteria due to their outer membrane barrier, a rise in the literature suggests that this no longer poses a constraint on lysin use in Gram-negatives (Thandar et al., 2016; Peng et al., 2017; Larpin et al., 2018).
Advances have also been made in human phage therapy trials. A key case in the United States involved the first intravenous administration of phage therapy and resulted in the successful treatment and recovery of a patient with A. baumannii pancreatic pseudocyst infection (Schooley et al., 2017). This has led to increased phage therapy exposure to the public and arguably increased clinical awareness regarding this alternative therapeutic. More recently another case study involving infection at a craniectomy site with a MDR-A. baumannii, applied a personalized phage cocktail intravenously in an attempt to improve patient outcomes (Lavergne et al., 2018). Unfortunately, the patient passed away after life support efforts were ceased following the family's request. In cases such as this it is difficult to navigate the regulatory issues in a timely manner; while personalized therapy is ideal to adapt to patient needs it can be a challenge.
Pseudomonas Aeruginosa
P. aeruginosa is a major opportunistic pathogen and cause of nosocomial infections (Lyczak et al., 2000; Breidenstein et al., 2011). It is also a frequent cause of chronic lung infections in cystic fibrosis patients and as such has been assessed as a target for phage therapy (Olszak et al., 2015). Phage therapy for P. aeruginosa infections dates back more than 50 years (Bertoye et al., 1959; Soothill, 2013), but recent developments in use of both phage lysins and live phage are very promising. A review by Rossitto and colleagues describes the current literature in this field and the challenges associated with phage therapy in cystic fibrosis, in particular they suggest that future studies include testing on both mucoid and non-mucoid P. aeruginosa isolates and the use of both pulmonary and non-pulmonary host models (Rossitto et al., 2018). Spray-dried formulations of phages have also been thoroughly tested for inhaled application against P. aeruginosa lung infection (Chang et al., 2017, 2018). Immunogenicity data has been assessed using an in vitro human lung model and demonstrated an increase in IL-6 and TNF-a for one of two phages (Shiley et al., 2017). The human immune response is an important consideration when assessing therapeutic phage application (Krut and Bekeredjian-Ding, 2018) however, beneficial effects of the immune response conducive to positive phage therapy outcomes have also been reported (Roach et al., 2017).
A cocktail of six phages was observed to successfully treat respiratory P. aeruginosa infection in mice and, additionally, sepsis in Galleria mellonella models (Forti et al., 2018). Ability of some phages to penetrate P. aeruginosa biofilms is another major advantage over conventional treatments (Fong et al., 2017; Waters et al., 2017), while co-administration of phages and antibiotics has been reported as a mechanism of restoring antibiotic sensitivity (Chan et al., 2016). In the latter case, where phages utilize components of multidrug efflux pump systems as receptors, mutation to confer phage-resistance alters the pump mechanism, leading to antibiotic re-sensitization. A case study of aortic prosthetic graft infection by P. aeruginosa with direct administration to the graft of a combination of phage and ceftazidime was successful in resolving and possibly eradicating infection (Chan et al., 2018). Finally, phage lysin research is also on the increase: Guo et al. described a novel endolysin with in vitro activity against P. aeruginosa and other Gram-negative bacteria on the critical priority pathogens list (Guo et al., 2017), with similar reports from other groups (Larpin et al., 2018).
Enterobacteriaceae
Within the family Enterobacteriaceae, Escherichia coli, and Klebsiella spp. ranked highest in the WHO critical priority list of antibiotic-resistant bacteria followed by Enterobacter, Serratia and Proteus spp. (Tacconelli et al., 2018). While occupying many commensal niches, E. coli isolates include significant intestinal and extraintestinal pathogens (Bolocan et al., 2016). The majority of early phage research was undertaken with coliphages (phages that infect E. coli), particularly T4 (Stahl, 1989; Edgar, 2004), evidenced by numerous studies, some of which are summarized by Bolocan et al. (2016). More recently, in vitro and in vivo studies have shown promising results, for example control of enteropathogenic E. coli in mice with hospital sewage-isolated phage (Vahedi et al., 2018) and effect of coliphages against planktonic and biofilm-associated infections (Tkhilaishvili et al., 2018).
Klebsiella spp. are frequent nosocomial and community-acquired pathogens recognized for their MDR status. Cao et al. administered intranasal phage to treat K. pneumoniae lung infection in mice, resulting in protection against lethal infection and lower inflammatory cytokine levels in the lung (Cao et al., 2015). Similarly, in a burn wound mouse model of K. pneumoniae infection, topical phage application resulted in a significant reduction in mortality (Kumari et al., 2011) and a liposome loaded phage cocktail enhanced bacterial clearance and rate of healing (Chadha et al., 2017).
Other phage therapeutic uses include prevention of biofilm formation. Depolymerase producing K. pneumoniae phage, in combination with iron antagonizing agents, showed ability to eradicate early biofilms of K. pneumoniae: a promising preventative strategy (Chhibber et al., 2013). Progress has also been made in lysin research: Yan et al. described a novel fusion protein that combines the receptor binding domains of colicin A with an E. coli phage lysin to overcome the blocking effect of the Gram-negative outer membrane, with successful control of E. coli both in vitro and in a mouse model (Yan et al., 2017).
While many studies have addressed phage therapy in vitro and in vivo, there is much further work required for translation into humans. Case reports have been discussed, but lack the robust evidence of clinical trials.
Phage Therapy in Humans
Phage use in Eastern Europe and the former Soviet Union has been widespread since their discovery; as a result therapeutic phage use is integrated within their health care systems. However, this potential therapy is only recently being investigated according to rigorous scientific standards (Kutter et al., 2010; Villarroel et al., 2017). Abedon has presented a list of key criteria that should be thoroughly considered and reported in phage therapy studies (Abedon, 2017). Information critical to the success of clinical trials includes the adequate characterization and selection of phages as well as of the subjects (humans) and the target bacteria. Additional data are also required such as formulations, dosing and efficacy, however, without the foundation of characterized and well-planned targets these are of no value. Detailed reporting would improve the quality of future research and enable replication and extension of previous studies. Another consideration is the choice of appropriate disease targets for phage therapy (Harper, 2018). For example, the species specificity characteristic of most phages is generally highly desirable in monomicrobial diseases, however, this specificity can be a major limitation in cases of polymicrobial infections unless, perhaps, the phage is administered in combination with a suitable antibiotic. Such considerations are imperative for patient safety in clinical trials, as removal of one pathogen and consequent overgrowth of a second could potentially have fatal consequences (Harper, 2018). On the other hand, it may be that broad-host-range phages are more common than is currently believed, due in part to biases in phage isolation methods (De Jonge et al., 2018): this disparity deserves much further research.
Clinical Trials Involving Phages
One of the current challenges of progressing phage therapy into the clinic is the lack of validated and adequately controlled clinical trials. Additional care should be taken in the planning and design of such trials as, while clinical trial design for phage therapy will naturally share many parallels with standard drug clinical trials, there are several factors that are unique to phages. These include pharmacological considerations such as the dosage (Payne and Jansen, 2003). As these are self-replicating viruses, their dose has the potential to exponentially increase upon reaching the bacteria of interest. This leads to another consideration of application: phages require direct contact with the bacteria and if distributed too broadly they will be less efficacious. Topical applications have been widely used to address this, however, as mentioned other methods have been used with success. When considering monotherapy or combination therapy approaches, phage cocktails offer broad spectrum activity and reduce the chances of resistance formation, however, it should be noted that combination therapy greatly increases the challenge of assessing inflammatory effects, potential for gene transfer and phage resistance development for all phages in a cocktail (Parracho et al., 2012).
Some have argued that exposure to bacteriophages occurs in humans every day and is evidence of their safety, however, in the context of clinical trials there are a number of considerations that should be addressed. The first of these relates to the sterility and purity of the phage preparation. It is imperative that products exclude toxins and bacterial debris to comply with good manufacturing practice or equivalent quality assurance standards. Parracho et al. described the quality parameters recommended for bacteriophage products from the point of phage identification through to manufacturing processes (Parracho et al., 2012). Secondly, concerns surrounding the potential for the onset of toxic shock as a result of the bactericidal effect of phages must also be addressed. While this has been reported to not be an issue (Speck and Smithyman, 2016) and this method of bacterial killing is shared by bactericidal antibiotics (Dufour et al., 2017), this is a necessary safety consideration prior to clinical trials.
Previous clinical trials involving phage therapy have been described in detail by Kutter et al. and include those undertaken in Georgia and Poland (Kutter et al., 2010). Worth noting are two phage therapy clinical trials that are used as examples throughout the literature, addressing safety of phages for treating venous leg ulcers (Rhoads et al., 2009) and safety and efficacy in chronic otitis (Wright et al., 2009). Rhoads and colleagues reported on safety in a small phase I trial in patients with venous leg ulcers and reported no adverse events with the administration of phages (Rhoads et al., 2009). Wright et al. demonstrated efficacy and safety of anti-Pseudomonal phages against late stage recurrent otitis which was dominated by MDR-P. aeruginosa. These are among the first controlled clinical trials in humans conducted in the western world. More recently, a number of clinical trials have been registered (https://clinicaltrials.gov/ and https://globalclinicaltrialdata.com/) as summarized in Figure 1 (Miedzybrodzki et al., 2012; Sarker et al., 2016; Leitner et al., 2017). At both web sites, use of the search phrase “phage therapy” resulted in 15 studies/trials in the former site, of which nine were phage therapy-related, with a focus on treatment of infection. Two additional studies were identified using the global clinical trials resource. Search results did not all represent standard clinical trials, for example sputum collections for in vitro phage testing and expanded access interventional trials were also included. Additional, scientifically sound clinical trials are vital to increasing the western clinical worlds' acceptance of phage therapy applications. While many observational studies have been conducted, these have been limited by small sample sizes and many are poorly controlled. Conversely, promising case studies do exist, however, robust clinical trial data is what is required by regulators in order to progress clinical guidelines for phage therapy.
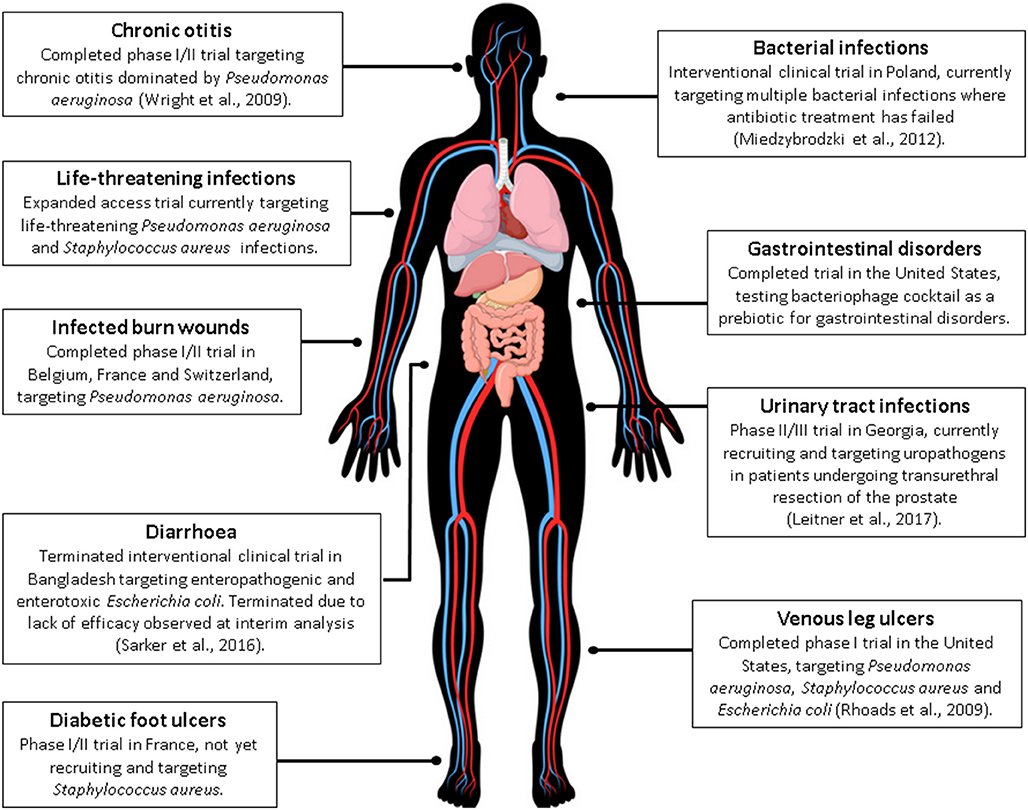
Figure 1. A current summary of human phage therapy trials and the range of target sites/infections (see www.clinicaltrials.gov or https://globalclinicaltrialdata.com/ where citation is not given). This figure includes a licensed image obtained by the authors.
Regulation and Policy Development
No framework currently exists that explicitly defines phages in the context of medicinal products for use in humans, however, in Georgia these are embedded in the healthcare system as a standard medical application (Kutateladze, 2015). Specifically, the Eliava Institute of Bacteriophages, Microbiology and Virology has several phage preparations readily available (over-the-counter) and a broader range of products, specifically supplied to medical practitioners (Kutter et al., 2010; Kutateladze, 2015). Similarly, Poland has the Hirszfeld Institute of Immunology and Experimental Therapy, although this center supplies personalized phage products directly to physicians using a more tailored approach (Kutter et al., 2010). In other parts of the world, however, bacteriophages present a unique regulatory agenda.
Gorski summarizes the current access schemes around the world and identifies the main inclusion of compassionate use cases in most countries as a last resort option (Gorski et al., 2018). Schemes vary, however, all respond to the situation of a critically ill or chronically suffering patient for whom all authorized treatment options have been exhausted. While these schemes are beneficial in the short-term, it has been recognized that a dedicated phage therapy legal framework is essential for the smooth introduction of natural phage therapy into western medicine. Regulatory calls to action have been made in Europe with discussions around regulatory hurdles and future steps required to achieve appropriate phage-based therapeutic guidelines (Huys et al., 2013; Verbeken et al., 2014).
Working Towards a Solution
A thorough analysis of key stakeholder opinions on the regulatory status of phage therapy was reported by Verbeken et al. (2014). Calls for two regulatory pathways were proposed, including product market placement of natural phage-based products and hospital exemption pathways for tailored phage therapy. The consensus among surveyed stakeholders was the need for a dedicated new regulatory framework for phage therapy and one which acknowledges the specific properties of phages and their interactions, in addition to the role of hospitals as providers of phage therapy (Verbeken et al., 2014). In the same vein, a workshop with the European Medicines Agency (EMA) set out to work together with all stakeholders to provide a solution to regulatory hurdles faced by phage researchers, while maintaining the standards of quality and safety (Pelfrene et al., 2016). Here, the EMA confirmed that none of the current regulations were suitable for phage therapy and discussed options for the way forward.
Magistral Phages
Political progression in Belgium has resulted in a magistral phage regulatory framework: a pragmatic framework to encompass tailored phage therapy (Pirnay et al., 2018). This regulatory framework includes a magistral formula in which non-authorized phage products can be prepared by a pharmacist, given the external quality assessment of the phage preparations. Quality assurance and good manufacturing practice are of extreme importance for any therapeutic agent and considerations for phage banks would include the characterization of all phages so that amongst other parameters, identity, viability, potency and purity are ensured (Pirnay et al., 2015; Pelfrene et al., 2016).
A Therapeutic Classification for Phages
Questions regarding the biological status of phages include whether they are living or not, which highlights the need for defined phage-specific terms of policy. As it currently stands, phage therapy in many cases represents the epitome of personalized medicine as it is a process involving tailor-made phage combinations specific for an individual patient's bacterial infection/s. This presents difficulties in the regulatory pipeline, as this move toward personalized medicine breaks the mold of regulatory conventions. It must be acknowledged that other therapeutics, for example cancer therapy (Daly, 2007), have faced a similar hurdle in the past and refinement is certainly possible. The Food and Drug Administration in the United States has recently provided an opportunity for the new Center for Innovative Phage Applications and Therapeutics (IPATH) to utilize phage therapy via the Emergency Investigational New Drug scheme. These initiatives are likely to improve clinical understanding and acceptance, while also providing supporting evidence of the need for dedicated regulatory guidelines.
A Future for Phages
There is no doubt that phage therapy is an attractive solution to combating escalating antibiotic resistance. Numerous studies highlight the in vitro and in vivo potential of therapeutic phages and while a number of clinical trials have taken place over the last decade, further data is needed to present a robust regulatory case for clinical use. There remain obvious challenges ahead for phage therapy, particularly regarding management of regulatory policy. Progression toward novel schemes based around knowledge of phage applications should guide these processes and work toward a reasonable implementation structure. Ideally, regulatory developments should be reached in a standardized and global manner; however, this is understandably a challenge. While the field is rapidly progressing toward therapeutics, fuelled by the evident need for antibiotic alternatives, regulatory processes must be refined and approached from a novel phage-based perspective. One size does not fit all and collaborative efforts to build models that suit phages will surely result in better health outcomes for all. We must also remember that despite the frustrations of legislative parameters, it is of utmost importance to conserve high standards of safety, quality, and efficacy. It is vital that scientists and clinicians continue having these discussions with the appropriate regulatory bodies and move this area forward sooner rather than later.
Author Contributions
LF conceived the review topic and focus, drafted the manuscript and approved the final version to be published. Both BC and MP contributed to the structure and content, critically revised the drafted manuscript and approved the final version to be published.
Funding
LF is supported by an Australian Government Research Training Program Scholarship and a Professor Gordon King Postgraduate Scholarship, provided by the Women and Infants Research Foundation. MP is supported by an NHMRC project grant [1144040].
Conflict of Interest Statement
The authors declare that the research was conducted in the absence of any commercial or financial relationships that could be construed as a potential conflict of interest.
References
Abedon, S. T. (2017). Information phage therapy research should report. Pharmaceuticals 10:E43. doi: 10.3390/ph10020043
Bassetti, M., Poulakou, G., Ruppe, E., Bouza, E., Van Hal, S. J., and Brink, A. (2017). Antimicrobial resistance in the next 30 years, humankind, bugs and drugs: a visionary approach. Intensive Care Med. 43, 1464–1475. doi: 10.1007/s00134-017-4878-x
Bertoye, A., Gaillard, L., and Courtieu, A. L. (1959). Adapted bacteriophages in the treatment of infections caused by antibiotic-resistant microorganisms. J. Med. Lyon 40, 465–471.
Bolocan, A. S., Callanan, J., Forde, A., Ross, P., and Hill, C. (2016). Phage therapy targeting Escherichia coli-a story with no end? FEMS Microbiol. Lett. 363:fnw256. doi: 10.1093/femsle/fnw256
Breidenstein, E. B., De La Fuente-Nunez, C., and Hancock, R. E. (2011). Pseudomonas aeruginosa: all roads lead to resistance. Trends Microbiol. 19, 419–426. doi: 10.1016/j.tim.2011.04.005
Burrowes, B., Harper, D. R., Anderson, J., Mcconville, M., and Enright, M. C. (2011). Bacteriophage therapy: potential uses in the control of antibiotic-resistant pathogens. Expert Rev. Anti Infect Ther. 9, 775–785. doi: 10.1586/eri.11.90
Cao, F., Wang, X., Wang, L., Li, Z., Che, J., Wang, L., et al. (2015). Evaluation of the efficacy of a bacteriophage in the treatment of pneumonia induced by multidrug resistance Klebsiella pneumoniae in mice. Biomed. Res. Int. 2015:752930. doi: 10.1155/2015/752930
Cha, K., Oh, H. K., Jang, J. Y., Jo, Y., Kim, W. K., Ha, G. U., et al. (2018). Characterization of two novel bacteriophages infecting multidrug-resistant (MDR) Acinetobacter baumannii and evaluation of their therapeutic efficacy in vivo. Front. Microbiol. 9:696. doi: 10.3389/fmicb.2018.00696
Chadha, P., Katare, O. P., and Chhibber, S. (2017). Liposome loaded phage cocktail: enhanced therapeutic potential in resolving Klebsiella pneumoniae mediated burn wound infections. Burns 43, 1532–1543. doi: 10.1016/j.burns.2017.03.029
Chan, B. K., Sistrom, M., Wertz, J. E., Kortright, K. E., Narayan, D., and Turner, P. E. (2016). Phage selection restores antibiotic sensitivity in MDR Pseudomonas aeruginosa. Sci. Rep. 6:26717. doi: 10.1038/srep26717
Chan, B. K., Turner, P. E., Kim, S., Mojibian, H. R., Elefteriades, J. A., and Narayan, D. (2018). Phage treatment of an aortic graft infected with Pseudomonas aeruginosa. Evol. Med. Public Health 2018, 60–66. doi: 10.1093/emph/eoy005
Chang, R. Y., Wong, J., Mathai, A., Morales, S., Kutter, E., Britton, W., et al. (2017). Production of highly stable spray dried phage formulations for treatment of Pseudomonas aeruginosa lung infection. Eur. J. Pharm. Biopharm. 121, 1–13. doi: 10.1016/j.ejpb.2017.09.002
Chang, R. Y. K., Chen, K., Wang, J., Wallin, M., Britton, W., Morales, S., et al. (2018). Proof-of-principle study in a murine lung infection model of antipseudomonal activity of phage PEV20 in a dry-powder formulation. Antimicrob. Agents Chemother. 62:e01714–e01717.
Chhibber, S., Nag, D., and Bansal, S. (2013). Inhibiting biofilm formation by Klebsiella pneumoniae B5055 using an iron antagonizing molecule and a bacteriophage. BMC Microbiol. 13:174. doi: 10.1186/1471-2180-13-174
De Jonge, P. A., Nobrega, F. L., Brouns, S. J. J., and Dutilh, B. E. (2018). Molecular and evolutionary determinants of bacteriophage host range. Trends Microbiol. 7:1352. doi: 10.1016/j.tim.2018.08.006
De Kraker, M. E., Stewardson, A. J., and Harbarth, S. (2016). Will 10 million people die a year due to antimicrobial resistance by 2050? PLoS Med. 13:e1002184. doi: 10.1371/journal.pmed.1002184
Dufour, N., Delattre, R., Ricard, J. D., and Debarbieux, L. (2017). The lysis of pathogenic Escherichia coli by bacteriophages releases less endotoxin than by beta-lactams. Clin. Infect. Dis. 64, 1582–1588. doi: 10.1093/cid/cix184
Fong, S. A., Drilling, A., Morales, S., Cornet, M. E., Woodworth, B. A., Fokkens, W. J., et al. (2017). Activity of bacteriophages in removing biofilms of Pseudomonas aeruginosa isolates from chronic rhinosinusitis patients. Front. Cell Infect. Microbiol. 7:418. doi: 10.3389/fcimb.2017.00418
Forti, F., Roach, D. R., Cafora, M., Pasini, M. E., Horner, D. S., Fiscarelli, E. V., et al. (2018). Design of a broad-range bacteriophage cocktail that reduces Pseudomonas aeruginosa biofilms and treats acute infections in two animal models. Antimicrob. Agents Chemother. 62:e02573–17. doi: 10.1128/AAC.02573-17
Garcia-Quintanilla, M., Pulido, M. R., Lopez-Rojas, R., Pachon, J., and Mcconnell, M. J. (2013). Emerging therapies for multidrug resistant Acinetobacter baumannii. Trends Microbiol. 21, 157–163. doi: 10.1016/j.tim.2012.12.002
Ghajavand, H., Esfahani, B. N., Havaei, A., Fazeli, H., Jafari, R., and Moghim, S. (2017). Isolation of bacteriophages against multidrug resistant Acinetobacter baumannii. Res. Pharm. Sci. 12, 373–380. doi: 10.4103/1735-5362.213982
Gorski, A., Miedzybrodzki, R., Lobocka, M., Glowacka-Rutkowska, A., Bednarek, A., Borysowski, J., et al. (2018). Phage therapy: what have we learned? Viruses 10:E288. doi: 10.3390/v10060288
Guo, M., Feng, C., Ren, J., Zhuang, X., Zhang, Y., Zhu, Y., et al. (2017). A novel antimicrobial endolysin, LysPA26, against Pseudomonas aeruginosa. Front. Microbiol. 8:293. doi: 10.3389/fmicb.2017.00293
Harper, D. R. (2018). Criteria for selecting suitable infectious diseases for phage therapy. Viruses 10:E177. doi: 10.3390/v10040177
Huys, I., Pirnay, J. P., Lavigne, R., Jennes, S., De Vos, D., Casteels, M., et al. (2013). Paving a regulatory pathway for phage therapy. Europe should muster the resources to financially, technically and legally support the introduction of phage therapy. EMBO Rep. 14, 951–954. doi: 10.1038/embor.2013.163
Keen, E. C. (2015). A century of phage research: bacteriophages and the shaping of modern biology. Bioessays 37, 6–9. doi: 10.1002/bies.201400152
Krut, O., and Bekeredjian-Ding, I. (2018). Contribution of the immune response to phage therapy. J. Immunol. 200, 3037–3044. doi: 10.4049/jimmunol.1701745
Kumari, S., Harjai, K., and Chhibber, S. (2011). Bacteriophage versus antimicrobial agents for the treatment of murine burn wound infection caused by Klebsiella pneumoniae B5055. J. Med. Microbiol. 60, 205–210. doi: 10.1099/jmm.0.018580-0
Kusradze, I., Karumidze, N., Rigvava, S., Dvalidze, T., Katsitadze, M., Amiranashvili, I., et al. (2016). Characterization and testing the efficiency of Acinetobacter baumannii phage vB-GEC_Ab-M-G7 as an antibacterial agent. Front. Microbiol. 7:1590. doi: 10.3389/fmicb.2016.01590
Kutateladze, M. (2015). Experience of the eliava institute in bacteriophage therapy. Virol. Sinica 30, 80–81. doi: 10.1007/s12250-014-3557-0
Kutter, E., De Vos, D., Gvasalia, G., Alavidze, Z., Gogokhia, L., Kuhl, S., et al. (2010). Phage therapy in clinical practice: treatment of human infections. Curr. Pharm. Biotechnol. 11, 69–86. doi: 10.2174/138920110790725401
Larpin, Y., Oechslin, F., Moreillon, P., Resch, G., Entenza, J. M., and Mancini, S. (2018). In vitro characterization of PlyE146, a novel phage lysin that targets Gram-negative bacteria. PLoS ONE 13:e0192507. doi: 10.1371/journal.pone.0192507
Lavergne, S., Hamilton, T., Biswas, B., Kumaraswamy, M., Schooley, R. T., and Wooten, D. (2018). Phage therapy for a multidrug-resistant Acinetobacter baumannii craniectomy site infection. Open Forum Infect. Dis. 5:ofy064. doi: 10.1093/ofid/ofy064
Leitner, L., Sybesma, W., Chanishvili, N., Goderdzishvili, M., Chkhotua, A., Ujmajuridze, A., et al. (2017). Bacteriophages for treating urinary tract infections in patients undergoing transurethral resection of the prostate: a randomized, placebo-controlled, double-blind clinical trial. BMC Urol. 17:90. doi: 10.1186/s12894-017-0283-6
Liu, Y., Mi, Z., Niu, W., An, X., Yuan, X., Liu, H., et al. (2016). Potential of a lytic bacteriophage to disrupt Acinetobacter baumannii biofilms in vitro. Future Microbiol. 11, 1383–1393. doi: 10.2217/fmb-2016-0104
Lood, R., Winer, B. Y., Pelzek, A. J., Diez-Martinez, R., Thandar, M., Euler, C. W., et al. (2015). Novel phage lysin capable of killing the multidrug-resistant Gram-negative bacterium Acinetobacter baumannii in a mouse bacteremia model. Antimicrob Agents Chemother. 59, 1983–1991. doi: 10.1128/AAC.04641-14
Lyczak, J. B., Cannon, C. L., and Pier, G. B. (2000). Establishment of Pseudomonas aeruginosa infection: lessons from a versatile opportunist. Microbes Infect. 2, 1051–1060. doi: 10.1016/S1286-4579(00)01259-4
Mcconnell, M. J., Actis, L., and Pachón, J. (2013). Acinetobacter baumannii: human infections, factors contributing to pathogenesis and animal models. FEMS Microbiol. Rev. 37, 130–155. doi: 10.1111/j.1574-6976.2012.00344.x
Miedzybrodzki, R., Borysowski, J., Weber-Dabrowska, B., Fortuna, W., Letkiewicz, S., Szufnarowski, K., et al. (2012). Clinical aspects of phage therapy. Adv. Virus Res. 83, 73–121. doi: 10.1016/B978-0-12-394438-2.00003-7
Olszak, T., Zarnowiec, P., Kaca, W., Danis-Wlodarczyk, K., Augustyniak, D., Drevinek, P., et al. (2015). In vitro and in vivo antibacterial activity of environmental bacteriophages against Pseudomonas aeruginosa strains from cystic fibrosis patients. Appl. Microbiol. Biotechnol. 99, 6021–6033. doi: 10.1007/s00253-015-6492-6
O'neill, J. (2014). Antimicrobial resistance: tackling a crisis for the health and wealth of nations. Rev. Antimicrob. Resist. Available online at: http://amr-review.org/
Parracho, H. M., Burrowes, B. H., Enright, M. C., Mcconville, M. L., and Harper, D. R. (2012). The role of regulated clinical trials in the development of bacteriophage therapeutics. J. Mol. Genet. Med. 6, 279–286. doi: 10.4172/1747-0862.1000050
Payne, R. J., and Jansen, V. A. (2003). Pharmacokinetic principles of bacteriophage therapy. Clin. Pharmacokinet 42, 315–325. doi: 10.2165/00003088-200342040-00002
Pelfrene, E., Willebrand, E., Cavaleiro Sanches, A., Sebris, Z., and Cavaleri, M. (2016). Bacteriophage therapy: a regulatory perspective. J. Antimicrob. Chemother. 71, 2071–2074. doi: 10.1093/jac/dkw083
Peng, S. Y., You, R. I., Lai, M. J., Lin, N. T., Chen, L. K., and Chang, K. C. (2017). Highly potent antimicrobial modified peptides derived from the Acinetobacter baumannii phage endolysin LysAB2. Sci. Rep. 7:11477. doi: 10.1038/s41598-017-11832-7
Pirnay, J. P., Blasdel, B. G., Bretaudeau, L., Buckling, A., Chanishvili, N., Clark, J. R., et al. (2015). Quality and safety requirements for sustainable phage therapy products. Pharm. Res. 32, 2173–2179. doi: 10.1007/s11095-014-1617-7
Pirnay, J. P., Verbeken, G., Ceyssens, P. J., Huys, I., De Vos, D., Ameloot, C., et al. (2018). The magistral phage. Viruses 10:E64. doi: 10.3390/v10020064
Regeimbal, J. M., Jacobs, A. C., Corey, B. W., Henry, M. S., Thompson, M. G., Pavlicek, R. L., et al. (2016). Personalized therapeutic cocktail of wild environmental phages rescues mice from Acinetobacter baumannii wound infections. Antimicrob. Agents Chemother. 60, 5806–5816. doi: 10.1128/AAC.02877-15
Rhoads, D. D., Wolcott, R. D., Kuskowski, M. A., Wolcott, B. M., Ward, L. S., and Sulakvelidze, A. (2009). Bacteriophage therapy of venous leg ulcers in humans: results of a phase I safety trial. J. Wound Care 18, 237–238, 240–233. doi: 10.12968/jowc.2009.18.6.42801
Roach, D. R., Leung, C. Y., Henry, M., Morello, E., Singh, D., Di Santo, J. P., et al. (2017). Synergy between the host immune system and bacteriophage is essential for successful phage therapy against an acute respiratory pathogen. Cell Host Microbe 22, 38–47.e34. doi: 10.1016/j.chom.2017.06.018
Rossitto, M., Fiscarelli, E. V., and Rosati, P. (2018). Challenges and promises for planning future clinical research into bacteriophage therapy against Pseudomonas aeruginosa in cystic Fibrosis. An argumentative review. Front. Microbiol. 9:775. doi: 10.3389/fmicb.2018.00775
Sarker, S. A., Sultana, S., Reuteler, G., Moine, D., Descombes, P., Charton, F., et al. (2016). Oral phage therapy of acute bacterial diarrhea with two coliphage preparations: a randomized trial in children from Bangladesh. EBioMedicine 4, 124–137. doi: 10.1016/j.ebiom.2015.12.023
Schooley, R. T., Biswas, B., Gill, J. J., Hernandez-Morales, A., Lancaster, J., Lessor, L., et al. (2017). Development and use of personalized bacteriophage-based therapeutic cocktails to treat a patient with a disseminated resistant Acinetobacter baumannii infection. Antimicrob Agents Chemother. 61:e00954–17. doi: 10.1128/AAC.00954-17
Shiley, J. R., Comfort, K. K., and Robinson, J. B. (2017). Immunogenicity and antimicrobial effectiveness of Pseudomonas aeruginosa specific bacteriophage in a human lung in vitro model. Appl. Microbiol. Biotechnol. 101, 7977–7985. doi: 10.1007/s00253-017-8504-1
Soothill, J. (2013). Use of bacteriophages in the treatment of Pseudomonas aeruginosa infections. Expert Rev. Anti Infect Ther. 11, 909–915. doi: 10.1586/14787210.2013.826990
Soothill, J. S. (1992). Treatment of experimental infections of mice with bacteriophages. J. Med. Microbiol. 37, 258–261. doi: 10.1099/00222615-37-4-258
Speck, P., and Smithyman, A. (2016). Safety and efficacy of phage therapy via the intravenous route. FEMS Microbiol. Lett. 363:fnv242. doi: 10.1093/femsle/fnv242
Tacconelli, E., Carrara, E., Savoldi, A., Harbarth, S., Mendelson, M., Monnet, D. L., et al. (2018). Discovery, research, and development of new antibiotics: the WHO priority list of antibiotic-resistant bacteria and tuberculosis. Lancet Infect Dis. 18, 318–327. doi: 10.1016/S1473-3099(17)30753-3
Thandar, M., Lood, R., Winer, B. Y., Deutsch, D. R., Euler, C. W., and Fischetti, V. A. (2016). Novel engineered peptides of a phage lysin aseffective antimicrobials against multidrug-resistant Acinetobacter baumannii. Antimicrob Agents Chemother. 60, 2671–2679. doi: 10.1128/AAC.02972-15
Tkhilaishvili, T., Di Luca, M., Abbandonato, G., Maiolo, E. M., Klatt, A. B., Reuter, M., et al. (2018). Real-time assessment of bacteriophage T3-derived antimicrobial activity against planktonic and biofilm-embedded Escherichia coli by isothermal microcalorimetry. Res. Microbiol. doi: 10.1016/j.resmic.2018.05.010. [Epub ahead of print].
Vahedi, A., Dallal, M. M. S., Douraghi, M., Nikkhahi, F., Rajabi, Z., Yousefi, M., et al. (2018). Isolation and identification of specific bacteriophage against enteropathogenic Escherichia coli (EPEC) and in vitro and in vivo characterization of bacteriophage. FEMS Microbiol. Lett. 365:fny136. doi: 10.1093/femsle/fny136
Verbeken, G., Pirnay, J. P., Lavigne, R., Jennes, S., De Vos, D., Casteels, M., et al. (2014). Call for a dedicated European legal framework for bacteriophage therapy. Arch. Immunol. Ther. Exp. 62, 117–129. doi: 10.1007/s00005-014-0269-y
Villarroel, J., Larsen, M. V., Kilstrup, M., and Nielsen, M. (2017). Metagenomic analysis of therapeutic PYO phage cocktails from 1997 to 2014. Viruses 9:E328. doi: 10.3390/v9110328
Waters, E. M., Neill, D. R., Kaman, B., Sahota, J. S., Clokie, M. R. J., Winstanley, C., et al. (2017). Phage therapy is highly effective against chronic lung infections with Pseudomonas aeruginosa. Thorax 72:666. doi: 10.1136/thoraxjnl-2016-209265
Wright, A., Hawkins, C. H., Anggard, E. E., and Harper, D. R. (2009). A controlled clinical trial of a therapeutic bacteriophage preparation in chronic otitis due to antibiotic-resistant Pseudomonas aeruginosa; a preliminary report of efficacy. Clin. Otolaryngol. 34, 349–357. doi: 10.1111/j.1749-4486.2009.01973.x
Yan, G., Liu, J., Ma, Q., Zhu, R., Guo, Z., Gao, C., et al. (2017). The N-terminal and central domain of colicin A enables phage lysin to lyse Escherichia coli extracellularly. Antonie Van Leeuwenhoek 110, 1627–1635. doi: 10.1007/s10482-017-0912-9
Yin, S., Huang, G., Zhang, Y., Jiang, B., Yang, Z., Dong, Z., et al. (2017). Phage Abp1 rescues human cells and mice from infection by pan-drug resistant Acinetobacter baumannii. Cell Physiol. Biochem. 44, 2337–2345. doi: 10.1159/000486117
Keywords: bacteriophage, phage therapy, regulations, clinical trials, antimicrobial resistance, alternative treatments
Citation: Furfaro LL, Payne MS and Chang BJ (2018) Bacteriophage Therapy: Clinical Trials and Regulatory Hurdles. Front. Cell. Infect. Microbiol. 8:376. doi: 10.3389/fcimb.2018.00376
Received: 11 August 2018; Accepted: 05 October 2018;
Published: 23 October 2018.
Edited by:
Maria Tomas, Complexo Hospitalario Universitario A Coruña, SpainReviewed by:
Katrine L. Whiteson, University of California, Irvine, United StatesJose Ramos-Vivas, Instituto de Investigación Marques de Valdecilla (IDIVAL), Spain
Copyright © 2018 Furfaro, Payne and Chang. This is an open-access article distributed under the terms of the Creative Commons Attribution License (CC BY). The use, distribution or reproduction in other forums is permitted, provided the original author(s) and the copyright owner(s) are credited and that the original publication in this journal is cited, in accordance with accepted academic practice. No use, distribution or reproduction is permitted which does not comply with these terms.
*Correspondence: Lucy L. Furfaro, lucy.furfaro@uwa.edu.au