- 1Istituto di Chimica Biomolecolare, Consiglio Nazionale delle Ricerche, Pozzuoli, Italy
- 2Canada Excellence Research Chair on the Microbiome-Endocannabinoidome Axis in Metabolic Health, Faculty of Medicine and Faculty of Agricultural and Food Sciences, Centre de Recherche de l’Institut de Cardiologie et Pneumologie de l’Université et Institut sur la Nutrition et les Aliments Fonctionnels, Centre NUTRISS, Université Laval, Quebec, Canada
A simple and sustainable propylphosphonic anhydride (T3P)-assisted methodology for the synthesis of N-acyl tryptamines, an interesting class of gut microbiota-derived endocannabinoid-like lipid mediators, was proposed. This protocol is characterized by great operational simplicity, and all products were obtained at room temperature, without the use of an inert atmosphere and by using limited amounts of non-halogenated solvents. Finally, the possibility to realize the reaction under mechanochemical conditions was explored with interesting results.
1 Introduction
Tryptamine is a metabolite of tryptophan produced by the gut microbiota through the indole pathway (Roth et al., 2021). Recently, it was demonstrated that the gut microbiota can also biosynthesize N-acyl tryptamines, i.e., compounds in which tryptamine is linked to bioavailable endogenous/dietary fatty acids (Chang et al., 2021). These amides, from a structural point of view, are very similar to endogenous signaling molecules belonging to the endocannabinoidome (Di Marzo, 2020) [e.g., N-acyl serotonins (Verhoeckx et al., 2011)], and, as such, they often compete for the same molecular targets, thus resulting bioactive (Chang et al., 2021; Ki et al., 2020).
Considering the interesting bioactivities that are emerging for N-acyl tryptamines, the design of new synthetic strategies (Nicolaou, 2014; Villano et al., 2022) is fundamental in order to have sufficient quantities of these molecules to test with the aim of 1) extending the screening of their bioactivities and understanding their role and mechanisms of action and 2) inserting appropriate structural modifications in the original scaffold for structure–activity relationship (SAR) studies. In this context, the development of new sustainable methodologies, based on the use of low-toxicity reagents, that maximize the quantity of the product and reduce the quantity of waste, as an alternative to obsolete high-impact methodologies, represents the real challenge (Horváth and Anastas, 2007; Li and Trost, 2008).
Some methods for the synthesis of N-acyl tryptamines are reported in the literature (Scheme 1): their synthesis was carried out at high temperatures (approximately 200°C) with complex reaction mixtures being produced. Thus, difficult purification was often required, and the method was not suitable for thermolabile products or carboxylic acids (Hahn and Friedel Gudjons, 1938); alternatively, carboxylic acid could be converted into acyl chloride with SOCl2 or oxalyl chloride before a subsequent reaction with tryptamine. However, this alternative method is not very sustainable due to the use of toxic chlorinating agents and, often, halogenated solvents, especially during acyl halide synthesis (Schmidt et al., 2010).
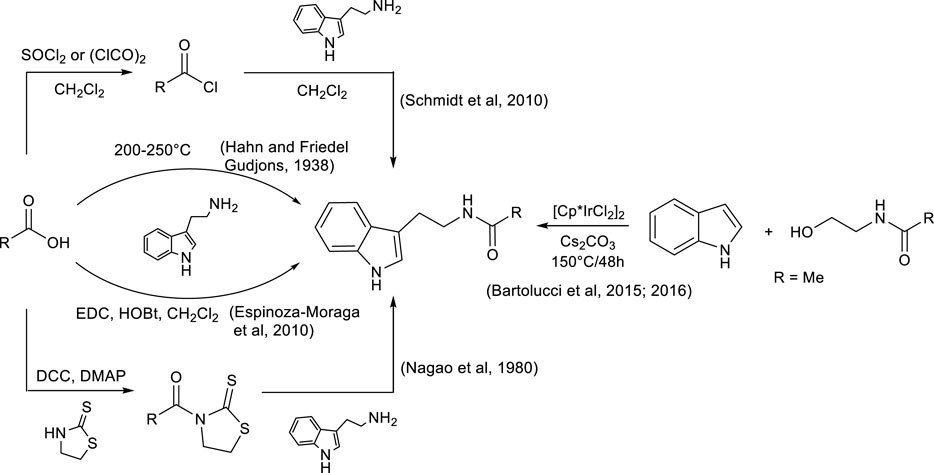
Scheme 1. Some synthetic strategies reported in the literature for the production of N-acyl tryptamines.
More recently, N-acetyl tryptamines were synthesized by the direct alkylation of indoles with N-acetylethanolamine, using [Cp*IrCl2]2 and Cs2CO3 at high temperature; the corresponding products were isolated with satisfactory yields, although the methodology was limited to the production of acetyl derivatives (Bartolucci et al., 2015; Bartolucci et al., 2016).
Some coupling reagents, such as DCC/thiazolidine-2-thione (Nagao et al., 1980) or the EDC/HOBt (Espinoza-Moraga et al., 2010) system, were also used to promote the reaction between carboxylic acid and tryptamine, but, even in this case, despite good yields, the coupling reagents are not sustainable. DCC and EDC are substances characterized by acute toxicity and can cause organ damage (Jordan et al., 2021), while some additives such as HOBt, in addition to being harmful to health, show explosive properties at high temperatures or under mechanical stress (Wehrstedt et al., 2005), and therefore, many precautions must be taken during their use and storage. Furthermore, the reactions promoted by DCC or EDC are often carried out in halogenated solvents and at high dilutions, conditions that further reduce the sustainability of the process. Finally, another problem associated with the use of these classical coupling reagents is that their by-products are insoluble in water but show partial solubility in organic solvents, and therefore, during the extraction step, they remain in the mixture with the reaction product. Consequently, laborious chromatographic purifications are often required to obtain the pure reaction product, with a large increase in the volume of solvents, chromatographic materials, and energy used.
Propylphosphonic anhydride (T3P) (Basavaprabhu et al., 2013) is emerging as a good alternative to other less sustainable coupling reagents (such as the mentioned DCC and EDC); it was successfully used in peptide synthesis (Wissmann and Kleiner, 1980; Mattellone et al., 2023a; Mattellone et al., 2023b) even in the solid state (Al Musaimi et al., 2021) and, recently, also in the reaction between tryptamine and carboxylic acids (Ábrányi-Balogh et al., 2016). However, in the latter case, the experimental conditions (no base, MW irradiation, 120°C–150°C, and high pressure) did not allow the isolation of N-acyl tryptamines but favored the synthesis of 1-substituted-3,4-dihydro-β-carbolines via a cascade mechanism (acylation of tryptamine and subsequent Bischler–Napieralski cyclization of the amide, in a consecutive and one-pot manner).
Based on the indications of the ACS Green Chemistry Institute (Isidro-Llobet et al., 2019), T3P is considered a green reagent, thanks to its stability and low-toxicity/allergy profile, characteristics that make it an excellent candidate for the development of sustainable methodologies. Furthermore, it is commercially available in solution with different organic solvents (EtOAc, 2-MeTHF, etc.), and these formulations increase its stability and also make it suitable for use in small-scale reactions. Finally, unlike as with other classic coupling reagents (e.g., DCC and EDC), T3P by-products are soluble in water, and therefore, at the end of the reaction, they can be easily separated from the reaction mixture by simple aqueous extraction (Davidson et al., 2021), thus making the subsequent purification phase particularly simple while significantly reducing the volume of organic solvents and chromatographic materials. These aspects are particularly interesting, considering that the purification step often represents the most critical phase for the sustainability of the process (Duarte et al., 2015).
In view of this background and the biological importance of N-acyl tryptamines, in this study, we report a simple one-pot methodology for the synthesis of this class of molecules, using T3P as a coupling reagent and minimal volumes of non-halogenated solvents (for the reaction, workup, and purification steps).
2 Methods
2.1 General
All reagents and solvents (HPLC grade) were used without further purification. Reactions were monitored by thin-layer chromatography (TLC) on Merck silica gel plates (0.25 mm) and visualized using UV light at 254 nm and a cerium sulfate reagent. Column chromatographic purification of products was carried out using silica gel 60 (particle size: 0.040–0.063 mm; Merck). 1H NMR and 13C NMR spectra were recorded on a Bruker DRX 600 spectrometer equipped with an inverse TCI CryoProbe at room temperature (RT) in CDCl3. Chemical shifts are reported in ppm, and multiplicities are indicated by s (singlet), d (doublet), t (triplet), q (quartet), m (multiplet), and br (broad). Coupling constants (J) are reported in Hz. Yields are given for isolated products showing one spot on a TLC plate and no impurities detectable in the NMR spectrum. Spectroscopic characterizations of products 3aa (Schmidt et al., 2010), 3ba (Schmidt et al., 2010), 3ea (Sládeková et al., 2023), 3da (Singh and Singh, 2009), 3eb (Takao et al., 2015), and 3be (Blickenstaff et al., 1994) matched those reported in the literature.
2.2 Synthetic procedures and characterization of products
2.2.1 General procedure for the synthesis of N-acyl tryptamines 3
In an 8-mL vial, tryptamine 2 (1.2 eq, 0.12 mmol), carboxylic acid 1 (0.1 mmol), Et3N (2.0 eq, 0.2 mmol), T3P (50 wt% solution in EtOAc, 1.5 eq, 0.15 mmol), and EtOAc (60 µL) were added. The resulting mixture was stirred for 24 h at room temperature, and then, it was quenched by the addition of H2O (2 mL) and EtOAc (2 mL). The phases were separated, and the H2O phase was extracted with EtOAc (2 × 2 mL). The combined organic phase was dried (Na2SO4) and concentrated. The residue was purified using a short pad of silica gel (petroleum ether/EtOAc: 7/3–1/1) to yield products 3. All new compounds were fully characterized.
2.2.2 General procedure for the synthesis of N-acyl tryptamine 3aa under mechanochemical conditions
In an 8-mL vial, tryptamine 2a (1.2 eq, 0.12 mmol), carboxylic acid 1a (0.1 mmol), Et3N (2.0 eq, 0.2 mmol), T3P (50 wt% solution in EtOAc, 1.5 eq, 0.15 mmol), and two stainless steel balls (diameter: 5 mm) were added. The resulting mixture was ground at 2,500 rpm for 10 min at RT using a vortex mixer (IKA Vortex Genius 3, speed 6), and then, the vial was left closed at RT for an additional 10 min before the usual workup with H2O (1 mL) and EtOAc (1 mL). The phases were separated, and the H2O phase was extracted with EtOAc (2 × 1 mL). The combined organic phase was dried (Na2SO4) and concentrated. The residue was purified using a short pad of silica gel (petroleum ether/EtOAc: 7/3–1/1) to yield product 3aa.
N-(2-(1H-indol-3-yl)ethyl)dodecanamide or N-lauroyl tryptamine (3ca). Prepared according to the general procedure from 1c and 2a. Colorless amorphous solid, 27.4 mg (0.08 mmol), and 80% yield. Rf value: 0.1 (petroleum ether/EtOAc: 7/3). 1H NMR (CDCl3, 600 MHz) δ: 8.1 (bs, 1H), 7.61 (d, J = 7.9 Hz, 1H), 7.38 (d, J = 8.2 Hz, 1H), 7.21 (t, J = 7.6 Hz, 1H), 7.13 (t, J = 7.6 Hz, 1H), 7.04 (bs, 1H), 5.48 (bs, 1H), 3.61 (q, J = 6.2 Hz, 2H), 2.98 (t, J = 6.7 Hz, 2H), 2.09 (t, J = 7.5 Hz, 2H), 1.61–1.54 (m, overlapping H2O residual peak, 2H), 1.31–1.21 (m, 16H), and 0.88 (t, J = 6.8 Hz, 3H). 13C NMR (CDCl3, 150 MHz) δ: 173.1, 136.4, 127.4, 122.3, 122.0, 119.5, 118.8, 113.2, 111.2, 39.6, 36.9, 31.9, 29.6, 29.5, 29.4, 29.3, 25.7, 25.4, 22.7, and 14.1.
N-(2-(1H-indol-3-yl)ethyl)arachidonamide or N-arachidonoyl tryptamine (3fa). Prepared according to the general procedure from 1f and 2a. Pale yellow oil, 23.0 mg (0.055 mmol). Rf value: 0.1 (petroleum ether/EtOAc: 7/3). 1H NMR (CDCl3, 600 MHz) δ: 8.20 (bs, 1H), 7.61 (d, J = 7.9 Hz, 1H), 7.38 (d, J = 8.1 Hz, 1H), 7.21 (t, J = 7.2 Hz, 1H), 7.13 (t, J = 7.3 Hz, 1H), 7.03 (bs, 1H), 5.52 (bs, 1H), 5.42–5.31 (m, 8H), 3.61 (q, J = 6.6 Hz, 2H), 2.98 (t, J = 6.8 Hz, 2H), 2.85–2.76 (m, 6H), 2.14–2.02 (m, 6H), 1.68 (m, 2H), 1.38–1.26 (m, 6H), and 0.89 (t, J = 6.4 Hz, 3H). 13C NMR (CDCl3, 150 MHz) δ: 172.9, 136.4, 130.5, 129.2, 128.7, 128.6, 128.3, 128.2, 127.9, 127.5, 127.4, 122.2, 122.0, 119.5, 118.7, 113.0, 111.3, 39.7, 36.2, 31.5, 29.3, 27.2, 26.7, 25.6, 25.5, 25.4, 22.6, and 14.1.
N-(2-(5-hydroxy-1H-indol-3-yl)ethyl)palmitamide or N-palmitoyl serotonin (3bb). Prepared according to the general procedure from 1b and 2b. Colorless amorphous solid, 15.6 mg (0.038 mmol). Rf value: 0.1 (petroleum ether/EtOAc: 7/3). 1H NMR (CDCl3, 600 MHz) δ: 7.88 (bs, 1H), 7.23 (d, J = 8.6 Hz, 1H), 7.04 (d, J = 2.3 Hz, 1H), 7.01 (d, J = 2.2 Hz, 1H), 6.79 (dd, J = 8.6, 2.4 Hz, 1H), 5.50 (bs, 1H), 4.89 (bs, 1H), 3.57 (q, J = 6.7 Hz, 2H), 2.90 (t, J = 6.9 Hz, 2H), 2.11 (t, J = 7.5 Hz, 2H), 1.60–1.55 (m, overlapping H2O residual peak, 2H), 1.31–1.21 (m, 24H), and 0.88 (t, J = 6.8 Hz, 3H). 13C NMR (CDCl3, 150 MHz) δ: 173.2, 149.6, 131.6, 128.1, 123.0, 112.5, 112.1, 111.9, 103.3, 39.5, 36.9, 31.9, 29.7, 29.6, 29.5, 29.4, 29.3, 25.8, 25.5, 22.7, and 14.1.
N-(2-(5-methyl-1H-indol-3-yl)ethyl)palmitamide or N-palmitoyl 5-methyltryptamine (3bc). Prepared according to the general procedure from 1b and 2c. Colorless amorphous solid, 34.3 mg (0.083 mmol). Rf value: 0.1 (petroleum ether/EtOAc: 7/3). 1H NMR (CDCl3, 600 MHz) δ: 7.96 (bs, 1H), 7.39 (bs, 1H), 7.27 (d, overlapping solvent peak, 1H), 7.04 (d, J = 8.2 Hz, 1H), 6.99 (d, J = 2.0 Hz, 1H), 5.48 (bs, 1H), 3.59 (q, J = 6.5 Hz, 2H), 2.94 (t, J = 6.7, 2H), 2.46 (s, 3H), 2.09 (t, J = 7.5 Hz, 2H), 1.66–1.52 (m, overlapping H2O residual peak, 2H), 1.30–1.20 (m, 24H), and 0.88 (t, J = 6.8 Hz, 3H). 13C NMR (CDCl3, 150 MHz) δ: 173.1, 134.7, 128.8, 127.6, 123.9, 122.1, 118.4, 112.7, 110.9, 39.7, 37.0, 31.9, 29.7, 29.67, 29.63, 29.5, 29.4, 29.3, 25.8, 25.4, 22.7, 21.5, and 14.1.
N-(2-(5-methyl-1H-indol-3-yl)ethyl)oleamide or N-oleoyl 5-methyltryptamine (3ec). Prepared according to the general procedure from 1e and 2c. Colorless wax, 35.1 mg (0.080 mmol). Rf value: 0.2 (petroleum ether/EtOAc: 7/3). 1H NMR (CDCl3, 600 MHz) δ: 8.25 (bs, 1H), 7.38 (bs, 1H), 7.26 ((d, J = 8.3 Hz, overlapping solvent peak, 1H), 7.03 (d, J = 8.2 Hz, 1H), 6.97 (bs, 1H), 5.58 (bs, 1H), 5.34 (m, 2H), 3.59 (q, J = 6.5 Hz, 2H), 2.94 (t, J = 6.7 Hz, 2H), 2.46 (s, 3H), 2.09 (t, J = 7.6 Hz, 2H), 2.01 (m, 4H), 1.58 (m, 2H), 1.36–1.23 (m, 20H), and 0.89 (t, J = 5.9 Hz, 3H). 13C NMR (CDCl3, 150 MHz) δ: 173.3, 134.8, 130.0, 129.8, 128.7, 127.6, 123.8, 122.2, 118.4, 112.4, 111.0, 39.8, 36.9, 31.9, 29.8, 29.7, 29.5, 29.3, 29.2, 27.24, 27.2, 25.8, 25.4, 22.7, 21.5, and 14.1.
N-(2-(5-fluoro-1H-indol-3-yl)ethyl)palmitamide or N-palmitoyl 5-fluorotryptamine (3bd). Prepared according to the general procedure from 1b and 2d. Amorphous solid, 30.8 mg (0.074 mmol). Rf value: 0.1 (petroleum ether/EtOAc 7/3). 1H NMR (CDCl3, 600 MHz) δ: 8.08 (bs, 1H), 7.28 (dd, J = 8.8, 4.3 Hz, 1H), 7.23 (dd, J = 9.5, 2.3 Hz, 1H), 7.08 (d, J = 1.9 Hz, 1H), 6.95 (td, J = 9.0, 2.4 Hz, 1H), 5.48 (bs, 1H), 3.58 (q, J = 6.7 Hz, 2H), 2.92 (t, J = 6.7 Hz, 2H), 2.11 (t, J = 7.6 Hz, 2H), 1.60–1.55 (m, overlapping H2O residual peak, 2H), 1.29–1.23 (m, 24H), and 0.88 (t, J = 6.9 Hz, 3H). 13C NMR (CDCl3, 150 MHz) δ 173.2, 158.6, 157.0, 132.8, 127.8 (d, JC-F = 9.6 Hz), 123.7, 113.4, 111.8 (d, JC-F = 9.6 Hz), 110.6 (d, JC-F = 26.2 Hz), 103.6 (d, JC-F = 23.3 Hz), 39.5, 36.9, 31.9, 29.7, 29.66, 29.63, 29.5, 29.4, 29.3, 25.7, 25.4, 22.7, and 14.1.
N-(2-(5-fluoro-1H-indol-3-yl)ethyl)oleamide or N-oleoyl 5-fluorotryptamine (3ed). Prepared according to the general procedure from 1e and 2d. Amorphous solid, 24.7 mg (0.056 mmol). Rf value: 0.1 (petroleum ether/EtOAc: 7/3). 1H NMR (CDCl3, 600 MHz) δ: 8.26 (bs, 1H), 7.28 (dd, J = 8.8, 4.3 Hz, 1H), 7.23 (bd, J = 9.5 Hz, 1H), 7.07 (bs, 1H), 6.95 (bt, J = 8.9 Hz, 1H), 5.52 (bs, 1H), 5.34 (m, 2H), 3.58 (q, J = 6.5 Hz, 2H), 2.92 (t, J = 6.7 Hz, 2H), 2.11 (t, J = 7.6 Hz, 2H), 2.00 (m, 4H), 1.60–1.55 (m, 2H), 1.34–1.23 (m, 20H), and 0.88 (t, J = 6.9 Hz, 3H). 13C NMR (CDCl3, 150 MHz) δ: 173.2, 158.6, 157.0, 132.9, 130.0, 129.8, 127.8 (d, JC-F = 9.4 Hz), 123.8, 113.3, 111.9 (d, JC-F = 9.4 Hz), 110.6 (d, JC-F = 26.2 Hz), 103.6 (d, JC-F = 23.3 Hz), 39.6, 36.9, 31.9, 29.8, 29.7, 29.5, 29.3, 29.2, 29.1, 27.2, 27.18, 25.7, 25.4, 22.7, and 14.1.
N-(2-(5-methoxy-1H-indol-3-yl)ethyl)oleamide or N-oleoyl 5-methoxytryptamine (3ee). Prepared according to the general procedure from 1e and 2e. Amorphous solid, 33.8 mg (0.074 mmol). Rf value: 0.1 (petroleum ether/EtOAc: 7/3). 1H NMR (CDCl3, 600 MHz) δ: 8.11 (bs, 1H), 7.26 (d, J = 8.7 Hz, overlapping solvent peak, 1H), 7.03 (d, J = 2.3 Hz, 1H), 7.00 (bs, 1H), 6.87 (dd, J = 8.8, 2.3 Hz, 1H), 5.53 (bs, 1H), 5.34 (m, 2H), 3.86 (s, 3H), 3.60 (q, J = 6.5 Hz, 2H), 2.94 (t, J = 6.7, 2H), 2.10 (t, J = 7.5 Hz, 2H), 2.00 (m, 4H), 1.60–1.55 (m, 2H), 1.35–1.23 (m, 20H), and 0.88 (t, J = 6.8 Hz, 3H). 13C NMR (CDCl3, 150 MHz) δ: 173.2, 154.1, 131.5, 130.0, 129.8, 127.7, 122.8, 112.8, 112.4, 112.0, 100.5, 55.9, 39.5, 36.9, 31.9, 29.8,29.7, 29.5, 29.3, 29.28, 29.1, 27.2, 27.19, 25.8, 25.4, 22.7, and 14.1.
N-(2-(5-chloro-1H-indol-3-yl)ethyl)palmitamide or N-palmitoyl 5-chlorotryptamine (3bf). Prepared according to the general procedure from 1b and 2f. Amorphous solid, 31.0 mg (0.072 mmol). Rf value: 0.1 (petroleum ether/EtOAc: 7/3). 1H NMR (CDCl3, 600 MHz) δ: 8.12 (bs, 1H), 7.56 (d, J = 1.6 Hz, 1H), 7.29 (d, J = 8.6 Hz, 1H), 7.15 (dd, J = 8.6, 1.9 Hz, 1H), 7.06 (bd, J = 1.9 Hz, 1H), 5.47 (bs, 1H), 3.58 (q, J = 6.7 Hz, 2H), 2.93 (t, J = 6.7 Hz, 2H), 2.11 (t, J = 7.5 Hz, 2H), 1.60–1.55 (m, overlapping H2O residual peak, 2H), 1.30–1.23 (m, 24H), and 0.88 (t, J = 6.8 Hz, 3H). 13C NMR (CDCl3, 150 MHz) δ: 173.2, 134.7, 128.6, 125.3, 123.3, 122.5, 118.3, 113.1, 112.2, 39.6, 36.9, 31.9, 29.7, 29.66, 29.63, 29.5, 29.4, 29.3, 25.7, 25.3, 22.7, and 14.1.
N-(2-(5-chloro-1H-indol-3-yl)ethyl)oleamide or N-oleoyl 5-chlorotryptamine (3ef). Prepared according to the general procedure from 1e and 2f. Colorless oil, 35.1 mg (0.076 mmol). Rf value: 0.1 (petroleum ether/EtOAc: 7/3). 1H NMR (CDCl3, 600 MHz) δ: 8.51 (bs, 1H), 7.54 (bs, 1H), 7.27 (bd, J = 8.5 Hz, 1H), 7.13 (m, 1H), 7.02 (m, 1H), 5.58 (bs, 1H), 5.34 (m, 2H), 3.57 (q, J = 6.0 Hz, 2H), 2.91 (t, J = 6.2 Hz, 2H), 2.12 (t, J = 7.6 Hz, 2H), 2.00 (m, 4H), 1.61–1.55 (m, 2H), 1.35–1.23 (m, 20H), and 0.87 (t, J = 6.8 Hz, 3H). 13C NMR (CDCl3, 150 MHz) δ: 173.3, 134.7, 130.0, 129.8, 128.5, 125.1, 123.5, 122.4, 118.2, 112.3, 39.8, 36.9, 31.9, 29.8, 29.7, 29.5, 29.3, 29.2, 29.1, 27.2, 27.19, 25.8, 25.3, 22.7, and 14.1.
3 Results and discussion
In a preliminary study, stearic acid 1a (0.1 mmol) was chosen as the model substrate and subjected to a coupling reaction with tryptamine 2a (Scheme 2). The reaction was initially carried out for 24 h at RT, using 1 eq of T3P (commercially available as a 50 wt% solution in EtOAc) and adding a further small volume of EtOAc (60 µL) in order to solubilize the solid reagents and allow homogeneous mixing. The procedure was one-pot; hence, T3P was added together with the other reagents, without acid preactivation. Under these conditions, 3aa was obtained only in trace amounts (entry 1, Table 1), and a slight improvement in yield was observed using a small excess of tryptamine (entry 2, Table 1).
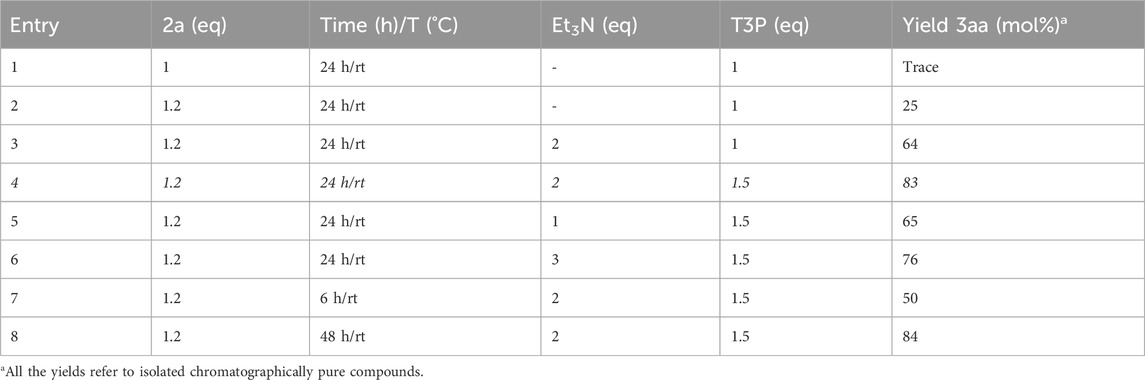
Table 1. Optimization of the T3P-assisted coupling reaction between stearic acid 1a and tryptamine 2a.
In agreement with the hypothesized mechanism (Scheme 3), a clear improvement in the reaction yield was observed by adding 2 eq of a base (Et3N), which was necessary to deprotonate carboxylic acid and start the reaction mechanism (entry 3). An excess of T3P significantly improved the efficiency of the reaction (entry 4), while no further improvement was observed by using a smaller (entry 5) or a larger (entry 6) amount of base, of which 2 eq was the optimal quantity. Finally, 24 h was necessary to obtain high yields of product 3aa (compare entry 4 with entries 7 and 8 in Table 1).
In order to test the general validity of the developed methodology, several carboxylic acids were submitted to the protocol using the optimized reaction conditions given in Table 1, entry 4.
As shown in Scheme 4, good results were obtained in 24 h at RT with short-, medium-, and long-chain (saturated, monounsaturated, and polyunsaturated) carboxylic acids, confirming the validity and generality of the developed methodology.
With the aim of extending the scope of the reaction, two bioavailable long-chain carboxylic acids (palmitic acid 1b and oleic acid 1e) were chosen as representative substrates, and the reaction was repeated with some tryptamine derivatives 2 exhibiting a substituent Rʹ at the 5-position (Scheme 5).
As shown in Scheme 5, most of the products were isolated with satisfactory yields. The worst efficiencies were obtained with serotonin 2b, which was probably due to the interference of the unprotected phenolic OH with T3P and, consequently, with the reaction mechanism.
These data further confirm the validity and generality of the developed methodology, which also provided interesting results using 5-substituted tryptamines 2, thus also allowing easy access to other classes of bioactive molecules, such as long-chain N-acyl serotonins (Ortar et al., 2007) (3bb and 3eb) or melatonins (Blickenstaff et al., 1994) (3be and 3ee).
It is important to note that, when using the 5-substituted tryptamine derivative 2 commercially available as hydrochloride salt (2b, 2c, 2e, and 2f), the best yields were obtained using 3.2 eq of Et3N instead of 2 eq, in order to balance the HCl derived from the starting material (as shown for the product 3bb in Scheme 5).
The impact of this new methodology for the synthesis of N-acyl tryptamines 3 can be better described by considering the importance that some products reported in this study have already proven to have. For example, N-lauroyl tryptamine 3ca (Scheme 4) was found to not only activate serotonin receptors but also inhibit EBI2 (or GPR183), a receptor associated with inflammatory bowel disease (Chang et al., 2021). N-oleoyl tryptamine 3ea (Scheme 4) was found to activate the pregnane X receptor (PXR), which is seemingly involved in several physiological responses (regulation of immune response, embryogenesis, metabolic disorders, etc.) (Sládeková et al., 2023). Furthermore, the bioactivities of some products given in Scheme 5 are described in the literature; for example, N-oleoyl serotonin 3eb inhibits capsaicin-induced TRPV1 channel activation (Ortar et al., 2007); N-palmitoyl serotonin 3bb inhibits L-dopa-induced dyskinesia in the mouse Parkinson model (Park et al., 2016); finally, for homologs of melatonin, such as N-palmitoyl 5-methoxytryptamine 3be, radioprotective activity was highlighted (Blickenstaff et al., 1994).
Finally, the model reaction between stearic acid 1a and tryptamine 2a was repeated under mechanochemical conditions (Fantozzi et al., 2023) (Scheme 6). Mechanochemistry explores the possibility of inducing chemical reactions through mechanical forces, and recently, the IUPAC included it in the list of 10 chemical innovations that could change the world (https://www.chemistryworld.com/news/iupac-names-10-chemistry-innovations-that-will-change-the-world/3010335.article). In 2012, Stojakovic et al. (Stojakovic et al., 2012) demonstrated that an automated grinding method based on a vortex mixer could replace manual mortar-and-pestle grinding (a time-consuming and impractical procedure) and allow for the realization of processes under mechanochemical conditions.
When using this approach, all solid reagents were added to a transparent borosilicate vial with two stainless steel beads (diameter: 5 mm) in the presence of T3P (1.5 eq) and Et3N (2 eq), without adding a further solvent, and the non-homogeneous mixture was mixed for 10 min at RT using a vortex mixer (commonly used for the preparation of biological samples).
At the end of the process, the mixture appeared homogeneous, thanks to the mechanical stress produced by the grinding in the vortex due to the collisions among reagents, beads, and vial. This alternative procedure provided product 3aa in only few minutes with a yield of 78%, comparable to the yield obtained with the conventional methodology under the optimized conditions (83% in 24 h/RT, as reported in entry 4, Table 1), with a significant reduction in reaction times and energy consumption.
To the best of our knowledge, this is the first synthesis of N-stearoyl tryptamine 3aa under mechanochemical conditions. Starting from this very encouraging preliminary result, further experiments for the development of a general mechanochemical methodology for N-acyl tryptamines 3 are in progress. In particular, a scrupulous screening of the experimental conditions will be carried out, with the aim of reducing excess reagents and improving the yields and “greenness” associated with the methodology, further considering the biological importance of the products with this scaffold and the need to elaborate new sustainable protocols for their production. For this purpose, more sustainable bases will also be tested as an alternative to Et3N, and the best reaction conditions will finally be applied to the reaction with different carboxylic acids 1 and tryptamine derivatives 2, using alternative milling devices.
4 Conclusions
In conclusion, a simple and effective methodology for the synthesis of an interesting class of gut microbiota-derived endocannabinoid-like lipid mediators, called N-acyl tryptamines, was proposed. The reaction was promoted by T3P, a sustainable coupling reagent that allowed the realization of a one-pot process, without preactivation of carboxylic acid. A key advantage of this methodology was the use of limited amounts of organic solvents in each phase of the process (reaction, workup, and purification) and no halogenated solvent. Furthermore, the reactions were carried out at room temperature, without the use of an inert atmosphere. The by-products of T3P were highly soluble in water, so they could be completely removed from the reaction mixture by a simple aqueous extraction, thus also making the subsequent purification phase particularly simple and with reduced environmental impact. Finally, the possibility of carrying out the reaction under mechanochemical conditions was explored with interesting prospects for a future development, thanks to the drastic reduction in reaction times and energy consumption.
Data availability statement
The original contributions presented in the study are included in the article further inquiries can be directed to the corresponding author.
Author contributions
RV: Conceptualization, Data curation, Formal Analysis, Funding acquisition, Investigation, Methodology, Project administration, Resources, Validation, Visualization, Writing–original draft. VD: Conceptualization, Funding acquisition, Project administration, Writing–review and editing.
Funding
The authors declare that financial support was received for the research, authorship, and/or publication of this article. The authors acknowledge the financial support from the Unité Mixte Internationale (UMI) for Chemical and Biomolecular Research on the Microbiome and its impact on Metabolic Health and Nutrition (MicroMeNu), which is partly funded by the Sentinelle Nord project, supported by the Apogée (Canada First) program from the Federal Tri-Agency of Canada.
Conflict of interest
The authors declare that the research was conducted in the absence of any commercial or financial relationships that could be construed as a potential conflict of interest.
The author(s) declared that they were an editorial board member of Frontiers, at the time of submission. This had no impact on the peer review process and the final decision.
Publisher’s note
All claims expressed in this article are solely those of the authors and do not necessarily represent those of their affiliated organizations, or those of the publisher, the editors, and the reviewers. Any product that may be evaluated in this article, or claim that may be made by its manufacturer, is not guaranteed or endorsed by the publisher.
References
Ábrányi-Balogh, P., Földesi, T., Grün, A., Volk, B., Keglevich, G., and Milen, M. (2016). Synthetic study on the T3P®-promoted one-pot preparation of 1-substituted-3,4-dihydro-β-carbolines by the reaction of tryptamine with carboxylic acids. Tetrahedron Lett. 57, 1953–1957. doi:10.1016/j.tetlet.2016.03.067
Al Musaimi, O., Wisdom, R., Talbiersky, P., De La Torre, B. G., and Albericio, F. (2021). Propylphosphonic anhydride (T3P®) as coupling reagent for solid-phase peptide synthesis. ChemistrySelect 6, 2649–2657. doi:10.1002/slct.202100123
Bartolucci, S., Mari, M., Bedini, A., Piersanti, G., and Spadoni, G. (2015). Iridium-catalyzed direct synthesis of tryptamine derivatives from indoles: exploiting N-protected β-amino alcohols as alkylating agents. J. Org. Chem. 80, 3217–3222. doi:10.1021/acs.joc.5b00195
Bartolucci, S., Mari, M., Di Gregorio, G., and Piersanti, G. (2016). Observations concerning the synthesis of tryptamine homologues and branched tryptamine derivatives via the borrowing hydrogen process: synthesis of psilocin, bufotenin, and serotonin. Tetrahedron 72, 2233–2238. doi:10.1016/j.tet.2016.03.007
Basavaprabhu, B., Vishwanatha, T. M., Panguluri, N. R., and Sureshbabu, V. V. (2013). Propanephosphonic acid anhydride (T3P®) - a benign reagent for diverse applications inclusive of large-scale synthesis. Synthesis 45, 1569–1601. doi:10.1055/s-0033-1338989
Blickenstaff, R. T., Brandstadter, S. M., Reddy, S., and Witt, R. (1994). Potential radioprotective agents. 1. Homologs of melatonin. J. Pharm. Sci. 83, 216–218. doi:10.1002/jps.2600830220
Chang, F.-Y., Siuti, P., Laurent, S., Williams, T., Glassey, E., Sailer, A. W., et al. (2021). Gut-inhabiting Clostridia build human GPCR ligands by conjugating neurotransmitters with diet- and human-derived fatty acids. Nat. Microbiol. 6, 792–805. doi:10.1038/s41564-021-00887-y
Davidson, A., Foley, D. A., Frericks-Schmidt, H., Ruggeri, S. G., Herman, M., LaCasse, S., et al. (2021). pH-dependent degradation of T3P-related byproducts. Org. Process Res. Dev. 25, 621–626. doi:10.1021/acs.oprd.0c00431
Di Marzo, V. (2020). The endocannabinoidome as a substrate for noneuphoric phytocannabinoid action and gut microbiome dysfunction in neuropsychiatric disorders. Dialogues Clin. Neurosci. 22, 259–269. doi:10.31887/dcns.2020.22.3/vdimarzo
Duarte, R. C. C., Ribeiro, M. G. T. C., and Machado, A. A. S. C. (2015). Using green star metrics to optimize the greenness of literature protocols for syntheses. J. Chem. Educ. 92, 1024–1034. doi:10.1021/ed5004096
Espinoza-Moraga, M., Petta, T., Vasquez-Vasquez, M., Laurie, V. F., Moraes, L. A. B., and Silva Santos, L. (2010). Bioreduction of β-carboline imines to amines employing Saccharomyces bayanus. Tetrahedron Asymmetry 21, 1988–1992. doi:10.1016/j.tetasy.2010.06.036
Fantozzi, N., Volle, J.-N., Porcheddu, A., Virieux, D., Garcıa, F., and Colacino, E. (2023). Green metrics in mechanochemistry. Chem. Soc. Rev. 52, 6680–6714. doi:10.1039/d2cs00997h
Hahn, G., and Friedel Gudjons, H. (1938). Synthese von 3-alkyl-4-carbolinen und 3.3′-polymethylen-di-4-carbolinen. Chem. Berichte 71, 2175–2182. doi:10.1002/cber.19380711025
Horváth, I. T., and Anastas, P. T. (2007). Innovations and green chemistry. Chem. Rev. 107, 2169–2173. doi:10.1021/cr078380v
Isidro-Llobet, A., Kenworthy, M. N., Mukherjee, S., Kopach, M. E., Wegner, K., Gallou, F., et al. (2019). Sustainability challenges in peptide synthesis and purification: from R&D to production. J. Org. Chem. 84, 4615–4628. doi:10.1021/acs.joc.8b03001
Jordan, A., Whymark, K. D., Sydenham, J., and Sneddon, H. F. (2021). A solvent-reagent selection guide for Steglich-type esterification of carboxylic acids. Green Chem. 23, 6405–6413. doi:10.1039/d1gc02251b
Ki, D.-W., El-Desokya, A. H., Kodama, T., Wong, C. P., Ghani, M. A., El-Beih, A. A., et al. (2020). New cytotoxic polyacetylene amides from the Egyptian marine sponge Siphonochalina siphonella. Fitoterapia 142, 104511. doi:10.1016/j.fitote.2020.104511
Li, C.-J., and Trost, B. M. (2008). Green chemistry for chemical synthesis. PNAS 105, 13197–13202. doi:10.1073/pnas.0804348105
Mattellone, A., Corbisiero, D., Cantelmi, P., Martelli, G., Palladino, C., Tolomelli, A., et al. (2023b). Fast solution-phase and liquid-phase peptide syntheses (SolPSS and LPPS) mediated by biomimetic cyclic propylphosphonic anhydride (T3P®). Molecules 28, 7183. doi:10.3390/molecules28207183
Mattellone, A., Corbisiero, D., Ferrazzano, L., Cantelmi, P., Martelli, G., Palladino, C., et al. (2023a). Speeding up sustainable solution-phase peptide synthesis using T3P® as a green coupling reagent: methods and challenges. Green Chem. 25, 2563–2571. doi:10.1039/d3gc00431g
Nagao, Y., Seno, K., Kawabata, K., Miyasaka, T., Takao, S., and Fujita, E. (1980). Monitored aminolysis of 3-acylthiazolidine-2-thione: a new convenient synthesis of amide. Tetrahedron Lett. 21, 841–844. doi:10.1016/s0040-4039(00)71520-6
Nicolaou, K. C. (2014). Organic synthesis: the art and science of replicating the molecules of living nature and creating others like them in the laboratory. Proc. R. Soc. A 470, 20130690. doi:10.1098/rspa.2013.0690
Ortar, G., Cascio, M. G., De Petrocellis, L., Morera, E., Rossi, F., Schiano-Moriello, A., et al. (2007). New N-arachidonoyl serotonin analogues with potential “dual” mechanism of action against pain. J. Med. Chem. 50, 6554–6569. doi:10.1021/jm070678q
Park, H.-Y., Ryu, Y.-K., Go, J., Son, E., Kim, K.-S., and Kim, M. R. (2016). Palmitoyl serotonin inhibits L-dopa-induced abnormal involuntary movements in the mouse Parkinson model. Exp. Neurobiol. 25, 174–184. doi:10.5607/en.2016.25.4.174
Roth, W., Zadeh, K., Vekariya, R., Ge, Y., and Mohamadzadeh, M. (2021). Tryptophan metabolism and gut-brain homeostasis. Int. J. Mol. Sci. 22, 2973. doi:10.3390/ijms22062973
Schmidt, F., Le Douaron, G., Champy, P., Amar, M., Séon-Méniel, B., Raisman-Vozari, R., et al. (2010). Tryptamine-derived alkaloids from Annonaceae exerting neurotrophin-like properties on primary dopaminergic neurons. Bioorg. Med. Chem. 18, 5103–5113. doi:10.1016/j.bmc.2010.05.067
Singh, K., and Singh, K. (2009). N1,N3-Diacyl-3,4-dihydropyrimidin-2(1H)-ones: neutral acyl group transfer reagents. Tetrahedron 65, 10395–10399. doi:10.1016/j.tet.2009.10.037
Sládeková, L., Zgarbová, E., Vrzal, R., Vanda, D., Soural, M., Jakubcová, K., et al. (2023). Switching on/off aryl hydrocarbon receptor and pregnane X receptor activities by chemically modified tryptamines. Toxicol. Lett. 387, 63–75. doi:10.1016/j.toxlet.2023.09.012
Stojakovic, J., Farris, B. S., and MacGillivray, L. R. (2012). Vortex grinding for mechanochemistry: application for automated supramolecular catalysis and preparation of a metal–organic framework. Chem. Commun. 48, 7958–7960. doi:10.1039/c2cc33227b
Takao, K., Noguchi, K., Hashimoto, Y., Shirahata, A., and Sugita, Y. (2015). Synthesis and evaluation of fatty acid amides on the N-oleoylethanolamide-like activation of peroxisome proliferator activated receptor α. Chem. Pharm. Bull. 63, 278–285. doi:10.1248/cpb.c14-00881
Verhoeckx, K. C. M., Voortman, T., Balvers, M. G. J., Hendriks, H. F. J., Wortelboer, H. M., and Witkamp, R. F. (2011). Presence, formation and putative biological activities of N-acyl serotonins, a novel class of fatty-acid derived mediators, in the intestinal tract. Biochimica Biophysica Acta 1811, 578–586. doi:10.1016/j.bbalip.2011.07.008
Villano, R., Tinto, F., and Di Marzo, V. (2022). Facile and sustainable synthesis of commendamide and its analogues. Front. Chem. 10, 858854. doi:10.3389/fchem.2022.858854
Wehrstedt, K. D., Wandrey, P. A., and Heitkamp, D. (2005). Explosive properties of 1-hydroxybenzotriazoles. J. Hazard. Mater. A126, 1–7. doi:10.1016/j.jhazmat.2005.05.044
Keywords: N-acyl tryptamines, microbiota, green chemistry, organic synthesis, propylphosphonic anhydride
Citation: Villano R and Di Marzo V (2024) A sustainable protocol for the synthesis of N-acyl tryptamines, a class of potential gut microbiota-derived endocannabinoid-like mediators. Front. Chem. 12:1436008. doi: 10.3389/fchem.2024.1436008
Received: 21 May 2024; Accepted: 18 September 2024;
Published: 10 October 2024.
Edited by:
Tamer S. Saleh, National Research Centre, EgyptReviewed by:
Michele Mari, University of Urbino Carlo Bo, ItalyMohmmad Younus Wani, Jeddah University, Saudi Arabia
Copyright © 2024 Villano and Di Marzo. This is an open-access article distributed under the terms of the Creative Commons Attribution License (CC BY). The use, distribution or reproduction in other forums is permitted, provided the original author(s) and the copyright owner(s) are credited and that the original publication in this journal is cited, in accordance with accepted academic practice. No use, distribution or reproduction is permitted which does not comply with these terms.
*Correspondence: Rosaria Villano, cm9zYXJpYS52aWxsYW5vQGljYi5jbnIuaXQ=