- 1Departamento de Infectómica y Patogénesis Molecular, CINVESTAV-IPN, Mexico City, Mexico
- 2Área Académica de Gerontología, Instituto de Ciencias de la Salud, Universidad Autónoma del Estado de Hidalgo, Pachuca, Mexico
Entamoeba histolytica is the etiologic agent of human amoebiasis, disease that causes 40,000 to 100,000 deaths annually worldwide. The cytopathic activity as well as the growth and differentiation of this microorganism is dependent on both, extracellular and free cytoplasmic calcium. However, few is known about the proteins that regulate the calcium flux in this parasite. In many cells, the calcium extrusion from the cytosol is performed by plasma membrane Ca2+-ATPases and calcium/cation exchangers. The aim of this work was to identify a calcium/cation exchanger of E. histolytica and to analyze its possible role in some cellular processes triggered by calcium flux, such as the programmed cell death and in vitro virulence. By searching putative calcium/cation exchangers in the genome database of E. histolyica we identified a protein belonging to the CCX family (EhCCX). We generated a specific antibody against EhCCX, which showed that this protein was expressed in higher levels in E. histolytica than its orthologous in the non-pathogenic amoeba E. dispar. In addition, the expression of EhCCX was increased in trophozoites incubated with hydrogen peroxide. This E. histolytica exchanger was localized in the plasma membrane and in the membrane of some cytoplasmic vesicles. However, after 10 min of erythrophagocytosis, EhCCX was found predominantly in the plasma membrane of the trophozoites. On the other hand, the parasites that overexpress this exchanger contained higher cytosolic calcium levels than control, but the extrusion of calcium after the addition of hydrogen peroxide was more efficient in EhCCX-overexpressing trophozoites; consequently, the programmed cell death was retarded in these parasites. Interestingly, the overexpression of EhCCX increased the in vitro virulence of trophozoites. These results suggest that EhCCX plays important roles in the programmed cell death and in the in vitro virulence of E. histolytica.
Introduction
The ion calcium (Ca2+) is a highly versatile secondary messenger that operates over a wide temporal range to regulate many different cellular processes (Berridge et al., 2003). Thus, the concentration of Ca2+ in cytosol and some organelles, such as the endoplasmic reticulum, the Golgi apparatus, and nucleus, is strongly regulated by channels, transporters and pumps, which dynamically adjust the Ca2+ amount in agreement with a specific physiological demand (Berridge et al., 2003). In many cells Ca2+ extrusion from the cytosol is performed by plasma membrane Ca2+-ATPases (PMCAs) and by Ca2+/cations exchangers (Berridge et al., 2003). PMCAs have high Ca2+ affinity, but low turnover rates, while exchangers have a lower Ca2+ affinity, but higher turnover rates (Blaustein and Lederer, 1999). Consequently, PMCAs are involved in the maintenance of the resting cytoplasmic concentration of Ca2+, whereas exchangers are important in the restoration of cytoplasmic concentration of Ca2+ after it has been elevated during signaling (Harper and Sage, 2016). Interestingly, exchangers also participate in the entry of Ca2+ to cytoplasm, since they can reverse its transport in response to changes in the concentration of the transported ions and membrane potential (Harper and Sage, 2016). The superfamily of calcium exchangers comprises five branches (Lytton, 2007; Emery et al., 2012): (i) H+/ Ca2+ exchangers (CAX), mainly found in plants; (ii) bacteria exchangers (YRGB); (iii) Na+/Ca2+ exchangers (NCX or SLC8); (iv) Na+/Ca2+ + K+ exchangers (NCKX or SLC24); and (v) Ca2+/cation exchangers (CCX), which contains one mammalian member, the Na+/Ca2+ or Li+ exchanger (NCLX) that is also named as NCKX6.
Entamoeba histolytica is the etiological agent of human amoebiasis, a disease that produces 40,000 to 100,000 deaths per year worldwide (Stanley, 2003). The cytolytic activity of this parasite is dependent on both, extracellular and free cytoplasmic Ca2+ (Ravdin et al., 1982, 1985). In addition, Ca2+ flux participates in the adherence of trophozoites to fibronectin (Carbajal et al., 1996), as well as in growth and differentiation of E. histolytica and E. invadens (Makioka et al., 2001, 2002; Martínez-Higuera et al., 2015). However, little is known about the proteins that regulate the Ca2+ flux in this parasite. Our previous studies showed that E. histolytica contains at least five Ca2+-ATPases: three related to PMCAs, one to Sarco/Endoplasmic reticulum Ca2+-ATPases (SERCA), and another to Secretory Pathway Ca2+-ATPases (SPCA) (Martinez-Higuera et al., 2013; Rodríguez et al., 2018). Indeed, we detected the SPCA-related pump in the Golgi apparatus of E. histolytica (Rodríguez et al., 2018) and the SERCA-related pump in the endoplasmic reticulum of E. histolytica and E. invadens (Martinez-Higuera et al., 2013; Martínez-Higuera et al., 2015). In addition, we demonstrated that specific inhibitors of SERCA affected the encystation of E. invadens (Martínez-Higuera et al., 2015), suggesting that calcium flux through SERCA is involved in the development of Entamoeba sp. However, other proteins involved in the Ca2+ movement, such as channels or exchangers, and their role in the Enatamoeba biology have not been described.
In this work, we identified a calcium/cation exchanger of E. histolytica related to members of the CCX family (EhCCX). This exchanger was higher expressed in E. histolytica that its orthologous in the non-pathogenic amoeba E. dispar. EhCCX expression was increased in trophozoites incubated with hydrogen peroxide. The exchanger was located in the plasma membrane of trophozoites and in the membrane of some cytoplasmic vesicles, but after 10 min of erythrophagocytosis, EhCCX was mainly detected in the plasma membrane. On the other hand, the overexpression of EhCCX augmented the cytosolic calcium levels under basal conditions, but the calcium extrusion after the addition of hydrogen peroxide was more efficient. In addition, the overexpression of EhCCX retarded the programmed cell death and increased the in vitro virulence. These results suggest that the Ca2+ flux through EhCCX plays an important role in the programmed cell death and the virulence of E. histolytica.
Materials and Methods
Entamoeba Cultures
Trophozoites of E. histolytica clone A, strain HM1:IMSS (Orozco et al., 1983) were axenically cultured in TYI-S-33 medium (Diamond et al., 1978), whereas trophozoites of E. dispar (strain SAW 760) were axenically cultured in YI-S medium (Diamond et al., 1995). Cells were harvested during the logarithmic growth phase as previously described (Diamond et al., 1978).
Identification and in silico Characterization of a Calcium/Cation Exchanger of E. histolytica
To identify possible calcium/cation exchangers in Entamoeba spp., a BLAST search was performed on the databases of the Amoeba Genomics Resource (http://amoebaDb.org/amoeba/) using as probes the α1 and α2 repeats of human calcium/sodium exchangers (NCX, NCKX, and NCLX). These motifs are characteristics of these transporters and participate in the ions transport (Philipson and Nicoll, 2000). Then, the retrieved protein of E. histolytica was characterized in silico using the software deposited in the Expasy Bioinformatics Resource Portal (http://expasy.org) and in the NCBI Home Page (http://www.ncbi.nlm.nih.gov). The amino acid sequence of this protein was compared, by ClustalW, with sequences of proteins belonging to the different families of calcium exchangers (CAX, YRGB, NCX, NCKX, and CCX). Then, a phylogenetic analysis was performed using the Unweighted Pair Group Method with Arithmetic Mean (UPGMA) employing the MEGA 5.05 software package (Tamura et al., 2011). Bootstrapping was performed for 1000 replicates.
The 3D molecular model was built with the I-TASSER server (http://zhanglab.ccmb.med.umich.edu/I-TASSER) (Zhang, 2008) and with the Raptor X Structure Prediction (http://raptorx.uchicago.edu) using as a template the crystalized structure of the Methanococcus jannaschii NCX (Protein Data Bank: 3V5S) (Liao et al., 2012).
PCR and RT-PCR
The genomic DNA of E. histolytica was obtained with the Wizard Genomic DNA purification kit (Promega), following the manufacturer's recommendations. Total RNA was isolated using the Trizol reagent (Invitrogen), following the manufacturer's recommendations, and cDNA was synthesized using an oligo dT primer and the Superscrip II reverse transcriptase (Invitrogen). Amplifications of the Ehccx full-length gene was performed in a select Cycler (Select BioProducts) using 200 ng of DNA or cDNA and specific primers situated at the 5′- and 3′-ends of the gene and containing the BamHI and KpnI restriction sites, respectively (forward, 5′- CCCCGGTACCATGAAACAGATGAATAAAATTTATATTATATTA-3′; reverse, 5′-CCCCGGATCCTTAACCAAACAGTTTAAAAACGTTAA-3′). The assays were performed in a 50 μl volume reaction containing 1 μM of each primer, dNTPs 1.5 mM, MgCl2 2 mM, and 1 U of the high-fidelity enzyme KAPA HiFi DNA polymerase (KAPABIOSYSTEMS). Amplification cycles comprised: (i) 1 min of denaturing step at 94°C; (ii) 35 cycles of 1 min of denaturing step at 94°C, 1 min of annealing step at 55°C; and 3 min of elongation step at 72°C; and (iii) 10 min of elongation step at 72°C. The amplified products were analyzed by electrophoresis in 1% agarose gels.
Production of the α-EhCCX Antibody and Western Blot
To obtain antigenic peptides of the predicted calcium/cation exchanger of E. histolytica (EhCCX), its amino acid sequence was analyzed by the ABCpred program (http://www.imtech.res.in/raghava/abcpred/). Peptides with higher scores were used as probes in BLAST searches on the E. histolytica genome database; then, a specific peptide at position 216-229 (ISEQLDSENKTKLI) was synthesized (GL Biochem) linked to the KLH (Keyhole limpet Hemocyanin) tag to increase its immunogenicity. Next, Wistar rats were intradermally immunized three times, in an interval of 2 weeks, with 100 μg of the synthetic polypeptide resuspended in Titermax Gold adjuvant (1:1) (Sigma). All animals used in this study were handled in accordance with the guidelines of the Institutional Animal Care and Use Committee. Our institution fulfills all the technical specifications for the production, care and use of laboratory animals and it is certified by national law (NOM-062-ZOO-1999).
For Western blot assays, total extracts of E. histolytica or E. dispar trophozoites obtained in the presence of protease inhibitors (Complete Mini, Roche-Mannheim) were separated by 10% SDS-PAGE, transferred to nitrocellulose membranes and probed with the α-EhCCX antibody (1: 3,000). Then membranes were incubated with a peroxidase-coupled secondary antibody (1: 10,000) (ZYMED) and finally, the reaction was developed by chemiluminescence (ECL Plus GE-Healthcare). To compare the expression level of EhCCX and EdCCX or to analyze the expression level of EhCCX in trophozoites under different conditions (in the presence of H202 1 mM for 10 min, or incubated at 42°C for 30 and 60 min) we performed Western blot assays using the α-EhCCX antibody. Then, membranes were exposed to an α-actin antibody (1: 20,000) (kindly provided by Dr. Jose Manuel Hernandez-Hernandez at CINVESTAV-IPN, Mexico), used as an internal control of loading. The band detected by the α-EhCCX antibody was analyzed by scanning densitometry and the data were normalized to the actin content according to the reactivity of the α-actin antibody. For semi-quantitative comparisons, the protein level in E. histolytica trophozoites under basal conditions was arbitrary taken as 1.
Immunofluorescence and Confocal Microscopy
Trophozoites grown on coverslides were fixed and permeabilized with methanol for 10 min and non-specific binding sites were blocked with 10% FBS in phosphate buffered saline (PBS). Then, cells were incubated for 1 h at 37°C with the rat antibody against EhCCX (1:50 dilution). After several washes with PBS, samples were incubated with an Alexa 555-conjugated secondary antibody (Zymed) (1:400). Finally, the nuclei were stained with 4',6-diamidino-2-Phenylindole (DAPI) and samples were observed through a confocal microscope (Carl Zeiss LSM 700). Observations were performed in ~20 optical sections from the top to the bottom of each sample. For the immunolocalization of EhCCX during phagocytosis, before performing the immunofluorescence, trophozoites were incubated with fresh human red blood cells (RBCs) from healthy donors (1:25 ratio) for 5, 10, and 15 min at 37°C. As a control, cells were incubated in the presence of EGTA 0.3 mM.
For co-localization assays, after incubation with α-EhCCX, the samples were incubated with rabbit antibodies against EhSERCA (Martinez-Higuera et al., 2013) (dilution 1:50), EhRabB (Rodriguez et al., 2000) (dilution 1:25), or EhNPC-1 (Bolaños et al., 2016) (dilution 1:100); or with mouse antibodies against the Gal/GalNac lectin (Petri et al., 1989) (dilution 1:50). Subsequently, the samples were incubated with anti-rat IgGs labeled with Alexa 555, and with anti-rabbit IgGs labeled with Alexa 488, or anti-mouse IgGs labeled with FITC (Zymed; 1:100) as appropriate. For detection of the Golgi apparatus, trophozoites were incubated with 5 μM of NBD C6-Ceramide (Thermo Fisher Scientific) for 90 min at 37°C. To evaluate the co-localization between molecules, Pearson coefficients were obtained from at least 15 confocal independent images (laser sections: 0.5 μm) using the ImageJ 1.45v software and the JACoP plugin.
Immunoelectron Microscopy
Immunoelectron microscopy assays were performed as described (Segovia-Gamboa et al., 2011). E. histolytica trophozoites were fixed in 4% paraformaldehyde and 0.1% glutaraldehyde in serum-free DMEM for 1 h at room temperature. Samples were embedded in LR white resin (London Resin Co) and polymerized under UV at 4°C for 48 h. Then, thin sections (60 nm) were mounted on formvar-covered nickel grids followed by overnight incubation with the α-EhNCX (1:20) antibody and later, by overnight incubation with the secondary antibody (1:60) conjugated to 15 nm gold particles (Ted Pella Inc.). Finally, sections were observed with a morgana 268 D Philips transmission electron microscope (FEI Company).
Overexpression of the EhCCX Gene
The full-length Ehccx gene was obtained by PCR using genomic DNA as template and using the conditions described above. Then, the amplicon was sequenced and cloned into the BamHI and Kpnl restriction sites of the pExEhNeo (pNeo) plasmid (Hamann et al., 1995), obtaining the pNeo/Ehccx construct.
For transfection, 3 × 105 trophozoites were cultivated overnight at 37°C in a 5% CO2 environment; then, cells were washed with M199 medium (Sigma), resuspended in 1.8 ml of M199 medium supplemented with 15% of fetal bovine serum (FBS), and placed in 35-mm Petri dishes. Subsequently, a mix of 10 μg of plasmid (pNeo or pNeo/Ehccx) and 10 μg of Superfect (Qiagen) in 100 μl of M199 medium was added to the cultures and incubated for 4 h at 37°C in a 5% CO2 environment. Afterwards, cells were transferred to a tube containing 10 ml of TYI-S-33 pre-warmed medium and incubated 48 h at 37°C. Finally, transfected parasites were selected in the presence of 10 μg/ml of G-418 (Sigma-Aldrich). Overexpression was confirmed by qRT-PCR and Western blot assays.
For qRT-PCR, 100 ng of cDNA, 0.15 μM of each primer (forward, 5′-CACTGAAACACAAATCCCTTC-3′; reverse, 5′-CCAACTGAATTTCCCCAACA-3′) and the KAPA SYBR FAST PCR Master Mix (Kapa Biosystems) were used in a StepOne TM Real-Time PCR System (Applied Biosystem). As normalizer we used the gene encoding for the protein S2 of the 40S ribosomal subunit (primers: forward, 5′-ATTCGGAAATAGAAGAGGAGG-3′; reverse, 5′- CTATTCTTCCAAGCTTGGT-3′). Data from three independent cDNA preparations were analyzed using the 2−ΔΔCT method.
Western blot assays and semi-quantitative comparisons were performed as described above to analyze the expression level of the EhCCX protein in transfected trophozoites. The protein level in trophozoites transfected with the empty vector (pNeo) was arbitrary taken as 1.
Intracellular Ca2+ Levels
The trophozoites (1 × 106) were suspended in 1 ml of PBS and incubated for 30 min at 37°C in the dark with 4 μM of the Ca2+-sensitive dye Fluo-4 AM (Invitrogen). To remove the extracellular dye, the parasites were washed three times with PBS and resuspended in 1 ml of Ca2+/Mg2+ PBS (CaCl2 0.1 mM, MgCl2 1 mM). The fluorescence signal was detected by flow cytometry using the 520/40 filters in a BD FACSCalibur analyzer (BD Biosciences). To analyze the cytosolic calcium levels during the programmed cell death, H2O2 1 mM was added to the samples and the fluorescence intensity was measured every 40 s.
Cell Death Induced by Hydrogen Peroxide
The trophozoites (5 × 105) were treated with H2O2 1 mM in serum-free TYI medium and incubated at 37°C. Then, the cell viability was measured at different times by Trypan Blue exclusion.
Erythrophagocytosis
Trophozoites suspended in serum-free TYI medium were incubated with fresh RBCs (1:100 ratio) at 37°C with slight agitation for 5, 10, and 15 min. Non-ingested erythrocytes were lysed by incubation with distilled water for 10 min at room temperature. Then, the samples were washed three times with PBS and parasites with internalized RBCs, were lysed with 1 ml of concentrated formic acid (J.T. Baker). Finally, phagocytosis was determined by measuring the hemoglobin released by the ingested erythrocytes (absorbance at 405 nm using a Beckman Coulter DU800 spectrophotometer).
Cytopathic Assays
Cytopathic assays, defined as the ability of live trophozoites to destroy cultured cells, were carried out as previously described (Rodríguez and Orozco, 1986). Briefly, MDCK cell monolayers (1 × 105 cells) were incubated for 2 h at 37°C with 1 × 105 trophozoites. Then, the trophozoites were eliminated by incubation at 4°C during 10 min, and the remaining mammalian cells were fixed with 2.5% (v/v) glutaraldehyde and stained with 1% (w/v) methylene blue. After exhaustive washes, the dye captured by cells was extracted with 0.1 M HCl and measured in a spectrophotometer (Beckman Coulter DU800) at 660 nm.
Migration Assays
Serum-starved (3 h) trophozoites (7.5 × 104) were placed in the upper chamber of transwell inserts (5 μm pore size, 24 well, Costar) and 500 μl of adult bovine serum was added to the lower chamber. Then, trophozoites were incubated for 3 h at 37°C and migration was determined by counting the number of trophozoites at the lower chamber of the well.
Statistical Analysis
Values of all assays were expressed as the mean ± standard error of three independent experiments by duplicate. Statistical analyzes were carried out using the GraphPad Prism V5.01 software by two-way ANOVA and Student's t-test.
Results
E. histolytica Has a Calcium Exchanger Belonging to the CCX Family
We search for putative calcium/cation exchangers in the Amoeba Genomics Resource (http://www.amoebaDb.org) using as probes the α1 and α2 domains of the different human calcium/cation exchangers (NCX, NCKX, or NCLX). By this analysis, the α1 domains of NCLX and NCX2, as well as the α2 domains of NCLX, NCKX1, and NCKX2 retrieved just one putative cation/calcium exchanger in E. histolytica (EHI_001770), E. nuttali (ENVI_134420), E. dispar (EDI_138590), and E. invadens (EIN_083040), whereas they retrieved two exchangers of E. moshkovskii (EMO_063580 and EMO_049830). The identity of the E. histolytica exchanger with the orthologous proteins of the other Entamoeba species ranged from 69 to 99%, whereas similarity varied from 83 to 99% (Table 1). On the other hand, the E. histolytica exchanger displayed 16–30% identity and 42–51% similarity with calcium/sodium exchangers from plants, mammals, amphibians, insects, and bacteria (Table 1).
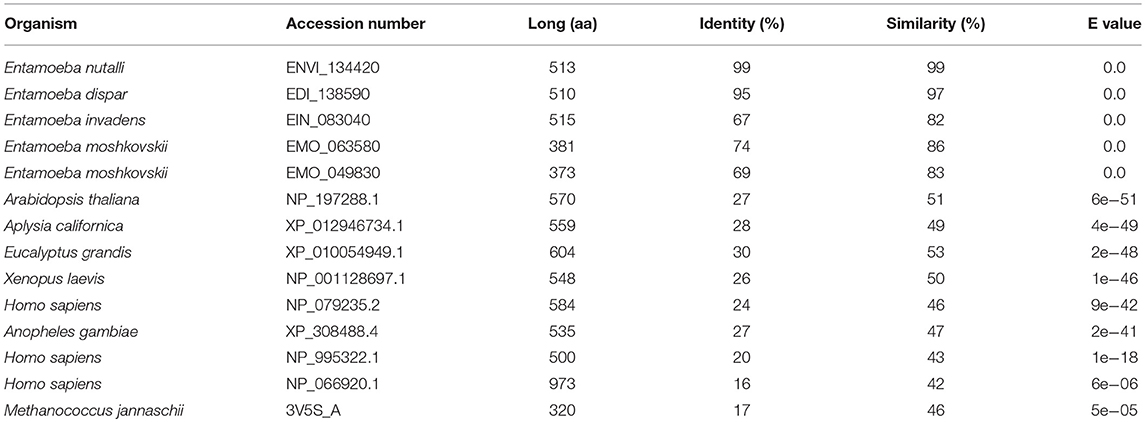
Table 1. Comparison of the calcium/sodium exchanger of E. histolytica with orthologous proteins of different organisms.
The putative calcium/cation exchanger of E. histolytica has 513 amino acid residues. It contains a signal peptide in positions 1 to 19 and its secondary structure model showed a similar organization to the human NCLX protein (NP_079235.2) (Figure 1A). Both proteins contain: (i) two sodium/calcium exchanger (NCX) domains (positions 62-206 and 352-503 in EHI_001770) that include the α1 and α2 repeats (positions 101-141 and 391-447 in EHI_001770); and (ii)10 putative transmembrane segments (positions 65-75, 108-126, 127-147, 165-181, 187-200, 363-382, 387-403, 422-444, 461-479, and 484-501 in EHI_001770) (Figure 1A). Then, we constructed a predicted 3D model of the putative E. histolytica exchanger using the crystallized structure of the sodium/calcium exchanger of Methanococcus jannaschii (MjNCX) as template. This 3D model confirmed the presence of 10 transmembrane segments in the amoebic protein, but it showed a modest structural identity with MjNCX (9.38%) and with the human NCLX (11.13%) (Figure 1B). However, the structural identity between human and bacteria exchangers was also moderate (17.19%) (Figure 1B). Nevertheless, the predicted structures of the α1 and α2 repeats of the E. histolytica protein showed a higher identity with those of NCLX (43.9 and 43.14%, respectively) (Figure 1B).
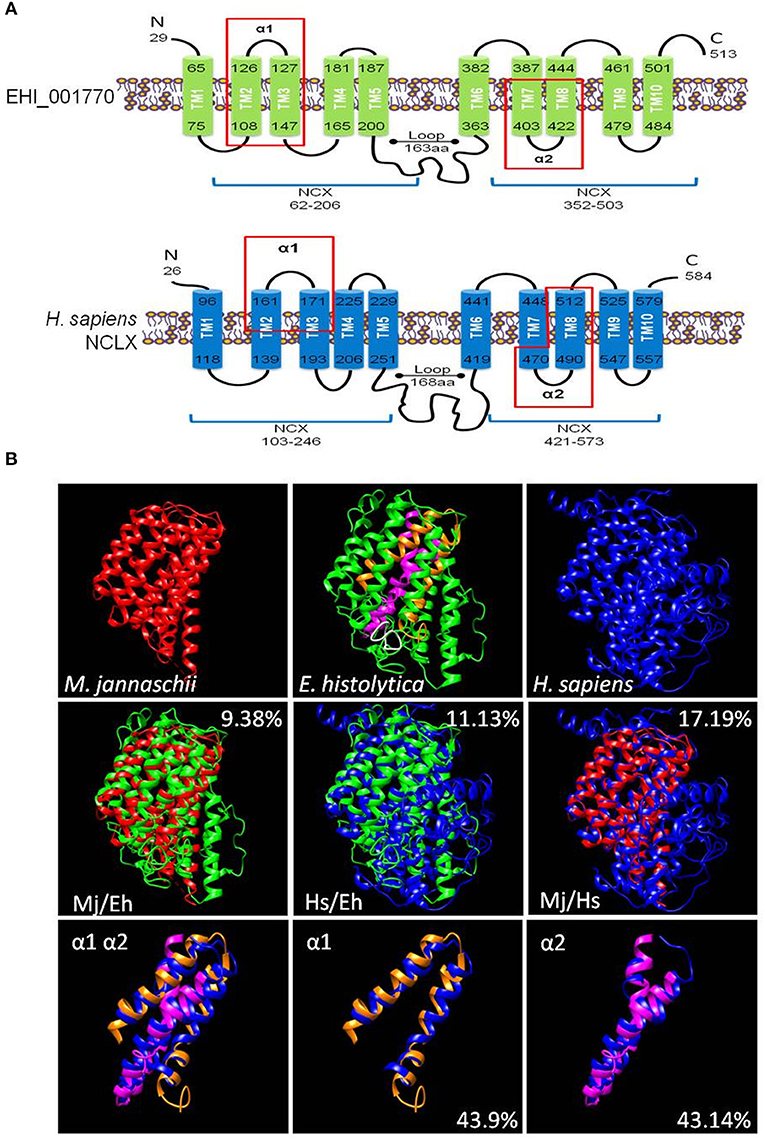
Figure 1. In silico characterization of a calcium/cation exchanger of E. histolytica. (A) Secondary structure models of the putative calcium/cation exchanger of E. histolytica and the human NCLX. The signal peptides were eliminated in the models. Numbers indicate the amino acid position. Bars represent the transmembrane regions (TM1 to TM10). The protein segments that correspond to the NCX domains are indicated by blue lines below the models. Red rectangles showed the protein segments corresponding to the α1 and α2 repeats. (B) The 3D models of the E. histolytica exchanger (green) and the human NCLX (blue) were predicted by the I-TASSER server using the crystallized sodium/calcium exchanger of Methanococcus jannaschii NCX (red) as a template. The white portion in the 3D model of the E. histolytica protein represents the peptide used to obtain specific antibodies against it, whereas orange and magenta segments correspond to the α1 and α2 repeats, respectively. The overlapping of the 3D models of the whole proteins as well as of the α1 and α2 domains are also shown. Numbers indicate the percentage of structural identity.
To determine the family of calcium exchangers to which the putative E. histolytica protein belongs, we compared its amino acid sequence with those of different members of the YRGB (bacterial exchangers), NCX (Ca2+/Na+ exchangers), NCKX (Ca2+/Na+ + K+ exchangers), CAX (Ca2+/H+ exchangers), and CCX (Ca2+/cation exchangers) families. Then, we constructed a phylogenetic tree, where the proteins of the different Entamoeba species were clustered into the clade comprising the CCX family, whereas the exchangers of other protozoa parasites were grouped in the clade of the CAX family (Figure 2A). Moreover, the E. histolytica exchanger contains the sequences GNG(A/T)PD and GNSIGD, corresponding to distinctive motives of the CCX family, which include the Ca2+/Na+ or Li+ exchangers (NCLX) (Sharma and O'Halloran, 2014) (Figure 2B). Concordantly, the E. histolytica protein shares all the amino acid residues that were identified as responsible of transporting Ca2+, Na+, or Li+ in the human NCLX (Roy et al., 2017) (Figure 2B); therefore, we named the E. histolytica exchanger as EhCCX.
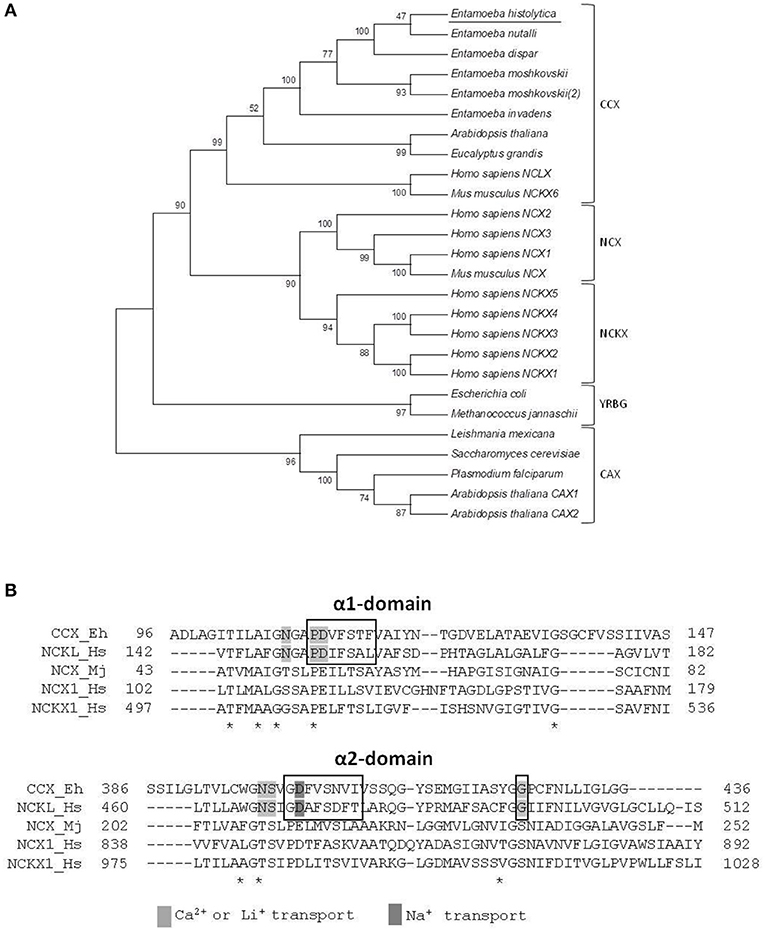
Figure 2. Phylogenetic analysis of the E. histolytica exchanger. (A) The predicted amino acid sequence of the E. histolytica exchanger was aligned by ClustalW with sequences of several calcium/cation exchangers from different organisms and data were submitted to phylogenetic analysis by UPGMA using the MEGA 5.05 software package. The protein sequences used for this analysis were: E. histolytica (accession number, EHI_001770); E. nutalli (ENVI_134420); E. dispqr (EDI_138590); E. invadens (EIN_083040); E. moshcovskii (EMO_063580 and EMO_049830); Homo sapiens NCXL (NP_079235.2), NCKX 1-5 (NP_004718.1, NP_065077.1, NP_065740.2, NP_705932.2, and NP_995322.1), and NCX1-3 (NP_066920.1, NP_055878.1, and NP_150287.1); Mus musculus NCKX6 (NP_573484.2) and NCX (AAB69167.1); Arabidopsis thaliana NCKX (NP_197288.1), and CAX1-2 (NP_181352.1 and NP_566452.1); Eucalyptus grandis CCX (XP_010054949.1); Trichomonas vaginalis CAX (XP_001582908.1); Plasmodium falciparum CAX (SCP05226.1); Leishmania Mexicana CAX (XP_003873397.1); Saccharomyces cerevisiae CVX1 (NP_010155.1); Escherichia coli NCX (WP_000922901.1); and Methanococcus jannaschii NCX (3V5S_A). Numbers at the branch nodes indicate the confidence percentages of the tree topology from bootstrap analysis of 1000 replicates. Proteins are grouped as cation/Ca2+ exchangers (CCX), Na+/Ca2+ exchangers (NCX), Na+/Ca2+ + K+ exchangers (NCKX), bacterial exchangers (YRGB), and H+/Ca2+ exchangers (CAX). (B) Amino acid sequence alignment of the α1 and α2 repeats of the E. histolytica exchanger with those of representative members of the different exchanger families. Asterisks indicate identical residues in all sequences. Boxes show the distinctive motives of the CCX family. Residues involved in calcium, sodium, or lithium transport are shadowed.
Expression and Localization of EhCCX
To investigate whether the transcript of the Ehccx gene is found in the trophozoites we performed RT-PCR assays. Results showed the amplification of a cDNA fragment with the expected molecular size (Figure 3A), indicating that the Ehccx gene is transcriptionally active under basal conditions. Next, with the purpose to immunodetect the EhCCX protein, we produced an antibody against a specific peptide located at position 216-229. Based on the 3D model, this peptide is into an exposed segment (Figure 2A). In Western blot assays, the antibody detected a single band of 56 kDa (Figure 3B), the expected molecular weight for EhCCX. By immunofluorescence assays, this protein was detected in the plasma membrane and in the membrane of numerous cytoplasmic vesicles (Figure 3C). Immunoelectron microscopy confirmed the presence of EhCCX in the plasma membrane and in the membrane of some cytoplasmic vesicles (Figure 4).
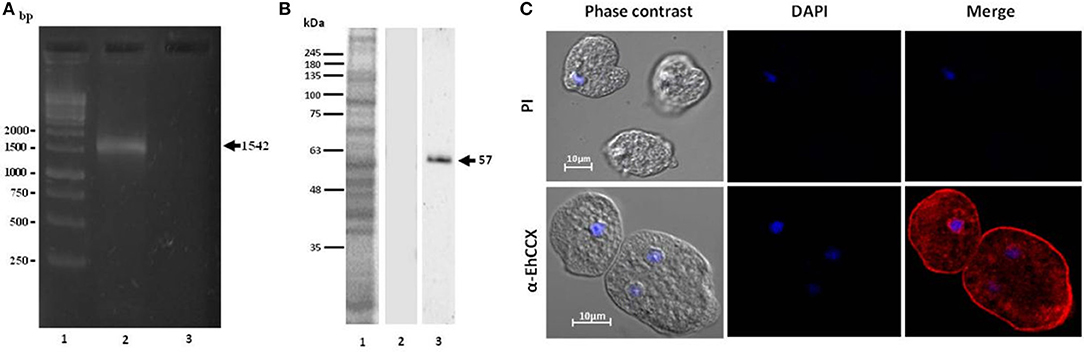
Figure 3. Expression of the Ehccx transcript and immunolocalization of the EhCCX protein. (A) Total RNA was obtained from E. histolytica trophozoites, cDNA was synthesized, and RT-PCR assays were performed using specific primers for Ehccx. Lane 1, 1 kb size markers. Lane 2, PCR assay using cDNA as template. Lane 3, PCR using RNA (omitting the cDNA synthesis) as template. (B) A specific peptide of EhCCX was synthesized and inoculated in rats to obtain antibodies against this exchanger. Then, to analyze their specificity, these antibodies were used in Western blot assays on total extracts of trophozoites. Lane 1, total proteins of E. histolytica trophozoites stained with Coomassie blue; lane 2, Western blot assay using the pre-immune serum; lane 3, Western blot assay using α-EhCCX; (C) E. histolytica trophozoites were fixed, permeabilized and incubated with rat antibodies against EhCCX. Subsequently, samples were incubated with Alexa 555-conjugated secondary antibodies. Finally, nuclei were stained with DAPI and samples were analyzed by confocal microscopy. PI, pre-immune serum.
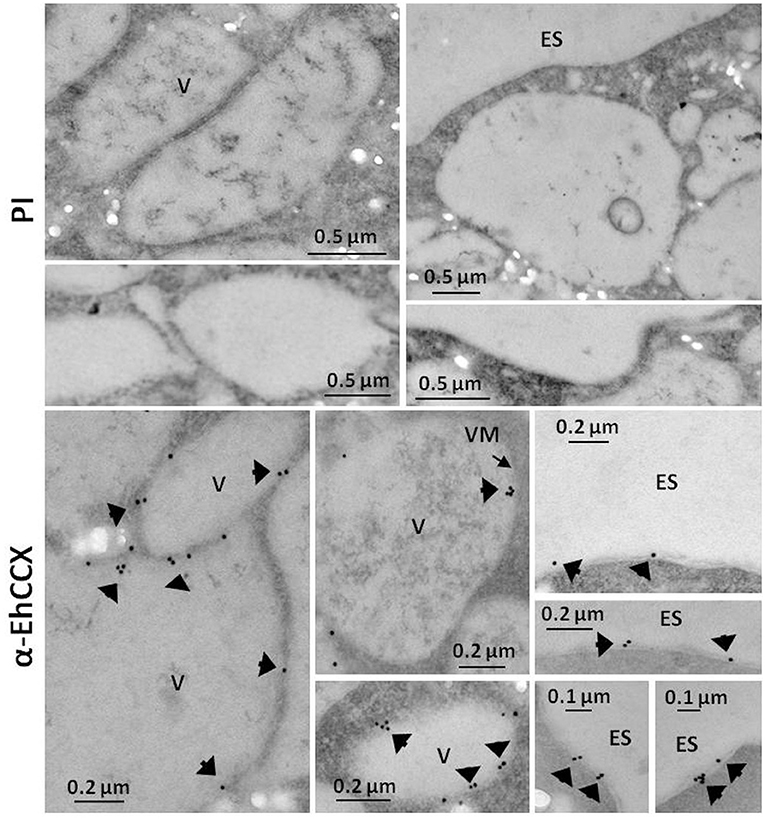
Figure 4. Immunoelectron microscopy. Thin sections (60 nm) of trophozoites were incubated with pre-immune serum (PI) or with antibodies against EhCCX (α-EhCCX) and then, with anti-rat IgGs conjugated to 15 nm gold particles. Afterwards, samples were analyzed by transmission electron microscopy. ES, extracellular space; V, vacuoles.
To identify the possible cytoplasmic compartments in which EhCCX might be situated, we analyzed its co-localization with markers for endoplasmic reticulum (EhSERCA), Golgi apparatus (NBD C6-Ceramide), late endosomes (NPC-1), and endocytic vesicles (EhRabB). We also confirmed the presence of EhCCX in the plasma membrane by its co-localization with the Gal/GalNac lectin. In these assays, EhCCX showed some spots of co-localization with the Gal/GalNac lectin and EhRabB into or close to the plasma membrane (Figure 5A). This exchanger also showed co-localization with NBD C6-Ceramide in some vesicles close to the plasma membrane (Figure 5A), suggesting its presence in the trans-Golgi network. On the other hand, EhCCX did not display significant co-localization with EhSERCA, NPC-1 or cytoplasmic EhRabB (Figure 5A). The Pearson coefficient correlation confirmed a significant co-localization of EhCCX only with the Gal/GalNac lectin, EhRabB and NBD C6-Ceramide (Figure 5B). These results suggested that EhCCX in the plasma membrane could be involved in the extrusion of Ca2+ from cytosol, whereas its presence in in trans-Golgi could be due to its cytoplasmic transport. Alternatively, the posttranslational modifications needed for the maturation process of EhCCX could explain its presence in the Golgi apparatus. Nevertheless, we do not know the identity of most of the cytoplasmic vesicles that contain EhCCX nor its function in these structures.
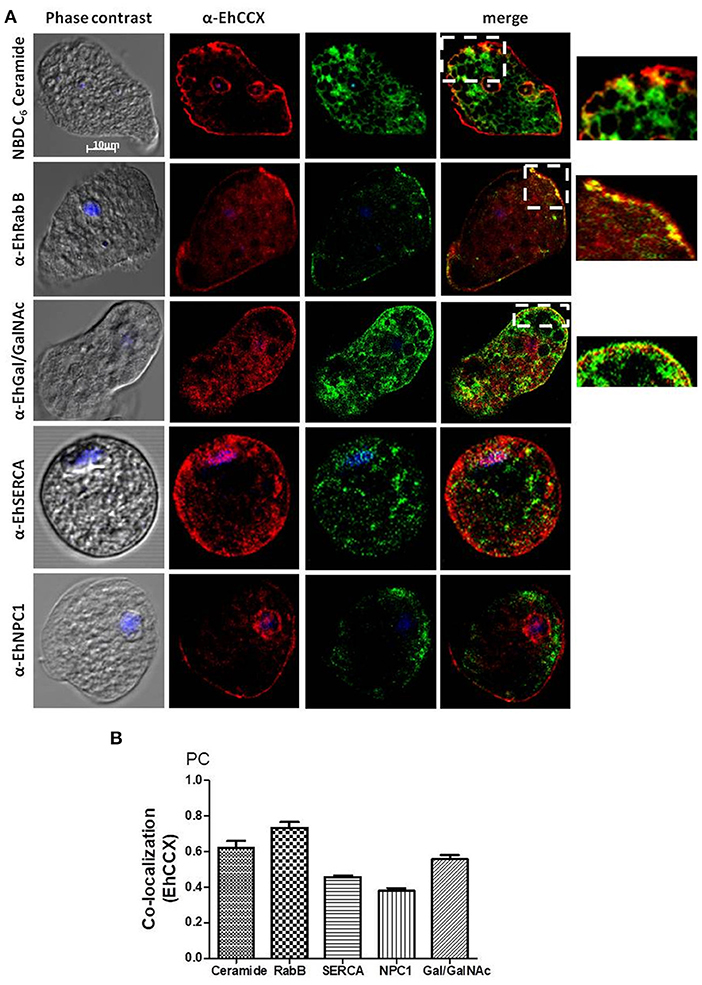
Figure 5. Co-localization assays. (A) E. histolytica trophozoites were fixed, permeabilized and incubated with rat antibodies against EhCCX and subsequently with rabbit antibodies against EhSERCA, EhRabB, or EhNPC1, or mouse antibodies against the Gal/GalNac lectin. Afterwards, samples were incubated with anti-rat IgGs labeled with Alexa 555, and with anti-rabbit IgGs labeled with Alexa 488 or anti-mouse IgGs labeled with FITC. Alternatively, after incubation with α-EhCCX and the anti-rat secondary antibody, trophozoites were incubated with NBD C6-ceramide. Finally, nuclei were stained with DAPI and samples were analyzed by confocal microscopy. On the right are shown magnifications of the squares marked in the merge images of co-localization with NBD C6-ceramide, EhRabB, and Gal/GalNac lectin. (B) Pearson coefficients of co-localization between EhCCX and the different markers.
The non-pathogenic amoeba E. dispar has a lower erythrophagocytic capacity that the pathogenic E. histolytica (Talamás-Lara et al., 2014). To analyze whether EhCCX could participate in virulence, we compared the location and expression of the exchanger in E. histolytica (EhCCX) and E. dispar (EdCCX). Western blot assays showed that E. histolytica expresses a higher amount of the exchanger compared with E. dispar (Figure 6A). Immunofluorescence assays using the α-EhCCX antibody showed that the localization of the exchanger in the trophozoites of E. histolytica and E. dispar is similar, but the fluorescent signal was apparently lower in E. dispar (Figure 6B). The minor expression of the exchanger in E. dispar supports the hypothesis that EhCCX could participates in the virulence mechanism of E. histolytica.
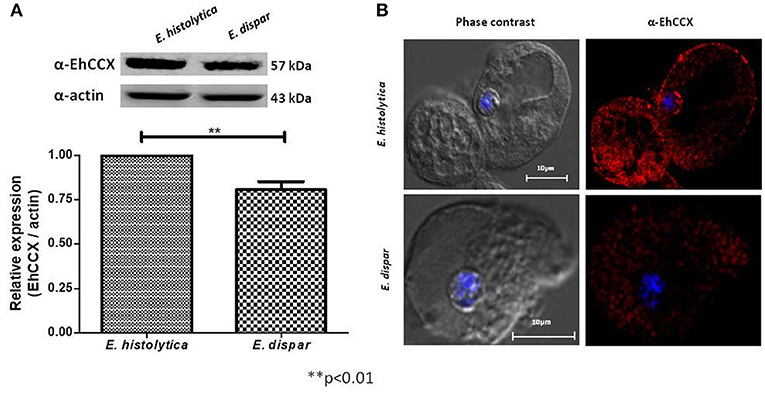
Figure 6. Expression and localization of CCX in E. dispar. (A) The comparison of the expression level of the CCX protein in E. histolytica (EhCCX) and E. dispar (EdCCX) was performed by Western blot using the α-EhCCX antibody. As an internal control, the membranes were probed with an α-actin antibody. The band detected by the α-EhCCX antibody was analyzed by densitometry and the data were normalized to the actin content. The relative expression in E. histolytica was taken as 1. Data are expressed as the mean ± standard error of three independent experiments. (B) E. histolytica and E. dispar trophozoites were fixed, permeabilized and incubated with α-EhCCX antibodies. Next, they were incubated with Alexa 555-conjugated secondary antibodies, nuclei were stained with DAPI, and samples were analyzed by confocal microscopy.
EhCCX Is Stress-Inducible
Calcium/cation exchangers are important in the restoration of the cytoplasmic concentration of Ca2+ after it has been elevated by different stimuli, including oxidative stress. Thus, we analyzed the expression level and localization of EhCCX in trophozoites exposed to H2O2 1 mM during 10 min. Western blot assays showed that this exchanger slightly increased its expression (~0.4 times) under this condition (Figure 7A), suggesting that an augment of this protein could be needed to enhance the calcium extrusion trying to avoid the cellular damage. By immunofluorescence we observed a small accumulation of EhCCX in the plasma membrane and in some big vacuoles (Figure 7B).
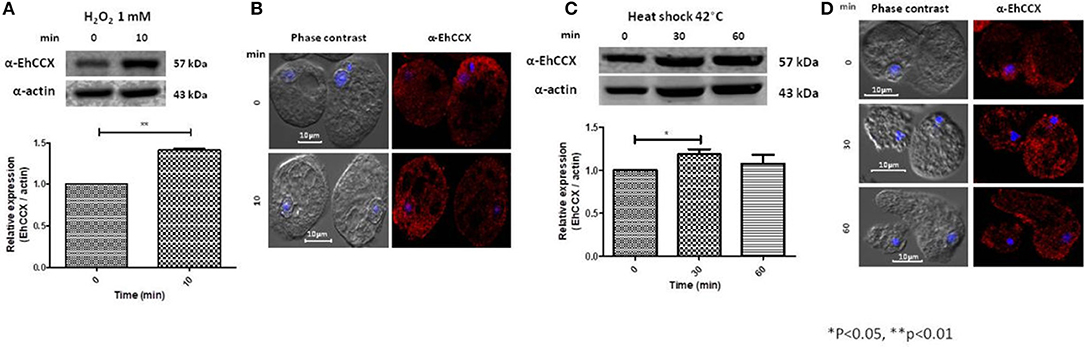
Figure 7. Expression and localization of EhCCX in trophozoites exposed to hydrogen peroxide and to heat shock. (A,B) E. histolytica trophozoites were incubated in the absence (0 min) or in the presence of hydrogen peroxide 1 mM during 10 min. (C,D) E. histolytica trophozoites were incubated at 37 °C (0 min) or at 42 °C during 30 and 60 min. (A,C) The expression of EhCCX was analyzed by Western blot. As an internal control, the membranes were probed with an α-actin antibody. The band detected by the α-EhCCX antibody was analyzed by densitometry and the data were normalized to the actin content. The relative expression in trophozoites at 0 min was taken as 1. Data are expressed as the mean ± standard error of three independent experiments. (B,D) Cells were fixed, permeabilized and incubated with α-EhCCX antibodies. Next, they were incubated with Alexa 555-conjugated secondary antibodies, nuclei were stained with DAPI, and samples were analyzed by confocal microscopy.
We also analyzed the expression of EhCCX in trophozoites submitted to heat shock. After 30 min of incubation at 42°C, the expression of EhCCX showed a slight increase (about 0.2 times) that descend to approximately the basal levels after 60 min of heat shock (Figure 7C). We do not observe any change of the location of this exchanger under 30 and 60 min of incubation at 42°C (Figure 7D). Results suggest that EhCCX could has a minor participation or does not participate in the response to heat shock.
Phagocytosis Alters EhCCX Localization
It has been described that the accumulation of cytosolic calcium plays a major role in several cellular processes of E. histolytica trophozoites, including phagocytosis (Jain et al., 2008). Therefore, to investigate the possible role of EhCCX in this cellular event we performed Western blot assays on total extracts of trophozoites obtained at different times of erythrophagocytosis. No differences in the protein level were detected in these experiments (Figure 8A). However, we observed changes in its localization during the erythrophagocytosis. At 5 min, the localization of EhCCX was similar to the basal conditions; but at 10 min the exchanger was mainly detected in the plasma membrane, and after 15 min the presence of this protein diminished in the plasma membrane and it was concentrated in some cytoplasmic spots (Figure 8B). The accumulation of EhCCX in the plasma membrane at 10 min of phagocytosis suggested that this exchanger could participate in the signaling triggered by the interaction with the target cells and this signaling could be interrupted at 15 min by the internalization of EhCCX. In the presence of the calcium chelator EGTA, few erythrocytes were phagocyted by trophozoites, even after 30 min (Figure 8B), confirming that calcium flux is involved in this process. In this condition the movement of EhCCX to the plasma membrane was not observed, but it was concentrated in some small points in the cytoplasm (Figure 8B). These results suggest that the signaling pathway triggered by the presence of target cells promotes the recruitment of EhCCX in the amoeba surface.
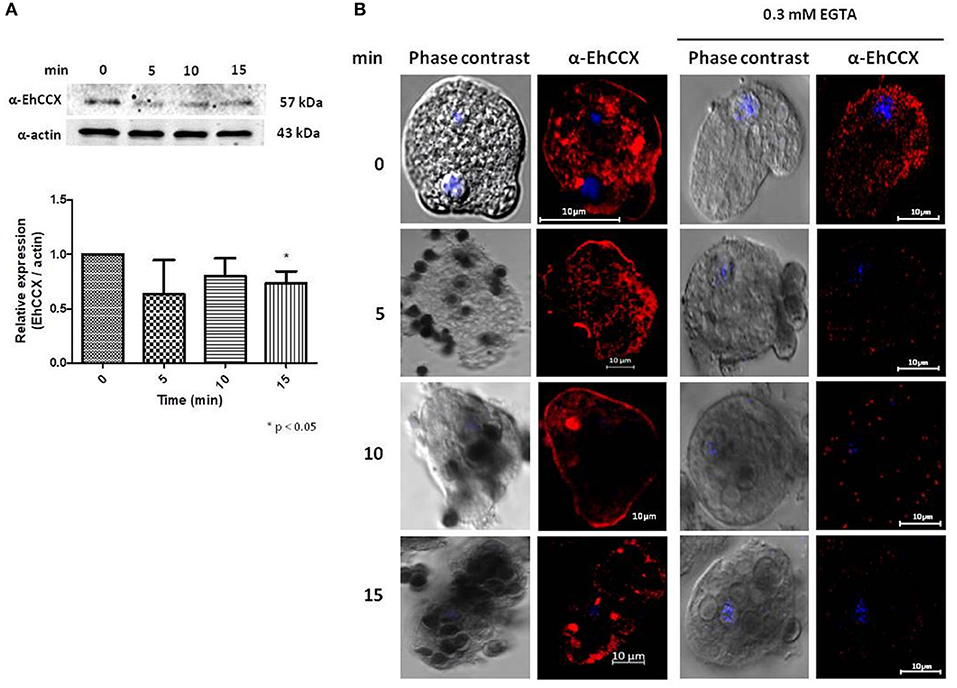
Figure 8. Expression and localization of EhCCX during phagocytosis. E. histolytica trophozoites were incubated at 37°C without erythrocytes (0 min) or in the presence of human erythrocytes during 5, 10, and 15 min. (A) The expression of EhCCX was analyzed by Western blot. As an internal control, the membranes were probed with an α-actin antibody. The band detected by the α-EhCCX antibody was analyzed by densitometry and the data were normalized to the actin content. The relative expression in trophozoites at 0 min was taken as 1. Data are expressed as the mean ± standard error of three independent experiments. (B) Cells were fixed, permeabilized and incubated with α-EhCCX antibodies. Next, they were incubated with Alexa 555-conjugated secondary antibodies, nuclei were stained with DAPI, and samples were analyzed by confocal microscopy. Images at the right correspond to assays performed in the presence of EGTA 0.3 mM.
EhCCX Overexpression Modifies the Cytoplasmic Calcium Level and PCD
To confirm the role of EhCCX in calcium movement and virulence we overexpressed this putative exchanger in trophozoites. Thus, we amplified the Ehccx gene by PCR using genomic DNA as a template and a high-fidelity DNA polymerase. The sequence of the amplicon showed two nucleotide changes with respect to the sequence EHI_001770 deposited in the genome database of E. histolytica: T to C in the position 220 and G to A in the position 328 (data not shown). These nucleotide substitutions modify the amino acid residues 74 (Ser to Pro) and 110 (Ala to Thr), which are into the α1 repeat (Supplementary Figure 1A); however, they did not produce significant alterations in the predicted 3D structure of this motif (Supplementary Figure 1B). In addition, although the residue in position 110 is into one of the distinctive sequences of the CCX members (Figure 2B), the amino acid change fits into the GNG(A/T)PD consensus sequence (Sharma and O'Halloran, 2014), and previous functional studies indicated that the residue situated in this place in the human NCLX does not participate in the ions transport (Roy et al., 2017). Thus, the amplicon was cloned into the pExEhNeo (pNeo) vector (Hamann et al., 1995) and trophozoites were transfected with the resultant construct (pNeo/Ehccx) to overexpress this exchanger. qRT-PCR assays showed that parasites transfected with the pNeo/Ehccx plasmid (pNeo/EhCCX trophozoites) overexpressed about 3.5 times the Ehccx transcript (Figure 9A). On the other hand, Western blot assays revealed that the EhCCX protein was overexpressed 2.8 times in pNeo/EhCCX trophozoites compared with the control (Figure 9B).
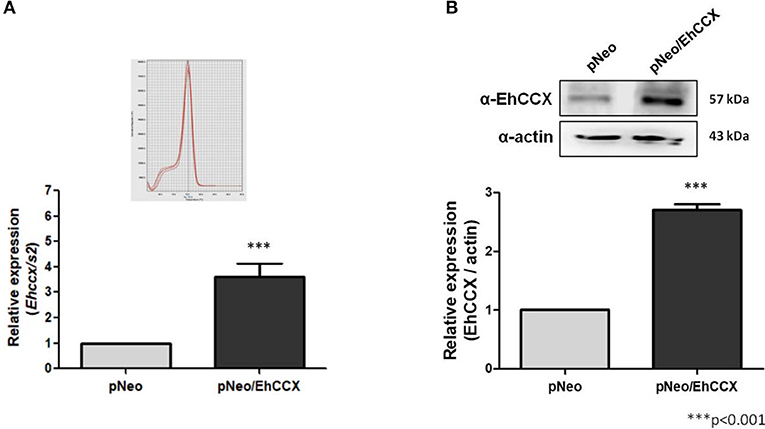
Figure 9. Overexpression of EhCCX. The Ehccx full-length gene was cloned into the pNeo vector and trophozoites were transfected with this construct (pNeo/EhCCX). Trophozoites transfected with the empty vector (pNeo) were used as controls. (A) RNA isolated from trophozoites was used for qRT-PCR assays. As gene normalizer we used the gene coding for the S2 protein of the 40S ribosome subunit. Relative expression was determined using the 2−ΔΔCT method. Inset showed the melt curve that demonstrates the specificity of the Ehccx amplification. (B) Western bolt assays using the α-EhCCX antibody. As an internal control, the membranes were probed with an α-actin antibody. The band detected by the α-EhCCX antibody was analyzed by densitometry and the data were normalized to the actin content. The relative expression in pNeo trophozoites was taken as 1. Data are expressed as the mean ± standard error of three independent experiments.
Then, to confirm that EhCCX participates in Ca2+ flux, we analyzed the cytosolic calcium levels in pNeo and pNeo/EhCCX cells using the Ca2+-sensitive dye Fluo-4 AM and flow cytometry. In these assays the fluorescence intensity in pNeo/EhCCX cells was about twice higher with respect to that showed by the pNeo trophozoites (Figure 10A). Then, to analyze the calcium flux in these trophozoites, we added H2O2 1 mM and analyzed the fluorescence intensity every 40 s. In these assays, the signal gradually increased in pNeo cells (Figure 10B). In contrast, in pNeo/EhCCX trophozoites the florescence intensity augmented after 40 s of incubation with hydrogen peroxide, but subsequently the signal progressively diminished until at 400 s the fluorescence intensity was similar to that of pNeo cells under basal conditions (Figure 10B). These results confirm the role of EhCCX in Ca2+ transport, because the higher expression of the exchanger produced a greater influx of calcium under basal conditions and improve the extrusion of the cytosolic calcium during the programmed cell death (PCD) induced by H2O2.
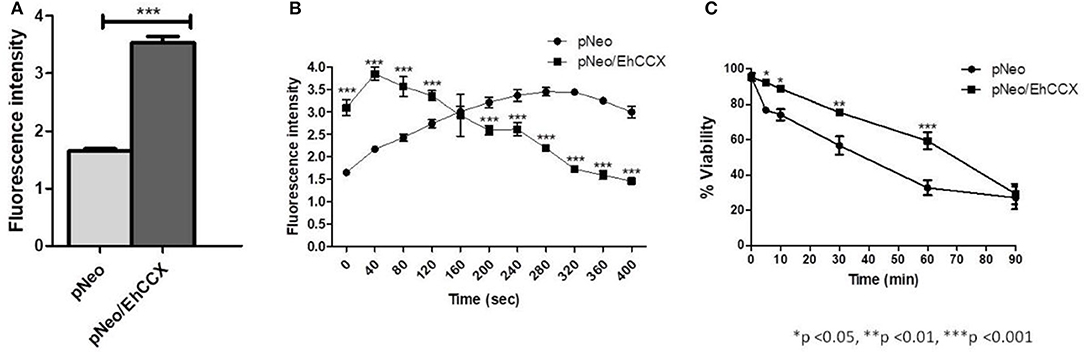
Figure 10. Effect of the EhCCX overexpression on the cytosolic calcium levels and PCD. (A) The cytosolic calcium level of pNeo and pNeo/EhCCX trophozoites in basal conditions was determined by using Fluo4 AM as described in Materials and Methods. (B) pNeo and pNeo/EhCCX trophozoites charged with Fluo4 AM were treated with hydrogen peroxide 1 mM and the fluorescence intensity was measured every 40 s. (C) The cell viability of trophozoites incubated with hydrogen peroxide 1 mM was determined by Trypan blue exclusion at 5, 10, 30, 60, and 90 min. Data are expressed as the mean ± standard error of three independent experiments performed by duplicate.
In concordance with the augmented extrusion of calcium during the PCD-induction, after 30 and 60 min of incubation with H2O2, the cell death of pNeo/EhCCX was significantly lower than pNeo (Figure 10C); however, we did not observe differences in the cell death after 90 min of treatment (Figure 10C). These results suggest that the higher extrusion of cytosolic calcium driven by the overexpressed exchanger retarded the PCD of trophozoites.
EhCCX Overexpression Is Increasing the in vitro Virulence of E. histolytica
We analyzed the erythrophagocytosis, cytopathic activity and migration of pNeo/EhCCX to corroborate that EhCCX is also involved in the in vitro virulence of E. histolytica. Results showed that pNeo/EhCCX cells displayed a significant increase in erythrophagocytosis, compared with pNeo (Figure 11A). EhCCX-overexpressing trophozoites also enhanced more than twice their ability to destroy mammalian cells (Figure 11B), and remarkably, migration of trophozoites augmented almost four times compared to pNeo (Figure 11C). All these results support the hypothesis that Ca2+ flux mediated by EhCCX participates in the in vitro virulence of E. histolytica.
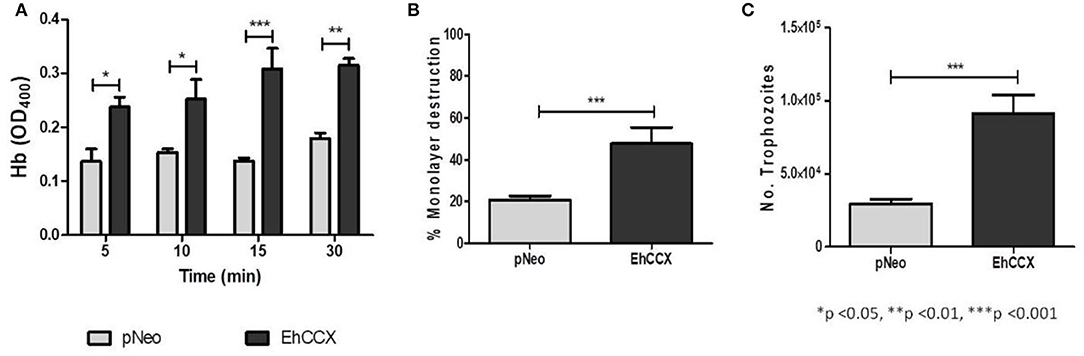
Figure 11. Effect of the EhCCX overexpression on the in vitro virulence. (A) pNeo and pNeo/EhCCX trophozoites were incubated with human erythrocytes at 37°C during 5, 10, 15, and 30 min. Then, non-ingested erythrocytes were lysed by incubation by 10 min with distilled water. After several washes with PBS, trophozoites were lysed with formic acid; and the hemoglobin released from the erythrocytes ingested was determined by absorbance at 405 nm. (B) MDCK cell monolayers were incubated with pNeo or pNeo/EhCCX trophozoites during 2 h at 37°C, and the monolayer destruction was evaluated as described in Material and Methods. (C) Serum-starved trophozoites were placed in the upper chamber of transwell inserts and 500 μl of adult bovine serum was added to the lower chamber. Then, trophozoites were incubated for 3 h at 37°C and migration was determined by counting the number of trophozoites at the lower chamber of the well. Data are expressed as the mean ± standard error of three independent experiments performed by duplicate.
Discussion
The Ca2+ extrusion from the cytosol is carried out mainly by Ca2+-ATPases of the PMCA family and calcium/cations exchangers, therefore, these proteins play a critical role in Ca2+ homeostasis (Berridge et al., 2003). Calcium/cation exchangers transport Ca2+ against its electrochemical gradient coupled to the exchange of different cations, such as H+ (YRGB and CAX), Na+ (NCX), Na+ + K+ (NCKX), and Na+ or Li+ (CCX) (Lytton, 2007; Emery et al., 2012). All these exchangers share structural similarities, including two α-repeats involved in the ion binding/transport events (Giladi et al., 2016). The differences in the ion-coordinating residues among the members of the Ca2+/cation superfamily are responsible of the divergences in ion selectivity (Refaeli et al., 2016).
Ca2+ homeostasis is involved in the host invasion by different protozoa parasites (Ravdin et al., 1985; Lu et al., 1997; Vieira and Moreno, 2000; Lovett and Sibley, 2003; Moreno et al., 2007). It has been described the presence of PMCAs in most of these microorganisms (Moreno and Docampo, 2003), however, until now there are not reports about the existence of Ca2+/Na+ exchangers for any parasite protozoa. A genomic analysis in Apicomplexans revealed that Toxoplasma gondii, Cryptosporidium spp., and Plasmodium spp. contain orthologs of Ca2+/H+ exchangers (CAX) found in plants and yeast, but not in animals. Conversely, Ca2+/Na+ exchangers, which are common in animals, are not found in these parasites (Nagamune and Sibley, 2006). In Plasmodium berghei, the CAX protein participates in ookinete development and differentiation (Guttery et al., 2013). On the other hand, biochemical evidence suggests that trypanosomatids also have a Ca2+/H+ antiporter (Verseci et al., 1994), which was proposed to be involved in Ca2+ release by the increase of sodium mediated by a Na+/H+ antiporter (Versesi and Docampo, 1996). Concordantly, by a in silico analysis we found a gene coding for a putative Ca2+/H+ exchanger in Leishmania mexiana. We also identify a gene coding for a putative Ca2+/H+ exchanger in Trichomonas vaginalis, but we did not discover Ca2+/Na+ exchangers in any parasite protozoa different to Entamoeba spp.
The E. histolytica exchanger showed a higher similarity with the CCX family and contains the same amino acid residues involved in the transport of calcium, sodium, and lithium of human NCLX. It is known that the intracellular concentration of Li+ is very low (0.6–0.8 mM); thus, the Li+ transport by NCLX members just could be related to some functional properties. For instance, Ca2+ transport by NCX is driven by a steep Na+ gradient and a moderate (~-70 mV) membrane potential, whereas Ca2+ efflux by NCLX is primarily driven by a much steeper (~−200 mV) membrane potential (Roy et al., 2017).
Based on the disulfide cross-linking data, a model for Ca2+/Na+ exchangers was proposed (Nicoll et al., 1999). In this model, the transmembrane segments 5 and 6 (TMS5 and TMS6) are separated by a large (~500 amino acids) f-loop that faces the cytosolic side. This loop is responsible for the regulation of the ions transport activity by the binding of several cytoplasmic messengers, protons, NO, PIP2, phosphoarginine, phosphocreatinine, ATP, and endogenous inhibitors (Khananshvili, 2013). Interestingly, NCLX members have a very short f-loop with no regulatory domains on it (Khananshvili, 2013). Similarly, EhCCX has a small f-loop (163 aa), suggesting that this protein, as NCLX members, has no controlling domains for “secondary” regulation.
The human NCLX is found in the inner membrane of the mitochondria (Palty et al., 2010); however, it was also detected in the plasma membrane of ventricular myocytes and pancreatic β-cells (Cai and Lytton, 2004; Han et al., 2015). Indeed, it has been reported that in pancreatic β-cells the NCLX of plasma membrane is involved in the calcium flux that contributes to the vesicle recruitment for sustained exocytosis in response to repetitive depolarization (Han et al., 2015). Likewise, the EhCCX located in the surface of trophozoites may participates in the calcium flux through the plasma membrane. This hypothesis is supported by the increase of its expression in trophozoites exposed to H202 and by the changes in the cytosolic calcium levels in EhCCX-overexpressing trophozoites during the incubation with H202.
On the other hand, EhCCX seems to be involved in the amoeba virulence. This hypothesis is supported by: (i) the expression level of this protein is higher in the pathogenic E. histolytica than in the non-pathogenic E. dispar; and (ii) the overexpression of EhCCX augmented the in vitro virulence properties of trophozoites, such as phagocytosis, destruction of mammalian cell monolayers and migration. Similarly, the overexpression of NCX proteins enhances the heart rate mediated by beta-adrenergic (Kaese et al., 2017), and the relaxation of gastric fundus (Fujimoto et al., 2016). We are hypothesizing that, as calcium/cation exchangers can reverse the ions transport under certain conditions (Harper and Sage, 2016), the different stimuli that are involved in the E. histolytica virulence could increase the Ca2+ influx via EhCCX, extending the period of high Ca2+ levels in cytoplasm, maintaining the response to the signal. Such mechanism of Ca2+ influx via NCX has also been proposed to explain the enhanced contraction displayed by the urinary bladder smooth muscles of transgenic mice overexpressing NCX1.3 (Yamamura et al., 2013).
In conclusion, in this work we identified a calcium/cation exchanger of E. histolytica (EhCCX). The expression of this exchanger, belonging to the CCX family, is higher in pathogenic than in non-pathogenic amoeba. Moreover, the cellular localization of EhCCX changed during phagocytosis and its overexpression increased the in vitro virulence and retarded the PCD induced by H2O2. These results suggest that Ca2+ flux through EhCCX participates in the PCD and the in vitro virulence of E. histolytica.
Author Contributions
MV-S conceived and carried out experiments, analyzed data, and drafted the manuscript. JB performed experiments and analyzed data. EO and MH participated in the design of the study and analyzed data. GG-R carried out the transfection of trophozoites and participates in their characterization. AS-C realized the measurements of cytosolic calcium levels. BC-M performed the immunoelectron microscopy. MR conceived and designed the study, analyzed data and drafted the manuscript.
Conflict of Interest Statement
The authors declare that the research was conducted in the absence of any commercial or financial relationships that could be construed as a potential conflict of interest.
Acknowledgments
MV-S was a recipient of a fellowship from Consejo Nacional de Ciencia y Tecnología (Grant 418500). We are grateful for the excellent technical assistance of Carlos Vázquez-Calzada and Victor Rosales-García. We also thank Dr. José Manuel Hernández-Hernández (CINVESTAV-IPN) for providing the α-actin antibody.
Supplementary Material
The Supplementary Material for this article can be found online at: https://www.frontiersin.org/articles/10.3389/fcimb.2018.00342/full#supplementary-material
Abbreviations
CCX, calcium/cation exchanger; EhCCX, calcium/cation exchanger of E. histolytica; EdCCX, calcium/cation exchanger of E. dispar; EhNPC1, Niemann-Pick type C protein 1 of E. histolytica; EhRabB, RabB protein of E. histolytica; EhSERCA, sarco/endoplasmic reticulum Ca2+-ATPase of E. histolytica; Gal/GalNac lectin, lectin of E. histolytica inhibited by galactose and N-acetyl-D-galactosamine; NCLX, sodium/calcium/lithium exchanger; NCX, sodium/calcium exchanger; MjNCX, sodium/calcium exchanger of Methanococcus jannaschii; PMCA, plama membrane Ca2+-ATPase; PCD, programmed cell death.
References
Berridge, M. J., Bootman, M. D., and Roderick, H. L. (2003). Calcium signalling: dynamics, homeostasis and remodelling. Nat. Rev. Mol. Cell Biol. 4, 517–529. doi: 10.1038/nrm1155
Blaustein, M. P., and Lederer, W. J. (1999). Sodium/calcium exchange: its physiological implications. Physiol. Rev. 79, 763–854.
Bolaños, J., Betanzos, A., Javier-Reyna, R., García-Rivera, G., Huerta, M., Pais-Morales, J., et al. (2016). EhNPC1 and EhNPC2 proteins participate in trafficking of exogenous cholesterol in Entamoeba histolytica trophozoites: relevance for phagocytosis. PLoS Pathog. 12:e1006089. doi: 10.1371/journal.ppat.1006089
Cai, X., and Lytton, J. (2004). Molecular cloning of a sixth member of the K+-dependent Na+/Ca2+ exchanger gene family, NCKX6. J. Biol. Chem. 279, 5867–5876. doi: 10.1074/jbc.M310908200
Carbajal, M. E., Manning-Cela, R., Pina, A., Franco, E., and Meza, I. (1996). Fibronectin-induced intracellular calcium rise in Entamoeba histolytica trophozoites: effect on adhesion and the actin cytoskeleton. Exp. Parasitol. 82, 11–20.
Diamond, L. S., Clark, C. G., and Cunnick, C. C. (1995). YI-S, a casein-free medium for axenic cultivation of Entamoeba histolytica, related Entamoeba, Giardia intestinalis and Trichomonas vaginalis. J. Eukariot. Microbiol. 4, 277–278.
Diamond, L. S., Harlow, D. R., and Cunnick, C. C. (1978). A new medium for the axenic cultivation of Entamoeba histolytica and other Entamoeba. Trans. R. Soc. Trop. Med. Hyg. 72, 431–432.
Emery, L., Whelan, S., Hirschi, K. D., and Pittman, J. K. (2012). Protein phylogenetic analysis of Ca2+/cation antiporters and insights into their evolution in plants. Front. Plant Sci. 3:1. doi: 10.3389/fpls.2012.00001
Fujimoto, Y., Hayashi, S., Azuma, Y. T., Mukai, K., Nishiyama, K., Kita, S., et al. (2016). Overexpression of Na+/Ca2+ exchanger 1 display enhanced relaxation in the gastric fundus. J. Pharmacol. Sci. 132, 181–186. doi: 10.1016/j.jphs.2016.10.003
Giladi, M., Shor, R., Lisnyansky, M., and Khananshvili, D. (2016). Structure-functional basis of ion transport in sodium-calcium exchanger (NCX) proteins. Int. J. Mo.l Sci. 17:1949. doi: 10.3390/ijms17111949
Guttery, D. S., Pittman, J. K., Frénal, K., Poulin, B., McFarlane, L. R., Slavic, K., et al. (2013). The Plasmodium berghei Ca2+/H+ exchanger, PbCAX, is essential for tolerance to environmental Ca2+) during sexual development. PLoS Pathog. 9:e1003191. doi: 10.1371/journal.ppat.1003191
Hamann, L., Nickel, R., and Tannich, E. (1995). Transfection and continuous expression of heterologous genes in the protozoan parasite Entamoeba histolytica. Proc. Natl. Acad. Sci. U.S.A. 92, 8975–8979.
Han, Y. E., Ryu, S. Y., Park, S. H., Lee, K. H., Lee, S. H., and Ho, W. K. (2015). Ca2+ clearance by plasmalemmal NCLX, Li+-permeable Na+/Ca2+ exchanger, is required for thesustained exocytosis in rat insulinoma INS-1 cells. Pflugers Arch. 467, 2461–2472. doi: 10.1007/s00424-015-1715-3
Harper, A. G., and Sage, S. O. (2016). TRP-Na+/Ca2+ exchanger coupling. Adv. Exp. Med. Biol. 898, 67–85. doi: 10.1007/978-3-319-26974-0_4
Jain, R., Santi-Rocca, J., Padhan, N., Bhattacharya, S., Guillen, N., and Bhattacharya, A. (2008). Calcium-binding protein 1 of Entamoeba histolytica transiently associates with phagocytic cups in a calcium-independent manner. Cell. Microbiol. 10, 1373–1389. doi: 10.1111/j.1462-5822.2008.01134.x
Kaese, S., Bögeholz, N., Pauls, P., Dechering, D., Olligs, J., Kölker, K., et al. (2017). Increased sodium/calcium exchanger activity enhances beta-adrenergic-mediated increase in heart rate: whole-heart study in a homozygous sodium/calcium exchanger overexpressor mouse model. Heart Rhythm. 14, 1247–1253. doi: 10.1016/j.hrthm.2017.05.001
Khananshvili, D. (2013). The SLC8 gene family of sodium-calcium exchangers (NCX) - structure, function, and regulation in health and disease. Mol. Aspects Med. 34, 220–235. doi: 10.1016/j.mam.2012.07.003
Liao, J., Li, H., Zeng, W., Sauer, D. B., Belmares, R., and Jiang, Y. (2012). Structural insight into the ion-exchange mechanism of the sodium/calcium exchanger. Science 335, 686–690. doi: 10.1126/science.1215759
Lovett, J. L., and Sibley, L. D. (2003). Intracellular calcium stores in Toxoplasma gondii govern invasion of host cells. J. Cell Sci. 116, 3009–3016. doi: 10.1242/jcs.00596
Lu, H. G., Zhong, L., Chang, K. P., and Docampo, R. (1997). Intracellular Ca2+ pool content and signaling and expression of a calcium pump are linked to virulence in Leishmania mexicana amazonensis. J. Biol. Chem. 272, 9646–9473.
Lytton, J. (2007). Na+/Ca2+ exchangers: three mammalian gene families control Ca2+ transport. Biochem. J. 406, 365–382. doi: 10.1042/BJ20070619
Makioka, A., Kumagai, M., Kobayashi, S., and Takeuchi, T. (2002). Possible role of calcium ions, calcium channels and calmodulin in excystation and metacystic development of Entamoeba invadens. Parasitol. Res. 88, 837–843. doi: 10.1007/s00436-002-0676-6
Makioka, A., Kumagai, M., Ohtomo, H., Kobayashi, S., and Takeuchi, T. (2001). Effect of calcium antagonists, calcium channel blockers and calmodulin inhibitors on the growth and encystation of Entamoeba histolytica and E. invadens. Parasitol. Res. 87, 833–837. doi: 10.1007/s0043601004
Martínez-Higuera, A., Herrera-Martínez, M., Chávez-Munguía, B., Valle-Solís, M., Muñiz-Lino, M. A., Cázares-Apátiga, J., et al. (2015). Entamoeba invadens: identification of a SERCA protein and effect of SERCA inhibitors on encystation. Microb. Pathog. 89, 18–26. doi: 10.1016/j.micpath.2015.08.016
Martinez-Higuera, A., Salas-Casas, A., Calixto-Gálvez, M., Chávez-Munguía, B., Pérez-Ishiwara, D. G., Ximénez, C., et al. (2013). Identification of calcium-transporting ATPases of Entamoeba histolytica and cellular localization of the putative SERCA. Exp. Parasitol. 135, 79–86. doi: 10.1016/j.exppara.2013.06.004
Moreno, S. N., and Docampo, R. (2003). Calcium regulation in protozoan parasites. Curr. Opin. Microbiol. 6, 359–364. doi: 10.1016/S1369-5274(03)00091-2
Moreno, S. N. J., Miranda, K., Fang, J., Rohloff, P., and de Souza, W. (2007). “Calcium storage and homeostasis in Toxoplasma gondii,” in Toxoplasma gondii. The model Apicomplexan: Perspectives and Methods, eds L. M. Weiss and K. Kim (Amsterdam: Elsevier), 245–263.
Nagamune, K., and Sibley, L. D. (2006). Comparative genomic and phylogenetic analyses of calcium ATPases and calcium-regulated proteins in the apicomplexa. Mol. Biol. Evol. 23, 1613–1627. doi: 10.1093/molbev/msl026
Nicoll, D. A., Ottolia, M., Lu, L., Lu, Y., and Philipson, K. D. (1999). A new topological model of the cardiac sarcolemmal Na+-Ca2+ exchanger. J. Biol. Chem. 274, 910–917.
Orozco, E., Guarneros, G., Martinez-Palomo, A., and Sánchez, T. (1983). Entamoeba histolytica. Phagocytosis as a virulence factor. J. Exp. Med. 158, 1511–1521.
Palty, R., Silverman, W. F., Hershfinkel, M., Caporale, T., Sensi, S. L., Parnis, J., et al. (2010). NCLX is an essential component of mitochondrial Na+/Ca2+ exchange. Proc. Natl. Acad. Sci. U.S.A. 107, 436–441. doi: 10.1073/pnas.0908099107
Petri, W. A. Jr., Chapman, M. D., Snodgrass, T., Mann, B. J., Broman, J., and Ravdin, J. I. (1989). Subunit structure of the galactose and N-acetyl-D-galactosamine-inhibitable adherence lectin of Entamoeba histolytica. J. Biol. Chem. 264, 3007–3012.
Philipson, K. D., and Nicoll, D. A. (2000). Sodium-calcium exchange: a molecular perspective. Annu. Rev. Physiol. 62, 111–133. doi: 10.1146/annurev.physiol.62.1.111
Ravdin, J. I., Murphy, C. F., Guerrant, R. L., and Long-Krug, S. A. (1985). Effect of antagonists of calcium and phospholipase A on the cytopathogenicity of Entamoeba histolytica. J. Infect. Dis. 152, 542–549.
Ravdin, J. I., Sperelakis, N., and Guerrant, R. L. (1982). Effect of ion channel inhibitors on the cytopathogenicity of Entamoeba histolytica. J. Infect. Dis. 146, 335–340.
Refaeli, B., Giladi, M., Hiller, R., and Khananshvili, D. (2016). Structure-based engineering of lithium-transport capacity in an archaeal sodium-calcium exchanger. Biochemistry 55, 1673–1676. doi: 10.1021/acs.biochem.6b00119
Rodriguez, M. A., Garcia-Perez, R. M., Garcia-Rivera, G., Lopez-Reyes, I., Mendoza, L., Ortiz-Navarrete, V., et al. (2000). An Entamoeba histolytica rab-like encoding gene and protein: function and cellular location. Mol. Biochem. Parasitol. 10, 199–206. doi: 10.1016/S0166-6851(00)00216-4
Rodríguez, M. A., Martínez-Higuera, A., Valle-Solis, M. I., Hernandes-Alejandro, M., Chávez-Munguía, B., Figueroa-Gutiérrez, A. H., et al. (2018). A putative Calcium-ATPase of the secretory pathway family may regulate calcium/manganese levels in the Golgi apparatus of Entamoeba histolytica. Parasitol. Res. doi: 10.1007/s00436-018-6030-4. [Epub ahead of print].
Rodríguez, M. A., and Orozco, E. (1986). Isolation and characterization of phagocytosis- and virulence-deficient mutants of Entamoeba histolytica. J. Infect. Dis. 154, 27–32.
Roy, S., Dey, K., Hershfinkel, M., Ohana, E., and Sekler, I. (2017). Identification of residues that control Li+ versus Na+ dependent Ca2+ exchange at the transport site of the mitochondrial NCLX. Biochim. Biophys. Acta 1864, 997–1008. doi: 10.1016/j.bbamcr.2017.01.011
Segovia-Gamboa, N. C., Talamás-Rohana, P., Ángel-Martínez, A., Cázares-Raga, F. E., González-Robles, A., Hernández-Ramírez, V. I., et al. (2011). Differentiation of Entamoeba histolytica: a possible role for enolase. Exp. Parasitol. 129, 65–71. doi: 10.1016/j.exppara.2011.05.001
Sharma, V., and O'Halloran, D. M. (2014). Recent structural and functional insights into the family of sodium calcium exchangers. Genesis 52, 93–109. doi: 10.1002/dvg.22735
Talamás-Lara, D., Chávez-Munguía, B., González-Robles, A., Talamás-Rohana, P., Salazar- Villatoro, L., Durán-Díaz, A., et al. (2014). Erythrophagocytosis in Entamoeba histolytica and Entamoeba dipar: a comparative study. Biomed. Res. Int. 2014:626259. doi: 10.1155/2014/626259
Tamura, K., Peterson, D., Peterson, N., Stecher, G., Nei, M., and Kumar, S. (2011). MEGA5: molecular evolutionary genetics analysis using maximum likelihood, evolutionary distance, and maximum parsimony methods. Mol. Biol. Evol. 28, 2731–2739. doi: 10.1093/molbev/msr121
Verseci, A. E., Moreno, S. N., and Docampo, R. (1994). Ca2+/H+ exchange in acidic vacuoles of Trypanosoma brucei. Biochem J. 30, 227–233.
Versesi, A. E., and Docampo, R. (1996). Sodium-proton exchange stimulates Ca2+ release from acidocalcisomes of Trypanosoma brucei. Biochem J. 315, 265–270.
Vieira, M. C., and Moreno, S. N. (2000). Mobilization of intracellular calcium upon attachment of Toxoplasma gondii tachyzoites to human fibroblasts is required for invasion. Molec. Biochem. Parasitol. 106, 157–162. doi: 10.1016/S0166-6851(99)00182-6
Yamamura, H., Cole, W. C., Kita, S., Hotta, S., Murata, H., Suzuki, Y., et al. (2013). Overactive bladder mediated by accelerated Ca2+ influx mode of Na+/Ca2+ exchanger in smooth muscle. Am. J. Physiol. Cell Physiol. 305, C299–308. doi: 10.1152/ajpcell.00065.2013
Keywords: Entamoeba histolytica, calcium/cation exchanger, protein overexpression, programmed cell death, virulence
Citation: Valle-Solis M, Bolaños J, Orozco E, Huerta M, García-Rivera G, Salas-Casas A, Chávez-Munguía B and Rodríguez MA (2018) A Calcium/Cation Exchanger Participates in the Programmed Cell Death and in vitro Virulence of Entamoeba histolytica. Front. Cell. Infect. Microbiol. 8:342. doi: 10.3389/fcimb.2018.00342
Received: 27 April 2018; Accepted: 10 September 2018;
Published: 01 October 2018.
Edited by:
Margaret E. Bauer, School of Medicine, Indiana University Bloomington, United StatesReviewed by:
César López-Camarillo, Universidad Autónoma de la Ciudad de México, MexicoAbhijeet Anil Bakre, University of Georgia, United States
Copyright © 2018 Valle-Solis, Bolaños, Orozco, Huerta, García-Rivera, Salas-Casas, Chávez-Munguía and Rodríguez. This is an open-access article distributed under the terms of the Creative Commons Attribution License (CC BY). The use, distribution or reproduction in other forums is permitted, provided the original author(s) and the copyright owner(s) are credited and that the original publication in this journal is cited, in accordance with accepted academic practice. No use, distribution or reproduction is permitted which does not comply with these terms.
*Correspondence: Mario A. Rodríguez, bWFyb2RyaUBjaW52ZXN0YXYubXg=