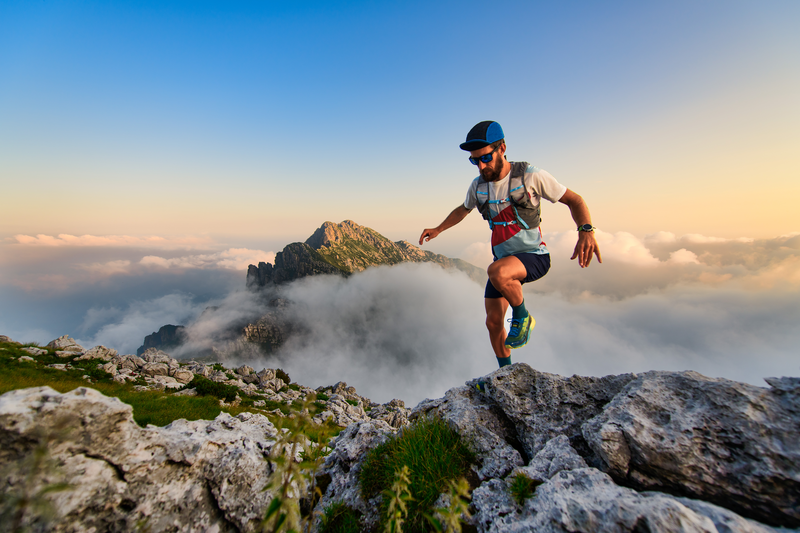
94% of researchers rate our articles as excellent or good
Learn more about the work of our research integrity team to safeguard the quality of each article we publish.
Find out more
ORIGINAL RESEARCH article
Front. Cell. Infect. Microbiol. , 28 April 2017
Sec. Parasite and Host
Volume 7 - 2017 | https://doi.org/10.3389/fcimb.2017.00129
This article is part of the Research Topic Tick-host-pathogen Interactions View all 39 articles
Rickettsia rickettsii is a tick-borne obligate intracellular bacterium that causes Rocky Mountain Spotted Fever (RMSF). In Brazil, two species of ticks in the genus Amblyomma, A. sculptum and A. aureolatum, are incriminated as vectors of this bacterium. Importantly, these two species present remarkable differences in susceptibility to R. rickettsii infection, where A. aureolatum is more susceptible than A. sculptum. In the current study, A. aureolatum and A. sculptum ticks were fed on suitable hosts previously inoculated with R. rickettsii, mimicking a natural infection. As control, ticks were fed on non-infected animals. Both midgut and salivary glands of all positively infected ticks were colonized by R. rickettsii. We did not observe ticks with infection restricted to midgut, suggesting that important factors for controlling rickettsial colonization were produced in this organ. In order to identify such factors, the total RNA extracted from the midgut (MG) was submitted to next generation RNA sequencing (RNA-seq). The majority of the coding sequences (CDSs) of A. sculptum differentially expressed by infection were upregulated, whereas most of modulated CDSs of A. aureolatum were downregulated. The functional categories that comprise upregulated CDSs of A. sculptum, for instance, metabolism, signal transduction, protein modification, extracellular matrix, and immunity also include CDSs of A. aureolatum that were downregulated by infection. This is the first study that reports the effects of an experimental infection with the highly virulent R. rickettsii on the gene expression of two natural tick vectors. The distinct transcriptional profiles of MG of A. sculptum and A. aureolatum upon infection stimulus strongly suggest that molecular factors in this organ are responsible for delineating the susceptibility to R. rickettsii. Functional studies to determine the role played by proteins encoded by differentially expressed CDSs in the acquisition of R. rickettsii are warranted and may be considered as targets for the development of strategies to control the tick-borne pathogens as well as to control the tick vectors.
Rocky Mountain Spotted Fever (RMSF), also known as Brazilian Spotted Fever (BSF), is a severe tick-borne illness caused by Rickettsia rickettsii. In Brazil, R. rickettsii is transmitted to humans by Amblyomma sculptum, formerly named Amblyomma cajennense (Nava et al., 2014), and Amblyomma aureolatum (Labruna, 2009). The prevalence rates of R. rickettsii-infected ticks in endemic areas are low, oscillating around 1%. These low prevalence rates seems to be associated with lower reproductive and survival rates of infected ticks, suggesting that R. rickettsii is also pathogenic to its vectors (Burgdorfer, 1988; Niebylski et al., 1999; Labruna et al., 2008). Previous experimental infections with R. rickettsii have demonstrated that 80–100% of A. aureolatum ticks from a laboratory colony acquire this bacterium, whereas only 10 to 60% of A. sculptum ticks become infected (Labruna et al., 2008). These results showed that A. aureolatum is more susceptible to rickettsial infection than A. sculptum.
The midgut (MG) is the first tick organ that blood meal-acquired microbes (including tick-borne pathogens) interact with. The neutral pH and the nutrients of the host blood favor microbial proliferation in the lumen of the tick MG. In addition, microbes do not directly contact digestive enzymes, as digestion in ticks is intracellular (Sojka et al., 2008). Therefore, to efficiently prevent microbial growth, ticks must present a robust immune response in MG (Hajdusek et al., 2013). Indeed, different antimicrobial peptides (AMPs) and/or their transcripts have been detected in this organ, such as hemocidins (host hemoglobin-derived antimicrobial peptides) (Fogaca et al., 1999; Nakajima et al., 2003; Sonenshine et al., 2005; Belmonte et al., 2012), defensins (Nakajima et al., 2001, 2002; Hynes et al., 2005; Rudenko et al., 2005; Ceraul et al., 2007; Zhou et al., 2007), and lysozymes (Kopacek et al., 1999; Grunclova et al., 2003; Simser et al., 2004; Ceraul et al., 2007). Moreover, transcriptomics data suggest that tick MG contains proteins involved in the production of reactive oxygen species (ROS), which may also help to control microorganism growth (Anderson et al., 2008). To be successfully transmitted to another host, tick-borne pathogens must then be able to resist to the immune reactions of tick MG, transpose this barrier, and reach the salivary glands through hemolymph.
In the current work, we present the first global transcription profile of MG of A. sculptum and A. aureolatum in response to infection with R. rickettsii. Importantly, ticks were infected by feeding on hosts previously inoculated with R. rickettsii, mimicking a natural infection. The analyses were performed using next generation RNA sequencing (RNA-seq) and data were validated by quantitative polymerase chain reaction preceded by reverse transcription (RT-qPCR). The proteins encoded by differentially expressed CDSs in the colonization of tick MG by R. rickettsii should be functionally characterized and may be considered as targets for the development of strategies to control the tick borne pathogens as well as to control the tick vectors.
All animal experiments were performed in strict accordance with the Institutional Animal Care and Use Committees from of the Faculty of Veterinary Medicine and Animal Husbandry (protocol number 1423/2008) and the Institute of Biomedical Sciences (protocol number 128/11) of the University of São Paulo, São Paulo, Brazil. Animal purchases and euthanize procedures were performed as described in Galletti et al. (2013).
Ticks were obtained from laboratory colonies of A. sculptum (Pedreira strain; state of São Paulo, Brazil) and A. aureolatum (Atibaia strain; state of São Paulo, Brazil). A. sculptum larvae, nymphs, and adults were fed on rabbits (Oryctolagus cuniculus), as previously described (Pinter et al., 2002). Feeding of A. aureolatum developmental stages was accomplished following the methodology detailed in Galletti et al. (2013). To obtain infected ticks, larvae were fed on hosts previously infected with the highly virulent Taiaçu strain of R. rickettsii, using the procedure described by Pinter and Labruna (2006) and Galletti et al. (2013). Off-host phases were held in an incubator at 25°C and 95% relative humidity.
Adult ticks from the R. rickettsii-infected and non-infected groups were manually removed from the vertebrate hosts after 3 days of feeding. The ticks were washed in 70% ethanol and sterile phosphate-buffered saline (PBS) (10 mM NaH2PO4, 1.8 mM KH2PO4, 140 mM NaCl, and 2.7 mM KCl, pH 7.4) for 10 min each. Midgut (MG) and salivary glands (SG) of each tick were dissected and separately transferred to 100 μL of RNAlater® Solution (ThermoFisher Scientific).
The SG and MG of each adult tick were homogenized and submitted to a simultaneous isolation of genomic DNA (gDNA) and total RNA using the InviTrap® Spin Cell RNA Mini Kit (Stratatec), according to the manufacturer's specifications.
gDNA was used to quantify the total number of rickettsiae in tick MG by real-time quantitative PCR (qPCR) using a hydrolysis probe for the citrate synthase gene (gltA) of R. rickettsii, as previously described by Galletti et al. (2013). All samples were analyzed in three technical replicates. Samples of gDNA extracted from control ticks were also tested to confirm the absence of infection.
To perform mRNA sequencing (RNA-seq) analysis, total RNA extracted from the MG of adult females were pooled, according to the following description: eight A. sculptum harboring 2.2 × 107 ± 2.4 × 107 rickettsiae and ten A. aureolatum presenting 4.8 × 107 ± 2.7 × 107 rickettsiae composed the infected samples (AsI and AaI, respectively); and eight non-infected adult females of A. sculptum and 10 non-infected A. aureolatum composed the control samples (AsC and AaC, respectively). Each tick contributed equally for the composition of the RNA pool samples. Samples were tagged and multiplex sequenced in four lanes using a HiSeq™ sequencing system (Illumina) at the North Carolina State University facility (North Carolina, USA).
Near 567 million reads of 101 base pairs were obtained using the single read mode (these reads also include the transcriptomes of SG of A. aureolatum and A. sculptum; data not shown). Reads for each species were assembled together using Abyss and Soapdenovo Trans programs with K-values varying from 21 to 91 (in 10 interval increments). Resulting assemblies were concatenated and clustered using the blastn tool (performed locally from executables obtained at the NCBI FTP site ftp://ftp.ncbi.nih.gov/blast/executables/) (Altschul et al., 1990) and CAP3 assembler (Huang and Madan, 1999) by a decreasing word size inclusion strategy, as described by Karim et al. (2011), starting at 300 and ending in 60. Coding sequences (CDSs) were extracted as detailed by Karim et al. (2011), based on matches to public databases or longer open reading frames with a signal peptide as an indicative of secretion. Data were organized in a hyperlinked spreadsheet as described by Ribeiro et al. (2004). The blastx (Altschul et al., 1997) tool was used to compare the predict amino acid sequences translated from the nucleotide sequences to the NR protein database of the NCBI and to the Gene Ontology (GO) database (Ashburner et al., 2000). The tool reverse position-specific BLAST (rpsblast) (Altschul et al., 1997) was used to search for conserved protein domains in the Pfam (Bateman et al., 2000), SMART (Schultz et al., 2000), KOG (Tatusov et al., 2003), and conserved domains databases (CDD) (Marchler-Bauer et al., 2002). To identify putative secreted proteins, predicted proteins starting with a methionine residue were submitted to the SignalP server (Nielsen et al., 1997). In addition, the program NetOGlyc (Julenius et al., 2005) was used to predict glycosylation sites on the proteins. The functional annotation of the CDSs was carried out according to the their e-values and their order of appearance on the comparisons described above, as detailed in Karim et al. (2011).
To compare the gene expression between samples [A. sculptum infected (AsI) vs. non-infected (AsC) and A. aureolatum infected (AaI) vs. non-infected (AaC)], paired comparisons of the number of reads hitting each contig were calculated by X2 tests to detect significant differences between samples, where the minimum considered value was larger than 5 and p < 0.05. Normalized fold-ratios of the sample reads were computed by adjusting the numerator by a factor based on the ratio of the total number of reads in each sample, and adding one to the denominator to avoid division by zero.
The complete dataset of A. sculptum and A. aureolatum can be downloaded from the National Center for Biotechnology Information (NCBI). The raw data were deposited to the Sequence Read Archives (SRA) of the NCBI under bioprojects numbers PRJNA343654 (A. sculptum, raw reads runs SRR4277085, SRR4277086, SRR4277087, SRR4277088, and SRR4277089), and PRJNA344771 (A. aureolatum, raw reads runs SRR4301100, SRR4301108, SRR4301110, and SRR4301120). This Transcriptome Shotgun Assembly (TSA) project has been deposited at DDBJ/EMBL/GenBank under the accession GFAA00000000 (for A. sculptum) and GFAC00000000 (for A. aureolatum). Only CDS representing 90% of known proteins or larger than 250 amino acids were deposited.
When required, phylogenetics and topology analyses of predicted proteins were performed using the program Clustal omega (European Bioinformatics Institute; EMBL-EBI) with default parameters.
Five hundred nanogram of the total RNA extracted from the MG of infected (AsI and AaI) or control (AsC and AaC) ticks were treated with RQ1 RNase-free DNase (Promega) and reverse transcribed (RT) in cDNA using M-MLV Reverse Transcriptase (ThermoFisher Scientific), as detailed by manufacturer. Resulting cDNA was used as template in qPCR with the Maxima SYBR Green/ROX qPCR MasterMix (ThermoFisher Scientific) and specific primers for selected CDSs (Supplementary Table 1). Primers were designed using Primer3 (Rozen and Skaletsky, 2000) and synthesized by ThermoFisher Scientific. qPCR was performed on a StepOnePlus™ Real-Time PCR System (ThermoFisher Scientific), using the following program: 95°C for 10 min followed by 40 cycles at 95°C for 15 s, 60°C for 60 s, and 72°C for 20 s. In addition, a melting curve analysis was carried out to check the specificity of the primers. To determine the efficiency of each pair of primers, standard curves were generated using different concentrations of cDNA (400–3.12 ng; 2-fold dilution).
The 2−ΔΔCt equation was utilized to calculate the relative expression of select CDSs in infected vs. non-infected ticks (Livak and Schmittgen, 2001). The CDS of the ribosomal protein S3A was used as reference (Supplementary Table 1). Eight biological replicates from A. sculptum and nine from A. aureolatum were analyzed. Each biological replicate corresponds to one tick MG. Student's t-test was used to statistically validate the differentially expressed CDSs. To verify the reproducibility of both gene expression measurement techniques, we calculated the Pearson's correlation coefficient between RNA-seq and RT-qPCR.
Initially, adult females of A. sculptum and A. aureolatum infected with R. rickettsii were obtained by a laboratory-controlled experimental infection. The presence of rickettsiae in midgut (MG) and salivary glands (SG) of ticks was assessed by qPCR. Only fifteen among 100 A. sculptum adult females (15%) were positively infected, while 27 from 29 A. aureolatum adult females (93%) acquired R. rickettsii. Importantly, both MG and SG of all infected ticks were colonized by R. rickettsii.
The RNA extracted from the MG of control and infected A. sculptum and A. aureolatum [A. sculptum control (AsC), A. aureolatum control (AaC), A. sculptum infected (AsI), and A. aureolatum infected (AaI)] were multiplex sequenced using an Illumina HiSeq platform. The sequencing of A. scultpum RNA samples resulted in 324 million reads of 101 base pairs, which were assembled in 9,560 CDSs. For A. aureolatum, near 242 million reads were obtained and assembled in 11,906 CDSs (Table 1).
Table 1. Functional classification of CDSs identified in the MG of A. sculptum and A. aureolatum by RNA-seq.
To identify coding sequences (CDSs) differentially expressed by infection with R. rickettsii, we compared the number of reads mapped to each contig in infected vs. non-infected A. sculptum (Table 2 and Supplementary Tables 2, 3) and A. aureolatum (Table 3 and Supplementary Tables 4, 5). Infection modulated 479 CDSs in the MG of A. sculptum, among which 416 were upregulated (Supplementary Table 3) and only 63 were downregulated (Supplementary Table 2). Differently, from 310 CDSs of A. aureolatum modulated by infection, 237 were downregulated (Supplementary Table 4) and only 73 were upregulated (Supplementary Table 5). In order to validate the transcription pattern obtained by RNA-seq, selected CDSs were analyzed by RT-qPCR (Tables 2, 3 for A. sculptum and A. aureolatum, respectively). The correlation between transcriptional data obtained by these two methods was 0.69 (p = 0.037) for A. sculptum and 0.73 (p = 0.039) for A. aureolatum, strengthening the transcriptional data obtained by RNA-seq.
Table 2. Selected CDSs of A. sculptum midgut differentially expressed by infection with R. rickettsii.
Table 3. Selected CDSs of A. aureolatum midgut differentially expressed by infection with R. rickettsii.
Most of the CDSs upregulated by R. rickettsii infection in A. sculptum belongs to metabolism, protein modification, secreted, signal transduction, transporter and channels, and transposon elements functional categories (Figure 1). Differently, CDSs in these same functional categories were majorly downregulated in A. aureolatum. Moreover, CDSs of A. sculptum in protein synthesis and storage categories were majorly downregulated by infection, while CDSs in immunity, proteasome, transcription factor, and virus categories were mostly upregulated. In A. aureolatum, CDSs in cytoskeletal category were remarkably downregulated by infection, whereas CDSs in transcription machinery and detoxification categories were upregulated (Figure 1).
Figure 1. Functional classification of CDSs differentially expressed in the midgut of R. rickettsii-infected ticks. AsR, downregulated CDSs of A. sculptum; AsI, upregulated CDSs of A. sculptum; AaR, downregulated CDSs of A. aureolatum; AaI, upregulated CDSs of A. aureolatum.
In metabolism category, 11 CDSs were upregulated by infection in A. sculptum (Supplementary Table 3), while none was downregulated (Supplementary Table 2). On the other hand, 10 CDSs inside this same category were downregulated in A. aureolatum (Supplementary Table 4), while three were upregulated (Supplementary Table 5). Among them, only one share the same annotation (pancreatic lipase-like) in both A. sculptum (Acaj-69206) and A. aureolatum (Ambaur-63833). In addition, two sequences coding glucose dehydrogenases were downregulated in A. aureolatum (AmbarSigP-52021 and AmbarSigP-66386; Table 3 and Supplementary Table 4). In A. sculptum, two glycolate oxidase CDSs (AcajSigP-74567 and AcajSigP-79349) were upregulated (Table 2 and Supplementary Table 3).
In protein modification category, three CDSs of serine carboxipeptidases (Acaj-72629, Acaj-72628, and Acaj-72627) were upregulated by infection in A. sculptum MG (Table 2 and Supplementary Table 3), while none was downregulated. Differently, in A. aureolatum, two serine carboxipeptidases CDSs (Ambaur-58705 and AmbarSigP-15638, this last one included in secreted category) were downregulated (Table 3 and Supplementary Table 4) and one (AmbarSigP-13149) was upregulated (Table 3 and Supplementary Table 5). Moreover, 15 metalloprotease CDSs (predicted to be secreted or not) were upregulated in A. sculptum (Supplementary Table 3), while eight were downregulated in A. aureolatum (Supplementary Table 4). None metalloprotease CDS was detected to be downregulated in A. sculptum or upregulated in A. aureolatum. Phylogenetics and amino acid alignment analysis showed that, among the metalloproteinases of A. aureolatum, six are reprolysins and two are astacins (Supplementary Figure 1). In relation to the metalloproteases of A. sculptum, 14 are reprolysins and only one is astacin (Supplementary Figure 1).
Among the differentially expressed sequences that code secreted proteins with annotated function, we highlight lipocalins, serine proteinase inhibitors, principally members of Kunitz family, and mucins. Twenty six lipocalin CDSs were upregulated in A. sculptum MG (Supplementary Table 3) and only two in A. aureolatum (Supplementary Table 5). On the other hand, 30 lipocalin CDSs were downregulated in A. aureolatum (Supplementary Table 4), while none was downregulated in A. sculptum. In addition, five sequences encoding Kunitz inhibitors were downregulated by infection in A. aureolatum (Table 3 and Supplementary Table 4) and only one was upregulated (Table 3 and Supplementary Table 5). An opposite panorama was observed in A. sculptum, with eight CDSs of Kunitz inhibitors upregulated by R. rickettsii infection (Table 2 and Supplementary Table 3) and none downregulated. In relation to sequences encoding mucins, one CDS (Acaj-39037) was detected to be downregulated in A. sculptum by RNA-seq analysis (Table 2 and Supplementary Table 2). In A. aureolatum, four secreted mucin CDSs (Ambaur-60941, Ambaur-63342, Ambaur-63341, and AmbarSigP-64116) were downregulated (Table 3 and Supplementary Table 4).
In signal transduction category, R. rickettsii upregulated the transcription of 11 CDSs in A. sculptum (Supplementary Table 3) and downregulated 13 CDSs in A. aureolatum (Supplementary Table 4). Two sequences inside this category, coding K+-dependent Ca2+/Na+ exchanger NCKX1, are common to both A. sculptum (AcajSigP-12807) and A. aureolatum (Ambaur-41603). Transporter and channels category also contains CDSs of A. sculptum that were upregulated by infection as well as CDSs of A. aureolatum that were downregulated (Supplementary Tables 3, 4, respectively). Nonetheless, none of those CDSs shares the same annotation in both tick species.
Cuticle proteins CDSs, which belong to external matrix category, were downregulated by infection in both A. sculptum (Acaj-76542, Acaj-80620, and Acaj-75348; Table 2 and Supplementary Table 2) and A. aureolatum (Ambaur-64626 and Ambaur-58882; Table 3 and Supplementary Table 4). In this same category, two peritrophin CDSs (Acaj-81446 and Acaj-SigP85359) were downregulated exclusively in infected A. sculptum (Table 2 and Supplementary Table 2).
In A. sculptum, infection downregulated two vitellogenin receptor CDSs (AcajSigP-26714 and AcajSigP-19478), which are comprised in storage category (Table 2 and Supplementary Table 2). Moreover, two CDSs in transcription factors (Acaj-72151: alcohol dehydrogenase transcription factor Myb/SANT-like; and Acaj-62642: transcription factor NERF) and one in proteasome machinery (AcajTE-85582: E3 ubiquitin -protein ligase RBBP6-like isoform X3) were exclusively upregulated in A. sculptum (Supplementary Table 3). In A. aureolatum, nine CDSs in detoxification category were upregulated by infection, among which seven code cytochrome P450 (Supplementary Table 5). In A. sculptum, two cytochrome P450 CDSs (AcajSigP-19690 and AcajSigP-26888) were also upregulated by infection (Supplementary Table 3), while one superoxide dismutase (SOD) CDS (Acaj-52926) was downregulated (Supplementary Table 2). Seven CDSs in transcription machinery category, among which six code the regulator of rDNA transcription protein 15, were also upregulated by R. rickettsii in A. aureolatum MG (Supplementary Table 5).
Infection upregulated a higher number of CDS of immune-related proteins in A. sculptum than in A. aureolatum (Figure 1). Lysozyme, ixoderin, and peptidoglycan recognition protein (PGRP) CDSs were upregulated by infection in both tick species (Tables 2, 3, and Supplementary Tables 3, 5). In A. sculptum, R. rickettsii also upregulated the expression of two CDSs (Acaj-48379 and Acaj-74395) that code antimicrobial peptides (AMPs) similar to the defensin of Amblyomma americanum, named amercin (Table 2 and Supplementary Table 3). In addition, one CDS of a protein containing ML domain (MD-2-related lipid-recognition) (AcajSigP-6765) was upregulated in A. sculptum by infection (Table 2 and Supplementary Table S3). In A. aureolatum, sequences coding one 5.3 kDa AMP (Ambaur-19862) and one AMP similar to the ixodidin of Rhipicephalus microplus (Ambaur-53938), which exhibit chymotrypsin-elastase inhibitory activity, were downregulated (Table 3 and Supplementary Table 4). In A. sculptum, three tumor necrosis factor receptor-associated factor (TRAF) CDSs (Acaj-43466, Acaj-36823, and Acaj-70988; Table 2 and Supplementary Table 2) were downregulated, while one was upregulated (Acaj-51987; Table 2 and Supplementary Table 3). In A. aureolatum, only one CDS encoding TRAF (Ambaur-58673) was detected as downregulated (Table 3 and Supplementary Table 4).
As expected, several differentially expressed sequences encode hypothetical proteins or unknown products in both tick species (Figure 1 and Supplementary Tables 2–5). Remarkably, the majority of those sequences encode proteins that are predicted to be secreted in A. sculptum (140 among 204 sequences) and in A. aureolatum (61 among 117 sequences).
In the current study, laboratory colonies of two different species of ticks that are vectors of R. rickettsii in Brazil were fed on infected hosts, mimicking a natural infection. While 93% of A. aureolatum females acquired R. rickettsii, only 15% of A. sculptum females were positively infected. This result reinforces the differences in susceptibility of these two tick species to infection with R. rickettsii, as previously reported by Labruna et al. (2008).
It is known that pathogens acquired within the blood meal have to overcome several barriers in ticks to be successfully transmitted to another host (Kopacek et al., 2010; Hajdusek et al., 2013). Firstly, the pathogen must colonize MG and later the salivary glands (SG) through hemolymph. Our data showed that both MG and SG of all positively infected A. aureolatum and A. sculptum ticks were colonized by R. rickettsii. We did not observed ticks with infection restricted to MG, suggesting that if this barrier is broken, this pathogen is able to reach the SG. Therefore, the tick MG probably plays a key role in controlling rickettsial infection. To get better insights on the factors that control infection in tick MG, the global gene expression profile of infected vs. non-infected (control) ticks was determined by RNA sequencing (RNA-seq) and validated using RT-qPCR. The correlation between RNA-seq and RT-qPCR (around 70%) strengthened the transcriptional findings of the current study.
Most CDSs of A. aureolatum that were differentially expressed by infection with R. rickettsii were downregulated (87%). On the contrary, the majority of CDSs of infected A. sculptum were induced (76%). Interestingly, many upregulated CDSs of A. sculptum belong to the same functional categories of most downregulated CDSs of A. aureolatum, for instance, metabolism, protein modification, and secreted proteins. Further studies are required to determine the role played by CDSs within those functional categories in delineating the differences in susceptibility of these two tick species to infection.
Among CDSs associated to metabolism that were induced by infection in A. sculptum, we highlight two glycolate oxidases. In plants, this enzyme converts glycolate to oxalate producing hydrogen peroxide (H2O2) (Rojas and Mysore, 2012). The upregulation of such oxidases upon infection might represent an attempt of ticks to control R. rickettsii through production of H2O2. Indeed, production of ROS, including superoxide () and H2O2, is an ancient immune response against invader pathogens (Zug and Hammerstein, 2015). It was previously reported that R. rickettsii infection induces a pro-oxidant response in human endothelial cells (Santucci et al., 1992; Devamanoharan et al., 1994), causing oxidative cell injury (Eremeeva et al., 2001). The stimulation of hemocytes of the tick R. microplus with the Gram-positive bacterium Micrococcus luteus also leaded to production of ROS. This activity was abolished by addition of superoxide dismutase (SOD) and catalase, suggesting that both superoxide and H2O2 are produced upon microbial challenge (Pereira et al., 2001). Besides upregulation of glycolate oxidase, one SOD CDS was downregulated by infection in A. sculptum, strengthening that the MG is under oxidative stress upon infection. In A. aureolatum, two glucose dehydrogenase encoding genes were downregulated. This enzyme reduces NADP to NADPH. Electrons from NADPH can be used by glutathione reductase to regenerate the intracellular pool of reduced glutathione (Mishra and Imlay, 2012), which, in turn, is important to reduce H2O2 into H2O by action of glutathione peroxidase (Wang et al., 2013). Alternatively, intracellular NADPH can act as the electron donor to the NADPH oxidase complex to catalyze the reduction of molecular oxygen to superoxide in phagocyte membranes (Wang et al., 2013). Therefore, glucose dehydrogenase play an important role in maintaining the cellular redox homeostasis. In a previous study, our research group showed that antioxidant enzymes of R. rickettsii (thioredoxin peroxidase 1, glutaredoxin 3, ferredoxin, and also one hypothetical protein A1G_00185 with a thioredoxin domain) are upregulated in MG of fed A. aureolatum, which may protect R. rickettsii against the deleterious effects of free radicals (Galletti et al., 2013). Then, it is plausible to suppose that the MG of fed infected A. aureolatum is under oxidative stress.
It is known that the main proteolytic activities in the MG of ticks are acidic aspartic and cysteine proteinases, whereas exopeptidases (the cysteine amino- and carboxy-dipeptidases cathepsins C and B, respectively) may participate in a later stage of digestion (Horn et al., 2009; Cruz et al., 2010). In addition, two monopeptidases, namely serine carboxypeptidase (Motobu et al., 2007) and leucine aminopeptidase (Hatta et al., 2006), would be possibly involved in the final stage of digestion, liberating free amino acids (Horn et al., 2009). Digestion of hemoglobin, as well as other proteins within the blood meal, is important not only to provide nutrients and energy for molting and vitellogenesis processes, but also to generate fragments with antimicrobial properties (Fogaca et al., 1999; Nakajima et al., 2003; Sonenshine et al., 2005; Belmonte et al., 2012). These antimicrobial fragments, named hemocidins, are probably involved in protection of tick MG against proliferation of pathogens (Kopacek et al., 2010; Hajdusek et al., 2013). We did not detect the transcriptional regulation of aspartic and cysteine proteinases by infection. However, three serine carboxipeptidase CDSs were upregulated in A. sculptum, while two were downregulated in A. aureolatum. As serine carboxypeptidases are involved in the final stage of hemoglobin digestion (Motobu et al., 2007), studies to determine the contents of hemocidins in MG of Amblyomma ticks are warranted to determine the role of these peptides in controlling infection.
Infection also modulated the expression of metalloproteases in tick MG, upregulating 15 CDSs in A. sculptum and downregulating eight CDSs in A. aureolatum. No metalloprotease CDS was detected to be upregulated in A. aureolatum or downregulated in A. sculptum. It is known that metalloproteinases of tick saliva, which belong to metzincin family, are essential for both the initial and late feeding stages (Francischetti et al., 2003, 2005a). During the initial feeding stage, these enzymes inhibit blood clotting and degrade extracellular matrix proteins, which is essential for the preparation of the feeding site. As metalloproteases also present anti-angiogenic activity, they are also important in the late feeding stage, inhibiting host tissue repair. Metalloproteases from metzincin family present a zinc-binding consensus motif (HEXXHXXG/NXXH/D, where X corresponds to any amino acid residue) in the active site and a conserved methionine-containing turn (Met-turn) that underlies the active site. According to their structure, metzincins are subdivided in other families, among which are reprolysin and astacin family (Gomis-Ruth, 2009), where tick metalloproteases are included. It was previously reported that reprolysins are majorly expressed in the SG of the tick R. microplus, while astacin transcripts are more abundant in the MG and ovaries (Barnard et al., 2012). Importantly, the knockdown of both reprolysins and astacins leaded to a reduction of both oviposition and egg weight, demonstrating that those proteins correspond to promising targets for development of strategies to protect the cattle against ticks (Barnard et al., 2012). Indeed, the immunization of bovines with the reprolysin BrRm-MP4 diminished the feeding and reproductive rates of R. microplus females (Ali et al., 2015), strengthening the potential of metalloproteinases as vaccine candidates. Among the metalloproteinases from A. aureolatum, six are reprolysins and two are astacins, while 14 of the metalloproteases of A. sculptum are reprolysins and only one is astacin.
Many CDSs of both annotated and hypothetical/unknown function proteins that contain signal peptide for secretion were modulated by infection. Importantly, most of the proteins predicted to be secreted were downregulated in A. aureolatum and induced in A. sculptum. Among them, lipocalins, proteins containing Kunitz domain, and mucins are prominent. R. rickettsii infection upregulated 26 lipocalin CDSs in A. sculptum and downregulated 30 CDSs in A. aureolatum. It is known that lipocalins bind histamine and serotonin (Paesen et al., 1999; Sangamnatdej et al., 2002; Francischetti et al., 2009), which in high concentrations on the feeding pool can affect attachment, feeding efficiency, and reproductive success (Kemp and Bourne, 1980; Paine et al., 1983). Therefore, these anti-inflammatory proteins are extensively detected to be present in tick saliva (Francischetti et al., 2005b; Ribeiro et al., 2006; Alarcon-Chaidez et al., 2007; Karim et al., 2011; Karim and Ribeiro, 2015). The unique non-salivary gland lipocalin described in ticks is savicalin from hemocytes of Ornithodoros savignyi. Importantly, savicalin transcripts were also detected to be present in MG and ovaries (Cheng et al., 2010). Therefore, additional studies should be addressed to determine the site of production and the role played by lipocalins in protection of Amblyomma ticks against infection.
In addition, CDSs of secreted proteins encoding serine protease inhibitors, predominantly Kunitz inhibitors, were upregulated in A. sculptum and downregulated in A. aureolatum. Serine proteinase inhibitors were already enrolled in immune reactions of arthropods, mediating both coagulation and melanization processes of the hemolymph and also the production of AMPs (Gulley et al., 2013). These inhibitors may also control pathogen proliferation by inhibiting proteinases they use to colonize the host tissues and evade immune system (Armstrong, 2001). In addition, Kunitz inhibitors are widely described to be produced by ticks, inhibiting blood coagulation and facilitating tick feeding (Corral-Rodriguez et al., 2009). The differential expression of tick Kunitz inhibitors by Dermacentor variabilis upon an infection with the avirulent Rickettsia montanensis was previously reported (Ceraul et al., 2008). Moreover, it was shown that this Kunitz inhibitor exhibits a bacteriostatic effect against R. montanensis (Ceraul et al., 2008) and that its transcription knockdown leads to an increase in the susceptibility of D. variabilis to infection (Ceraul et al., 2011).
Some mucins predicted to be secreted were also detected to be differentially expressed in infected ticks. These proteins are lipid binding proteins that constitute an acellular barrier that promote protection of the MG epithelium. Mucins were already reported to be present in MG of ticks, such as D. variabilis (Anderson et al., 2008), as well as in SG (Karim et al., 2011; Ribeiro et al., 2011). Interestingly, D. variabilis mucins exhibit similarity to insect peritrophins (Anderson et al., 2008). Two peritrophin CDSs and one secreted mucin CDS were detected to be downregulated in A. sculptum by RNA-seq analysis. Peritrophin encoding sequences were not detected to be modulated by infection in A. aureolatum. However, four secreted mucin CDSs were downregulated in this tick species. It was previously reported that the knockdown of the peritrophin 1 of Ixodes scapularis reduces the thickness of the peritrophic matrix, impairing the colonization of the tick MG by Borrelia burgdorferi, causative agent of Lyme disease (Narasimhan et al., 2014). Similar effects of peritrophin 1 knockdown was observed when ticks were treated with antibiotics, altering the MG microbiota (Narasimhan et al., 2014). Indeed, ticks harbor a microbiota that can be modified, for instance, upon the treatment with antibiotics (Narasimhan et al., 2014; Clayton et al., 2015; Abraham et al., 2017). Interestingly, a recent study showed that infection with Anaplasma phagocytophilum, causative agent of human granulocytic anaplasmosis, also alter the composition of the microbiota of I. scapularis MG through the upregulation of the tick anti-freeze glycoprotein (iafgp) (Abraham et al., 2017). Iafgp binds bacterial peptidoglycan, negatively affecting biofilm formation and, consequently, altering microbiota. Differentially from the effect previously observed for B. burgdorferi (Narasimhan et al., 2014), the remodeling of the microbiota of I. scapularis MG, which diminishes the thickness peritrophic matrix, increased the load of A. phagocytophilum (Abraham et al., 2017). It is possible that the MG of A. sculptum and A. aureolatum harbor a distinct microbiota, which might play a role in delineating susceptibility to infection. In addition, the distinct transcriptional profile triggered by R. rickettsii infection may also exert a different impact on the MG microbiota of these two ticks, resulting in differences in susceptibility. Then, studies to determine the composition of the microbiota of the MG of A. sculptum and A. aureolatum infected or not with R. rickettsii are warranted.
It is known that R. rickettsii exerts a detrimental effect on ticks, reducing both survival and reproductive rates (Burgdorfer and Brinton, 1975; Niebylski et al., 1999; Labruna et al., 2008; Soares et al., 2012). Our data showed that CDSs of cuticle proteins were downregulated by infection in both A. sculptum and in A. aureolatum. The expansion of the cuticle is crucial for the engorgement of the tick female (Flynn and Kaufman, 2011), assuring that an enough amount of blood be acquired for egg production. In addition to cuticle proteins, two vitellogenin receptor CDSs were detected to be downregulated by infection in A. sculptum. Therefore, it is possible that the downregulation of cuticle proteins and vitellogenin receptors might be involved in the reduction of both oviposition and survival rates in infected ticks.
Infection modulated CDSs of immune-related proteins in both species of ticks. Ixoderins, which are recognition proteins that possesses a C-terminal domain with a high homology to fibrinogen and fibrinogen-related proteins (FREPs), were upregulated by infection in both A. sculptum and A. aureolatum. Two isoforms of ixoderins, A and B, were identified in the hard tick Ixodes ricinus (Rego et al., 2005) and possess similarity to the lectin Dorin M of the soft tick Ornithodorus moubata (Kovar et al., 2000). Infection with R. rickettsii also induced expression of PGRP CDSs in MG of both A. sculptum and A. aureolatum. Depending on the presence of an amidase catalytic site, those proteins are classified into non-catalytic or catalytic. Non-catalytic PGRPs function as pathogen pattern recognition receptors and activate the Toll and Imd pathways in Drosophila upon infection, while catalytic PGRPs cleaves peptidoglycan, acting, therefore, as negative regulators of the immune response (by removing peptidoglycan) or as effectors (by killing bacteria) (Palmer and Jiggins, 2015). The PGRPs of both A. sculptum and A. aureolatum exhibit amidase catalytic site, suggesting they might play a role as effectors or negative regulators of tick immune signaling pathways. One protein containing ML domain was also induced in A. sculptum by infection. Genes encoding ML-domain containing proteins were detected to be expressed in MG of I. ricinus (Rudenko et al., 2005; Horackova et al., 2010). The function of such proteins in tick gut is not known, but is likely that they might be involved in response against invader pathogens and/or lipid metabolism (Inohara and Nunez, 2002).
The production of AMPs in tick MG is important, as the slow intracellular digestion of nutrients and neutral pH may favor the proliferation of microorganisms (Kopacek et al., 2010; Hajdusek et al., 2013). One lysozyme CDS was induced by infection in both A. sculptum and A. aureolatum. In A. sculptum, infection also induced the expression of two CDSs that code AMPs similar to the defensin of A. americanum, named amercin (Todd et al., 2007). Conversely, sequences encoding one 5.3-kDa AMP and one AMP with chymotrypsin-elastase inhibitory activity, similar to the ixodidin of R. microplus (Fogaca et al., 2006), were downregulated in the MG of infected A. aureolatum. These results suggest that the MG of A. sculptum might be more hostile to R. rickettsii than the MG of A. aureolatum. Then, functional studies are required to determine the importance of AMPs in protection of Amblyomma ticks against rickettsiae.
In insects, it is well-known that transcription of AMPs, as well as other immune effectors, is mainly regulated by the intracellular signaling pathways Toll, Immune deficiency (Imd), JNK (Jun-N-terminal kinase), and Jak/Stat (Ferrandon et al., 2007; Souza-Neto et al., 2009; Kleino and Silverman, 2014). Much less information is available on the immune signaling pathways in ticks. Immune signaling pathway components were identified in the genome of I. scapularis (Smith and Pal, 2014; Kotsyfakis et al., 2015; Gulia-Nuss et al., 2016). It was recently reported that Toll and Jak-Stat insect immune signaling pathway components are well conserved in ticks. Conversely, ticks lack some IMD signaling pathway components, such as the adaptor protein IMD, its associated molecule FAAD (Fas associated protein with death domain), the caspase DREDD (death related ced-3/Nedd2-like), and the negative regulators Pirk (poor IMD response upon knock-in) and Dnr1 (defense repressor 1) (Rosa et al., 2016). This same study has also shown that A. marginale downregulates the expression of immune signaling pathway components in an embryonic cell line (BME26) of R. microplus, its biological vector, while other microbial stimuli, including R. rickettsii, induce their expression. In addition, it was previously reported that the Jak/Stat pathway regulates the expression of 5.3-kDa AMP, which is essential to prevent A. phagocytophilum infection in I. scapularis (Liu et al., 2012). Jak/Stat pathway was also enrolled in controlling the production of peritrofin-1, which is essential for the successful colonization of I. scapularis MG by B. burgdorferi (Narasimhan et al., 2014). Interestingly, TRAF, a downstream member of the Toll pathway, was differentially expressed in both A. sculptum and A. aureolatum. Therefore, functional studies are warranted to determine the role played by immune pathways in the control of rickettsial infection in Amblyomma ticks.
In conclusion, the current study shows that infection with R. rickettsii elicits a distinct transcriptional profile in the MG of Amblyomma sculptum and A. aureolatum, which might be responsible for their differences in susceptibility to infection. The proteins encoded by differentially expressed CDSs should be functionally characterized and might be targets for development of blocking vaccines. As the genome of A. sculptum and A. aureolatum is unavailable, this study provides a rich source of sequences in the genus Amblyomma.
Designed the experiments: ACF. Generated biological samples: LM, MG, and FC. Performed the experiments: LM and MG. Analyzed RT-qPCR data: LM, MG, and AF. Performed bioinformatics data analysis: JR. Performed statistic data analysis: JR, AF. Contributed reagents/materials/analysis tools: JR, AF, ML, SD, and ACF. Wrote the paper: LM, MG, and ACF. All authors read and approved the final manuscript.
This work was supported by funds from the São Paulo Research Foundation (FAPESP; Grants 2008/053570-0, 2013/26450-2, and 2014/11513-1), the National Council for Scientific and Technological Development [CNPq; grants CNPq 573959/2008-0; The National Institutes of Science and Technology Program in Molecular Entomology (INCT-EM)], the Coordination for the Improvement of Higher Education Personnel (CAPES), and the Provost for Research of the University of São Paulo [Research Support Center on Bioactive Molecules from Arthropod Vectors (NAP-MOBIARVE 12.1.17661.1.7)]. MG and LM were respectively supported by doctoral and master's fellowships from FAPESP.
The authors declare that the research was conducted in the absence of any commercial or financial relationships that could be construed as a potential conflict of interest.
We would like to thank Adriano Pinter, Camila Dantas Malossi, João F. Soares, and Hebert S. Soares for technical assistance in tick colony maintenance.
The Supplementary Material for this article can be found online at: http://journal.frontiersin.org/article/10.3389/fcimb.2017.00129/full#supplementary-material
MG, Midgut; SG, salivary glands; AsC, Amblyomma sculptum control; AaC, Amblyomma aureolatum control; AsI, Amblyomma sculptum infected; AaI, Amblyomma aureolatum infected; CDSs, coding sequences.
Abraham, N. M., Liu, L., Jutras, B. L., Yadav, A. K., Narasimhan, S., Gopalakrishnan, V., et al. (2017). Pathogen-mediated manipulation of arthropod microbiota to promote infection. Proc. Natl. Acad. Sci. U.S.A. 114, E781–E790. doi: 10.1073/pnas.1613422114
Alarcon-Chaidez, F. J., Sun, J., and Wikel, S. K. (2007). Transcriptome analysis of the salivary glands of Dermacentor andersoni Stiles (Acari: Ixodidae). Insect Biochem. Mol. Biol. 37, 48–71. doi: 10.1016/j.ibmb.2006.10.002
Ali, A., Parizi, L. F., Guizzo, M. G., Tirloni, L., Seixas, A., Vaz Ida, S. Jr., et al. (2015). Immunoprotective potential of a Rhipicephalus (Boophilus) microplus metalloprotease. Vet. Parasitol. 207, 107–114. doi: 10.1016/j.vetpar.2014.11.007
Altschul, S. F., Gish, W., Miller, W., Myers, E. W., and Lipman, D. J. (1990). Basic local alignment search tool. J. Mol. Biol. 215, 403–410. doi: 10.1016/S0022-2836(05)80360-2
Altschul, S. F., Madden, T. L., Schaffer, A. A., Zhang, J., Zhang, Z., Miller, W., et al. (1997). Gapped BLAST and PSI-BLAST: a new generation of protein database search programs. Nucleic Acids Res. 25, 3389–3402. doi: 10.1093/nar/25.17.3389
Anderson, J. M., Sonenshine, D. E., and Valenzuela, J. G. (2008). Exploring the mialome of ticks: an annotated catalogue of midgut transcripts from the hard tick, Dermacentor variabilis (Acari: Ixodidae). BMC Genomics. 9:552. doi: 10.1186/1471-2164-9-552
Armstrong, P. B. (2001). The contribution of proteinase inhibitors to immune defense. Trends Immunol. 22, 47–52. doi: 10.1016/S1471-4906(00)01803-2
Ashburner, M., Ball, C. A., Blake, J. A., Botstein, D., Butler, H., Cherry, J. M., et al. (2000). Gene ontology: tool for the unification of biology. Gene Ontol. Consort. Nat. Genet. 25, 25–29. doi: 10.1038/75556
Barnard, A. C., Nijhof, A. M., Gaspar, A. R., Neitz, A. W., Jongejan, F., and Maritz-Olivier, C. (2012). Expression profiling, gene silencing and transcriptional networking of metzincin metalloproteases in the cattle tick, Rhipicephalus (Boophilus) microplus. Vet. Parasitol. 186, 403–414. doi: 10.1016/j.vetpar.2011.11.026
Bateman, A., Birney, E., Durbin, R., Eddy, S. R., Howe, K. L., and Sonnhammer, E. L. (2000). The Pfam protein families database. Nucleic Acids Res. 28, 263–266. doi: 10.1093/nar/28.1.263
Belmonte, R., Cruz, C. E., Pires, J. R., and Daffre, S. (2012). Purification and characterization of Hb 98-114: a novel hemoglobin-derived antimicrobial peptide from the midgut of Rhipicephalus (Boophilus) microplus. Peptides 37, 120–127. doi: 10.1016/j.peptides.2012.05.017
Burgdorfer, W. (1988). “Ecological and epidemiological considerations of rock mountain spotted fever and scrub typhus,” in Biology of Rickettsial Diseases, Vol. 1, ed D. H. Walker (Boca Raton, FL: CRC Inc), 33–50.
Burgdorfer, W., and Brinton, L. P. (1975). Mechanisms of transovarial infection of spotted fever Rickettsiae in ticks. Ann. N. Y. Acad. Sci. 266, 61–72. doi: 10.1111/j.1749-6632.1975.tb35088.x
Ceraul, S. M., Chung, A., Sears, K. T., Popov, V. L., Beier-Sexton, M., Rahman, M. S., et al. (2011). A Kunitz protease inhibitor from Dermacentor variabilis, a vector for spotted fever group rickettsiae, limits Rickettsia montanensis invasion. Infect. Immun. 79, 321–329. doi: 10.1128/IAI.00362-10
Ceraul, S. M., Dreher-Lesnick, S. M., Gillespie, J. J., Rahman, M. S., and Azad, A. F. (2007). New tick defensin isoform and antimicrobial gene expression in response to Rickettsia montanensis challenge. Infect. Immun. 75, 1973–1983. doi: 10.1128/IAI.01815-06
Ceraul, S. M., Dreher-Lesnick, S. M., Mulenga, A., Rahman, M. S., and Azad, A. F. (2008). Functional characterization and novel rickettsiostatic effects of a Kunitz-type serine protease inhibitor from the tick Dermacentor variabilis. Infect. Immun. 76, 5429–5435. doi: 10.1128/IAI.00866-08
Cheng, P. H., Mans, B. J., Neitz, A. W., and Gaspar, A. R. (2010). Savicalin, a lipocalin from hemocytes of the soft tick, Ornithodoros savignyi. Exp. Appl. Acarol. 52, 313–326. doi: 10.1007/s10493-010-9368-6
Clayton, K. A., Gall, C. A., Mason, K. L., Scoles, G. A., and Brayton, K. A. (2015). The characterization and manipulation of the bacterial microbiome of the Rocky Mountain wood tick, Dermacentor andersoni. Parasit. Vectors. 8:632. doi: 10.1186/s13071-015-1245-z
Corral-Rodriguez, M. A., Macedo-Ribeiro, S., Barbosa Pereira, P. J., and Fuentes-Prior, P. (2009). Tick-derived Kunitz-type inhibitors as antihemostatic factors. Insect Biochem. Mol. Biol. 39, 579–595. doi: 10.1016/j.ibmb.2009.07.003
Cruz, C. E., Fogaca, A. C., Nakayasu, E. S., Angeli, C. B., Belmonte, R., Almeida, I. C., et al. (2010). Characterization of proteinases from the midgut of Rhipicephalus (Boophilus) microplus involved in the generation of antimicrobial peptides. Parasit. Vectors. 3:63. doi: 10.1186/1756-3305-3-63
Devamanoharan, P. S., Santucci, L. A., Hong, J. E., Tian, X., and Silverman, D. J. (1994). Infection of human endothelial cells by Rickettsia rickettsii causes a significant reduction in the levels of key enzymes involved in protection against oxidative injury. Infect. Immun. 62, 2619–2621.
Eremeeva, M. E., Dasch, G. A., and Silverman, D. J. (2001). Quantitative analyses of variations in the injury of endothelial cells elicited by 11 isolates of Rickettsia rickettsii. Clin. Diagn. Lab. Immunol. 8, 788–796. doi: 10.1128/cdli.8.4.788-796.2001
Ferrandon, D., Imler, J. L., Hetru, C., and Hoffmann, J. A. (2007). The Drosophila systemic immune response: sensing and signalling during bacterial and fungal infections. Nat. Rev. Immunol. 7, 862–874. doi: 10.1038/nri2194
Flynn, P. C., and Kaufman, W. R. (2011). Female ixodid ticks grow endocuticle during the rapid phase of engorgement. Exp. Appl. Acarol. 53, 167–178. doi: 10.1007/s10493-010-9393-5
Fogaca, A. C., Almeida, I. C., Eberlin, M. N., Tanaka, A. S., Bulet, P., and Daffre, S. (2006). Ixodidin, a novel antimicrobial peptide from the hemocytes of the cattle tick Boophilus microplus with inhibitory activity against serine proteinases. Peptides 27, 667–674. doi: 10.1016/j.peptides.2005.07.013
Fogaca, A. C., da Silva, P. I. Jr., Miranda, M. T., Bianchi, A. G., Miranda, A., Ribolla, P. E., et al. (1999). Antimicrobial activity of a bovine hemoglobin fragment in the tick Boophilus microplus. J. Biol. Chem. 274, 25330–25334. doi: 10.1074/jbc.274.36.25330
Francischetti, I. M., Mather, T. N., and Ribeiro, J. M. (2003). Cloning of a salivary gland metalloprotease and characterization of gelatinase and fibrin(ogen)lytic activities in the saliva of the Lyme disease tick vector Ixodes scapularis. Biochem. Biophys. Res. Commun. 305, 869–875. doi: 10.1016/S0006-291X(03)00857-X
Francischetti, I. M., Mather, T. N., and Ribeiro, J. M. (2005a). Tick saliva is a potent inhibitor of endothelial cell proliferation and angiogenesis. Thromb. Haemost. 94, 167–174. doi: 10.1160/TH04-09-0566
Francischetti, I. M., My Pham, V., Mans, B. J., Andersen, J. F., Mather, T. N., Lane, R. S., et al. (2005b). The transcriptome of the salivary glands of the female western black-legged tick Ixodes pacificus (Acari: Ixodidae). Insect Biochem. Mol. Biol. 35, 1142–1161. doi: 10.1016/j.ibmb.2005.05.007
Francischetti, I. M., Sa-Nunes, A., Mans, B. J., Santos, I. M., and Ribeiro, J. M. (2009). The role of saliva in tick feeding. Front. Biosci. 14, 2051–2088. doi: 10.2741/3363
Galletti, M. F., Fujita, A., Nishiyama, M. Y. Jr., Malossi, C. D., Pinter, A., Soares, J. F., et al. (2013). Natural blood feeding and temperature shift modulate the global transcriptional profile of Rickettsia rickettsii infecting its tick vector. PLoS ONE 8:e77388. doi: 10.1371/journal.pone.0077388
Gomis-Ruth, F. X. (2009). Catalytic domain architecture of metzincin metalloproteases. J. Biol. Chem. 284, 15353–15357. doi: 10.1074/jbc.R800069200
Grunclova, L., Fouquier, H., Hypsa, V., and Kopacek, P. (2003). Lysozyme from the gut of the soft tick Ornithodoros moubata: the sequence, phylogeny and post-feeding regulation. Dev. Comp. Immunol. 27, 651–660. doi: 10.1016/S0145-305X(03)00052-1
Gulia-Nuss, M., Nuss, A. B., Meyer, J. M., Sonenshine, D. E., Roe, R. M., Waterhouse, R. M., et al. (2016). Genomic insights into the Ixodes scapularis tick vector of Lyme disease. Nat. Commun. 7:10507. doi: 10.1038/ncomms10507
Gulley, M. M., Zhang, X., and Michel, K. (2013). The roles of serpins in mosquito immunology and physiology. J. Insect Physiol. 59, 138–147. doi: 10.1016/j.jinsphys.2012.08.015
Hajdusek, O., Sima, R., Ayllon, N., Jalovecka, M., Perner, J., de la Fuente, J., et al. (2013). Interaction of the tick immune system with transmitted pathogens. Front. Cell. Infect. Microbiol. 3:26. doi: 10.3389/fcimb.2013.00026
Hatta, T., Kazama, K., Miyoshi, T., Umemiya, R., Liao, M., Inoue, N., et al. (2006). Identification and characterisation of a leucine aminopeptidase from the hard tick Haemaphysalis longicornis. Int. J. Parasitol. 36, 1123–1132. doi: 10.1016/j.ijpara.2006.05.010
Horackova, J., Rudenko, N., Golovchenko, M., and Grubhoffer, L. (2010). Der-p2 (Dermatophagoides pteronyssinus) allergen-like protein from the hard tick Ixodes ricinus-a novel member of ML (MD-2-related lipid-recognition) domain protein family. Parasitology 137, 1139–1149. doi: 10.1017/S0031182009992083
Horn, M., Nussbaumerova, M., Sanda, M., Kovarova, Z., Srba, J., Franta, Z., et al. (2009). Hemoglobin digestion in blood-feeding ticks: mapping a multipeptidase pathway by functional proteomics. Chem. Biol. 16, 1053–1063. doi: 10.1016/j.chembiol.2009.09.009
Huang, X., and Madan, A. (1999). CAP3: a DNA sequence assembly program. Genome Res. 9, 868–877. doi: 10.1101/gr.9.9.868
Hynes, W. L., Ceraul, S. M., Todd, S. M., Seguin, K. C., and Sonenshine, D. E. (2005). A defensin-like gene expressed in the black-legged tick, Ixodes scapularis. Med. Vet. Entomol. 19, 339–344. doi: 10.1111/j.1365-2915.2005.00579.x
Inohara, N., and Nunez, G. (2002). ML–a conserved domain involved in innate immunity and lipid metabolism. Trends Biochem. Sci. 27, 219–221. doi: 10.1016/S0968-0004(02)02084-4
Julenius, K., Molgaard, A., Gupta, R., and Brunak, S. (2005). Prediction, conservation analysis, and structural characterization of mammalian mucin-type O-glycosylation sites. Glycobiology 15, 153–164. doi: 10.1093/glycob/cwh151
Karim, S., and Ribeiro, J. M. (2015). An Insight into the sialome of the lone star tick, Amblyomma americanum, with a Glimpse on its time dependent gene expression. PLoS ONE 10:e0131292. doi: 10.1371/journal.pone.0131292
Karim, S., Singh, P., and Ribeiro, J. M. (2011). A deep insight into the sialotranscriptome of the gulf coast tick, Amblyomma maculatum. PLoS ONE 6:e28525. doi: 10.1371/journal.pone.0028525
Kemp, D. H., and Bourne, A. (1980). Boophilus microplus: the effect of histamine on the attachment of cattle-tick larvae–studies in vivo and in vitro. Parasitology 80, 487–496. doi: 10.1017/S0031182000000950
Kleino, A., and Silverman, N. (2014). The Drosophila IMD pathway in the activation of the humoral immune response. Dev. Comp. Immunol. 42, 25–35. doi: 10.1016/j.dci.2013.05.014
Kopacek, P., Hajdusek, O., Buresova, V., and Daffre, S. (2010). Tick innate immunity. Adv. Exp. Med. Biol. 708, 137–162. doi: 10.1007/978-1-4419-8059-5_8
Kopacek, P., Vogt, R., Jindrak, L., Weise, C., and Safarik, I. (1999). Purification and characterization of the lysozyme from the gut of the soft tick Ornithodoros moubata. Insect Biochem. Mol. Biol. 29, 989–997. doi: 10.1016/S0965-1748(99)00075-2
Kotsyfakis, M., Kopacek, P., Franta, Z., Pedra, J. H., and Ribeiro, J. M. (2015). Deep sequencing analysis of the ixodes ricinus Haemocytome. PLoS Negl. Trop. Dis. 9:e0003754. doi: 10.1371/journal.pntd.0003754
Kovar, V., Kopacek, P., and Grubhoffer, L. (2000). Isolation and characterization of Dorin, M., a lectin from plasma of the soft tick Ornithodoros moubata. Insect Biochem. Mol. Biol. 30, 195–205. doi: 10.1016/S0965-1748(99)00107-1
Labruna, M. B. (2009). Ecology of rickettsia in South America. Ann. N. Y. Acad. Sci. 1166, 156–166. doi: 10.1111/j.1749-6632.2009.04516.x
Labruna, M. B., Ogrzewalska, M., Martins, T. F., Pinter, A., and Horta, M. C. (2008). Comparative susceptibility of larval stages of Amblyomma aureolatum, Amblyomma cajennense, and Rhipicephalus sanguineus to infection by Rickettsia rickettsii. J. Med. Entomol. 45, 1156–1159. doi: 10.1093/jmedent/45.6.1156
Liu, L., Dai, J., Zhao, Y. O., Narasimhan, S., Yang, Y., Zhang, L., et al. (2012). Ixodes scapularis JAK-STAT pathway regulates tick antimicrobial peptides, thereby controlling the agent of human granulocytic anaplasmosis. J. Infect. Dis. 206, 1233–1241. doi: 10.1093/infdis/jis484
Livak, K. J., and Schmittgen, T. D. (2001). Analysis of relative gene expression data using real-time quantitative PCR and the 2−ΔΔCT Method. Methods 25, 402–408. doi: 10.1006/meth.2001.1262
Marchler-Bauer, A., Panchenko, A. R., Shoemaker, B. A., Thiessen, P. A., Geer, L. Y., and Bryant, S. H. (2002). CDD: a database of conserved domain alignments with links to domain three-dimensional structure. Nucleic Acids Res. 30, 281–283. doi: 10.1093/nar/30.1.281
Mishra, S., and Imlay, J. (2012). Why do bacteria use so many enzymes to scavenge hydrogen peroxide? Arch. Biochem. Biophys. 525, 145–160. doi: 10.1016/j.abb.2012.04.014
Motobu, M., Tsuji, N., Miyoshi, T., Huang, X., Islam, M. K., Alim, M. A., et al. (2007). Molecular characterization of a blood-induced serine carboxypeptidase from the ixodid tick Haemaphysalis longicornis. FEBS J. 274, 3299–3312. doi: 10.1111/j.1742-4658.2007.05852.x
Nakajima, Y., van der Goes van Naters-Yasui, A., Taylor, D., and Yamakawa, M. (2001). Two isoforms of a member of the arthropod defensin family from the soft tick, Ornithodoros moubata (Acari: Argasidae). Insect Biochem. Mol. Biol. 31, 747–751. doi: 10.1016/S0965-1748(01)00066-2
Nakajima, Y., van der Goes van Naters-Yasui, A., Taylor, D., and Yamakawa, M. (2002). Antibacterial peptide defensin is involved in midgut immunity of the soft tick, Ornithodoros moubata. Insect Mol. Biol. 11, 611–618. doi: 10.1046/j.1365-2583.2002.00372.x
Nakajima, Y., Ogihara, K., Taylor, D., and Yamakawa, M. (2003). Antibacterial hemoglobin fragments from the midgut of the soft tick, Ornithodoros moubata (Acari: Argasidae). J. Med. Entomol. 40, 78–81. doi: 10.1603/0022-2585-40.1.78
Narasimhan, S., Rajeevan, N., Liu, L., Zhao, Y. O., Heisig, J., Pan, J., et al. (2014). Gut microbiota of the tick vector Ixodes scapularis modulate colonization of the Lyme disease spirochete. Cell Host Microbe. 15, 58–71. doi: 10.1016/j.chom.2013.12.001
Nava, S., Beati, L., Labruna, M. B., Caceres, A. G., Mangold, A. J., and Guglielmone, A. A. (2014). Reassessment of the taxonomic status of Amblyomma cajennense () with the description of three new species, Amblyomma tonelliae n. sp., Amblyomma interandinum n. sp. and Amblyomma patinoi n. sp., and reinstatement of Amblyomma mixtum, and Amblyomma sculptum (Ixodida: Ixodidae). Ticks Tick Borne Dis. 5, 252–276. doi: 10.1016/j.ttbdis.2013.11.004
Niebylski, M. L., Peacock, M. G., and Schwan, T. G. (1999). Lethal effect of Rickettsia rickettsii on its tick vector (Dermacentor andersoni). Appl. Environ. Microbiol. 65, 773–778.
Nielsen, H., Engelbrecht, J., Brunak, S., and von Heijne, G. (1997). Identification of prokaryotic and eukaryotic signal peptides and prediction of their cleavage sites. Protein Eng. 10, 1–6. doi: 10.1093/protein/10.1.1
Paesen, G. C., Adams, P. L., Harlos, K., Nuttall, P. A., and Stuart, D. I. (1999). Tick histamine-binding proteins: isolation, cloning, and three-dimensional structure. Mol. Cell. 3, 661–671. doi: 10.1016/S1097-2765(00)80359-7
Paine, S. H., Kemp, D. H., and Allen, J. R. (1983). In vitro feeding of Dermacentor andersoni (Stiles): effects of histamine and other mediators. Parasitology 86, 419–428. doi: 10.1017/S0031182000050617
Palmer, W. J., and Jiggins, F. M. (2015). Comparative genomics reveals the origins and diversity of Arthropod immune systems. Mol. Biol. Evol. 32, 2111–2129. doi: 10.1093/molbev/msv093
Pereira, L. S., Oliveira, P. L., Barja-Fidalgo, C., and Daffre, S. (2001). Production of reactive oxygen species by hemocytes from the cattle tick Boophilus microplus. Exp. Parasitol. 99, 66–72. doi: 10.1006/expr.2001.4657
Pinter, A., and Labruna, M. B. (2006). Isolation of Rickettsia rickettsii and Rickettsia bellii in cell culture from the tick Amblyomma aureolatum in Brazil. Ann. N. Y. Acad. Sci. 1078, 523–529. doi: 10.1196/annals.1374.103
Pinter, A., Labruna, M. B., and Faccini, J. L. (2002). The sex ratio of Amblyomma cajennense (Acari: Ixodidae) with notes on the male feeding period in the laboratory. Vet. Parasitol. 105, 79–88. doi: 10.1016/S0304-4017(01)00650-1
Rego, R. O., Hajdusek, O., Kovar, V., Kopacek, P., Grubhoffer, L., and Hypsa, V. (2005). Molecular cloning and comparative analysis of fibrinogen-related proteins from the soft tick Ornithodoros moubata and the hard tick Ixodes ricinus. Insect Biochem. Mol. Biol. 35, 991–1004. doi: 10.1016/j.ibmb.2005.04.001
Ribeiro, J. M., Alarcon-Chaidez, F., Francischetti, I. M., Mans, B. J., Mather, T. N., Valenzuela, J. G., et al. (2006). An annotated catalog of salivary gland transcripts from Ixodes scapularis ticks. Insect Biochem. Mol. Biol. 36, 111–129. doi: 10.1016/j.ibmb.2005.11.005
Ribeiro, J. M., Anderson, J. M., Manoukis, N. C., Meng, Z., and Francischetti, I. M. (2011). A further insight into the sialome of the tropical bont tick, Amblyomma variegatum. BMC Genomics 12:136. doi: 10.1186/1471-2164-12-136
Ribeiro, J. M., Topalis, P., and Louis, C. (2004). AnoXcel: an Anopheles gambiae protein database. Insect Mol. Biol. 13, 449–457. doi: 10.1111/j.0962-1075.2004.00503.x
Rojas, C., and Mysore, K. S. (2012). Glycolate oxidase is an alternative source for H2O2 production during plant defense responses and functions independently from NADPH oxidase. Plant Signal. Behav. 7, 752–755. doi: 10.4161/psb.20429
Rosa, R. D., Capelli-Peixoto, J., Mesquita, R. D., Kalil, S. P., Pohl, P. C., Braz, G. R., et al. (2016). Exploring the immune signalling pathway-related genes of the cattle tick Rhipicephalus microplus: from molecular characterization to transcriptional profile upon microbial challenge. Dev. Comp. Immunol. 59, 1–14. doi: 10.1016/j.dci.2015.12.018
Rozen, S., and Skaletsky, H. (2000). Primer3 on the WWW for general users and for biologist programmers. Methods Mol. Biol. 132, 365–386. doi: 10.1385/1-59259-192-2:365
Rudenko, N., Golovchenko, M., Edwards, M. J., and Grubhoffer, L. (2005). Differential expression of Ixodes ricinus tick genes induced by blood feeding or Borrelia burgdorferi infection. J. Med. Entomol. 42, 36–41. doi: 10.1093/jmedent/42.1.36
Sangamnatdej, S., Paesen, G. C., Slovak, M., and Nuttall, P. A. (2002). A high affinity serotonin- and histamine-binding lipocalin from tick saliva. Insect Mol. Biol. 11, 79–86. doi: 10.1046/j.0962-1075.2001.00311.x
Santucci, L. A., Gutierrez, P. L., and Silverman, D. J. (1992). Rickettsia rickettsii induces superoxide radical and superoxide dismutase in human endothelial cells. Infect. Immun. 60, 5113–5118.
Schultz, J., Copley, R. R., Doerks, T., Ponting, C. P., and Bork, P. (2000). SMART: a web-based tool for the study of genetically mobile domains. Nucleic Acids Res. 28, 231–234. doi: 10.1093/nar/28.1.231
Simser, J. A., Macaluso, K. R., Mulenga, A., and Azad, A. F. (2004). Immune-responsive lysozymes from hemocytes of the American dog tick, Dermacentor variabilis and an embryonic cell line of the Rocky Mountain wood tick, D., andersoni. Insect Biochem. Mol. Biol. 34, 1235–1246. doi: 10.1016/j.ibmb.2004.07.003
Smith, A. A., and Pal, U. (2014). Immunity-related genes in Ixodes scapularis–perspectives from genome information. Front. Cell. Infect. Microbiol. 4:116. doi: 10.3389/fcimb.2014.00116
Soares, J. F., Soares, H. S., Barbieri, A. M., and Labruna, M. B. (2012). Experimental infection of the tick Amblyomma cajennense, Cayenne tick, with Rickettsia rickettsii, the agent of Rocky Mountain spotted fever. Med. Vet. Entomol. 26, 139–151. doi: 10.1111/j.1365-2915.2011.00982.x
Sojka, D., Franta, Z., Horn, M., Hajdusek, O., Caffrey, C. R., Mares, M., et al. (2008). Profiling of proteolytic enzymes in the gut of the tick Ixodes ricinus reveals an evolutionarily conserved network of aspartic and cysteine peptidases. Parasit. Vectors. 1:7. doi: 10.1186/1756-3305-1-7
Sonenshine, D. E., Hynes, W. L., Ceraul, S. M., Mitchell, R., and Benzine, T. (2005). Host blood proteins and peptides in the midgut of the tick Dermacentor variabilis contribute to bacterial control. Exp. Appl. Acarol. 36, 207–223. doi: 10.1007/s10493-005-2564-0
Souza-Neto, J. A., Sim, S., and Dimopoulos, G. (2009). An evolutionary conserved function of the JAK-STAT pathway in anti-dengue defense. Proc. Natl. Acad. Sci. U.S.A. 106, 17841–17846. doi: 10.1073/pnas.0905006106
Tatusov, R. L., Fedorova, N. D., Jackson, J. D., Jacobs, A. R., Kiryutin, B., Koonin, E. V., et al. (2003). The COG database: an updated version includes eukaryotes. BMC Bioinformatics. 4:41. doi: 10.1186/1471-2105-4-41
Todd, S. M., Sonenshine, D. E., and Hynes, W. L. (2007). Tissue and life-stage distribution of a defensin gene in the Lone Star tick, Amblyomma americanum. Med. Vet. Entomol. 21, 141–147. doi: 10.1111/j.1365-2915.2007.00682.x
Wang, K., Zhang, T., Dong, Q., Nice, E. C., Huang, C., and Wei, Y. (2013). Redox homeostasis: the linchpin in stem cell self-renewal and differentiation. Cell Death Dis. 4:e537. doi: 10.1038/cddis.2013.50
Zhou, J., Liao, M., Ueda, M., Gong, H., Xuan, X., and Fujisaki, K. (2007). Sequence characterization and expression patterns of two defensin-like antimicrobial peptides from the tick Haemaphysalis longicornis. Peptides 28, 1304–1310. doi: 10.1016/j.peptides.2007.04.019
Keywords: spotted fever, tick, rickettsiae, Rickettsia rickettsii, Amblyomma, midgut, RNA-seq, transcriptome
Citation: Martins LA, Galletti MFBM, Ribeiro JM, Fujita A, Costa FB, Labruna MB, Daffre S and Fogaça AC (2017) The Distinct Transcriptional Response of the Midgut of Amblyomma sculptum and Amblyomma aureolatum Ticks to Rickettsia rickettsii Correlates to Their Differences in Susceptibility to Infection. Front. Cell. Infect. Microbiol. 7:129. doi: 10.3389/fcimb.2017.00129
Received: 27 January 2017; Accepted: 29 March 2017;
Published: 28 April 2017.
Edited by:
Ard Menzo Nijhof, Freie Universität Berlin, GermanyReviewed by:
Sean Phillip Riley, Louisiana State University, USACopyright © 2017 Martins, Galletti, Ribeiro, Fujita, Costa, Labruna, Daffre and Fogaça. This is an open-access article distributed under the terms of the Creative Commons Attribution License (CC BY). The use, distribution or reproduction in other forums is permitted, provided the original author(s) or licensor are credited and that the original publication in this journal is cited, in accordance with accepted academic practice. No use, distribution or reproduction is permitted which does not comply with these terms.
*Correspondence: Andréa C. Fogaça, ZGVhZm9nQHVzcC5icg==
†These authors have contributed equally to this work.
Disclaimer: All claims expressed in this article are solely those of the authors and do not necessarily represent those of their affiliated organizations, or those of the publisher, the editors and the reviewers. Any product that may be evaluated in this article or claim that may be made by its manufacturer is not guaranteed or endorsed by the publisher.
Research integrity at Frontiers
Learn more about the work of our research integrity team to safeguard the quality of each article we publish.