- 1Department of Molecular Genetics, Groningen Biomolecular Sciences and Biotechnology Institute, University of Groningen, Groningen, Netherlands
- 2Department of Bioinformatics and Biotechnology, Government College University Faisalabad, Faisalabad, Pakistan
- 3Department of Microbiology, Tumor and Cell Biology, Karolinska Institutet, Stockholm, Sweden
By using a transcriptomic approach, we have elucidated the effect of Ni2+ on the global gene expression of S. pneumoniae D39 by identifying several differentially expressed genes/operons in the presence of a high extracellular concentration of Ni2+. The genes belonging to the AdcR regulon (adcRCBA, adcAII-phtD, phtA, phtB, and phtE) and the PsaR regulon (pcpA, prtA, and psaBCA) were highly upregulated in the presence of Ni2+. We have further studied the role of Ni2+ in the regulation of the AdcR regulon by using ICP-MS analysis, electrophoretic mobility shift assays and transcriptional lacZ-reporter studies, and demonstrate that Ni2+ is directly involved in the derepression of the AdcR regulon via the Zn2+-dependent repressor AdcR, and has an opposite effect on the expression of the AdcR regulon compared to Zn2+.
Introduction
In bacteria, the transition metal ions play an important role in the proper functioning of many enzymes, transporters, and transcriptional regulators. Transition metal ions are the prerequisite for the proper bacterial growth at low concentrations, but metal ions can be lethal at higher concentrations (Blencowe and Morby, 2003; Finney and O'Halloran, 2003; Moore and Helmann, 2005; Ge et al., 2012). Therefore, proper homeostasis of metal ions is very important for the survival of bacteria, which is maintained by the dedicated metal transport- and efflux-systems (Tottey et al., 2008; Waldron and Robinson, 2009; Lisher et al., 2013). These systems are tightly regulated by metal-responsive transcriptional regulators to ensure the proper functioning of the cell by maintaining the minimum levels of metal ions inside the cell.
Streptococcus pneumoniae is one of the most common human pathogens that reside asymptomatically in the human nasopharynx (Mitchell, 2003). However, it may occasionally translocate to the lungs, the eustachian tube, the blood, and the nervous system, causing pneumoniae, otitis media, bacteremia, and meningitis, respectively (Obaro and Adegbola, 2002; Bogaert et al., 2004). During translocation from the nasopharynx to other infection sites, S. pneumoniae may encounter different environmental conditions including varying metal ions concentrations, which might affect the expression of different genes including virulence genes (Gupta et al., 2009; Shafeeq et al., 2011b, 2013; Plumptre et al., 2014a). However, the exact conditions that S. pneumoniae might face during infections, are poorly understood.
The role of manganese (Mn2+), zinc (Zn2+), copper (Cu2+), iron (Fe2+), cobalt (Co2+), and cadmium (Cd2+) on the gene regulation of S. pneumoniae have already been established and several metal-specific acquisition- and efflux-systems have been characterized. These systems include AdcRCBA (the Zn2+-uptake system), CzcD (the Zn2+-efflux system), PsaBCA (the Mn2+-uptake system), MntE (the Mn2+-efflux system), the cop operon (the Cu2+-efflux system), and PiaABCD, PiuBCDA, and PitADBC (the Fe2+- and Fe3+-uptake systems) (Kloosterman et al., 2007, 2008; Hendriksen et al., 2009; Rosch et al., 2009; Bayle et al., 2011; Shafeeq et al., 2011a, 2013; Manzoor et al., 2015c). These systems have further been shown to be regulated by metal-specific transcriptional regulators in S. pneumoniae. The Zn2+-uptake system (AdcRCBA) is repressed by transcriptional regulator AdcR in the presence of Zn2+ (Shafeeq et al., 2011a). Similarly, the psaBCA operon encoding Mn2+-uptake system are repressed by transcriptional regulator PsaR in the presence of Mn2+ (Johnston et al., 2006; Kloosterman et al., 2008), whereas, this PsaR-mediated repression is relieved by the addition of Zn2+, Co2+, Cd2+, or Ni2+ (Kloosterman et al., 2008; Jacobsen et al., 2011; Begg et al., 2015; Manzoor et al., 2015a,b,c).
Ni2+ is an essential micronutrient for certain bacteria, due to its role in various cellular processes like methane formation, hydrolysis of urea, and consumption of molecular hydrogen (Chen and Burne, 2003; Mulrooney and Hausinger, 2003; Rodionov et al., 2006; Anwar et al., 2007). In Escherichia coli, the nik operon (nikABCDE) involved in the transport of Ni2+ is shown to regulate by transcriptional regulator NikR (De Pina et al., 1999). Moreover, the expression of NmtA, an ATP-dependent transporter involved in the efflux of Ni2+ and Co2+, is tightly regulated by Ni2+-responsive transcriptional regulator NmtR in Mycobacterium tuberculosis (Cavet et al., 2002). Ni2+ is also shown to regulate the expression of urease activity in Streptococcus salivarius and Helicobacter pylori (van Vliet et al., 2001; Chen and Burne, 2003). The amount of Ni2+ in the human blood is estimated to be 0.83 ng ml−1 (Alimonti et al., 2005) and it is likely that S. pneumoniae may encounter Ni2+ during infection in blood. So far, very little is known about the impact of Ni2+ on the global gene expression of S. pneumoniae. Previously, the role of Ni2+ in the regulation of the Zn2+-efflux system czcD was reported (Kloosterman et al., 2007). It was shown that the SczA-mediated expression of czcD was highly increased in the presence of Zn2+, Co2+, or Ni2+ (Kloosterman et al., 2007). Moreover, a number of proteins and motif with Co2+- and Ni2+-binding capacity has been identified by Immobilized metal affinity column (IMAC) and LTQ-Orbitrap mass spectrometry (MS) that have diverse functions in the S. pneumoniae (Sun et al., 2013). In a recent study, we demonstrated the role of Ni2+ in regulation of the PsaR regulon and showed that Ni2+ not only alleviates the Mn2+-dependent binding of PsaR to the promoter regions of the PsaR regulon genes, but also cause Mn2+ deficiency possibly by blocking Mn2+-uptake via PsaA, hence leading to the high expression of the PsaR regulon in the presence of Ni2+ (Manzoor et al., 2015b).
In this current study, we used a transcriptomic analysis approach for the identification of differentially expressed genes/operons in response to high extracellular Ni2+ in S. pneumoniae. The expression of genes belonging to the AdcR regulon and the PsaR regulon was highly upregulated in the presence of Ni2+. We further studied the role of Ni2+ in the AdcR-mediated regulation of the adcRCBA, adcAII-phtD, phtA, phtB, and phtE by using transcriptional lacZ-reporter studies, inductively coupled plasma-mass spectrometry (ICP-MS) analysis and electrophoretic mobility shift assays (EMSAs), and showed that Ni2+ and Zn2+ play an opposite role in the regulation of the adcRCBA, adcAII-phtD, phtA, phtB, and phtE.
Materials and Methods
Bacterial Strains and Media
Bacterial strains used in this study are listed in Table 1. Growth of bacteria and DNA manipulation were performed as described (Shafeeq et al., 2011a; Manzoor et al., 2015a). All experiments in this study were performed in chemically defined medium (CDM). Salts of metal ion ZnSO4.7H2O and NiSO4.6H2O were used as specified in the Results section. Primers used in this study are based on the genome sequence of S. pneumoniae D39 and are listed in Table 2.
DNA Microarray and Data Analysis
For microarray analysis in response to Ni2+, S. pneumoniae D39 wild-type was grown in two biological replicates in CDM with and without the addition of 0.5 mM NiSO4.6H2O. To analyze the impact of adcR deletion on the transcirptome of S. pneumoniae in the presence of Ni2+, D39 wild-type and ΔadcR (SS200) (Shafeeq et al., 2011a) were grown in two biological replicates in CDM with 0.3 mM of NiSO4.6H2O. All other procedures regarding microarray experiments and data analysis were done as described before (Shafeeq et al., 2011b; Afzal et al., 2015). For the identification of differentially expressed genes a Bayesian p < 0.001 and a fold change cut-off of 2 was applied. The DNA microarray data have been submitted to gene expression omnibus (GEO) database under the accession number GSE73852.
Construction of Transcriptional lacZ-fusions and β-galactosidase Assays
Chromosomal transcriptional lacZ-fusions to the promoter regions of adcR, adcAII, phtA, phtB, and phtE were constructed in plasmid pPP2 (Halfmann et al., 2007) with the primer pairs listed in Table 2, resulting in pIM501-505. These plasmids were introduced into D39 wild-type and ΔadcR (SS200) (Shafeeq et al., 2011a) resulting in strains IM501-505 and IM551-554, respectively. All plasmids were checked for the presence of correct insert by means of PCR and DNA sequencing. For β-galactosidase activity, the derivatives of S. pneumoniae were grown in triplicate in CDM supplemented with different metal ion concentrations (w/v) mentioned in the Results and harvested at the mid-exponential growth phase. The β-galactosidase activity was measured as described before (Kloosterman et al., 2006). Standard deviations were calculated from three independent replicates of each sample.
Inductively Coupled Plasma-mass Spectrometry (ICP-MS) Analysis
To determine the cell-associated concentration of metal ions, an ICP-MS analysis was performed on the cells grown in triplicates in CDM with and without the addition of 0.5 mM Ni2+ till the mid-exponential growth phase. Cell cultures were centrifuged at 4°C and washed twice with overnight Chelex (Sigma) treated phosphate-buffered saline (PBS) with 1 mM nitrilotriacetic acid. Cells were dried overnight in a Speedvac at room temperature. The dried cells were dissolved in 2.5% nitric acid (Ultrapure, Sigma Aldrich) and lysed at 95oC for 10 min by vigorous vortexing after each 30 s. The lysed cell samples were used for ICP-MS analysis as described (Jacobsen et al., 2011). Metal ion concentrations were expressed as μg g−1 dry weight of cells.
Overexpression and Purification of Strep-tagged AdcR
The nisin-inducible (NICE) expression system (Kuipers et al., 1998) in Lactococcus lactis strain NZ9000 was used for the overexpression of C-terminally Strep-tagged AdcR (Shafeeq et al., 2011a). Cells were grown until an OD600 of 0.4 in 1 L culture followed by the induction with 10 ng ml−1 nisin. The purification of AdcR-Strep tag was performed using the Streptactin column from IBA according to the supplier's instructions (www.iba-go.com). The purified protein was eluted in buffers without EDTA and stored at a concentration of 0.5 mg/ml in the elution buffer (100 mM Tris-HCl [pH 8], 150 mM NaCl, 2.5 mM desthiobiotin, and 1 mM β-mercaptoethanol) with 10% glycerol at −80°C.
Electrophoretic Mobility Shift Assays
Electrophoretic mobility shift assays (EMSAs) were performed as described (Kloosterman et al., 2008). In short, PCR products of the promoter regions of adcR, adcAII, phtA, phtB, and pcpA were labeled with [γ-33P] ATP. All the EMSAs were performed with 5000 cpm of [γ-33P] ATP-labeled PCR products in buffer containing 20 mM Tris-HCL (pH 8.0), 5 mM MgCl2, 8.7% (w/v) glycerol, 62.5 mM KCl, 25 μg/ml bovine serum albumin and 25 μg/ml poly (dI-dC). Various metal ions were added in concentrations as described in the Results section. Reactions were incubated at 30°C for 30 min before loading on gels. Gels were run in 1 M Tris-borate buffer (pH 8.3) at 95 V for 90 min.
Results
Identification of Ni2+-dependent Genes in S. pneumoniae
To investigate the impact of Ni2+ on the transcriptome of S. pneumoniae, a DNA microarray-based comparison of D39 wild-type grown in CDM with 0.5 mM Ni2+ to the same strain grown in CDM with 0 mM Ni2+ was performed. Table 3 summarizes the list of differentially expressed genes in the presence of 0.5 mM Ni2+. The PsaR regulon consisting of the operon psaBCA (encoding Mn2+-dependent ABC transporters, PsaBCA), pcpA (encoding a choline binding protein, PcpA), and prtA (encoding a serine protease PrtA) were highly upregulated in the presence of Ni2+. The Ni2+-dependent upregulation of the PsaR regulon in the presence of Ni2+ is consistent with our recent study, where we have explored the Ni2+-dependent regulation of the PsaR regulon in more details (Manzoor et al., 2015b). Expression of a gene cluster including the cation efflux system gene czcD, the MerR family transcriptional regulator, and the Zn2+-containing alcohol dehydrogenase adhB was increased more than 35-fold in the presence of Ni2+. The cation efflux system CzcD was shown to protect S. pneumoniae against the intracellular Zn2+-stress (Kloosterman et al., 2007). A novel TetR family transcriptional regulator SczA has been shown to activate the expression of czcD in the presence of Zn2+, Co2+, or Ni2+ (Kloosterman et al., 2007). Therefore, the upregulation of czcD in our transcriptomic analysis is consistent with the finding presented in previous study (Kloosterman et al., 2007). Furthermore, genes encoding a heat shock protein (HtpX) and a Dpr homolog (spd_1402) were also differentially expressed. The Dpr protein has been shown to protect bacterial cells from oxidative stress (Pulliainen et al., 2003).
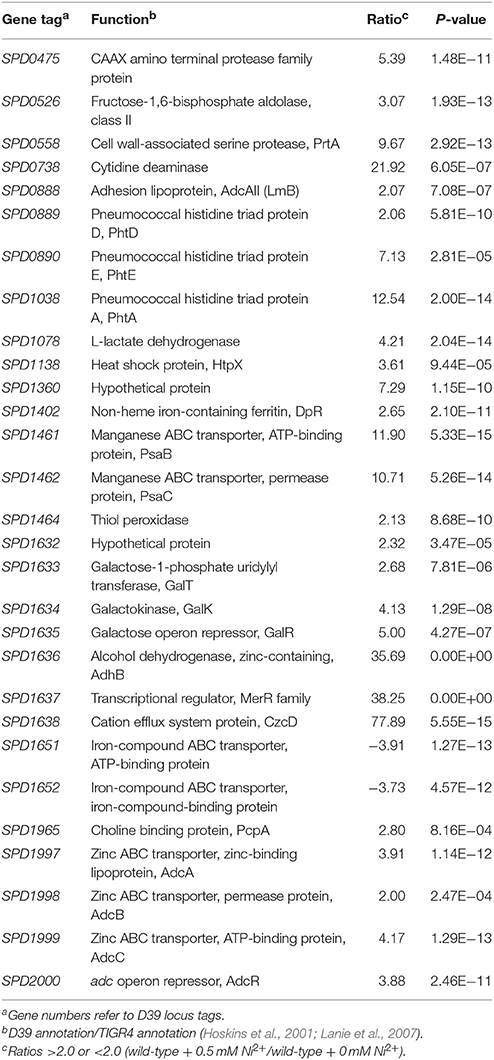
Table 3. Summary of transcriptome comparison of S. pneumoniae D39 wild-type grown in CDM plus 0.5 mM Ni2+ to CDM plus 0 mM Ni2+.
The genes belonging to the AdcR regulon were also upregulated in the presence of Ni2+. The expression of the adc operon was 4-fold upregulated. The expression of adcAII-phtD operon was upregulated 2-fold. The expression of other genes encoding for Pht family proteins (PhtA and PhtE), was upregulated more then 7-fold. Previously, it was shown that the expression of the AdcR regulon is repressed by the transcriptional regulator AdcR in the presence of Zn2+ (Shafeeq et al., 2011a). Transcriptome data was further validated by qRT-PCR analysis (Supplementary data: Table S1). Upregulation of the AdcR regulon in the presence of Ni2+ might also indicate the putative role of Ni2+ in the regulation of the AdcR regulon by the transcriptional regulator AdcR. Therefore, we decided to further explore the role of Ni2+ in the regulation of the AdcR regulon and to determine the intracellular concentrations of metal ions in S. pneumoniae D39 grown in the presence of either 0.5 mM Ni2+ or 0 mM Ni2+ in CDM.
S. pneumoniae Accumulates More Ni2+ When Grown in the Presence of 0.5 mM Ni2+
To investigate whether the observed transcriptomic responses correlated with high cell-associated concentration of Ni2+, we performed an ICP-MS analysis on the same conditions used for performing the transcriptome analysis, i.e., cells grown either in the presence of 0.5 mM Ni2+ or 0 mM Ni2+ in CDM. Our ICP-MS data revealed that the cells grown in the presence of 0.5 mM Ni2+ accumulate 30-fold more cell-associated Ni2+ compared to the cells grown in 0 mM Ni2+ (30 μg g−l dry mass of cells vs. < 1 μg g−l dry mass of cells) (Figure 1). Moreover, 2.6-fold decrease in the cell-associated concentration of Mn2+ was observed. The cell-associated concentration of other metal ions was not changed in the presence of 0.5 mM Ni2+ compared to 0 mM Ni2+. Therefore, it is likely that the transcriptomic changes observed in the presence of 0.5 M Ni2+ are due to the high intracellular concentration of Ni2+.
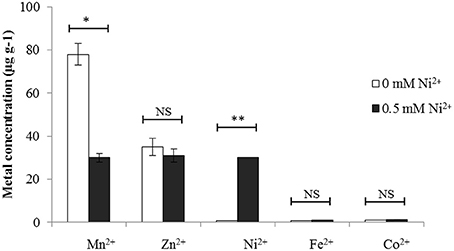
Figure 1. Cell-associated metal ion concentrations (expressed ug g−1) of S. pneumoniae D39 wild-type when grown in CDM with either 0 mM or 0.5 mM Ni2+. The statistical significance of the differences in the mean metal ion concentrations was determined by One-way ANOVA (NS not significant, *P < 0.05, and **P < 0.001).
Ni2+-dependent Expression of the AdcR Regulon
To explore the transcriptional regulation of the genes/operons belonging to the AdcR regulon (adcRCBA, adcAII-phtD, phtA, phtB, and phtE) found in our microarray analysis, transcriptional lacZ-fusions were constructed to the promoter regions of adcR, adcAII, phtA, phtB, and phtE in plasmid pPP2 (Halfmann et al., 2007) and transferred to S. pneumoniae D39 wild-type. The expression of PadcR-lacZ, PadcAII-lacZ, PphtA-lacZ, PphtB-lacZ, and PphtE-lacZ was measured in CDM and CDM-Zn2+ (Zn2+ depleted medium) with the addition of 0, 0.1, 0.3, or 0.5 mM Ni2+. As AdcR represses the expression of the AdcR regulon in the presence of Zn2+, we also used Zn2+-depleted medium (CDM-Zn2+). β-galactosidase activity (Miller Units) showed that the elevated concentration of Ni2+ led to the high expression of all these promoters in CDM and CDM-Zn2+ (Figures 2A,B). However, the expression of these promoters was much higher in CDM-Zn2+ compared to CDM. The full CDM contains minor amounts of Zn2+ (around 883 μg l−1) (Manzoor et al., 2015a), which could explain the lower expression of these promoters in CDM compared to CDM-Zn2+. This data not only suggests the role of Ni2+ in the regulation of the adcRCBA, adcAII-phtD, phtA, phtB, and phtE, but also indicate the ability of Ni2+ to derepress the Zn2+-dependent repression of these genes.
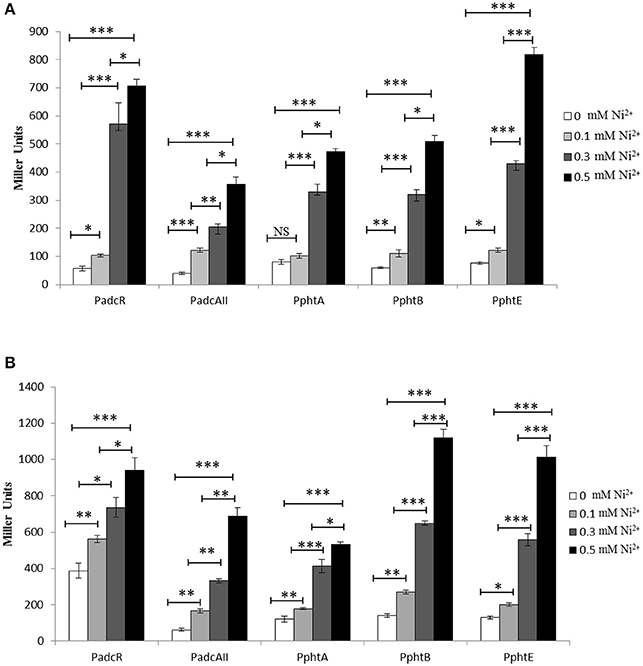
Figure 2. Expression level (in Miller units) of the D39 wild-type containing transcriptional lacZ-fusions to PadcR, PadcAII, PphtA, PphtB, and PphtE, grown in CDM (A) and CDM-Zn2+ (Zn2+-depleted medium) (B) with different added concentrations of Ni2+. Standard deviation of three independent replications is indicated with error bars. Statistical significance of the differences in the expression levels was determined by One-way ANOVA (NS, not significant, *P < 0.05, **P < 0.001, and ***P < 0.0001).
Opposite Effect of Zn2+ and Ni2+ on the Expression of the AdcR Regulon
β-galactosidase activities shown above indicate that Ni2+ might compete with Zn2+ and that both metal ions have opposite effects on the expression of the adcRCBA, adcAII-phtD, phtA, phtB, and phtE. In order to study the interplay of Ni2+ and Zn2+ in the regulation of adcRCBA, adcAII-phtD, phtA, phtB, and phtE in more details, we performed β-galactosidase assays with PadcR-lacZ, PadcAII-lacZ, PphtA-lacZ, PphtB-lacZ, and PphtE-lacZ in CDM with the addition of varying concentrations of Ni2+ and Zn2+ together. β-galactosidase data (Miller Units) showed that addition of Zn2+ in the medium leads to the repression of PadcR-lacZ, PadcAII-lacZ, PphtA-lacZ, PphtB-lacZ, and PphtE-lacZ, even in the presence of Ni2+. However, repression caused by Zn2+ was much weaker at higher concentrations of Ni2+ (Figures 3A–E). This data confirm that Ni2+ and Zn2+ have an opposite effects on the expression of adcRCBA, adcAII-phtD, phtA, phtB, and phtE, where Zn2+ represses and Ni2+ derepresses the expression of these genes.
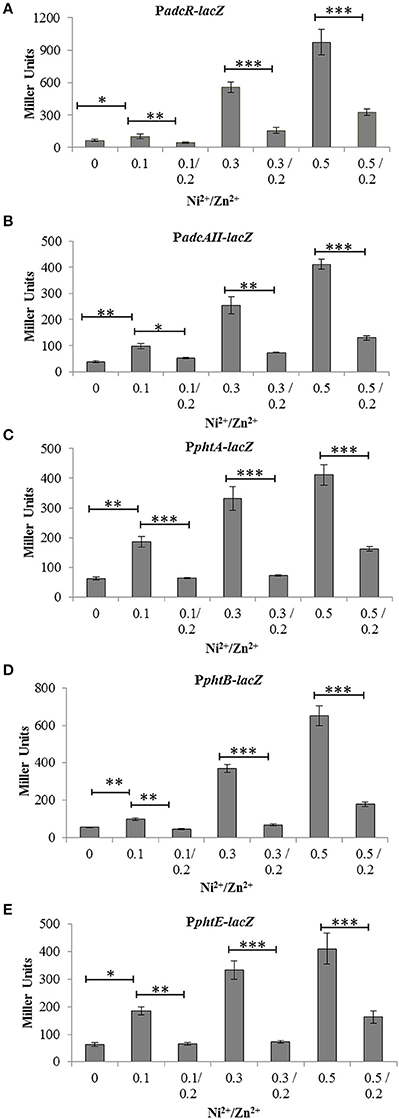
Figure 3. Expression level (in Miller units) of the D39 wild-type containing transcriptional lacZ-fusions to PadcR (A), PadcAII (B), PphtA (C), PphtB (D), and PphtE (E), grown in CDM with or without addition of different concentrations of Ni2+ and Zn2+. Standard deviation of three independent replications is indicated with error bars. Statistical significance of the differences in the expression levels was determined by One-way ANOVA (*P < 0.05, **P < 0.001, and ***P < 0.0001).
Role of the Transcriptional Regulator AdcR in the Ni2+-dependent Expression of the AdcR Regulon
Previously, it has been shown that the transcriptional regulator AdcR represses the expression of adcRCBA, adcAII-phtD, phtA, phtB, and phtE in the presence of Zn2+ (Shafeeq et al., 2011a). In this study, our transcriptomic analysis and transcriptional lacZ-reporter data indicate that Ni2+ derepresses the expression of these genes. To identify whether the transcriptional regulator AdcR is also responsible for the Ni2+-dependent expression of adcRCBA, adcAII-phtD, phtA, phtB, and phtE, we have transformed PadcR-lacZ, PadcAII-lacZ, PphtA-lacZ, PphtB-lacZ, and PphtE-lacZ into the adcR mutant (SS200) and performed β-galactosidase assays. β-galactosidase data revealed that the deletion of adcR leads to increase expression of PadcR-lacZ, PadcAII-lacZ, PphtA-lacZ, PphtB-lacZ, and PphtE-lacZ even in the absence of Ni2+ (Figure 4). Upregulation of these transcriptional lacZ-fusions in the adcR mutant indicates that Ni2+-dependent expression of adcRCBA, adcAII-phtD, phtA, phtB, and phtE is mediated by transcriptional regulator AdcR.
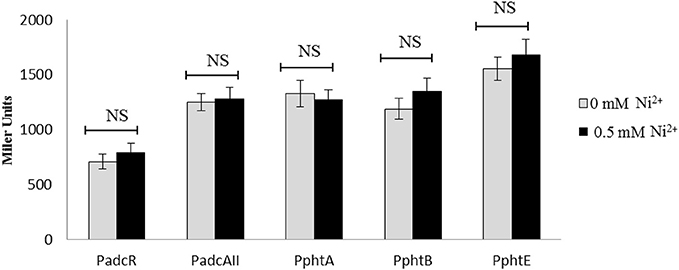
Figure 4. Expression level (in Miller units) of the adcR mutant containing transcriptional lacZ-fusions to PadcR, PadcAII, PphtA, PphtB, and PphtE grown in CDM with or without addition of 0.5 mM Ni2+. Standard deviation of three independent replications is indicated with error bars. Statistical significance of the differences in the expression levels was determined by One-way ANOVA (NS, not significant).
To elucidate the Ni2+-dependent role of AdcR in more details and find more targets of AdcR in the presence of Ni2+, microarray comparison of the adcR mutant with D39 wild-type was performed in CDM with 0.3 mM Ni2+. As expected, the expression of genes belonging to the AdcR regulon was highly upregulated (Table 4), except for the adc operon, which was downregulated in our transcriptome analysis (Table 4). For creating an adcR mutant in previous study, an erythromycin-resistance gene cassette was used to replace the adcR gene (Shafeeq et al., 2011a). Therefore, downregulation of the adc operon might be due to the polar effect of adcR deletion on the downstream genes of adcR (Shafeeq et al., 2011a). We further validated our DNA microarray data by qRT-PCR. qRT-PCR data is also in agreement with our transcriptome data (Supplementary data: Table S2).
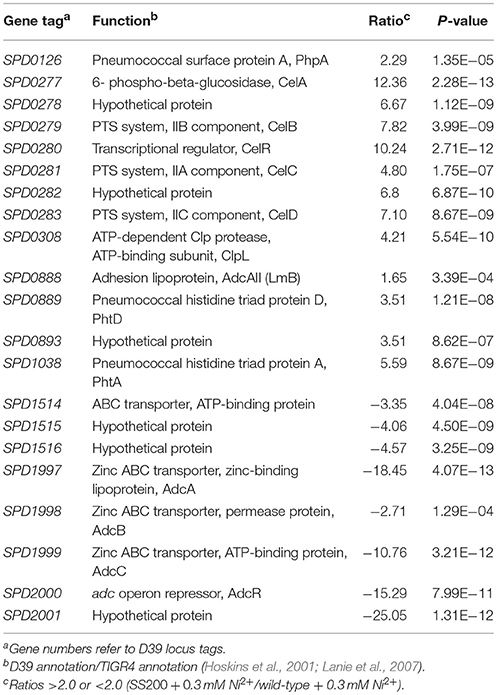
Table 4. Summary of transcriptome comparison of S. pneumoniae D39 wild-type with ΔadcR (SS200) grown in CDM with 0.3 mM Ni2+.
Binding of AdcR to Its Target is Zn2+-and Ni2+-dependent
To study the direct interaction of AdcR with the promoter regions of the genes belonging to the AdcR regulon in the presence of Ni2+, we performed EMSAs with purified Strep-tagged AdcR (Ad-Strep tag) and 33P-labeled promoters of adcR, adcAII, phtA, phtB, and pcpA. To prevent the interference of metal ions with Ad-Strep tag, all the experiments were performed in EDTA free gels and buffers. The pcpA promoter region was taken as a negative control. Ad-Strep tag was unable to shift the promoter regions of adcR, adcAII, phtA, and phtB in the absence of metal ions (Lane 2 in Figure 5). However, the addition of 0.2 mM Zn2+ led to the binding of Ad-Strep tag to the promoter regions of adcR, adcAII, phtA, and phtB (Lane 3 in Figures 5A–D), which is consistent with our previous study (Shafeeq et al., 2011a). Interestingly, 0.2 and 0.4 mM Ni2+ were unable to stimulate the binding of Ad-Strep tag with the promoter regions of adcR, adcAII, phtA, and phtB (Lane 4 and 5 in Figures 5A–D). In our transcriptome data mentioned above, Ni2+ showed a derepressive effect on the expression of the AdcR regulon. Therefore, we also decided to check the interaction of Ad-Strep tag with the promoter regions of adcR, adcAII, phtA, and phtB in the presence of both Zn2+ and Ni2+ together. The Zn2+-dependent interaction of AdcR with these promoters in the presence of 0.2 mM Zn2+ was alleviated with the addition of 0.2 mM or 0.4 mM Ni2+ (Lane 6 and 7 in Figures 5A–D). Under the same conditions, we did not see any band shift with the promoter region of pcpA as a negative control (Figure 5E). Thus, this data indicates that Zn2+ and Ni2+ have an opposite effects on the interaction of AdcR with the promoter regions of adcR, adcAII, phtA, and phtB.
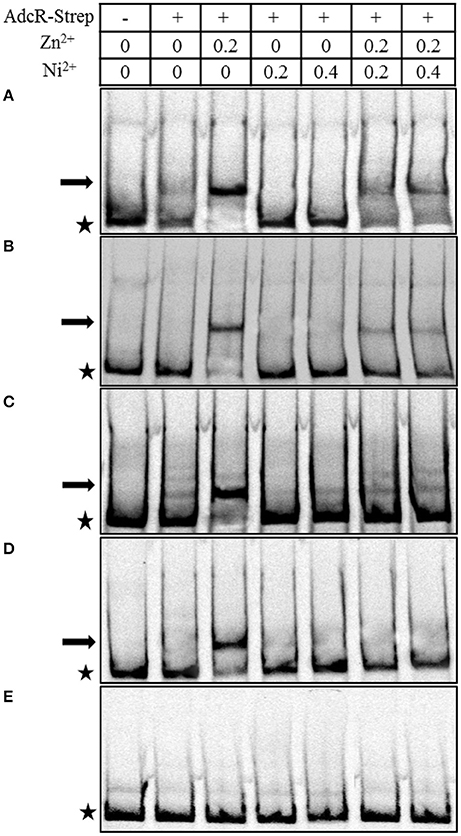
Figure 5. In vitro interaction of Ad-Strep tag with the promoter regions of adcR (A), adcAII (B), phtA (C), phtB (D), and pcpA (E). Ad-Strep was added at a concentration of 30 nM as indicated above panel, while lane 1 is without added protein. Arrows indicate the position of shifted probe and asterisks indicate the position of free probe. 0.2 mM Zn2+ was added in lanes 3, 6, and 7. Whereas, Ni2+ was added at the concentration of 0.2 mM in lane 4 and 6, and 0.4 mM in lanes 5 and 7.
Effect of Ni2+on SczA-mediated Expression of the Zn2+-efflux system czcD
To investigate the regulation of czcD in the presence of Ni2+, we studied the transcriptional response of PczcD-lacZ grown in complete CDM with the addition of different concentrations of Ni2+. β-galactosidase assays showed that PczcD-lacZ responded to Ni2+ and its expression was highly increased with an increasing concentration of Ni2+ (Figure 6). This data is in agreement with our transcriptomic data mentioned above and suggests the putative role of CzcD in Ni2+ homeostasis.
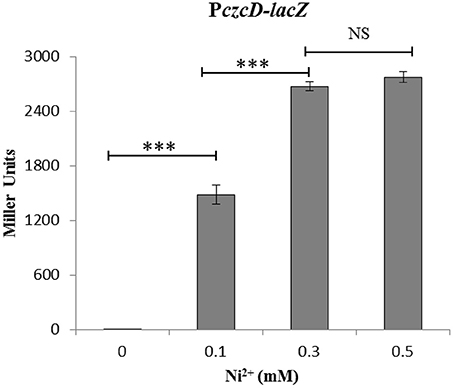
Figure 6. Expression level (miller units) of the D39 wild-type containing transcriptional lacZ-fusion to PczcD grown in CDM with different added concentrations of Ni2+. Standard deviation of three independent replications is indicated with error bars. Statistical significance of the differences in the expression levels was determined by One-way ANOVA (NS, not significant and ***P < 0.0001).
Discussion
Transition metal ions such as Mn2+, Zn2+, Cu2+, Fe2+, Co2+, and Cd2+ have been shown to play a pivotal role in the metabolism and virulence of S. pneumoniae (Brown et al., 2001; Kloosterman et al., 2008; Shafeeq et al., 2011b; Begg et al., 2015). However, the role of Ni2+ on the global gene expression of S. pneumoniae has not been studied before. In this study, we analyze the transcriptome changes in S. pneumoniae D39 wild-type in response to high Ni2+ concentration. The expression of a number of important genes and operons with diverse functions, including the AdcR regulon (adcRCBA, adcAII-phtD, phtA, phtB, and phtE), the PsaR regulon (pcpA, prtA, and psaBCA) regulon, and the Zn2+-efflux system czcD were significantly altered in the presence of Ni2+. We further studied the role of Ni2+ in the regulation of the AdcR regulon and demonstrated that Ni2+ plays an opposite role compared to Zn2+ in the regulation of the AdcR regulon.
The AdcR regulon consists of adcRCBA, adcAII-phtD, phtA, phtB, phtE, and adhC in S. pneumoniae. The adc operon (adcRCBA) is involved in Zn2+ acquisition, and encodes for a Zn2+-responsive MarR family transcriptional regulator, AdcR, two ABC transporter proteins AdcC and AdcB, and an extracellular Zn2+-binding protein AdcA (Dintilhac et al., 1997; Dintilhac and Claverys, 1997; Bayle et al., 2011). The adcAII gene encodes an adhesion lipoprotein which has an overlapping specificity with AdcA for Zn2+ (Bayle et al., 2011). AdcAII belongs to the LraI-lipoprotein family and is organized in an operon with a phtD gene encoding pneumococcal histidine triade protein precursor D (PhtD). phtA, phtB, and phtE encodes for pneumococcal histidine triade protein A, B, and E, respectively. Recent studies have demonstrated the role of the PhT family proteins (PhtA, PhtB, PhtE, and PhtD) in intracellular Zn2+ acquisition and pathogenesis in S. pneumoniae (Hava and Camilli, 2002; Ogunniyi et al., 2009; Plumptre et al., 2014b). The adhC gene encodes for a Zn2+-containing alcohol dehydrogenase. Previously, it was demonstrated that the expression of adcRCBA, adcAII-phtD, phtA, phtB, and phtE is repressed, while the expression of adhC is activated by the transcriptional regulator AdcR in the presence of Zn2+ (Shafeeq et al., 2011a). Here, we show that Ni2+ also plays a role in the regulation of adcRCBA, adcAII-phtD, phtA, phtB, and phtE. Our β-galactosidase assays showed that the expression of adcRCBA, adcAII-phtD, phtA, phtB, and phtE was increased with increasing concentrations of Ni2+. However, we did not find any significant change in the expression of adhC in our both transcriptome analysis performed in this study. This might exclude the role of Ni2+ in the AdcR mediated regulation of adhC.
High concentrations of Ni2+ can be very toxic for bacteria (Macomber and Hausinger, 2011). Therefore, bacteria must limit the toxic amount of Ni2+ to perform normal cellular functions. In many bacteria, CDF-family efflux pumps help to maintain proper concentrations of heavy metals in the cell. For example, in Bacillus subtilis, the CzcD heavy metal efflux pump is involved in the homeostasis of Zn2+, Co2+, Cu2+, and Ni2+, and is regulated by CzrA (Moore et al., 2005). It is also important to note that the expression of czcD is highly upregulated in our transcriptome analysis in response to Ni2+. Expression of czcD is regulated by the TetR family transcriptional regulator SczA in the presence of Zn2+, Co2+, or Ni2+ (Kloosterman et al., 2007). Moreover, Zn2+, Co2+, or Ni2+ has been shown to stimulate the binding of SczA to the promoter region of czcD (Kloosterman et al., 2007). In this study, we further confirmed the expression of czcD in the presence of Ni2+ by transcriptional lacZ-reporter study with PczcD-lacZ and our results are consistent with a previous study (Kloosterman et al., 2007).
The PsaR regulon consists of psaBCA, pcpA, and prtA that encodes for the Mn2+ uptake system (PsaBCA), a choline binding protein (PcpA), and a serine protease (PrtA), respectively. The expression of the PsaR regulon is shown to be repressed by the DtxR family transcriptional regulator PsaR in the presence of Mn2+ (Johnston et al., 2006). Notably, Zn2+ and Co2+ can bind with PsaR to relieve the Mn2+-dependent repression of the PsaR regulon (Kloosterman et al., 2008; Manzoor et al., 2015a). Recently, we have studied the regulation of the PsaR regulon in the presence of Ni2+ and demonstrated that like Zn2+ and Co2+, Ni2+ also has the ability to derepress the Mn2+-dependent repression of the PsaR regulon, and that high concentrations of Ni2+ leads to cell-associated Mn2+ deficiency (Manzoor et al., 2015b). In this study, we have also observed the significant upregulation of the PsaR regulon in our transcriptome analysis performed in the presence of Ni2+ (Table 3). Upregulation of the PsaR regulon in our transcriptome further verifies our previous results (Manzoor et al., 2015b). Moreover, we have also observed the cell-associated deficiency of Mn2+ in our ICP-MS analysis performed in this study (Figure 1), which is also in consistent with our previous results (Manzoor et al., 2015b).
The interplay, or competition, of metal ions plays an important role in the regulation of metal responsive genes. In S. pneumoniae, competition of Mn2+ with Zn2+, Co2+, or Ni2+ in the regulation of the PsaR regulon by transcriptional regulator PsaR has already extensively been studied (Kloosterman et al., 2008; Manzoor et al., 2015a,b). Similarly, the interplay of Cu2+ and Zn2+ in the regulation of cop operon by transcriptional regulator CopY was studied before, where Cu2+ induces and Zn2+ represses the CopY-mediated expression of cop operon (Shafeeq et al., 2011b). Here, we elaborated for the first time the interplay of Ni2+ and Zn2+ in the regulation of genes belonging to the AdcR regulon. Our lacZ-reporter studies determined the ability of Ni2+, in derepressing the Zn2+-dependent repression of adcRCBA, adcAII-phtD, phtA, phtB, and phtE. Our in vitro data showed that the Zn2+-dependent binding of AdcR to the promoter regions of the genes belonging to the AdcR regulon was alleviated by the addition of Ni2+. Recently, it has been shown that Cd2+-uptake reduces the accumulation of cell-associated Mn2+ and Zn2+ (Begg et al., 2015). Our ICP-MS comparison of cells grown in CDM with 0.5 mM to 0 mM Ni2+ has not shown any difference in the concentration of Zn2+ or other metal ions, which also indicates the direct role of Ni2+ in the regulation of adcRCBA, adcAII-phtD, phtA, phtB, and phtE. Moreover, the role of genes belonging to the AdcR regulon in the pathogenesis of S. pneumoniae has already been demonstrated, which also suggests the important role of Ni2+ in pneumococcal virulence.
Conflict of Interest Statement
The authors declare that the research was conducted in the absence of any commercial or financial relationships that could be construed as a potential conflict of interest.
Supplementary Material
The Supplementary Material for this article can be found online at: http://journal.frontiersin.org/article/10.3389/fcimb.2015.00091
References
Afzal, M., Manzoor, I., and Kuipers, O. P. (2015). A fast and reliable pipeline for bacterial transcriptome analysis case study: serine-dependent gene regulation in Streptococcus pneumoniae. J. Vis. Exp. e52649. doi: 10.3791/52649
Alimonti, A., Bocca, B., Mannella, E., Petrucci, F., Zennaro, F., Cotichini, R., et al. (2005). Assessment of reference values for selected elements in a healthy urban population. Ann. Ist. Super. Sanità 41, 181–187.
Anwar, H. A., Aldam, C. H., Visuvanathan, S., and Hart, A. J. (2007). The effect of metal ions in solution on bacterial growth compared with wear particles from hip replacements. J. Bone Joint Surg. Br. 89-B, 1655–1659. doi: 10.1302/0301-620X.89B12.19714
Bayle, L., Chimalapati, S., Schoehn, G., Brown, J., Vernet, T., and Durmort, C. (2011). Zinc uptake by Streptococcus pneumoniae depends on both AdcA and AdcAII and is essential for normal bacterial morphology and virulence. Mol. Microbiol. 82, 904–916. doi: 10.1111/j.1365-2958.2011.07862.x
Begg, S. L., Eijkelkamp, B. A., Luo, Z., Couñago, R. M., Morey, J. R., Maher, M. J., et al. (2015). Dysregulation of transition metal ion homeostasis is the molecular basis for cadmium toxicity in Streptococcus pneumoniae. Nat. Commun. 6:6418. doi: 10.1038/ncomms7418
Blencowe, D. K., and Morby, A. P. (2003). Zn(II) metabolism in prokaryotes. FEMS Microbiol. Rev. 27, 291–311. doi: 10.1016/S0168-6445(03)00041-X
Bogaert, D., De Groot, R., and Hermans, P. W. M. (2004). Streptococcus pneumoniae colonisation: the key to pneumococcal disease. Lancet Infect. Dis. 4, 144–154. doi: 10.1016/S1473-3099(04)00938-7
Brown, J. S., Gilliland, S. M., and Holden, D. W. (2001). A Streptococcus pneumoniae pathogenicity island encoding an ABC transporter involved in iron uptake and virulence. Mol. Microbiol. 40, 572–585. doi: 10.1046/j.1365-2958.2001.02414.x
Cavet, J. S., Meng, W., Pennella, M. A., Appelhoff, R. J., Giedroc, D. P., and Robinson, N. J. (2002). A nickel-cobalt-sensing ArsR-SmtB family repressor. Contributions of cytosol and effector binding sites to metal selectivity. J. Biol. Chem. 277, 38441–38448. doi: 10.1074/jbc.M207677200
Chen, Y.-Y. M., and Burne, R. A. (2003). Identification and characterization of the nickel uptake system for urease biogenesis in Streptococcus salivarius 57.I. J. Bacteriol. 185, 6773–6779. doi: 10.1128/JB.185.23.6773-6779.2003
De Pina, K., Desjardin, V., Mandrand-Berthelot, M. A., Giordano, G., and Wu, L. F. (1999). Isolation and characterization of the nikR gene encoding a nickel-responsive regulator in Escherichia coli. J. Bacteriol. 181, 670–674.
Dintilhac, A., Alloing, G., Granadel, C., and Claverys, J. P. (1997). Competence and virulence of Streptococcus pneumoniae: Adc and PsaA mutants exhibit a requirement for Zn and Mn resulting from inactivation of putative ABC metal permeases. Mol. Microbiol. 25, 727–739. doi: 10.1046/j.1365-2958.1997.5111879.x
Dintilhac, A., and Claverys, J. P. (1997). The adc locus, which affects competence for genetic transformation in Streptococcus pneumoniae, encodes an ABC transporter with a putative lipoprotein homologous to a family of streptococcal adhesins. Res. Microbiol. 148, 119–131. doi: 10.1016/S0923-2508(97)87643-7
Finney, L. A., and O'Halloran, T. V. (2003). Transition metal speciation in the cell: insights from the chemistry of metal ion receptors. Science 300, 931–936. doi: 10.1126/science.1085049
Ge, R., Chen, Z., and Zhou, Q. (2012). The actions of bismuth in the treatment of Helicobacter pylori infections: an update. Met. Integr. Biometal Sci. 4, 239–243. doi: 10.1039/c2mt00180b
Gupta, R., Shah, P., and Swiatlo, E. (2009). Differential gene expression in Streptococcus pneumoniae in response to various iron sources. Microb. Pathog. 47, 101–109. doi: 10.1016/j.micpath.2009.05.003
Halfmann, A., Hakenbeck, R., and Brückner, R. (2007). A new integrative reporter plasmid for Streptococcus pneumoniae. FEMS Microbiol. Lett. 268, 217–224. doi: 10.1111/j.1574-6968.2006.00584.x
Hava, D. L., and Camilli, A. (2002). Large-scale identification of serotype 4 Streptococcus pneumoniae virulence factors. Mol. Microbiol. 45, 1389–1406. doi: 10.1046/j.1365-2958.2002.03106.x
Hendriksen, W. T., Bootsma, H. J., van Diepen, A., Estevão, S., Kuipers, O. P., de Groot, R., et al. (2009). Strain-specific impact of PsaR of Streptococcus pneumoniae on global gene expression and virulence. Microbiol. Read. Engl. 155, 1569–1579. doi: 10.1099/mic.0.025072-0
Hoskins, J., Alborn, W. E. Jr., Arnold, J., Blaszczak, L. C., Burgett, S., DeHoff, B. S., et al. (2001). Genome of the bacterium Streptococcus pneumoniae strain R6. J. Bacteriol. 183, 5709–5717. doi: 10.1128/JB.183.19.5709-5717.2001
Jacobsen, F. E., Kazmierczak, K. M., Lisher, J. P., Winkler, M. E., and Giedroc, D. P. (2011). Interplay between manganese and zinc homeostasis in the human pathogen Streptococcus pneumoniae. Met. Integr. Biometal Sci. 3, 38–41. doi: 10.1039/C0MT00050G
Johnston, J. W., Briles, D. E., Myers, L. E., and Hollingshead, S. K. (2006). Mn2+-dependent regulation of multiple genes in Streptococcus pneumoniae through PsaR and the resultant impact on virulence. Infect. Immun. 74, 1171–1180. doi: 10.1128/IAI.74.2.1171-1180.2006
Kloosterman, T. G., Hendriksen, W. T., Bijlsma, J. J. E., Bootsma, H. J., van Hijum, S. A. F. T., Kok, J., et al. (2006). Regulation of glutamine and glutamate metabolism by GlnR and GlnA in Streptococcus pneumoniae. J. Biol. Chem. 281, 25097–25109. doi: 10.1074/jbc.M601661200
Kloosterman, T. G., van der Kooi-Pol, M. M., Bijlsma, J. J. E., and Kuipers, O. P. (2007). The novel transcriptional regulator SczA mediates protection against Zn2+ stress by activation of the Zn2+-resistance gene czcD in Streptococcus pneumoniae. Mol. Microbiol. 65, 1049–1063. doi: 10.1111/j.1365-2958.2007.05849.x
Kloosterman, T. G., Witwicki, R. M., van der Kooi-Pol, M. M., Bijlsma, J. J. E., and Kuipers, O. P. (2008). Opposite effects of Mn2+ and Zn2+ on PsaR-mediated expression of the virulence genes pcpA, prtA, and psaBCA of Streptococcus pneumoniae. J. Bacteriol. 190, 5382–5393. doi: 10.1128/JB.00307-08
Kuipers, O. P., de Ruyter, P. G. G., Kleerebezem, M., and de Vos, W. M. (1998). Quorum sensing-controlled gene expression in lactic acid bacteria. J. Biotechnol. 64, 15–21. doi: 10.1016/S0168-1656(98)00100-X
Lanie, J. A., Ng, W.-L., Kazmierczak, K. M., Andrzejewski, T. M., Davidsen, T. M., Wayne, K. J., et al. (2007). Genome sequence of Avery's virulent serotype 2 strain D39 of Streptococcus pneumoniae and comparison with that of unencapsulated laboratory strain R6. J. Bacteriol. 189, 38–51. doi: 10.1128/JB.01148-06
Lisher, J. P., Higgins, K. A., Maroney, M. J., and Giedroc, D. P. (2013). Physical Characterization of the manganese-sensing pneumococcal surface antigen repressor from Streptococcus pneumoniae. Biochemistry (Mosc.) 52, 7689–7701. doi: 10.1021/bi401132w
Macomber, L., and Hausinger, R. P. (2011). Mechanisms of nickel toxicity in microorganisms. Met. Integr. Biometal Sci. 3, 1153–1162. doi: 10.1039/c1mt00063b
Manzoor, I., Shafeeq, S., Kloosterman, T. G., and Kuipers, O. P. (2015a). Co2+-dependent gene expression in Streptococcus pneumoniae: opposite effect of Mn2+ and Co2+ on the expression of the virulence genes psaBCA, pcpA and prtA. Microb. Physiol. Metab. 6, 748. doi: 10.3389/fmicb.2015.00748
Manzoor, I., Shafeeq, S., and Kuipers, O. P. (2015b). Ni2+-dependent and PsaR-mediated regulation of the virulence genes pcpA, psaBCA and prtA in Streptococcus pneumoniae. PLoS ONE 10:e0142839. doi: 10.1371/journal.pone.0142839
Manzoor, I., Shafeeq, S., and Kuipers, O. P. (2015c). Transcriptome analysis of Streptococcus pneumoniae D39 in the presence of cobalt. Genomics Data 6, 151–153. doi: 10.1016/j.gdata.2015.08.033
Mitchell, T. J. (2003). The pathogenesis of streptococcal infections: from tooth decay to meningitis. Nat. Rev. Microbiol. 1, 219–230. doi: 10.1038/nrmicro771
Moore, C. M., Gaballa, A., Hui, M., Ye, R. W., and Helmann, J. D. (2005). Genetic and physiological responses of Bacillus subtilis to metal ion stress. Mol. Microbiol. 57, 27–40. doi: 10.1111/j.1365-2958.2005.04642.x
Moore, C. M., and Helmann, J. D. (2005). Metal ion homeostasis in Bacillus subtilis. Curr. Opin. Microbiol. 8, 188–195. doi: 10.1016/j.mib.2005.02.007
Mulrooney, S. B., and Hausinger, R. P. (2003). Nickel uptake and utilization by microorganisms. FEMS Microbiol. Rev. 27, 239–261. doi: 10.1016/S0168-6445(03)00042-1
Obaro, S., and Adegbola, R. (2002). The pneumococcus: carriage, disease and conjugate vaccines. J. Med. Microbiol. 51, 98–104. doi: 10.1099/0022-1317-51-2-98
Ogunniyi, A. D., Grabowicz, M., Mahdi, L. K., Cook, J., Gordon, D. L., Sadlon, T. A., et al. (2009). Pneumococcal histidine triad proteins are regulated by the Zn2+-dependent repressor AdcR and inhibit complement deposition through the recruitment of complement factor H. FASEB J. 23, 731–738. doi: 10.1096/fj.08-119537
Plumptre, C. D., Eijkelkamp, B. A., Morey, J. R., Behr, F., Couñago, R. M., Ogunniyi, A. D., et al. (2014a). AdcA and AdcAII employ distinct zinc acquisition mechanisms and contribute additively to zinc homeostasis in Streptococcus pneumoniae. Mol. Microbiol. 91, 834–851. doi: 10.1111/mmi.12504
Plumptre, C. D., Hughes, C. E., Harvey, R. M., Eijkelkamp, B. A., McDevitt, C. A., and Paton, J. C. (2014b). Overlapping Functionality of the Pht Proteins in Zinc Homeostasis of Streptococcus pneumoniae. Infect. Immun. 82, 4315–4324. doi: 10.1128/IAI.02155-14
Pulliainen, A. T., Haataja, S., Kähkönen, S., and Finne, J. (2003). Molecular basis of H2O2 resistance mediated by Streptococcal Dpr. Demonstration of the functional involvement of the putative ferroxidase center by site-directed mutagenesis in Streptococcus suis. J. Biol. Chem. 278, 7996–8005. doi: 10.1074/jbc.M210174200
Rodionov, D. A., Hebbeln, P., Gelfand, M. S., and Eitinger, T. (2006). Comparative and functional genomic analysis of prokaryotic nickel and cobalt uptake transporters: evidence for a novel group of ATP-binding cassette transporters. J. Bacteriol. 188, 317–327. doi: 10.1128/JB.188.1.317-327.2006
Rosch, J. W., Gao, G., Ridout, G., Wang, Y.-D., and Tuomanen, E. I. (2009). Role of the manganese efflux system mntE for signalling and pathogenesis in Streptococcus pneumoniae. Mol. Microbiol. 72, 12–25. doi: 10.1111/j.1365-2958.2009.06638.x
Shafeeq, S., Kloosterman, T. G., and Kuipers, O. P. (2011a). Transcriptional response of Streptococcus pneumoniae to Zn2+) limitation and the repressor/activator function of AdcR. Met. Integr. Biometal Sci. 3, 609–618. doi: 10.1039/c1mt00030f
Shafeeq, S., Kuipers, O. P., and Kloosterman, T. G. (2013). The role of zinc in the interplay between pathogenic streptococci and their hosts. Mol. Microbiol. 88, 1047–1057. doi: 10.1111/mmi.12256
Shafeeq, S., Yesilkaya, H., Kloosterman, T. G., Narayanan, G., Wandel, M., Andrew, P. W., et al. (2011b). The cop operon is required for copper homeostasis and contributes to virulence in Streptococcus pneumoniae. Mol. Microbiol. 81, 1255–1270. doi: 10.1111/j.1365-2958.2011.07758.x
Sun, X., Yu, G., Xu, Q., Li, N., Xiao, C., Yin, X., et al. (2013). Putative cobalt- and nickel-binding proteins and motifs in Streptococcus pneumoniae. Met. Integr. Biometal Sci. 5, 928–935. doi: 10.1039/c3mt00126a
Tottey, S., Waldron, K. J., Firbank, S. J., Reale, B., Bessant, C., Sato, K., et al. (2008). Protein-folding location can regulate manganese-binding versus copper- or zinc-binding. Nature 455, 1138–1142. doi: 10.1038/nature07340
van Vliet, A. H. M., Kuipers, E. J., Waidner, B., Davies, B. J., de Vries, N., Penn, C. W., et al. (2001). Nickel-responsive induction of urease expression inhelicobacter pylori is mediated at the transcriptional level. Infect. Immun. 69, 4891–4897. doi: 10.1128/IAI.69.8.4891-4897.2001
Keywords: metal homeostasis, pneumococcus, nickel, zinc, AdcR, Pht family proteins, AdcR regulon, PsaR regulon
Citation: Manzoor I, Shafeeq S, Afzal M and Kuipers OP (2015) The Regulation of the AdcR Regulon in Streptococcus pneumoniae Depends Both on Zn2+- and Ni2+-Availability. Front. Cell. Infect. Microbiol. 5:91. doi: 10.3389/fcimb.2015.00091
Received: 27 August 2015; Accepted: 17 November 2015;
Published: 08 December 2015.
Edited by:
Jorge Eugenio Vidal, Emory University, USAReviewed by:
Hilde De Reuse, Institut Pasteur, FranceStephen Peter Kidd, University of Adelaide, Australia
Copyright © 2015 Manzoor, Shafeeq, Afzal and Kuipers. This is an open-access article distributed under the terms of the Creative Commons Attribution License (CC BY). The use, distribution or reproduction in other forums is permitted, provided the original author(s) or licensor are credited and that the original publication in this journal is cited, in accordance with accepted academic practice. No use, distribution or reproduction is permitted which does not comply with these terms.
*Correspondence: Oscar P. Kuipers, o.p.kuipers@rug.nl
†These authors have contributed equally to this work.