- 1Hainan Women and Children’s Medical Center, Haikou, China
- 2Hainan General Hospital/Hainan Affiliated Hospital of Hainan Medical University, Haikou, China
- 3NHC (National Health Commission of the People’s Republic of China) Key Laboratory of Tropical Disease Control, School of Tropical Medicine, Hainan Medical University, Haikou, Hainan, China
Numerous local herbal extract species have been investigated as potential medicinal ingredients due to their promising anti-cancer properties. However, the primary constraint of the class of plant flavonoids lies in their low solubility and limited membrane permeability, leading to chemical instability and restricted bioavailability that impede biomedical applications. In this study, we have developed an ideal nanozyme-Galazyme, comprising galangin-loaded copper Nanozyme coated by DSPE-PEG, which amplifies oxidative stress to induce apoptosis via the regulation of reactive oxygen species (ROS) generation and mitogen-activated protein kinase (MAPK) activation. Galazyme exhibited significant peroxidase mimetic activity, demonstrating its potential to generate ROS and elevate oxidative stress. Upon uptake by HepG-2 cells, Galazyme efficiently converts excess hydrogen peroxide (H2O2) into highly reactive •OH radicals and upregulates MAPK expression, leading to the activation of Bax and Caspase 3, thereby promoting irreversible tumor cell apoptosis. Both in vitro and in vivo results demonstrate that Galazyme inhibits tumor cell growth and induces apoptosis by generating ample ROS and activating the MAPK pathway. Our study offers novel evidence supporting the enhancement of Galazyme-induced apoptosis through the upregulation of Bax and Caspase 3, along with the elucidation of the interaction between MAPK and apoptosis.
1 Introduction
Flavonoids, as one of the most common herbal metabolites, have garnered significant attention in the treatment of various diseases (Ren et al., 2003; Kandaswami et al., 2005; Zhu et al., 2024a; Chen et al., 2024). Flavonoids and derivatives have been demonstrated to possess a range of biological properties, including antitumor, antioxidant, antibacterial, and anti-inflammatory activities (Cushnie and Lamb, 2005; Farhadi et al., 2019; Zhu et al., 2024b). Interestingly, numerous preclinical studies have shown that flavonoids can modulate various forms of regulated cell death (RCD) (Wu et al., 2019; Wu et al., 2022). Regulated cell deaths (RCDs), such as pyroptosis, apoptosis, necroptosis, and ferroptosis, play crucial roles in different stages of disease progression and are closely linked to treatment strategies (Tang et al., 2019; Niu et al., 2023). Galangin (3,5,7-trihydroxyflavone) is regarded as the bioactive constituent of galangal, a perennial plant native of Indonesia but cultivated in many parts of Asia. The anticancer effects of galangin are mostly due to its abilities to inhibit cell cycle progression, inhibiting mitogen-activated protein kinase (MAPK) (Liu et al., 2015), protein kinase B (Akt) (Li et al., 2018), or mammalian target of rapamycin (mTOR) activity thereby inducing apoptotic cell death by activating caspase-9/-8/-3 and inhibiting tumor invasion and metastasis by reducing the upregulation of matrix metalloproteinase-2/-9 (Kong et al., 2019; Wu et al., 2024). These molecular pathways regulated by galangin are involved in suppressing different malignancies, such as lung cancer, hepatic cancer, ovarian cancer, breast cancer, gastric cancer, colorectal cancer, retinoblastoma, and osteosarcoma (Liang et al., 2021). Based on the effective tumor intervention, the rational development of new drug formulations related to galangin is of great significance in overcoming the challenges of RCD-related tumor treatment.
Nanozyme, nanoparticle endowed with inherent enzyme-mimic activities, has motivated the rapid advancement for novel tumor therapy strategy (Huang Y. et al., 2019; Zhu et al., 2021a; Tang et al., 2021; Zhu D. et al., 2022; Zhu et al., 2023). Nanozyme emerge as a promising candidate for regulating cellular bioprocesses due to their satisfactory enzymatic activities, excellent stability, cost-effectiveness, and superior membrane permeability compared to natural enzymes (Zhu et al., 2021b; Xu et al., 2022; Wang et al., 2023). A great deal of nanozymes have been reported to display impressive catalytic performances for cancer therapy (Jiang et al., 2019; Zhu Y. et al., 2021). Significantly, nanozymes with peroxidase-catalytic activity (POD-like activity), such as copper nanozymes (Zhu Y. et al., 2022; Niu et al., 2023), single atom nanozymes, manganese nanozymes, and iron nanozymes (Huang L. et al., 2019; Cheng et al., 2021), have been employed to generate sufficient reactive oxygen species (ROS) to destroy tumor cells. Chen et al. present a chemodynamic nanomedicine, comprising oleanolic acid-loaded iron single atom nanozyme coated by red cell membrane, which enhances lipid peroxidation to boost anticancer efficacy via the regulation of cell membrane unsaturation. Therefore, nanozyme can serve as a potential therapeutic agent for conquering clinical cancer treatment challenges.
In this study, we developed an impressive nanozyme-Galazyme (Scheme 1), comprising galangin-loaded copper Nanozyme coated by DSPE-PEG, which strengthens oxidative stress to induce apoptosis by regulating ROS initiation and MAPK activation. Galazyme exhibited significant peroxidase mimetic activity, demonstrating its potential to generate ROS and elevate oxidative stress. Upon uptake by cancer cells, Galazyme not only catalytically converted overproduced hydrogen peroxide (H2O2) into highly active •OH but also activated the MAPK pathway, leading to the upregulation of Bax and Caspase 3, thereby promoting irreversible apoptosis. Both in vitro and in vivo results demonstrated that Galazyme suppressed tumor cell growth and induced tumor apoptosis through initiating ROS and activating MAPK pathway. Our work offers a new proof-of-concept to construct natural product Nanozymes which enhance apoptosis via upregulating Bax and Caspase three while establishing MAPK and apoptosis interaction.
2 Materials and methods
2.1 Materials
Galangin was purchased from MedChemExpress (MCE, China). Dimethylimidazole, copper acetylacetonate, and zinc nitrate hexahydrate were bought from Sigma. Hoechst, Annexin V-FITC, propidium iodide (PI), 2′,7′-dichlorofluorescin diacetate (DCFH-DA) and cell count kit-8 (CCK-8), were bought from Beyotime (Shanghai, China). Paraformaldehyde (PFA), Tween 20, Triton X-100 were purchased from Sinopharm Chemical Reagents (Shanghai, China). Mycoplasma Removal Agent (cat #60703) were purchased from Yeasen, Shanghai, China. Deionized (DI) water was obtained from a Milli-Q water purification system.
2.2 Synthesis of galazyme
Solution A consisted of 2.36 g of dimethylimidazole and 200 mg of copper acetylacetonate dissolved in 80 mL of methyl alcohol, while solution B comprised 1.07 g of zinc nitrate hexahydrate dissolved in 80 mL of methyl alcohol. Solution B was then slowly added to solution A under magnetic stirring and mixed for 6 h. The resulting product was collected by centrifugation and washed with methyl alcohol three times. Finally, the product underwent vacuum drying at 60°C. Subsequently, the product was subjected to pyrolysis at 600°C for 2 h to yield Nanozyme.
The 10 mg Nanozyme was dissolved in 20 mL of 20 mL DD H2O. Separately, 2 mg of galangin was dissolved in 1 mL of dimethyl sulfoxide (DMSO). The galangin-containing DMSO solution was then added to the Nanozyme solution. Then, the Galazyme was collected by centrifugation and washed with PBS for three times.
2.3 Drug loading and entrapment efficiency
The galangin were loaded into Nanozyme with a simple impregnation method under magnetic stirring. Then, it was subjected to ultracentrifugation for 2 h at 6,000 r. The amount of drug load and entrapment efficiency (EE) within EVs were quantified using a spectrophotometer (UV-2600, SHIMADZU, Kyoto, Japan). The EE was calculated according to the following Equations:
2.4 Cellar uptake
HepG2 cells were seeded in confocal dishes for 12 h. After 4 h of incubation with Galazyme, tumor cells were collected and the content was measured by high-performance liquid chromatography (HPLC).
2.5 Proliferation assay
Cell viability was measured by the CCK-8 assay. HepG2 cells were planted for 24 h. Then, the cells were incubated with various concentrations of Galazyme. After treatment for 24 h, the medium was replaced with fresh medium containing 10 μL CCK-8 and quantified by the absorbance at 450 nm using a microplate reader.
2.6 Reactive oxygen species (ROS) generation
HepG2 cells were seeded in confocal dish. The cells were treated with different formulations for 4 h. Then, the cells were co-stained with DCFH-DA (10 μM) and Hoechst (10 μM). The fluorescence imaging of cells was imaged by confocal microscopy.
2.7 In vivo anti-tumor assay
Animal experiments were performed according to the protocol approved by The Ethical Committee of Hainan Medical University. HepG2 tumor-bearing mice were randomly divided into four groups (5 mice per group) and intravenously administrated with 5 mg/kg Galazyme for 3 times every 3 days. After 14 days of treatment, the mice were euthanatized for histological examination.
2.8 Statistical analysis
Data represent the mean ± s.d. From indicated independent replicates. Statistical analysis was conducted using GraphPad Prism. For comparisons between two groups, means were compared using the unpaired two-tailed Student’s t-test. A value of p < 0.05 was considered statistically significant.
3 Results and discussion
3.1 Synthesis and characterization of galazyme
In this study, ZIF-8 nanoparticles, with a particle size of approximately 40 nm, were fabricated using a Host-Guest Template and observed via transmission electron microscopy (TEM) (Figure 1A). The TEM image revealed that there are numerous pores on the surface of Nanozyme, attributing to the efficient loading of the galangin (Figure 1B). The zeta-potential of the nanoparticles was measured as ZIF-8: 21.3 mV, Galazyme: 20.5 mV. The long-term measurement of the stability was studied by dynamic light scattering (DLS). The DLS result showed that the diameter of Galazyme remained stable for 48 h, which indicated the stability of Galazyme in biological environments (Supplementary Figure S1). No apparent crystal peak was noticed in the X-ray powder diffraction (XRD) spectrum, implying the poor crystallinity of Cu element in Galazyme (Figure 1C). And the X-ray photoelectron spectroscopy (XPS) was selected to measure the Cu valences in Galazyme. As shown in Figure 1D, Cu 2p XPS spectrum showed that two characteristic Cu peaks at Cu2+ (937.5 eV) and Cu+ 954.1 eV, implying the remarkable Fenton reactivity of Galazyme. In addition, the Fenton activity of Galazyme was assessed using 3,3′,5,5′-tetramethylbenzidine (TMB) as a chromogenic substrate. As shown in Figure 1E, Galazyme converted TMB into oxidized TMB (ox-TMB) with absorbance at 652 nm and acidic pH in the presence of hydrogen peroxide (H2O2), implying the pH-dependent catalytic activity of Galazyme. The peroxidase (POD) activity of Galazyme in different pH were studied by UV-vis. The results demonstrated that Galazyme can convert TMB into oxidized TMB (ox-TMB) under acidic pH while no obvious chromogenic reaction was observed at neutral pH 7.4, indicating that Galazyme exhibited a pH-dependent Fenton reaction to produce plentiful ROS (Supplementary Figure S2). Furthermore, the Fenton activity of Galazyme exhibited a concentration-dependent manner. Moreover, 5,5-Dimethyl-1-pyrroline N-oxide (DMPO), a spin trapper reacting with the intermediate •OH, was employed as the •OH product indicator. Electron spin resonance (ESR) spectrum demonstrated a characteristic quartet signal (1:2:2:1) of DMPO/•OH adduct when Galazyme was treated with H2O2 at acidic pH (Figure 1F). These finding confirmed the catalytic activity of Galazyme and its enhanced potential to initiate a ROS storm.
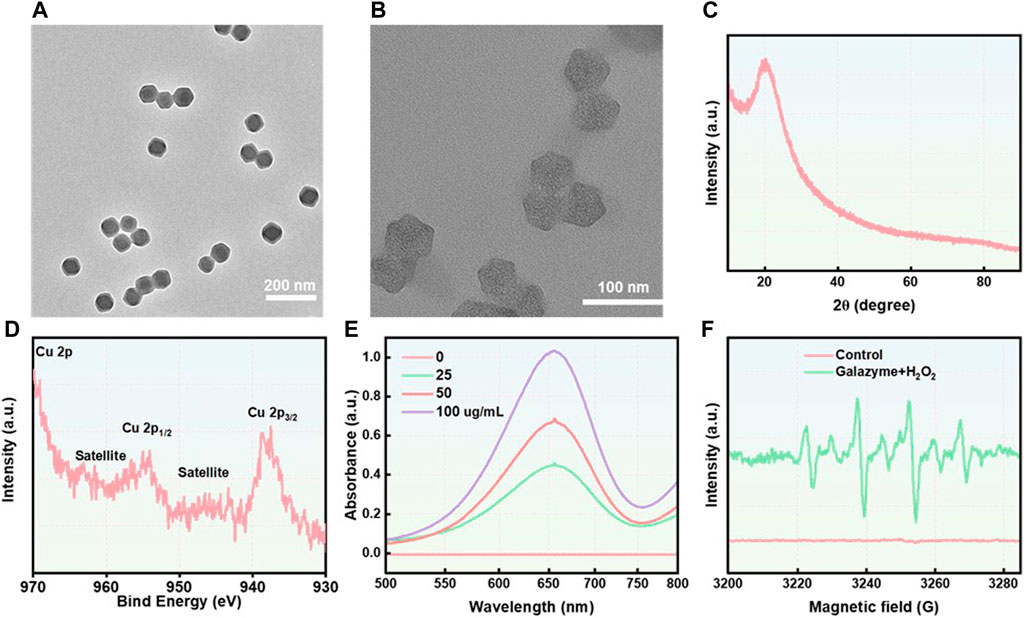
Figure 1. (A) The TEM image of ZIF-8. Scale ber: 200 nm. (B) The TEM image of Nanozyme. Scale ber: 100 nm. (C) The XRD spectrum of Galazyme. (D) The XPS spectrum of Galazyme. (E) The UV-vis spectra of TMB treated with Galazyme and H2O2 at different concentrations. (F) The ESR spectra of •OH trapped using DMPO.
3.2 In Vitro cytotoxicity of galazyme
The in vitro anti-proliferation effect was evaluated using various assays based on the impressive excellent catalytic activity of Galazyme and the galangin-induced chemotherapy toxicity. The cellular uptake of Galazyme and galangin were quantified by HPLC. Figure 2A demonstrated that Galazyme showed higher internalization efficiency compared to free galangin, ascribing to the passive uptake of nanoparticles by tumor cells. The cell counting kit-8 (CCK-8) assay was conducted to quantitatively measure the in vitro cellular cytotoxicity of Galazyme on HepG2 cells. As shown in Figure 2B and Supplementary Figure S3, free nanozyme or galangin partially inhibited tumor cell growth, whereas Galazyme markedly suppressed it, validating its remarkable catalytic activity and enhanced chemotherapy toxicity. In addition, the bright fields images of tumor cells were imaged to assess the cytotoxicity of Galazyme. As anticipated, Galazyme can effectively induce tumor cell death (Supplementary Figure S4). Furthermore, the live/dead assay was employed to evaluate the tumor cell-killing effect of Galazyme using the Annexin-V/propidium iodide (PI). Galazyme demonstrated remarkably brighter fluorescence (indicating apoptosis) than the Nanozyme or galangin alone group (Figure 2C; Supplementary Figure S5). The cytometry analysis further proved the tumor cell killing effect (Supplementary Figure S6). These finding confirmed that Galazyme inhibited tumor cell growth via synergetic catalytic therapy and chemotherapy toxicity.
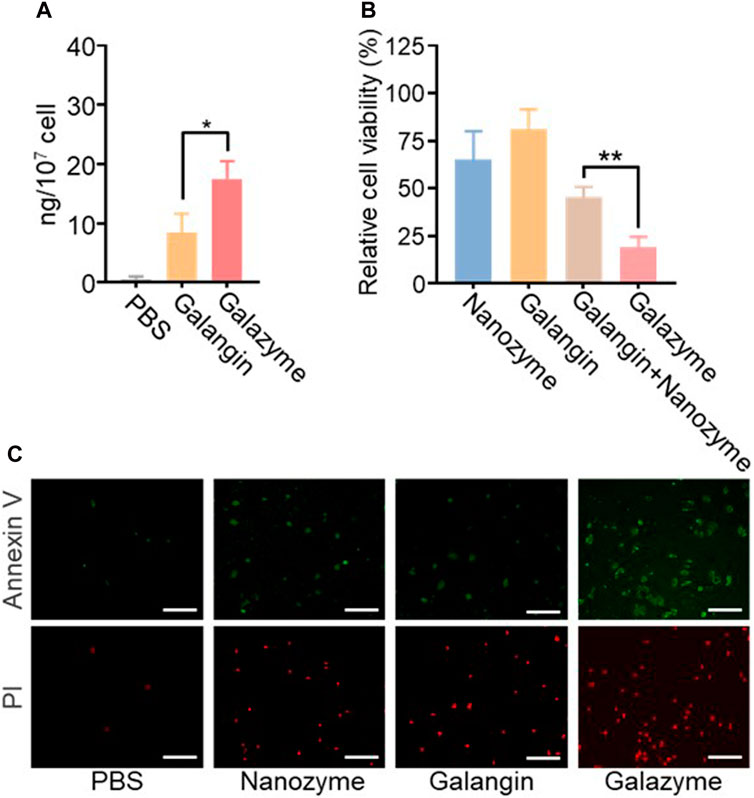
Figure 2. (A) Intracellular content analysis of the treatment of Galangin and Galazyme. (B) Cell viability analysis of cells after the indicated treatments. The drug concentration was based on 10 μM galangin. Data are expressed as mean ± SEM of three independent experiments. (C) Cell apoptosis analysis of cells after the treatment of PBS, Nanoenzyme, Galangin, and Galazyme. Scale bar: 100 μm. (*p < 0.05, **p < 0.01).
3.3 Therapeutic mechanisms of galazyme-induced cell death
The releated tumor cell death pathway was investigated to assess the potential mechanism of Galazyme-induced therapeutic efficacy. As previous reported, galangin can effective upregulate the MAPK expression to induce tumor cell apoptosis. The immunofluorescence staining was employed to measure the phosphorylated MAPK levels in tumor cells after treatment with different formulations. As shown in Figure 3A, both galangin and Galazyme effective elevated the expression in tumor cells. Then the excessive cellular ROS level was assessed using a fluorescence probe (2′,7′-dichlorofluorescin diacetate, DCFH-DA). It can be seen in Figure 3B that Galazyme displayed brighter red fluorescence intensity compared to free galangin, attributing to the excellent catalytic activity. Moreover, the qRCR was conducted to measure the Bax level and Caspase 3 level in tumor cells after treated with different formulations. As expected, Galazyme greatly elevated the expression of Bax and Caspase-3, ascribing the satisfactory •OH generation and MAPK activation (Figures 3C, D). These results indicated that Galazyme convert H2O2 into highly toxic •OH and activated MAPK pathway, inducing tumor cell apoptosis.
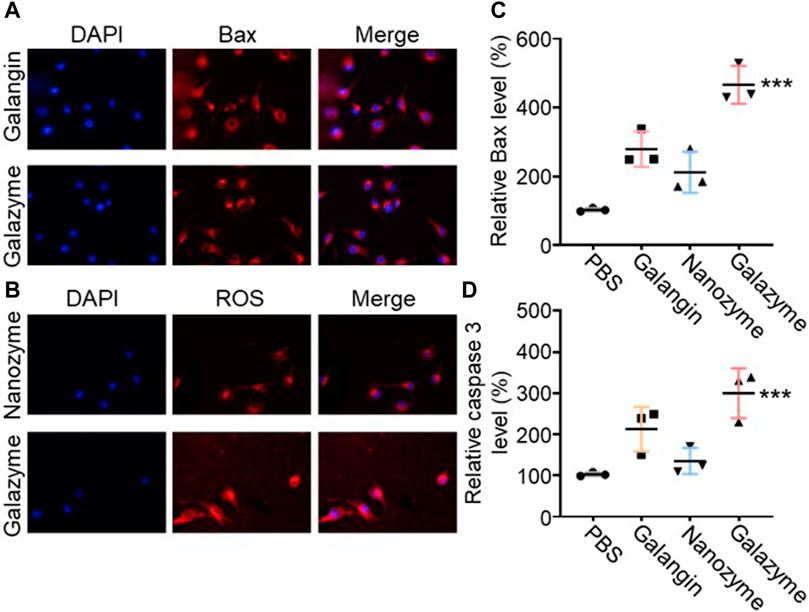
Figure 3. (A) The phosphorylated MAPK levels in tumor cells after treatment with different formulations Nucleus was stained with DAPI. (B) The ROS levels in tumor cells after treatment with different formulations. Nucleus was stained with DAPI. (C) Bax expression in tumor cells treated with different formulations. (D) Cleaved caspase three expression in tumor cells treated with different formulations. (***p < 0.001).
3.4 In vivo antitumor effect of galazyme
The Ethical Committee of Hainan Medical University approved the protocol for the animal experiments. The synergistic therapeutic efficacy triggered by Galazyme was studied in tumor-bearing nude mice post-intravenous injection. The tumor-bearing nude mice were randomly divided into four groups: PBS, Galangin, nanozyme, and Galazyme groups. As can be seen in Figure 4A, Nanozyme partially suppressed tumor growth, ascribed to the impressive Fenton activity. In comparison, Galazyme demonstrated a significant inhibition of tumor growth, attrubuted to the synergistic chemodynamic and apoptosis involving ROS generation (Supplementary Figure S7). Importantly, no significant variation in body weight was observed among the different groups, suggesting the favorable biosafety profile of Galazyme. Moreover, the antitumor efficacy of Galazyme was confirmed through immunochemical TUNEL and immunofluorescence staining of tumor sections. Figure 4B showed that Galazyme induced the highest level of cell death and activated MAPK expression compared to free galangin and Nanozyme. And Figure 4C revealed that Galazyme effectively induced the MAPK activation in tumor cells. Collectively, these results supported the enhanced antitumor efficacy of Galazyme via activating MAPK pathways and generating plentiful ROS.
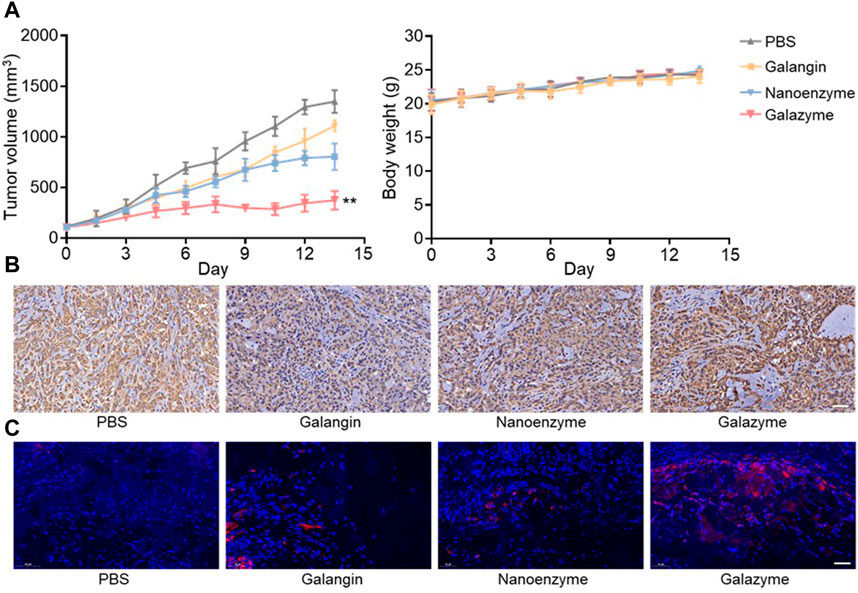
Figure 4. (A) Tumor volume and body weight change of each treatment group. (B) Images of immunochemical staining TUNEL assays of tumor tissues after different treatments. Scale bar: 50 μm. (C) Images of immunofluorescence staining of the MAPK. Nucleus was stained with DAPI. Scale bar: 50 μm. (**p < 0.01).
4 Conclusion
In conclusion, we developed an impressive nanozyme-Galazyme with MAPK activation and ROS production capabilities. Upon uptake of cancer cells, Galazyme not only catalytically converted overproduced H2O2 into highly active •OH but also activated MAPK pathway, causing the upregulation of Bax2 and Caspase 3, which in return promoted irreversible apoptosis. Both in vitro and in vivo results revealed that Galazyme suppressed tumor cell growth and triggered tumor apoptosis through initiating ROS and activating MAPK pathway. Our work highlights the potential of Galazyme as a promising apoptosis nanomedicine to deliver effective tumor therapy.
Data availability statement
The original contributions presented in the study are included in the article/Supplementary Material, further inquiries can be directed to the corresponding author.
Ethics statement
The animal study was approved by The Ethical Committee of Hainan Medical University. The study was conducted in accordance with the local legislation and institutional requirements.
Author contributions
EW: Conceptualization, Data curation, Formal Analysis, Methodology, Writing–original draft. YuW: Formal Analysis, Validation, Writing–review and editing. YaW: Formal Analysis, Software, Validation, Writing–review and editing. JL: Writing–review and editing. XuL: Writing–review and editing. ZW: Data curation, Methodology, Writing–review and editing. XaL: Formal Analysis, Writing–review and editing. FF: Writing–review and editing. JM: Formal Analysis, Writing–review and editing. YZ: Writing–review and editing. LL: Funding acquisition, Investigation, Supervision, Writing–review and editing.
Funding
The author(s) declare that financial support was received for the research, authorship, and/or publication of this article. Funding for the construction project of Hainan Clinical Medical Center (QWYH202175). Youth Fund Project of Natural Science Foundation of Hainan Province, China (320QN263); Research of Molecular Epidemiology and Development of Prevention&Control Technology for Tropical Diseases (2020-PT310-009).
Conflict of interest
The authors declare that the research was conducted in the absence of any commercial or financial relationships that could be construed as a potential conflict of interest.
Publisher’s note
All claims expressed in this article are solely those of the authors and do not necessarily represent those of their affiliated organizations, or those of the publisher, the editors and the reviewers. Any product that may be evaluated in this article, or claim that may be made by its manufacturer, is not guaranteed or endorsed by the publisher.
Supplementary material
The Supplementary Material for this article can be found online at: https://www.frontiersin.org/articles/10.3389/fchem.2024.1426634/full#supplementary-material
References
Chen, X., Zhao, M., Xie, Q., Zhou, S., Zhong, X., Zheng, J., et al. (2024). Click-hydrogel delivered aggregation-induced emissive nanovesicles for simultaneous remodeling and antibiosis of deep burn wounds. Aggregate 5, e406. doi:10.1002/agt2.406
Cheng, J., Zhu, Y., Xing, X., Xiao, J., Chen, H., Zhang, H., et al. (2021). Manganese-deposited iron oxide promotes tumor-responsive ferroptosis that synergizes the apoptosis of cisplatin. Theranostics 11, 5418–5429. doi:10.7150/thno.53346
Cushnie, T. P. T., and Lamb, A. J. (2005). Antimicrobial activity of flavonoids. Int. J. Antimicrob. Ag. 26, 343–356. doi:10.1016/j.ijantimicag.2005.09.002
Farhadi, F., Khameneh, B., Iranshahi, M., and Iranshahy, M. (2019). Antibacterial activity of flavonoids and their structure–activity relationship: an update review. Phytother. Res. 33, 13–40. doi:10.1002/ptr.6208
Huang, L., Chen, J., Gan, L., Wang, J., and Dong, S. (2019b). Single-atom nanozymes. Sci. Adv. 5, eaav5490. doi:10.1126/sciadv.aav5490
Huang, Y., Ren, J., and Qu, X. (2019a). Nanozymes: classification, catalytic mechanisms, activity regulation, and applications. Chem. Rev. 119, 4357–4412. doi:10.1021/acs.chemrev.8b00672
Jiang, D., Ni, D., Rosenkrans, Z. T., Huang, P., Yan, X., and Cai, W. (2019). Nanozyme: new horizons for responsive biomedical applications. Chem. Soc. Rev. 48, 3683–3704. doi:10.1039/c8cs00718g
Kandaswami, C., Lee, L. T., Lee, P. P., Hwang, J. J., Ke, F. C., Huang, Y. T., et al. (2005). The antitumor activities of flavonoids. Vivo 19, 895–909. Available at: https://iv.iiarjournals.org/content/19/5/895.short.
Kong, Y., Feng, Z., Chen, A., Qi, Q., Han, M., Wang, S., et al. (2019). The natural flavonoid galangin elicits apoptosis, pyroptosis, and autophagy in glioblastoma. Fron. Oncol. 9, 942. doi:10.3389/fonc.2019.00942
Li, Y., Tong, L., Zhang, J., Zhang, Y., and Zhang, F. (2018). Galangin alleviates liver ischemia-reperfusion injury in a rat model by mediating the PI3K/AKT pathway. Cell. Physiol. biochem. 51, 1354–1363. doi:10.1159/000495553
Liang, X., Wang, P., Yang, C., Huang, F., Wu, H., Shi, H., et al. (2021). Galangin inhibits gastric cancer growth through enhancing STAT3 mediated ROS production. Fron. Pharmacol. 12, 646628. doi:10.3389/fphar.2021.646628
Liu, Y.-N., Zha, W. J., Ma, Y., Chen, F. F., Zhu, W., Ge, A., et al. (2015). Galangin attenuates airway remodelling by inhibiting TGF-β1-mediated ROS generation and MAPK/Akt phosphorylation in asthma. Sci. Rep. 5, 11758. doi:10.1038/srep11758
Niu, X., Zhu, Y., Ding, C., Ma, J., Wei, P., Lin, Y., et al. (2023). Dual-active center AgFeCu nanocatalyst for tumor destruction via self-catalytically enhanced mild photothermal therapy. Adv. Funct. Mat. 33, 2306778. doi:10.1002/adfm.202306778
Ren, W., Qiao, Z., Wang, H., Zhu, L., and Zhang, L. (2003). Flavonoids: promising anticancer agents. Med. Res. Revi. 23, 519–534. doi:10.1002/med.10033
Tang, D., Kang, R., Berghe, T. V., Vandenabeele, P., and Kroemer, G. (2019). The molecular machinery of regulated cell death. Cell Res. 29, 347–364. doi:10.1038/s41422-019-0164-5
Tang, G., He, J., Liu, J., Yan, X., and Fan, K. (2021). Nanozyme for tumor therapy: surface modification matters. Exploration 1, 75–89. doi:10.1002/exp.20210005
Wang, J., Ding, H., Zhu, Y., Liu, Y., Yu, M., Cai, H., et al. (2023). Iron-siRNA nanohybrids for enhanced chemodynamic therapy via ferritin heavy chain downregulation. Angew. Chem. Int. Ed. 62, e202302255. doi:10.1002/ange.202302255
Wu, T., Cao, Y., Liu, Q., Wu, X., Shang, Y., Piao, J., et al. (2022). Genetically encoded double-stranded DNA-based nanostructure folded by a covalently bivalent CRISPR/dCas system. J. Am. Chem. Soc. 144, 6575–6582. doi:10.1021/jacs.2c01760
Wu, T., Fu, Y., Guo, S., Shi, Y., Zhang, Y., Fan, Z., et al. (2024). Self-assembly multifunctional DNA tetrahedron for efficient elimination of antibiotic-resistant bacteria. Aggregate 5, e402. doi:10.1002/agt2.402
Wu, T., Liu, J., Liu, M., Liu, S., Zhao, S., Tian, R., et al. (2019). A nanobody-conjugated DNA nanoplatform for targeted platinum-drug delivery. Angew. Chem. Int. Ed. 58, 14224–14228. doi:10.1002/anie.201909345
Xu, B., Li, S., Zheng, L., Liu, Y., Han, A., Zhang, J., et al. (2022). A bioinspired five-coordinated single-atom iron nanozyme for tumor catalytic therapy. Adv. Mat. 34, 2107088. doi:10.1002/adma.202107088
Zhu, D., Chen, H., Huang, C., Li, G., Wang, X., Jiang, W., et al. (2022a). H2O2 self-producing single-atom nanozyme hydrogels as light-controlled oxidative stress amplifier for enhanced synergistic therapy by transforming “cold” tumors. Adv. Funct. Mat. 32, 2110268. doi:10.1002/adfm.202110268
Zhu, D., Zhang, J., Luo, G., Duo, Y., and Tang, B. Z. (2021b). Bright bacterium for hypoxia-tolerant photodynamic therapy against orthotopic colon tumors by an interventional method. Adv. Sci. 8, 2004769. doi:10.1002/advs.202004769
Zhu, D., Zheng, Z., Luo, G., Suo, M., Li, X., Duo, Y., et al. (2021a). Single injection and multiple treatments: an injectable nanozyme hydrogel as AIEgen reservoir and release controller for efficient tumor therapy. Nano Today 37, 101091. doi:10.1016/j.nantod.2021.101091
Zhu, Y., Jin, D., Liu, M., Dai, Y., Li, L., Zheng, X., et al. (2022b). Oxygen self-supply engineering-ferritin for the relief of hypoxia in tumors and the enhancement of photodynamic therapy efficacy. Small 18, 2200116. doi:10.1002/smll.202200116
Zhu, Y., Liao, Y., Zou, J., Cheng, J., Pan, Y., Lin, L., et al. (2023). Engineering single-atom nanozymes for catalytic biomedical applications. Small 19, 2300750. doi:10.1002/smll.202300750
Zhu, Y., Niu, X., Ding, C., Lin, Y., Fang, W., Yan, L., et al. (2024a). Carrier-free self-assembly nano-sonosensitizers for sonodynamic-amplified cuproptosis-ferroptosis in glioblastoma therapy. Adv. Sci., e2402516. doi:10.1002/advs.202402516
Zhu, Y., Niu, X., Wu, T., Cheng, J., Zou, J., Pan, Y., et al. (2024b). Metal-phenolic nanocatalyst rewires metabolic vulnerability for catalytically amplified ferroptosis. Chem. Eng. J. 485, 150126. doi:10.1016/j.cej.2024.150126
Keywords: galangin, nanozyme, reactive oxygen species, apoptosis, fenton reaction
Citation: Wang E, Wu Y, Wang Y, Li J, Liang X, Wang Z, Liu X, Feng F, Mao J, Zhu Y and Li L (2024) Natural product nanozymes of herbal extract galangin in managing hepatocellular carcinoma. Front. Chem. 12:1426634. doi: 10.3389/fchem.2024.1426634
Received: 01 May 2024; Accepted: 24 May 2024;
Published: 10 June 2024.
Edited by:
Junjie Cheng, University of Science and Technology of China, ChinaReviewed by:
Yang Zhu, National University of Singapore, SingaporeDaoming Zhu, Southern Medical University, China
Copyright © 2024 Wang, Wu, Wang, Li, Liang, Wang, Liu, Feng, Mao, Zhu and Li. This is an open-access article distributed under the terms of the Creative Commons Attribution License (CC BY). The use, distribution or reproduction in other forums is permitted, provided the original author(s) and the copyright owner(s) are credited and that the original publication in this journal is cited, in accordance with accepted academic practice. No use, distribution or reproduction is permitted which does not comply with these terms.
*Correspondence: Le Li, bGVsaUBoYWlubWMuZWR1LmNu