- College of Materials and Chemistry, China Jiliang University, Hangzhou, China
Water pollution caused by antibiotics is a growing problem and photodegradation by efficient catalysts is an environmentally friendly technology that can effectively degrade organic pollutants in water. Here, a novel method was innovatively used to synthesize niobium oxyfluoride (Nb3O7F) nanosheets decorated with Au nanoparticles, which is the first report for the composites of Au and Nb3O7F. We prepared the Nb3O7F nanosheets via hydrothermal synthesis followed by deposition of Au nanoparticles on their surface using HAuCl4. The prepared samples were characterized by XRD, HRTEM, XPS, and UV–Vis. The diameters of most Au NPs are ranging from 5 to 25 nm with an average size of about 16.9 nm, as well as the Nb3O7F nanosheets in size ranging from 200 nm to 700 nm. The chemical composition of the Au-Nb3O7F showed a Au/Nb atomic ratio of 1/10, as well as a Nb/O/F ratio of 3/7/1. UV–Vis spectrum reveals a largest absorption peak at 520 nm for the Au-Nb3O7F nanosheets. The prepared Au-Nb3O7F nanomaterials were applied to the visible-light photodegradation of tetracycline hydrochloride, with the photocatalytic degradation rate reached more than 50% under the optimal conditions within 1 h. Capture experiments indicated that h+ and •O2- are the main active substances involved during the course of the photodegradation. Furthermore, the proposed mechanism for the photodegradation of the novel Au-Nb3O7F nanosheets was given.
Introduction
Development of new efficient photocatalysts addressed to environmentally friendly processes, aiming at the elimination of organic pollutants and transformations of waste is still an emerging issue (Yang et al., 2021a; Shurbaji et al., 2021; Zhang Y. et al., 2022; De Ilurdoz et al., 2022; Balakrishnan et al., 2023; Li et al., 2023; Lanjwani et al., 2024; Vinayagam et al., 2024). Environmentally friendly semiconductors, such as TiO2, ZnO, Nb2O5, are efficient heterogeneous photocatalysts (Lang et al., 2014; Ani et al., 2018; Anwer et al., 2019; Wetchakun et al., 2019; Jiang et al., 2020; Zhao et al., 2020). In most cases, these semiconductors only respond to UV light irradiation and cannot be efficiently excited under visible light due to their wide band gaps (ca. 3.2 eV for TiO2, ZnO, and Nb2O5), leading to limited utility for photosynthesis and photodegradations. According to the literature, one of the effective methods to improve their activity under visible light irradiation and achieve this goal is to design more complex catalytic systems and construct heterojunction containing plasmonic metals, in order to broaden the absorption spectrum as well as to improve the carrier separation efficiency (Leong et al., 2018; Prakash et al., 2018; Yang et al., 2021b; Gao et al., 2022; Liu et al., 2022; Ly et al., 2023; Mishra et al., 2023). Up to now, many researchers have reported that these large band gap semiconductors combined with noble metal nanoparticles (such as Au, Ag, etc.) are effective photocatalysts for photoelectrochemical water splitting and photocatalytic degradation of organic dyes and pollutants (Leong et al., 2018; Prakash et al., 2018; Ly et al., 2023).
Nb3O7F is a metal-oxyfluoride semiconductor with a band gap of ca. 2.9–3.2 eV, as well as low cost and high chemical stability. Nb3O7F is a commonly used material for a variety of applications including catalysts, sensors, recording materials, electrochemical supercapacitors, Li-ion batteries, and field emission materials (Idrees et al., 2014; Hirai et al., 2018; Chen et al., 2021; Li et al., 2021; Zhang et al., 2021). However, Au combined with Nb3O7F had been never investigated before, including photocatalytic degradation of contaminants in water. Furthermore, tetracycline is one of the mostly occurring synthetic antibiotics and is commonly used, leading to a growing problem of water pollution. As a result, degradation of such highly stable antibiotic is much needed (Xiao et al., 2020; Qin et al., 2021; Zhang J. et al., 2022; Huang et al., 2022; Zhao L. et al., 2023; Umair et al., 2023; Yuan et al., 2023). Common methods like adsorption on activated carbons and reverse osmosis are adopted for this purpose. However, these are not effective in destructing and demolishing the contaminants. Ozonation and chlorination are other methods for antibiotics degradation and contaminants elimination from water but they are considered as expensive and inefficient to use (Choi et al., 2008; Sánchez-Polo et al., 2015; Su et al., 2020; Calcio Gaudino et al., 2021; Lu et al., 2021). Hence there is a need of the production of such photocatalyst, which are less toxic and more effective as well as can easily degrade these contaminants from water.
Herein, we successfully synthesized a kind of novel Au-Nb3O7F composite nanosheets for the first time, which could be used in the visible light photocatalytic degradation of tetracycline hydrochloride. The Nb3O7F nanosheets were prepared via different methods followed by the deposition of gold nanoparticles on their surface using HAuCl4. The prepared nanosheets were characterized by XRD, HRTEM, XPS, and UV–Vis. The composite nanoparticles of Nb3O7F nanosheets decorated with gold nanoparticles demonstrate distinct and robust surface plasmon resonance (SPR) effects within the visible light range. Photocatalytic activity of the nanomaterials was evaluated under visible light irradiation and demonstrated that the decoration of the gold nanoparticles with the Nb3O7F nanosheets led to an enhancement of the photocatalyst performance. Furthermore, preliminary mechanistic studies indicated that h+ and •O2− are the main active substances in the degradation of TC-HCl.
Materials and methods
Materials
Chemicals such as niobium nanopowder (Nb, 99.9%, 60–80 nm), anatase titanium dioxide (TiO2, 99.8%, 60 nm), zinc oxide (ZnO, 99.9%, 30 ± 10 nm), niobium pentoxide (Nb2O5, 99.9%, AR), tert-butanol (C4H10O, TBA, >99.0%, AR), ethylene diamine tetraacetic acid disodium salt dihydrate (C10H14N2Na2O8.2H2O, EDTA·2Na, 98%, AR), sodium sulfate (Na2SO4, AR), tetracycline hydrochloride (C22H24N2O8·HCl, TC-HCl, BR), polyvinyl pyrrolidone ((C6H9NO)n, PVP, Average Mw: 8000, K16-K18, AR), L-ascorbic acid (C6H8O6, >99.0%, AR), hydrofluoric acid (HF, 40%, AR), Nafion (D520, 5wt% in mixture of lower aliphatic alcohols) had been all purchased from Macklin Chemical Co., Ltd. Ethanol (C2H6O, 99.7%, AR), 2,2,6,6-tetramethylpiperidinooxy (C9H18NO, TEMPO, 98%, AR) had been all purchased from Hangzhou Shuanglinchem Co., Ltd. Chloroauric acid (HAuCl4, 99%, AR) was purchased from CIVI(Shanghai). Ultrapure water was used in the process of all the experiments. All chemical reagents have been used as received without any further purification.
Synthesis of Nb3O7F nanosheets
The Nb3O7F nanosheets were synthesized through a novel simple hydrothermal method. Niobium nanopowder (diameter: 60–80 nm, 0.500 g, 5.38 mmol), hydrofluoric acid (1.000 mL, w/w: 40%) were added into 50.0 mL deionized-water and stirred. After stirring for 2 h at room temperature, the solution was transferred into a Teflon-lined stainless steel autoclave for a hydrothermal reaction at 160°C for 12 h. The samples were cooled down to room temperature, centrifuged, and washed with deionized water and ethanol. This grey sample was dried and labeled as Nb3O7F nanosheets.
Synthesis of Au-Nb3O7F nanosheets
The Au NPs were prepared by the chemical reduction method in a previous report (Leung et al., 2012). 0.100 g Nb3O7F nanosheets, 0.700 g Polyvinyl pyrrolidone, 1.200 g L-ascorbic acid was added into a 100 mL round-bottomed flask. Put it into an oil bath and heated until 90°C. Then a certain volume of HAuCl4 aqueous solution (0.050 g/mL) was added into the mixture. The color of the solution turns into wine red in the boiling state for 3 h. Finally, the sample was cooled down to room temperature, centrifuged, washed with deionized water and ethanol for three times respectively and putted into an oven at 60°C for 12 h. The dark-red solid sample was labeled as xAu-Nb3O7F (x refers to the mass ratio of HAuCl4 and Nb3O7F nanosheets, the exact amounts of HAuCl4 for each synthesis are shown in Table 1).
Sample characterization
X-ray diffraction (XRD) patterns were obtained on a Rigaku Ultima IV in the range 2θ was from 10° to 90° with the scan speed of 5°/min to identify the crystalline structure of Au-Nb3O7F nanosheets with graphite monochromatic Cu−Kα radiation (λ = 0.154178 nm). Scanning electron microscope (SEM) images and energy dispersive spectroscopy (EDS) spectra were captured using a Hitachi S-4800. For transmission electron microscopy (TEM) analysis, a drop of nanosheet dispersion in deionized water was dropped onto a nonfixed carbon-coated copper grid. Ultra high resolution transmission electron microscopy (HRTEM) images were acquired using a JEM-2100F with an accelerating voltage of 200 kV. X-ray photoelectron spectroscopy (XPS, Thermo Scientific K-Alpha) with a monochromatic Al Kα radiation was used to analyze the elemental composition on the surface and oxidation state of material. The ultraviolet−visible absorption spectrum was measured using Shimadzu UV-3600 integrating sphere.
Photoelectrochemical measurements
A 300 W Xe lamp assembled with a cutoff filter (λ > 400 nm) was used as the light source in the photocatalytic degradation experiment. First, 10 mg of catalyst is added to 50 mL of a certain concentration tetracycline hydrochloride (TC-HCl) solution in a beaker. Before the light irradiation, the solution with the photocatalyst was magnetically stirred in darkness for 1 h in order to establish adsorption equilibrium. During the light irradiation, 3.5 mL of the solution was taken out every 10 min and centrifuged to separate the solid. The concentration of TC-HCl was investigated at its maximum adsorption of 356 nm on the UV 2600 spectrophotometer. The photocatalytic degradation rate was then calculated. The photodegradation rate (DR) (%) of TC-HCl by the catalyst in this experiment was determined by using the following equation:
where Ai is the absorbance of the sample at the time of irradiation and A0 is the initial absorbance of the reactive degradates after reaching adsorption-desorption equilibrium.
Electrochemical tests were operated at the CHI660E electrochemical workstation. By using a standard three-electrode cell with a platinum wire as the counter electrode, a standard calomel electrode (SCE) as the reference electrode, and 10 mg of prepared Nb3O7F or 0.4 Au-Nb3O7F were separately dissolved in 1.5 mL of Nafion solution and coated on the FTO conducting glass (1 cm × 1 cm) by spin coating to form the working electrode, respectively. Forty milliliters of Na2SO4 (0.5 M) was used as an electrolyte and a 300 W Xe lamp assembled with a cutoff filter (λ > 400 nm) was used as the light source.
Results and discussion
Synthesis and characterization of Au-Nb3O7F nanoparticles
A schematic diagram of the synthesis process of Nb3O7F nanosheets and Au-Nb3O7F nanosheets is shown in Figure 1. These Au-Nb3O7F nanosheets were synthesized by a combined process of niobium nanopowder hydrothermal process and followed by a reduction process of HAuCl4 to form the gold nanoparticles to deposit on the Nb3O7F nanosheets.
Powder X-Ray Diffraction (XRD) was used to detect the crystal phase of the Au and Nb3O7F composite nanostructure. XRD diagrams for Au-Nb3O7F nanosheets and Nb3O7F nanosheets are presented in Figure 2, which shows that the XRD pattern accurately corresponds to monoclinic phase of Nb3O7F and Au, respectively. Peaks at 2θ values of 17.15°, 22.62°, 23.59°, 25.85°, 31.81°, 32.91°, 34.69°, 47.39°, 53.13°, and 84.76°, etc., corresponding to the Nb3O7F (JCPDS No. 74-2363), and the strong diffraction peaks observed also suggest that the product’s crystallinity is high. In the image we can also observe the reflection peak of Au NPs at 38.18°, 44.38°, 64.57°, and 77.56°, correspond to the peaks of Au (JCPDS No. 99-0056) on the Nb3O7F, which is weaker than the peaks correspond to Nb3O7F, without any detectable diffraction peaks of NbO2 or any other crystalline phase, thereby indicating that the product is the Au-Nb3O7F nanosheets.
The high resolution transmission electron microscopy (HRTEM) images of the Nb3O7F and the Au-Nb3O7F composites are shown in Figures 3A–D, respectively, indicated that the sample is in size ranging from 200–700 nm. These Au nanoparticles were successfully loaded on the surface of the Nb3O7F nanoparticles and the deposition of Au NPs does not change the original appearance of Nb3O7F nanosheets (Figures 3B–D). There are Au NPs on the surface of Nb3O7F nanosheets. Furthermore, energy dispersive spectroscopy (EDS) characterizations suggested that the sample contained Au, Nb, F, and O signals (Figure 3E). The EDS mapping images give the elemental distribution of the Nb3O7F nanosheets, which clearly proves that Nb, O, and F elements are uniformly distributed on these nanosheets, with the Au element distributed on these nanosheets as the nanoparticles. The HRTEM image indicates that the lattice spacings of 0.15 nm correspond to the (220) crystal planes of Au (Figure 3B). The diameter of most Au NPs on the surface of the nanosheets are ranging from 5 nm to 25 nm with an average size of about 16.9 nm (Figure 3F).
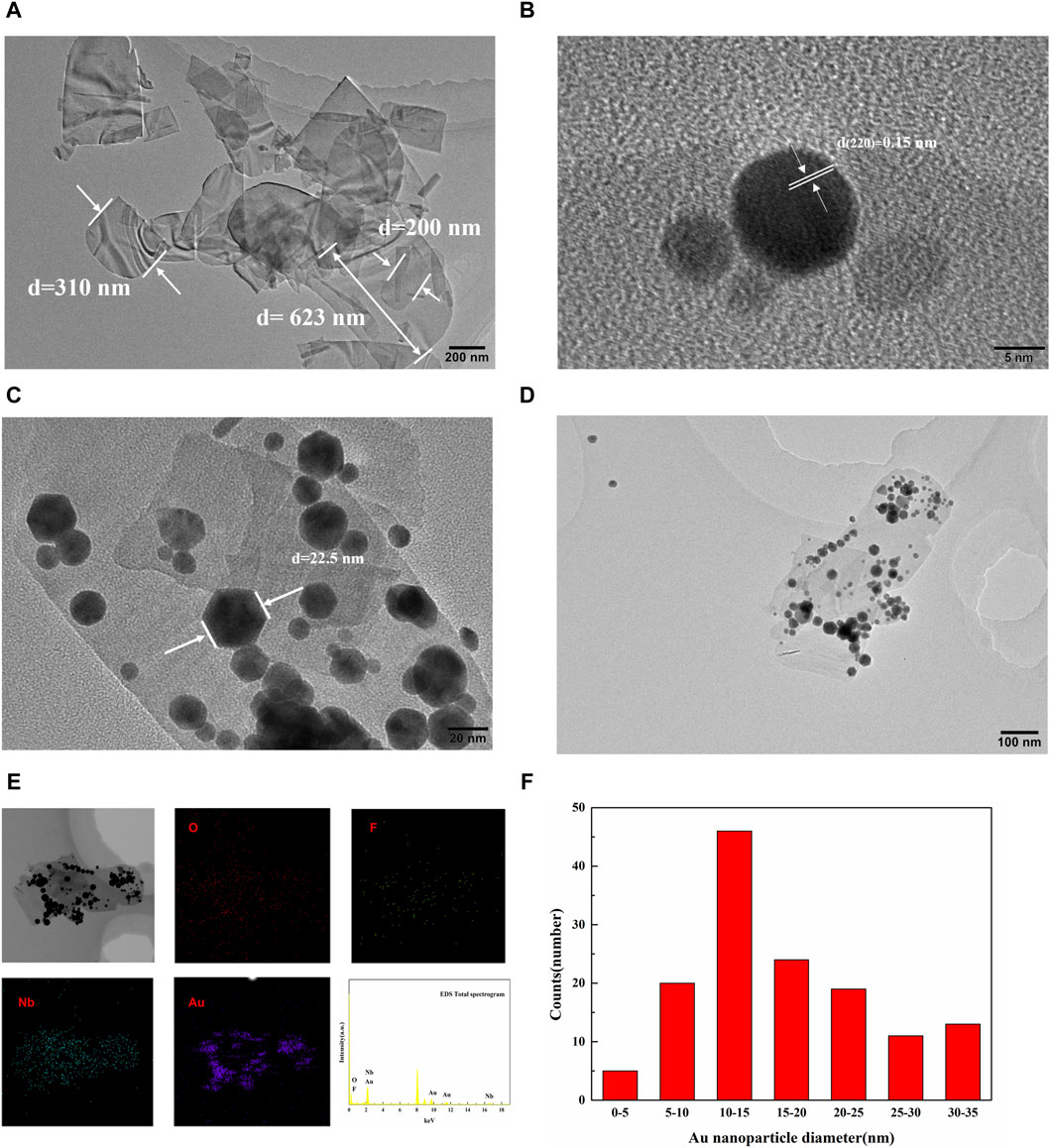
Figure 3. (A) HRTEM images of Nb3O7F; (B–D) HRTEM images of 0.4 Au-Nb3O7F; (E) EDS Mapping image of 0.4 Au-Nb3O7F; (F) histogram of the size distribution of Au NPs.
X-ray photoelectron spectroscopy (XPS) was used to analyze the composition and oxidation state of the atoms in the Au-Nb3O7F nanosheets. As shown in Figure 4A, the survey spectrum proves the existence of Au, Nb, O, and F elements. The peak at 684.3 eV is typically attributed to F 1s (Li et al., 2018). The peak at 530.2 eV is typically attributed to O 1s (Li et al., 2018). The oxidation state of the nanosheets are indicated that the nanosheets are composed of oxygen. The oxidation state of niobium, present as Nb5+, were differentiated by peak fitting of the Nb 3d spectra and the spectra of Nb 3d are shown in Figure 4B. The predominant peaks located at lower binding energies of 207.1 eV, was classified as characteristic of Nb 3d5/2. The higher binding energies of 209.8 eV are characteristic of the Nb 3d3/2 spin-orbit components. Two peaks were attributed to Nb5+ 3d (207.1 and 209.8 eV) (Li et al., 2018). Above results confirm the existence of Nb-F bond and Nb-O band (Li et al., 2018). The XPS of Au 4f5/2 and Au 4f7/2 are also shown in Figure 4C. The main peaks located at BEs of 83.1 and 86.8 eV representing metallic Au (Au0) (Dong et al., 2019; Lin et al., 2020). Based on these results, it can be confirmed that Au-Nb3O7F has been synthesized successfully. In addition, the XPS spectrum shows a Au/Nb atomic ratio of 1/10, as well as the nanosheets have a Nb/O/F atomic ratio of 3/7/1 (see Supplementary Table S2).
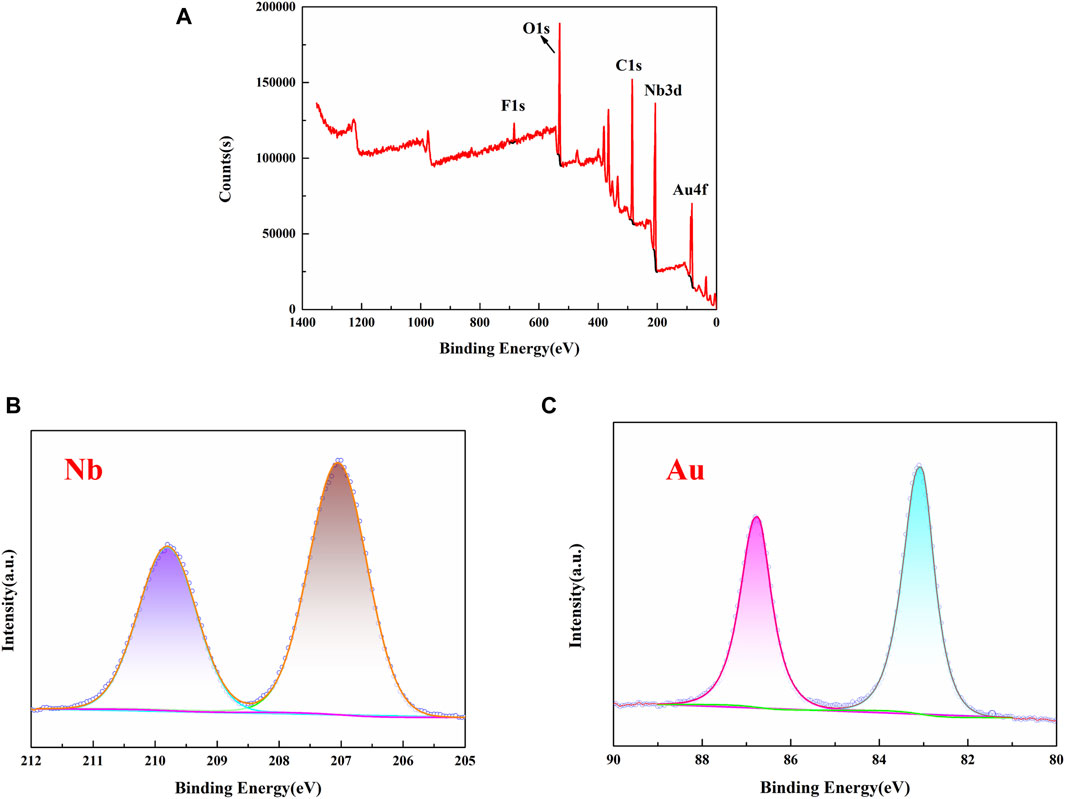
Figure 4. (A) XPS full spectrum of 0.4 Au-Nb3O7F; XPS fine spectra of (B) Nb 3d, and (C) Au 4f of 0.4 Au-Nb3O7F.
The photoresponse range of the catalyst is one of the important factors affecting the photocatalytic effect. The ultraviolet−visible (UV−Vis) absorption spectrum of 0.4 Au-Nb3O7F and Nb3O7F with wavelengths ranging from 200 nm to 800 nm were displayed in Figure 5. It can be seen from the figure that the Nb3O7F nanosheets without Au NPs mainly absorbs light less than 425 nm. However, the optical absorption of 0.4 Au-Nb3O7F nanosheets increases ranging greater than 400 nm in visible light region, as well as the largest absorption peak at 520 nm. It reveals a broad absorption ranging from 400 nm to 800 nm for the Au-Nb3O7F nanosheets. This optical phenomenon is likely attributed to the LSPR effect, which is believed to be closely related to the Au nanoparticles, and the largest absorption peak at 520 nm of 0.4 Au-Nb3O7F nanosheets was attributed to the Au NPs. The 0.4 Au-Nb3O7F nanosheets possess strong LSPR effect and are expected to an excellent candidate for visible light photodegradation (Cui et al., 2022; Guo et al., 2023; Zhao et al., 2024). In addition, the bandgap calculated by the Tauc’s plot is shown in Supplementary Figure S8. The band gaps of 0.4 Au-Nb3O7F nanosheets and Nb3O7F nanosheets are 2.38 eV and 3.28 eV, respectively, indicating that the forbidden bandwidth of the 0.4 Au-Nb3O7F became narrower than that of Nb3O7F, and the narrower forbidden bandwidth made the catalysts easier to be excited, resulting in a better photocatalytic effect.
Photocatalytic properties of the Au-Nb3O7F nanosheets
The degradation ability of the prepared photocatalysts was examined using tetracycline hydrochloride as the target pollutant. Figure 6 shows the graphs of the degradation of tetracycline hydrochloride under the same conditions for Nb3O7F nanosheets without gold nanoparticles, as well as the Au-Nb3O7F nanosheets with different ratios of gold nanoparticles. In Figure 6A, without any catalyst, the concentration of the tetracycline hydrochloride was almost not changed after 1 h of visible-light irradiation, and the degradation effect of Nb3O7F nanoparticles without Au NPs was the worst, with only 18.0% degradation after 1 h of visible-light irradiation. The degradation effect of the Nb3O7F nanosheets combined with any ratio of gold nanoparticles was better than that of the single Nb3O7F nanosheets, and the degradation effect was related to the mass ratio of the gold nanoparticles and the Nb3O7F nanosheets. The photocatalytic degradation rate of the prepared 0.4 Au-Nb3O7F composites reached 50.6% within 1 h. The degradation efficiency showed an increasing trend with the increasing loading of gold nanoparticles, which might be attributed to the fact that the loading of gold nanoparticles would improve the effect of the photocatalytic degradation. The degradation of tetracycline hydrochloride by all the photocatalysts was in accordance with the first order kinetic equation. Figure 6B shows the first-order kinetic fitting curves of Nb3O7F nanosheets, 0.1 Au-Nb3O7F, 0.2 Au-Nb3O7F and 0.4 Au-Nb3O7F, with rate constants of 7.43 × 10−3, 1.52 × 10−2, 2.16 × 10−2, and 2.80 × 10−2 min-1, respectively, and the reaction rate constant of 0.4 Au-Nb3O7F nanosheets was about 4 times as high as those of Nb3O7F nanosheets. HPLC was used to monitor whether the byproducts or intermediates were formed during the photocatalytic degradation of 0.4 Au-Nb3O7F nanosheets. The test results are shown in the Supplementary Material.
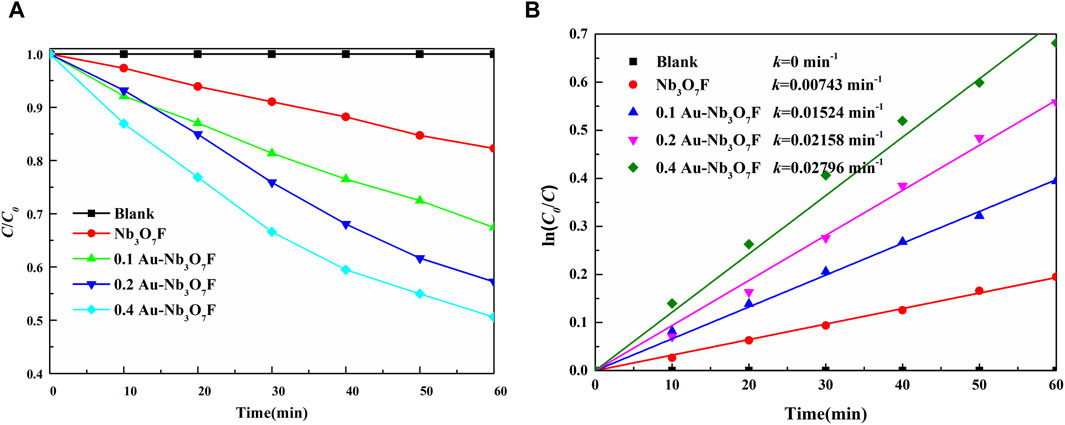
Figure 6. Photodegradation curves of tetracycline hydrochloride (A) photodegradation versus time curves; (B) photodegradation rate curves.
The reusability/sustainability of photocatalysts plays an important role in their practical usage. The selected 0.4 Au-Nb3O7F nanosheets were collected and washed after 1 h of the photocatalytic process and operated repeatedly for another three cycles according to the same procedures (see Supplementary Figure S4). However, the activity is significantly reduced at the 3rd cycle, which may result from the aggregation and dissociation of the gold nanoparticles from the Nb3O7F nanosheets. Additionally, aggregations of the gold nanoparticles are presented in the HRTEM image of 0.4 Au-Nb3O7F nanosheets after the 3rd cycle of the photocatalytic reaction (see Supplementary Figure S5). These features as well as the mass loss during the photodegradation process and the recycle process may contribute for the significant decrease of photocatalytic efficiency in the 3rd cycle.
The photocurrent at the light on/off conversion is a robust approach to analyzing the separation/transport of the photogenerated carriers, which is closely connected with the photocatalytic performance. Figure 7 shows the photocurrent response of Nb3O7F nanosheets and 0.4 Au-Nb3O7F nanoparticles under visible irradiation. Obvious photocurrents are present in both the photocatalysts when the irradiation is turned on, and 0.4 Au-Nb3O7F nanosheets have the greater photocurrent compared to Nb3O7F. This indicates that the 0.4 Au-Nb3O7F nanosheets may have the higher separation/migration efficiency of photoexcited carriers compared with Nb3O7F nanosheets without gold nanoparticles. This finding indicates that the combination of the Au nanoparticles as well as the Nb3O7F nanosheets may be benefit for the charge separation, leading to the improved photocatalytic performance (Liang et al., 2020; Guo et al., 2023).
Free radical capture experiments were used to investigate the active substance of 0.4 Au-Nb3O7F nanosheets in photocatalytic reactions. Tert-butanol (TBA), ethylene diamine tetraacetic acid disodium salt (EDTA·2Na), and TEMPO were added to the aqueous TC-HCl solution to capture the ·OH, h+, and ·O2− produced during the photodegradation process, respectively (Lang and Zhao, 2018; Zhang B. et al., 2022; Feng et al., 2022). From Figure 8, it can be seen that EDTA·2Na and TEMPO have a greater effect on the photocatalytic degradation effect, while TBA has little effect on the photocatalytic degradation effect, which proves that h+ and ·O2− are the main active substances in the degradation of TC-HCl by 0.4 Au-Nb3O7F nanosheets (Guo et al., 2019; Zhao Y. et al., 2023; Yang et al., 2023).
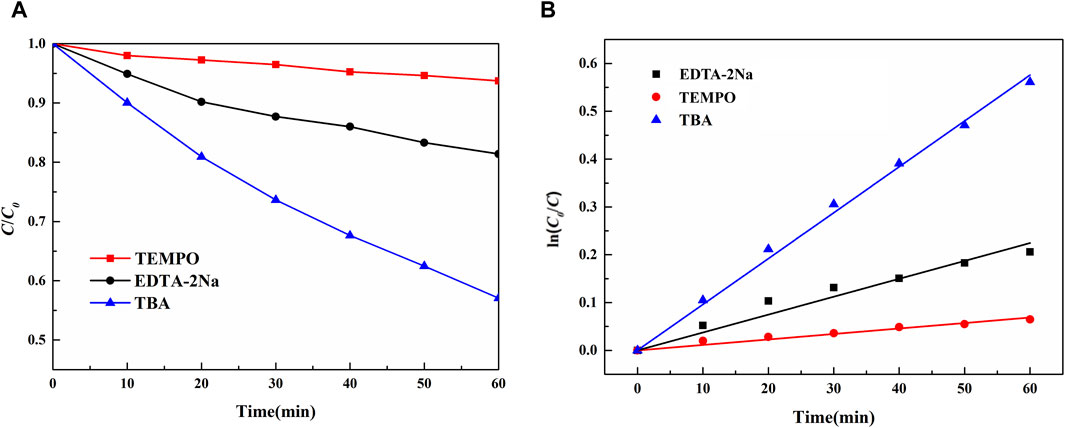
Figure 8. Photodegradation of tetracycline hydrochloride with different radical scavengers. (A) photodegradation versus time curves; (B) photodegradation rate curves.
According to the kinetic study, the presence of Au nanoparticles was able to increase the apparent rate constant of the reaction by 4 folds (Figure 6), which might be attributed to the fact that the loading of gold nanoparticles would improve the effect of the photocatalytic degradation. In order to determine the conduction band and valence band positions, the valence band spectra was obtained by XPS test, as shown in Figure 9A, and the valence band position of Nb3O7F was 2.54 eV (Kamat, 2002) (VB(Vs NHE)=Ф+2.78-4.44 =4.2+2.78-4.44 =2.54 eV, see ref. (Feng et al. 2020), indicating that the conduction band position of Nb3O7F was higher than the fermi level of gold nanoparticles, since the fermi level of gold is more positive (0.75 V) (Kamat, 2002). Combining the above data and previous reports, the photocatalytic mechanism for the photodegradation of the novel Au-Nb3O7F nanosheets was outlined in Figure 9(b). It is suggested that the most plausible mechanism for the plasmonic enhancement of photocatalytic in this study is via charge transfer and local electric field enhancement. Previous reports have suggested that when exposed to visible light radiation, the SPR process excites electrons in Au, which could then be transferred to the conduction band of the adjacent semiconductor Tian and Tatsuma, 2005; Mubeen et al., 2011; Khalil et al., 2019. Furthermore, the presence of SPR can also generate intense local electric fields creating “hot spot” regions near the surface of Au nanoparticles Khalil et al., 2019; Le et al. 2008. As a result, the population of electrons in the “hot spot” regions that can be migrated to CB of the adjacent semiconductor is also increased, which are essential for photocatalytic reactions. With electron transfer from the Au nanoparticles fill into the conduction band (CB) of Nb3O7F, leading to the formation of superoxide radicals, and the degradation of TC-HCl could occur. In addition, when exposed to visible light radiation, the positive hole (or positive charge) left in gold nanoparticles would get electrons from the TC-HCl, with the degradation of TC-HCl could occur.
Conclusion
In summary, we have successfully innovatively synthesized a kind of novel composite nanosheets composed of Au nanoparticles and Nb3O7F for the first time, which could be used for the photocatalytic degradation of tetracycline hydrochloride. The niobium oxyfluoride (Nb3O7F) nanosheets were prepared via hydrothermal synthesis, followed by deposition of gold nanoparticles on their surface. The hybrid nanosheets of Nb3O7F combined with gold nanoparticles demonstrate surface plasmon resonance (SPR) effects within the visible light range. Compared with Nb3O7F nanosheets without gold nanoparticles, the Au-Nb3O7F composite nanosheets have better photocurrent response efficiency, and exhibit better photocatalytic performance in the visible-light degradation of tetracycline hydrochloride, which could be applied in the treatment of organic pollutants in water.
Data availability statement
The original contributions presented in the study are included in the article/Supplementary Material, further inquiries can be directed to the corresponding authors.
Author contributions
ZW: Formal Analysis, Methodology, Writing–original draft, Investigation. LR: Formal Analysis, Funding acquisition, Investigation, Supervision, Writing–original draft, Writing–review and editing. ZC: Writing–review and editing. YC: Writing–review and editing, Methodology. XT: Methodology, Writing–review and editing. GW: Funding acquisition, Supervision, Writing–review and editing.
Funding
The author(s) declare that financial support was received for the research, authorship, and/or publication of this article. This work was financially supported by the Natural Science Foundation of Zhejiang Province (Grant No. LQ20B020011), National Natural Science Foundation of China (Grant No. 52171083), and China Jiliang University.
Conflict of interest
The authors declare that the research was conducted in the absence of any commercial or financial relationships that could be construed as a potential conflict of interest.
The handling editor EF is currently organizing a Research Topic with the author ZC.
Publisher’s note
All claims expressed in this article are solely those of the authors and do not necessarily represent those of their affiliated organizations, or those of the publisher, the editors and the reviewers. Any product that may be evaluated in this article, or claim that may be made by its manufacturer, is not guaranteed or endorsed by the publisher.
Supplementary material
The Supplementary Material for this article can be found online at: https://www.frontiersin.org/articles/10.3389/fchem.2024.1412457/full#supplementary-material
References
Ani, I. J., Akpan, U. G., Olutoye, M. A., and Hameed, B. (2018). Photocatalytic degradation of pollutants in petroleum refinery wastewater by TiO2- and ZnO-based photocatalysts: recent development. J. Clean. Prod. 205, 930–954. doi:10.1016/j.jclepro.2018.08.189
Anwer, H., Mahmood, A., Lee, J., Kim, K. H., Park, J. W., and Yip, A. C. K. (2019). Photocatalysts for degradation of dyes in industrial effluents: opportunities and challenges. Nano Res. 12, 955–972. doi:10.1007/s12274-019-2287-0
Balakrishnan, A., Chinthala, M., Polagani, R. K., and Vo, D. V. N. (2023). Removal of tetracycline from wastewater using g-C3N4 based photocatalysts: a review. Environ. Res. 216, 114660. doi:10.1016/j.envres.2022.114660
Calcio Gaudino, E., Canova, E., Liu, P., Wu, Z., and Cravotto, G. (2021). Degradation of antibiotics in wastewater: new advances in cavitational treatments. Molecules 26, 617. doi:10.3390/molecules26030617
Chen, Z., Li, Z., He, W., An, Y., Shen, L., Dou, H., et al. (2021). Nb3O7F mesocrystals: orientation formation and application in lithium-ion capacitors. CrystEngComm 23, 6012–6022. doi:10.1039/d1ce00600b
Choi, K. J., Kim, S. G., and Kim, S. H. (2008). Removal of tetracycline and sulfonamide classes of antibiotic compound by powdered activated carbon. Environ. Technol. 29, 333–342. doi:10.1080/09593330802102223
Cui, Z., Zhang, L., Wang, Y., and He, W. (2022). Plasmon excitation facilitating generation of electrons and reactive oxygen species for broad spectrum photocatalytic activity. Appl. Sur. Sci. 584, 152655. doi:10.1016/j.apsusc.2022.152655
De Ilurdoz, M. S., Sadhwani, J. J., and Reboso, J. V. (2022). Antibiotic removal processes from water and wastewater for the protection of the aquatic environment – a review. J. Water Process. Eng. 45, 102474. doi:10.1016/j.jwpe.2021.102474
Dong, L., Xin, Y., Liu, X., Guo, Y., Pao, C. W., Chen, J. L., et al. (2019). Selective hydrodeoxygenation of lignin oil to valuable phenolics over Au/Nb2O5 in water. Green Chem. 21, 3081–3090. doi:10.1039/c9gc00327d
Feng, C., Tang, L., Deng, Y., Wang, J., Luo, J., Liu, Y., et al. (2020). Synthesis of leaf-vein-like g-C3N4 with tunable band structures and charge transfer properties for selective photocatalytic H2O2 evolution. Adv. Funct. Mat. 30, 2001922. doi:10.1002/adfm.202001922
Feng, X. Y., Yu, Z., Shan, M., Long, R., Li, X., and Liao, K. (2022). Z-type ZnAl-LDO/Ag2S heterojunction activated peroxysulfate to degrade tetracycline hydrochloride under visible light efficiently. Chem. Eng. J. 443, 136422. doi:10.1016/j.cej.2022.136422
Gao, J., Qian, X., Wei, Q., Chen, Z., Liu, C., Wang, W., et al. (2022). Construction of core-shell cesium lead bromide-silica by precipitation coating method with applications in aqueous photocatalysis. J. Colloid Interface Sci. 623, 974–984. doi:10.1016/j.jcis.2022.05.116
Guo, J., Gan, W., Chen, R., Zhang, M., and Sun, Z. (2023). Au nanoparticle sensitized blue TiO2 nanorod arrays for efficient Gatifloxacin photodegradation. RSC Adv. 13, 28299–28306. doi:10.1039/d3ra05552c
Guo, J., Li, P., and Yang, Z. (2019). A novel Z-scheme g-C3N4/LaCoO3 heterojunction with enhanced photocatalytic activity in degradation of tetracycline hydrochloride. Catal. Commun. 122, 63–67. doi:10.1016/j.catcom.2019.01.022
Hirai, D., Sawai, O., Nunoura, T., and Hiroi, Z. (2018). Facile synthetic route to transition metal oxyfluorides via reactions between metal oxides and PTFE. J. Fluor. Chem. 209, 43–48. doi:10.1016/j.jfluchem.2018.02.008
Huang, S., Yu, J., Li, C., Zhu, Q., Zhang, Y., Lichtfouse, E., et al. (2022). The effect review of various biological, physical and chemical methods on the removal of antibiotics. Water 14, 3138. doi:10.3390/w14193138
Idrees, F., Cao, C., Butt, F. K., Tahir, M., Shakir, I., Rizwan, M., et al. (2014). Synthesis of novel hollow microflowers (NHMF) of Nb3O7F, their optical and hydrogen storage properties. Int. J. Hydrog. Energy. 39, 13174–13179. doi:10.1016/j.ijhydene.2014.06.142
Jiang, H., Li, Y., Wang, D., Hong, X., and Liang, B. (2020). Recent advances in heteroatom doped graphitic carbon nitride (g-C3N4) and g-C3N4/metal oxide composite photocatalysts. Curr. Org. Chem. 24, 673–693. doi:10.2174/1385272824666200309151648
Kamat, P. V. (2002). Photoinduced transformations in semiconductormetal nanocomposite assemblies. Pure Appl. Chem. 74, 1693–1706. doi:10.1351/pac200274091693
Khalil, M., Anggraeni, E. S., Ivandini, T. A., and Budianto, E. (2019). Exposing TiO2 (001) crystal facet in nano Au-TiO2 heterostructures for enhanced photodegradation of methylene blue. Appl. Surf. Sci. 487, 1376–1384. doi:10.1016/j.apsusc.2019.05.232
Lang, X., Chen, X., and Zhao, J. (2014). Heterogeneous visible light photocatalysis for selective organic transformations. Chem. Soc. Rev. 43, 473–486. doi:10.1039/c3cs60188a
Lang, X., and Zhao, J. (2018). Integrating TEMPO and its analogues with visible-light photocatalysis. Chem. Asian J. 13, 599–613. doi:10.1002/asia.201701765
Lanjwani, M. F., Tuzen, M., Khuhawar, M. Y., and Saleh, T. A. (2024). Trends in photocatalytic degradation of organic dye pollutants using nanoparticles: a review. Inorg. Chem. Commun. 159, 111613. doi:10.1016/j.inoche.2023.111613
Le, F., Brandl, D. W., Urzhumonv, Y. A., Wang, H., Kundu, J., Halas, N. J., et al. (2008). Metallic nanoparticle arrays: a common substrate for both surface-enhanced Raman scattering and surface-enhanced infrared absorption. ACS Nano 2, 707–718. doi:10.1021/nn800047e
Leong, K. H., Aziz, A. A., Sim, L. C., Saravanan, P., Jang, M., and Bahnemann, D. (2018). Mechanistic insights into plasmonic photocatalysts in utilizing visible light. Beilstein J. Nanotechnol. 9, 628–648. doi:10.3762/bjnano.9.59
Leung, D. Y. C., Fu, X., Ye, D., and Huang, H. (2012). Effect of oxygen mobility in the lattice of Au/TiO2 on formaldehyde oxidation. Kinet. Catal. 53, 239–246. doi:10.1134/s0023158412020048
Li, C., Wang, X., Wu, J., Gao, J., Zhao, R., Xia, S., et al. (2023). Harnessing ultrasound in photocatalysis: synthesis and piezo-enhanced effect: a review. Ultrason. Sonochem. 99, 106584. doi:10.1016/j.ultsonch.2023.106584
Li, Z., Huang, F., Peng, B., Yan, A., Dong, H., Feng, H., et al. (2018). A kind of economical, environment-friendly and controllable synthesis of Nb3O7F nanowalls and their photocatalytic properties. Mat. Lett. 214, 165–169. doi:10.1016/j.matlet.2017.11.124
Li, Z., Huang, F., Xu, Y., Yan, A., Dong, H., Luo, S., et al. (2021). 2D/2D Nb3O7F/g-C3N4 heterojunction photocatalysts with enhanced hydrogen evolution activity. ACS Appl. Energy Mat. 4, 839–845. doi:10.1021/acsaem.0c02727
Liang, X., Wang, P., Gao, Y., Huang, H., Tong, F., Zhang, Q., et al. (2020). Design and synthesis of porous M-ZnO/CeO2 microspheres as efficient plasmonic photocatalysts for nonpolar gaseous molecules oxidation: insight into the role of oxygen vacancy defects and M=Ag, Au nanoparticles. Appl. Catal. B Environ. 260, 118151. doi:10.1016/j.apcatb.2019.118151
Lin, M., Mochizuki, C., An, B., Honma, T., Haruta, M., Ishida, T., et al. (2020). Ligand effect of gold colloid in the preparation of Au/Nb2O5 for CO oxidation. J. Catal. 389, 9–18. doi:10.1016/j.jcat.2020.05.014
Liu, C. X., Qian, X. X., Wei, Q. Y., Chen, Z., Chen, J., Wang, W., et al. (2022). Construction of hydrostable cesium lead bromide-titania for visible-light degradation of tetracycline hydrochloride in water. J. Clean. Prod. 365, 132830. doi:10.1016/j.jclepro.2022.132830
Lu, T., Gao, Y., Yang, Y., Ming, H., Huang, Z., Liu, G., et al. (2021). Efficient degradation of tetracycline hydrochloride by photocatalytic ozonation over Bi2WO6. Chemosphere 283, 131256. doi:10.1016/j.chemosphere.2021.131256
Ly, N. H., Vasseghian, Y., and Joo, S. W. (2023). Plasmonic photocatalysts for enhanced solar hydrogen production: a comprehensive review. Fuel 344, 128087. doi:10.1016/j.fuel.2023.128087
Mishra, K., Devi, N., Siwal, S. S., Gupta, V. K., and Thakur, V. K. (2023). Hybrid semiconductor photocatalyst nanomaterials for energy and environmental applications: fundamentals, designing, and prospects. Adv. Sustain. Syst. 7. doi:10.1002/adsu.202300095
Mubeen, S., Hernandez-Sosa, G., Moses, D. J., Lee, J., and Moskovits, M. (2011). Plasmonic photosensitization of a wide band gap semiconductor: converting plasmons to charge carriers. Nano Lett. 11, 5548–5552. doi:10.1021/nl203457v
Prakash, J., Sun, S., Swart, H. C., and Gupta, R. K. (2018). Noble metals-TiO2 nanocomposites: from fundamental mechanisms to photocatalysis, surface enhanced Raman scattering and antibacterial applications. Appl. Mat. Today. 11, 82–135. doi:10.1016/j.apmt.2018.02.002
Qin, K., Zhao, Q., Yu, H., Xia, X., Li, J., He, S., et al. (2021). A review of bismuth-based photocatalysts for antibiotic degradation: insight into the photocatalytic degradation performance, pathways and relevant mechanisms. Environ. Res. 199, 111360. doi:10.1016/j.envres.2021.111360
Sánchez-Polo, M., Velo-Gala, I., López-Peñalver, J. J., and Rivera-Utrilla, J. (2015). Molecular imprinted polymer to remove tetracycline from aqueous solutions. Microporous Mesoporous Mater 203, 32–40. doi:10.1016/j.micromeso.2014.10.022
Shurbaji, S., Huong, P. T., and Altahtamouni, T. M. (2021). Review on the visible light photocatalysis for the decomposition of ciprofloxacin, norfloxacin, tetracyclines, and sulfonamides antibiotics in wastewater. Catalysts 11, 437. doi:10.3390/catal11040437
Su, Y., Wang, X., Dong, S., Fu, S., Zhou, D., and Rittmann, B. E. (2020). Towards a simultaneous combination of ozonation and biodegradation for enhancing tetracycline decomposition and toxicity elimination. Bioresour. Technol. 304, 123009. doi:10.1016/j.biortech.2020.123009
Tian, Y., and Tatsuma, T. (2005). Mechanisms and applications of plasmon-induced charge separation at TiO2 films loaded with gold nanoparticles. J. Am. Chem. Soc. 127, 7632–7637. doi:10.1021/ja042192u
Umair, M., Kanwal, T., Loddo, V., Palmisano, L., and Bellardita, M. (2023). Review on recent advances in the removal of organic drugs by advanced oxidation processes. Catalysts 13, 1440. doi:10.3390/catal13111440
Vinayagam, V., Palani, K. N., Ganesh, S., Rajesh, S., Akula, V. V., Avoodaiappan, R., et al. (2024). Recent developments on advanced oxidation processes for degradation of pollutants from wastewater with focus on antibiotics and organic dyes. Environ. Res. 240, 117500. doi:10.1016/j.envres.2023.117500
Wetchakun, K., Wetchakun, N., and Sakulsermsuk, S. (2019). An overview of solar/visible light-driven heterogeneous photocatalysis for water purification: TiO2- and ZnO-based photocatalysts used in suspension photoreactors. J. Ind. Eng. Chem. 71, 19–49. doi:10.1016/j.jiec.2018.11.025
Xiao, K., Huang, D., Kang, C., and Sun, S. (2020). Removal of tetracyclines from aqueous solutions by electrocoagulation/pecan nutshell coupling processes: synergistic effect and mechanism. Water Sci. Technol. 82, 683–694. doi:10.2166/wst.2020.367
Yang, L., Liu, Y., Tan, P., Lu, Y., Ding, Q., and Pan, J. (2023). Anchoring TiO2@CsPbBr3 on g-C3N4 nanosheet for enhanced photocatalytic degradation activity of tetracycline hydrochloride. Diam. Relat. Mat. 133, 109727. doi:10.1016/j.diamond.2023.109727
Yang, X., Chen, Z., Zhao, W., Liu, C., Qian, X., Chang, W., et al. (2021b). Construction of porous-hydrangea BiOBr/BiOI n-n heterojunction with enhanced photodegradation of tetracycline hydrochloride under visible light. J. Alloys Compd. 864, 158784. doi:10.1016/j.jallcom.2021.158784
Yang, X., Chen, Z., Zhao, W., Liu, C., Qian, X., Zhang, M., et al. (2021a). Recent advances in photodegradation of antibiotic residues in water. Chem. Eng. J. 405, 126806. doi:10.1016/j.cej.2020.126806
Yuan, Q., Qu, S., Li, R., Huo, Z. Y., Gao, Y., and Luo, Y. (2023). Degradation of antibiotics by electrochemical advanced oxidation processes (EAOPs): performance, mechanisms, and perspectives. Sci. Total Environ. 856, 159092. doi:10.1016/j.scitotenv.2022.159092
Zhang, B., He, X., Yu, C., Liu, G., Ma, D., Cui, C., et al. (2022c). Degradation of tetracycline hydrochloride by ultrafine TiO2 nanoparticles modified g-C3N4 heterojunction photocatalyst: influencing factors, products and mechanism insight. Chin. Chem. Lett. 33, 1337–1342. doi:10.1016/j.cclet.2021.08.008
Zhang, J., Xiang, S., Wu, P., Wang, D., Lu, S., Wang, S., et al. (2022b). Recent advances in performance improvement of Metal-organic Frameworks to remove antibiotics: mechanism and evaluation. Sci. Total Environ. 811, 152351. doi:10.1016/j.scitotenv.2021.152351
Zhang, W., Shen, P., Qian, L., Wang, Y., Arandiyan, H., Mahmoud, H., et al. (2021). Nanoscale phase engineering in two-dimensional niobium pentoxide anodes toward excellent electrochemical lithium storage. ACS Appl. Energy Mat. 4, 4551–4560. doi:10.1021/acsaem.1c00186
Zhang, Y., Zhao, Y. G., Maqbool, F., and Hu, Y. (2022a). Removal of antibiotics pollutants in wastewater by UV-based advanced oxidation processes: influence of water matrix components, processes optimization and application: a review. J. Water Process. Eng. 45, 102496. doi:10.1016/j.jwpe.2021.102496
Zhao, L., Vora, L. K., Kelly, S. A., Li, L., Larrañeta, E., McCarthy, H. O., et al. (2023a). Hydrogel-forming microarray patch mediated transdermal delivery of tetracycline hydrochloride. J. Control. Release. 356, 196–204. doi:10.1016/j.jconrel.2023.02.031
Zhao, W., Chen, Z., Yang, X., Qian, X., Liu, C., Zhou, D., et al. (2020). Recent advances in photocatalytic hydrogen evolution with high-performance catalysts without precious metals. Renew. Sust. Energy Rev. 132, 110040. doi:10.1016/j.rser.2020.110040
Zhao, Y., Chang, L., Li, Y., He, W., Liu, K., Cui, M., et al. (2023b). High-gravity photocatalytic degradation of tetracycline hydrochloride under simulated sunlight. J. Water Process Eng. 53, 103753. doi:10.1016/j.jwpe.2023.103753
Keywords: niobium oxyfluoride, gold nanoparticles, tetracycline hydrochloride, visible light, photodegradation
Citation: Wang Z, Ren L, Chen Z, Chen Y, Tian X and Wei G (2024) Preparation of novel Au-Nb3O7F nanosheets for the photodegradation of tetracycline hydrochloride. Front. Chem. 12:1412457. doi: 10.3389/fchem.2024.1412457
Received: 05 April 2024; Accepted: 02 May 2024;
Published: 22 May 2024.
Edited by:
Ermelinda Falletta, University of Milan, ItalyReviewed by:
Suresh Kumar Dash, Siksha O Anusandhan University, IndiaIstván Székely, Babeș-Bolyai University, Romania
Hugo Salazar, Basque Center for Materials, Applications and Nanostructures, Spain
Copyright © 2024 Wang, Ren, Chen, Chen, Tian and Wei. This is an open-access article distributed under the terms of the Creative Commons Attribution License (CC BY). The use, distribution or reproduction in other forums is permitted, provided the original author(s) and the copyright owner(s) are credited and that the original publication in this journal is cited, in accordance with accepted academic practice. No use, distribution or reproduction is permitted which does not comply with these terms.
*Correspondence: Li Ren, cmVubGlAY2psdS5lZHUuY24=; Guoying Wei, Z3VveWluZ3dlaUBjamx1LmVkdS5jbg==