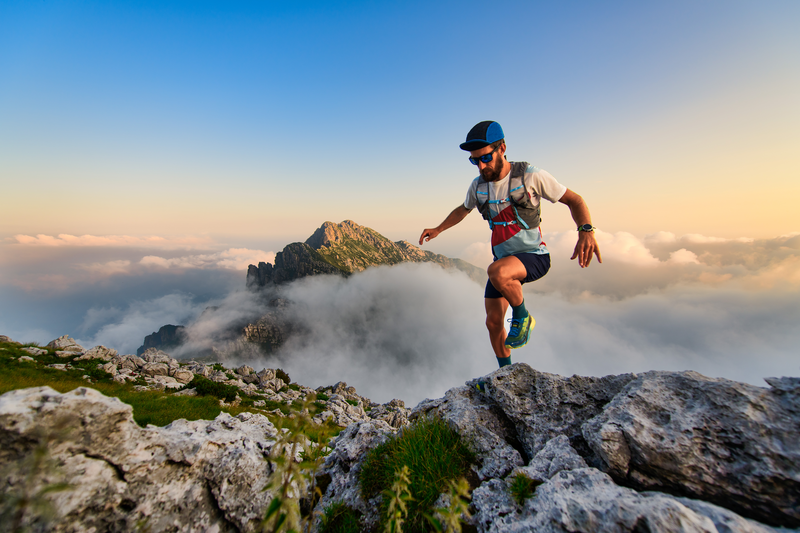
94% of researchers rate our articles as excellent or good
Learn more about the work of our research integrity team to safeguard the quality of each article we publish.
Find out more
ORIGINAL RESEARCH article
Front. Chem. , 12 February 2025
Sec. Analytical Chemistry
Volume 13 - 2025 | https://doi.org/10.3389/fchem.2025.1554453
Background: Siloxanes have been widely used in various products and have been detected to varying degrees in the environment. It has been reported that methylsiloxane has toxic effects on the nervous, immune and reproductive systems of aquatic animals, and is carcinogenic and mutagenic. Therefore, in order to protect human health, it is urgent to establish a method for the determination of siloxane content in drinking water and source water.
Methods: Herein, this paper proposes a precise, fast, and selective method using solid-phase microextraction combined with gas chromatography-mass spectrometry (GC-MS/MS) method for the simultaneous detection of 11 kinds of siloxanes in drinking water and source water. Quantification of siloxanes was carried out by internal standard method. The parameters that affect the extraction and desorption processes were optmised. The extraction efficiency of four commercially available SPME fibers was evaluated. The results showed that divinylbenzene/polydimethyl-siloxane or divinylbenzene/Carboxen/polydimethylsiloxane was the best coating for the extraction of siloxane.
Results: This method provided good linearity (r > 0.9946) and precision (RSD% <8.0%) with the minimum detection mass concentration ranging from 0.008 to 0.025 μg/L under the optimized extraction and GC-MS/MS analysis conditions. The developed method has been applied to the simultaneous analysis of 11 kinds of siloxanes in drinking water and source water, and the results showed that decamethylcyclopentasiloxane (D5) and dodecamethylcyclohexasiloxane (D6) were found in two source water samples at concentrations ranging from 0.008 to 0.012 μg/L and 0.015–0.019 μg/L, respectively.
Conclusion: This developed method was simple, quick, and effective and our satisfactory results demonstrated its suitability for the simultaneous determination of 11 kinds of siloxanes in drinking water and source water.
Siloxanes are a type of synthetic, high molecular weight organosilicon polymer bearing silico-oxygen bonds (-Si-O-Si-) in their backbone with organic groups connected to the silicon atoms. Due to the volatility of its oligomers, the silicon and oxygen atoms appear alternately in a synthetic ring structure, named cyclic volatile methylsiloxane (cVMS) by the International Chemical Organization, which may break in the environment to form linear methylsiloxane (Capela et al., 2017).
Recently, siloxanes have been widely used in various products encompassing industrial, household, and personal care products, cleaning agents, and medical devices (Homem et al., 2017; Lassen et al., 2005; Graiver et al., 2003; Horii and Kannan, 2008; Genualdi et al., 2011; Lu et al., 2011). In 2023, China was expected to place into operation new capacity plans to achieve 582,000 tons/year of polysiloxane with an annual production capacity of 2.893 million tons/year, an increase of 25.2%, which will be the world’s largest supply and demand market (Zhong, 2023).
During their use, ∼100% of the volatile siloxanes enter the surrounding environment, of which 90% is distributed in the atmosphere, indoor dust, soil, and other media through volatilization and settlement. The other 10% is discharged with domestic sewage and enters environmental water bodies through surface run-off and other pathways. Consequently, siloxanes have been detected to varying degrees in the environment (Kierkegaard and McLachlan, 2010; Wang et al., 2001; Hodgson et al., 2003; Kierkegaard et al., 2013; Genualdi et al., 2011; Raich-Montiu et al., 2014; Xu et al., 2013; Guo et al., 2019; Zhang et al., 2024). In 2005, Kaj et al. (2005) reported the content of cVMS in various water environments in Northern Europe (including natural surface water bodies, seawater, stormwater run-off, water inlet, and outlets from municipal sewage treatment plants, landfill leachate, etc.). Although there are some defects in the detection method and technology, these results indicate the future direction for the detection of cVMS in the environment. Wang et al. (2013b) investigated the content of cVMS in wastewater (wastewater inlets and outlets, and receiving surface water) of a wastewater treatment plant in Canada, and the concentrations of octamethylcyclotetrasiloxane (D4), decamethylcyclopentasiloxane (D5), and dodecamethylcyclohexasiloxane (D6) in wastewater effluent were 6.69, 135 and 26.9 μg/L, respectively. The highest detected amounts of D4, D5, and D6 in surface water ranging from 0.005 to 3.1 km away from the sewage outlet were 0.023, 1.48, and 0.151 μg/L, respectively. It has been reported that methylsiloxane has toxic effects on the nervous, immune and reproductive systems of aquatic animals, and is carcinogenic and mutagenic. However, there are few research studies on siloxanes in drinking water and there is no limiting standard for siloxanes. Meanwhile, several methods, including headspace, liquid-liquid microextraction, and purge/capture techniques combined with gas chromatography-mass spectrometry analysis have been developed for the determination of siloxanes in solid and natural water samples (Companioni-Damas et al., 2012; Wang et al., 2023; Luciana et al., 2018; Sparham et al., 2008; Cortada et al., 2014; Sparham et al., 2011; Zhang et al., 2011; Wang et al., 2013a). However, Previous studies normally require a sample preparation step because organic compounds are contained at trace levels or in a matrix that interferes. Solid phase microextraction (SPME)has attracted much attention because it has the advantages of integrating sampling, extraction, concentration and sampling, and the need for solvent-free micro-extraction of samples. Headspace-solid phase microextraction combined with gas chromatography-mass spectrometry technology has the advantages of rapidity, sensitivity, accuracy and high efficiency. Organic compounds with different concentrations and chemical structures can be analyzed. The target compound loss rate in the sample is low, which is suitable for the analysis of multi-component mixture samples, and has been used as the first choice for the determination of siloxane in recent years. Xu et al. (2013) reported that determination of 6 volatile siloxanes in Water by headspace solid phase microextraction/Gas chromatogram-mass spectrometry. The detection limit of this method is about 5 ng/L, and its sensitivity and precision can meet the requirements of water quality detection. The DB-5MS chromatographic column has been used for the separation and detection. However, there are some problems in the detection method and technology of siloxanes in drinking water and source water. The DB-5MS column bleeding compound were the target compound for detection. When the column bleeding is large, it cannot accurately reflect the true content of cyclic siloxanes (D4, D5, D6, etc.) in the sample. In addition, the high volatility of siloxanes and the potential sources of background contamination affecting their final determination are the main limiting factors for their analysis (Luciana et al., 2018). Meanwhile, these methods are limited to the detection of only a few siloxanes, which fails to meet the requirements for comprehensive detection. Therefore, it is imperative to further investigate and refine the SPME experimental conditions to ensure the detection of a broader range of siloxane compounds, particularly when analyzing trace or ultra-trace levels of siloxanes in water samples.
In this study, we have established a precise, fast, and selective method using solid-phase microextraction combined with gas chromatography-mass spectrometry (GC-MS/MS) method for the simultaneous detection of 11 kinds of siloxanes in drinking water and source water. Quantification of siloxanes was carried out by internal standard method. This method was successfully applied to identification and quantification of 11 kinds of siloxanes in drinking water and source water.
Hexamethylcyclotrisiloxane (D3), octamethyltrisiloxane (L3), octamethylcyclotetrasiloxane (D4), decamethyltetrasiloxane (L4), decamethylcyclopentasiloxane (D5), dodecamethylpentasiloxane (L5), dodecamethylcyclohexasiloxane (D6), tetradecamethylhexasiloxane (L6), tetradecamethylcycloheptasiloxane (D7), hexadecamethylcyclooctasiloxane (D8), and octadecamethylcyclononasiloxane (D9) with ≥98% purity were supplied by CATO Research Chemicals Inc. (Guangzhou. China). The internal standard, 2,4,6-triethylene-2,4,6-trimethylcyclotrisiloxane (D3V), with ≥97% purity was supplied by Macklin Biochemical Co., Ltd. (Shanghai. China).
Acetone and sodium chloride were purchased from Beijing Chemical Works. Ultrapure water (resistivity of 18.2 MΩ cm at 25°C) was obtained using a Milli-Q Gradient water system (Millipore, Bedford, MA, United States).
Stock standard solutions with concentrations of 1,000 μg/mL of the 11 kinds of siloxanes and internal standard were prepared in acetone, respectively and stored at 4°C in brown glass bottles.
Samples were analyzed on a GCMS-QP2010 Ultra gas chromatography-mass spectrometer (Shimadzu, Japan). Chromatographic separation was conducted on a VF-WAX elastic quartz capillary column (30 m × 0.25 mm I.D. and 0.25 μm film thickness).
An solid-phase microextraction (SPME) device including SPME sampling table, SPME handle, sample catheter, and SPME fiber (using divinylbenzene/polydimethylsiloxane (DVB/PDMS) fiber or divinylbenzene/carboxen/polydimethylsiloxane (DVB/PDMS/CAR) fiber or equivalent) was used. The liner for solid phase microextraction had dimensions of 78.5 mm × 6.3 mm, 0.75 mm.
Brown glass bottles with Teflon pads were used for sample collection. During sampling, water was collected into a full bottle, sealed, and stored at 4°C. The determination needed to be completed within 24 h because the components to be detected in the sample were volatile. A 0.22 μm water filter membrane was used to filter the water sample if the sample was turbid.
A magnetic stirrer was placed into a 60-mL sampling bottle and 4 g of sodium chloride added. 40 mL of water sample were added, followed by 80 μL of the internal standard solution (100 μg/L), and the bottle cap tightened. The bottle was placed on the sampling table and stirred at 800 rpm. After 15 s of stirring, the extracted fiber was pressed to the top space for adsorption extraction. After 45 min of extraction, the extracted fiber was removed, the needle dried and the water was absorbed, and the extracted fiber inserted into the gas chromatographic inlet and desorbed at 220°C for 2 min.
All samples were analyzed using GC-MS/MS. For GC detection, the separation was conducted on a VF-WAX elastic quartz capillary column. The oven temperature was programmed from 35°C (held for 2 min) to 240°C at 10°C/min (held for 5 min). Helium (99.999%) was used as a carrier gas at a constant flow rate of 1.0 mL/min. The injector temperature was maintained at 220°C and a 50:1 split injection mode with the split closed for 2 min used for our experiments.
Electron ionization (EI) mode operated at 70 eV was applied for MS/MS detection. The mass spectrometer source and transfer line were set at 230°C and 280°C, respectively. Selected ion monitoring (SIM) mode was used for data acquisition. Table 1 shows the ions selected for the quantification and confirmation of 11 kinds of siloxanes using the GC-MS method. The representative total ion flow diagram of the 11 siloxanes and internal standard solutions is shown in Figure 1.
According to the physical and chemical properties of siloxanes, three pretreatment methods for solid phase extraction, solid phase microextraction, and purge and capture were selected for comparison. The absolute recoveries of D3, D4, and L3 were <30% in the solid phase extraction method and the small ring siloxanes were highly volatile, so it was not suitable for this method. The principles of purge capture and SPME are similar. The experiment was conducted using SPME first considering the operability of the method and the availability of consumables, the molecular weight of siloxanes D3 to D9 were very different, and stirring SPME was more beneficial to the volatilization of the tested substances.
Forty milliliters the mixed standard solution (100 ng/L) were added to a 60-mL headspace bottle. Under the extraction conditions of room temperature, pH 7.0, extraction time of 60 min, and desorption time at gas chromatographic inlet (220°C) of 2 min, the extraction efficiency of four kinds of fibers (polyacrylate (PA), Polyethylene glycol/divinylbenzene (CW/DVB), DVB/PDMS, and DVB/PDMS/CAR) on the 11 kinds of siloxanes was investigated. Information regarding the four kinds of fibers is shown in Table 2.
Our results showed that the extraction efficiency of the DVB/PDMS and DVB/PDMS/CAR fibers for the 11 kinds of siloxanes was significantly higher than that of the PA and CW/DVB fibers, which may be attributed to the main component of DVB/PDMS and DVB/PDMS/CAR being weakly polar methicone polymer (PDMS). Consequently, the DVB/PDMS and DVB/PDMS/CAR fibers exhibited a higher extraction efficiency than the polar PA and CW/DVB fibers. DVB/PDMS and DVB/PDMS/CAR had similar extraction efficiencies for D4, L4, D5, L5, D6, L6, D7, D8. and D9. For D3 and L3, the extraction efficiency of DVB/PDMS/CAR was slightly higher than that of DVB/PDMS. However, the reproducibility (RSD of 2.6%–7.8%) of DVB/PDMS extraction was better than that observed for the 11 kinds of siloxanes using DVB/PDMS/CAR (RSD of 5.6%–17.4%), as shown in Figure 2.
After a complete SPME/GC-MS process of the 500 ng/L target solution, the two fibers were directly inserted into the chromatographic inlet for secondary desorption. Our results showed that the peak area of the 11 kinds of siloxanes during the second desorption of DVB/PDMS was 0.5% lower than that of the first desorption, while the peak area of the target siloxanes during the second desorption of DVB/PDMS/CAR was ∼1% of that of the first desorption. Although the absolute recovery of DVB/PDMS fiber was slightly better than that of DVB/PDMS/CAR fiber in the extraction of the target materials, there was no significant difference when applying the internal standard method to determination. Based on the reproducibility and residue of the two fibers, the DVB/PDMS and DVB/PDMS/CAR fibers were used in this experiment.
Under the following experimental conditions, DVB/PDMS fiber, 40 mL of the standard solution (100 ng/L) was mixed with the 11 kinds of siloxanes added to a 60-mL headspace bottle, pH 7.0, extraction time of 60 min, and desorption for 2 min at 220°C at the gas chromatography inlet. The effect of the extraction temperature on the extraction efficiency was investigated. Figure 3 shows that the extraction efficiency decreased with an increase in the extraction temperature, and the extraction efficiency of L3, L4, D3, and D4 reached its maximum value at 24°C (room temperature). Our results indicated that the extraction efficiency of L3, L4, D3, and D4 could be satisfactory at room temperature due to their high volatility, but the extraction efficiency was not significantly improved upon heating. On the contrary, high temperature reduced the partition coefficient between the fiber coating and top space, resulting in a reduction in the extraction efficiency (peak area). D5, L5, D6, and L6 exhibited the highest extraction efficiency at 35°C, while D7, D8, and D9 had the highest extraction efficiency at 45°C. Room temperature (24°C) was chosen as the optimal extraction temperature when considering the main polluents in the environment are D3, D4, D5, and D6.
Under the following experimental conditions of DVB/PDMS fiber, 40 mL of the standard solution (100 ng/L) was mixed with the 11 kind of siloxanes added to a 60-mL headspace bottle, pH 7.0, extraction temperature of 24°C, and desorption for 2 min at 220°C at the gas chromatography inlet. It can be seen from our experimental results that with an increase in the extraction time, the extraction efficiency of the 11 kinds of siloxanes increased. When the extraction time reached 45 min, the extraction reached equilibrium, and the peak area changed little when the extraction time reached 60 min (see Figure 4). To reduce the analysis time, 45 min was used as the optimized extraction time.
The addition of inorganic salts (such as NaCl) to the aqueous solution induced a salting-out effect, thereby increasing the ionic strength of the solution. This effectively reduced the solubility of the analyte in the solution and enhanced its volatility towards the headspace phase. Consequently, it enabled greater absorption of the target components by the fiber coating for the purpose of analysis, which ultimately improved the extraction efficiency. The effects of salinity on the extraction efficiency were investigated by adding different concentrations of NaCl to the water samples. The experimental conditions were as follows: DVB/PDMS fiber, 40 mL of standard solution (100 ng/L) mixed with the 11 kind of siloxanes added to a 60-mL headspace bottle, pH 7.0, extraction temperature of 24°C, extraction time of 45 min, and desorption for 2 min at 220°C at the gas chromatography inlet. Our results showed that the extraction efficiency of L3 and D3 could not be significantly increased upon adding NaCl to the water samples. However, the extraction efficiency of L4, D4, D5, L5, D6, L6, D7, D8, and D9 slowly increased with an increase in the NaCl concentration and when the NaCl concentration reached 0.10 g/mL, the extraction efficiency reached its maximum value (see Figure 5).
The experimental conditions were as follows: DVB/PDMS fiber, 40 mL of standard solution (100 ng/L) mixed with the 11 kinds of siloxanes added to a 60-mL headspace bottle, extraction temperature of 24°C, pH 7.0, extraction time of 45 min, and desorption at 220°C at the gas chromatography inlet. The influence of the desorption time at the gas chromatography inlet on the extraction efficiency of the target substance was investigated (Figure 6). Our results showed that the extraction efficiency (peak area) of L3, D3, and L4 was largest when the desorption time was 2 min, and the extraction efficiency significantly decreased upon extending the desorption time. The extraction efficiency of D4, D5, L5, L6, and D6 was highest at 3 min of desorption and the extraction efficiency of D7, D8, and D9 was highest at 5 min of desorption.
Although D4–D6 did not reach the maximum extraction efficiency at 2 min, the difference between D4 and D6 was not obvious. Therefore, considering that the extraction efficiency of L3 and L4 was significantly reduced when the desorption time was greater than 2 min, 2 min was selected as the final optimized desorption time.
We compared the DB-5MS (30 m × 0.25 mm, 0.25 μm) elastic quartz capillary column with a VF-WAX MS (30 m × 0.25 mm, 0.25 μm) elastic quartz capillary column. The DB-5MS column bleeding compound was the target compound of detection. When the column bleeding was large, it could not accurately reflect the true content of cyclic siloxane (D3, D4, D5, D6, etc.) in the sample. The VF-WAXms column was a relatively low loss column among all of the commercially available products and had less interference on target compounds, so this column was used in our study.
Because D3, D4, and L3 were extremely volatile, the initial temperature of the chromatography should be as low as possible under the premise of ensuring the complete separation of each target compound. Finally, the initial temperature was selected to be 35°C, held for 2 min, and the temperature was raised to 240°C at 10°C/min. The other conditions were as follows: Inlet temperature: 220°C; carrier gas: high purity helium; column flow rate: 1.0 mL/min; shunt injection, shunt ratio: 50:1.
At present, D3V is not detected in water and air, its physical and chemical properties are similar to the target objects to be measured, and its retention time was in the middle of the 11 kinds of siloxanes studied, so it was selected as the internal standard.
The method was assessed in terms of the detection limit, linearity range, calibration curve, precision, and accuracy using drinking and source water samples, respectively. The minimum detection mass concentration of the method was D3, 0.020 μg/L; L3, 0.012 μg/L; D4, 0.015 μg/L; L4, 0.010 μg/L; D5, 0.012 μg/L; L5, 0.020 μg/L; D6, 0.008 μg/L; L6, 0.022 μg/L; D7, 0.018 μg/L; D8, 0.018 μg/L; and D9, 0.025 μg/L. The linearity of the method was tested at six different concentration levels within the range of 0–10.0 μg/L. Each concentration level was analyzed in sextuplicate. The linearity of the calibration curve was assessed using the coefficient of determination. A good linearity was observed in the range of 0–10.0 μg/L for the 11 kinds of siloxanes. The precision and accuracy of the method were determined using spiked samples with three different levels (0.1, 0.5, and 2.0 ng/L). The accuracies of the method were in the range of 80%–98% and precision of the method was 1.8%–8.0%, respectively, indicating a compliance with the requirements of residual analysis. All of the data are summarized in Tables 3, 4.
This method was successfully applied to the identification and quantification of 11 kinds of siloxanes in 10 drinking water and 10 source water samples collected from Jilin province of China. None of the 11 substances were detected in the 10 samples of drinking water. D5 and D6 were detected in two samples of source water with D5 concentrations of 0.012 and 0.008 μg/L and D6 concentrations of 0.015 and 0.019 μg/L, respectively.
In this study, we have established a precise, fast, and selective method using solid-phase microextraction combined with gas chromatography-mass spectrometry (GC-MS/MS) method for the simultaneous detection of 11 types of siloxanes in drinking water and source water. Quantification of siloxanes was carried out by internal standard method. The results show that DVB/PDMS or DVB/PDMS/CAR fiber is the most effective coating for extracting siloxanes. This method provided good linearity (r > 0.9946) and precision (RSD%<8.0%), and low limits of quantification, from 0.008 to 0.025 μg/L. The developed method has been applied to the simultaneous analysis of 11 kinds of siloxanes in drinking water and source water, and the results showed that decamethylcyclopentasiloxane (D5) and dodecamethylcyclohexasiloxane (D6) were found in two source water samples at concentrations ranging from 0.008 to 0.012 μg/L and 0.015–0.019 μg/L, respectively.
To the best of our knowledge, this is the first report on using solid-phase microextraction combined with gas chromatography-mass spectrometry (GC-MS/MS) method for the identification and quantitation of 11 types of siloxanes in drinking water and source water. The extraction was performed using solid-phase microextraction to reduce the interference during the analysis. Meanwhile, D3V was used as the internal standard during the sample preparation step. This method only required 15 min for separation and detection, and was capable to achieve rapid qualitative detection, making it more suitable for the detection of emergent public health events. Therefore, the developed method was simple, quick, and effective and our satisfactory results demonstrated its suitability for the simultaneous determination of 11 kinds of siloxanes in drinking water and source water.
As the fillers of gas chromatography columns, methylpolysiloxane compounds including D3, D4, D5, D6, D7, D8, and D9 are commonly used and can contribute to column loss. In this study, various brands and models of columns were evaluated through extensive testing, leading to the selection of the VF-WAX column due to its minimal column loss and negligible detection of target siloxanes. PDMS/DVB and PDMS/DVB/CAR fibers contain limited amounts of siloxanes, making them suitable for detecting 11 siloxanes. However, to detect a broader range of siloxanes, future research should focus on improving methodologies and techniques. Therefore, it is imperative to further investigate and refine the solid-phase microextraction experimental conditions to ensure the detection of a broader range of siloxane compounds, particularly when analyzing trace or ultra-trace levels of siloxanes in water samples.
The original contributions presented in the study are included in the article/supplementary material, further inquiries can be directed to the corresponding author.
SL: Methodology, Writing–original draft, Writing–review and editing, Data curation, Formal Analysis, Resources. JM: Data curation, Formal Analysis, Methodology, Resources, Writing–original draft. SJ: Data curation, Formal Analysis, Writing–original draft.
The author(s) declare that financial support was received for the research, authorship, and/or publication of this article. This work was supported by key research and development project of department of science and technology of Jilin Province (grant number: 20240304116SF).
The authors declare that the research was conducted in the absence of any commercial or financial relationships that could be construed as a potential conflict of interest.
The author(s) declare that no Generative AI was used in the creation of this manuscript.
All claims expressed in this article are solely those of the authors and do not necessarily represent those of their affiliated organizations, or those of the publisher, the editors and the reviewers. Any product that may be evaluated in this article, or claim that may be made by its manufacturer, is not guaranteed or endorsed by the publisher.
Capela, D., Ratola, N., Alves, A., and Homem, V. (2017). Volatile methylsiloxanes through wastewater treatment plants – a review of levels and implications. Environ. Int. 102, 9–29. doi:10.1016/j.envint.2017.03.005
Companioni-Damas, E. Y., Santos, F. J., and Galceran, M. T. (2012). Analysis of linear and cyclic methylsiloxanes in water by headspace-solid phase microextraction and gas chromatographymass spectrometry. Talanta 89, 63–69. doi:10.1016/j.talanta.2011.11.058
Cortada, C., Costa dos Reis, L., Vidal, L., Llorca, J., and Canals, A. (2014). Determination of cyclic and linear siloxanes in wastewater samples by ultrasound-assisted dispersive liquid-liquid microextraction followed by gas chromatography-mass spectrometry. Talanta 120, 191–197. doi:10.1016/j.talanta.2013.11.042
Genualdi, S., Harner, T., Cheng, Y., MacLeod, M., Hansen, K. M., Egmond, R. V., et al. (2011). Global distribution of linear and cyclic volatile methyl siloxanes in air. Sci. Technol. 45 (8), 3349–3354. doi:10.1021/es200301j
Graiver, D., Farminer, K. W., and Narayan, R. (2003). A review of the fate and effects of silicones in the environment. J. Polym. Environ. 11 (4), 129–136. doi:10.1023/a:1026056129717
Guo, J. Y., Zhou, Y., Zhang, B. Y., and Zhang, J. B. (2019). Distribution and evaluation of the fate of cyclic volatile methyl siloxanes in the largest lake of southwest China. Sci. Total. Environ. 657, 87–95. doi:10.1016/j.scitotenv.2018.11.454
Hodgson, A. T., Faulkner, D., Sullivan, D. P., DiBartolomeo, D. L., Rusell, M. L., and Fisk, W. J. (2003). Effect of outside air ventilation rate on volatile organic compound concentrations in a call center. Atmos. Environ. 37, 5517–5527. doi:10.1016/j.atmosenv.2003.09.028
Homem, V., Capela, D., Silva, J. A., Cincinelli, A., Santos, L., Alves, A., et al. (2017). An approach to the environmental prioritisation of volatile methylsiloxanes in several matrices. Sci. Total. Environ. 579, 506–513. doi:10.1016/j.scitotenv.2016.11.068
Horii, Y., and Kannan, K. (2008). Survey of organosilicone compounds, including cyclic and linear siloxanes, in personal-care and household products. Archives. Environ. Contam. Toxi. 55, 701–710. doi:10.1007/s00244-008-9172-z
Kaj, L., Andersson, J., Cousins, A. P., Remberger, M., Ekheden, Y., Dusan, B., et al. (2005). Results from the Swedish national screening programme 2004, subreport 4: siloxanes IVL report B1643. Stockholm: Swedish National Research Institute. Available at: http://www.ivl.se/download/18.2f3a7b311a7c806443800055371/B1643.pdf (Accessed March 10, 2010).
Kierkegaard, A., Bignert, A., and McLachlan, M. S. (2013). Cyclic volatile methylsiloxanes in fish from the Baltic Sea. Chemosphere 93 (5), 774–778. doi:10.1016/j.chemosphere.2012.10.048
Kierkegaard, A., and McLachlan, M. S. (2010). Determination of decamethylcyclopentasiloxane in air using commercial solid phase extraction cartridges. J. Chromatogr. A 1217 (21), 3557–3560. doi:10.1016/j.chroma.2010.03.045
Lassen, C. L., Hansen, S. H., and Mikkelsen, J. M. (2005). Siloxanes – consumption, toxicity and alternatives, Danish ministry of the environment, environmental protection agency. Available at: http://www.miljoestyrelsen.dk/udgiv/publications/2005/87-7614-756-8/pdf/87-7614-757-6.pdf (Accessed March 3, 2010).
Lu, Y., Yuan, T., Wang, W. H., and Kannan, K. (2011). Concentrations and assessment of exposure to siloxanes and synthetic musks in personal care products from China. Environ. Pollut. 159 (12), 3522–3528. doi:10.1016/j.envpol.2011.08.015
Luciana, R., Lorena, V., and Antonio, C. (2018). Determination of siloxanes in water samples employing graphene oxide/Fe3O4 nanocomposite as sorbent for magnetic solid-phase extraction prior to gas chromatography-mass spectrometry. J. Separa Sci. 41 (22), 4177–4184. doi:10.1002/jssc.201800577
Raich-Montiu, J., Ribas-Font, C., Arespacochaga, N., Roig-Torres, E., Broto-Puig, F., Crest, M., et al. (2014). Analytical methodology for sampling and analysing eight siloxanes and trimethylsilanol in biogas from different wastewater treatment plants in Europe. Anal. Chim. Acta. 812, 83–91. doi:10.1016/j.aca.2013.12.027
Sparham, C., Van Egmond, R., Hastie, C., O’Connor, S., Gore, D., and Chowdhuryet, N. (2011). Determination of decamethylcyclopentasiloxane in river and estuarine sediments in the UK. J. Chromatogr. A 1218 (6), 817–823. doi:10.1016/j.chroma.2010.12.030
Sparham, C., Van Egmond, R., O'Connor, S., Hastie, C., Whelan, M., Kanda, R., et al. (2008). Determination of decamethylcyclopentasiloxane in river water and final effluent by headspace gas chromatography/mass spectrometry. J. Chromatogr. A 1212, 124–129. doi:10.1016/j.chroma.2008.10.014
Wang, D. G., Alaee, M., Steer, H., Tait, T., Williams, Z., Brimble, S., et al. (2013a). Determination of cyclic volatile methylsiloxanes in water, sediment, soil, biota, and biosolid using large-volume injection–gas chromatography–mass spectrometry. Chemosphere 93 (5), 741–748. doi:10.1016/j.chemosphere.2012.10.044
Wang, D. G., Norwood, W., Alaee, M., Byer, J. D., and Brimble, S. (2013b). Review of recent advances in research on the toxicity, detection, occurrence and fate of cyclic volatile methyl siloxanes in the environment. Chemosphere 93 (5), 711–725. doi:10.1016/j.chemosphere.2012.10.041
Wang, W. L., Zhang, Y., Sun, D. M., Chen, Z. Y., Qian, M., Zhou, Y., et al. (2023). Volatile Methylsiloxanes in complex samples: recent updates on pretreatment and analysis methods. Crit. Rev. Anal. Chem. 21, 3377–3397. doi:10.1080/10408347.2023.2245050
Wang, X. M., Lee, S. C., Sheng, G. Y., Chan, L. Y., Fu, J. M., Li, X. D., et al. (2001). Cyclic organosilicon compounds in ambient air in guangzhou, Macau and nanhai, pearl river delta. Appl. Geochem. 16, 1447–1454. doi:10.1016/s0883-2927(01)00044-0
Xu, L., Shi, Y., and Cai, Y. (2013). Occurrence and fate of volatile siloxanes in a municipal wastewater treatment Plant of Beijing, China. Water Res. 47 (2), 715–724. doi:10.1016/j.watres.2012.10.046
Zhang, Y. M., Yin, G., Sheng, G. D., Yu, Z. Y., and Yin, D. Q. (2024). Distribution and spatial variation of volatile methylsiloxanes in surface water and wastewater from the Yangtze River Basin, China. China. Sci. total. Environ. 929, 172541. doi:10.1016/j.scitotenv.2024.172541
Zhang, Z. F., Qi, H., Ren, N. Q., Li, Y. F., Gao, D. W., and Kannan, K. (2011). Survey of cyclic and linear siloxanes in sediment from the Songhua River and in sewage sludge from wastewater treatment plants, Northeastern China. Arch. Environ. Contam. Toxicol. 60 (2), 204–211. doi:10.1007/s00244-010-9619-x
Zhong, S. (2023). 2023 China polysiloxane production capacity forecast and product import and export analysis. Available at: http://www.askci.com/news/chanye/20230628/101632268791859125417668.shtml (Accessed July 28, 2023).
Keywords: drinking water, gas chromatography-mass spectrometry, internal standard method, siloxanes, solid-phase microextraction
Citation: Liu S, Ma J and Jiang S (2025) Simultaneous determination of 11 kinds of siloxanes in drinking water and source water using solid-phase microextraction combined with gas chromatography-mass spectrometry. Front. Chem. 13:1554453. doi: 10.3389/fchem.2025.1554453
Received: 02 January 2025; Accepted: 21 January 2025;
Published: 12 February 2025.
Edited by:
Valentina Gianotti, University of Eastern Piedmont, ItalyReviewed by:
Shima Salehinia, University of the Balearic Islands, SpainCopyright © 2025 Liu, Ma and Jiang. This is an open-access article distributed under the terms of the Creative Commons Attribution License (CC BY). The use, distribution or reproduction in other forums is permitted, provided the original author(s) and the copyright owner(s) are credited and that the original publication in this journal is cited, in accordance with accepted academic practice. No use, distribution or reproduction is permitted which does not comply with these terms.
*Correspondence: Sijie Liu, MDkyOGxzakAxNjMuY29t
Disclaimer: All claims expressed in this article are solely those of the authors and do not necessarily represent those of their affiliated organizations, or those of the publisher, the editors and the reviewers. Any product that may be evaluated in this article or claim that may be made by its manufacturer is not guaranteed or endorsed by the publisher.
Research integrity at Frontiers
Learn more about the work of our research integrity team to safeguard the quality of each article we publish.