- 1Faculty of Forestry and Wood Sciences, Czech University of Life Sciences Prague, Prague, Czechia
- 2Department of Analytical Chemistry, Institute of Chemistry, Faculty of Science, P. J. Šafárik University, Košice, Slovakia
- 3Department of Analytical Chemistry, Faculty of Chemistry, Gdańsk University of Technology, Gdańsk, Poland
- 4Department of Process Engineering and Chemical Technology, Faculty of Chemistry, Gdańsk University of Technology, Gdańsk, Poland
Polyphenolic compounds play an essential role in plant growth, reproduction, and defense mechanisms against pathogens and environmental stresses. Extracting these compounds is the initial step in assessing phytochemical changes, where the choice of extraction method significantly influences the extracted analytes. However, due to environmental factors, analyzing numerous samples is necessary for statistically significant results, often leading to the use of harmful organic solvents for extraction. Therefore, in this study, a novel DES-based shaking-assisted extraction procedure for the separation of polyphenolic compounds from plant samples followed by LC-ESI-QTOF-MS analysis was developed. The DES was prepared from choline chloride (ChCl) as the hydrogen bond acceptor (HBA) and fructose (Fru) as the hydrogen bond donor (HBD) at various molar ratios with the addition of 30% water to reduce viscosity. Several experimental variables affecting extraction efficiency were studied and optimized using one-variable-at-a-time (OVAT) and confirmed by response surface design (RS). Nearly the same experimental conditions were obtained using both optimization methods and were set as follows: 30 mg of sample, 300 mg of ChCl:Fru 1:2 DES containing 30% w/w of water, 500 rpm shaking speed, 30 min extraction time, 10°C extraction temperature. The results were compared with those obtained using conventional solvents, such as ethanol, methanol and water, whereby the DES-based shaking-assisted extraction method showed a higher efficiency than the classical procedures. The greenness of the developed method was compared with the greenness of existing procedures for the extraction of polyphenolic substances from solid plant samples using the complementary green analytical procedure index (ComplexGAPI) approach, while the results for the developed method were better or comparable to the existing ones. In addition, the practicability of the developed procedure was evaluated by application of the blue applicability grade index (BAGI) metric. The developed procedure was applied to the determination of spruce root samples with satisfactory results and has the potential for use in the analysis of similar plant samples.
1 Introduction
Secondary plant metabolites, mainly polyphenolic compounds, play an important role in the processes of growth, reproduction and the defense mechanisms of plants against pathogens and environmental stresses (Hartmann and Trumbore, 2016; Pang et al., 2021). They possess adaptive traits that have undergone natural selection through evolution or climate change. The extraction of secondary plant metabolites is the first step in evaluating phytochemical changes in plants. However, it should be noted that the extracted substances may depend on the extraction method chosen (Bentley et al., 2020). Due to the various environmental factors affecting the synthesis of certain compounds in plants, it is necessary to analyze a large number of samples to obtain statistically significant results, leading to the need to use a large amount of harmful organic solvents for extraction.
In line with the requirements of green chemistry, the current trend is to replace dangerous solvents with less harmful and environmentally friendly alternatives, as well as to reduce the total amount of solvents used. One possibility is the use of deep eutectic solvents (DES), which can be considered as promising green solvents for the extraction of bioactive compounds from complex matrix samples, such as fruits, vegetables and plants (Duan et al., 2016; Shishov et al., 2017; Vieira et al., 2018; Cai et al., 2019; El Kantar et al., 2019; Skarpalezos and Detsi, 2019; Bajkacz et al., 2020; Chen et al., 2020; Ivanović et al., 2020; Koel et al., 2020; Hikmawanti et al., 2021; Kalyniukova et al., 2021; Chen et al., 2022; Mansour et al., 2024). However, the high viscosity of most DESs is a fundamental limitation that can affect the extraction efficiency of the target compounds and subsequently complicate the quantification of analytes by instrumental methods. The addition of water can reduce the viscosity of DESs and thus increase the extraction efficiency (El Achkar et al., 2019; Rozas et al., 2021).
One of the most commonly used types of DES involves systems based on choline chloride as a hydrogen bond acceptor (HBA) in combination with various hydrogen bond donors (HBD), such as organic acids, urea or sugars. Recently, articles have been published devoted to DESs prepared from choline chloride and sugars to the study of the influence of the nature of the HBD (sucrose, glucose, fructose, and xylose) on the distribution behavior of selected compounds in a two-phase liquid–liquid system (Liu et al., 2022); to the use in an ultrasound-assisted dispersive liquid–liquid microextraction procedure for the extraction of aflatoxin M1 in milk samples prior to its determination by UV–VIS spectrophotometry (Gürsoy et al., 2022); and as potential absorbents for NH3 capture (Li et al., 2020). DESs based on choline chloride and d-fructose in different ratios were prepared, and their physical properties, such as density, viscosity, surface tension, refractive index and pH, were investigated at different temperatures (25°C–85°C). The measured physical properties indicated that DESs have the potential to be used for various applications, including extraction processes (Hayyan et al., 2012).
In the extraction of bioactive compounds from solid plant samples, the mass transfer is usually supported by some auxiliary energy, for example, by ultrasound or shaking. Here are a few examples of using choline chloride-fructose DES for plant sample analysis: Razboršek et al. investigated different natural deep eutectic solvents (NADESs) based on choline chloride for the ultrasound-assisted extraction (UAE) of phenolic compounds from dried chokeberries and found that the highest values of total phenolics and total flavonoids were obtained using the choline chloride-fructose system. Additionally, the results were compared with those obtained using conventional methanol extraction, and their high extraction efficiency was demonstrated (Razboršek et al., 2020). Takla et al. presented a comparison of the potential of NADES and nonionic surfactants with conventional solvents (methanol, ethanol and water) for the ultrasound extraction of alkaloids from plant material. High-performance thin-layer chromatography was used for quantification. The highest extraction yields were obtained using a DES consisting of choline chloride:fructose (5:2) with 35% water. The results showed that a NADES and surfactants were much more efficient in extracting alkaloids than previous methods (Takla et al., 2018). NADESs prepared from choline chloride (ChCl) and sucrose, fructose, glucose and xylose were used for ultrasound-assisted extraction of antioxidants from the halophyte Polygonum maritimum L. (sea knotgrass) and compared with conventional solvents, such as ethanol and acetone. The obtained extracts were evaluated for in vitro antioxidant properties and profiled using liquid chromatography analysis. The results indicate that a NADES containing ChCl and sucrose/fructose can replace conventional solvents in the extraction of antioxidants from sea knotgrass (Rukavina et al., 2021).
Zhang et al. developed a selective shaking-assisted extraction of astaxanthin ester and free astaxanthin from Haematococcus pluvialis by aqueous biphasic systems (ABS) composed of ionic liquids and deep eutectic solutions. ABS composed of tributyloctylphosphine chloride and choline chloride:D-fructose performed the best. The results was compared with organic solvent extraction and prior methods (Zhang et al., 2024). A DES-based pretreatment followed by microwave-assisted hydrodistillation (MAHD) for the extraction of essential oil from dry fruits of white and black peppers was developed. The DES comprised of choline chloride and fructose at a molar ratio of 3:1 was used. The obtained essential oils were analyzed using gas chromatography-mass spectrometry (GC-MS), identifying more compounds than hydrodistillation (Yu et al., 2017). García et al. developed an electrokinetic chromatography method enabling separation of the four stereoisomers of the acetamide herbicide dimethenamid. They tested different anionic cyclodextrins (CDs) based on the use of single and dual CD systems with the addition of ionic liquids and DESs and found that choline chloride-D-fructose, when added to the CDs dual system, enabled separating the four stereoisomers of dimethenamid (Angeles Garcia et al., 2022).
The aim of this work was to develop a novel shaking-assisted extraction method for phenolic compounds from plant samples using a green extraction solvent, a DES based on choline chloride as the HBA and fructose as the HBD. Despite the popularity of ChCl-based DES for analytical purposes, the mixtures of ChCl with Fru are less investigated, especially for plant analysis. That is why we focused on this combination. The parameters influencing the extraction efficiency were investigated by the one variable-at-a-time method (OVAT) and a response surface design (RS) based on face-centered central composite design. The method was subsequently applied to the analysis of Norway spruce root samples in conjunction with LC-ESI-QTOF-MS quantification of polyphenolic compounds.
2 Methods
2.1 Chemicals
The DES was composed of choline chloride (ChCl) (purity ≥99%) obtained from Gentham Life Science, UK, and D (−)fructose (Fru) (purity ≥99%) purchased from Acros Organics, United States. The solvents, such as methanol (purity ≥99.9%), acetonitrile (purity ≥99.9%) and water (LC-MS grade), were obtained from Honeywell, Germany. The chemical standards were obtained as follows: piceatannol (purity ≥98%), epicatechin (purity ≥98%), procyanidin B1 (purity ≥98%), (−)-epigallocatechin (purity ≥98%) and taxifolin (purity ≥98%) from Chem Faces, China; isorhapontin (purity ≥98%) from Toronto Research Chemicals, Canada; 4-coumaric acid (purity ≥98%) and (+)-catechin (purity ≥98%) from Extrasynthese, France. Ultra-pure water was obtained using a Millipore Milli-Q Plus water treatment system (Millipore Bedford Corp., Bedford, MA).
2.2 LC-ESI-QTOF-MS/MS analysis
LC-ESI-QTOF-MS/MS analysis was carried out using an Agilent 1,290 Infinity II system coupled to an Agilent 6546 LC/MS QTOF mass spectrometer (Agilent, United States). The LC separation was conducted using a Zorbax Eclipse Plus C18 column (2.1 × 50 mm, 1.8 µm), (Agilent, United States). The optimal conditions were as follows: mobile phase A consisted of water containing 0.05% formic acid, and mobile phase B consisted of acetonitrile; flow rate, 1.1 mL min–1; injection volume, 1 μL; and column temperature, 35°C. For separation of polyphenolic compounds, the gradient elution was set as follows: 0–0.1 min, 95% A; 0.one to eight min, 72% A; 8–9.1 min, 25% A; 9.1–11 min, 95% A.
The determination of polyphenolic compounds (Table 1) was carried out by LC-MS/MS in negative ionization mode. The QTOF parameters were optimized using the standards and were set as follows: scan range 100–1,000 m/z; drying gas temperature, 350°C; sheath gas flow rate, 12.0 L/min; sheath gas temperature, 400°C; capillary voltage, 5.0 kV; nozzle voltage 0.9 kV; fragmentor, 140 V; collision energy at 10, 20 and 40 eV. MS/MS data were acquired at a scan range of 50–800 m/z; retention time window, 0.5 min; isolation window, 1.3 amu and an acquisition rate of two spectra s–1. During the analysis, two reference masses (112.9855 and 966.0007 m/z) were continuously measured to mass correction. The data collection was carried out using the Agilent Mass Hunter Acquisition software, and data analysis was performed using Mass Hunter Qualitative Analysis 10.0 and Q-TOF Quantitative analysis (Agilent, United States).
2.3 Sample collection
Norway spruce root samples (Picea abies L.) were collected from a depth of 15 cm approximately 1 m from the stem base in the Ore Mts (GPS coordinates 50.65 lat and 13.63 long). The roots were rinsed from the soil, inserted into conical tubes and placed into liquid nitrogen immediately. Samples were freeze-dried, homogenized, passed through 50 µm analytical sieve, and stored at −80°C before LC-ESI-QTOF-MS analysis.
2.4 Preparation of the deep eutectic solvents
Choline chloride (HBA) was mixed with fructose (HBD) in different molar ratios (1:1, 1:2, 2:1 and 5:2) with the addition of 30% water w/w to reduce the viscosity, and the mixtures were stirred at 350 rpm at 60°C on a heating magnetic stirrer (Witeg MSH-20D, Germany) until a transparent liquid was formed.
2.5 General extraction procedure
A 30 mg sample was precisely weighed in 2-mL test tube and 300 mg of DES was added. Then, 200 µL of water was added, and the sample was vortexed and placed into the thermoshaker (Bioer, China) for 30 min at 500 rpm. After extraction, the sample was centrifuged at 13,000 rpm for 5 min; 200 µL of collected supernatant was transferred to a new dry test tube, diluted with 800 µL of methanol, filtered through a 0.22 µm PTFE filter and injected into the LC system.
2.6 Response surface design
In order to validate findings from OVAT optimization, the response surface design was performed. The selected face-centered central composite design with five replicates on the center point was used to find optimal values of shaking speed, extraction time and extraction temperature. The outcome of the RS was a maximizing of the area under the peak of procyanidin B1, catechin, epicatechin and taxifolin. The RS was performed using Minitab v. 17.1.0 (LEAD Technologies, Inc.), and surface plots were generated using in-house Python code. The residues distribution of the obtained models were checked to determine their close to normal distribution characteristics. The residues distribution for catechin and procyanidin B1 were found to be asymmetric; thus Box-Cox transformation of the model input data for those compounds was proposed (the ‘find optimal λ′ option was implemented) giving satisfactory results. The RS is presented in detail in the Supplementary Materials.
2.7 Green assessment tools
The complementary green analytical procedure index (ComplexGAPI) (Płotka-Wasylka and Wojnowski, 2021) was used as the green assessment tool. In addition to the green character evaluation, an assessment of the method practicality was performed. For this purpose, the blue applicability grade index (BAGI) (Manousi et al., 2023) was applied.
3 Results and discussion
Parameters affecting the extraction efficiency, such as the choline chloride and fructose molar ratio, shaking speed, extraction time, extraction temperature and addition of water, were investigated.
3.1 Effect of choline chloride and fructose molar ratio
In general, we can consider DESs as promising solvents in extraction and separation processes (Zainal-Abidin et al., 2017). However, the ability of a DES to extract bioactive compounds from plant samples depends on various factors, such as the type of HBA and HBD used as well as their molar ratio. Therefore, ChCl:Fru DESs with various molar ratios were tested for the extraction of polyphenolic compounds from spruce roots. Supplementary Figure S1 shows that the highest extraction yield was obtained for the DES at a 1:2 M ratio; therefore, this ratio was selected for further studies. The results indicate that an increase in the fructose content within the DES structure correlates with enhanced extraction efficiency. This can be attributed to the presence of active groups in the fructose structure, notably five -OH groups and one = O group, capable of forming robust hydrogen bonds with bioactive substances. In contrast, the ChCl structure possesses only one hydroxyl group, leading to a reduction in extraction efficiency. Another influential factor affecting extraction efficiency is dynamic viscosity, impacting the mass transfer process. With an elevation in the concentration of ChCl, the viscosity of the DES increases, consequently leading to a decrease in extraction efficiency. The extraction efficiency of DES was compared with conventional solvents, such as methanol, ethanol and water. Figure 1 shows that the extraction efficiency of DES is higher compared to methanol at 4%–22%, at ethanol 15%–25%, at 42%–58%, depending on bioactive compounds.
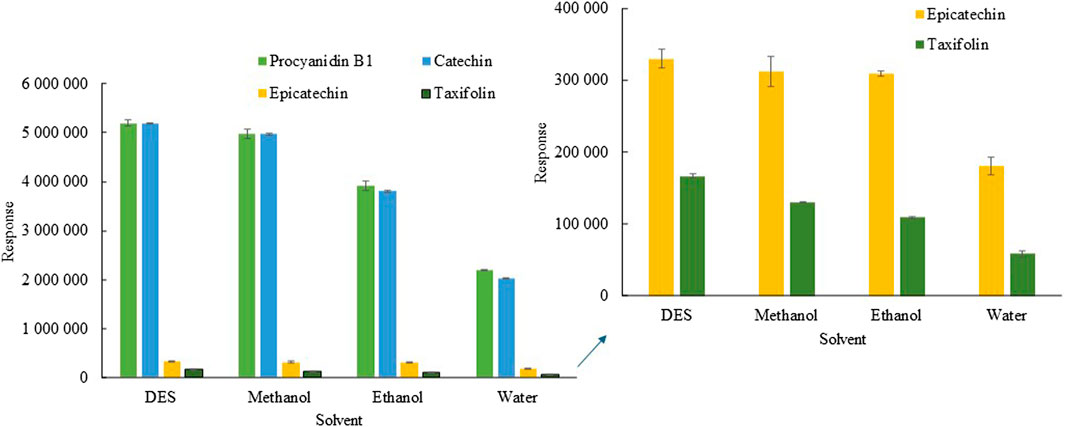
Figure 1. Comparison of DES extraction ability with conventional solvent. Extraction conditions: sample amount, 30 mg; DES amount, 300 mg; solvent amount, 500 μL, water volume, 200 µL (in the case of DES); extraction temperature, 10°C; shaking speed, 500 rpm, extraction time, 5 min.
3.2 Effect of shaking speed
Another parameter that influences the extraction yield is shaking speed, which ensures the rate of mass transfer from solid phase into the DES phase. The shaking speed was examined in the range from 100 to 1,500 rpm. From Supplementary Figure S2 is evident that the shaking speed has no significant effect on the extraction efficiency; however, a slight increase was observed at 500 rpm which then remains stable up to 1,500 rpm. Therefore, 500 rpm was selected for further optimization steps.
3.3 Effect of extraction time
The effect of extraction time was examined in the range of 5–60 min. Supplementary Figure S3 reveals that the extraction yield slowly but gradually and clearly increases with time, reaching a maximum value at 30 min, then remains stable. This can be attributed to the heightened solubility of bioactive compounds in the DES during agitation, thereby facilitating the efficient mass transfer of bioactive compounds from the spruce roots to the DES phase. To ensure high extraction yield in the shortest possible analysis time, 30 min was chosen as the optimum value.
3.4 Effect of extraction temperature
Temperature can affect the extraction efficiency; therefore, its influence was studied in a wide range from 5°C to 90°C. In principle, elevating the extraction temperature is expected to decrease the dynamic viscosity of DES, thereby enhancing the efficiency of the mass exchange process. However, excessively high temperatures are undesirable, because the interactions between the DES and bioactive compounds involve an exothermic reaction, in accordance with Van ‘t Hoff’s law. This law asserts that in an exothermic reaction heat is liberated, resulting in a negative net enthalpy change. This, in turn, directly affects the partition coefficient value between the DES and bioactive compounds (Tellinghuisen, 2006). Furthermore, heightened temperatures can lead to the degradation of D-fructose. This degradation may manifest as caramelization followed by pyrolysis of the sugars (Woo et al., 2011).
As can be seen from Supplementary Figure S4, the efficiency of catechin extraction clearly increases with increasing temperature up to 30°C and subsequently decreases. In the case of procyanidin B1, the extraction efficiency is highest and stable up to 20°C; with a further increase in temperature, a lowering of the inter-sample repeatability is observed (30°C–60°C) and at temperatures above 60°C, the extraction efficiency decreases. The extraction efficiency of epicatechin is highest at lower temperatures up to 20°C, and from 30°C it gradually decreases. The extraction efficiency of taxifolin is less affected by temperature, but even in this case we can observe a gentle and slow increase to a maximum of 30°C, followed by a slow but clear decrease. Therefore, choosing the optimal extraction temperature is difficult, and it was necessary to choose a temperature that would provide the best results for the maximum number of analytes. Therefore 10°C was selected for further analysis.
3.5 Effect of water volume
In general, DESs are viscous, which complicates their use for the extraction of substances from solid samples such as plants. The addition of a small amount of water can affect the viscosity, but also the density and polarity of the DES, which can lead to improved extraction properties (El Achkar et al., 2019). On the other hand, an excessive amount of water can weaken the interactions between the DES and the target compounds, as well as the interactions between the DES components, which can lead to disruption of the DES supramolecular structure (El Achkar et al., 2019; Vilková et al., 2020). Therefore, the effect of adding water up to 600 µL was investigated and based on the obtained results (Supplementary Figure S5), 200 µL was selected for further experiments.
3.6 Results of experimental design
In order to confirm the optimal conditions, experimental design was applied. Parameters such as extraction time, extraction temperature and shaking speed were selected for optimization, since the results obtained with OVAT did not include interactions between each optimized factor; thus, it needed to be investigated. In Figure 2 the surface plots of catechin RS optimization are depicted (for others, see Supplementary Materials). Based on the calculations, optimal conditions for catechin determination are a shaking time of 32.5 min, a temperature of 10°C and a shaking speed of 300 rpm. These results are very close to the OVAT optimization. However, for all other optimized compounds strong saddle-shape surface plots were obtained. This can justify findings from OVAT optimization, where the impact of shaking speed and time was insignificant. The insignificance of some factors used for RS also results in a low lack of fit (LoF), where only the RS model for catechin (LoF 0.1 > 0.05) and taxifolin (LoF 0.09 > 0.05) were valid. Therefore, the authors decided to rely on the OVAT results and keep shaking for 30 min at 500 rpm in 10°C.
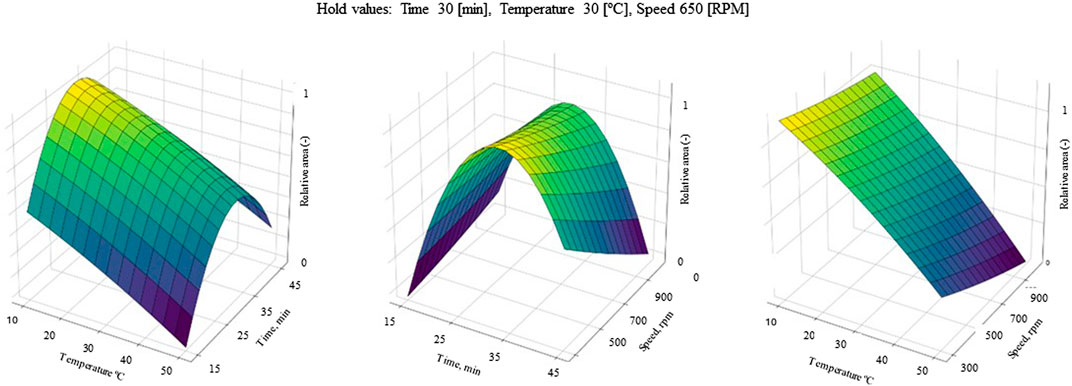
Figure 2. RS surface plots for catechin peak area optimization. Factors taken for optimization: extraction time (15–45 min), extraction temperature (10°C–50°C) and shaking speed (300–1,000 rpm).
3.7 Application to real samples
To show the applicability of the developed method for the extraction of bioactive compounds, a series of experiments was performed by analyzing Norway root samples spiked with standard solutions at a concentration level of 5 μg g-1. The precision and trueness were evaluated by inter-day and intra-day (five consecutive days) measurements. The results are given in Table 2. The recoveries were between 83% and 117%, with RSD less than 9.9% for inter-day and between 93% and 111% with RSD less than 14% for intra-day experiments, respectively.
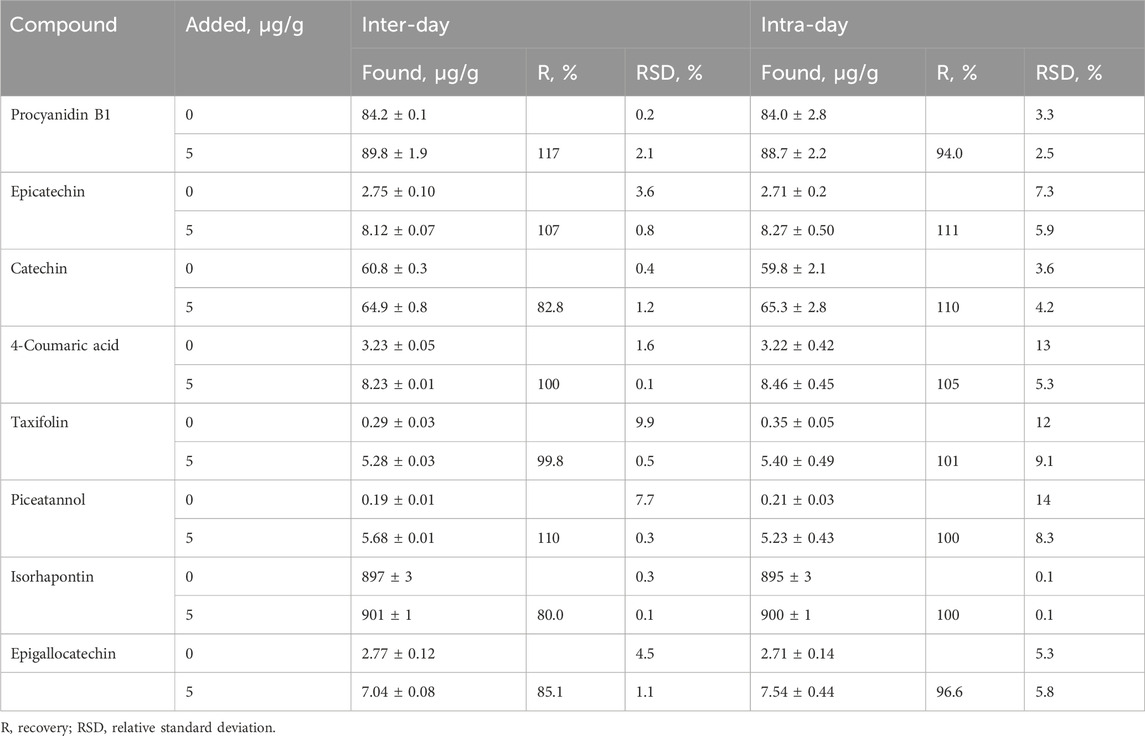
Table 2. Inter- and intra-day precision and accuracy of phenolic compounds determination in Norway spruce roots (n = 5).
3.8 Assessment of greenness
The ComplexGAPI tool was applied for the evaluation of the green character of the developed procedure. This metric not only allows the evaluation of the analytical protocol in terms of its environmental friendliness but also those processes which precede the analytical procedure itself (Płotka-Wasylka and Wojnowski, 2021; Locatelli et al., 2023). And so, the greenness aspect of the following parameters was evaluated: yield, conditions, reagents and solvents, instrumentation and workup, and purification which may occur before analytical protocol. Considering the generated waste, E-factor is calculated and presented in the middle of the lower part of the pictogram. As these elements can be applied to assess the synthesis of NADES, ComplexGAPI seems be perfect for use in the evaluation of the developed procedure.
Looking at the generated ComplexGAPI (Figure 3A) pictogram it can be concluded that the DES-based shaking-assisted extraction procedure coupled to LC-ESI-QTOF-MS for determination of bioactive compounds from Norway spruce roots can be considered green. This is mainly because the processes related to the synthesis of the DES are based on non-hazardous reagents. In fact, DES synthesis is a very simple process. The synthesis occurs in a 100% yield and no waste is generated during this process (E-factor = 0). The procedure requires small amounts of reagents for the analytical separation; however, what needs to be noted is that moderate toxic solvents (methanol and acetonitrile) are used here. In addition, a few milliliters of waste per sample are generated. The critical point of the procedure is the necessity to transport and store the sample. In order to show the potential of the developed protocol, the greenness of the developed method was compared with that of existing procedures for the extraction of polyphenolic substances from solid plant samples using the ComplexGAPI approach, and the results for the developed method were better or comparable to existing ones (Table 3).
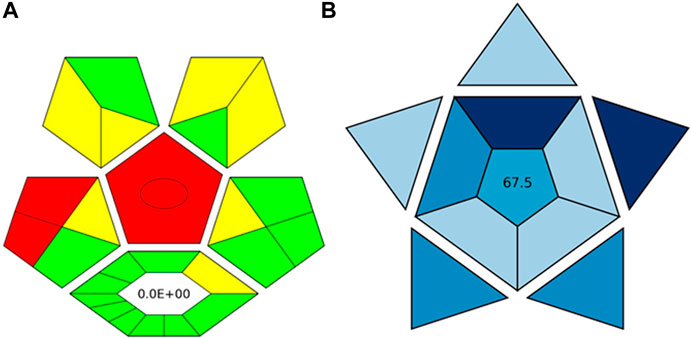
Figure 3. The pictograms of ComplexGAPI (A) and BAGI (B) generated for the DES-based shaking-assisted extraction coupled to LC-ESI-QTOF-MS for determination of bioactive compounds from Norway spruce roots.
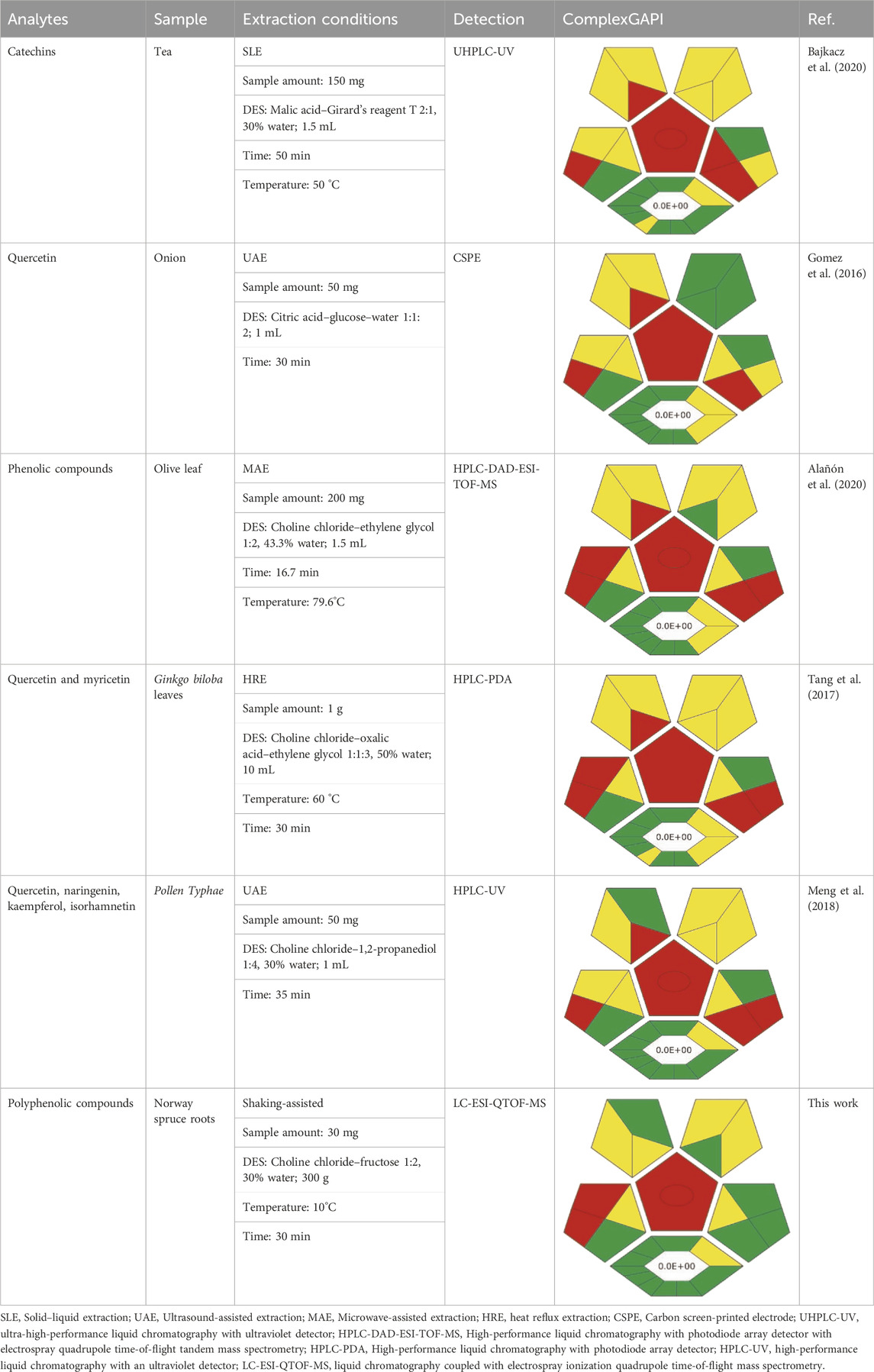
Table 3. Comparison of the greenness of the developed method with other reported methods for the extraction of phenolic compounds by means of ComplexGAPI analysis.
In addition to the green character evaluation, an assessment of method’s applicability was performed. For this purposes, blue applicability grade index (BAGI) was applied. This metric can be considered complementary to the ComplexGAPI (and other metrics), and it is mainly focused on the practical aspects of White Analytical Chemistry (WAC) (Manousi et al., 2023). Two different types of results can be obtained using the BAGI metric tool and they are correlated to the obtained pictogram and the obtained score as is visible in Figure 3B. In order to be considered practical, it is recommended that the method attains at least 60 points.
Looking at the BAGI pictogram, a great deal of information can be found at first glance. First of all, the information of the analysis was both quantitative and confirmatory due to the employment of the MS detector. The determination enabled the quantification of eight compounds belonging to two different classes (polyphenols and acids). Since the DES needs to be synthesized in the lab in a relatively simple and straightforward way using simple equipment, the procedure lost few points. Regarding the instrumentation, sophisticated equipment was employed. The simultaneous sample preparation can be easily performed using a multichannel pipet. As demonstrated by the results, a one-step preconcentration was needed. The autosampler of LC was used to inject the samples. As for the sample preparation, miniaturized extraction was employed, and the sample volume for the plant matrix was 30 mg. Thus, a BAGI score of 67.5 is attained for the method and the whole protocol shows good applicability potential.
4 Conclusion
In conclusion, this study introduced an environmentally friendly shaking-assisted extraction procedure utilizing a deep eutectic solvent based on choline chloride and fructose for the efficient extraction of polyphenolic compounds from Norway root samples coupled with LC-ESI-QTOF-MS detection. For the optimization of extraction efficiency, two optimization approaches were utilized: one-variable-at-a-time and confirmed by response surface design. Almost identical experimental conditions were achieved using both optimization techniques, as follows: 30 mg of the sample, 300 mg of ChCl:Fru 1:2 DES with 30% w/w water content, shaking speed set at 500 rpm, extraction time of 30 min, and an extraction temperature of 10°C. The DES was compared with conventional solvents and the results showed that the extraction efficiency of DES is higher compared to methanol, ethanol, and water. The environmental sustainability of the developed method was evaluated using the ComplexGAPI, while the practicality of the developed procedure was assessed through the blue applicability grade index. Furthermore, the method was successfully applied to the analysis of spruce root samples, yielding satisfactory results. We can assume that the suggested approach can (of course, after appropriate validation) also be used for samples of other plants with a similar structure or for the roots of other plants.
Data availability statement
The raw data supporting the conclusion of this article will be made available by the authors, without undue reservation.
Author contributions
AK: Conceptualization, Formal Analysis, Investigation, Supervision, Validation, Visualization, Writing–original draft, Writing–review and editing. AV: Writing–review and editing, Investigation. JP-W: Formal Analysis, Writing–original draft. TM: Formal Analysis, Writing–original draft. PM-C: Writing–original draft. IT: Resources, Writing–review and editing. VP: Resources, Writing–review and editing. FP: Resources, Writing–review and editing. AJ: Resources, Writing–review and editing. VA: Conceptualization, Supervision, Writing–original draft, Writing–review and editing.
Funding
The author(s) declare financial support was received for the research, authorship, and/or publication of this article.
AK, IT, VP, and FP thank “Advanced research supporting the forestry and wood-processing sector's adaptation to global change and the 4th industrial revolution”, No. CZ.02.1.01/0.0/0.0/16_019/0000803 financed by OP RDE. VA and AV would like to express their thank to the Scientific Grant Agency of the Ministry of Education, Science, Research and Sport of the Slovak Republic (VEGA 1/0220/21). AJ thanks “EXTEMIT-K,” No. CZ.02.1.01/0.0/0.0/15_003/0000433.
Conflict of interest
The authors declare that the research was conducted in the absence of any commercial or financial relationships that could be construed as a potential conflict of interest.
Publisher’s note
All claims expressed in this article are solely those of the authors and do not necessarily represent those of their affiliated organizations, or those of the publisher, the editors and the reviewers. Any product that may be evaluated in this article, or claim that may be made by its manufacturer, is not guaranteed or endorsed by the publisher.
Supplementary material
The Supplementary Material for this article can be found online at: https://www.frontiersin.org/articles/10.3389/fchem.2024.1385844/full#supplementary-material
Abbreviations
ABS, aqueous biphasic systems; CD, cyclodextrin; BAGI, blue applicability grade index; ChCl, choline chloride; ComplexGAPI, complementary green analytical procedure index; DES, deep eutectic solvents; Fru, fructose; HBA, hydrogen bond acceptor; HBD, hydrogen bond donor; NADES, natural deep eutectic solvent; OVAT, one variable-at-a-time method; RS, response surface design; UAE, ultrasound-assisted extraction; MAHD, microwave-assisted hydrodistillation; LoF, lack of fit; WAC, White Analytical Chemistry.
References
Alañón, M. E., Ivanović, M., Gómez-Caravaca, A. M., Arráez-Román, D., and Segura-Carretero, A. (2020). Choline chloride derivative-based deep eutectic liquids as novel green alternative solvents for extraction of phenolic compounds from olive leaf. Arabian J. Chem. 13, 1685–1701. doi:10.1016/j.arabjc.2018.01.003
Angeles Garcia, M., Jimenez-Jimenez, S., and Marina, M. L. (2022). Stereoselective separation of dimethenamid by cyclodextrin electrokinetic chromatography using deep eutectic solvents. J. Chromatogr. A 1673, 463114. doi:10.1016/j.chroma.2022.463114
Bajkacz, S., Adamek, J., and Sobska, A. (2020). Application of deep eutectic solvents and ionic liquids in the extraction of catechins from tea. Molecules 25, 3216. doi:10.3390/molecules25143216
Bentley, J., Olsen, E. K., Moore, J. P., and Farrant, J. M. (2020). The phenolic profile extracted from the desiccation-tolerant medicinal shrub Myrothamnus flabellifolia using Natural Deep Eutectic Solvents varies according to the solvation conditions. Phytochemistry 173, 112323. doi:10.1016/j.phytochem.2020.112323
Cai, C., Li, F., Liu, L., and Tan, Z. (2019). Deep eutectic solvents used as the green media for the efficient extraction of caffeine from Chinese dark tea. Sep. Purif. Technol. 227, 115723. doi:10.1016/j.seppur.2019.115723
Chen, Y., Dang, J., Yan, X., Lu, F., and Li, D. (2020). Optimization studies on the extraction of flavone di-C-glycosides from Premna fulva craib by deep eutectic solvents. J. Chem. 2020, 1–9. doi:10.1155/2020/7240535
Chen, Z., Wu, K., Zhu, W., Wang, Y., Su, C., and Yi, F. (2022). Chemical compositions and bioactivities of essential oil from perilla leaf (Perillae Folium) obtained by ultrasonic-assisted hydro-distillation with natural deep eutectic solvents. Food Chem. 375, 131834. doi:10.1016/j.foodchem.2021.131834
Duan, L., Dou, L. L., Guo, L., Li, P., and Liu, E. H. (2016). Comprehensive evaluation of deep eutectic solvents in extraction of bioactive natural products. ACS Sustain. Chem. Eng. 4, 2405–2411. doi:10.1021/acssuschemeng.6b00091
el Achkar, T., Fourmentin, S., and Greige-Gerges, H. (2019). Deep eutectic solvents: an overview on their interactions with water and biochemical compounds. J. Mol. Liq. 288, 111028. doi:10.1016/j.molliq.2019.111028
el Kantar, S., Rajha, H. N., Boussetta, N., Vorobiev, E., Maroun, R. G., and Louka, N. (2019). Green extraction of polyphenols from grapefruit peels using high voltage electrical discharges, deep eutectic solvents and aqueous glycerol. Food Chem. 295, 165–171. doi:10.1016/j.foodchem.2019.05.111
Gomez, F. J. V., Espino, M., de Los Angeles Fernandez, M., Raba, J., and Silva, M. F. (2016). Enhanced electrochemical detection of quercetin by natural deep eutectic solvents. Anal. Chim. Acta 936, 91–96. doi:10.1016/j.aca.2016.07.022
Gürsoy, N., SıRTBAŞı, B., Şimşek, S., Elik, A., and Altunay, N. (2022). Optimization and application of ultrasound-assisted sugar based deep eutectic solvent dispersive liquid–liquid microextraction for the determination and extraction of aflatoxin M1 in milk samples. Microchem. J. 172, 106974. doi:10.1016/j.microc.2021.106974
Hartmann, H., and Trumbore, S. (2016). Understanding the roles of nonstructural carbohydrates in forest trees - from what we can measure to what we want to know. New phytologist 211, 386–403. doi:10.1111/nph.13955
Hayyan, A., Mjalli, F. S., Alnashef, I. M., al-Wahaibi, T., al-Wahaibi, , and Hashim, M. A. (2012). Fruit sugar-based deep eutectic solvents and their physical properties. Thermochim. Acta 541, 70–75. doi:10.1016/j.tca.2012.04.030
Hikmawanti, N. P. E., Ramadon, D., Jantan, I., and Mun’Im, A. (2021). Natural deep eutectic solvents (Nades): phytochemical extraction performance enhancer for pharmaceutical and nutraceutical product development. Plants 10, 2091. doi:10.3390/plants10102091
Ivanović, M., Razboršek, M. I., and Kolar, M. (2020). Innovative extraction techniques using deep eutectic solvents and analytical methods for the isolation and characterization of natural bioactive compounds from plant material. Plants 9, 1–29. doi:10.3390/plants9111428
Kalyniukova, A., Holuša, J., Musiolek, D., Sedlakova-Kadukova, J., Płotka-Wasylka, J., and Andruch, V. (2021). Application of deep eutectic solvents for separation and determination of bioactive compounds in medicinal plants. Industrial Crops Prod. 172, 114047. doi:10.1016/j.indcrop.2021.114047
Koel, M., Kuhtinskaja, M., and Vaher, M. (2020). Extraction of bioactive compounds from Catharanthus roseus and Vinca minor. Sep. Purif. Technol., 252. doi:10.1016/j.seppur.2020.117438
Li, Z. L., Zhong, F. Y., Huang, J. Y., Peng, H. L., and Huang, K. (2020). Sugar-based natural deep eutectic solvents as potential absorbents for NH3 capture at elevated temperatures and reduced pressures. J. Mol. Liq., 317. doi:10.1016/j.molliq.2020.113992
Liu, F., Chen, L., Yin, K., Fan, T., and Yan, Z. (2022). Sugars as hydrogen-bond donors tune the phase behavior in a novel liquid–liquid biphasic system formed by hydrophilic deep eutectic solvents and n-propanol. Fluid Phase Equilibria 556, 113393. doi:10.1016/j.fluid.2022.113393
Locatelli, M., Kabir, A., Perrucci, M., Ulusoy, S., Ulusoy, H. I., and Ali, I. (2023). Green profile tools: current status and future perspectives. Adv. Sample Prep. 6, 100068. doi:10.1016/j.sampre.2023.100068
Manousi, N., Wojnowski, W., Płotka-Wasylka, J., and Samanidou, V. (2023). Blue applicability grade index (BAGI) and software: a new tool for the evaluation of method practicality. Green Chem. 25, 7598–7604. doi:10.1039/d3gc02347h
Mansour, F. R., Bedair, A., Hamed, M., Magdy, G., Ali, I., and Locatelli, M. (2024). Applications of (natural) deep eutectic solvents in liquid phase microextraction: a review. Microchem. J. 198, 110178. doi:10.1016/j.microc.2024.110178
Meng, Z., Zhao, J., Duan, H., Guan, Y., and Zhao, L. (2018). Green and efficient extraction of four bioactive flavonoids from Pollen Typhae by ultrasound-assisted deep eutectic solvents extraction. J. Pharm. Biomed. Analysis 161, 246–253. doi:10.1016/j.jpba.2018.08.048
Pang, Z., Chen, J., Wang, T., Gao, C., Li, Z., Guo, L., et al. (2021). Linking plant secondary metabolites and plant microbiomes: a review. Front. Plant Sci. 12, 621276. doi:10.3389/fpls.2021.621276
Płotka-Wasylka, J., and Wojnowski, W. (2021). Complementary green analytical procedure index (ComplexGAPI) and software. Green Chem. 23, 8657–8665. doi:10.1039/d1gc02318g
Razboršek, M. I., Ivanović, M., Krajnc, P., and Kolar, M. (2020). Choline chloride based natural deep eutectic solvents as extraction media for extracting phenolic compounds from chokeberry (Aronia melanocarpa). Molecules 25, 1619. doi:10.3390/molecules25071619
Rozas, S., Benito, C., Alcalde, R., Atilhan, M., and Aparicio, S. (2021). Insights on the water effect on deep eutectic solvents properties and structuring: the archetypical case of choline chloride + ethylene glycol. J. Mol. Liq., 344. doi:10.1016/j.molliq.2021.117717
Rukavina, I., Rodrigues, M. J., Pereira, C. G., Mansinhos, I., Romano, A., Ślusarczyk, S., et al. (2021). Greener is better: first approach for the use of natural deep eutectic solvents (nades) to extract antioxidants from the medicinal halophyte polygonum maritimum l. Molecules 26, 6136. doi:10.3390/molecules26206136
Shishov, A., Bulatov, A., Locatelli, M., Carradori, S., and Andruch, V. (2017). Application of deep eutectic solvents in analytical chemistry. A review. Microchem. J. 135, 33–38. doi:10.1016/j.microc.2017.07.015
Skarpalezos, D., and Detsi, A. (2019). Deep eutectic solvents as extraction media for valuable flavonoids from natural sources, 9. Switzerland: Applied Sciences.
Takla, S. S., Shawky, E., Hammoda, H. M., and Darwish, F. A. (2018). Green techniques in comparison to conventional ones in the extraction of Amaryllidaceae alkaloids: best solvents selection and parameters optimization. J. Chromatogr. A 1567, 99–110. doi:10.1016/j.chroma.2018.07.009
Tang, W., Li, G., Chen, B., Zhu, T., and Row, K. H. (2017). Evaluating ternary deep eutectic solvents as novel media for extraction of flavonoids from Ginkgo biloba. Sep. Sci. Technol. Phila. 52, 91–99. doi:10.1080/01496395.2016.1247864
Tellinghuisen, J. (2006). Van't Hoff analysis of K° (T): how good.or bad? Biophys. Chem. 120, 114–120. doi:10.1016/j.bpc.2005.10.012
Vieira, V., Prieto, M. A., Barros, L., Coutinho, J. A. P., Ferreira, I. C. F. R., and Ferreira, O. (2018). Enhanced extraction of phenolic compounds using choline chloride based deep eutectic solvents from Juglans regia L. Industrial Crops Prod. 115, 261–271. doi:10.1016/j.indcrop.2018.02.029
Vilková, M., Płotka-Wasylka, J., and Andruch, V. (2020). The role of water in deep eutectic solvent-base extraction. J. Mol. Liq. 304, 112747. doi:10.1016/j.molliq.2020.112747
Woo, K. S., Hwang, I. G., Kim, H. Y., Jang, K. I., Lee, J., Kang, T. S., et al. (2011). Thermal degradation characteristics and antioxidant activity of fructose solution with heating temperature and time. J. Med. Food 14, 167–172. doi:10.1089/jmf.2010.1166
Yu, G. W., Cheng, Q., Nie, J., Wang, P., Wang, X. J., Li, Z. G., et al. (2017). DES-based microwave hydrodistillation coupled with GC-MS for analysis of essential oil from black pepper (Piper nigrum) and white pepper. Anal. Methods 9, 6777–6784. doi:10.1039/c7ay02072d
Zainal-Abidin, M. H., Hayyan, M., Hayyan, A., and Jayakumar, N. S. (2017). New horizons in the extraction of bioactive compounds using deep eutectic solvents: a review. Anal. Chim. Acta 979, 1–23. doi:10.1016/j.aca.2017.05.012
Keywords: deep eutectic solvents, Norway spruce roots, green chemistry, polyphenolic compounds, choline chloride
Citation: Kalyniukova A, Várfalvyová A, Płotka-Wasylka J, Majchrzak T, Makoś-Chełstowska P, Tomášková I, Pešková V, Pastierovič F, Jirošová A and Andruch V (2024) Deep eutectic solvent-based shaking-assisted extraction for determination of bioactive compounds from Norway spruce roots. Front. Chem. 12:1385844. doi: 10.3389/fchem.2024.1385844
Received: 13 February 2024; Accepted: 14 March 2024;
Published: 02 April 2024.
Edited by:
Halil Ibrahim Ulusoy, Cumhuriyet University, TürkiyeReviewed by:
Songul Ulusoy, Cumhuriyet University, TürkiyeAmarsinh Jadhav, D. Y. Patil College of Engineering and Technology, India
Marcello Locatelli, University of Studies G. d’Annunzio Chieti and Pescara, Italy
Copyright © 2024 Kalyniukova, Várfalvyová, Płotka-Wasylka, Majchrzak, Makoś-Chełstowska, Tomášková, Pešková, Pastierovič, Jirošová and Andruch. This is an open-access article distributed under the terms of the Creative Commons Attribution License (CC BY). The use, distribution or reproduction in other forums is permitted, provided the original author(s) and the copyright owner(s) are credited and that the original publication in this journal is cited, in accordance with accepted academic practice. No use, distribution or reproduction is permitted which does not comply with these terms.
*Correspondence: Alina Kalyniukova, ZGl1emhldmFAZmxkLmN6dS5jeg==