- 1Department of Chemistry, G.B. Pant University of Agriculture and Technology, Pantnagar, India
- 2Regional Ayurveda Research Institute, Ministry of Ayush, Gwalior, India
- 3Department of Botany and Microbiology, College of Science, King Saud University Riyadh, Riyadh, Saudi Arabia
A new bidentate Schiff base ligand (C16H16Cl2N4), condensation product of ethylene diamine and 4-chloro N-phenyl formamide, and its metal complexes [M(C16H16Cl2N4)2(OAc)2] (where M = Mn(II) and Zn(II)) were synthesized and characterized using various analytical and spectral techniques, including high-resolution mass spectrometry (HRMS), elemental analysis, ultraviolet–visible (UV–vis), Fourier-transform infrared (FTIR) spectroscopy, AAS, molar conductance, 1H NMR, and powder XRD. All the compounds were non-electrolytes and nanocrystalline. The synthesized compounds were assessed for antioxidant potential by DPPH radical scavenging and FRAP assay, with BHT serving as the positive control. Inhibitory concentration at 50% inhibition (IC50) values were calculated and used for comparative analysis. Furthermore, the prepared compounds were screened for antibacterial activity against two Gram-negative bacteria (Staphylococcus aureus and Bacillus subtilis) and two Gram-positive bacteria (Escherichia coli and Salmonella typhi) using disk-diffusion methods, with amikacin employed as the standard reference. The comparison of inhibition zones revealed that the complexes showed better antibacterial activity than the ligand. To gain insights into the molecular interactions underlying the antibacterial activity, the ligand and complexes were analyzed for their binding affinity with S. aureus tyrosyl–tRNA synthetase (PDB ID: 1JIL) and S. typhi cell membrane protein OmpF complex (PDB ID: 4KR4). These analyses revealed robust interactions, validating the observed antibacterial effects against the tested bacterial strains.
1 Introduction
Schiff bases, also referred to as azomethines (>C=N-) or imines, are compounds synthesized through the reaction of primary amines with ketones or aldehydes under specific conditions (Kanwal et al., 2022). These compounds are extensively studied because of their sigma donor tendency toward metal and π acceptor properties in imine nitrogen atoms. This distinctive property renders it a valuable donating ligand in coordination chemistry (Bruns et al., 2010; Abd El-Lateef et al., 2018). Schiff base ligands containing nitrogen, along with other donor atoms such as oxygen and sulfur in their molecular structures, serve as chelating agents and easily form complexes with various metal ions (Abd El Wahed et al., 2004; Mishra et al., 2005; El-Sherif and Eldebss, 2011). In recent years, these complexes gained significant attention due to their diverse use in biology (Ghanghas et al., 2021), as models for metal-containing sites in metalloproteins (Ueno et al., 2004; Shahraki, 2022), catalysts for some organic reactions (Juyal et al., 2023a), and complexing ability toward some toxic metals (Vaghasiya et al., 2004) encompassing antibacterial (Kargar et al., 2021; Muthukumar et al., 2022), antifungal (Joshi et al., 2020; Shiryaev et al., 2021; Borrego-Muñoz et al., 2022), anticancer (Tadele and Tsega, 2019; Aslan et al., 2020; Alorini et al., 2022), antioxidant (Buldurun et al., 2019; Bingöl and Turan, 2020; Yadav et al., 2021), anti-inflammatory (Devi et al., 2019; Krishna et al., 2023), and antiviral activities (Abd El-Hamid et al., 2023; Bhandarkar et al., 2023).
The formylation-driven amination process, typically achieved by combining amines with formic acids, results in the formation of formamide. These compounds hold significant importance in both organic and industrial chemistry (Liang et al., 2023). N-Alkylformamides, polar solvents, like diethylformamide and dimethylformamide, are extensively utilized in both chemical laboratories and industrial settings for the synthesis of films, artificial fibers, and leather products (Amato et al., 2001). Formamides exhibit dual reactivity as both electrophilic and nucleophilic agents (Muzart, 2009), serving as a versatile source of key intermediates that mediate various reactions. Their structural flexibility allows engagement in diverse reactions functioning as building blocks for diverse units like -CHO, -O, -CONMe2, -NMe2, - CO, and -Me (Ding and Jiao, 2012). Formamides serve as intermediates in the synthesis of pharmaceutically active molecules including fluoroquinolones like 1,2-dihydroquinolines (Kobayashi et al., 1995), norfloxacin, and ciprofloxacin (Jackson and Meth-Cohn, 1995) and nitrogen-bridged heterocycles such as oxazolidinones (Lohray et al., 1999) and benzimidazole (Mohanty et al., 2018). However, metal complexes of formamide-based moieties are not widely studied, so there is untapped potential for discovery and advancement in the understanding of metal complexes with formamide-based moieties.
The overproduction of reactive oxygen species, like OH. and superoxide anions, is recognized for inducing oxidative harm to DNA, lipids, and proteins. This contributes significantly to cancer, aging, inflammation, cardiovascular diseases, and neurodegenerative disorders (Beyazit et al., 2020). To counteract these harmful effects, antioxidants are crucial. Various studies have indicated the potential of Schiff base metal complexes as efficient scavengers of these free radicals (Sharma et al., 2015; Mumtaz et al., 2020). Furthermore, pathogenic microorganisms pose a substantial threat through life-threatening bacterial infections (Xu et al., 2020). Although antibiotics and antibacterial agents have been the traditional means to combat harmful bacterial growth, their prolonged use has raised concerns about bacterial resistance (Kaya et al., 2021). Consequently, there is an ongoing demand for novel antibacterial agents. Antioxidants can modulate the redox balance within microbial cells by scavenging free radicals and maintaining cellular redox homeostasis. Disruption of the redox balance can interfere with essential metabolic processes in microbes, leading to growth inhibition or cell death (Zandi and Schnug, 2022). In the given context, metal complexes of Schiff bases have emerged as promising candidates (Chen et al., 2017; El-Gammal et al., 2021).
Molecular docking techniques are instrumental in drug design and mechanistic studies, enabling the precise positioning of molecules within the binding sites of target macromolecules without forming chemical bonds (Juyal et al., 2023b). The process of docking utilizes computer simulations to predict how small compounds or macromolecules will interact with receptors at a molecular level, utilizing specialized docking software such as DOCK (Ismael et al., 2018) and AutoDock (Cheng et al., 2009; Junaid et al., 2018). These techniques offer accurate and efficient predictions of favorable interactions between proteins and ligands. These programs function as powerful computational filters, streamlining the search for potential bioactive compounds before experimental screening, thus reducing both costs and labor. Additionally, they contribute significantly to the understanding of molecular mechanisms post-experimental screening (Pagadala et al., 2017).
The present study aims to create potent antioxidant and antibacterial compounds with metal complexes of formamide-based Schiff base moieties due to their unique structural framework. The inclusion of the imine group enhances biological activity through hydrogen bonding, thus motivating the synthesis of the targeted molecules. The ligand precursor formamide was synthesized through chloroanilines and formic acid, utilizing NH2OH.HCl as the catalyst. The ligand was then synthesized by a reaction of the ligand precursor with ethylene diamine.
Manganese (Mn) and zinc (Zn) are essential trace elements crucial for numerous biological functions. Mn supports the activities of enzymes like arginase and manganese superoxide dismutase, aiding the urea cycle and oxidative stress protection (Bowman et al., 2011). Zn is integral to over 300 enzymes, including DNA polymerase, and is vital for DNA replication and repair, protein integrity, and immune signaling (Chasapis et al., 2020). Their unique properties of Mn(II) and Zn(II) make them indispensable in cellular roles and drives the synthesis of complexes. The incorporation of Mn(II) and Zn(II) into the ligand was achieved by chelation of the synthesized ligand to different metal salts at a 2:1 M ratio. The synthesized compounds were subjected to biological assessments, including assessment of antioxidant and antibacterial activities, to evaluate the responsiveness of compounds to various microorganisms. Additionally, a molecular docking study was conducted to gain insights into the bioactive mechanisms of these compounds.
2 Materials and methods
2.1 Reagents
The reagents Mn(OAc)2.4H2O (Sigma-Aldrich), Zn(OAc)2.2H2O (Sigma-Aldrich), ethylene diamine (Sigma-Aldrich), formic acid (HiMedia), methanol (Molychem), 4-chloroaniline (Molychem), ethanol (Molychem), and hydroxylamine hydrochloride (Sigma-Aldrich) were utilized in their highest degree of purity (AR grade). The bacterial culture was collected from the Department of Microbiology, CVAS, GB Pant University of Agriculture and Technology, Pantnagar.
2.2 Instruments
Molar conductivity of all compounds was determined using the Systronics conductivity TDS meter 308, while the melting point was determined using the Decibel DB-3135H MP apparatus. Elemental analysis was carried out using the vario MICRO cube (Elementar Analysensysteme, Germany). Moreover, the metal analysis was performed using the AAS instrument Element AS AAS 4141, and 1H NMR was carried out using the JEOL JNM ECS400 (400 MHz, dimethyl sulfoxide (DMSO)-d6). The magnetic molar susceptibilities (χM) were gauged using Quincke’s tube in conjunction with the Digital Gauss Meter, DGM-102, and µeff was then calculated using the expression µeff = 2.828 (χM.T)1/2 B.M. (Abou-Hussein and Linert, 2015). Powder XRD (PXRD) was conducted using the Bruker D8 ADVANCE diffractometer, while the Fourier-transform infrared (FTIR) spectroscopy data were collected by using the PerkinElmer FTIR spectrophotometer (350–4,000 cm−1). The ultraviolet–visible (UV–vis) spectra were measured (0.05 g/L) using the US GENESYS 10S spectrophotometer (Thermo Fisher Scientific).
2.3 Synthesis
2.3.1 Preparation of the ligand
The preparation of the ligand involves two steps. First, the precursor formamide was synthesized and then ethylene diamine was attached to the formamide structure to attain the Schiff base ligand moiety.
2.3.1.1 Synthesis of ligand precursors 4-chloro-(N-phenyl)formamide
Formic acid (2 mmol) and 4-chloroaniline (1 mmol) were refluxed together at 80°C with the catalyst NH2OH.HCl (Scheme 1). The reaction progress was monitored through thin-layer chromatography (TLC.), and once the reaction was concluded, EtOAc was poured into the mixture. Subsequently, the reaction mixture was washed with H2O and 5% HCl solution (three times). The mixture was then dried with anhydrous Na2SO4, and the solvent was removed by vacuum distillation. A white powder product was obtained with good yield.
Ligand precursor [C7H6ClNO]: White; yield: 84%; MP 53°C; 1H NMR (400 MHz, DMSO-d6, TMS) δ (ppm): 3.8 (s, 1H, NH), 7.1–7.6 (m, 4H, C6H6), and 7.8 (s, 1H, CHO); and FTIR (KBr, cm−1): 3,515 (N-H), 3,052 (-CH), and 1,715 (C=O).
2.3.1.2 Synthesis of the Schiff base ligand
A methanolic solution of ethylene diamine (1 mmol) was added dropwise to a methanolic solution of 4-chloro-(N-phenyl)formamide (2 mmol) in the presence of NH2OH.HCl at 72°C (Scheme 2). Once the reaction was concluded, EtOAc was introduced to the reaction mixture. Subsequently, the reaction mixture underwent successive washes with H2O and a 5% HCl solution (three times). Subsequently, the mixture was dried using anhydrous Na2SO4, and the solvent was removed through vacuum distillation. A light-yellow crude product was obtained, which was dried, washed, and recrystallized with C2H5OH.
Schiff base ligand [C16H16Cl2N4]: Light yellow; yield: 84%; MP 94°C; 1H NMR (400 MHz, DMSO-d6, TMS) δ (ppm): 1.5 (t, 2H, CH2), 4.2 (s, 1H, NH), 6.6–7.5 (m, 4H, C6H6), and 7.8 (s, 1H, CH); FTIR (KBr, cm−1): 3,536 (N-H), 2,972 (C-H), and 1,621 (C=N).
2.3.2 General methods for the preparation of metal complexes
The Schiff base ligand (2 mmol methanolic solution) was added dropwise to the 1 mmol methanolic solution of metal salts (Mn(OAc)2.4H2O and Zn(OAc)2.2H2O). The resulting mixture was stirred for 8 h at a temperature range of 70°C–80°C (Scheme 3). Following this, the mixture was allowed to stand overnight, resulting in the precipitation of colored crystals with a yield of 72%–78%.
Mn(II) complex [(C16H16Cl2N4)2Mn(OAc)2]: Light pink; yield: 78%; decomposition temperature: 281°C; FTIR (KBr, cm−1): 3,547 (N-H), 2,971 (=CH), 1,713 (C=O), 1,675 (C=N), 1,521 (COO)asymm., 1,325 (COO)symm., 468 (Mn-N), and 378 (Mn-O).
Zn(II) complex [(C16H16Cl2N4)2Zn(OAc)2]: Yellow; yield: 75%; decomposition temperature: 277°C; 1H NMR (400 MHz, DMSO-d6, TMS) δ (ppm): 1.2 (t, 2H, CH2), 2.6 (s, 3H, COCH3), 3.4 (s, 2H, NH), 6.6–7.6 (m, 4H, C6H6), and 7.8 (s, 2H, CH); FTIR (KBr, cm−1): 3,568 (N-H), 2,972 (=C-H), 1,711 (C=O), 1,625 (C=N), 1,508 (COO)asymm., 1,334 (COO)symm., 456 (Zn-O), and 396 (Zn-N).
2.4 Biological activities
2.4.1 Antioxidant activity
To assess the antioxidant potential of the compounds, ferric reducing antioxidant power (FRAP) methods and 1,1-diphenylpicrylhydrazyl (DPPH) radical scavenging methods were utilized. The samples were tested at varying concentrations (250–1,000 μg/mL).
2.4.1.1 FRAP assay
The FRAP method (Benzie and Strain, 1996) was employed to examine the reduction capacity of the compounds. To prepare the FRAP assay, a 10:1:1 solution of phosphate buffer (pH, 7.6), TPTZ, and FeCl3 was heated at 70°C for 10 min and then added to the compounds. The reduction potential was determined by measuring the conversion of Fe(III) to Fe(II). The amount of Fe(II) complex in the solution was quantified by the intensity of blue color at 593 nm. A higher increase in absorbance was related to the greater reduction potential of the compounds. The FRAP was determined utilizing the standard curve of ferrous sulfate with butylated hydroxytoluene (BHT) used as a reference standard.
2.4.1.2 DPPH radical scavenging assay
To evaluate the antioxidant affinity, the DPPH radical scavenging procedure was conducted as detailed by Brand-Williams et al. (1995) and Aljohani et al. (2023). Here, 1 mL of the test solution was added to 0.004% methanolic solution of DPPH (4 mL) at varying concentrations (250–1,000 μg/mL). The solution was incubated in the dark for 30 min, and absorbance at 517 nm was recorded using a UV–vis spectrophotometer. BHT served as the standard here. Higher reductions in absorbance are associated with higher antioxidant activity. The following expression was used to calculate DPPH scavenging affinity %:
where AC and AS denote the absorbance of the control and sample, respectively. A comparative analysis was conducted by determining the inhibitory concentration at 50% inhibition (IC50) values using the graph between the concentration and DPPH scavenging affinity %.
2.4.2 Antibacterial activity
The minimum inhibitory concentration (MIC), quantified as the minimal concentration that inhibits bacterial growth post-overnight incubation, was ascertained utilizing the protocol followed by Devi and Batra (2015). Evaluations were executed on a pair of Gram-positive microorganisms, Bacillus subtilis and Staphylococcus aureus, alongside Gram-negative species, Salmonella typhi and Escherichia coli. Initially, a stock solution of the synthesized compounds was prepared in DMSO at a concentration of 100 μg/mL. This stock solution was then serially diluted to achieve concentrations of 50, 25, 12.5, 6.25, and 3.125 μg/mL. Hinton–Muller agar was prepared and uniformly spread across sterilized Petri dishes, which were subsequently incubated to achieve solidification. Thereafter, bacterial strains were inoculated on these plates, and aliquots spanning concentrations of 3.125–100 μg/mL were administered via 5-mm paper disks. The MIC values were recorded after an overnight incubation period.
2.5 Molecular docking study
A molecular docking study was performed to observe the molecular interactions between the prepared compounds and the protein receptors of S. aureus and S. typhi. The cell membrane protein OmpF complex of S. typhi (PDB ID: 4KR4) and S. aureus tyrosyl–tRNA synthetase (PDB ID: 1JIL) was used as the protein for the study (Sanner, 1999; De et al., 2020). The 3D structure of the protein in PDB file format was obtained from the PDB database (https://www.rcsb.org), and the ligand molecule in PDB file format was obtained from PubChem (https://pubchem.ncbi.nlm.nih.gov/). The grid and docking parameter files (GPFs and DPFs, respectively) were prepared by using AutoDock tools. The GPF defines the size and location of the grid that will be used to search for potential binding sites on the protein, while the DPF specifies the parameters for the genetic algorithm that will be used to dock the ligand to the protein. AutoGrid is used to generate a grid map of the protein based on the GPF. Blind docking was conducted to study the protein–ligand interactions. For each docking simulation, a grid box of dimensions 126 × 126 × 126 Å was used to encompass the entire protein structure, with the center coordinates set differently for each molecule and given in Table 3. The DPF was generated using the Lamarckian genetic algorithm (LGA), with a maximum of 2,500,000 energy evaluations. All other values were used as default. This will help identify the most favorable binding sites for the ligand. The docking simulation was performed using the AutoDock genetic algorithm. The docking results were analyzed using AutoDock 4.2 and BIOVIA Discovery Studio.
3 Results and discussion
Various methods such as magnetic, analytical, and spectral methods were used to characterize the synthesized compounds. Table 1 summarizes the yields, colors, magnetic moments, melting points, molar conductivity, and elemental compositions of the ligand and complexes. The molar conductivity of all compounds was low, indicating that they were non-electrolytic (Geary, 1971). The ligand precursor and ligand were soluble in CH3OH and DMSO, while the metal complexes were soluble in DMSO.
3.1 Characterization
3.1.1 Ultraviolet–visible spectroscopy and magnetic properties
The ligand precursor exhibited a λmax at 238 nm, likely associated with a π–π* transition. Additionally, a band at 484 nm can be ascribed to an n–π* transition, indicative of bonding with a system possessing a lone pair of electrons. In the case of the ligand, λmax appears at 288 nm (π–π* transition), and the other band appears at 356 nm (n–π* transition). The emergence of a distinct shoulder band in all complexes signifies the occurrence of charge transfer bands, providing conclusive evidence for the formation of a complex between the ligand and metal ions (Damiche and Chafaa, 2017). The Mn(II) complex was found to be paramagnetic, having a magnetic moment of 5.83 BM, suggesting the octahedral nature of the complex. The Mn(II) complex exhibited λmax at 372 nm and an LMCT band at 413 nm. Owing to the d-d transition bands being spin-forbidden and Laporte-forbidden, they are either absent or possess such minimal intensity that they are not observed. The same types of results were also reported by Devi et al. (2012) and Keypour et al. (2013). The Zn(II) complex was diamagnetic, and no d-d transition was observed for the complex. λmax and a charge transfer band were observed at 283 and 387 nm, respectively (Figure 1). The maximum molar absorption coefficients of the ligand precursor, Schiff base ligand, Mn(II) complex, and Zn(II) complex were 84,267, 75,976, 83,560, and 75,977 Lmol−1cm−1, respectively.
3.1.2 Infrared spectroscopy
FTIR spectroscopy serves as a pivotal tool for elucidating the structural intricacies of Schiff base ligands and their complexes. The infrared (IR) spectral analysis of the ligand precursor unveiled characteristic absorptions, notably the C=N stretching vibration at 1,621 cm−1, indicative of imine formation, and the N-H stretching at 3,515 cm−1, associated with amine functionalities. Notably, the C=O stretch, initially present at 1,715 cm−1, vanishes upon Schiff base formation, affirming the condensation of the carbonyl group with a primary amine to yield the imine linkage. Upon metal coordination, these bands undergo a discernible shift to lower wavenumbers, a phenomenon attributed to bond length augmentation. Concurrently, a new band emerges in the vicinity of 1,711–1,735 cm−1, ascribed to the carbonyl stretch within the acetate moiety, a common feature observed in metal–acetate complexes (Supplementary Figure S1).
Further scrutiny of the metal complexes through FT-IR spectroscopy reveals the presence of metal–ligand vibrational modes in the far-infrared region. For the Mn(II) complex, the emergence of bands at 468 cm−1 and 378 cm−1 corresponds to the Mn-N and Mn-O stretches, respectively. Similarly, the Zn(II) complex exhibits bands at 396 cm−1 for Zn-O and 456 cm−1 for Zn-N vibrations (Table 2). These bands are quintessential indicators of metal–nitrogen and metal–oxygen coordination, providing empirical evidence for the successful synthesis of the respective metal complexes.
The carboxylate group (from acetate) in the complexes shows two stretching bands: asymmetrical and symmetrical. The asymmetric stretching band typically appears at a higher wavenumber than the symmetric stretching band due to differences in bond strengths and the molecular vibrations associated with these modes. For the Mn(II) complex, the asymmetric stretching band is observed at 1,521 cm−1, while the symmetric stretching band is observed at 1,325 cm−1 (Abdallah et al., 2010). In contrast, for the Zn(II) complex, the asymmetric stretching band appears at 1,508 cm−1, and the symmetric stretching band appears at 1,334 cm−1 (Soliman and Mohamed, 2004); the M-O band is present in both complexes, suggesting the acetate coordination mode. The difference between the asymmetric and symmetric frequency Λ[ʋasymm (COO)- ʋsymm (COO)] is large (175–200 cm−1), which suggests monodentate legation (Belal et al., 2015).
3.1.3 1H NMR spectroscopy
1H NMR spectra of the prepared compounds confirm the proposed structure (Scheme 3). For the ligand precursor, the -NH bond appears at 3.8 ppm, the aldehydic proton appears at 7.8 ppm, and signals from 7.1 ppm to 7.4 ppm represent the aromatic region (Figure 2). In the case of the Schiff base ligand, the existing band of -NH shifted toward lower chemical shift, and a new band of -CH2 (ethylene diamine) appeared at 1.5 ppm (Supplementary Figure S2). The Mn(II) complex did not show the NMR signals, which may be attributed to the paramagnetism of the Mn(II) complex. For the Zn(II) complex, the band due to -NH shifted toward lower chemical shifts due to chelation of the ligand to the metal, and a new band appeared at approximately 2.4 ppm due to the -OCOCH3 group. NMR of the ligand precursor and Zn(II) complex is given in Supplementary Material (Supplementary Figure S3).
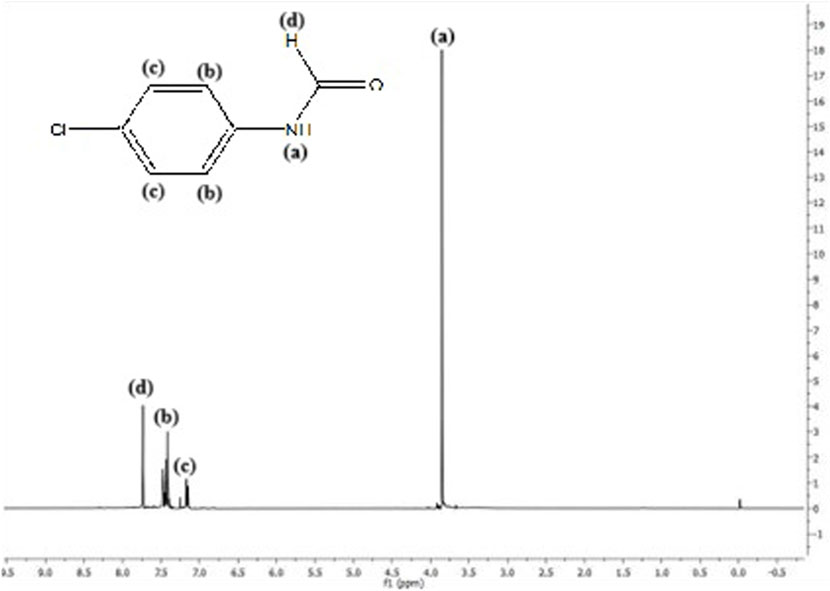
Figure 2. 1H NMR spectra of the Schiff base ligand precursor where (a), (b), (c) and (d) represent -NH, -CH, -CH and aldehydic H respectively.
3.1.4 Powder XRD study
Powder X-ray diffraction was performed in the 10 < 2θ < 80 range to study their lattice dynamics (Figure 3). The particle size (D) was obtained using the Debye–Scherrer expression:
where K is a constant of the Cu grid (0.94), λ denotes the wavelength of the X-ray, θ denotes the Bragg diffraction angle, and β is the integral peak width (Sundararajan et al., 2014).
The sizes of the ligand precursor, ligand, and Mn(II) and Zn(II) metal complexes were identified as nanocrystalline with 41.07, 96.6, 40.21, and 122.20 nm, respectively. The degree of crystallinity for the ligand precursor, Schiff base ligand, and Mn(II) and Zn(II) metal complexes was found to be 46.07, 30.09, 35.10, and 21.07%, respectively.
The XRD pattern reveals distinct crystalline patterns for both the ligand and its complexes, exhibiting different degrees of crystallinity. The complexes exhibit extra peaks compared to the ligand, confirming metal ion chelation and signaling complex formation (El-Megharbel and Hamza, 2022).
3.2 Biological activities
3.2.1 Antioxidant activities
3.2.1.1 FRAP assay
The ability to reduce ferric ions was evaluated through the FRAP assay. The standard curve of FeSO4.7H2O was utilized for obtaining FRAP values (Supplementary Figure S4). The findings indicated that all tested compounds possessed ferric-reducing antioxidant potential, with the ligand showing the highest reducing potential among all the compounds. The Zn(II) complex showed the highest FRAP among the metal complexes, and the activity follows the order BHT > Schiff base ligand > Zn(II) complex > Mn(II) complex > ligand precursor (Figure 4).
3.2.1.2 DPPH radical scavenging activity
Figure 5 demonstrates the DPPH radical scavenging potential of the examined compounds. Antioxidant activity was determined by evaluating the hydrogen-donating capacity or radical scavenging potential against the stable radical DPPH. Upon hydrogen radical abstraction, a color transition from dark purple to pale yellow was noted in the DPPH solution, and this change was quantified by assessing the reduction in the absorbance at 517 nm. The results indicated that the Zn(II) complex exhibited the highest scavenging potential among the examined compounds. The general order of DPPH radical scavenging potential was as follows: BHT > Zn(II) complex > ligand > Mn(II) complex > ligand precursor.
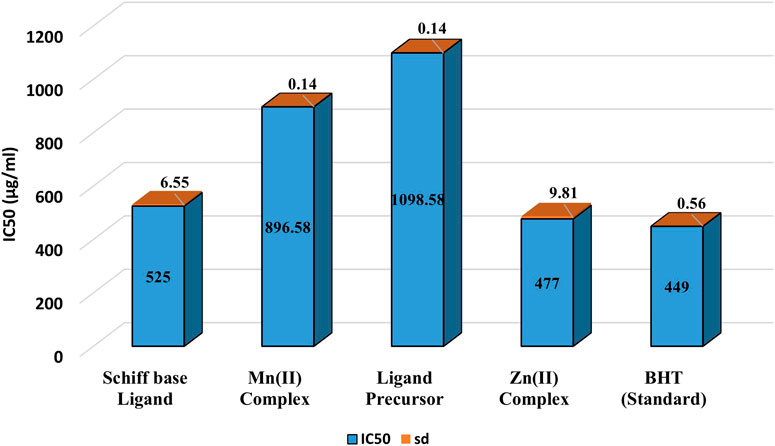
Figure 5. IC50 values of DPPH radical scavenging activity of Ligand precursor, Schiff base Ligand, Mn(II) Complex, Zn(II) Complex and Standard (BHT).
3.2.2 Antibacterial activity
Figure 6 illustrates the antibacterial screening results of all the tested compounds. Good antibacterial activity was observed for all the tested compounds. The metal complexes showed higher antibacterial activity than the ligand precursor and ligand. This could be attributed to Tweedy’s chelation theory, which proposes that chelation increases the bactericidal power of metal complexes as the metals share their positive charge with ligands, leading to p-electron delocalization throughout the compound. Thus, the complexes acquired a lipophilic nature, making it easier for them to permeate through membranes (Tweedy, 1964; Abu-Dief et al., 2019). Both Gram-positive and Gram-negative bacteria were tested, and the results showed that the tested compounds were more active for Gram-positive bacteria. Gram-positive bacteria are often considered to have a somewhat more permeable cell membrane because of the absence of an outer membrane, which simplifies their structure, allowing for potentially easier diffusion of molecules (Malanovic and Lohner, 2016). The highest activity was observed for S. typhi, although the activity of the examined compounds was lower than that of amikacin (standard). Among the complexes, the Mn(II) complex showed higher activities than the Zn(II) complex.
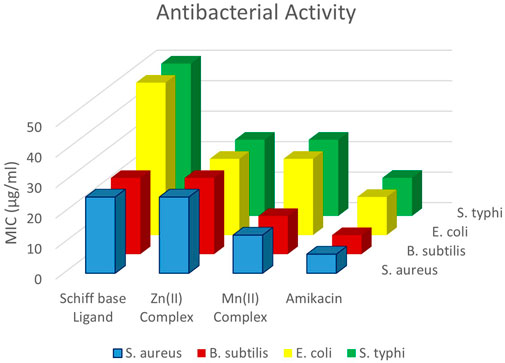
Figure 6. Antibacterial activity against Gram-negative bacteria (S. aureus and B. subtilis) and Gram-positive bacteria (E. coli and S. typhi).
3.3 Molecular docking study
The best 3D binding interaction of test compounds with the S. aureus tyrosyl–tRNA synthetase (Figure 7) and S. typhi cell membrane protein OmpF complex (Figure 8) is demonstrated. The binding energy represents the thermodynamic stability of the ligand–receptor complex. In molecular docking studies, a lower (more negative) binding energy is generally considered indicative of a more favorable and stronger interaction between a ligand (docked compound) and its target receptor (Wolohan and Reichert, 2004). These observations indicate substantial binding between the ligand and its complexes with the protein receptor, resulting in a favorable free binding energy.
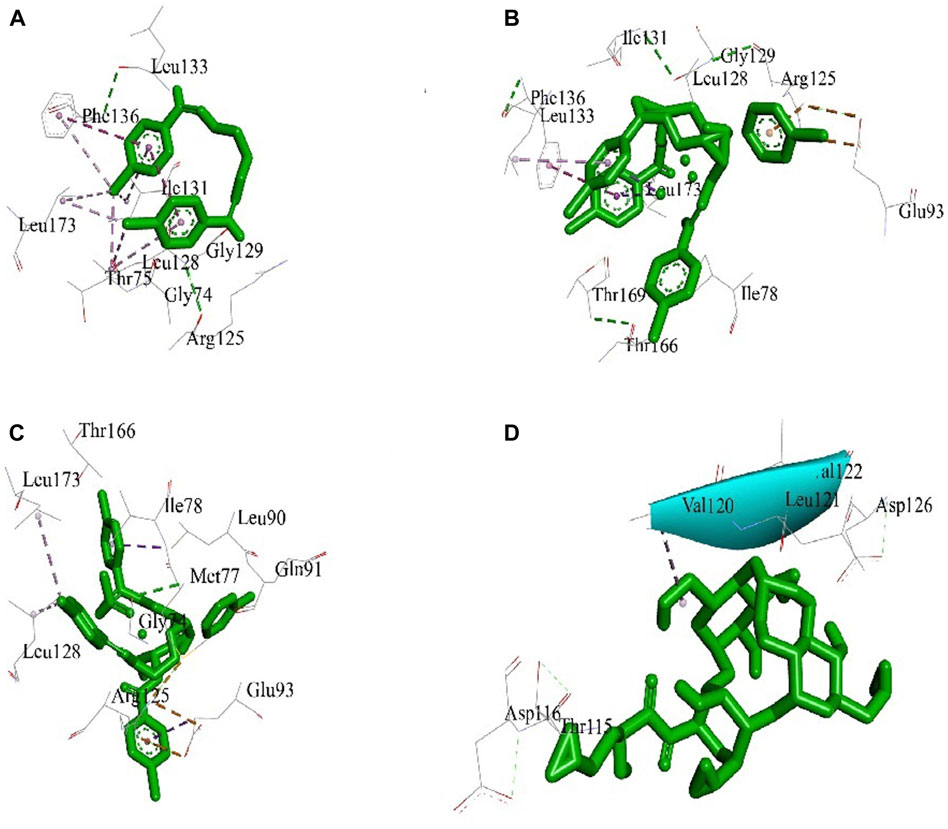
Figure 7. Docking interaction diagrams of the (A) Schiff base ligand, (B) manganese(II) complex, (C) zinc(II) complex, and (D) amikacin against S. aureus tyrosyl–tRNA synthetase.
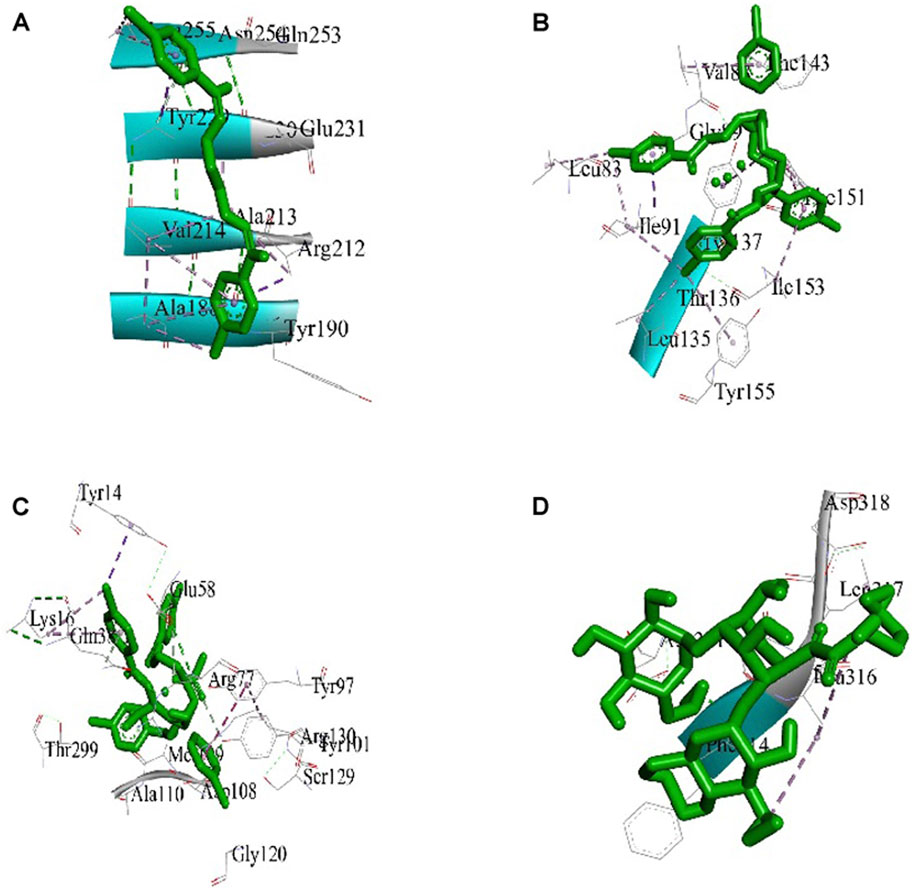
Figure 8. Docking interaction diagrams of the (A) Schiff base ligand, (B) manganese(II) complex, (C) zinc(II) complex, and (D) amikacin against the S. typhi protein OmpF complex.
In the case of the S. typhi protein OmpF complex, the best activity was shown by the Mn(II) complex (binding energy, −7.34 kcal/mol), followed by the Zn(II) complex (binding energy, −4.97 kcal/mol) and the ligand (binding energy, −4.47 kcal/mol). However, in the case of S. aureus tyrosyl–tRNA synthetase, the Mn (II)complex (binding energy, −4.43 kcal/mol) showed the highest activity, followed by the ligand (binding energy, −3.68 kcal/mol) and Zn(II) complex (binding energy, −3.28 kcal/mol) (Table 3).
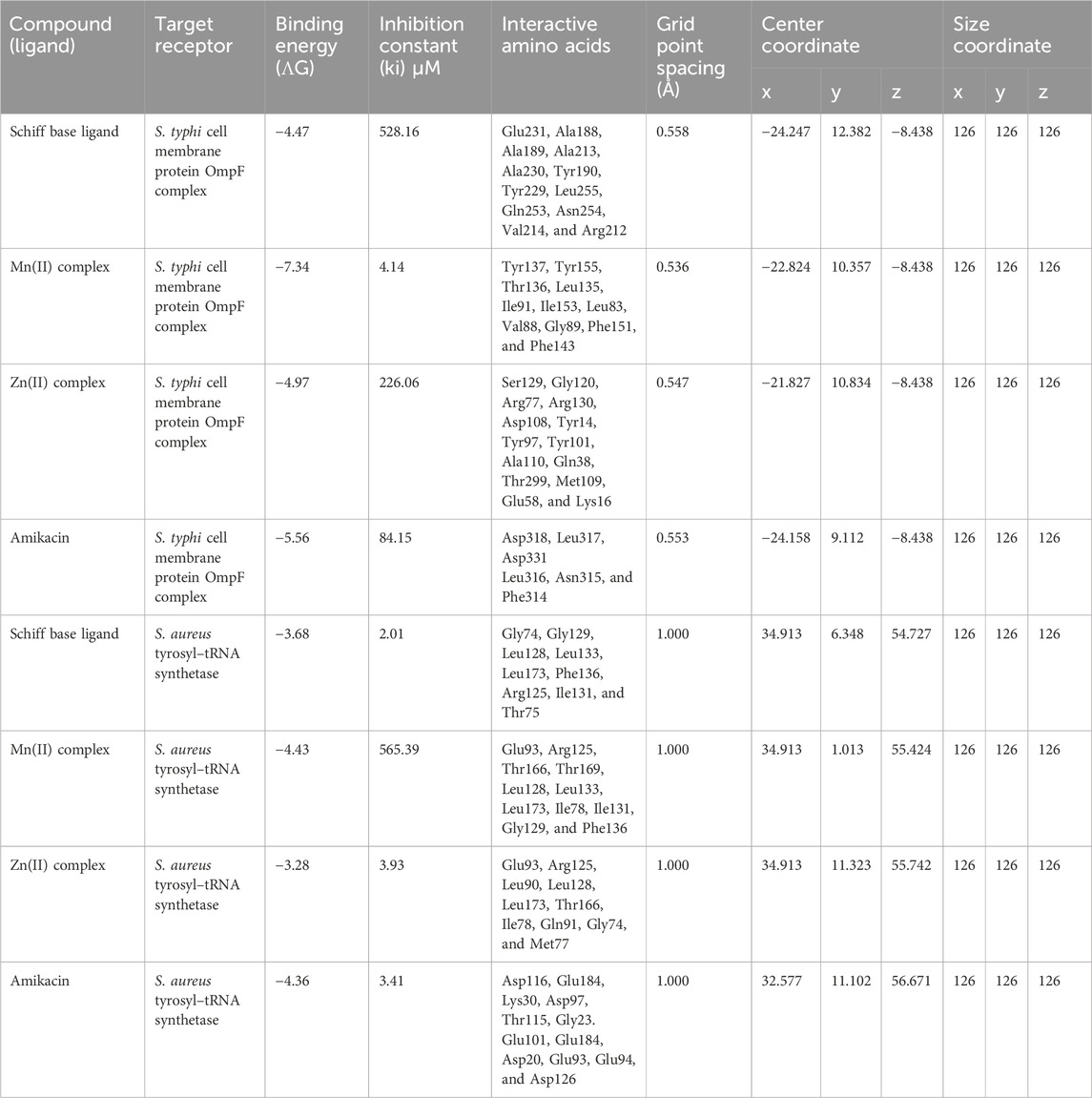
Table 3. Molecular docking results of docked compounds against the S. typhi cell membrane protein OmpF complex and S. aureus tyrosyl–tRNA synthetase.
4 Conclusion
In summary, Mn(II) and Zn(II) complexes of the formamide Schiff base ligand, derived from ethylene diamine and 4-chloro (N-phenyl)formamide, were synthesized and characterized by various analytical, spectral, and magnetic methods. The antioxidant potential of the prepared compounds was evaluated through DPPH radical scavenging activity assay and the FRAP method, and the following general trend was found: BHT > ligand ∼ Zn(II) complex > Mn(II) complex > ligand precursor. Afterward, all the synthesized compounds underwent assessment for their antibacterial efficacy against two Gram-negative bacteria (S. aureus and B. subtilis) and two Gram-positive bacteria (E. coli and S. typhi). The Mn(II) complex exhibited better bactericidal capacity than other compounds, which was validated by molecular docking interaction study. In studies involving the S. typhi cell membrane protein OmpF complex and S. aureus tyrosyl–tRNA synthetase, the Mn(II) complex demonstrated the highest binding affinity, followed by the ligand and Zn(II) complex.
Data availability statement
The original contributions presented in the study are included in the article/Supplementary Material; further inquiries can be directed to the corresponding authors.
Author contributions
VJ: conceptualization, data curation, formal analysis, investigation, methodology, writing–original draft, and writing–review and editing. ST: software and writing–review and editing. MP: data curation and writing–review and editing. Rashmi: data curation, visualization, and writing–review and editing. OP: methodology, visualization, and writing–review and editing. KP: funding acquisition, investigation, supervision, validation, and writing–review and editing. NB: funding acquisition, supervision, validation, and writing–review and editing. VN: resources, supervision, visualization, and writing–review and editing.
Funding
The author(s) declare financial support was received for the research, authorship, and/or publication of this article. The authors acknowledge the support provided by the Researchers Supporting Project, Number RSP2024R358, King Saud University, Riyadh, Saudi Arabia.
Acknowledgments
The author acknowledges GBPUAT, Pantnagar, for granting access to research facilities and the UGC for the CSIR-NET-SRF. The author acknowledges IIT Roorkee (IIC) for PXRD, Indian Agriculture Research Institute, Delhi, for AAS, IIT Ropar (CRF) for NMR characterization, LPU Punjab (CIF) for FTIR, and USIC DU for elemental analysis.
Conflict of interest
The authors declare that the research was conducted in the absence of any commercial or financial relationships that could be construed as a potential conflict of interest.
Publisher’s note
All claims expressed in this article are solely those of the authors and do not necessarily represent those of their affiliated organizations, or those of the publisher, the editors, and the reviewers. Any product that may be evaluated in this article, or claim that may be made by its manufacturer, is not guaranteed or endorsed by the publisher.
Supplementary material
The Supplementary Material for this article can be found online at: https://www.frontiersin.org/articles/10.3389/fchem.2024.1414646/full#supplementary-material
Abbreviations
AAS, atomic absorption spectra; B. subtilis, Bacillus subtilis; BHT, butylated hydroxytoluene; DMSO, dimethyl sulfoxide; DPPH, 1,1-diphenylpicrylhydrazyl; E. coli, Escherichia coli; FTIR, Fourier-transform infrared; MHz, megahertz; NMR, nuclear magnetic resonance; PDB, Protein Data Bank; ppm, parts per million; PXRD, powder X-ray diffractometer; S. aureus, Staphylococcus aureus; S. typhi, Salmonella typhi; TLC, thin-layer chromatography; TMS, tetra methyl silane; TPTZ, 2,4, 6-tri (2-pyridyl)-s-triazine; UV–vis, ultraviolet–visible.
References
Abdallah, S. M., Zayed, M. A., and Mohamed, G. G. (2010). Synthesis and spectroscopic characterization of new tetradentate Schiff base and its coordination compounds of NOON donor atoms and their antibacterial and antifungal activity. Arab. J. Chem. 3 (2), 103–113. doi:10.1016/j.arabjc.2010.02.006
Abd El-Hamid, S. M., Sadeek, S. A., Mohammed, S. F., Ahmed, F. M., and El-Gedamy, M. S. (2023). N2O2-chelate metal complexes with Schiff base ligand: synthesis, characterisation and contribution as a promising antiviral agent against human cytomegalovirus. Appl. Organomet. Chem. 37 (2), e6958. doi:10.1002/aoc.6958
Abd El-Lateef, H. M., Adam, M. S. S., and Khalaf, M. M. (2018). Synthesis of polar unique 3d metal-imine complexes of salicylidene anthranilate sodium salt. Homogeneous catalytic and corrosion inhibition performance. J. Taiwan Inst. Chem. Eng. 88, 286–304. doi:10.1016/j.jtice.2018.04.024
Abd El Wahed, M., Nour, E., Teleb, S., and Fahim, S. (2004). Thermodynamic and thermal investigation of Co (II), Ni (II), and Cu (II) complexes with adenine. J. Therm. Anal. Calorim. 76 (1), 343–348. doi:10.1023/b:jtan.0000027834.34263.48
Abou-Hussein, A. A., and Linert, W. (2015). Synthesis, spectroscopic studies and inhibitory activity against bactria and fungi of acyclic and macrocyclic transition metal complexes containing a triamine coumarine Schiff base ligand. Spectrochim. Acta A Mol. Biomol. Spectrosc. 141, 223–232. doi:10.1016/j.saa.2015.01.063
Abu-Dief, A. M., El-Sagher, H. M., and Shehata, M. R. (2019). Fabrication, spectroscopic characterization, calf thymus DNA binding investigation, antioxidant and anticancer activities of some antibiotic azomethine Cu (II), Pd (II), Zn (II) and Cr (III) complexes. Appl. Organomet. Chem. 33 (8), e4943. doi:10.1002/aoc.4943
Aljohani, F. S., Omran, O. A., Ahmed, E. A., Al-Farraj, E. S., Elkady, E. F., Alharbi, A., et al. (2023). Design, structural inspection of new bis (1H-benzo [d] imidazol-2-yl) methanone complexes: biomedical applications and theoretical implementations via DFT and docking approaches. Inorg. Chem. Commun. 148, 110331. doi:10.1016/j.inoche.2022.110331
Alorini, T. A., Al-Hakimi, A. N., Saeed, S. E. S., Alhamzi, E. H. L., and Albadri, A. E. (2022). Synthesis, characterization, and anticancer activity of some metal complexes with a new Schiff base ligand. Arab. J. Chem. 15 (2), 103559. doi:10.1016/j.arabjc.2021.103559
Amato, G., Grasso, E., Longo, V., and Gervasi, P. G. (2001). Oxidation of N, N-dimethylformamide and N, N-diethylformamide by human liver microsomes and human recombinant P450s. Toxicol. Lett. 124 (1-3), 11–19. doi:10.1016/S0378-4274(01)00324-1
Aslan, H. G., Akkoç, S., and Kökbudak, Z. (2020). Anticancer activities of various new metal complexes prepared from a Schiff base on A549 cell line. Inorg. Chem. Commun. 111, 107645. doi:10.1016/j.inoche.2019.107645
Belal, A. A. M., El-Deen, I. M., Farid, N. Y., Zakaria, R., and Refat, M. S. (2015). Synthesis, spectroscopic, coordination and biological activities of some transition metal complexes containing ONO tridentate Schiff base ligand. Spectrochim. Acta A Mol. Biomol. Spectrosc. 149, 771–787. doi:10.1016/j.saa.2015.05.005
Benzie, I. F., and Strain, J. J. (1996). The ferric reducing ability of plasma (FRAP) as a measure of “antioxidant power”: the FRAP assay. Anal. Biochem. 239 (1), 70–76. doi:10.1006/abio.1996.0292
Beyazit, N., Çakran, H. S., Cabir, A., Akışcan, Y., and Demetgül, C. (2020). Synthesis, characterization and antioxidant activity of chitosan Schiff base derivatives bearing (−)-gossypol. Carbohydr. Polym. 240, 116333. doi:10.1016/j.carbpol.2020.116333
Bhandarkar, S. E., Pathare, P. P., and Khobragade, B. P. (2023). New nickel (II), copper (II) and cobalt (II) complexes based salicyaldehyde schiff base: synthesis, characterisation, and antiviral activity. Mat. Today Proc. 92, 807–816. doi:10.1016/j.matpr.2023.04.381
Bingöl, M., and Turan, N. (2020). Schiff base and metal (II) complexes containing thiophene-3-carboxylate: synthesis, characterization and antioxidant activities. J. Mol. Struct. 1205, 127542. doi:10.1016/j.molstruc.2019.127542
Borrego-Muñoz, P., Becerra, L. D., Ospina, F., Coy-Barrera, E., and Quiroga, D. (2022). Synthesis (Z) vs (E) selectivity, antifungal activity against Fusarium oxysporum, and structure-based virtual screening of novel Schiff bases derived from L-tryptophan. ACS omega 7 (28), 24714–24726. doi:10.1021/acsomega.2c02614
Bowman, A. B., Kwakye, G. F., Hernández, E. H., and Aschner, M. (2011). Role of manganese in neurodegenerative diseases. J. Trace Elem. Med. Biol. 25 (4), 191–203. doi:10.1016/j.jtemb.2011.08.144
Brand-Williams, W., Cuvelier, M. E., and Berset, C. L. W. T. (1995). Use of a free radical method to evaluate antioxidant activity. LWT - Food Sci. Technol. 28 (1), 25–30. doi:10.1016/S0023-6438(95)80008-5
Bruns, H., Patil, M., Carreras, J., Vázquez, A., Thiel, W., Goddard, R., et al. (2010). Synthesis and coordination properties of nitrogen (I)-based ligands. Angew. Chem. Int. Ed. 21 (49), 3680–3683. doi:10.1002/anie.200906168
Buldurun, K., Turan, N., Aras, A., Mantarcı, A., Turkan, F., and Bursal, E. (2019). Spectroscopic and structural characterization, enzyme inhibitions, and antioxidant effects of new Ru (II) and Ni (II) complexes of Schiff base. Chem. Biodivers. 16 (8), e1900243. doi:10.1002/cbdv.201900243
Chasapis, C. T., Ntoupa, P. S. A., Spiliopoulou, C. A., and Stefanidou, M. E. (2020). Recent aspects of the effects of zinc on human health. Arch. Toxicol. 94, 1443–1460. doi:10.1007/s00204-020-02702-9
Chen, Z., Li, H., Cao, G., Xu, J., Miao, M., and Ren, H. (2017). Copper-Catalyzed double C–N bond formation for the synthesis of diverse benzimidazoles from N-Alkyl-2-iodoaniline and sodium azide. Synlett 28 (04), 504–508. doi:10.1055/s-0036-1588086
Cheng, K., Zheng, Q. Z., Qian, Y., Shi, L., Zhao, J., and Zhu, H. L. (2009). Synthesis, antibacterial activities and molecular docking studies of peptide and Schiff bases as targeted antibiotics. Bioorg. Med. Chem. 17 (23), 7861–7871. doi:10.1016/j.bmc.2009.10.037
Damiche, R., and Chafaa, S. (2017). Synthesis of new bioactive aminophosphonates and study of their antioxidant, anti-inflammatory and antibacterial activities as well the assessment of their toxicological activity. J. Mol. Struct. 1130, 1009–1017. doi:10.1016/j.molstruc.2016.10.054
De, A., Ray, H. P., Jain, P., Kaur, H., and Singh, N. (2020). Synthesis, characterization, molecular docking and DNA cleavage study of transition metal complexes of o-vanillin and glycine derived Schiff base ligand. J. Mol. Struct. 1199, 126901. doi:10.1016/j.molstruc.2019.126901
Devi, J., and Batra, N. (2015). Synthesis, characterization and antimicrobial activities of mixed ligand transition metal complexes with isatin monohydrazone Schiff base ligands and heterocyclic nitrogen base. Spectrochim. Acta A Mol. Biomol. Spectrosc. 135, 710–719. doi:10.1016/j.saa.2014.07.041
Devi, J., Batra, N., and Malhotra, R. (2012). Ligational behavior of Schiff bases towards transition metal ion and metalation effect on their antibacterial activity. Spectrochim. Acta A Mol. Biomol. Spectrosc. 97, 397–405. doi:10.1016/j.saa.2012.06.026
Devi, J., Yadav, J., and Singh, N. (2019). Synthesis, characterisation, in vitro antimicrobial, antioxidant and anti-inflammatory activities of diorganotin (IV) complexes derived from salicylaldehyde Schiff bases. Res. Chem. Intermed. 45, 3943–3968. doi:10.1007/s11164-019-03830-3
Ding, S., and Jiao, N. (2012). N, N-dimethylformamide: a multipurpose building block. Angew. Chem. Int. Ed. 51 (37), 9226–9237. doi:10.1002/anie.201200859
El-Gammal, O. A., Mohamed, F. S., Rezk, G. N., and El-Bindary, A. A. (2021). Synthesis, characterization, catalytic, DNA binding and antibacterial activities of Co (II), Ni (II) and Cu (II) complexes with new Schiff base ligand. J. Mol. Liq. 326, 115223. doi:10.1016/j.molliq.2020.115223
El-Megharbel, S. M., and Hamza, R. Z. (2022). Synthesis, spectroscopic characterizations, conductometric titration and investigation of potent antioxidant activities of gallic acid complexes with Ca (II), Cu (II), Zn (III), Cr (III) and Se (IV) metal ions. J. Mol. Liq. 358, 119196. doi:10.1016/j.molliq.2022.119196
El-Sherif, A. A., and Eldebss, T. M. (2011). Synthesis, spectral characterization, solution equilibria, in vitro antibacterial and cytotoxic activities of Cu (II), Ni (II), Mn (II), Co (II) and Zn (II) complexes with Schiff base derived from 5-bromosalicylaldehyde and 2-aminomethylthiophene. Spectrochim. Acta A Mol. Biomol. Spectrosc. 79 (5), 1803–1814. doi:10.1016/j.saa.2011.05.062
Geary, W. J. (1971). The use of conductivity measurements in organic solvents for the characterisation of coordination compounds. Coord. Chem. Rev. 7 (1), 81–122. doi:10.1016/S0010-8545(00)80009-0
Ghanghas, P., Choudhary, A., Kumar, D., and Poonia, K. (2021). Coordination metal complexes with Schiff bases: useful pharmacophores with comprehensive biological applications. Inorg. Chem. Commun. 130, 108710. doi:10.1016/j.inoche.2021.108710
Ismael, M., Abdou, A., and Abdel-Mawgoud, A. M. (2018). Synthesis, characterization, modeling, and antimicrobial activity of FeIII, CoII, NiII, CuII, and ZnII complexes based on tri-substituted imidazole ligand. Z. Anorg. Allg. Chem. 644 (20), 1203–1214. doi:10.1002/zaac.201800230
Jackson, A., and Meth-Cohn, O. (1995). A new short and efficient strategy for the synthesis of quinolone antibiotics. J. Chem. Soc. Chem. Commun. 13, 1319. doi:10.1039/C39950001319
Joshi, R., Kumari, A., Singh, K., Mishra, H., and Pokharia, S. (2020). Triorganotin (IV) complexes of Schiff base derived from 1, 2, 4-triazole moiety: synthesis, spectroscopic investigation, DFT studies, antifungal activity and molecular docking studies. J. Mol. Struct. 1206, 127639. doi:10.1016/j.molstruc.2019.127639
Junaid, M., Alam, M. J., Hossain, M. K., Halim, M. A., and Ullah, M. O. (2018). Molecular docking and dynamics of Nickel-Schiff base complexes for inhibiting β-lactamase of Mycobacterium tuberculosis. Silico Pharmacol. 6, 6–11. doi:10.1007/s40203-018-0044-6
Juyal, V. K., Pathak, A., Panwar, M., Thakuri, S. C., Prakash, O., Agrwal, A., et al. (2023a). Schiff base metal complexes as a versatile catalyst: a review. J. Org. Chem. 999, 122825. doi:10.1016/j.jorganchem.2023.122825
Juyal, V. K., Thakuri, S. C., Panwar, M., Prakash, O., and Nand, V. (2023b). Synthesis, characterization, and in silico molecular docking study of bidentate hydroxy α-aminophosphonates derivative and its Mn (II), Fe (III) and Zn (II) metal complexes as potent antioxidant and antibacterial agents. J. Indian Chem. Soc. 100 (8), 101041. doi:10.1016/j.jics.2023.101041
Kanwal, A., Parveen, B., Ashraf, R., Haider, N., and Ali, K. G. (2022). A review on synthesis and applications of some selected Schiff bases with their transition metal complexes. J. Coord. Chem. 75 (19-24), 2533–2556. doi:10.1080/00958972.2022.2138364
Kargar, H., Ardakani, A. A., Tahir, M. N., Ashfaq, M., and Munawar, K. S. (2021). Synthesis, spectral characterization, crystal structure and antibacterial activity of nickel (II), copper (II) and zinc (II) complexes containing ONNO donor Schiff base ligands. J. Mol. Struct. 1233, 130112. doi:10.1016/j.molstruc.2021.130112
Kaya, S., Erkan, S., and Karakaş, D. (2021). Computational investigation of molecular structures, spectroscopic properties and antitumor-antibacterial activities of some Schiff bases. Spectrochim. Acta A Mol. Biomol. Spectrosc. 244, 118829. doi:10.1016/j.saa.2020.118829
Keypour, H., Shayesteh, M., Rezaeivala, M., Chalabian, F., and Valencia, L. (2013). Synthesis and characterization of a series of transition metal complexes with a new symmetrical polyoxaaza macroacyclic Schiff base ligand: X-ray crystal structure of cobalt (II) and nickel (II) complexes and their antibacterial properties. Spectrochim. Acta A Mol. Biomol. Spectrosc. 101, 59–66. doi:10.1016/j.saa.2012.09.048
Kobayashi, K., Nagato, S., Kawakita, M., Morikawa, O., and Konishi, H. (1995). Synthesis of 1-formyl-1, 2-dihydroquinoline derivatives by a Lewis acid-catalyzed cyclization of o-(1-hydroxy-2-alkenyl) phenyl isocyanides. Chem. Lett. 24 (7), 575–576. doi:10.1246/cl.1995.575
Krishna, G. A., Dhanya, T. M., Shanty, A. A., Raghu, K. G., and Mohanan, P. V. (2023). Transition metal complexes of imidazole derived Schiff bases: antioxidant/anti-inflammatory/antimicrobial/enzyme inhibition and cytotoxicity properties. J. Mol. Struct. 1274, 134384. doi:10.1016/j.molstruc.2022.134384
Liang, H., Zhao, T., Ou, J., Liu, J., and Hu, X. (2023). Catalyst-free N-formylation of amines using formic acid as a sustainable C1 source. ACS Sustain. Chem. Eng. 11 (39), 14317–14322. doi:10.1021/acssuschemeng.3c04457
Lohray, B. B., Baskaran, S., Rao, B. S., Reddy, B. Y., and Rao, I. N. (1999). A short synthesis of oxazolidinone derivatives linezolid and eperezolid: a new class of antibacterials. Tetrahedron Lett. 40 (26), 4855–4856. doi:10.1016/S0040-4039(99)00893-X
Malanovic, N., and Lohner, K. (2016). Gram-positive bacterial cell envelopes: the impact on the activity of antimicrobial peptides. Biochim. Biophys. Acta, Biomembr. 1858 (5), 936–946. doi:10.1016/j.bbamem.2015.11.004
Mishra, A. K., Manav, N., and Kaushik, N. K. (2005). Organotin (IV) complexes of thiohydrazones: synthesis, characterization and antifungal study. Spectrochim. Acta A Mol. Biomol. 61 (13-14), 3097–3101. doi:10.1016/j.saa.2004.11.035
Mohanty, S. K., Khuntia, A., Yellasubbaiah, N., Ayyanna, C., Sudha, B. N., and Harika, M. S. (2018). Design, synthesis of novel azo derivatives of benzimidazole as potent antibacterial and antitubercular agents. Beni-Suef Univ. J. basic Appl. Sci. 7, 646–651. doi:10.1016/j.bjbas.2018.07.009
Mumtaz, A., Mahmud, T., Khalid, M., Khan, H., Sadia, A., Samra, M. M., et al. (2020). Biological evaluation of synthesized schiff base-metal complexes derived from sulfisomidine. J. Pharm. Innov. 17, 37–45. doi:10.1007/s12247-020-09476-8
Muthukumar, R., Karnan, M., Elangovan, N., Karunanidhi, M., and Thomas, R. (2022). Synthesis, spectral analysis, antibacterial activity, quantum chemical studies and supporting molecular docking of Schiff base (E)-4-((4-bromobenzylidene) amino) benzenesulfonamide. J. Indian Chem. Soc. 99 (5), 100405. doi:10.1016/j.jics.2022.100405
Muzart, J. (2009). N, N-Dimethylformamide: much more than a solvent. Tetra 65 (40), 8313–8323. doi:10.1016/j.tet.2009.06.091
Pagadala, N. S., Syed, K., and Tuszynski, J. (2017). Software for molecular docking: a review. Biophys. Rev. 9, 91–102. doi:10.1007/s12551-016-0247-1
Sanner, M. F. (1999). Python: a programming language for software integration and development. J. Mol. Graph Model. 17 (1), 57–61.
Shahraki, S. (2022). Schiff base compounds as artificial metalloenzymes. Colloids Surf. B Biointerfaces 218, 112727. doi:10.1016/j.colsurfb.2022.112727
Sharma, M., Singh, S., and Sharma, S. (2015). New generation antibiotics/antibacterials: deadly arsenal for disposal of antibiotic resistant bacteria. J. Microb. Biochem. Technol. 7, 374–379. doi:10.4172/1948-5948.1000241
Shiryaev, A. A., Goncharenko, A. N., Burkhanova, T. M., Alkhimova, L. E., Babashkina, M. G., Chandrasekaran, R., et al. (2021). A chiral (1 R, 2 R)-N, N′-bis-(salicylidene)-1, 2-diphenyl-1, 2-ethanediamine Schiff base dye: synthesis, crystal structure, Hirshfeld surface analysis, computational study, photophysical properties and in silico antifungal activity. J. Iran. Chem. Soc. 18, 2897–2911. doi:10.1007/s13738-021-02237-5
Soliman, A. A., and Mohamed, G. G. (2004). Study of the ternary complexes of copper with salicylidene-2-aminothiophenol and some amino acids in the solid state. Thermo. Acta 421 (1-2), 151–159. doi:10.1016/j.tca.2004.03.010
Sundararajan, M. L., Jeyakumar, T., Anandakumaran, J., and Selvan, B. K. (2014). Synthesis of metal complexes involving Schiff base ligand with methylenedioxy moiety: spectral, thermal, XRD and antimicrobial studies. Spectrochim. Acta A Mol. Biomol. Spectrosc. 131, 82–93. doi:10.1016/j.saa.2014.04.055
Tadele, K. T., and Tsega, T. W. (2019). Schiff Bases and their metal complexes as potential anticancer candidates: a review of recent works. Anticancer Agents Med. Chem. 19 (15), 1786–1795. doi:10.2174/1871520619666190227171716
Tweedy, B. G. (1964). Plant extracts with metal ions as potential antimicrobial agents. J. Phytopathol. 55, 910–914.
Ueno, T., Ohashi, M., Kono, M., Kondo, K., Suzuki, A., Yamane, T., et al. (2004). Crystal structures of artificial metalloproteins: tight binding of FeIII (Schiff-Base) by mutation of Ala71 to Gly in apo-myoglobin. Inorg. Chem. 43 (9), 2852–2858. doi:10.1021/ic0498539
Vaghasiya, Y. K., Nair, R., Soni, M., Baluja, S., and Shanda, S. (2004). Synthesis, structural determination and antibacterial activity of compounds derived from vanillin and 4-aminoantipyrine. J. Serb. Chem. Soc. 69 (12), 991–998. doi:10.2298/JSC0412991V
Wolohan, P., and Reichert, D. E. (2004). Use of binding energy in comparative molecular field analysis of isoform selective estrogen receptor ligands. J. Mol. Graph. Model. 23 (1), 23–38. doi:10.1016/j.jmgm.2004.03.002
Xu, Y., Shi, Y., Lei, F., and Dai, L. (2020). A novel and green cellulose-based Schiff base-Cu (II) complex and its excellent antibacterial activity. Carbohydr. Polym. 230, 115671. doi:10.1016/j.carbpol.2019.115671
Yadav, M., Sharma, S., and Devi, J. (2021). Designing, spectroscopic characterization, biological screening and antioxidant activity of mononuclear transition metal complexes of bidentate Schiff base hydrazones. J. Chem. Sci. 133, 21–22. doi:10.1007/s12039-020-01854-6
Keywords: formamide, Schiff base, antibacterial, antioxidant, in silico molecular docking
Citation: Juyal VK, Thakuri SC, Panwar M, Rashmi , Prakash O, Perveen K, Bukhari NA and Nand V (2024) Manganese(II) and Zinc(II) metal complexes of novel bidentate formamide-based Schiff base ligand: synthesis, structural characterization, antioxidant, antibacterial, and in-silico molecular docking study. Front. Chem. 12:1414646. doi: 10.3389/fchem.2024.1414646
Received: 09 April 2024; Accepted: 18 June 2024;
Published: 19 July 2024.
Edited by:
Magdalena Barwiolek, Nicolaus Copernicus University in Toruń, PolandReviewed by:
Rodica Olar, University of Bucharest, RomaniaJoanna Masternak, Jan Kochanowski University, Poland
Copyright © 2024 Juyal, Thakuri, Panwar, Rashmi, Prakash, Perveen, Bukhari and Nand. This is an open-access article distributed under the terms of the Creative Commons Attribution License (CC BY). The use, distribution or reproduction in other forums is permitted, provided the original author(s) and the copyright owner(s) are credited and that the original publication in this journal is cited, in accordance with accepted academic practice. No use, distribution or reproduction is permitted which does not comply with these terms.
*Correspondence: Vijay Kumar Juyal, vijayjuyal1795@gmail.com; Viveka Nand, imvivekanand@gmail.com