- 1Department of Chemistry, Guru Ghasidas Vishwavidyalaya, Bilaspur, India
- 2Department of Physics, School of Basic and Applied Science, Galgotias University, Greater Noida, India
- 3Department of Chemistry, Rensselaer Polytechnic Institute, Troy, NY, United States
- 4Department of Chemistry, Indian Institute of Engineering Science and Technology, Howrah, India
We report the fabrication of a novel spinel-type Pd₀.₁Cu₀.₉Co₂O₄ nano-flake material designed for Mizoroki-Heck and Suzuki coupling-cum-transesterification reactions. The Pd₀.₁Cu₀.₉Co₂O₄ material was synthesized using a simple co-precipitation method, and its crystalline phase and morphology were characterized through powder XRD, UV-Vis, FESEM, and EDX studies. This material demonstrated excellent catalytic activity in Mizoroki-Heck and Suzuki cross-coupling reactions, performed in the presence of a mild base (K₂CO₃), ethanol as the solvent, and microwave irradiation under ligand-free conditions. Notably, the Heck coupling of acrylic esters proceeded concurrently with transesterification using various alcohols as solvents. The catalyst exhibited remarkable stability under reaction conditions and could be recycled and reused up to ten times while maintaining its catalytic integrity.
Introduction
Transition-metal-catalyzed cross-coupling reactions, namely, Mizoroki-Heck and Suzuki reactions, have gained recognition for both their utility and versatility in the construction of carbon-carbon bonds (Miyaura and Buchwald, 2002; Diederich and Stang, 2008; Negishi, 2011; Johansson Seechurn et al., 2012; De Meijere et al., 2013), which has widespread applications in the synthesis of biologically and pharmaceutically important scaffolds (Heck, 1979; Miyaura and Suzuki, 1995; Beletskaya and Cheprakov, 2000; Yin and Liebscher, 2007; Buchwald, 2008). Palladium was the first transition metal to be used as a catalyst for key organic reactions on an industrial level (Matos and Soderquist, 1998). Historically, homogeneous palladium catalysts in the form of metal salts with phosphines, N-heterocyclic carbenes (NHCs), and other organic ligands have been widely used in catalyzing cross-coupling reactions (Suzuki, 1999; Maureen, 2004; Jiang et al., 2007; Wu et al., 2010b; Wu et al., 2010a). However, growing economic and environmental concerns of homogeneous palladium catalysts have kickstarted research into the heterogenization of these catalysts. Researchers aim to create catalysts that maintain high catalytic activity while addressing economic and environmental concerns by immobilizing palladium on various inorganic and organic support materials. However, catalysts containing transition metals other than palladium, such as copper (Babu et al., 2013; Liwosz and Chemler, 2013; Gurung et al., 2014; Basnet et al., 2016; Tang et al., 2018; Budiman et al., 2019), cobalt (Asghar et al., 2017; Ludwig et al., 2020), or nickel (Gøgsig et al., 2012; Ramgren et al., 2013), have also been used to conduct various cross-coupling reactions. In recent years, spinels have gained recognition as active catalysts for organic transformations (Jagadeesh et al., 2013; Payra et al., 2016b; Payra et al., 2016a; Payra et al., 2018; Anke et al., 2019; Dong et al., 2019; Patel et al., 2020b; Ghazzy et al., 2022; Patel et al., 2022). These materials, also known as perovskites, are binary and ternary mixed metal oxides composed of mixed-valence transition metals, with a general formula of AB₂O₄, where A and B represent different metal cations. The presence of two mixed-valence metal cations facilitates electron transport between multiple transition metal cations, requiring relatively low activation energy (Jadhav et al., 2016; Kuang et al., 2016). Recently, spinel oxide-supported palladium catalysts such as PdAl2O4(Kannan, 2017), Pd/Fe3O4(Baran and Nasrollahzadeh, 2019), Pd/NiFe2O(Borhade and Waghmode, 2011), Pd/ZnFe2O4 (Singh et al., 2013), PdCuFe2O4 (Tong et al., 2016), PdCoFe2O4 (Senapati et al., 2012) have been reported to catalyze various cross-coupling reactions. Similarly to other spinels, Co3O4 adopts a normal spinel structure, consisting of Co2+ at tetrahedral sites and Co3+ at octahedral sites (Gao et al., 2016). In addition to the high activity, spinels provide additional benefits including low cost, ease of preparation, and high stability (Hamdani et al., 2010), Furthermore, the electrocatalytic efficiency of Co3O4 can be enhanced by the incorporation of additional metal ions (M = Zn, Cu, Ni, Mg, Fe, and Pd) into the oxide (Wu and Scott, 2011; Grewe et al., 2013; Rosen et al., 2013; Grewe et al., 2014; Liu et al., 2014; Kumar and Srivastava, 2020). The cobalt cation is partially substituted by a transition metal cation, which occupies the octahedral sites, while Co. occupies both the tetrahedral and octahedral sites, which in turn forms an inverse spinel structure (Liu et al., 2016). The use of cobalt catalysts, particularly CuCo2O4 have been reported in oxidation of alcohols (Jiang et al., 2019) and in the oxidative aza-coupling of amines. (Patel A. R. et al., 2020).
Transesterification is a classic organic reaction that involves the conversion of one ester into another through the exchange of alkoxy groups between an alcohol and the ester. Esters represent one of the most important functional groups found in polymers, agrochemicals, natural products, and biological systems, thereby making them widely applicable as key intermediates and/or protecting groups in organic transformations. (Larock, 1989; Otera and Nishikido, 2009; Nguyen et al., 2012). Transesterification reactions are widely used in organic synthesis and chemical industries (Otera, 1993; Grasa et al., 2004; Otera, 2004) as well as in polymer industries (Capelot et al., 2012) and biodiesel synthesis (Hindryawati et al., 2014; Lam et al., 2019). Recently, transesterification reactions that utilize diverse catalysts such as Lewis acids (Bosco and Saikia, 2004; Sheng and Kady, 2009), organic and inorganic bases (Jagtap et al., 2008; Watson et al., 2008; Sridharan et al., 2010), and N-heterocyclic carbenes (Grasa et al., 2002; Nyce et al., 2002; Singh et al., 2004; Zeng et al., 2009) have also been reported. However, there is currently no known methodology for performing one-pot cross-coupling reactions combined with transesterification.
As part of our ongoing efforts to develop novel transition metal-catalyzed reactions (Pati et al., 2018; Pati et al., 2020; Pati et al., 2024) and green synthetic methodologies using heterogeneous nanomaterials (Banerjee and Saha, 2013; Banerjee, 2015; Saha et al., 2015; Saha et al., 2017a; Saha et al., 2017b; Saha et al., 2018; Patel et al., 2019a; Patel et al., 2019b; Saha et al., 2019) we report the synthesis of hybrid Pd₀.₁Cu₀.₉Co₂O₄ spinel nano-flakes (Scheme 1), which effectively catalyze Mizoroki-Heck and Suzuki coupling reactions along with concomitant transesterification in a one-pot process. This occurs under ligand-free microwave irradiation conditions using an alcohol solvent (see Scheme 2).
Result and discussion
Firstly, we synthesized CuCo₂O₄ and Pd-doped CuCo₂O₄ using a simple co-precipitation method. The CuCo₂O₄ was prepared following a previously reported procedure (Sudha et al., 2019), while the Pd-doped CuCo₂O₄ was synthesized by doping an appropriate amount of palladium into the CuCo₂O₄ structure (details in ESI).
To investigate the crystalline form of the samples, X-ray powder diffraction (XRD) measurements were performed. The XRD patterns of the CuCo₂O₄ and Pd₀.₁Cu₀.₉Co₂O₄ samples are presented in Figure 1. For the CuCo₂O₄ sample, diffraction peaks were observed at 2θ values of 18.77°, 31.08°, 36.86°, 38.66°, 44.63°, 56.45°, 59.30°, and 65.55°, corresponding to the (111) (220) (311) (222) (400) (422) (511), and (440) planes, respectively.
The diffraction peaks observed correspond to the polycrystalline cubic spinel phase of CuCo₂O₄ (JCPDS Card No. 01–1155). The Pd₀.₁Cu₀.₉Co₂O₄ sample exhibits diffraction peaks at the same 2θ values as the CuCo₂O₄ sample, confirming that Pd is fully doped into the Cu site without forming any impurity phases (Bikkarolla and Papakonstantinou, 2015; Patel A. R. et al., 2020). The crystallite sizes (D) for both the pure and Pd-doped samples were calculated using the Debye–Scherrer formula (
The morphology of the Pd₀.₁Cu₀.₉Co₂O₄ sample was examined using field emission scanning electron microscopy (FESEM). Figure 2 presents the FESEM image, revealing the formation of a flake-like structure in the material. The average nano-flake size ranges from 760 nm for CuCo₂O₄ to 205 nm for Pd₀.₁Cu₀.₉Co₂O₄, indicating that the Pd-doped samples have a higher surface area compared to the pure CuCo₂O₄ samples.
The elemental composition and purity of the Pd₀.₁Cu₀.₉Co₂O₄ sample were determined using energy-dispersive X-ray spectroscopy (EDX). The EDX spectrum, shown in Figure 3, confirms the presence of dispersive peaks corresponding to the elements C, O, Co., Cu, Pd, and Pt (the latter due to the Pt coating applied during SEM measurements). The absence of dispersive peaks for other elements, within the statistical limits of detection, indicates the high purity of the Pd₀.₁Cu₀.₉Co₂O₄ material. (Patel A. R. et al., 2020).
Additionally, the optical properties of the Pd₀.₁Cu₀.₉Co₂O₄ sample were investigated using UV–Vis spectroscopy. Figure 4 shows the UV–Vis absorbance spectra of the as-prepared Pd₀.₁Cu₀.₉Co₂O₄ nano-flakes, which exhibit a broad absorption range spanning both the UV and visible regions. Two distinct absorption bands were observed at 500 nm and 750 nm. The band gap was determined using Tauc’s relation: αhν = C (hν−E.g.,) n\alpha h\nu = C(h\nu - E_g)∧nαhν = C(hν−E.g.,) n, where hνh\nuhν represents the photon energy, EgE_gEg is the optical band gap, and CCC is the band tailing parameter. For direct allowed transitions, nnn was set to 2. Figure 4B presents the Tauc’s plot used to estimate the direct optical band gap of the Pd₀.₁Cu₀.₉Co₂O₄ sample, which was found to be 1.82 eV.
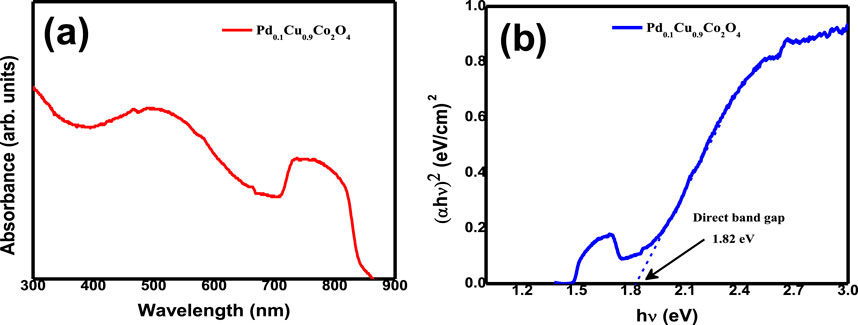
Figure 4. (A) UV-Vis spectrum of Pd₀.₁Cu₀.₉Co₂O₄ nano-flakes and (B) Tauc’s plot for the estimation of direct optical band gap for Pd₀.₁Cu₀.₉Co₂O₄ nano-flakes.
The catalytic activity of well-characterized Pd₀.₁Cu₀.₉Co₂O₄ nano-flakes was next evaluated in cross-coupling reactions. We began with the Heck coupling reaction of one-iodo-4-nitrobenzene (1) and acrylonitrile as a model system. When a mixture of one-iodo-4-nitrobenzene (1.0 mmol), acrylonitrile (1.5 mmol), K₂CO₃ (2.0 mmol), and Pd₀.₁Cu₀.₉Co₂O₄ nano-flakes (4 mol %) was stirred in DMF at 100°C, a 30% yield of (E)-3-(4-nitrophenyl) acrylonitrile (2) was obtained after 10 h (entry 1, Table 1).
In microwave-assisted reactions, the solvent absorbs microwave energy through dielectric heating, rapidly increasing the temperature and speeding up the reaction. Polar solvents, which have a higher dipole moment, absorb microwaves more efficiently compared to nonpolar solvents with zero or low dipole moments. This enhances reaction rates and selectivity, enabling superheating that improves yields and reaction outcomes under conditions not achievable with conventional heating. We then proceeded to optimize the reaction conditions, starting with the screening of various solvents (entries 1–5, Table 1). Ethanol (EtOH) emerged as the optimal solvent, yielding a 60% product yield in 6 h (entry 5, Table 1). Further optimization involved varying the base, catalyst amount, and reaction time. Increasing the catalyst amount to 8 mol % improved the yield (entry 6, Table 1), but further increases in catalyst quantity did not enhance the yield (entry 7, Table 1). Among the bases tested, K₂CO₃ proved to be the most effective (entries 8–10, Table 1).
Finally, we conducted the model reaction under microwave (MW) irradiation, which offers several advantages over conventional heating methods. These include significantly reduced reaction times, selective and direct heating of reactants and reagents without heating the reaction vessel, improved yields, and reduced by-product formation (Payra et al., 2016b; Roberts and Strauss, 2005; Kappe and Dallinger, 2006; Patel et al., 2021).
Initially, when the reaction was conducted under microwave irradiation at 50 W and 100°C for 10 min using 8 mol % of catalyst, a significant improvement in yield (100% conversion, 99% yield) was achieved using 2.0 equivalents of K₂CO₃ in 1 mL of ethanol (entry 11, Table 1). A similar conversion was observed when the catalyst amount was reduced to 4 mole% (entry 12, Table 1). However, further reducing the catalyst to 2 mol% led to a slight decrease in yield (entry 13, Table 1). Additionally, shortening the reaction time to 5 min resulted in a reduced yield of 76% (entry 14, Table 1). Therefore, for 1 mmol of one-iodo-4-nitrobenzene, the optimized conditions for the model Heck coupling reaction were determined to be 4 mol% of Pd₀.₁Cu₀.₉Co₂O₄ nano-flakes in ethanol under microwave irradiation (50 W, 100°C, 10 min) (entry 12, Table 1).
Next, the scope of this methodology was explored under optimized reaction conditions by reacting various conjugated alkenes with aryl halides, following a general experimental procedure (see ESI for details). Notably, when methyl acrylate was used instead of acrylonitrile, the cross-coupling reaction led to transesterification when ethanol (EtOH) was used as the solvent. Encouraged by this finding, we investigated the cross-coupling of aryl halides with methyl acrylate in the presence of different alcohols as solvents. The results, summarized in Table 2, show that the Heck coupling reaction with methyl acrylate resulted in 100% transesterification when ethanol, n-propanol, or n-butanol was used (entries 1–4, 6, 9, Table 2). However, when propyl acrylate or butyl acrylate was used as the alkene, only the straight cross-coupling product was observed, with no transesterification occurring (entries 10–11, Table 2).
Both aryl iodides and bromides reacted efficiently with various alkenes, such as acrylonitrile, methyl acrylate, and butyl acrylate, under the optimized conditions. Aryl iodides reacted faster than their bromide counterparts, likely due to the weaker C–I bond compared to the C–Br bond, which results in better leaving group ability for iodides, leading to higher yields with iodo-analogues. Additionally, we investigated the electronic effects of aryl halides on yield and reaction time with this catalytic system. Groups such as–NO₂ and–OMe on the aryl halides were well tolerated and enhanced the reaction rate. The reaction scope is detailed in Table 3. When acrylic acid was used, the Pd₀.₁Cu₀.₉Co₂O₄ nano-flakes catalyst facilitated the cross-coupling reaction followed by esterification of cinnamic acid with alcohol, yielding cinnamic acid esters.
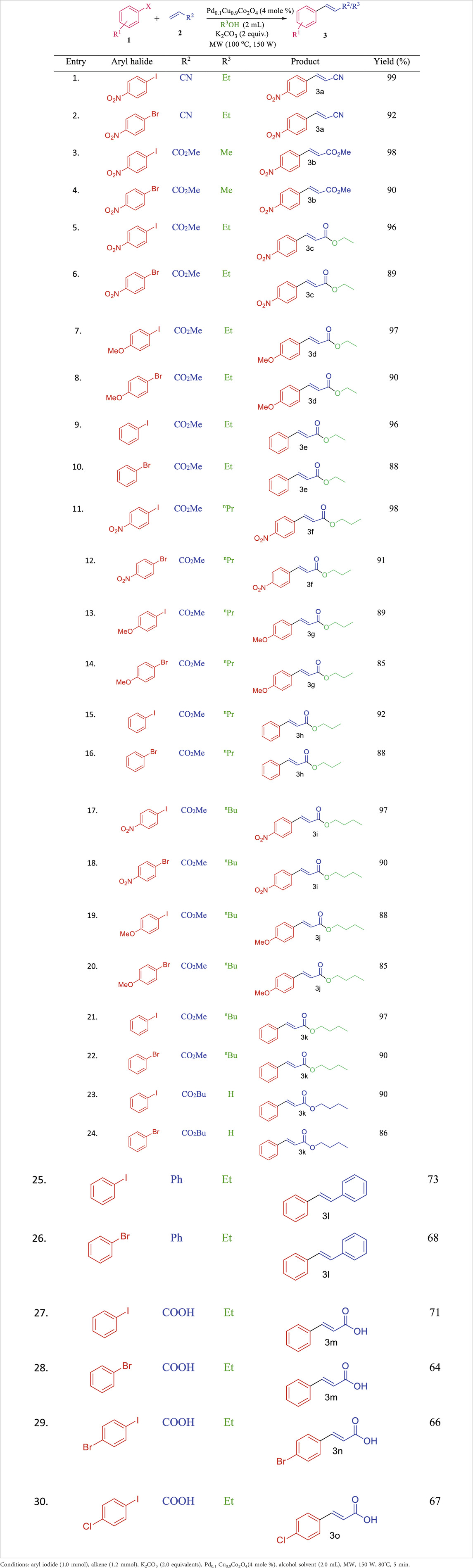
Table 3. Scope of Pd0.1Cu0.9Co2O4NFs-catalyzed Heck coupling with concomitant transesterification reactions.
We further assessed the catalytic performance of Pd₀.₁Cu₀.₉Co₂O₄ nano-flakes in another significant C–C bond-forming reaction: the Suzuki coupling of aryl halides with arylboronic acids to synthesize biaryl compounds. When a mixture of 1-nitro-4-iodobenzene (1 mmol), phenylboronic acid (1.2 mmol), and Pd₀.₁Cu₀.₉Co₂O₄ nano-flakes (10 mg) was heated at 100°C under microwave irradiation (150 W) in 2 mL of ethanol within a sealed microwave tube for 5 min, a quantitative yield of 4-nitrobiphenyl was obtained (Scheme 3).

Scheme 3. Synthesis of bi-aryl derivatives using Pd0.1Cu0.9Co2O4NFs catalyst via Suzuki coupling reactions.
The scope of the Suzuki coupling reaction for synthesizing biaryl derivatives was explored using a straightforward and general experimental procedure, with the results summarized in Table 4. The Pd₀.₁Cu₀.₉Co₂O₄ nano-flakes efficiently catalyzed the coupling of aryl halides with aryl boronic acids under microwave irradiation, yielding various substituted biaryl derivatives. Arylboronic acids with a wide range of substituents produced robust yields of cross-coupled products. Notably, substrates bearing a–CO₂Me group (5o and 5q) also underwent transesterification. Additionally, sterically hindered boronic acids, such as 2,4,6-trisubstituted boronic acids, delivered high yields of biaryl products under the optimized reaction conditions (5k). The stability and reusability of the Pd0.1Cu0.9Co2O4 nano-flakes were evaluated using the Heck coupling of 1-iodo-4-nitrobenzene with acrylonitrile to form (E)-3-(4-nitrophenyl)acrylonitrile as a model reaction on a 2 mmol scale. After the reaction, the organic component was dissolved in ethyl acetate, and the catalyst was recovered by centrifugation. The recovered catalyst was washed, dried at 80°C for 4 h, and reused for ten consecutive runs. The recycling results, shown in Figure 5, indicate that the catalyst remained stable and active throughout the ten cycles, with no significant loss in efficiency or product yield. The slight decrease in yield could be attributed to catalyst loss during recycling or agglomeration of the nano- flakes during the process.
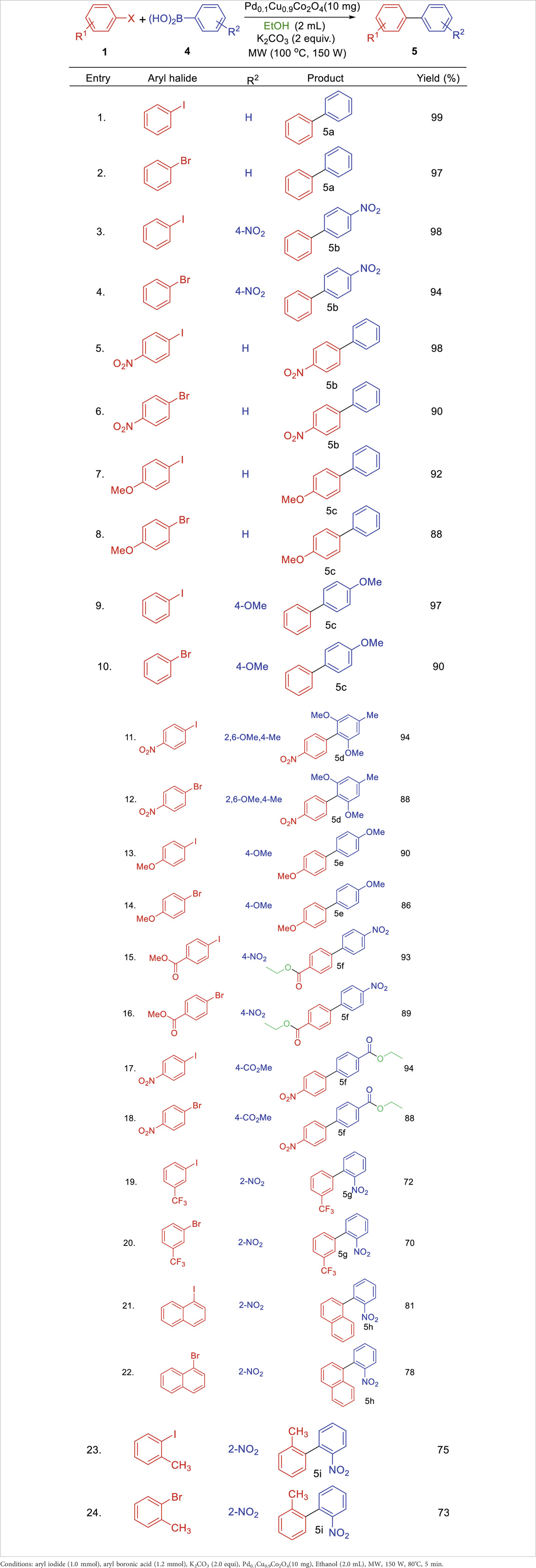
Table 4. Substrate scope of Pd0.1Cu0.9Co2O4NFs catalyzed Suzuki coupling of aryl halides and aryl boronic acids.
A hot filtration test was performed to assess the heterogeneity of the Pd0.1Cu0.9Co2O4 NFs catalyst through a leaching study. After 2 min of reaction (with 35% conversion achieved), the catalyst was removed from the reaction mixture using hot ultracentrifugation. The filtrate was then subjected to microwave (MW) irradiation for an additional 8 min, with reaction progress monitored at 2-min intervals. No further increase in product yield was observed after the catalyst was removed. As depicted in Figure 6, these results confirm that the Pd0.1Cu0.9Co2O4 NFs remained stable under the reaction conditions, with no detectable metal leaching from the catalyst.
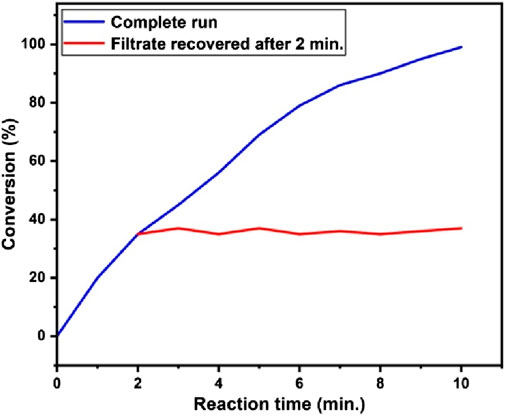
Figure 6. Results of the leaching study by hot-filtration test performed with (A) complete run (blue line), (B) filtrate removed after 2 min (red line) for the Heck-coupling cum transesterification reaction.
In the experiments, turnover number (TON) and turnover frequency (TOF) were determined using 10 mg of the Pd₀.₁Cu₀.₉Co₂O₄ catalyst, corresponding to a Pd content of 0.004 mol% in a 1 mmol scale reaction. For the Suzuki coupling reaction yielding biphenyl (5a), the calculated TON and TOF were 2500 and 15,000 h⁻1, respectively. Additionally, we conducted FESEM analysis to investigate the morphology of the reused catalyst. The FESEM image (Figure 7) of the catalyst after the 10th cycle confirmed that its flower-like structure remained intact, indicating stability and reusability of the catalyst.
The advantages of the Pd₀.₁Cu₀.₉Co₂O₄ nano-flakes catalyst for the Heck and Suzuki coupling reactions were highlighted by comparing it with previously reported Pd-based catalytic methods, as shown in Table 5. The comparison demonstrated that the Pd₀.₁Cu₀.₉Co₂O₄ catalyst outperforms other Pd-based spinel-structured catalysts, establishing it as a high-performance option in these reactions.
Conclusion
In conclusion, we synthesized spinel-type Pd₀.₁Cu₀.₉Co₂O₄ nano-flakes via a simple co-precipitation method and characterized them using powder XRD, UV-Vis, FESEM, and EDX. The material showed excellent catalytic activity in Mizoroki-Heck and Suzuki cross-coupling reactions under microwave irradiation. Key advantages include the use of a mild base (K₂CO₃), ethanol as a green solvent, ligand-free conditions, short reaction times (10 min), and high yields (86%–99%). Notably, methyl acrylate underwent complete transesterification, while butyl acrylate yielded only cross-coupling products. The catalyst was stable and reusable for up to ten cycles with minimal loss in activity.
Data availability statement
The original contributions presented in the study are included in the article/Supplementary Material, further inquiries can be directed to the corresponding authors.
Author contributions
AP: Methodology, Writing–original draft. GM: Data curation, Writing–original draft. TP: Conceptualization, Formal Analysis, Methodology, Supervision, Writing–original draft, Writing–review and editing. LA: Data curation, Methodology, Writing–original draft. CC: Writing–review and editing. SB: Conceptualization, Investigation, Supervision, Writing–original draft, Writing–review and editing.
Funding
The author(s) declare that no financial support was received for the research, authorship, and/or publication of this article.
Acknowledgments
Department of Chemistry, Guru Ghasidas Vishwavidyalaya Bilaspur Chhattisgarh for providing the platform.
Conflict of interest
The authors declare that the research was conducted in the absence of any commercial or financial relationships that could be construed as a potential conflict of interest.
Publisher’s note
All claims expressed in this article are solely those of the authors and do not necessarily represent those of their affiliated organizations, or those of the publisher, the editors and the reviewers. Any product that may be evaluated in this article, or claim that may be made by its manufacturer, is not guaranteed or endorsed by the publisher.
Supplementary material
The Supplementary Material for this article can be found online at: https://www.frontiersin.org/articles/10.3389/fchem.2024.1496234/full#supplementary-material
References
Anke, S., Bendt, G., Sinev, I., Hajiyani, H., Antoni, H., Zegkinoglou, I., et al. (2019). Selective 2-propanol oxidation over unsupported Co3O4 spinel nanoparticles: mechanistic insights into aerobic oxidation of alcohols. ACS Catal. 9, 5974–5985. doi:10.1021/acscatal.9b01048
Asghar, S., Tailor, S. B., Elorriaga, D., and Bedford, R. B. (2017). Cobalt-catalyzed suzuki biaryl coupling of aryl halides. Angew. Chem. 129, 16585–16588. doi:10.1002/ange.201710053
Babu, S. G., Neelakandeswari, N., Dharmaraj, N., Jackson, S. D., and Karvembu, R. (2013). Copper (II) oxide on aluminosilicate mediated Heck coupling of styrene with aryl halides in water. RSC Adv. 3, 7774–7781. doi:10.1039/c3ra23246h
Banerjee, S. (2015). The remarkable catalytic activity of ultra-small free-CeO2 nanoparticles in selective carbon–carbon bond formation reactions in water at room temperature. New J. Chem. 39, 5350–5353. doi:10.1039/c5nj00500k
Banerjee, S., and Saha, A. (2013). Free-ZnO nanoparticles: a mild, efficient and reusable catalyst for the one-pot multicomponent synthesis of tetrahydrobenzo [b] pyran and dihydropyrimidone derivatives. New J. Chem. 37, 4170–4175. doi:10.1039/c3nj00723e
Baran, T., and Nasrollahzadeh, M. (2019). Facile synthesis of palladium nanoparticles immobilized on magnetic biodegradable microcapsules used as effective and recyclable catalyst in Suzuki-Miyaura reaction and p-nitrophenol reduction. Carbohydr. Polym. 222, 115029. doi:10.1016/j.carbpol.2019.115029
Basnet, P., Thapa, S., Dickie, D. A., and Giri, R. (2016). The copper-catalysed Suzuki–Miyaura coupling of alkylboron reagents: disproportionation of anionic (alkyl)(alkoxy) borates to anionic dialkylborates prior to transmetalation. Chem. Commun. 52, 11072–11075. doi:10.1039/c6cc05114f
Beletskaya, I. P., and Cheprakov, A. V. (2000). The Heck reaction as a sharpening stone of palladium catalysis. Chem. Rev. 100, 3009–3066. doi:10.1021/cr9903048
Bikkarolla, S. K., and Papakonstantinou, P. (2015). CuCo2O4 nanoparticles on nitrogenated graphene as highly efficient oxygen evolution catalyst. J. Power Sources 281, 243–251. doi:10.1016/j.jpowsour.2015.01.192
Borhade, S. R., and Waghmode, S. B. (2011). Studies on Pd/NiFe2O4 catalyzed ligand-free Suzuki reaction in aqueous phase: synthesis of biaryls, terphenyls and polyaryls. Beilstein J. Org. Chem. 7, 310–319. doi:10.3762/bjoc.7.41
Bosco, J., and Saikia, A. K. (2004). Palladium (II) chloride catalyzed selective acetylation of alcohols with vinyl acetate. Chem. Commun. 35, 1116–1117. doi:10.1039/b401218f
Buchwald, S. L. (2008). Cross Coupling. Acc. Chem. Res. [ACS Publications] 41 (11), 1439. doi:10.1021/ar8001798
Budiman, Y. P., Friedrich, A., Radius, U., and Marder, T. B. (2019). Copper-Catalysed suzuki-miyaura cross-coupling of highly fluorinated aryl boronate esters with aryl iodides and bromides and Fluoroarene− arene π-stacking interactions in the products. ChemCatChem 11, 5387–5396. doi:10.1002/cctc.201901220
Capelot, M., Montarnal, D., Tournilhac, F., and Leibler, L. (2012). Metal-catalyzed transesterification for healing and assembling of thermosets. J. Am. Chem. Soc. 134, 7664–7667. doi:10.1021/ja302894k
De Meijere, A., Bräse, S., and Oestreich, M. (2013). Metal catalyzed cross-coupling reactions and more. John Wiley and Sons.
Diederich, F., and Stang, P. J. (2008). Metal-catalyzed cross-coupling reactions. John Wiley and Sons.
Dong, C., Qu, Z., Qin, Y., Fu, Q., Sun, H., and Duan, X. (2019). Revealing the highly catalytic performance of spinel CoMn2O4 for toluene oxidation: involvement and replenishment of oxygen species using in situ designed-TP techniques. Acs Catal. 9, 6698–6710. doi:10.1021/acscatal.9b01324
Gao, S., Jiao, X., Sun, Z., Zhang, W., Sun, Y., Wang, C., et al. (2016). Ultrathin Co3O4 layers realizing optimized CO2 electroreduction to formate. Angew. Chem. Int. Ed. 55, 698–702. doi:10.1002/anie.201509800
Ghazzy, A., Yousef, L., and Al-Hunaiti, A. (2022). Visible light induced nano-photocatalysis trimetallic Cu0. 5Zn0. 5-Fe: synthesis, characterization and application as alcohols oxidation catalyst. Catalysts 12, 611. doi:10.3390/catal12060611
Gøgsig, T. M., Kleimark, J., Nilsson Lill, S. O., Korsager, S., Lindhardt, A. T., Norrby, P.-O., et al. (2012). Mild and efficient nickel-catalyzed Heck reactions with electron-rich olefins. J. Am. Chem. Soc. 134, 443–452. doi:10.1021/ja2084509
Grasa, G. A., Kissling, R. M., and Nolan, S. P. (2002). N-heterocyclic carbenes as versatile nucleophilic catalysts for transesterification/acylation reactions. Org. Lett. 4, 3583–3586. doi:10.1021/ol0264760
Grasa, G. A., Singh, R., and Nolan, S. P. (2004). Transesterification/acylation reactions catalyzed by molecular catalysts. Synthesis., 971–985. doi:10.1055/s-2004-822323
Grewe, T., Deng, X., and TüYsüZ, H. (2014). Influence of Fe doping on structure and water oxidation activity of nanocast Co3O4. Chem. Mater. 26, 3162–3168. doi:10.1021/cm5005888
Grewe, T., Deng, X., Weidenthaler, C., SchüTh, F., and TüYsüZ, H. (2013). Design of ordered mesoporous composite materials and their electrocatalytic activities for water oxidation. Chem. Mater. 25, 4926–4935. doi:10.1021/cm403153u
Gurung, S. K., Thapa, S., Kafle, A., Dickie, D. A., and Giri, R. (2014). Copper-catalyzed Suzuki–Miyaura coupling of arylboronate esters: transmetalation with (PN) CuF and identification of intermediates. Org. Lett. 16, 1264–1267. doi:10.1021/ol500310u
Hamdani, M., Singh, R., and Chartier, P. (2010). Co3O4 and Co-based spinel oxides bifunctional oxygen electrodes. Int. J. Electrochem. Sci. 5, 556–577. doi:10.1016/s1452-3981(23)15306-5
Heck, R. F. (1979). Palladium-catalyzed reactions of organic halides with olefins. Accounts Chem. Res. 12, 146–151. doi:10.1021/ar50136a006
Hindryawati, N., Maniam, G. P., Karim, M. R., and Chong, K. F. (2014). Transesterification of used cooking oil over alkali metal (Li, Na, K) supported rice husk silica as potential solid base catalyst. Eng. Sci. Technol. Int. J. 17, 95–103. doi:10.1016/j.jestch.2014.04.002
Jadhav, H. S., Pawar, S. M., Jadhav, A. H., Thorat, G. M., and Seo, J. G. (2016). Hierarchical mesoporous 3D flower-like CuCo2O4/NF for high-performance electrochemical energy storage. Sci. Rep. 6, 31120. doi:10.1038/srep31120
Jagadeesh, R. V., Junge, H., Pohl, M.-M., Radnik, J. R., BrüCkner, A., and Beller, M. (2013). Selective oxidation of alcohols to esters using heterogeneous Co3O4–N@ C catalysts under mild conditions. J. Am. Chem. Soc. 135, 10776–10782. doi:10.1021/ja403615c
Jagtap, S. R., Bhor, M. D., and Bhanage, B. M. (2008). Synthesis of dimethyl carbonate via transesterification of ethylene carbonate with methanol using poly-4-vinyl pyridine as a novel base catalyst. Catal. Commun. 9, 1928–1931. doi:10.1016/j.catcom.2008.03.029
Jiang, X., Sclafani, J., Prasad, K., Repič, O., and Blacklock, T. J. (2007). Pd− Smopex-111: a new catalyst for heck and suzuki cross-coupling reactions. Org. process Res. and Dev. 11, 769–772. doi:10.1021/op7000657
Jiang, Y.-W., Chai, K., Wang, Y.-Q., Zhang, H.-D., Xu, W., Li, W., et al. (2019). Mesoporous silica-supported CuCo2O4 mixed-metal oxides for the aerobic oxidation of alcohols. ACS Appl. Nano Mater. 2, 4435–4442. doi:10.1021/acsanm.9b00828
Johansson Seechurn, C. C., Kitching, M. O., Colacot, T. J., and Snieckus, V. (2012). Palladium-catalyzed cross-coupling: a historical contextual perspective to the 2010 Nobel Prize. Angew. Chem. Int. Ed. 51, 5062–5085. doi:10.1002/anie.201107017
Kannan, V. (2017). PdAl2O4 spinel type nanoparticle synthesis through sol-gel route: an effective catalyst for Suzuki-Miyaura coupling reaction. IJCS 5, 923–927.
Kappe, C. O., and Dallinger, D. (2006). The impact of microwave synthesis on drug discovery. Nat. Rev. Drug Discov. 5, 51–63. doi:10.1038/nrd1926
Khazaei, A., Khazaei, M., and Nasrollahzadeh, M. (2017). Nano-Fe3O4@ SiO2 supported Pd (0) as a magnetically recoverable nanocatalyst for Suzuki coupling reaction in the presence of waste eggshell as low-cost natural base. Tetrahedron 73, 5624–5633. doi:10.1016/j.tet.2017.05.054
Kuang, M., Han, P., Wang, Q., Li, J., and Zheng, G. (2016). CuCo hybrid oxides as bifunctional electrocatalyst for efficient water splitting. Adv. Funct. Mater. 26, 8555–8561. doi:10.1002/adfm.201604804
Kumar, A., and Srivastava, R. (2020). Pd-decorated magnetic spinels for selective catalytic reduction of furfural: interplay of a framework-substituted transition metal and solvent in selective reduction. ACS Appl. Energy Mater. 3, 9928–9939. doi:10.1021/acsaem.0c01625
Lam, Y.-P., Wang, X., Tan, F., Ng, W.-H., Tse, Y.-L. S., and Yeung, Y.-Y. (2019). Amide/iminium zwitterionic catalysts for (trans) esterification: application in biodiesel synthesis. ACS Catal. 9, 8083–8092. doi:10.1021/acscatal.9b01959
Le, X., Dong, Z., Jin, Z., Wang, Q., and Ma, J. (2014). Suzuki–Miyaura cross-coupling reactions catalyzed by efficient and recyclable Fe3O4@ SiO2@ mSiO2–Pd (II) catalyst. Catal. Commun. 53, 47–52. doi:10.1016/j.catcom.2014.04.025
Li, W., Zhang, B., Li, X., Zhang, H., and Zhang, Q. (2013). Preparation and characterization of novel immobilized Fe3O4@ SiO2@ mSiO2–Pd (0) catalyst with large pore-size mesoporous for Suzuki coupling reaction. Appl. Catal. A General 459, 65–72. doi:10.1016/j.apcata.2013.04.010
Liu, S., San Hui, K., Hui, K. N., Yun, J. M., and Kim, K. H. (2016). Vertically stacked bilayer CuCo 2 O 4/MnCo 2 O 4 heterostructures on functionalized graphite paper for high-performance electrochemical capacitors. J. Mater. Chem. A 4, 8061–8071. doi:10.1039/c6ta00960c
Liu, X., Chang, Z., Luo, L., Xu, T., Lei, X., Liu, J., et al. (2014). Hierarchical ZnxCoxO4 nanoarrays with high activity for electrocatalytic oxygen evolution. Chem. Mater. 26, 1889–1895. doi:10.1021/cm4040903
Liwosz, T. W., and Chemler, S. R. (2013). Copper-catalyzed oxidative heck reactions between alkyltrifluoroborates and vinyl arenes. Org. Lett. 15, 3034–3037. doi:10.1021/ol401220b
Ludwig, J. R., Simmons, E. M., Wisniewski, S. R., and Chirik, P. J. (2020). Cobalt-catalyzed C (sp2)–C (sp3) Suzuki–Miyaura cross coupling. Org. Lett. 23, 625–630. doi:10.1021/acs.orglett.0c02934
Matos, K., and Soderquist, J. A. (1998). Alkylboranes in the Suzuki− miyaura coupling: stereochemical and mechanistic studies. J. Org. Chem. 63, 461–470. doi:10.1021/jo971681s
Maureen, R. (2004). A. Suzuki-coupling chemistry takes hold in commercial practice, from small-scale synthesis of screening compounds to industrial production of active ingredients. Chem. Eng. News 82, 49–58.
Miyaura, N., and Suzuki, A. (1995). Palladium-catalyzed cross-coupling reactions of organoboron compounds. Chem. Rev. 95, 2457–2483. doi:10.1021/cr00039a007
Negishi, E.-I. (2011). Magical power of transition metals: past, present, and future (nobel lecture). Angew. Chem. Int. Ed. 50, 6738–6764. doi:10.1002/anie.201101380
Nguyen, J. D., D'amato, E. M., Narayanam, J. M., and Stephenson, C. R. (2012). Engaging unactivated alkyl, alkenyl and aryl iodides in visible-light-mediated free radical reactions. Nat. Chem. 4, 854–859. doi:10.1038/nchem.1452
Nyce, G. W., Lamboy, J. A., Connor, E. F., Waymouth, R. M., and Hedrick, J. L. (2002). Expanding the catalytic activity of nucleophilic N-heterocyclic carbenes for transesterification reactions. Org. Lett. 4, 3587–3590. doi:10.1021/ol0267228
Otera, J. (2004). Toward ideal (trans) esterification by use of fluorous distannoxane catalysts. Accounts Chem. Res. 37, 288–296. doi:10.1021/ar030146d
Otera, J., and Nishikido, J. (2009). Esterification: methods, reactions, and applications. John Wiley and Sons.
Patel, A. R., Asatkar, A., Patel, G., and Banerjee, S. (2019a). Synthesis of rice husk derived activated mesoporous carbon immobilized palladium hybrid nano-catalyst for ligand-free mizoroki-heck/suzuki/sonogashira cross-coupling reactions. ChemistrySelect 4, 5577–5584. doi:10.1002/slct.201900384
Patel, A. R., Patel, G., and Banerjee, S. (2019b). Visible Light-Emitting Diode Light-Driven Cu0. 9Fe0. 1@ RCAC-catalyzed highly selective aerobic oxidation of alcohols and oxidative Azo-coupling of anilines: tandem one pot oxidation–condensation to imidazoles and imines. ACS omega 4, 22445–22455. doi:10.1021/acsomega.9b03096
Patel, A. R., Patel, G., Maity, G., Patel, S. P., Bhattacharya, S., Putta, A., et al. (2020a). Direct oxidative azo coupling of anilines using a self-assembled flower-like CuCo2O4 material as a catalyst under aerobic conditions. ACS omega 5, 30416–30424. doi:10.1021/acsomega.0c03562
Patel, G., Patel, A. R., Lambat, T. L., and Banerjee, S. (2021). Direct one-pot synthesis of imines/benzothiazoles/benzoxazoles from nitroarenes via sequential hydrogenation-condensation using Nano-NiFe2O4 as catalyst under microwave irradiation. Curr. Res. Green Sustain. Chem. 4, 100149. doi:10.1016/j.crgsc.2021.100149
Patel, G., Patel, A. R., Lambat, T. L., Mahmood, S. H., and Banerjee, S. (2020b). Rice husk derived nano-NiFe2O4@ CAGC-catalyzed direct oxidation of toluene to benzyl benzoate under visible LED light. FlatChem 21, 100163. doi:10.1016/j.flatc.2020.100163
Patel, G., Patel, A. R., Maity, G., Das, S., Patel, S. P., and Banerjee, S. (2022). Fabrication of self-assembled Co3O4 nano-flake for one-pot synthesis of tetrahydrobenzo [b] pyran and 1, 3-benzothazole derivatives. Curr. Res. Green Sustain. Chem. 5, 100258. doi:10.1016/j.crgsc.2021.100258
Pati, T. K., Ajarul, S., Kundu, M., Tayde, D., Khamrai, U., and Maiti, D. K. (2020). Synthesis of functionalized arylacetamido-2-pyridones through ortho-C (sp2)–H-activated installation of olefins and alkynes. J. Org. Chem. 85, 8563–8579. doi:10.1021/acs.joc.0c00941
Pati, T. K., Debnath, S., Kundu, M., Khamrai, U., and Maiti, D. K. (2018). 3-Amino-1-methyl-1 H-pyridin-2-one-Directed PdII catalysis: C (sp3)–H activated diverse arylation reaction. Org. Lett. 20, 4062–4066. doi:10.1021/acs.orglett.8b01618
Pati, T. K., Molla, S. A., Ghosh, N. N., Kundu, M., Ajarul, S., Maity, P., et al. (2024). 2-Pyridone-Directed CuII–catalyzed general method of C (sp2)-H activation for C–S, C–Se, and C–N cross-coupling: easy access to aryl thioethers, selenide ethers, and sulfonamides and DFT study. J. Org. Chem. 89, 6798–6812. doi:10.1021/acs.joc.4c00120
Payra, S., Saha, A., and Banerjee, S. (2016a). Nano-NiFe 2 O 4 as an efficient catalyst for regio-and chemoselective transfer hydrogenation of olefins/alkynes and dehydrogenation of alcohols under Pd-/Ru-free conditions. RSC Adv. 6, 52495–52499. doi:10.1039/c6ra09659j
Payra, S., Saha, A., and Banerjee, S. (2016b). Nano-NiFe 2 O 4 catalyzed microwave assisted one-pot regioselective synthesis of novel 2-alkoxyimidazo [1, 2-a] pyridines under aerobic conditions. RSC Adv. 6, 12402–12407. doi:10.1039/c5ra25540f
Payra, S., Saha, A., and Banerjee, S. (2018). Magnetically recoverable Fe3O4 nanoparticles for the one-pot synthesis of coumarin-3-carboxamide derivatives in aqueous ethanol. ChemistrySelect 3, 7535–7540. doi:10.1002/slct.201800523
Ramgren, S. D., Hie, L., Ye, Y., and Garg, N. K. (2013). Nickel-catalyzed Suzuki–Miyaura couplings in green solvents. Org. Lett. 15, 3950–3953. doi:10.1021/ol401727y
Roberts, B. A., and Strauss, C. R. (2005). Toward rapid, “green”, predictable microwave-assisted synthesis. Predict. microwave-assisted synthesis. Accounts Chem. Res. 38, 653–661. doi:10.1021/ar040278m
Rosen, J., Hutchings, G. S., and Jiao, F. (2013). Ordered mesoporous cobalt oxide as highly efficient oxygen evolution catalyst. J. Am. Chem. Soc. 135, 4516–4521. doi:10.1021/ja400555q
Saha, A., Payra, S., Akhtar, S., and Banerjee, S. (2017a). Fabrication of nano-CuO@ZnO for the synthesis of functionalized β-enaminone derivatives from β-nitrostyrenes, aliphatic/aromatic amines and 1,3-dicarbonyl/4-hydroxy coumarin. ChemistrySelect 2, 7319–7324. doi:10.1002/slct.201700890
Saha, A., Payra, S., and Banerjee, S. (2015). One-pot multicomponent synthesis of highly functionalized bio-active pyrano [2, 3-c] pyrazole and benzylpyrazolyl coumarin derivatives using ZrO 2 nanoparticles as a reusable catalyst. Green Chem. 17, 2859–2866. doi:10.1039/c4gc02420f
Saha, A., Payra, S., and Banerjee, S. (2017b). Synthesis of smart bimetallic nano-Cu/Ag@ SiO 2 for clean oxidation of alcohols. New J. Chem. 41, 13377–13381. doi:10.1039/c7nj02062g
Saha, A., Payra, S., Selvaratnam, B., Bhattacharya, S., Pal, S., Koodali, R. T., et al. (2018). Hierarchical mesoporous RuO2/Cu2O nanoparticle-catalyzed oxidative homo/hetero azo-coupling of anilines. ACS Sustain. Chem. and Eng. 6, 11345–11352. doi:10.1021/acssuschemeng.8b01179
Saha, A., Wu, C. M., Peng, R., Koodali, R., and Banerjee, S. (2019). Facile synthesis of 1, 3, 5-triarylbenzenes and 4-aryl-NH-1, 2, 3-triazoles using mesoporous Pd-MCM-41 as reusable catalyst. Eur. J. Org. Chem. 2019, 104–111. doi:10.1002/ejoc.201801290
Senapati, K. K., Roy, S., Borgohain, C., and Phukan, P. (2012). Palladium nanoparticle supported on cobalt ferrite: an efficient magnetically separable catalyst for ligand free Suzuki coupling. J. Mol. Catal. A Chem. 352, 128–134. doi:10.1016/j.molcata.2011.10.022
Sheng, D. P., and Kady, I. O. (2009). Catalysis by supported Lewis acids: an efficient method for transesterification of phosphotriesters. Appl. Catal. A General 365, 149–152. doi:10.1016/j.apcata.2009.05.061
Singh, A. S., Patil, U. B., and Nagarkar, J. M. (2013). Palladium supported on zinc ferrite: a highly active, magnetically separable catalyst for ligand free Suzuki and Heck coupling. Catal. Commun. 35, 11–16. doi:10.1016/j.catcom.2013.02.003
Singh, R., Kissling, R. M., Letellier, M.-A., and Nolan, S. P. (2004). Transesterification/acylation of secondary alcohols mediated by N-heterocyclic carbene catalysts. J. Org. Chem. 69, 209–212. doi:10.1021/jo035431p
Sridharan, V., Ruiz, M., and Menéndez, J. C. (2010). Mild and high-yielding synthesis of β-keto esters and β-ketoamides. Synthesis 2010, 1053–1057. doi:10.1055/s-0029-1217135
Stevens, P. D., Li, G., Fan, J., Yen, M., and Gao, Y. (2005). Recycling of homogeneous Pd catalysts using superparamagnetic nanoparticles as novel soluble supports for Suzuki, Heck, and Sonogashira cross-coupling reactions. Chem. Commun., 4435–4437. doi:10.1039/b505424a
Sudha, V., Annadurai, K., Kumar, S. M. S., and Thangamuthu, R. (2019). CuCo 2 O 4 nanobricks as electrode for enhanced electrochemical determination of hydroxylamine. Ionics 25, 5023–5034. doi:10.1007/s11581-019-03026-0
Suzuki, A. (1999). Recent advances in the cross-coupling reactions of organoboron derivatives with organic electrophiles, 1995–1998. J. Organomet. Chem. 576, 147–168. doi:10.1016/s0022-328x(98)01055-9
Tang, C., Zhang, R., Zhu, B., Fu, J., Deng, Y., Tian, L., et al. (2018). Directed copper-catalyzed intermolecular Heck-type reaction of unactivated olefins and alkyl halides. J. Am. Chem. Soc. 140, 16929–16935. doi:10.1021/jacs.8b10874
Tong, J., Su, L., Bo, L., Cai, X., Zhang, Q., and Wang, Q. (2016). Mesoporous palladium–copper ferrites as highly efficient and magnetically separable catalysts for Suzuki coupling reaction. Mater. Res. Bull. 73, 240–246. doi:10.1016/j.materresbull.2015.09.013
Vaddula, B. R., Saha, A., Leazer, J., and Varma, R. S. (2012). A simple and facile Heck-type arylation of alkenes with diaryliodonium salts using magnetically recoverable Pd-catalyst. Green Chem. 14, 2133–2136. doi:10.1039/c2gc35673b
Vibhute, S. P., Mhaldar, P. M., Shejwal, R. V., and Pore, D. M. (2020a). Magnetic nanoparticles-supported palladium catalyzed Suzuki-Miyaura cross coupling. Tetrahedron Lett. 61, 151594. doi:10.1016/j.tetlet.2020.151594
Vibhute, S. P., Mhaldar, P. M., Shejwal, R. V., Rashinkar, G. S., and Pore, D. M. (2020b). Palladium schiff base complex immobilized on magnetic nanoparticles: an efficient and recyclable catalyst for Mizoroki and Matsuda-Heck coupling. Tetrahedron Lett. 61, 151801. doi:10.1016/j.tetlet.2020.151801
Watson, D. A., Fan, X., and Buchwald, S. L. (2008). Carbonylation of aryl chlorides with oxygen nucleophiles at atmospheric pressure. Preparation of phenyl esters as acyl transfer agents and the direct preparation of alkyl esters and carboxylic acids. J. Org. Chem. 73, 7096–7101. doi:10.1021/jo800907e
Wu, X., and Scott, K. (2011). CuxCo3− xO4 (0≤ x< 1) nanoparticles for oxygen evolution in high performance alkaline exchange membrane water electrolysers. J. Mater. Chem. 21, 12344–12351. doi:10.1039/c1jm11312g
Wu, X. F., Anbarasan, P., Neumann, H., and Beller, M. (2010a). From noble metal to Nobel prize: palladium-catalyzed coupling reactions as key methods in organic synthesis. Angew. Chem. Int. Ed. 48, 9047–9050. doi:10.1002/anie.201006374
Wu, X. F., Anbarasan, P., Neumann, H., and Beller, M. (2010b). Palladium-catalyzed carbonylative C-H activation of heteroarenes. Angew. Chem. 40, 7474–7477.
Yin, L., and Liebscher, J. (2007). Carbon− carbon coupling reactions catalyzed by heterogeneous palladium catalysts. Chem. Rev. 107, 133–173. doi:10.1021/cr0505674
Keywords: spinel-type catalyst, nano-flake material, Mizoroki-Heck reaction, Suzuki coupling reaction, transesterification, microwave irradiation, recyclable catalyst, cross-coupling reactions
Citation: Patel AR, Maity G, Pati TK, Adak L, Cioffi CL and Banerjee S (2024) Hybrid Pd0.1Cu0.9Co2O4 nano-flakes: a novel, efficient and reusable catalyst for the one-pot heck and Suzuki couplings with simultaneous transesterification reactions under microwave irradiation. Front. Chem. 12:1496234. doi: 10.3389/fchem.2024.1496234
Received: 14 September 2024; Accepted: 07 October 2024;
Published: 30 October 2024.
Edited by:
Shuntaro Tsubaki, Kyushu University, JapanReviewed by:
Xin Gao, Tianjin University, ChinaSagnik Sengupta, University of Texas Southwestern Medical Center, United States
Copyright © 2024 Patel, Maity, Pati, Adak, Cioffi and Banerjee. This is an open-access article distributed under the terms of the Creative Commons Attribution License (CC BY). The use, distribution or reproduction in other forums is permitted, provided the original author(s) and the copyright owner(s) are credited and that the original publication in this journal is cited, in accordance with accepted academic practice. No use, distribution or reproduction is permitted which does not comply with these terms.
*Correspondence: Tanmay K. Pati, patit@rpi.edu, patitanmay@gmail.com; Subhash Banerjee, ocsb2006@gmail.com
†ORCID: Tanmay K. Pati, orcid.org/0000-0001-5652-4442