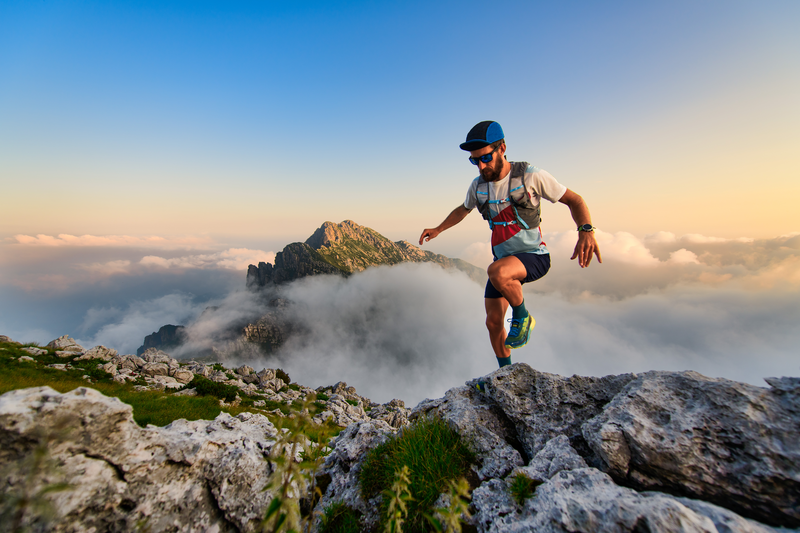
94% of researchers rate our articles as excellent or good
Learn more about the work of our research integrity team to safeguard the quality of each article we publish.
Find out more
ORIGINAL RESEARCH article
Front. Chem. , 07 August 2024
Sec. Chemical Biology
Volume 12 - 2024 | https://doi.org/10.3389/fchem.2024.1448171
This article is part of the Research Topic Advances in Bioactive Peptides and Functional Properties of Foods View all 6 articles
Chia (Salvia hispanica L.) flour seeds produce films with good barrier properties against water vapor and could be used as food packaging; however, their mechanical properties are poor, which limits their application. The incorporation of nanoparticles into natural polymers is a strategy used to improve the properties of films to increase their applications. Furthermore, nanoparticles can encapsulate antioxidant agents and generate active films. The objective of this study was to evaluate the influence of chia flour (4%–7%), glycerol (15%–25%), and chia extract-loaded chitosan nanoparticles (ChCNp) (0%–0.75%) on the physical, mechanical, barrier, structural and antioxidant properties of chia flour nanocomposite films. Chitosan nanoparticles loaded with antioxidant chia extract were synthesized by ionic gelation and incorporated into the films. The thickness, water vapor permeability, tensile strength, and antioxidant properties of the films were evaluated using a Box-Behnken experimental design. Structural analysis was conducted using the FTIR technique. The results of the ANOVA of the responses were adjusted to second and third order polynomial models obtaining determination coefficients of 0.96–0.99. The water vapor permeability of the films was 3.89 × 10-8–1.68 × 10−7 g mm/Pa s m2, tensile strength was 0.67–3.59 MPa and antioxidant activity was 57.12%–67.84%. The variables presented different effects on the films. Increasing the chia seed flour concentration negatively affected the water vapor permeability but improved the tensile strength and the antioxidant capacity of the films. The increase in glycerol concentration caused the films to become brittle. The nanoparticles had a significant effect on the thickness of the films and improved their mechanical and antioxidant properties. However, they did not show an effect on barrier properties. The results demonstrate that it is possible to obtain nanocomposite films with antioxidant capacity from chia seed flour and with the incorporation of chitosan nanoparticles loaded with antioxidants.
The interest in developing packaging films based on biopolymers and natural bioactive substances has increased recently (Rao et al., 2023). Films with antioxidant and antimicrobial functional properties help to extend the shelf life of food, reducing microbial growth and preventing spoilage reactions. They are also biodegradable, avoiding the environmental pollution caused by synthetic packaging. Chia (Salvia hispanica L.) is a native plant from Mexico and Guatemala. Its seeds contain biopolymers such as proteins (15%–25%), carbohydrates (25%–41%), dietary fibre (34%), and lipids (30%–33%) (Vera-Cespedes et al., 2023). These polymers can be used to develop films. The film-forming properties of chia mucilage have been widely studied (Muñoz et al., 2012; Dick et al., 2015a; Salazar et al., 2020; Urbizo-Reyes et al., 2020; Kosarsoy, 2022; Semwal et al., 2022). However, there are few studies on films made from chia seed flour. Dick et al. (2015b) evaluated the physicochemical, structural, barrier, and mechanical properties of chia flour-based films, reporting that the films presented excellent barrier properties but inferior mechanical properties, so their application is reduced. A strategy applied to improve the mechanical properties of films is the incorporation of nanoparticles. The nanostructures can interact with the polymeric matrix, thus increasing their resistance (Dash et al., 2019). Mujtaba et al. (2019a) incorporated cellulose nanofibers into chia mucilage films, and the particles improved the tensile strength. The addition of starch nanocrystals has also been reported; however, these particles did not significantly affect the mechanical properties of chia mucilage films (Mujtaba et al., 2019b). Not all nanoparticles interact in the same way with polymeric matrices, which is why it is not possible to generalize their action, and it is important to evaluate their effect in each of the possible polymeric matrices. Chitosan is a biocompatible, non-toxic, antimicrobial polymer derived from chitin that can form nanoparticles by ionic gelation (Ju et al., 2020). The incorporation of chitosan particles into films improves their barrier and mechanical properties, as reported by various authors (Garavand et al., 2020; Lee et al., 2021). The effect of adding chitosan particles in flour films of chia seeds has been little studied. Chitosan nanoparticles can also serve as a vehicle for the incorporation of bioactive substances and generate nanocomposite active packaging (Roy and Rhim, 2021). Active packaging with natural antioxidant compounds is a novel alternative applied to prevent lipid oxidation in foods. These reactions together with microbial growth are the main causes of the loss of sensory and nutritional quality of foods (Abiodun et al., 2023). Due to the growing demand for natural antioxidants in the food industry, new sources are being sought for the extraction of these compounds. In chia seeds, important phenolic compounds, such as kaempferol, quercetin, myricetin, and chlorogenic and caffeic acids have been identified (Morales-Olán et al., 2020) and the antioxidant capacity of the aqueous and ethanolic extracts of its seeds has been determined (Alcântara et al., 2019; Kulczyński et al., 2019). These extracts could be applied as antioxidant agents in the food industry. In previous studies, it was determined that the encapsulation of the antioxidant ethanolic extract of chia seeds in chitosan particles is possible, with good encapsulation efficiency (Morales-Olán et al., 2021). These nanoparticles could be added to chia flour films to improve their mechanical properties and obtain active nanocomposite packaging films. The objective of this study was to evaluate the effect of the content of chia flour (4%–7%), glycerol (15%–25%), and chia extract-loaded chitosan nanoparticles (ChCNp) (0%–0.75%) on the physical, barrier, mechanical, structural and antioxidant properties of chia flour nanocomposite films.
Chia seeds were acquired from local producers in Puebla City, Mexico. Seeds were ground in a food processor (Krups GX410011, Groupe SEB, Solingen, Germany), and the particle size was homogenized by passage through a 60-mesh screen to generate the flour. Sodium tripolyphosphate (TPP), chitosan (75%–85% deacetylated, medium molecular mass), glacial acetic acid (≥99.5%), glycerol, ethanol (≥99.5%) and 2,2-diphenyl-1-picrylhydrazyl (DPPH) were obtained from Sigma-Aldrich (Sigma Co., Saint Louis, United States).
The ChCNp were prepared following the methodology proposed by Morales-Olán et al. (2021). Briefly, chia extract (0.2 mg/mL) was mixed with the TPP (0.07%, w/v). The solution was slowly added to the chitosan solution (0.05%) with stirring at 25°C. Chitosan particles with chia extract were collected by centrifugation (MultifugeTM X3; Thermo ScientificTM) at 12,000 rpm for 30 min. Characterization of the particles included the determination of the encapsulation efficiency (EE), loading capacity (LC), size, morphology, and zeta potential. The size and morphology of the particles were examined by using a Scanning Electron Microscope (SEM-FE-JOL 7610F, JEOL, Tokyo, Japan) with a secondary electron detector and an Oxford EBSD detector (2.0 KV). The images were analyzed with ImageJ 1.52a to determine the particle size. The zeta potential (ζ) was measured using a Zetasizer Nano ZSP (Malvern Instruments, United Kingdom). Measurements were made in triplicate at 25°C. The EE and LC were calculated using Equations 1, 2.
Chia flour (4%–7%, w/v) was dissolved in distilled water, the pH was adjusted to 10 and the solution was stirred for 25 min. The solutions were heated at 80°C for 15 min, followed by the addition of glycerol (15%–25%, dry basis). The mixture was centrifuged at 5,000 rpm for 10 min. The ChCNp (0%, 0.375%, and 0.75%, based on the weight of chia flour) were added to the film-forming solution and magnetically stirred for 10 min at 25°C, placed in silicone molds (12.57 cm2) and dried for 12 h at 40°C. Before characterization, the films were conditioned with saturated NaBr solutions (58% RH) for 48 h.
The nanoparticle-free and nanocomposite films were characterized by FTIR spectroscopy (Vertex 70v; Bruker, Bremen, Germany) with an attenuated total reflectance (ATR) accessory. The measurement was performed in triplicate in the region of 4,000 to 500 cm−1.
The thickness of the films was measured with a micrometer (MCD-1” MX, 0-1 IN, Mitutoyo, Kawasaki, Japan). The measurements were made in different areas of the films. The mean of the measurements was used to determine the mechanical and barrier properties.
The cup method of the ASTM E96-95 standard was applied following the methodology of Beristain-Bauza et al. (2016) with slight modifications. The films were placed on top of beakers (25 mm diameter) with anhydrous silica (0% RH). The glasses were placed in a desiccator with NaCl solution (75% RH) at 25°C.
The water content transferred to the films was determined from the mass gain every 24 h for 120 h. WVP (g mm/Pa s m2) was calculated using Equation 3:
Where, WVT = water vapor transmission (g/Pa s m2), calculated from the slope of the linear regression of the mass gain versus time divided by beakers’ area (A, m2); L = film’s thickness; S = saturation vapor pressure at 25°C (Pa); R1 = RH of saturated NaCl solution; R2 = RH of desiccant.
A texture analyzer (EZS 500 N, Shimadzu, Kyoto, Japan) was used to measure the TS according to ASTM D882-18. The films were cut into 2.0 × 5.0 cm strips. The grip spacing was 45 mm and the crosshead speed was 0.5 mm/s.
The DPPH radical-scavenging activity was measured following the method reported by Chaichi et al. (2023) with brief modifications. The films were mixed with DPPH methanol solution (100 mM) for 30 min in the dark. The activity was determined with Equation 4, where A = Absorbance.
A Box-Behnken experimental design (BBD) was applied to evaluate the influence of variables on the nanocomposite films. The independent variables were the chia flour content (
Where Yk = response variables: T (mm), WVP (g mm/Pa s m2), TS (MPa), and DPPH radical-scavenging activity (%). Independent coded variables include the content of
The nanoparticles presented an EE of 93.0% ± 4.5%. The EE was higher than that reported in the encapsulation of other antioxidant extracts, such as aqueous grape extract loaded in chitosan particles (51.90% ± 1.33%) (Soleymanfallah et al., 2022). The concentration of the extract used in the encapsulation of the particles was determined according to the results obtained in previous studies (Morales-Olán et al., 2021). The highest encapsulation efficiency was achieved with this concentration. The LC of the particles was 16.2% ± 1.5%. The values found were higher than those reported in chitosan particles loaded with resveratrol and tannic acid (Wu et al., 2017; Leonida et al., 2023). The particles presented a spherical morphology and a size of 39.7 ± 8.4 (Figure 1A). The zeta potential was 26.3 mV, this value showed that the synthesized colloidal particles were unstable and tended to agglomerate. The results agree with those reported by Ivancica et al. (2020).
Figure 1. (A) SEM micrograph of chia extract loaded nanoparticles; (B) chia flour films without nanoparticles; (C) nanocomposite chia flour films with chia extract-loaded chitosan nanoparticles.
The results of the BBD employed to analyze the effect of the independent variables were as follows: the effects of chia flour (4%–7%), glycerol (15%–25%) and ChCNp (0.0%–0.75%) contents on the physical, mechanical, barrier, and antioxidant properties of chia films are shown in Table 1. The average measurements of the dependent variables were introduced into the design to obtain the fitting parameters and ANOVA (Table 2). The results showed that the quadratic regression models of the T and TS responses were statistically significant (p < 0.05). The lack of fit for the F values of the thickness and TS responses implies that the fit is not significant in relation to the pure error. There is a 9.9% and 5.9%, respectively, chance of lack of adjustment of the F value due to noise. The insignificance of the lack of fit indicates that the model fits the experimental data. All response variables presented a high R2 (Table 2), which shows a good correlation between the predicted and experimental values. Based on the results, these models are suitable to predict the responses in films made with chia flour containing chitosan particles encapsulating chia extract. The regression analysis for model fitting to assess the significance of the coefficient terms for all responses in the experimental design is shown in Table 3. For the T of the films, the regression coefficients for the factor chia flour (
Figure 2 shows the FTIR spectra of the films prepared without (Figure 2A) and with nanoparticles (Figure 2B). In both spectra, bands at 3,285 cm−1 related to the N-H vibrations of the proteins were observed. The bands present at 2,917 and 2,850 cm-1 correspond to the vibrations of the C-H bond of proteins, carbohydrates, and glycerol. On the other hand, the signals at 1744 cm−1 arise from the stretching vibration of the C=O group of the lipids. The bands related to amides I and II of the proteins were observed at 1,641 and 1,537 cm−1 (Wang et al., 2020). Signals from the methyl methylene groups present in lipids and proteins were observed at 1,452 cm−. Finally, the bands located at 1,039 and 994 cm−1 are due to the C–O and C–C vibrations of the plasticizing agent. No differences were observed between the spectra of the films without nanoparticles and for the nanocomposite films. The concentration of the nanoparticles is so low that their presence does not cause spectral changes.
Figure 2. FTIR spectrum of the film with (A): 0% nanoparticles, 7% chia flour, 20% glycerol, and (B): nanocomposite film with 0.75% nanoparticles, 7% chia flour and 20% glycerol.
Three-dimensional response surface graphs were constructed between two independent variables, keeping the third variable constant (Figure 3). The behaviours found in each of the study responses are described below.
Figure 3. Response surface plots of the combined effects of independent variables of the simple and nanocomposite chia flour films with chitosan particles loaded with chia extract (ChCNp). (A–C) thickness, (D–F) water vapor permeability (WVP), (G–I) tensile strength, and (J–L) antioxidant activity.
The films without nanoparticles were brown in color and homogeneous (Figure 1B). On the other hand, nanocomposite chia flour films were heterogeneous, flexible, and rough, due to the incorporation of the particles (Figure 1C). Thickness values of the chia flour nanocomposite films range from 0.02 to 0.04 mm (Table 1). The T was 10 times less than that of films of chia flour mixed with corn starch (Dick et al., 2015a) and similar to that of mucilage films without particles and mucilage films with cellulose nanofibers and starch nanocrystals (Dick et al., 2015b; Mujtaba et al., 2019a,b). Statistical analysis showed that chia flour, glycerol, and ChCNp had a significant impact on this parameter (Table 3) (Figures 3A–C). The addition of the nanoparticles generated a decrease in the T of the films (Figure 3C). The results are similar to those for mucilage films with starch nanocrystals (Mujtaba et al., 2019b). According to this research, the decrease can be attributed to the favorable interaction between the mucilage and the nanoparticles. Cabedo and Gamez-Pérez (2018) described that the nanoparticles in the films can be dispersed, intercalated, or aggregated, or there can be a combination of these. The intercalated particles generate a decrease in the thickness of the films according to Shariatinia et al. (2017). The final equation in terms of real variables for T is the following Equation 7:
The WVP of the films was 3.89 × 10−8–1.68 × 10−7 g mm/Pa s m2. These values were similar to those reported in chia flour and mucilage films (Dick et al., 2015a,b), but better than the WVP for the mucilage chia films combined with concentrated whey protein (Muñoz et al., 2012). The WVP of the nanocomposite chia flour films were higher than those reported for synthetic films such as polypropylene (PP) (4.7 × 10−9 g mm/Pa s m2) and polyethylene terephthalate (PET) (1.3 × 10−9 g mm/Pa s m2) (Janjarasskul and Krochta, 2010). The WVP was significantly affected by the chia flour content (Table 3). The WVP increased with the concentration of chia flour (Figures 3D–F). Chia seed flour contains mainly carbohydrates and proteins. Mucilage is made up of arabinose, xylose, glucose, glucuronic acid, galactose, and galacturonic acid (Beikzadeh et al., 2020) and has a high water-retention capacity (Urbizo-Reyes et al., 2020), so increasing its concentration in the film can favor the WVP. The ChCNp did not significantly influence the WVP of chia flour films. Comparable results have been reported by other authors with some concentrations of nanoparticles (Ju et al., 2020; Roy and Rhim, 2021). Possibly, the concentration of the particles used is low, so its effect on this property is not appreciated. In this work, the possibility of adding more nanoparticles was not considered because the film formed was very heterogeneous. The final equation in terms of real variables for the WVP is the Equation 8:
The TS of the films was 0.67–3.59 MPa. These values are comparable to those reported for chia flour films (0.77 MPa) and chia mucilage films mixed with whey protein (3.79 MPa) (Muñoz et al., 2012; Dick et al., 2015b). The TS was significantly influenced by the flour and glycerol content, as well as by the interactions between flour and glycerol and flour and nanoparticles (p < 0.05) (Table 3). The films with a higher concentration of chia flour presented a higher TS (Figure 3G). When the polymer concentration is increased, more interactions occur between them, increasing the resistance of the films. Glycerol negatively affected this parameter: its increase caused a decrease in TS (Figures 3G,I). These results agree with those reported by Urbizo-Reyes et al. (2020). The hydroxyl groups of glycerol contribute to the incorporation of water molecules, which can increase the molecular mobility of the films, decreasing their resistance. On the other hand, when the concentrations of flour and nanoparticles were simultaneously increased, the films presented a higher TS (Figure 3H). The greatest reinforcement was obtained with concentrations of chia flour of 6.4%–7% and 0.45%–0.75% of nanoparticles. The nanoparticles interacted best with chia flour at high concentrations. The main interactions that have been described between chitosan nanoparticles and polymeric matrices are intermolecular interactions due to hydrogen bonds (Zhao et al., 2023). Although the nanoparticles agglomerated (Figure 1C), they managed to reinforce the films. These results are similar to those reported by Shapi’i et al. (2022) for starch films incorporating chitosan nanoparticles and by Hosseini et al. (2015) for fish gelatine films reinforced with chitosan nanoparticles. The final equation in terms of real variables for TS is the Equation 9:
The films had a DPPH scavenging activity of 57.12%–67.84%. The antioxidant capacity found was greater than that reported in chitosan films added with chitosan particles loaded with quercetin (Roy and Rhim, 2021). This response was significantly affected by the flour content and by the interactions between flour, glycerol, and nanoparticles (Table 3). The highest antioxidant activity was found in the films made in the range of 4.6%–6.4% chia flour (Figure 3J). As described previously, phenolic compounds such as myricetin, quercetin, and kaempferol have been identified in chia flour and its ethanolic extracts, which can stabilize free radicals (Alcântara et al., 2019). On the other hand, it was observed that the antioxidant activity increases from 60% to 68% at low concentrations of glycerol and high concentrations of ChCNp (Figure 3L). ChCNp showed a positive effect on the antioxidant activity of the films. Similar results were reported by other authors in films added with nanoparticles loaded with antioxidants (Zhang and Zhao, 2017; Ezati and Rhim, 2021; Roy and Rhim, 2021). In previous studies carried out in the working group, it was found that the synthesized nanoparticles have an antioxidant capacity when they are subjected to 40°C (drying temperature of the films) and at pHs of 6 and 10 (Morales-Olán et al., 2021) by which the antioxidant capacity of these films could be increased by having contact with foods with these pHs. On the other hand, it is suggested to evaluate the antioxidant capacity of the films during their storage. The final equation in terms of real variables is the Equation 10:
In this study, the influence of the content of flour, glycerol and chitosan nanoparticles loaded with antioxidants on the physical, barrier, mechanical, structural and antioxidant properties of chia flour films was evaluated. The variables studied generated different effects on the properties of the films. Increasing the content of chia flour negatively affected the WVP; however, it improved the TS and antioxidant capacity of the films. On the other hand, the increase in glycerol concentration generated brittle films, due to its hydrophilic behavior and plasticizing capacity. The addition of nanoparticles produced heterogeneous films; however, the nanoparticles showed a significant effect on the thickness and improved the mechanical and antioxidant properties of the films. The results demonstrate that chitosan nanoparticles loaded with antioxidants improve the properties of chia flour films and allow the generation of nanocomposite films with antioxidant capacity. These films could be used as an active packaging alternative for food preservation.
The raw data supporting the conclusions of this article will be made available by the authors, without undue reservation.
GM-O: Formal Analysis, Investigation, Methodology, Writing–original draft. PM-Z: Formal Analysis, Writing–review and editing. MR-C: Formal Analysis, Writing–review and editing. AH-C: Formal Analysis, Writing–review and editing. MR-L: Data curation, Formal Analysis, Investigation, Methodology, Project administration, Resources, Supervision, Visualization, Writing–original draft, Writing–review and editing. SL-S: Data curation, Formal Analysis, Investigation, Methodology, Project administration, Resources, Supervision, Visualization, Writing–original draft, Writing–review and editing.
The author(s) declare financial support was received for the research, authorship, and/or publication of this article. Our thanks to the Consejo Nacional de Humanidades, Ciencias y Tecnologías (CONAHCYT) for the financial support.
The authors declare that the research was conducted in the absence of any commercial or financial relationships that could be construed as a potential conflict of interest.
All claims expressed in this article are solely those of the authors and do not necessarily represent those of their affiliated organizations, or those of the publisher, the editors and the reviewers. Any product that may be evaluated in this article, or claim that may be made by its manufacturer, is not guaranteed or endorsed by the publisher.
ChCNp, Chia extract-loaded chitosan nanoparticles; EE, Encapsulation efficiency; LC, Loading capacity; WVP, Water vapor permeability; TS, Tensile strength.
Abiodun, O. A., Abiodun, S. O., and Arise, A. K. (2023a). Antioxidant packaging, en Elsevier eBooks, 1–23. doi:10.1016/b978-0-323-95644-4.00005-x
Alcântara, M. A., De Lima Brito Polari, I., De Albuquerque Meireles, B. R. L., De Lima, A. E. A., Da Silva, J. C., Junior, De, et al. (2019). Effect of the solvent composition on the profile of phenolic compounds extracted from chia seeds. Food Chem. 275, 489–496. doi:10.1016/j.foodchem.2018.09.133
Beikzadeh, S., Khezerlou, A., Jafari, S. M., Pilevar, Z., and Mortazavian, A. M. (2020). Seed mucilages as the functional ingredients for biodegradable films and edible coatings in the food industry. Adv. Colloid Interface Sci. 280, 102164. doi:10.1016/j.cis.2020.102164
Beristain-Bauza, S. C., Mani-López, E., Palou, E., and López-Malo, A. (2016). Antimicrobial activity and physical properties of protein films added with cell-free supernatant of Lactobacillus rhamnosus. Food control. 62, 44–51. doi:10.1016/j.foodcont.2015.10.007
Cabedo, L., and Gamez-Pérez, J. (2018). “Inorganic-based nanostructures and their use in food packaging,” in Micro and nano technologies, nanomaterials for food packaging. Editors M. A. Ribeiro Cerqueira, J. M. Lagaron, L. M. Pastrana Castro, and A. A. Soares Vicente (Elsevier). doi:10.1016/B978-0-323-51271-8.00002-4
Chaichi, M., Badii, F., Mohammadi, A., and Hashemi, M. (2023). Novel bioactive composite films based on pectin-nanocellulose-synergistic triple essential oils: development and characterization. Food Bioprocess Tech. 16 (8), 1794–1805. doi:10.1007/s11947-023-03036-x
Dash, K. K., Ali, N. A., Das, D., and Mohanta, D. (2019). Thorough evaluation of sweet potato starch and lemon-waste pectin based-edible films with nano-titania inclusions for food packaging applications. Int. J. Biol. Macromol. 139, 449–458. doi:10.1016/j.ijbiomac.2019.07.193
Dick, M., Costa, T. M. H., Gomaa, A., Subirade, M., De Oliveira Rios, A., and Flôres, S. H. (2015a). Edible film production from chia seed mucilage: effect of glycerol concentration on its physicochemical and mechanical properties. Carbohydr. Polym. 130, 198–205. doi:10.1016/j.carbpol.2015.05.040
Dick, M., Pagno, C. H., Costa, T. M. H., Gomaa, A., Subirade, M., De Oliveira Rios, A., et al. (2015b). Edible films based on chia flour: development and characterization. J. Appl. Polym. Sci. 133 (2), 1–9. doi:10.1002/APP.42455
Ezati, P., and Rhim, J. (2021). Fabrication of quercetin-loaded biopolymer films as functional packaging materials. ACS Appl. Polym. Mater. 3 (4), 2131–2137. doi:10.1021/acsapm.1c00177
Garavand, F., Cacciotti, I., Vahedikia, N., Rehman, A., Tarhan, Ö., Alavijeh, A., et al. (2020). A comprehensive review on the nanocomposites loaded with chitosan nanoparticles for food packaging. Crit. Rev. Food Sci. Nutr. 62, 1383–1416. doi:10.1080/10408398.2020.1843133
Ivancica, T., Thompson, M. R., Pawlakb, J. L., and Lawtonc, D. (2020). Influence of anionic and non-ionic surfactants on nanoparticle synthesis by solvent-free extrusion emulsification. Colloids Surf. A Physicochem. Eng. Asp. 587, 124328. doi:10.1016/j.colsurfa.2019.124328
Janjarasskul, T., and Krochta, J. M. (2010). Edible packaging materials. Annu. Rev. Food Sci. 1 (1), 415–448. doi:10.1146/annurev.food.080708.100836
Ju, S., Zhang, F., Duan, J., and Jiang, J. (2020). Characterization of bacterial cellulose composite films incorporated with bulk chitosan and chitosan nanoparticles: a comparative study. Carbohydr. Polym. 237, 116167. doi:10.1016/j.carbpol.2020.116167
Kosarsoy, G. (2022). A new approach to nanocomposite carbohydrate polymer films: levan and chia seed mucilage. Int. J. Biol. Macromol. 218, 751–759. doi:10.1016/j.ijbiomac.2022.07.157
Kulczyński, B., Kobus-Cisowska, J., Taczanowski, M., Kmiecik, D., and Gramza-Michałowska, A. (2019). The chemical composition and nutritional value of chia seeds—current state of knowledge. Nutrients 11 (6), 1242. doi:10.3390/nu11061242
Lee, S., Zhang, M., Wang, G., Meng, W., Zhang, X., Wang, D., et al. (2021). Characterization of polyvinyl alcohol/starch composite films incorporated with p-coumaric acid modified chitosan and chitosan nanoparticles: a comparative study. Carbohydr. Polym. 262, 117930. doi:10.1016/j.carbpol.2021.117930
Leonida, M., Benzecry, A., Lozanovska, B., Mahmoud, Z., Reid, A., and Belbekhouche, S. (2023). Impact of tannic acid on nisin encapsulation in chitosan particles. Int. J. Biol. Macromol. 233, 123489. doi:10.1016/j.ijbiomac.2023.123489
Morales-Olán, G., Luna-Suárez, S., Figueroa-Cárdenas, J., Corea, M., and Rojas-López, M. (2021). Synthesis and characterization of chitosan particles loaded with antioxidant extracted from chia (Salvia hispanica L.) seeds. Int. J. Anal. Chem. 2021, 1–12. doi:10.1155/2021/5540543
Morales-Olán, G., Rojas-López, M., Díaz-Reyes, J., Rosas-Cárdenas, F., and Luna-Suárez, S. (2020). Effect of ethanol and methanol on the total phenolic content and antioxidant capacity of chia seeds (Salvia hispanica L.). Sains Malays 49, 1283–1292. doi:10.17576/jsm-2020-4906-06
Mujtaba, M., Akyuz, L., Koc, B., Kaya, M., Ilk, S., Cansaran-Duman, D., et al. (2019a). Novel, multifunctional mucilage composite films incorporated with cellulose nanofibers. Food Hydrocoll. 89, 20–28. doi:10.1016/j.foodhyd.2018.10.021
Mujtaba, M., Koc, B., Salaberria, A. M., Ilk, S., Cansaran-Duman, D., Akyuz, L., et al. (2019b). Production of novel chia-mucilage nanocomposite films with starch nanocrystals; an inclusive biological and physicochemical perspective. Int. J. Biol. Macromol. 133, 663–673. doi:10.1016/j.ijbiomac.2019.04.146
Muñoz, L. A., Aguilera, J. M., Rodriguez-Turienzo, L., Cobos, A., and Diaz, O. (2012). Characterization and microstructure of films made from mucilage of Salvia hispanica and whey protein concentrate. J. Food Eng. 111 (3), 511–518. doi:10.1016/j.jfoodeng.2012.02.031
Rao, Z., Lei, X., Chen, Y., Ling, J., Zhao, J., and Ming, J. (2023). Facile fabrication of robust bilayer film loaded with chitosan active microspheres for potential multifunctional food packing. Int. J. Biol. Macromol. 231, 123362. doi:10.1016/j.ijbiomac.2023.123362
Roy, S., and Rhim, J. (2021). Fabrication of chitosan-based functional nanocomposite films: effect of quercetin-loaded chitosan nanoparticles. Food Hydrocoll. 121, 107065. doi:10.1016/j.foodhyd.2021.107065
Salazar Vega, I. M., Quintana Owen, P., and Segura Campos, M. R. (2020). Physicochemical, thermal, mechanical, optical, and barrier characterization of chia (Salvia hispanica L.) mucilage-protein concentrate biodegradable films. J. Food Sci. 85, 892–902. doi:10.1111/1750-3841.14962
Semwal, A., Ambatipudi, K., and Kumar, N. K. (2022). Development and characterization of sodium caseinate based probiotic edible film with chia mucilage as a protectant for the safe delivery of probiotics in functional bakery. Food Hydrocoll. Hlth 2, 100065. doi:10.1016/j.fhfh.2022.100065
Shapi’i, R. A., Othman, S. H., Basha, R. K., and Naim, M. N. (2022). Mechanical, thermal, and barrier properties of starch films incorporated with chitosan nanoparticles. Nanotechnol. Rev. 11 (1), 1464–1477. doi:10.1515/ntrev-2022-0094
Shariatinia, Z., and Zahraee, Z. (2017). Controlled release of metformin from chitosan–based nanocomposite films containing mesoporous MCM-41 nanoparticles as novel drug delivery systems. J. Colloid Interface Sci. 501, 60–76. doi:10.1016/j.jcis.2017.04.036
Soleymanfallah, S., Khoshkhoo, Z., Hosseini, S. E., and Azizi, M. H. (2022). Preparation, physical properties, and evaluation of antioxidant capacity of aqueous grape extract loaded in chitosan-TPP nanoparticles. Food Sci. Nutr. 10 (10), 3272–3281. doi:10.1002/fsn3.2891
Urbizo-Reyes, U., Martín-González, M. F. S., García-Bravo, J., and Liceaga, A. M. (2020). Development of chia seed (Salvia hispanica) mucilage films plasticized with polyol mixtures: mechanical and barrier properties. Int. J. Biol. Macromol. 163, 854–864. doi:10.1016/j.ijbiomac.2020.07.023
Vera-Cespedes, N., Muñoz, L. A., Rincón, M. Á., and Haros, C. M. (2023). Physico-chemical and nutritional properties of chia seeds from Latin American countries. Foods 12 (16), 3013. doi:10.3390/foods12163013
Wang, Y., Zhang, R., Qin, W., Dai, J., Zhang, Q., Lee, K., et al. (2020). Physicochemical properties of gelatin films containing tea polyphenol-loaded chitosan nanoparticles generated by electrospray. Mater. Des. 185, 108277. doi:10.1016/j.matdes.2019.108277
Wu, J., Wang, Y., Yang, H., Liu, X., and Lu, Z. (2017). Preparation and biological activity studies of resveratrol loaded ionically cross-linked chitosan-TPP nanoparticles. Carbohydr. Polym. 175, 170–177. doi:10.1016/j.carbpol.2017.07.058
Zhang, S., and Zhao, H. (2017). Preparation and properties of zein–rutin composite nanoparticle/corn starch films. Carbohydr. Polym. 169, 385–392. doi:10.1016/j.carbpol.2017.04.044
Keywords: biodegradable films, sustainable packaging, food preservation, antioxidant activity, active packaging
Citation: Morales-Olán G, Moreno-Zarate P, Ríos-Corripio MA, Hernández-Cázares AS, Rojas-López M and Luna-Suárez S (2024) Nanocomposite films based on chia (Salvia hispanica L.) flour seeds incorporating antioxidant chitosan nanoparticles. Front. Chem. 12:1448171. doi: 10.3389/fchem.2024.1448171
Received: 12 June 2024; Accepted: 26 July 2024;
Published: 07 August 2024.
Edited by:
Olga Avrutina, Darmstadt University of Technology, GermanyReviewed by:
Karla A. Garrido Miranda, Scientific and Technological Bioresource Nucleus (BIOREN-UFRO), ChileCopyright © 2024 Morales-Olán, Moreno-Zarate, Ríos-Corripio, Hernández-Cázares, Rojas-López and Luna-Suárez. This is an open-access article distributed under the terms of the Creative Commons Attribution License (CC BY). The use, distribution or reproduction in other forums is permitted, provided the original author(s) and the copyright owner(s) are credited and that the original publication in this journal is cited, in accordance with accepted academic practice. No use, distribution or reproduction is permitted which does not comply with these terms.
*Correspondence: Silvia Luna-Suárez, c2lsdmlhbHMyMDA0QHlhaG9vLmNvbS5teA==; Marlon Rojas-López, bWFybG9ucmxAeWFob28uY29tLm14
Disclaimer: All claims expressed in this article are solely those of the authors and do not necessarily represent those of their affiliated organizations, or those of the publisher, the editors and the reviewers. Any product that may be evaluated in this article or claim that may be made by its manufacturer is not guaranteed or endorsed by the publisher.
Research integrity at Frontiers
Learn more about the work of our research integrity team to safeguard the quality of each article we publish.