- 1College of Chemistry and Chemical Engineering, Zhaotong University, Zhaotong, China
- 2Experimental Center for Teaching, Hebei Medical University, Shijiazhuang, China
- 3College of Chemistry and Materials Engineering, Wenzhou University, Wenzhou, China
Highly thermostable RhB@Zr-Eddc composites with the Rhodamine B (RhB) enclosed into the nanocages of Zr-Eddc was synthesized by one-pot method under hydrothermal conditions, whose structure, morphology and stability were characterized through the X-ray powder diffractometry (XRD), scanning electron microscopy (SEM) and thermogravimetric analysis (TGA). RhB@Zr-Eddc showed the highly thermal stability up to 550°C and emitted the bright red-light emission at 605 nm, which could highly selective detect the nitrofurazone (NFZ) among eleven other antibiotics in aqueous solution. Furthermore, via combining the RhB@Zr-Eddc with commercial green phosphor (Y3Al5O12:Ce3+, Ga3+), the mixture was encapsulated onto a 455 nm blue LED chip, creating an ex-cellent white light emitting diode (WLED) device with the correlated colour temperature (CCT) of 4710 K, luminous efficiency (LE) of 43.17 lm/w and Color Rendering Index (CRI) of 89.2.
1 Introduction
With the rapid expansion of livestock farming and aquaculture on a large scale, antibiotic veterinary drugs are extensively employed as feed additives to enhance animal growth and prevent/treat diseases in livestock and poultry (Hutchings et al., 2019; Svetlana et al., 2020; Divysnshi et al., 2022). Global statistics indicate an annual consumption of veterinary antibiotics exceeding 63,000 tons in areas like animal husbandry and aquaculture. This data is projected to rise to 106,600 tons by 2030, with quinolones, tetracyclines, sulphonamides, and others being the most commonly used veterinary drugs (Pepper et al., 2018; Kovalakova et al., 2020; Lyu et al., 2020). Due to the lack of effective regulation, a significant quantity of antibiotic-based veterinary drugs is consumed by animals. This results in enriched residues found in animal tissues, organs, and edible products, while livestock excretions and aquaculture wastewater discharge into the environment, causing environmental pollution and posing significant risks to human health (Baaloudj et al., 2021). Furthermore, due to the poor absorption of antibiotic veterinary drugs by organisms, these residues can persist in soil and water bodies, leading to microbial flora drug resistance and various ecological issues (Zhang et al., 2015; Chen et al., 2022; Zhang et al., 2024). This situation has a profound impact on human health and disrupts ecological balance. The complexity of antibiotic veterinary drug structures and the technical challenges in their detection make fluorescence detection methods, particularly those employing fluorescent sensors and signals, crucial. These methods offer high sensitivity, rapid response, and simplicity in operation, making them widely adopted in sensing detection (Chen et al., 2017; Zhou et al., 2018; Saboor et al., 2022). Given these considerations, the development of an easily operable, cost-effective and simple fluorescent sensor for detecting antibiotic residues is paramount for ensuring human health and environmental safety.
Metal-Organic Frameworks (MOFs) constitute a class of functional materials formed through the self-assembly of metal ions or clusters with organic ligands via ligand bonding. Among these, MOFs with fluorescent properties combine the stability of MOFs with high fluorescence efficiency. This unique combination allows for efficient fluorescence sensing through fluorescence bursts, rendering them highly applicable in the detection of antibiotic and pesticide residues (Hou et al., 2018; Zhu et al., 2018; Zhao et al., 2019; Duan et al., 2020; Yin et al., 2023). Despite their versatility, there is a scarcity of fluorescent MOFs-based composites exhibiting both high selectivity and sensitivity in the detection of antibiotics (Wang et al., 2022; Heng et al., 2023; Li et al., 2023; Luo et al., 2023; Zhong et al., 2023; Lei et al., 2024; Wu et al., 2024). Consequently, there is an urgent need to design and prepare more MOFs-based fluorescent composites tailored for high-selectivity, high-sensitivity antibiotic detection.
In this paper, the red Rhodamine B (RhB) fluorescent dye into the nanopores or cages of Zr-Eddc material to create highly thermally stable and efficient RhB@Zr-Eddc. The aim is to explore their potential applications in antibiotic detection and as components in white light emitting diode (WLED) devices. The findings from this research are expected to offer both experimental and theoretical guidance for the future development of MOFs fluorescent composites specifically designed for antibiotic detection and WLED devices.
2 Results and discussion
2.1 Preparation of materials, structural characterization and their structural analysis
H2Eddc, ZrCl4 and RhB dye were utilized in the preparation of RhB@Zr-Eddc through one-pot method under hydrothermal conditions, where the red RhB dye was incorporated into the nano-cage of Zr-Eddc (Figure 1A and Supplementary Figure S2). Characterization of the prepared Zr-Eddc and RhB@Zr-Eddc was conducted using XRD, revealing that the XRD peaks of RhB@Zr-Eddc align well with those of Zr-Eddc, confirming the successful synthesis of both materials (Figure 1B). Thermogravimetric analysis (TGA) of Zr-Eddc primarily indicated the loss of guest solvent molecules up to 300°C, while RhB@Zr-Eddc lost both guest solvent molecules and RhB dye molecules until 300°C. The frameworks of both materials began to decompose from 550°C, highlighting the highly thermal stability of Zr-Eddc and RhB@Zr-Eddc (Figure 1D and Supplementary Figure S5A). Additionally, the morphology of RhB@Zr-Eddc was more intuitively observed through SEM, revealing generally regular octahedral particles, albeit with some variation in size (Figure 1C).
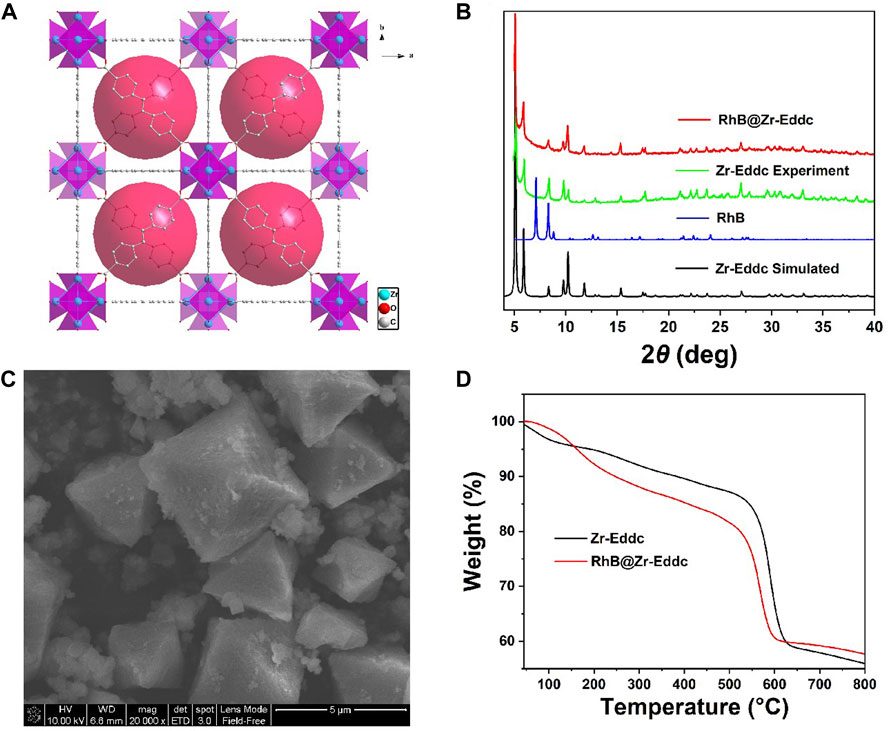
Figure 1. (A) Structure of the RhB@Zr-Eddc; (B) XRD powder diffraction diagram of RhB and RhB@Zr-Eddc; (C) SEM scanning diagram of RhB@Zr-Eddc; (D) Thermogravimetric curve of RhB@Zr-Eddc.
The structure of Zr-Eddc crystallizes in the cubic, and the linear carboxylates (μ2-COO) derived from the di H2Eddc ligand form the a Zr6O4(OH)4(CO2)12 cluster (Zhang et al., 2017). Structure of Zr-Eddc includes two types of polyhedral cages: an octahedral cage with a distance of 24.6 Å and tetrahedral cages with a distance of 17.4 Å (Supplementary Figure S1). The dimensions of the RhB dye molecule, measuring 15.6 Å × 13.5 Å × 4.2 Å (Supplementary Figure S3), are smaller than the nano-cage sizes of Zr-Eddc with the sizes of 24.6 Å and 17.4 Å. Structural analysis (Shen et al., 2022; Shen et al., 2023), as well as XRD data, support the conclusion that RhB dye has been encapsulated into the nano-cage of Zr-Eddc with the RhB dye concentration of 1.15 wt% (Supplementary Figure S4).
2.2 Analysis of the fluorescence properties
Due to RhB@Zr-Eddc was stable in conventional organic solvents and highly stable up to 550°C (Supplementary Figures S5A, B), the fluorescence emission wavelength of liquid-state RhB@Zr-Eddc was tested at room temperature with an excitation wavelength of 360 nm. RhB@Zr-Eddc exhibited a vibrant red-light emission with a maximum peak at 606 nm, primarily attributed to the characteristic emission of the RhB dye. To assess the potential of RhB@Zr-Eddc as a fluorescent probe for the detection of antibiotics, twelve different antibiotics, including furazolidone (FZD), nitrofurazone (NFZ), nitrofurantoin (NFT), ro-nidazole (RDZ), metronidazole (MDZ), dimetridazole (DTZ), ornidazole (ODZ), chlo-ramphenicol (CAP), thiamphenicol (THI), florfenicol (FFC), sulfadiazine (SDZ) and sul-famethazine (SMZ) (Supplementary Figure S6), were subjected to fluorescence detection using the RhB@Zr-Eddc.
5 mg of RhB@Zr-Eddc was immersed in 2 mL of twelve different antibiotic aqueous solutions with a concentration of 10 μg/mL. The mixtures underwent ultrasonic treatment to form suspensions, and their fluorescence spectra were determined under the same conditions. The experimental results revealed varying degrees of quenching effects of antibiotics on RhB@Zr-Eddc with the quenching effect ranking as follows: NFZ > NFT > RDZ > DTZ > FZD > CAP > ODZ > MDZ > THI > FFC > SDZ > SMZ. Notably, the antibiotic NFZ exhibited the most pronounced fluorescence quenching effect (Figures 2A,B).
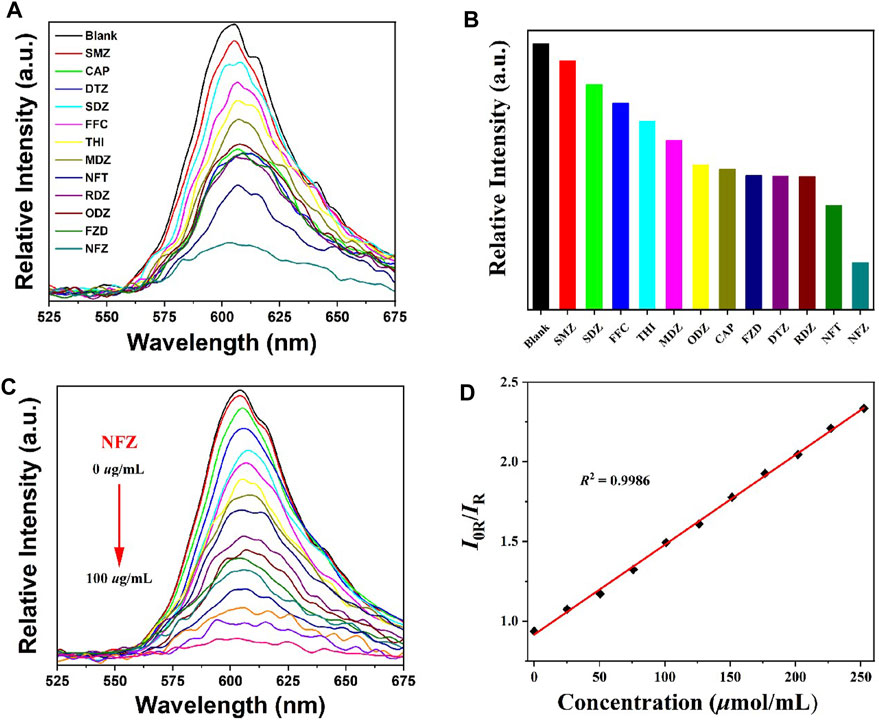
Figure 2. (A) and (B) The emission spectra and relatively intensity of RhB@Zr-Eddc with the different antibiotics; (C) Effects of different concentrations of NFZ on the RhB@Zr-Eddc fluorescence sensing; (D) The linear correlation of (I0/I) vs concentrations of NFZ antibiotic.
To investigate the efficacy of RhB@Zr-Eddc as a fluorescent probe for the NFZ antibiotic, 5 mg of RhB@Zr-Eddc was immersed in 2 mL NFZ aqueous solutions with different concentrations, whose fluorescence spectra were determined under uniform conditions. As the concentration of NFZ antibiotic increased, the fluorescence intensity of RhB@Zr-Eddc was significantly reduced (Figure 2C). At a concentration of 100 μg/mL NFZ, the fluorescence intensity of RhB@Zr-Eddc was nearly completely quenched. According to the Stern–Volmer equation, the quenching constants (Ksv) value of the RhB@Zr-Eddc acted the fluorescent probe for detecting the NFZ antibiotic is 7.08 × 104 M-1 with the range of 0–50 μg/mL (Figure 2D). In addition, according to the equation (LOD = 3σ/KSV, where σ is the standard deviation for eleven repeated luminescent measurements and Ksv is the quenching constants), the limits of detection (LODs) are 0.15 μM, indicating that RhB@Zr-Eddc demonstrates high detection performance for NFZ antibiotic compared with other MOFs (Supplementary Table S1).
To verify the high selectivity of RhB@Zr-Eddc for detecting NFZ antibiotic, 5 mg of RhB@Zr-Eddc was immersed in 2 mL solutions containing of NFZ antibiotic and interfering antibiotics with the concentration of 10 μg/mL, whose fluorescence spectra were then obtained under the same conditions. Experimental results showed a clear quenching effect on the emission intensities of RhB@Zr-Eddc with the addition of an equivalent amount of NFZ antibiotic (Supplementary Figure S7). This suggests that the emission intensities of RhB@Zr-Eddc can be rapidly quenched by NFZ antibiotic in the presence of eleven kinds of interfering antibiotics, indicating high selectivity and resistance to interference in the detection of NFZ antibiotic (Wang et al., 2016; Zhou et al., 2021; Wang et al., 2022; Li et al., 2023; Wang et al., 2023; Wen et al., 2023).
The sensing mechanism of RhB@Zr-Eddc for antibiotics can be attributed to the collision interaction between RhB@Zr-Eddc and antibiotic structures, and this interaction depletes energy transfer and resonance energy transfer (Wang et al., 2016; Cong et al., 2021; Lei et al., 2021; Rani et al., 2022; Su et al., 2022; Zhang et al., 2023; Fan et al., 2024). In summary, these findings indicate that RhB@Zr-Eddc demonstrates highly selective and sensitive detection capabilities for the NFZ antibiotic.
2.3 WLED device
Due to RhB@Zr-Eddc exhibits red-light emission, the prepared samples were utilized to create White Light Emitting Diode (WLED) devices aiming for excellent Correlated Color Temperature (CCT), Color Rendering Index (CRI), and Luminous Efficiency (LE) (Wen et al., 2017; Gutiérrez et al., 2020; Tang et al., 2020; Yuan et al., 2021; Atoini et al., 2023). The RhB@Zr-Eddc samples were combined with a commercial green phosphor (Y3Al5O12:Ce3+, Ga3+) in a mass ratio of 5: 1. The resulting composite phosphors were then applied to the curved surface of a commercial 455 nm blue LED chip to fabricate the WLED device. The WLED device exhibited peaks corresponding to the blue LED chip at 455 nm, the commercial green phosphor (Y3Al5O12:Ce3+, Ga3+) at 510 nm, and the RhB@Zr-Eddc at 612 nm (Figure 3A).
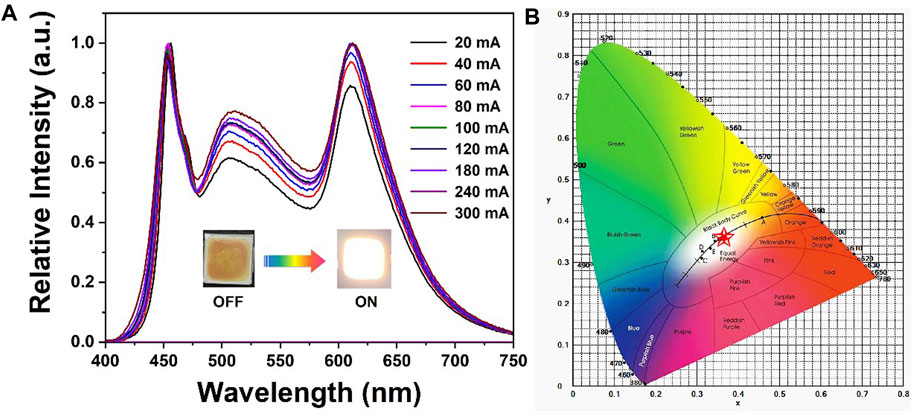
Figure 3. (A) and (B) The fluorescence emission spectra and CIE of WLED device based on the RhB@Zr-Eddc and commercial green phosphor with 455 nm blue LED chip.
Furthermore, as the current increased from 20 mA to 300 mA, the Correlated Color Temperature (CCT) and Color Rendering Index (CRI) values underwent changes. The maximum values for CCT and CRI were determined to be 4710 K and 89.2, respectively (Table 1). Conversely, the Luminous Efficiency (LE) value exhibited a decrease as the current decreased from 20 mA to 300 mA, reaching its maximum value at 43.17 lm/w (Table 1). Additionally, the CIE values were modified from (0.355, 0.353) to (0.354, 0.357) (Figure 3B and Table 1), aligning closely with the standard white light emission values of (0.333, 0.333) (Liu et al., 2019; Karmakar and Li, 2022; Huang et al., 2023; Wu et al., 2024).
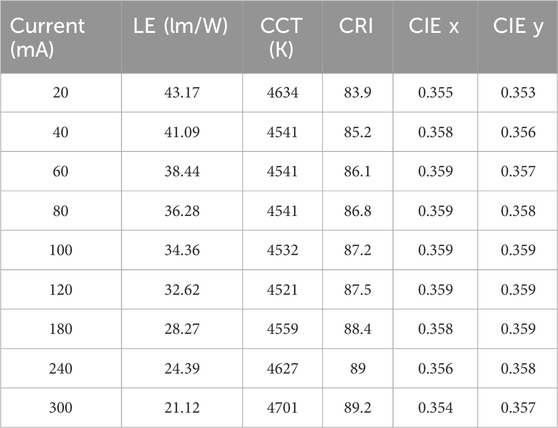
Table 1. The LE, CCT, CRI, and CIE Coordinates of the WLED device based on the RhB@Zr-Eddc and commercial green phosphor with 455 nm blue LED chip.
3 Materials and methods
3.1 Materials
All reagents were obtained commercially and used without further purification. Powder X-ray diffraction (XRD) patterns were recorded using a Desktop X-ray Diffractometer (Ultima-IV, Rigaku). Thermogravimetric experiments were conducted using a TGA/NETZSCH STA449C instrument, heated from 25°C–800°C with a heating rate of 10°C/min in a nitrogen stream. Scanning electron microscopy (JSM6700-F) was employed to characterize the morphology of the samples. Luminescent tests were performed using a PerkinElmer LS55 instrument. The White Light Emitting Diode (WLED) device was measured using a HAAS-2000 High Accuracy Array Spectroradiometer (Everfine) at room temperature under different current densities. The CIE data were computed with the GoCIE program and plotted on the 1931 CIE coordinate diagram.
3.2 Synthesis of RhB@Zr-Eddc
4,4′-Stilbenedicarboxylic acid (H2Eddc) (90 mg), ZrCl4 (70 mg), and rhodamine B (RhB) (100 mg) were combined in a 50 mL polytetrafluoroethylene reaction vessel. Subsequently, 20 mL N, N′-dimethylformamide (DMF) and 2 mL glacial acetic acid were added to the mixture. The resulting blend underwent ultrasonication and was then placed in an oven at 120°C for 50 h. After completion, the reaction vessel was cooled to room temperature. The obtained product was washed three times with DMF and methanol, separated through centrifugation, and dried at 85°C to obtain the RhB@Zr-Eddc.
3.3 Fluorescence sensing
To assess the interaction between RhB@Zr-Eddc and antibiotics, 5 mg of RhB@Zr-Eddc was immersed in 2 mL of twelve different antibiotic aqueous solutions, each with a concentration of 10 μg/mL. The mixtures were subjected to ultrasonic treatment under 20 min to form suspensions, and their fluorescence spectra were determined under the same conditions. Moreover, to specifically explore the effect of nitrofurazone, 5 mg of RhB@Zr-Eddc was immersed in 2 mL of nitrofurazone aqueous solutions with varying concentrations, and their fluorescence spectra were determined under the same conditions. To verify the selectivity of RhB@Zr-Eddc for the detecting NFZ antibiotic, 5 mg of RhB@Zr-Eddc was immersed in 2 mL solutions containing of NFZ antibiotic and interfering antibiotics with the concentration of 10 μg/mL and the volume ratio was 1:1, whose fluorescence spectra were then obtained under the same conditions.
3.4 WLED Encapsulation and determination experiment
The synthesis of White Light Emitting Diode (WLED) devices involved combining the as-synthesized RhB@Zr-Eddc and commercial green phosphor (Y3Al5O12:Ce3+, Ga3+) in a mass ratio of 5: 1. This mixture was then combined with silica gel (Dow Corning, OE6550 A/B) in a powder to glue mass ratio of 1: 1. The resulting composite acted as the phosphor material applied to the curved surface of a commercial 455 nm blue LED chip to create the WLED devices. Subsequently, the WLED devices were heated at 150°C for 2 h. The capabilities of the LED devices were then measured at room temperature under different current densities using the HAAS-2000.
4 Conclusion
Highly thermal stable RhB@Zr-Eddc was synthesized using a one-pot reaction method under hydrothermal conditions. The material was characterized through XRD, SEM, and TGA. RhB@Zr-Eddc demonstrated exceptional thermal stability, enduring temperatures up to 550°C, which also emitted a bright red-light at 605 nm and exhibited high selectivity in detecting nitrofurazone (NFZ) among eleven antibiotics. Additionally, RhB@Zr-Eddc was combined with commercial green phosphor powders and encapsulated onto a 455 nm blue LED chip, resulting in an excellent White Light Emitting Diode (WLED) device. The WLED device exhibited outstanding Correlated Color Temperature (CCT), Color Rendering Index (CRI), and Luminous Efficiency (LE). This suggests that RhB@Zr-Eddc holds potential for use in WLED devices, offering highly selective and sensitive detection capabilities for nitrofurazone antibiotics in future applications.
Data availability statement
The original contributions presented in the study are included in the article/Supplementary Material, further inquiries can be directed to the corresponding authors.
Author contributions
YS: Formal Analysis, Methodology, Writing–original draft. DM: Methodology, Writing–original draft, Investigation, Resources. MZ: Software, Supervision, Validation, Visualization, Writing–review and editing. JQ: Supervision, Writing–review and editing, Project administration. QL: Project administration, Supervision, Writing–review and editing.
Funding
The author(s) declare that financial support was received for the research, authorship, and/or publication of this article. This work was funded by the Special Basic Cooperative Research Programs of Yunnan Provincial Undergraduate Universities’ Association (202101BA070001-031, 202101BA070001-042, and 202301BA070001-093), the National Natural Science Foundation of China (21861044), the National College Student Innovation Training programmer (202210683008 and 201910683001), the Yunnan Province High-level Talent Training Support Program “Youth Top Talent” Project (2020), Yunnan Province Young and Middle-aged Academic and Technical Leaders Reserve Talent Project (202105 AC160060), the Project funded by China Postdoctoral Science Foundation (2018M633426) and the Project funded by the Yunnan Province Postdoctoral Science Foundation (2018).
Conflict of interest
The authors declare that the research was conducted in the absence of any commercial or financial relationships that could be construed as a potential conflict of interest.
Publisher’s note
All claims expressed in this article are solely those of the authors and do not necessarily represent those of their affiliated organizations, or those of the publisher, the editors and the reviewers. Any product that may be evaluated in this article, or claim that may be made by its manufacturer, is not guaranteed or endorsed by the publisher.
Supplementary material
The Supplementary Material for this article can be found online at: https://www.frontiersin.org/articles/10.3389/fchem.2024.1444036/full#supplementary-material
References
Atoini, Y., Cavinato, L. M., Cestau, J. F., Gmach, Y., Opdenbosch, D. V., and Costa, R. D. (2023). From blue to white: sustainable luminescent metal-organic framework for hybrid light-emitting diodes. Adv. Opt. Mater. 11, 2202643. doi:10.1002/adom.202202643
Baaloudj, O., Assadi, I., Nasrallah, N., ElJery, A., Khezami, L., and Assadi, A. A. (2021). Simultaneous removal of antibiotics and inactivation of antibiotic-resistant bacteria by photocatalysis: a review. J. Water Process Eng. 42, 102089. doi:10.1016/j.jwpe.2021.102089
Chen, X. W., Liang, Y. X., and Yu, X. F. (2017). The contamination and treatment technologies for antibiotics in water: a review. Environ. Sci Technol. 40, 125–132. doi:10.3969/i.issn.1003-6504.2017.S1.023
Chen, Y. L., Jiang, C. X., Wang, Y. L., Song, R. R., Tan, Y., Yang, Y. Y., et al. (2022). Sources, environmental fate, and ecological risks of antibiotics in sediments of Asia’s Longest River: a whole-basin investigation. Environ. Sci. Technol. 56, 14439–14451. doi:10.1021/acs.est.2c03413
Cong, Z. Z., Song, Z. F., Ma, Y. X., Zhu, M. C., Zhang, Y., Wu, S. Y., et al. (2021). Highly emissive metal-organic frameworks for sensitive and selective detection of nitrofuran and quinolone antibiotics. Chem-an Asian J. 16, 1773–1779. doi:10.1002/asia.202100352
Divysnshi, M., Ikram, S., Atul, S., and Saiqa, I. (2022). Critical review on adsorptive removal of antibiotics: present situation, challenges and future perspective. J. Hazard. Mater. 425, 127946. doi:10.1016/j.jhazmat.2021.127946
Duan, L. J., Zhang, C. C., Cen, P. P., Jin, X. Y., Liang, C., Yang, J. H., et al. (2020). Stable Ln-MOFs as multi-responsive photoluminescence sensors for the sensitive sensing of Fe3+, Cr2O72−, and nitrofuran. CrystEngComm. 22, 1695–1704. doi:10.1039/c9ce01995b
Fan, X. F., Deng, X. C., Cao, Q. L., Dong, G. Y., and Fu, L. S. (2024). Two robust dual-functional Cd(II) MOFs as luminescent sensors for quantitatively detection of nitrofurazone and Fe3+ ions. J. Mol. Struct. 1295, 136757. doi:10.1016/j.molstruc.2023.136757
Gutiérrez, M., Martín, C., Auweraer, M. V., Hofkens, J., and Tan, J. C. (2020). Electroluminescent guest@MOF nanoparticles for thin film optoelectronics and solid-state lighting. Adv. Opt. Mater. 8 (16), 2000670. doi:10.1002/adom.202000670
Heng, Y., Fang, Z., Li, J., Luo, L. Q., Zheng, M. Z., and Huang, H. L. (2023). Defective metal-organic framework derived from the waste plastic bottles for rapid and efficient nitroimidazole antibiotics removal. J. Colloid Interface Sci. 650, 836–845. doi:10.1016/j.jcis.2023.07.049
Hou, S. L., Dong, J., Jiang, X. L., Jiao, Z. H., Wang, C. M., and Zhao, B. (2018). Interpenetration-dependent luminescent probe in indium organic frameworks for selectively detecting nitrofurazone in water. Anal. Chem. 90, 1516–1519. doi:10.1021/acs.analchem.7b05088
Huang, M. Y., Liang, Z. X., Huang, J. L., Wen, Y. H., Zhu, Q. L., and Wu, X. T. (2023). Introduction of multicomponent dyes into 2D MOFs: a strategy to fabricate white light-emitting mof composite nanosheets. ACS Appl. Mater. Interfaces. 15, 11131–11140. doi:10.1021/acsami.2c22568
Hutchings, I. M., Truman, A. W., and Wilkinson, B. (2019). Antibiotics: past, present and future. Curr. Opin. Microbiol. 51, 72–80. doi:10.1016/j.mib.2019.10.008
Karmakar, A., and Li, J. (2022). Luminescent MOFs (LMOFs): recent advancement towards a greener WLED technology. Chem. Commun. 58, 10768–10788. doi:10.1039/d2cc03330e
Kovalakova, P., Cizmas, L., Mcdonald, T. J., Marsalek, B., Feng, M. B., and Sharma, V. (2020). Occurrence and toxicity of antibiotics in the aquatic environment: a review. Chemosphere 251, 126351. doi:10.1016/j.chemosphere.2020.126351
Lei, N. N., Li, W. C., Zhao, D. S., Li, W. Q., Liu, X., Liu, L. Y., et al. (2024). A bifunctional luminescence sensor for biomarkers detection in serum and urine based on chemorobust Nickel(II) metal-organic framework. Spectrochim. Acta A. 306, 123585. doi:10.1016/j.saa.2023.123585
Lei, Z., Hu, L., Yu, Z. H., Yao, Q. Y., Chen, X., Li, H., et al. (2021). Ancillary ligand enabled structural and fluorescence diversity in metal-organic frameworks: application for the ultra-sensitive detection of nitrofuran antibiotics. Inorg. Chem. Front. 8, 1290–1296. doi:10.1039/d0qi01098g
Li, G., Yan, T. G., Zhao, H. F., and Liu, D. H. (2023). Effective removal of nitroimidazole antibiotics in aqueous solution by an aluminum-based metal-organic framework: performance and mechanistic studies. J. Solid. State. Chem. 317, 123659. doi:10.1016/j.jssc.2022.123659
Liu, X. Y., Xing, K., Li, Y., Tsung, C. K., and Li, J. (2019). Three models to encapsulate multicomponent dyes into nanocrystal pores: a new strategy for generating high-quality white light. J. Am. Chem. Soc. 141 (37), 14807–14813. doi:10.1021/jacs.9b07236
Luo, D. W., Huang, J. F., Jian, Y. H., Sing, A., Kumar, A., Liu, J. Q., et al. (2023). Metal–organic frameworks (MOFs) as apt luminescent probes for the detection of biochemical analytes. J. Mater. Chem. B 11, 6802–6822. doi:10.1039/d3tb00505d
Lyu, J., Yang, L. S., Zhang, L., Ye, B. X., and Wang, L. (2020). Antibiotics in soil and water in China–a systematic review and source analysis. Environ. Pollut. 266, 115147. doi:10.1016/j.envpol.2020.115147
Pepper, I. L., Brooks, J. P., and Gerba, C. P. (2018). Antibiotic resistant bacteria in municipal wastes: is there reason for concern? Environ. Sci. Technol. 52 (7), 3949–3959. doi:10.1021/acs.est.7b04360
Rani, R., Deep, A., Mizaikoff, B., and Singh, S. (2022). Zirconium metal organic framework based opto-electrochemical sensor for nitrofurazone detection. J. Electroanal. Chem. 909, 116124. doi:10.1016/j.jelechem.2022.116124
Saboor, F. H., Nasirpour, N., Shahsavari, S., and Kazemian, H. (2022). The effectiveness of MOFs for the removal of pharmaceuticals from aquatic environments: a review focused on antibiotics removal. Chem-Asian J. 17, e202101105. doi:10.1002/asia.202101105
Shen, Y. Q., Geng, Y. C., Wang, J. B., Fan, X., and Li, Q. P. (2022). Preparation of r6g@γ-CD-MOFs composites with application in sensing of Fe3+ ion. Chin. J. Inorg. Chem. 38, 800–804. doi:10.11862/CJIC.2022.100
Shen, Y. Q., Ma, J., Li, S. J., Qian, J. J., and Li, Q. P. (2023). Highly luminescent and thermostable r6g@dut-52 composite for sensing Fe3+ ion and white light emitting diode. Chem. Eng. J. 466, 143290. doi:10.1016/j.cej.2023.143290
Su, P. C., Zhang, A. R., Yu, L., Ge, H. W., Wang, N., Huang, S. Y., et al. (2022). Dual-functional UiO-type metal-organic frameworks for the sensitive sensing and effective removal of nitrofurans from water. Sensors Actuators B Chem. 350, 130865. doi:10.1016/j.snb.2021.130865
Svetlana, I. P., Anca, E. G., Bela, K., Maria, G. Ş., and Felicia, L. (2020). Antibiotics in the environment: causes and consequences. Med. Pharm. Rep. 93 (3), 231–240. doi:10.15386/mpr-1742
Tang, Y., Wu, H. L., Cao, W. Q., Cui, Y. J., and Qian, G. D. (2020). Luminescent metal-organic frameworks for white LEDs. Adv. Opt. Mater. 9, 2001817. doi:10.1002/adom.202001817
Wang, B., Lv, X. L., Feng, D. W., Xie, L. H., Zhang, J., Li, M., et al. (2016). Highly stable Zr(IV)-based metal-organic frameworks for the detection and removal of antibiotics and organic explosives in water. J. Am. Chem. Soc. 138 (19), 6204–6216. doi:10.1021/jacs.6b01663
Wang, C. Y., Wang, C. C., Zhang, X. W., Ren, X. Y., Yu, B. Y., Wang, P., et al. (2022). A new Eu-MOF for ratiometrically fluorescent detection toward quinolone antibiotics and selective detection toward tetracycline antibiotics. Chin. Chem. Lett. 33, 1353–1357. doi:10.1016/j.cclet.2021.08.095
Wang, K. M., Bai, X. L., Zhao, X., Dong, Y. Q., Zhao, R. T., Zhou, J., et al. (2023). Highly sensitive fluorescence detection of nitrofurazone and nitrofurantoin in milk and honey using a hydrostable Cd(Ⅱ) metal-organic framework. J. Mol. Struct. 1295, 136114. doi:10.1016/j.molstruc.2023.136114
Wang, W. Z., Yang, F., Yang, Y. C., Wang, Y. Y., and Liu, B. (2022). Rational synthesis of a stable rod MOF for ultrasensitive detection of nitenpyram and nitrofurazone in natural water systems. J. Agric. Food Chem. 70, 15682–15692. doi:10.1021/acs.jafc.2c05780
Wen, M. Y., Fu, L. S., and Dong, G. Y. (2023). Two Cd(II)-MOFs containing pyridylbenzimidazole ligands as fluorescence sensors for sensing enrofloxacin, nitrofurazone and Fe3+. J. Mol. Struct. 1285, 135488. doi:10.1016/j.molstruc.2023.135488
Wen, Y. H., Sheng, T. L., Zhu, X. Q., Zhuo, C., Su, S. D., Li, H. R., et al. (2017). Introduction of red-green-blue fluorescent dyes into a metal-organic framework for tunable white light emission. Adv. Mater. 29, 1700778. doi:10.1002/adma.201700778
Wu, H. L., Tang, Y., Cui, Y. J., and Qian, G. D. (2024). Multi-luminescent center integrated metal organic frameworks for high-performance white light-emitting diodes. J. Mater. Chem. C 12, 1047–1054. doi:10.1039/d3tc03610c
Wu, X. Y., Gao, Y. X., and Bi, J. H. (2024). Understanding the structure-dependent adsorption behavior of four zirconium-based porphyrinic MOFs for the removal of pharmaceuticals. Micropor. Mesopor. Mat. 363, 112827. doi:10.1016/j.micromeso.2023.112827
Yin, J., Li, W., Li, W., Liu, L., Zhao, D., Liu, X., et al. (2023). Heterometallic ZnHoMOF as a dual-responsive luminescence sensor for efficient detection of hippuric acid biomarker and nitrofuran antibiotics. Molecules 28, 6274. doi:10.3390/molecules28176274
Yuan, Q. L., Wang, T., Yu, P. L., Zhang, H. Z., Zhang, H. J., and Wen, Y. J. (2021). A review on the electroluminescence properties of quantum-dot light-emitting diodes. Org. Electron. 90, 106086. doi:10.1016/j.orgel.2021.106086
Zhang, J., Yao, S., Liu, S., Liu, B., Sun, X. D., Zheng, B., et al. (2017). Enhancement of gas sorption and separation performance via ligand functionalization within highly stable zirconium-based metal-organic frameworks. Cryst. Growth Des. 17 (4), 2131–2139. doi:10.1021/acs.cgd.7b00090
Zhang, Q. Q., Ying, G. G., Pan, C. G., Liu, Y. S., and Zhao, J. L. (2015). Comprehensive evaluation of antibiotics emission and fate in the river basins of China: source analysis, multimedia modeling, and linkage to bacterial resistance. Environ. Sci. Technol. 49 (11), 6772–6782. doi:10.1021/acs.est.5b00729
Zhang, X. P., Fu, L. S., Blatova, O. A., and Cui, G. H. (2023). Four metal-organic frameworks for the sensing of oxytetracycline/nitrofurazone and Fe3+ ions. J. Solid State Chem. 324, 124124. doi:10.1016/j.jssc.2023.124124
Zhang, Z. X., Zhang, H. Y., Tian, D. H., Phan, A., Seididamyeh, M., Alanazi, M., et al. (2024). Luminescent sensors for residual antibiotics detection in food: recent advances and perspectives. Coord. Chem. Rev. 498, 215455. doi:10.1016/j.ccr.2023.215455
Zhao, Y., Wang, Y. J., Wang, N., Zheng, P., Fu, H. R., Han, M. L., et al. (2019). Tetraphenylethylene-decorated metal-organic frameworks as energy-transfer platform for the detection of nitro-antibiotics and white-light emission. Inorg. Chem. 58, 12700–12706. doi:10.1021/acs.inorgchem.9b01588
Zhong, Y. Y., Peng, Z. X., Peng, Y. Q., Li, B., Pan, Y., Ouyang, Q., et al. (2023). Construction of Fe-doped ZIF-8/DOX nanocomposites for ferroptosis strategy in the treatment of breast cancer. J. Mater. Chem. B 11, 6335–6345. doi:10.1039/d3tb00749a
Zhou, S. H., Lu, L., Liu, D., Wang, J., Sakiyama, H., Muddassir, M., et al. (2021). Series of highly stable Cd(ii)-based MOFs as sensitive and selective sensors for detection of nitrofuran antibiotic. CrystEngComm. 23, 8043–8052. doi:10.1039/d1ce01264a
Zhou, Y., Yang, Q., Zhang, D. N., Gan, N., Li, Q. P., and Cuan, J. (2018). Detection and removal of antibiotic tetracycline in water with a highly stable luminescent MOF. Sensors Actuators B 262, 137–143. doi:10.1016/j.snb.2018.01.218
Zhu, X. D., Zhang, K., Wang, Y., Long, W. W., Sa, R. J., Liu, T. F., et al. (2018). Fluorescent metal-organic framework (MOF) as a highly sensitive and quickly responsive chemical sensor for the detection of antibiotics in simulated wastewater. Inorg. Chem. 57 (3), 1060–1065. doi:10.1021/acs.inorgchem.7b02471
Keywords: RhB@Zr-Eddc, highly thermostable, nitrofurazone, selective detection, WLED
Citation: Shen Y, Ma D, Zhao M, Qian J and Li Q (2024) Highly thermostable RhB@Zr-Eddc for the selective sensing of nitrofurazone and efficient white light emitting diode. Front. Chem. 12:1444036. doi: 10.3389/fchem.2024.1444036
Received: 05 June 2024; Accepted: 24 July 2024;
Published: 02 August 2024.
Edited by:
Xuan Zhang, Donghua University, ChinaReviewed by:
Lei Liu, Harvard Medical School, United StatesZheng Wang, Shaanxi University of Science and Technology, China
Copyright © 2024 Shen, Ma, Zhao, Qian and Li. This is an open-access article distributed under the terms of the Creative Commons Attribution License (CC BY). The use, distribution or reproduction in other forums is permitted, provided the original author(s) and the copyright owner(s) are credited and that the original publication in this journal is cited, in accordance with accepted academic practice. No use, distribution or reproduction is permitted which does not comply with these terms.
*Correspondence: Mian Zhao, MTg2MDE1MjlAaGVibXUuZWR1LmNu; Jinjie Qian, amluamllcWlhbkB3enUuZWR1LmNu; Qipeng Li, cXBsaUB6dHUuZWR1LmNu