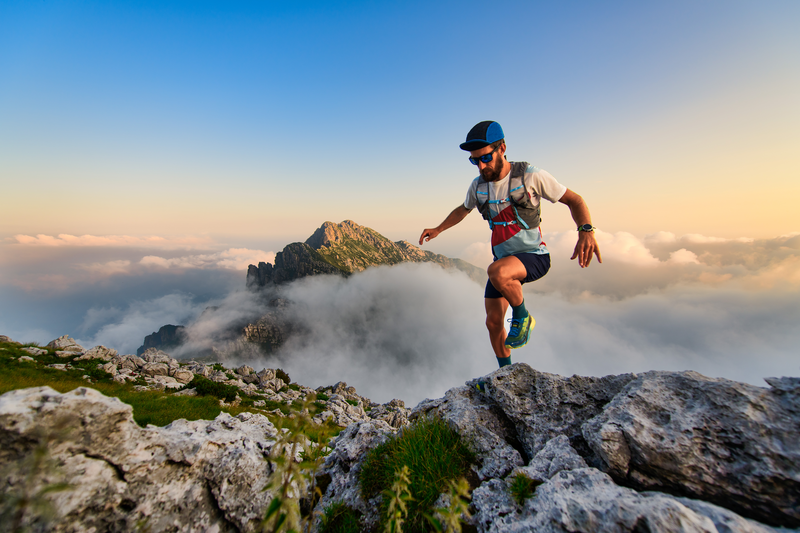
94% of researchers rate our articles as excellent or good
Learn more about the work of our research integrity team to safeguard the quality of each article we publish.
Find out more
MINI REVIEW article
Front. Chem. , 21 August 2024
Sec. Polymer Chemistry
Volume 12 - 2024 | https://doi.org/10.3389/fchem.2024.1441650
This article is part of the Research Topic Polymer Functional Composite View all articles
Plant-based materials and edible films have emerged as promising alternatives to conventional packaging materials, offering sustainable and environmentally friendly solutions. This mini-review highlights the significance of plant-based materials derived from polysaccharides, proteins, and lipids, showcasing their renewable and biodegradable nature. The properties of edible films, including mechanical strength, barrier properties, optical characteristics, thermal stability, and shelf-life extension, are explored, showcasing their suitability for food packaging and other applications. Moreover, the application of 3D printing technology allows for customized designs and complex geometries, paving the way for personalized nutrition. Functionalization strategies, such as active and intelligent packaging, incorporation of bioactive compounds, and antimicrobial properties, are also discussed, offering additional functionalities and benefits. Challenges and future directions are identified, emphasizing the importance of sustainability, scalability, regulation, and performance optimization. The potential impact of plant-based materials and edible films is highlighted, ranging from reducing reliance on fossil fuels to mitigating plastic waste and promoting a circular economy. In conclusion, plant-based materials and edible films hold great potential in revolutionizing the packaging industry, offering sustainable alternatives to conventional materials. Embracing these innovations will contribute to reducing plastic waste, promoting a circular economy, and creating a sustainable and resilient planet.
The use of plant-based materials and edible films can be traced back several decades. In the early 20th century, natural polymers, such as cellulose, were first recognized for their potential in packaging applications. However, it was not until the 1970s and 1980s that significant advancements were made in the development of plant-based materials and edible films (Teixeira-Costa and Andrade, 2021). During this period, researchers began exploring the use of various polysaccharides, proteins, and lipids derived from plants to create films with desirable properties for packaging purposes (Zubair et al., 2022).
Polysaccharides such as cellulose, starch, chitosan, and alginate gained attention due to their abundance, low cost, biodegradability, and film-forming abilities (Cazon et al., 2017). Proteins such as soy protein and wheat gluten were also investigated for their film-forming properties and potential applications in food packaging (Fu et al., 2022; Hadidi et al., 2022). Advancements in processing techniques, such as casting, compression molding, and extrusion, allowed for the production of plant-based films with improved mechanical and barrier properties (Hadidi et al., 2022). These films exhibited promising characteristics, including biocompatibility, flexibility, and resistance to moisture and oxygen (Wang et al., 2021).
In recent years, the focus on plant-based materials and edible films has intensified due to increased awareness of sustainability and environmental issues. Researchers and industry stakeholders have been actively exploring innovative approaches to enhance the properties and applications of plant-based materials, as well as to develop edible films with improved functionality.
There has been a growing global concern regarding the environmental impact of traditional packaging materials, such as plastics, which are non-biodegradable and contribute to pollution and waste accumulation (Kumar et al., 2021). This concern has led to a significant shift towards the development and utilization of sustainable packaging materials. Sustainable packaging materials aim to minimize environmental harm by utilizing renewable resources, reducing carbon footprint, and promoting biodegradability (Rai et al., 2021).
Among the various types of sustainable packaging materials, plant-based materials and edible films have emerged as promising alternatives. Plant-based materials are derived from renewable resources such as crops, fruits, and vegetables, making them environmentally friendly (Zuin et al., 2018). Edible films, on the other hand, are thin, edible layers that can be applied to food surfaces, providing an additional protective barrier while also being safe for consumption (Falguera et al., 2011).
By examining the advances and recent trends in plant-based materials and edible films, this comprehensive review provides valuable insights into the current state of research in this field. It highlights the potential of plant-based materials as sustainable alternatives to synthetic packaging and outlines the various production methods, properties, applications, and emerging technologies associated with edible films. The review also addresses the challenges and future directions, offering a roadmap for further research and development in this rapidly evolving area. The next sections of this review paper will delve into the advancements and recent trends in plant-based materials and edible films, including the production methods, properties, applications, and emerging technologies. Figure 1 below shows the overview of recent trends and advances in plant-based materials.
The widespread use of petrochemical-based plastics, particularly in packaging applications, is a significant contributor to environmental pollution. In the mid-1900s, only about 2 million tonnes were produced globally, but as of today, over 450 million tonnes are produced yearly (Ritchie et al., 2023). This is primarily due to its cheap production cost and versatility (flexible and lightweight), making it functional for industrial applications for a host of goods and services (Zimmermann et al., 2020). Its entire lifecycle (manufacturing, utilization, and disposal) contributes just under 2 billion tonnes of carbon emissions yearly (Waste and Resources Action Programme, 2024). In addition to this, around 42% of all petrochemical-based plastics used end up in landfills, and barely a 10th is recycled (Zhao et al., 2020; Lin et al., 2023). In global economic powers like the United States and China, around 60%–85% of plastic waste generated in municipalities is sent to landfills (Lin et al., 2023). The extensive production, utilization, and improper disposal of this form of plastic have resulted in global microplastic pollution, destruction of the aquatic ecosystem, and suspected morbidity (infertility and endocrine disruption) among humans (Daltry et al., 2021; Ullah et al., 2022; Chamanee et al., 2023). They are also potential sources of harmful chemicals like per- and poly-fluoroalkyl substances (PFAS) in the environment (Sangkham, 2024).
On the other hand, plant-based biofilms seem to offer more beneficial purposes, particularly in food packaging. For example, polysaccharide biofilms like alginate have been reported to enhance the shelf life of meat and meat products by regulating permeability and restricting dehydration (Kim et al., 2018). Chitosan biofilms have preserved food quality and extended shelf life by effectively limiting oxygen and carbon dioxide transfer through the coating (Casariego et al., 2009). Others have been reported to improve food shelf life via antimicrobial properties and by increasing oxidation stability (Heydari et al., 2015; Chang et al., 2023). In addition, biopolymers have also been employed to produce antimicrobial edible coatings. In one study, soft cheese covered with biofilm prepared using chitosan embedded with antimicrobial microcrystalline cellulose and probiotic organisms for 45 days had a high antimicrobial effect against a wide range of organisms (El-Sayed et al., 2021). The stability and sensory attributes of the cheese were also reasonably maintained throughout the duration (El-Sayed et al., 2021). However, such biopolymers face limitations in structural integrity. In comparison to petrochemical-based plastics, they have reduced impact strength, lower tensile elongation, and an increased flexural modulus (Haider et al., 2019; Filho et al., 2022). For example, Young’s modulus, tensile strength, and elongation at break for the fossil-based PP are 1,100–1,500 MPa, 31–43 MPa, and 500%–650%, while respective values for gelatin are 22 MPa, 9 MPa, and 45% (Nilsen-Nygaard et al., 2021). In addition, the water vapor transmission rate for PP was reported to be between 0.1 and 0.3, while that of cellulose acetate was reportedly 257.8 (Nilsen-Nygaard et al., 2021). Plant-based biofilms also have significantly higher permeation and water absorption characteristics (Gupta P. et al., 2022). This significantly limits the application of plant-based biofilms. Some polysaccharides (amylose starch and chitosan), however, have proven to possess comparative gas (oxygen and carbon dioxide) barrier properties and tensile strength values when compared to high-density polyethene films (Cazon et al., 2017). Thus, future research aims to explore possible modifications that can be made to improve the mechanical properties of plant-based biopolymers.
Considering the environmental implications of both plastic sources, Life cycle assessments (LCAs) indicate that bioplastics generally consume fewer non-renewable resources and emit fewer greenhouse gases compared to conventional plastics, particularly when biomass energy is utilized in their production (Kurdikar et al., 2000). However, the environmental benefits of bioplastics are not without caveats. The intensive agriculture required for bioplastic production, involving the use of pesticides, herbicides, and fertilizers, can negatively impact ecosystem quality, leading to issues such as acidification and eutrophication (Gironi and Piemonte, 2011). Despite these concerns, the overall benefits of plant-based materials in food packaging are substantial. They enhance food shelf life and prevent spoilage, reducing food waste and associated environmental impacts. Additionally, plant-based biofilms help prevent the proliferation of microplastics and reduce landfill burdens due to their compostable nature (Zhao et al., 2020). In contrast, the majority of petrochemical-based plastics end up in landfills and litter in the environment, where they persist for hundreds of years, exacerbating waste management issues and contributing to environmental degradation (Lin et al., 2023; Trinh et al., 2023). However, with a wide spectrum of biofilms emerging, more LCA studies need to be conducted to determine their precise environmental impact and compare them with conventional processes (Versino et al., 2023). Table 1 provides a comprehensive comparison between plant-based and traditional petrochemical-based packaging materials.
Polysaccharides are abundant carbohydrates found in plants and offer a wide range of properties that make them suitable for the development of plant-based materials and edible films. Some of the notable polysaccharides used in this context include cellulose and its derivatives, starch, chitosan, and alginate. Cellulose is the most abundant biopolymer on Earth and is derived from plant cell walls. It possesses excellent mechanical strength, thermal stability, and barrier properties (Liu et al., 2021). However, its insolubility in water limits its direct use in film formation (Xu et al., 2016). To overcome this, cellulose derivatives such as methylcellulose, carboxymethylcellulose, and hydroxypropyl cellulose are often employed (Acharya et al., 2021). These derivatives exhibit improved solubility and film-forming capabilities while retaining some of the cellulose’s desirable properties. Recent studies have also reported that acetylation of polysaccharides could improve their solubility properties (Yilmaz-Turan et al., 2020). In one study, acetylated xylan from sugarcane bagasse had significantly higher water vapor barrier and oil-resistant properties (Martins et al., 2024). Acetylation was performed using starch as blends and glycerol as a plasticizer at varying degrees of substitution (Martins et al., 2024).
Starch is another widely available polysaccharide obtained from various plant sources, primarily corn, potato, and wheat, and is highly favored due to its natural abundance and low cost (Matheus et al., 2023). It offers film-forming properties, biodegradability, and good oxygen barrier capabilities (Cheng et al., 2021). Starch-based materials can be modified through processes like plasticization, cross-linking, or blending with other polymers to enhance their mechanical strength, water resistance, and stability (Amaraweera et al., 2021). Additionally, the use of nanotechnology has enabled the development of nanocomposite films with improved properties.
Chitosan is derived from chitin, a polysaccharide found in the exoskeletons of crustaceans and cell walls of fungi. It possesses antimicrobial properties, good film-forming ability, and biodegradability (Priyadarshi and Rhim, 2020). Chitosan-based films have shown promise in various applications, including food packaging, due to their ability to extend the shelf life of perishable products by inhibiting microbial growth and maintaining product quality (Khubiev et al., 2023). Alginate is extracted from brown seaweeds and has been extensively studied for its film-forming properties. Alginate-based films are highly biodegradable, possess good water vapor barrier properties, and can be tailored to exhibit controlled release characteristics (Khorrami et al., 2021). These films find applications in food packaging, wound dressing, and pharmaceutical delivery systems. A specific example of such an application was in a recent study that developed an edible bioplastic film with increased strength and toughness by crosslinking composite films (soybean protein isolate and chitosan) with citric acid (Zhang et al., 2023). This packaging resulted in promising results like delaying fruit ripeness by about 5 days, maintaining the edible rate of cherries and strawberries by 75% 5–7 days post storage, and preventing meat spoilage via chemical inhibition and antibacterial properties (Zhang et al., 2023).
Proteins derived from plant sources have gained attention as potential alternatives for plant-based materials and edible films. Soy protein, wheat gluten, casein, and other plant-based proteins are commonly explored in this regard. Soy protein isolates and concentrates are obtained from soybeans and offer a range of functional properties suitable for film formation. Soy protein-based films possess good mechanical strength, flexibility, and oxygen barrier properties (Wu et al., 2019). They can also be modified by blending with other polymers, cross-linking, or incorporating additives to enhance their properties and expand their applications. A specific example of cross-linking can be found in a recent study that co-crosslinked keratin and gluten to improve the hydrophilic characteristics of the bioplastic film, among other physicochemical properties (Alshehhi et al., 2024). Specifically, the cross-linked films had better chain mobility and higher water uptake (by about 85%) (Alshehhi et al., 2024). In another study, the incorporation of by-products of Spanish Mackerel and pectin from passion fruit resulted in bioplastic with increased strength, flexibility, biodegradability, and water vapor permeability (Florentino et al., 2022).
Wheat gluten is a byproduct of wheat processing and has received considerable attention as a sustainable film-forming material. It exhibits excellent film-forming ability, biodegradability, and gas barrier properties (Zhang et al., 2022). Wheat gluten films have been explored for food packaging applications, offering a renewable and biodegradable alternative to petroleum-based plastics. Casein is a milk protein that can form films with excellent mechanical properties, water vapor barrier properties, and transparency (Karaca et al., 2019). Casein-based films are extensively used in food packaging due to their biodegradability, good oxygen barrier capabilities, and ability to inhibit moisture transfer (Chaudhary et al., 2022). Additionally, casein films can be modified by incorporating antimicrobial agents or incorporating nanoparticles to enhance their functionality (Khan et al., 2021).
Apart from soy protein, wheat gluten, and casein, various other plant-based proteins, such as pea protein, corn zein, and hemp protein, have been explored for their potential in developing plant-based materials and edible films. These proteins offer diverse properties and functionalities, such as film-forming ability, water resistance, and mechanical strength. The development of films from these proteins involves processes such as solubilization, extraction, and film casting, which can be optimized to achieve desired film properties. A recent study has reported an industrial proof of concept by producing bioplastic films from tofu manufacturing protein-rich byproducts (Bagnani et al., 2024). Around 0.5 m3 of soy whey was converted into 27 kg of bioplastic films by forming protein nanofibrils with okara using methylcellulose and glycerol (Bagnani et al., 2024).
Lipids derived from plant sources have also shown promise in the development of plant-based materials and edible films. Plant-based oils and fats, as well as wax-based materials, are notable examples in this category. Plant-based oils and fats, such as vegetable oils derived from soybean, corn, sunflower, or palm, can be utilized in the formulation of edible films. These films can exhibit good water vapor barrier properties, flexibility, and biodegradability (Zubair et al., 2021). For example, a study that formulated a film-forming emulsion with a hybrid of chitosan and oleic acid reported the lipid-limited transference of water vapor in the film (Aguirre-Loredo et al., 2014). By incorporating additives or modifying the lipid composition, properties such as mechanical strength and oxygen barrier performance can be improved.
Natural waxes, such as beeswax, carnauba wax, and candelilla wax, obtained from plant sources, have been explored for their potential in edible film development. These waxes offer desirable properties such as hydrophobicity, water vapor resistance, and film-forming capabilities (Saji, 2020). Wax-based films can be used as coatings on food surfaces to enhance their shelf life and preserve freshness (Singh et al., 2016). Additionally, blends of waxes with other biopolymers can be used to improve film properties. A specific example of using lipids in bioplastics for shelf-life enhancement was reported in a recent study that compounded essential oils (such as citral, carvacrol and α-terpineol) with blended films (Laorenza and Harnkarnsujarit, 2021). The addition of the essential oils improved the antimicrobial properties of the biofilm, prevented melanosis, and delayed spoilage of Pacific white shrimp (Laorenza and Harnkarnsujarit, 2021).
The selection of specific plant-based materials depends on their availability, functional properties, and compatibility with the desired application. Moreover, combinations of different plant-based materials, such as blending polysaccharides with proteins or lipids, can result in composite films with enhanced properties, offering a wide range of possibilities for sustainable packaging and edible film development. Figure 2 below shows plant-based sources for edible films.
Edible films and coatings are created from food-grade additives and biopolymers, forming thin layers that act as barriers to moisture, oxygen, and solute transfer in food products (Han, 2014; Díaz-Montes and Castro-Muñoz, 2021). These films can be used as coatings directly on food or as standalone films, gaining attention for their benefits over synthetic alternatives, such as being consumable along with the packaged product and reducing environmental pollution (Díaz-Montes and Castro-Muñoz, 2021). Recent research has concentrated on producing these films using agricultural by-products and food industry waste, integrating additives like antimicrobials, antioxidants, nutraceuticals, and flavourings to safeguard food from spoilage and pathogens, thereby improving food safety and quality (Campos et al., 2011; Díaz-Montes and Castro-Muñoz, 2021). The different types of edible films, including their applications and results, are presented in Table 2 below.
Edible films can be produced using various techniques, depending on the desired properties and application requirements. Figure 3 below illustrates different methods for producing edible films, showing the relationship between the methods, materials and unique characteristics. Some commonly employed production methods include:
Casting: Casting involves the preparation of a film-forming solution or dispersion, which is then poured onto a flat surface or mold and allowed to dry or solidify (Siqueira et al., 2021). This method enables the formation of uniform, thin films with controllable thickness (Suhag et al., 2020). Casting is suitable for films made from polysaccharides, proteins, and lipids, as well as their combinations.
Compression Molding: Compression molding involves applying heat and pressure to a mixture or powder to form a solid film (Tatara, 2024). This method is commonly used for thermoplastic materials, such as starch-based films, where the material is heated and compressed in a mold to achieve the desired shape and thickness (Yu et al., 2021). Compression molding allows for the production of films with good mechanical strength and barrier properties.
Extrusion: Extrusion is a widely used technique for producing continuous films from melt-processed materials. The process involves melting the film-forming material and forcing it through a die to form a continuous sheet. Extrusion allows for precise control of film thickness and facilitates the production of films with complex shapes (Shim, 2013). This method is particularly suitable for thermoplastic materials, including starch, proteins, and synthetic polymers.
Spray Coating: Spray coating involves the deposition of a film-forming solution or suspension onto a substrate using a spray system (Suhag et al., 2020). This method allows for the formation of thin, uniform films by spraying the solution in a controlled manner. Spray coating is commonly used for the production of edible films on irregularly shaped or delicate food surfaces (Bizymis and Tzia, 2021).
Edible films possess a range of properties that make them suitable for various applications. The key properties of edible films include:
Mechanical Properties: The mechanical properties of edible films, such as tensile strength, flexibility, and elasticity, are crucial for their performance as packaging materials (Shah et al., 2023). These properties determine the film’s ability to withstand handling, deformation, and stress during storage and transportation.
Barrier Properties: Edible films act as a barrier against moisture, oxygen, light, and other gases, protecting the packaged product from deterioration (Kaewprachu and Rawdkuen, 2016). Barrier properties, including water vapor transmission rate (WVTR), oxygen permeability, and light transmission, are important considerations for the selection and development of edible films for specific applications (Jeevahan et al., 2020).
Optical Properties: Optical properties, such as transparency, color, and gloss, play a significant role in the visual appeal of packaged products (Kim et al., 2014). Transparent and glossy films enhance product visibility, while color additives can be incorporated to impart a desired appearance.
Thermal Properties: Thermal properties, including glass transition temperature (Tg), melting point, and thermal stability, are essential for determining the temperature resistance and processing conditions of edible films (Gounga et al., 2010). These properties impact the film’s behavior during storage, cooking, and other food processing operations.
Shelf-Life Extension Properties: Edible films can possess properties that extend the shelf life of perishable products (Kaewprachu and Rawdkuen, 2016). For example, incorporating antimicrobial agents or antioxidants into the film matrix can help inhibit microbial growth or oxidative reactions, respectively, thereby preserving product quality and freshness.
Edible films find diverse applications in various industries, including:
Food Packaging and Preservation: Edible films offer an eco-friendly alternative to traditional plastic packaging materials (Petkoska et al., 2021). They can be used to wrap fresh fruits and vegetables, meat, dairy products, snacks, and bakery items, providing a protective barrier against moisture loss, oxygen, and microbial contamination (Mondal et al., 2021). Edible films can also incorporate active ingredients, such as antimicrobial agents or flavoring compounds, to further enhance food safety and extend shelf life.
Biomedical and Pharmaceutical Applications: Edible films have gained attention in the biomedical and pharmaceutical fields. They can be used as drug delivery systems, where medications are encapsulated within the film matrix, allowing for controlled release and targeted delivery (Zhang et al., 2021). Edible films have also been investigated for wound healing applications, as they can provide a protective barrier while promoting moisture retention and drug release at the wound site (Borbolla-Jiménez et al., 2023). Additionally, edible films can be used for oral drug delivery, where medications are formulated into thin strips or films that dissolve in the mouth for convenient administration.
Agricultural Applications: Edible films have found applications in agriculture to improve crop quality and post-harvest preservation (Kocira et al., 2021). They can be applied as coatings on fruits and vegetables to reduce water loss, maintain firmness, and inhibit microbial growth. Edible coatings can also enhance the effectiveness of post-harvest treatments, such as controlled atmosphere storage and modified atmosphere packaging, by providing an additional protective layer (Tabassum and Khan, 2020).
Other Emerging Applications: The versatility of edible films has led to the exploration of various emerging applications. These include the use of edible films as biodegradable packaging materials for personal care products, such as soap and shampoo bars. Edible films have also been utilized as biodegradable mulch films in agriculture to promote weed control, moisture retention, and soil temperature regulation.
Overall, edible films offer a sustainable and versatile solution for packaging, preservation, and controlled delivery in various industries. Ongoing research and advancements in formulation techniques, incorporation of active ingredients, and understanding of film properties continue to expand the potential applications of edible films in the pursuit of environmentally friendly and functional packaging materials.
Nanotechnology has emerged as a significant area of research in the development of plant-based materials and edible films. It involves manipulating materials at the nanoscale to enhance their properties and functionalities. Some recent trends include:
Nanostructured Plant-Based Materials: Nanostructuring involves incorporating nanoparticles or nanoscale structures into plant-based materials to improve their mechanical, barrier, and antimicrobial properties (Harish et al., 2022). For example, nanocellulose, derived from cellulose, has gained attention for its exceptional strength, high surface area, and biodegradability, making it a promising nanomaterial for enhancing the properties of edible films.
Nanocomposite Edible Films: Nanocomposite edible films involve the incorporation of nanofillers, such as nanoparticles or nanoclays, into the matrix of plant-based materials to enhance their mechanical strength, barrier properties, and functional attributes (Jafarzadeh et al., 2022). These nanocomposite films exhibit improved properties, such as increased tensile strength, reduced gas permeability, and enhanced stability as shown in Table 3 below. Nanoparticles, such as silver nanoparticles, can also be incorporated into edible films to confer antimicrobial properties.
Advances in 3D printing technology have opened up new possibilities for the fabrication of plant-based edible films with intricate structures and customized designs as shown in Table 3 below. Recent advancements in 3D printing have allowed for precise control over the deposition of plant-based materials, enabling the creation of complex geometries and customized shapes (Mittal et al., 2023). This technology enables the production of personalized edible films with tailored properties, such as thickness, texture, and release profiles. Additionally, the development of food-grade 3D printers and edible ink formulations has facilitated the direct printing of edible films.
While 3D printing of plant-based edible films offers exciting opportunities, challenges remain. Optimizing the rheological properties of the printing materials, ensuring their food safety and functionality, and achieving high printing resolution are ongoing areas of research. However, the potential for personalized nutrition, on-demand production, and novel sensory experiences makes 3D printing a promising technique in the field of plant-based edible films.
Functionalization involves incorporating additional properties or functionalities into plant-based materials and edible films to enhance their performance and value as shown in Figure 4. Active and intelligent packaging systems involve incorporating active components, such as antimicrobial agents, oxygen scavengers, or moisture absorbers, into the packaging materials (Dobrucka and Cierpiszewski, 2014). These components can help extend the shelf life of packaged products, improve food safety, and enhance product quality. Plant-based materials and edible films can be functionalized with active agents to create active packaging systems that interact with the packaged product and its environment (Dutta and Sit, 2022).
Plant-based materials and edible films can be functionalized by incorporating bioactive compounds, such as antioxidants, antimicrobials, or nutraceuticals (Daniloski et al., 2021). These compounds can provide additional health benefits, enhance food preservation, and improve the sensory attributes of packaged products. Plant extracts, essential oils, and natural antimicrobial agents can be incorporated into the film matrix to impart bioactive properties.
The development of plant-based materials and edible films with inherent antimicrobial properties has gained significant attention (Manzoor et al., 2023). This includes the incorporation of natural antimicrobial agents or the modification of the film surface to create antibacterial or antifungal properties. These antimicrobial edible films can help prevent microbial growth, reduce the need for chemical preservatives, and enhance food safety (Punia et al., 2021).
These recent trends and advances in plant-based materials and edible films highlight the continuous efforts to enhance their properties, functionality, and application potential. The integration of nanotechnology into plant-based materials has allowed for the development of nanostructured materials and nanocomposite edible films, which exhibit improved mechanical strength, barrier properties, and antimicrobial activity (Jafarzadeh et al., 2022). These advancements open up possibilities for creating sustainable and high-performance packaging materials. The utilization of 3D printing technology in the production of plant-based edible films offers opportunities for customized designs, complex geometries, and personalized nutrition. Despite some challenges in terms of material rheology and printing resolution, 3D printing holds promise for on-demand production and novel sensory experiences.
Functionalization of plant-based materials and edible films with active and intelligent packaging components allows for the creation of packaging systems that interact with the packaged product and its environment. Incorporating bioactive compounds into these materials enhances their health benefits, food preservation capabilities, and sensory attributes (Salgado et al., 2015). Furthermore, the development of plant-based materials with inherent antimicrobial properties provides a natural alternative to conventional antimicrobial agents, reducing the need for synthetic preservatives.
These recent trends and advances in plant-based materials and edible films contribute to the overall goal of sustainable and eco-friendly packaging solutions. By harnessing nanotechnology, 3D printing, and functionalization strategies, researchers and industries are continuously exploring innovative approaches to improve the properties, functionalities, and applications of these materials. The future of plant-based materials and edible films holds immense potential in addressing the growing demand for sustainable packaging and promoting a more environmentally conscious and healthier society.
While significant progress has been made in the development of plant-based materials and edible films, challenges related to sustainability, regulation, biodegradability, and performance optimization remain. Future directions aim to overcome these challenges through advanced processing techniques, circular economy approaches, multifunctional materials, and collaborative efforts. By addressing these challenges and advancing research in the field, plant-based materials and edible films have the potential to revolutionize the packaging industry and contribute to a more sustainable and environmentally friendly future.
One of the key challenges in the field of plant-based materials and edible films is ensuring sustainability throughout the entire lifecycle, from sourcing raw materials to disposal. The availability and scalability of plant-based resources need to be carefully considered to meet the growing demand for sustainable packaging materials. Additionally, the development of efficient and eco-friendly processing techniques is crucial to minimize energy consumption and environmental impact.
The regulatory landscape surrounding plant-based materials and edible films is evolving, and there is a need for clear guidelines and standards. It is important to ensure that these materials meet safety requirements and are compliant with relevant regulations for food contact materials. Moreover, consumer acceptance and perception play a vital role in the widespread adoption of plant-based packaging. Educating consumers about the benefits and sustainability aspects of these materials can contribute to their acceptance and market penetration.
Achieving true biodegradability and compostability is a significant challenge for plant-based materials and edible films. While many plant-based materials are biodegradable, their degradation rate and compatibility with existing waste management systems need to be considered (Moshood et al., 2022). Developing materials that can readily biodegrade in various environments, including home composting or industrial composting facilities, is crucial for reducing the environmental impact of packaging waste.
Further research and development efforts are needed to optimize the performance of plant-based materials and edible films (Gaspar and Braga, 2023). This includes improving mechanical strength, barrier properties, and stability to meet the diverse requirements of different applications. Enhancing the film’s functionality, such as incorporating active agents, improving printing resolution, and tailoring release profiles, will broaden the range of potential applications.
Future directions in the field of plant-based materials and edible films include:
1. Advanced Processing Techniques: Exploring advanced processing techniques, such as nanotechnology, electrospinning, and biofabrication, can further enhance the properties and functionalities of plant-based materials. These techniques enable precise control over material structures and properties, opening up new possibilities for tailored applications.
2. Circular Economy Approaches: Focusing on circular economy principles, such as designing materials for recyclability and developing closed-loop systems, can promote the sustainable use and disposal of plant-based packaging. Creating systems that enable efficient collection, recycling, and upcycling of plant-based materials will contribute to a more sustainable packaging industry.
3. Multifunctional Materials: Developing plant-based materials with multifunctional properties can offer added value and expand their application potential. These materials can possess not only barrier and mechanical properties but also functionalities such as antimicrobial activity, moisture management, and intelligent sensing capabilities.
4. Collaboration and Knowledge Sharing: Collaboration among researchers, industries, and regulatory bodies is essential for driving innovation, addressing challenges, and sharing knowledge in the field of plant-based materials and edible films. Open communication and interdisciplinary approaches can accelerate the development and adoption of sustainable packaging solutions.
The advancements in plant-based materials and edible films have the potential to revolutionize the packaging industry and promote sustainable practices. The use of renewable resources reduces reliance on fossil fuels and decreases carbon emissions. By replacing conventional plastic packaging with plant-based alternatives, we can mitigate environmental issues associated with plastic waste, such as pollution and landfill accumulation.
Furthermore, the future prospects of plant-based materials and edible films are promising. Ongoing research and development efforts are focused on addressing challenges related to sustainability, scalability, regulation, and consumer acceptance. Advancements in processing techniques, such as nanotechnology and 3D printing, offer exciting possibilities for tailoring materials and creating customized packaging solutions. Additionally, the functionalization of plant-based materials with active and intelligent components enhances food safety, preservation, and quality.
Collaboration among researchers, industries, and regulatory bodies is crucial for driving innovation and knowledge sharing in the field. This collaboration can facilitate the development of standardized guidelines and regulations for plant-based materials and edible films, ensuring their safety and compliance with food packaging requirements.
In conclusion, plant-based materials and edible films have the potential to significantly impact the packaging industry by providing sustainable alternatives to conventional materials. With ongoing research, technological advancements, and increased consumer awareness, these materials can contribute to a more environmentally friendly and sustainable future. By embracing these innovations, we can move towards a circular economy, reduce plastic waste, and create a more sustainable and healthier planet for future generations.
DBO: Conceptualization, Methodology, Resources, Writing–original draft, Writing–review and editing. OZW: Methodology, Writing–original draft, Writing–review and editing. AOI: Formal Analysis, Software, Writing–original draft, Writing–review and editing.
The author(s) declare that no financial support was received for the research, authorship, and/or publication of this article.
The authors declare that the research was conducted in the absence of any commercial or financial relationships that could be construed as a potential conflict of interest.
All claims expressed in this article are solely those of the authors and do not necessarily represent those of their affiliated organizations, or those of the publisher, the editors and the reviewers. Any product that may be evaluated in this article, or claim that may be made by its manufacturer, is not guaranteed or endorsed by the publisher.
Acharya, S., Liyanage, S., Parajuli, P., Rumi, S. S., Shamshina, J. L., and Abidi, N. (2021). Utilization of cellulose to its full potential: a review on cellulose dissolution, regeneration, and applications. Polymers 13 (24), 4344. doi:10.3390/polym13244344
Aguirre-Loredo, R. Y., Rodríguez-Hernández, A. I., and Chavarría-Hernández, N. (2014). Physical properties of emulsified films based on chitosan and oleic acid. CyTA - J. Food 12, 305–312. doi:10.1080/19476337.2013.853207
Ali, S., Anjum, M. A., Khan, A. S., Nawaz, A., Khaliq, G., Iqbal, S., et al. (2022). Carboxymethyl cellulose coating delays ripening of harvested mango fruits by regulating softening enzymes activities. Food Chem. 380, 131804. doi:10.1016/j.foodchem.2021.131804
Alshehhi, J. R. M. H., Wanasingha, N., Balu, R., Mata, J., Shah, K., Dutta, N. K., et al. (2024). 3D-Printable sustainable bioplastics from gluten and keratin. Gels 10, 136. doi:10.3390/gels10020136
Amaraweera, S. M., Gunathilake, C., Gunawardene, O. H. P., Fernando, N. M. L., Wanninayaka, D. B., Dassanayake, R. S., et al. (2021). Development of starch-based materials using current modification techniques and their applications: a review. Molecules 26 (22), 6880. doi:10.3390/molecules26226880
Badawy, M. E. I., Rabea, E. I., El-Nouby, M. a. M., Ismail, R. I. A., and Taktak, N. E. M. (2016). Strawberry shelf life, composition, and enzymes activity in response to edible chitosan coatings. Int. J. Fruit Sci. 17 (2), 117–136. doi:10.1080/15538362.2016.1219290
Bagnani, M., Peydayesh, M., Knapp, T., Appenzeller, E., Sutter, D., Kränzlin, S., et al. (2024). From soy waste to bioplastics: industrial proof of concept. Biomacromolecules 25, 2033–2040. doi:10.1021/acs.biomac.3c01416
Benbettaïeb, N., Debeaufort, F., and Karbowiak, T. (2018). Bioactive edible films for food applications: mechanisms of antimicrobial and antioxidant activity. Crit. Rev. Food Sci. Nutr. 59 (21), 3431–3455. doi:10.1080/10408398.2018.1494132
Bizymis, A., and Tzia, C. (2021). Edible films and coatings: properties for the selection of the components, evolution through composites and nanomaterials, and safety issues. Crit. Rev. Food Sci. Nutr. 62 (31), 8777–8792. doi:10.1080/10408398.2021.1934652
Borbolla-Jiménez, F. V., Peña-Corona, S. I., Farah, S. J., Jiménez-Valdés, M. T., Pineda-Pérez, E., Romero-Montero, A., et al. (2023). Films for wound healing fabricated using a solvent casting technique. Pharmaceutics 15 (7), 1914. doi:10.3390/pharmaceutics15071914
Campos, C. A., Gerschenson, L. N., and Flores, S. K. (2011). Development of edible films and coatings with antimicrobial activity. Food Bioproc Tech. 4, 849–875. doi:10.1007/s11947-010-0434-1
Casariego, A., Souza, B. W. S., Cerqueira, M. A., Teixeira, J. A., Cruz, L., Díaz, R., et al. (2009). Chitosan/clay films’ properties as affected by biopolymer and clay micro/nanoparticles’ concentrations. Food Hydrocoll. 23, 1895–1902. doi:10.1016/j.foodhyd.2009.02.007
Cazon, P., Velázquez, G., RamiRez, J. A., and Vázquez, M. (2017). Polysaccharide-based films and coatings for food packaging: a review. Food Hydrocoll. 68, 136–148. doi:10.1016/j.foodhyd.2016.09.009
Chamanee, G., Sewwandi, M., Wijesekara, H., and Vithanage, M. (2023). Global perspective on microplastics in landfill leachate; Occurrence, abundance, characteristics, and environmental impact. Waste Manag. 171, 10–25. doi:10.1016/j.wasman.2023.08.011
Chang, B. P., Trinh, B. M., Tadele, D. T., Bandara, N., and Mekonnen, T. H. (2023). Natural antioxidant and antimicrobial agents and processing technologies for the design of active food packaging polymers. Polym. Rev. 63, 961–1013. doi:10.1080/15583724.2023.2234464
Chaudhary, V., Kajla, P., Kumari, P., Bangar, S. P., Rusu, A. V., Tirf, M., et al. (2022). Milk protein-based active edible packaging for food applications: an eco-friendly approach. Front. Nutr. 9, 942524. doi:10.3389/fnut.2022.942524
Cheng, H., Chen, L., McClements, D. J., Yang, T., Zhang, Z., Ren, F., et al. (2021). Starch-based biodegradable packaging materials: a review of their preparation, characterization and diverse applications in the food industry. Trends Food Sci. Technol. 114, 70–82. doi:10.1016/j.tifs.2021.05.017
Daltry, A., Merone, L., and Tait, P. (2021). Plastic pollution: why is it a public health problem? Aust. N. Z. J. Public Health 45, 535–537. doi:10.1111/1753-6405.13149
Daniloski, D., Petkoska, A. T., Lee, N. A., Bekhit, A. E. A., Carne, A., Vaskoska, R., et al. (2021). Active edible packaging based on milk proteins: a route to carry and deliver nutraceuticals. Trends Food Sci. Technol. 111, 688–705. doi:10.1016/j.tifs.2021.03.024
Demircan, B., and Velioğlu, S. (2024). Control of browning, enzyme activity, and quality in stored fresh-cut fruit salads through chitosan coating enriched with bergamot juice powder. Foods 13 (1), 147. doi:10.3390/foods13010147
Díaz-Montes, E., and Castro-Muñoz, R. (2021). Edible films and coatings as food-quality preservers: an overview. Foods 10, 249. doi:10.3390/foods10020249
Dobrucka, R., and Cierpiszewski, R. (2014). Active and intelligent packaging food - research and development - a review. Pol. J. Food Nutr. Sci. - Tom 64, Numer 1 (2014) - Biblioteka Nauki - Yadda 64, 7–15. doi:10.2478/v10222-012-0091-3Available at: https://yadda.icm.edu.pl/yadda/element/bwmeta1.element.agro-840f6a8c-9921-49f7-a0f3-e0406a18c77c.
Dutta, D., and Sit, N. (2022). Application of natural extracts as active ingredient in biopolymer-based packaging systems. J. Food Sci. Technol. 60 (7), 1888–1902. doi:10.1007/s13197-022-05474-5
El-Sayed, H. S., El-Sayed, S. M., Mabrouk, A. M. M., Nawwar, G. A., and Youssef, A. M. (2021). Development of eco-friendly probiotic edible coatings based on chitosan, alginate and carboxymethyl cellulose for improving the shelf life of UF soft cheese. J. Polym. Environ. 29, 1941–1953. doi:10.1007/s10924-020-02003-3
Falguera, V., Quintero, J. P., Jiménez, A. J. G., Muñoz, J. A., and Ibarz, A. (2011). Edible films and coatings: structures, active functions, and trends in their use. Trends Food Sci. Technol. 22 (6), 292–303. doi:10.1016/j.tifs.2011.02.004
Filho, W. L., Barbir, J., Abubakar, I. R., Paço, A., Stasiskiene, Z., Hornbogen, M., et al. (2022). Consumer attitudes and concerns with bioplastics use: an international study. PLoS One 17, e0266918. doi:10.1371/journal.pone.0266918
Florentino, G. I. B., Lima, D. A. S., Santos, M. M. F., Ferreira, V. C. da S., Grisi, C. V. B., Madruga, M. S., et al. (2022). Characterization of a new food packaging material based on fish by-product proteins and passion fruit pectin. Food Packag Shelf Life 33, 100920. doi:10.1016/j.fpsl.2022.100920
Fu, M., Cao, M., Duan, J., Zhou, Q., Dong, M., Zhang, T., et al. (2022). Research on the properties of zein, soy protein isolate, and wheat gluten Protein-Based films containing cellulose nanocrystals. Foods 11 (19), 3010. doi:10.3390/foods11193010
Gaspar, M. C., and Braga, M. E. (2023). Edible films and coatings based on agrifood residues: a new trend in the food packaging research. Curr. Opin. Food Sci. 50, 101006. doi:10.1016/j.cofs.2023.101006
Ghidelli, C. (2015). Effect of soy protein-based edible coatings with antioxidant activity and modified atmosphere packaging on the quality of fresh-cut produces. doi:10.4995/thesis/10251/39104
Gironi, F., and Piemonte, V. (2011). Bioplastics and petroleum-based plastics: strengths and weaknesses. Energy Sources, Part A Recovery, Util. Environ. Eff. 33, 1949–1959. doi:10.1080/15567030903436830
Gounga, M. E., Xu, S., and Wang, Z. (2010). Film forming mechanism and mechanical and thermal properties of whey protein isolate-based edible films as affected by protein concentration, glycerol ratio and pullulan content. J. Food Biochem. doi:10.1111/j.1745-4514.2009.00294.x
Gupta, P., Toksha, B., and Rahaman, M. (2022). A review on biodegradable packaging films from vegetative and food waste. Chem. Rec. 22, e202100326. doi:10.1002/tcr.202100326
Gupta, R. K., Guha, P., and Srivastav, P. P. (2022). Natural polymers in bio-degradable/edible film: a review on environmental concerns, cold plasma technology and nanotechnology application on food packaging- A recent trends. Food Chem. Adv. 1, 100135. doi:10.1016/j.focha.2022.100135
Hadidi, M., Jafarzadeh, S., Forough, M., Garavand, F., Alizadeh, S. A., Salehabadi, A., et al. (2022). Plant protein-based food packaging films; recent advances in fabrication, characterization, and applications. Trends Food Sci. Technol. 120, 154–173. doi:10.1016/j.tifs.2022.01.013
Haider, T. P., Völker, C., Kramm, J., Landfester, K., and Wurm, F. R. (2019). Plastics of the future? The impact of biodegradable polymers on the environment and on society. Angew. Chem. Int. Ed. 58, 50–62. doi:10.1002/anie.201805766
Han, J. H. (2014). “Edible films and coatings,” in Innovations in food packaging (Elsevier), 213–255. doi:10.1016/B978-0-12-394601-0.00009-6
Harish, V., Tewari, D., Gaur, M., Yadav, A. B., Swaroop, S., Bechelany, M., et al. (2022). Review on nanoparticles and nanostructured materials: bioimaging, biosensing, drug delivery, tissue engineering, Antimicrobial, and Agro-Food Applications. Nanomaterials 12 (3), 457. doi:10.3390/nano12030457
Heydari, R., Bavandi, S., and Javadian, S. R. (2015). Effect of sodium alginate coating enriched with horsemint (M entha longifolia) essential oil on the quality of bighead carp fillets during storage at 4°C. Food Sci. Nutr. 3, 188–194. doi:10.1002/fsn3.202
Jafarzadeh, S., Forough, M., Amjadi, S., Kouzegaran, V. J., Almasi, H., Garavand, F., et al. (2022). Plant protein-based nanocomposite films: a review on the used nanomaterials, characteristics, and food packaging applications. Crit. Rev. Food Sci. Nutr. 63 (29), 9667–9693. doi:10.1080/10408398.2022.2070721
Jeevahan, J., Chandrasekaran, M., Venkatesan, S. P., Sriram, V., Joseph, G. B., Mageshwaran, G., et al. (2020). Scaling up difficulties and commercial aspects of edible films for food packaging: a review. Trends Food Sci. Technol. 100, 210–222. doi:10.1016/j.tifs.2020.04.014
Kaewprachu, P., and Rawdkuen, S. (2016). Application of active edible film as food packaging for food preservation and extending shelf life. Springer eBooks, 185–205. doi:10.1007/978-3-319-25277-3_10
Karaca, O. B., Oluk, C. A., Taşpınar, T., and Güven, M. (2019). New concept in packaging: milk protein edible films. Springer eBooks, 537–565. doi:10.1007/978-3-030-24903-8_19
Khan, M. R., Volpe, S., Valentino, M., Miele, N. A., Cavella, S., and Torrieri, E. (2021). Active casein coatings and films for perishable foods: structural properties and Shelf-Life extension. Coatings 11 (8), 899. doi:10.3390/coatings11080899
Khorrami, N. K., Radi, M., Amiri, S., and McClements, D. J. (2021). Fabrication and characterization of alginate-based films functionalized with nanostructured lipid carriers. Int. J. Biol. Macromol. 182, 373–384. doi:10.1016/j.ijbiomac.2021.03.159
Khosravi, Z., Fallah, A. A., and Abtahi, H. (2023). Nanocomposite films based on soy protein Isolate-Montmorillonite nanoclay containing emulsion and nanoemulsion of Zataria Multiflora essential oil for preserving chilled chicken burgers. jnfh.mums.Ac. Ir. 11 (4), 303–314. doi:10.22038/jnfh.2023.74440.1460
Khubiev, O., Egorov, A. R., Kirichuk, A. A., Хрусталев, В. Н., Tskhovrebov, A. G., and Kritchenkov, A. S. (2023). Chitosan-Based antibacterial films for biomedical and food applications. Int. J. Mol. Sci. 24 (13), 10738. doi:10.3390/ijms241310738
Kim, S., Baek, S.-K., and Song, K. B. (2018). Physical and antioxidant properties of alginate films prepared from Sargassum fulvellum with black chokeberry extract. Food Packag Shelf Life 18, 157–163. doi:10.1016/j.fpsl.2018.11.008
Kim, Y., Min, B. R., and Kim, K. W. (2014). General characteristics of packaging materials for food system. Elsevier Eb., 13–35. doi:10.1016/b978-0-12-394601-0.00002-3
Kocira, A., Kozłowicz, K., Panasiewicz, K., Staniak, M., Szpunar-Krok, E., and Hortyńska, P. (2021). Polysaccharides as edible films and coatings: characteristics and influence on fruit and vegetable quality—a review. Agronomy 11 (5), 813. doi:10.3390/agronomy11050813
Kumar, N., Pratibha, , Upadhyay, A., Petkoska, A. T., Gniewosz, M., and Kieliszek, M. (2022). Extending the shelf life of mango (Mangifera indica L.) fruits by using edible coating based on xanthan gum and pomegranate peel extract. J. Food Meas. Charact. 17 (2), 1300–1308. doi:10.1007/s11694-022-01706-6
Kumar, R., Verma, A., Shome, A., Sinha, R., Sinha, S., Jha, P. K., et al. (2021). Impacts of plastic pollution on ecosystem services, sustainable development goals, and need to focus on circular economy and policy interventions. Sustainability 13 (17), 9963. doi:10.3390/su13179963
Kurdikar, D., Fournet, L., Slater, S. C., Paster, M., Gruys, K. J., Gerngross, T. U., et al. (2000). Greenhouse gas profile of a plastic material derived from a genetically modified plant. J. Ind. Ecol. 4, 107–122. doi:10.1162/108819800300106410
Laorenza, Y., and Harnkarnsujarit, N. (2021). Carvacrol, citral and α-terpineol essential oil incorporated biodegradable films for functional active packaging of Pacific white shrimp. Food Chem. 363, 130252. doi:10.1016/j.foodchem.2021.130252
Lin, X., Zhang, S., Yang, S., Zhang, R., Shi, X., and Song, L. (2023). A landfill serves as a critical source of microplastic pollution and harbors diverse plastic biodegradation microbial species and enzymes: study in large-scale landfills, China. J. Hazard Mater 457, 131676. doi:10.1016/j.jhazmat.2023.131676
Liu, Y., Ahmed, S., Sameen, D. E., Wang, Y., Lü, R., Dai, J., et al. (2021). A review of cellulose and its derivatives in biopolymer-based for food packaging application. Trends Food Sci. Technol. 112, 532–546. doi:10.1016/j.tifs.2021.04.016
Lukum, A., Kadir, A. A., Sukamto, K., Mohamad, E., Thayban, T., and Paramata, A. R. (2023). Application of shrimp shell waste chitosan as edible coating to extend the shelf-life of tomato (Solanum lycopersicum L.). E3S Web Conf. 400, 04008. doi:10.1051/e3sconf/202340004008
Manzoor, A., Yousuf, B., Pandith, J. A., and Ahmad, S. (2023). Plant-derived active substances incorporated as antioxidant, antibacterial or antifungal components in coatings/films for food packaging applications. Food Biosci. 53, 102717. doi:10.1016/j.fbio.2023.102717
Martins, J. R., Llanos, J. H. R., Abe, M. M., Costa, M. L., and Brienzo, M. (2024). New blend of renewable bioplastic based on starch and acetylated xylan with high resistance to oil and water vapor. Carbohydr. Res. 537, 109068. doi:10.1016/j.carres.2024.109068
Matheus, J. R. V., Dalsasso, R. R., Rebelatto, E. A., Andrade, K. S., Andrade, L. M. de, Andrade, C. J. de, et al. (2023). Biopolymers as green-based food packaging materials: a focus on modified and unmodified starch-based films. Compr. Rev. Food Sci. Food Saf. 22, 1148–1183. doi:10.1111/1541-4337.13107
Mittal, S., Bhuiyan, M. H. R., and Ngadi, M. (2023). Challenges and prospects of plant-protein-based 3D printing. Foods 12 (24), 4490. doi:10.3390/foods12244490
Mondal, K., Ghosh, T., and Katiyar, V. (2021). Edible food packaging in targeted food preservation. Mater. horizons, 371–409. doi:10.1007/978-981-33-6169-0_14
Moshood, T. D., Nawanir, G., Mahmud, F., Mohamad, F., Ahmad, M. H., and AbdulGhani, A. (2022). Sustainability of biodegradable plastics: new problem or solution to solve the global plastic pollution? Curr. Res. Green Sustain. Chem. 5, 100273. doi:10.1016/j.crgsc.2022.100273
Nilsen-Nygaard, J., Fernández, E. N., Radusin, T., Rotabakk, B. T., Sarfraz, J., Sharmin, N., et al. (2021). Current status of biobased and biodegradable food packaging materials: impact on food quality and effect of innovative processing technologies. Compr. Rev. Food Sci. Food Saf. 20, 1333–1380. doi:10.1111/1541-4337.12715
Nunes, C., Silva, M., Farinha, D., Sales, H., Pontes, R., and Nunes, J. (2023). Edible coatings and future trends in active food packaging–fruits’ and traditional sausages’ shelf life increasing. Foods 12 (17), 3308. doi:10.3390/foods12173308
Petkoska, A. T., Daniloski, D., D’Cunha, N. M., Naumovski, N., and Broach, A. T. (2021). Edible packaging: sustainable solutions and novel trends in food packaging. Food Res. Int. 140, 109981. doi:10.1016/j.foodres.2020.109981
Pirayandeh, S., Fattahi, M. S., Akbarzade, M., Mohammadi, T., and Tofighy, M. A. (2024). “Nanofillers in membrane industry,” in Handbook of nanofillers. Editors S. Mallakpour, and C. M. Hussain (Singapore: Springer). doi:10.1007/978-981-99-3516-1_72-1
Priyadarshi, R., and Rhim, J. W. (2020). Chitosan-based biodegradable functional films for food packaging applications. Innovative Food Sci. Emerg. Technol. 62, 102346. doi:10.1016/j.ifset.2020.102346
Promhuad, K., Bumbudsanpharoke, N., Wadaugsorn, K., Sonchaeng, U., and Harnkarnsujarit, N. (2022). Maltol-Incorporated acetylated Cassava Starch films for Shelf-Life-Extension packaging of bakery products. Polymers 14 (24), 5342. doi:10.3390/polym14245342
Punia, S., Chaudhary, V., Thakur, N., Kajla, P., Kumar, M., and Trif, M. (2021). Natural antimicrobials as additives for Edible Food Packaging applications: a review. Foods 10 (10), 2282. doi:10.3390/foods10102282
Rai, P., Mehrotra, S., Priya, S., Gnansounou, E., and Sharma, S. (2021). Recent advances in the sustainable design and applications of biodegradable polymers. Bioresour. Technol. 325, 124739. doi:10.1016/j.biortech.2021.124739
Ritchie, H., Samborska, V., and Roser, M. (2023). Plastic pollution. Available at: https://ourworldindata.org/plastic-pollution.
Saji, V. S. (2020). Wax-based artificial superhydrophobic surfaces and coatings. Colloids Surfaces A Physicochem. Eng. Aspects 602, 125132. doi:10.1016/j.colsurfa.2020.125132
Salgado, P. R., Ortiz, C., Musso, Y. S., Di Giorgio, L., and Mauri, A. N. (2015). Edible films and coatings containing bioactives. Curr. Opin. Food Sci. 5, 86–92. doi:10.1016/j.cofs.2015.09.004
Sangkham, S. (2024). Global perspective on the impact of plastic waste as a source of microplastics and per- and polyfluoroalkyl substances in the environment. ACS ES&T Water 4, 1–4. doi:10.1021/acsestwater.3c00607
Shah, Y. A., Bhatia, S., Al-Harrasi, A., Afzaal, M., Saeed, F., Anwer, M. K., et al. (2023). Mechanical properties of Protein-Based food packaging materials. Polymers 15 (7), 1724. doi:10.3390/polym15071724
Shim, E. (2013). Bonding requirements in coating and laminating of textiles. Elsevier eBooks, 309–351. doi:10.1533/9780857093967.2.309
Silva, E. G. S., Cardoso, S., Bettencourt, A. F., and Ribeiro, I. a. C. (2022). Latest trends in sustainable Polymeric food Packaging films. Foods 12 (1), 168. doi:10.3390/foods12010168
Singh, S., Khemariya, P., Rai, A., Chandra, A., Koley, T. K., and Singh, B. (2016). Carnauba wax-based edible coating enhances shelf-life and retain quality of eggplant (Solanum melongena) fruits. LWT 74, 420–426. doi:10.1016/j.lwt.2016.08.004
Siqueira, L. D. V., La Fuente Arias, C. I., Maniglia, B. C., and Tadini, C. C. (2021). Starch-based biodegradable plastics: methods of production, challenges and future perspectives. Curr. Opin. Food Sci. 38, 122–130. doi:10.1016/j.cofs.2020.10.020
Suhag, R., Kumar, N., Petkoska, A. T., and Upadhyay, A. (2020). Film formation and deposition methods of edible coating on food products: a review. Food Res. Int. 136, 109582. doi:10.1016/j.foodres.2020.109582
Tabassum, N., and Khan, M. A. (2020). Modified atmosphere packaging of fresh-cut papaya using alginate based edible coating: quality evaluation and shelf life study. Sci. Hortic. 259, 108853. doi:10.1016/j.scienta.2019.108853
Tatara, R. A. (2024). Compression molding. Elsevier eBooks, 389–424. doi:10.1016/b978-0-323-88667-3.00011-4
Teixeira-Costa, B. E., and Andrade, C. T. (2021). Natural polymers used in edible food packaging—history, function and application trends as a sustainable alternative to synthetic plastic. Polysaccharides 3 (1), 32–58. doi:10.3390/polysaccharides3010002
Trinh, B. M., Chang, B. P., and Mekonnen, T. H. (2023). The barrier properties of sustainable multiphase and multicomponent packaging materials: a review. Prog. Mater Sci. 133, 101071. doi:10.1016/j.pmatsci.2023.101071
Ullah, S., Ahmad, S., Guo, X., Ullah, S., Ullah, S., Nabi, G., et al. (2022). A review of the endocrine disrupting effects of micro and nano plastic and their associated chemicals in mammals. Front. Endocrinol. (Lausanne) 13, 1084236. doi:10.3389/fendo.2022.1084236
Versino, F., Ortega, F., Monroy, Y., Rivero, S., López, O. V., and García, M. A. (2023). Sustainable and bio-based food packaging: a review on past and current design innovations. Foods 12, 1057. doi:10.3390/foods12051057
Wang, H., Yuan, T., Song, G., and Sun, R. (2021). Advanced and versatile lignin-derived biodegradable composite film materials toward a sustainable world. Green Chem. 23 (11), 3790–3817. doi:10.1039/d1gc00790d
Waste and Resources Action Programme (2024). Redesigning the plastics system. Available at: https://www.wrap.ngo/taking-action/plastic-packaging#:∼:text=The%20world%20produces%20141%20million,tonnes%20of%20carbon%20emissions%20annually.
Wu, H., Sang, S., Weng, P., Pan, D., Wu, Z., Yang, J., et al. (2023). Structural, rheological, and gelling characteristics of starch-based materials in context to 3D food printing applications in precision nutrition. Compr. Rev. Food Sci. Food Saf. 22 (6), 4217–4241. doi:10.1111/1541-4337.13217
Wu, J., Sun, Q., Huang, H., Duan, Y., Xiao, G., and Le, T. (2019). Enhanced physico-mechanical, barrier and antifungal properties of soy protein isolate film by incorporating both plant-sourced cinnamaldehyde and facile synthesized zinc oxide nanosheets. Colloids Surfaces B Biointerfaces 180, 31–38. doi:10.1016/j.colsurfb.2019.04.041
Xing, Y., Li, W., Wang, Q., Li, X., Xu, Q., Guo, X., et al. (2019). Antimicrobial nanoparticles incorporated in edible coatings and films for the preservation of fruits and vegetables. Molecules/Molecules Online/Molecules Annu. 24 (9), 1695. doi:10.3390/molecules24091695
Xu, Q., Chen, C., Rosswurm, K., Yao, T., and Janaswamy, S. (2016). A facile route to prepare cellulose-based films. Carbohydr. Polym. 149, 274–281. doi:10.1016/j.carbpol.2016.04.114
Yang, W., Zhang, Z., Chen, Y. P., and Luo, K. (2023). Evaluation of the use of Idesia polycarpa Maxim protein coating to extend the shelf life of European sweet cherries. Front. Nutr. 10, 1283086. doi:10.3389/fnut.2023.1283086
Yilmaz-Turan, S., Jiménez-Quero, A., Menzel, C., de Carvalho, D. M., Lindström, M. E., Sevastyanova, O., et al. (2020). Bio-based films from wheat bran feruloylated arabinoxylan: effect of extraction technique, acetylation and feruloylation. Carbohydr. Polym. 250, 116916. doi:10.1016/j.carbpol.2020.116916
Yu, X., Chen, L., Jin, Z., and Jiao, A. (2021). Research progress of starch-based biodegradable materials: a review. J. Mater. Sci. 56 (19), 11187–11208. doi:10.1007/s10853-021-06063-1
Zhang, L., Yu, D., Regenstein, J. M., Xia, W., and Dong, J. (2021). A comprehensive review on natural bioactive films with controlled release characteristics and their applications in foods and pharmaceuticals. Trends Food Sci. Technol. 112, 690–707. doi:10.1016/j.tifs.2021.03.053
Zhang, M., Jia, R., Ma, M., Yang, T., Sun, Q., and Li, M. (2022). Versatile wheat gluten: functional properties and application in the food-related industry. Crit. Rev. Food Sci. Nutr. 63 (30), 10444–10460. doi:10.1080/10408398.2022.2078785
Zhang, Y., Nian, Y., Shi, Q., and Hu, B. (2023). Protein fibrillation and hybridization with polysaccharides enhance strength, toughness, and gas selectivity of bioplastic packaging. J. Mater Chem. A Mater 11, 9884–9901. doi:10.1039/D3TA00446E
Zhao, X., Cornish, K., and Vodovotz, Y. (2020). Narrowing the gap for bioplastic use in food packaging: an update. Environ. Sci. Technol. 54, 4712–4732. doi:10.1021/acs.est.9b03755
Zheng, K., Li, B., Liu, Y., Wu, D., Bai, Y., and Xiang, Q. (2023). Effect of chitosan coating incorporated with oregano essential oil on microbial inactivation and quality properties of refrigerated chicken breasts. LWT 176, 114547. doi:10.1016/j.lwt.2023.114547
Zimmermann, L., Dombrowski, A., Völker, C., and Wagner, M. (2020). Are bioplastics and plant-based materials safer than conventional plastics? in vitro toxicity and chemical composition. Environ. Int. 145, 106066. doi:10.1016/j.envint.2020.106066
Zubair, M., Pradhan, R. A., Arshad, M., and Ullah, A. (2021). Recent advances in lipid derived Bio-Based materials for food packaging applications. Macromol. Mater. Eng. 306 (7). doi:10.1002/mame.202000799
Zubair, M., Shahzad, S. A., Hussain, A., Pradhan, R. A., Arshad, M., and Ullah, A. (2022). Current trends in the utilization of essential oils for Polysaccharide- and Protein-Derived food packaging materials. Polymers 14 (6), 1146. doi:10.3390/polym14061146
Keywords: plant-based materials, edible films, food packaging, plastic waste, nanocomposite
Citation: Olawade DB, Wada OZ and Ige AO (2024) Advances and recent trends in plant-based materials and edible films: a mini-review. Front. Chem. 12:1441650. doi: 10.3389/fchem.2024.1441650
Received: 31 May 2024; Accepted: 08 August 2024;
Published: 21 August 2024.
Edited by:
Faliang Luo, Ningxia University, ChinaReviewed by:
Tianyi Liu, Brewer Science, United StatesCopyright © 2024 Olawade, Wada and Ige. This is an open-access article distributed under the terms of the Creative Commons Attribution License (CC BY). The use, distribution or reproduction in other forums is permitted, provided the original author(s) and the copyright owner(s) are credited and that the original publication in this journal is cited, in accordance with accepted academic practice. No use, distribution or reproduction is permitted which does not comply with these terms.
*Correspondence: David B. Olawade, ZC5vbGF3YWRlQHVlbC5hYy51aw==
Disclaimer: All claims expressed in this article are solely those of the authors and do not necessarily represent those of their affiliated organizations, or those of the publisher, the editors and the reviewers. Any product that may be evaluated in this article or claim that may be made by its manufacturer is not guaranteed or endorsed by the publisher.
Research integrity at Frontiers
Learn more about the work of our research integrity team to safeguard the quality of each article we publish.