- 1School of Pharmacy, Changzhou University, Changzhou, Jiangsu, China
- 2Department of Chemistry and Biochemistry, Texas Tech University, Lubbock, TX, United States
The first photocatalytic trichloromethyl radical-triggered annulative reactions of amide-linked 1,7-diynes with polyhalomethanes were established for the flexible assembly of functionalized quinolin-2(1H)-ones with generally acceptable yields. With the installation of the aryl group (R1) into the alkynyl moiety, C-center radical-initiated Kharasch-type addition/nucleophilic substitution/elimination cascade to produce quinolin-2(1H)-ones-incorporating gem-dihaloalkene, whereas three examples of polyhalogenated quinolin-2(1H)-ones were afforded when amide-linked 1,7-diynes bearing two terminal alkyne units were subjected to BrCX3 by exploiting dry acetonitrile as a solvent.
Introduction
Aza-heterocyclic compounds are found in a wide variety of natural drugs and biologically active molecules, many of which are pharmacologically important (Pozharskii et al., 1997; Wen et al., 2022; Zhao et al., 2023; Liu et al., 2023a; Liu et al., 2023b). Among these, quinolin-2(1H)-one and its analogs are an important class of nitrogen-containing heterocycle scaffolds and are widely encountered in a myriad of pharmaceutical molecules and synthetic compounds (Sliskovic et al., 1991; Suzuki et al., 2001; Bach et al., 2002; Kuethe et al., 2005) which display versatile biological and pharmacological activities (McQuaid et al., 1992; Michael, 1995; Peifer et al., 2008), such as P2X7 receptor antagonist, rebamipide, and MAP kinase inhibitor (Figure 1) (Maignan et al., 2016; Tan et al., 2016; Miliutina et al., 2017; Wu et al., 2020). Various synthetic strategies have been achieved to construct the skeleton of such heterocycles, including Knorr synthesis (Liu et al., 2012; Ma et al., 2023), Friedlander reactions (Han et al., 2012), radical cyclization of acyclic precursors (Kadnikov and Larock, 2004; Manley and Bilodeau, 2004), and other methods (Fujita et al., 2004; Tsuritani et al., 2009; Berrino et al., 2012; Mai et al., 2014). The investigation of straightforward, atom-economic, environmentally acceptable, and green synthetic approaches to the construction of highly functionalized quinolin-2(1H)-ones remains a long-standing target and an active field of research in synthetic and medicinal chemistry. On the other hand, gem-dihaloalkenes are a unique structural unit with fascinating applications that range from organic synthesis to materials science (Rogawski, 2006; Meanwell, 2011) and can act as interesting synthetic intermediates in various chemical transformations for producing other useful molecules (Leriche et al., 2003; Okutami and Mori, 2009). Traditional approaches for the preparation of gem-dihaloalkenes include Wittig-type reactions, Julia–Kocienski reaction (Zhao et al., 2010; Chelucci, 2012; Zheng et al., 2013; Gao et al., 2015), and carbene insertion (Zeng et al., 2021) (Scheme 1A). With two geminal halogen atoms linked by an alkenyl carbon, these compounds exhibit higher reactivity for the oxidative addition of transition metal complexes than the corresponding monohaloolefins (London et al., 2014; Tian et al., 2016; Daniel et al., 2019), and the halogen atoms can be replaced by nucleophilic reagents through the additional elimination pathway (Yokota et al., 2007; Ichikawa et al., 2008). Despite significant progress in this field, the development of a new strategy for synthesizing a variety of valuable gem-dihaloalkenes remains a pressing need. To the best of our knowledge, the design and assembly of products incorporating a gem-dihaloalkene moiety and a quinolin-2(1H)-one skeleton using diynes as starting materials have not yet been reported.
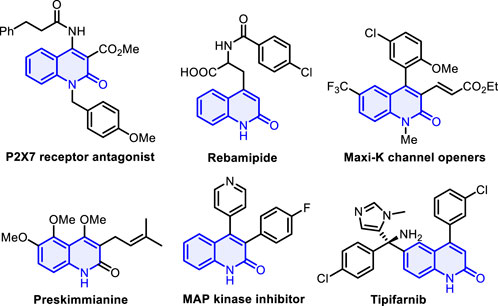
Figure 1. Selected examples of natural products and bioactive molecules containing quinolin-2(1H)-ones.
Over the years, the tandem annulation of 1,n-diynes has become an applicable and attractive tool for the collection of isocyclic and heterocyclic compounds via synergistic processes across its carbon–carbon triple-bond π system in an atom-economical manner (Singidi et al., 2010; Wang et al., 2017; Chintawar et al., 2019). For instance, Vidal and colleagues established Ru-catalyzed [2+2+2] cycloaddition of amide-linked 1,7-diynes with electron-rich cyanamide for forming benzo[c][2,7]naphthyridinones as a major product in good yields and regioselectivities (Scheme 1B) (Huvelle et al., 2022). Additionally, photocatalytic Kharasch-type-addition cyclization of 1,n-diynes provides another sustainable way of yielding various functionalized ring structures (Wang et al., 2021; Wu et al., 2021; Zheng et al., 2021). Recently, Jiang’s group elaborated a photocatalytic three-component biheterocyclization of heteroatom-linked 1,7-diynes with CBrCl3 and water as oxygen sources, leading to access of skeletally diverse fused-tricyclic heterocycles (Scheme 1C) (Wang et al., 2021). Intrigued by previous work and the continuation of our interest in radical cascade reactions (Wang et al., 2023a; Wang et al., 2023b; Wang et al., 2023c; Zhang et al., 2023), we believed that CCl3 radical derived from BrCCl3 under visible-light irradiation could add to preformed amide-linked 1,7-diynes followed by 6-exo-dig cyclization, 1,5-(SN″)-substitution, and dehydrohalogenation to furnish versatile functionalized quinolin-2(1H)-ones. No construction of quinolin-2(1H)-ones bearing gem-dihaloalkenes starting from 1,7-diynes and perhalogenated methanes has been reported. As anticipated, photocatalytic radical-induced addition-annulation was enabled by the reaction of amide-tethered 1,7-diynes 1 with bromotrichloromethane 2 in the presence of NaHCO3 to provide densely decorated 3-benzoyl-4-(2,2-dichlorovinyl)quinolin-2(1H)-ones 3 (Scheme 1D, path i). Notably, this reaction could obtain 3-(dibromomethyl)-4-(2,2-dichlorovinyl)quinolin-2(1H)-ones 4 when two terminal alkynes were installed into amide-tethered 1,7-diynes (Scheme 1D, path ii). We thus report these two types of interesting transformations.
Results and discussion
Initially, N-benzyl-N-(2-ethynylphenyl)-3-phenylpropiolamide 1a and CBrCl3 2a were selected as representative substrates under the irradiation of 30 W blue LEDs to identify the reaction conditions (Table 1). With eosin Y or Mes-Acr+ClO4− as photocatalysts, the reaction in the presence of K2CO3 in acetonitrile at room temperature did not detect the desired product 3a (entries 1–2). Fortunately, the use of fac-Ir(ppy)3 as a photocatalyst could drive the conversion of 1a into 3a, although the yield of quinolin-2(1H)-one 3a was 28% (entry 3). Next, we screened other inorganic and organic bases, comprising Na2CO3, KOAc, Na3PO4, NaHCO3, Na2HPO4, 4-dimethylaminopyridine (DMAP), and Et3N, for this photocatalysis by using fac-Ir(ppy)3 as the photocatalyst (entries 4–10). After careful screening, NaHCO3 was determined as the best choice, providing 3a at a higher 62% yield (entry 15). Based on fac-Ir(ppy)3 as a photocatalyst and NaHCO3 as a base, we then tested the solvent effect by screening several other solvents such as 1,2-dichloroethane (DCE, 33%), toluene (25%), 1,4-dioxane (22%), tetrahydrofuran (THF, NR), and EtOH (32%). The use of THF completely suppressed the reaction process, whereas other solvents we attempted gave more reduced yields than MeCN (entries 11–15).
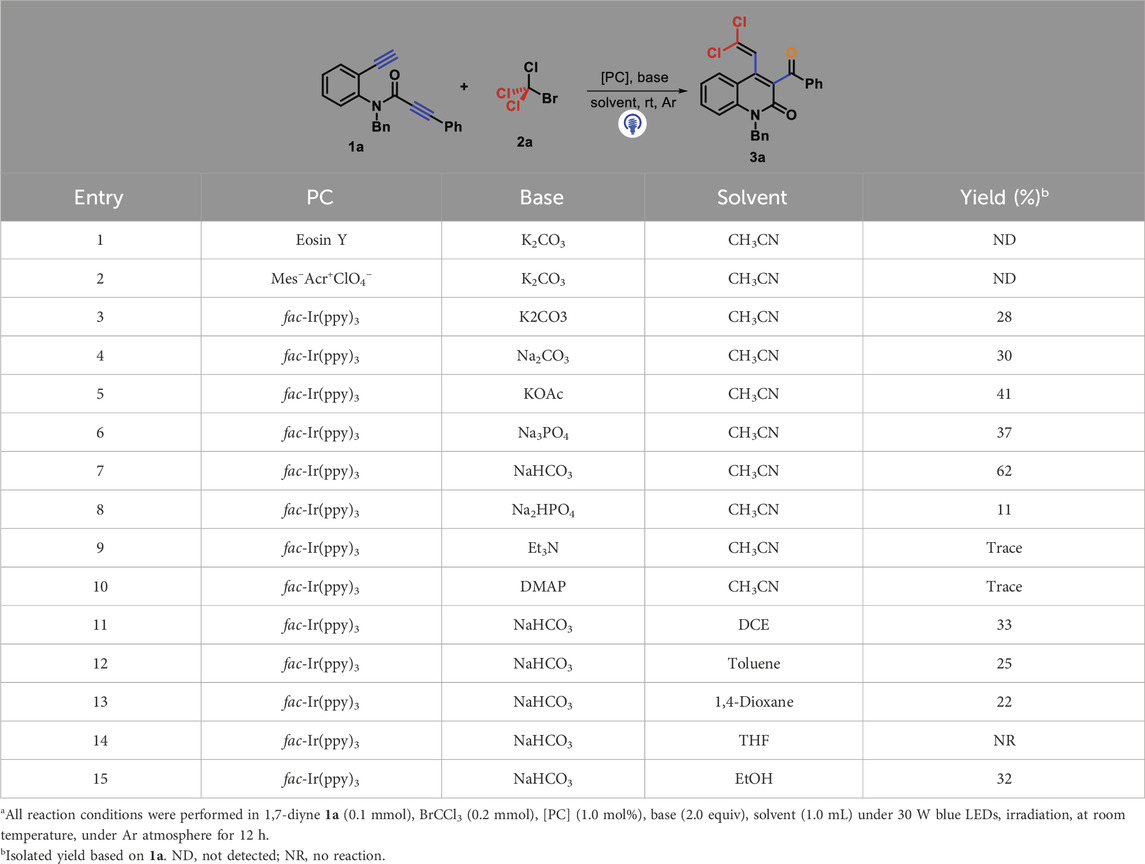
Table 1. Optimization conditions for forming 3aa.
Having establishing the optimal reaction conditions, we then evaluated the substrate scope and generality of an array of amide-linked 1,7-diynes for this photocatalytic radical tandem annulation toward synthesizing quinolin-2(1H)-ones bearing gem-dihaloalkenes; the results are summarized in Scheme 2. First, CBrCl3 (2a) reacted with 1,7-diynes 1 to investigate the influence of different the electronic properties and positions of substituents in the arylalkynyl units (R1), and all of them conveniently participated in the current cascade cyclization with acceptable yields. Both electron-donating (such as methyl 1b, methoxy 1c, and tert-butyl 1d) and electron-withdrawing (fluoro 1e) groups located at the para- or meta-position of the arylalkynyl moiety all performed well in this transformation, affording the corresponding gem-dichloroalkenes 3b–3e in 49%–59% yields. However, the obvious impact on steric hindrance and electronic effect was demonstrated because arylalkynyl with ortho-substituted or strong electron-withdrawing groups were suppressed during the reaction process, delivering almost no desired product. Subsequently, 1,7-diynes with different benzyl groups of nitrogen atoms could perform smoothly under standard conditions. The benzyl group bearing a functional group, including ether (o-methoxy 1f), alkyl (p-methyl 1i), and halogen (m-fluoro 1g, m-chloro 1h, p-fluoro 1j, p-chloro 1k, and p-bromo 1l), proved to be good candidates for the reaction, enabling their addition-cyclization to render the desired products 3f–3l with yields ranging from 48% to 66%. Subsequently, we chose methyl (1m and 1n) as the representative functional group to introduce the C4 or C5 position of the internal arene ring of 1,7-diynes to investigate its synthesis efficiency. The corresponding products 3m–3n were isolated in 41% and 46% yields, respectively. Furthermore, for the replacement of the benzyl group with a methyl group on the nitrogen atoms, amide-tethered 1,7-diynes 1o was a good reaction analog, giving the product 3o with a yield of 59%. Similarly, the substrate scope of this method was further assessed by taking advantage of CBr4 as the gem-dibromination reagent for assembling gem-dibromovinyl-incorporating quinolin-2(1H)-ones. We found that 1,7-diynes 1 with varied substitution patterns could effectively take part in the current system, furnishing corresponding products 3p–3s in 48%–65% yields. Unfortunately, N-unprotected amide-linked 1,7-diyne 1p and ester-linked 1,7-diyne 1q did not yield desired products. In addition, the preformed substrate 1r with two internal alkyne moieties was an unreactive reactant under standard conditions, and 1,7-diyne 1r was recovered, showing that terminal alkynes on starting material play an important role in this transformation.
To further expand the range of substrates for this transformation, amide-linked 1,7-diynes with two terminal alkynyl moieties 1s were subjected to the reaction of CBrCl3 under the above optimal conditions, but the reaction was completely suppressed. Surprisingly, the reaction can proceed smoothly in the presence of dry acetonitrile, and the unprecedented polyhalogenated quinolin-2(1H)-ones 4a was obtained in 54% yield via 1,5-(SN″)-substitution (Scheme 3A). Furthermore, a moderate chemical yield was observed for the 1,7-diynes with a methyl group located at the 5-position of the internal arene ring 1t for the assembly of the polyhalogenated products 4b–4c (Scheme 3B). The structures of densely functionalized quinolin-2(1H)-ones 3 and 4 were fully characterized by their NMR spectroscopy and HRMS date, and two cases of 3a and 4a were confirmed by X-ray diffraction analysis (see Supplementary Material).
The gram-scale experiments for the preparation of 3a on a 4.0 mmol scale were conducted under optimal conditions, and the product was delivered with a comparable yield (59%, Scheme 4A). The practicality of this methodology was further studied through the synthetic application of products. For example, the double nucleophilic vinylic substitution reaction 3a and p-toluenethiol proceeded smoothly by means of sodium hydride as base, which led to the product 5 in 81% yield (Scheme 4B) (Jiang et al., 2017).
Several control experiments were performed to gain insights into the reaction pathway mechanism. First, the use of a radical inhibitor TEMPO (2,2,6,6-tetramethyl-1-piperidinyloxy) successfully suppressed the reaction process, and the result confirmed that a trichloromethyl radical may be involved in these transformations (Scheme 5A). Next, the reaction occurred in the presence of H2O18, and the product containing O18 was isolated in 54% yield and identified by HR-MS (Scheme 5B). In addition, when dry CH3CN was employed as a solvent under standard conditions, the reaction progress was completely inhibited (Scheme 5C). These two survey results showed that the oxygen source of the carbonyl group in target products comes from water. Finally, several fluorescence quenching experiments indicated that CBrCl3 2a was a more efficient quencher of the excited state of fac-Ir(ppy)3* than 1,7-diyne 1a (Figure 2).
In light of these findings and previous related works (Wang et al., 2021; Wu et al., 2021; Zheng et al., 2021), we propose a plausible mechanism for this photo-catalyzed annulation of 1,7-diynes, as shown in Scheme 6. The photocatalytic cycle was initiated by the activation of Ir(III) with blue light irradiation to form the excited state Ir(III)* species, which reacts with BrCCl3 to yield trichloromethyl radical A and a bromine anion, together with Ir(IV) complex via a single electron transfer (SET). Next, the radical A can be trapped by the terminal carbon-carbon triple bond of 1,7-diyne 1 to provide the alkenyl radical B, which undergoes 6-exo-dig cyclization to give intermediate C. The resulting bromine anion was oxidized by Ir(IV) complex to produce Br radical (Bacauanu et al., 2018; Wang et al., 2019), followed by radical cross coupling with C to obtain intermediate D and regenerate Ir(III) species. Subsequently, the intermediate D reacts with OH− from H2O to afford the intermediate E through 1,5-(SN″)-substitution, which eliminates one molecule of HBr to assemble the desired product 3 (path i). The latter process, different from the above, undergoes 1,5-(SN″)-nucleophilic substitution with excess Br− in the photocatalytic system to give polyhalogenated products 4 (path ii).
Conclusion
Starting from new prepared amide-anchored 1,7-diynes, and easily available polyhalomethanes, we have illustrated a practical photocatalytic 6-exo-dig cyclization of 1,7-diynes, enabling substrate-controlled divergent synthesis of two types of functionalized quinolin-2(1H)-ones in moderate to excellent yields. When the aryl group (R1) was introduced into the alkynyl unit of 1,7-diynes, photoinduced radical cyclization cascades to access gem-dihaloalkene-containing quinolin-2(1H)-ones. Significantly, 1,7-diynes bearing two terminal alkynes were employed to react with BrCX3 by using dry acetonitrile as solvents, unexpectedly delivering three examples of polyhalogenated quinolin-2(1H)-ones. This reaction system features bond-forming efficiency, broad functional group compatibility, and mild reaction conditions. Further research on this amide-linked 1,7-diyne is currently being conducted by our group.
Data availability statement
The original contributions presented in the study are included in the article/Supplementary Material; further inquiries can be directed to the corresponding authors.
Author contributions
DC: Investigation, Writing–original draft. Z-JS: Data curation, Investigation, Writing–original draft. SY: Investigation, Writing–original draft. GL: Supervision, Writing–original draft. J-YW: Supervision, Writing–original draft. YZ: Supervision, Writing–original draft.
Funding
The author(s) declare that financial support was received for the research, authorship, and/or publication of this article. This research was supported by the School-level Research Projects of Changzhou University (No. ZMF23020007) and Robert A. Welch Foundation (D-1361-20210327, United States).
Conflict of interest
The authors declare that the research was conducted in the absence of any commercial or financial relationships that could be construed as a potential conflict of interest.
Publisher’s note
All claims expressed in this article are solely those of the authors and do not necessarily represent those of their affiliated organizations, or those of the publisher, the editors, and the reviewers. Any product that may be evaluated in this article, or claim that may be made by its manufacturer, is not guaranteed or endorsed by the publisher.
Supplementary material
The Supplementary Material for this article can be found online at: https://www.frontiersin.org/articles/10.3389/fchem.2024.1371978/full#supplementary-material
References
Bacauanu, V., Cardinal, S., Yamauchi, M., Kondo, M., Fernandez, D. F., Remy, R., et al. (2018). Metallaphotoredox difluoromethylation of aryl bromides. Angew. Chem. Int. Ed. 57, 12543–12548. doi:10.1002/anie.201807629
Bach, T., Bergmann, H., Grosch, B., and Harms, K. (2002). Highly enantioselective intra- and intermolecular [2 + 2] photocycloaddition reactions of 2-quinolones mediated by a chiral lactam host: host-guest interactions, product configuration, and the origin of the stereoselectivity in solution. J. Am. Chem. Soc. 124, 7982–7990. doi:10.1021/ja0122288
Berrino, R., Cacchi, S., Fabrizi, G., and Goggiamani, A. (2012). 4-Aryl-2-quinolones from 3,3-diarylacrylamides through intramolecular copper-catalyzed C–H functionalization/C–N bond formation. J. Org. Chem. 77, 2537–2542. doi:10.1021/jo202427m
Chelucci, G. (2012). Synthesis and metal-catalyzed reactions of gem-dihalovinyl systems. Chem. Rev. 112, 1344–1462. doi:10.1021/cr200165q
Chintawar, C.-C., Mane, M.-V., Tathe, A.-G., Biswas, S., and Patil, N.-T. (2019). Gold-catalyzed cycloisomerization of pyridine-bridged 1,8-diynes: an expedient access to luminescent cycl[3.2.2]azines. Org. Lett. 21, 7109–7113. doi:10.1021/acs.orglett.9b02677
Daniel, P. E., Onyeagusi, C. I., Ribeiro, A. A., Li, K., and Malcolmson, S. J. (2019). Palladium-catalyzed synthesis of α-trifluoromethyl benzylic amines via fluoroarylation of gem-Difluoro-2-azadienes enabled by phosphine-catalyzed formation of an azaallyl–silver intermediate. ACS Catal. 9, 205–210. doi:10.1021/acscatal.8b03999
Fujita, K.-i., Takahashi, Y., Owaki, M., Yamamoto, K., and Yamaguchi, R. (2004). Synthesis of five-six-and seven-membered ring lactams by cp*Rh complex-catalyzed oxidative N-heterocyclization of amino alcohols. Org. Lett. 6, 2785–2788. doi:10.1021/ol0489954
Gao, B., Zhao, Y., Hu, J., and Hu, J. (2015). Difluoromethyl 2-pyridyl sulfone: a versatile carbonyl gem-difluoroolefination reagent. Org. Chem. Front. 2, 163–168. doi:10.1039/C4QO00291A
Han, S.-Y., Choi, J. W., Yang, J., Chae, C. H., Lee, J., Jung, H., et al. (2012). Design and synthesis of 3-(4,5,6,7-tetrahydro-3Himidazo [4,5-c]pyridin-2-yl)-1H-quinolin-2-ones as VEGFR-2 kinase inhibitors. Bioorg. Med. Chem. Lett. 22, 2837–2842. doi:10.1016/j.bmcl.2012.02.073
Huvelle, S., Matton, P., Tran, C., Rager, M., Haddad, M., and Ratovelomanana-Vidal, V. (2022). Synthesis of benzo[c] [2,7]naphthyridinones and benzo[c] [2,6]naphthyridinones via ruthenium-catalyzed [2+2+2] cycloaddition between 1,7-diynes and cyanamides. Org. Lett. 24, 5126–5131. doi:10.1021/acs.orglett.2c01963
Ichikawa, J., Yokota, M., Kudo, T., and Umezaki, S. (2008). Efficient helicene synthesis: friedel–crafts-type cyclization of 1,1-Difluoro-1-alkenes. Angew. Chem. Int. Ed. 47, 4870–4873. doi:10.1002/anie.200801396
Jiang, M., Li, H., Yang, H., and Fu, H. (2017). Room-temperature arylation of thiols: breakthrough with aryl chlorides. Angew. Chem. Int. Ed. 56, 874–879. doi:10.1002/anie.201610414
Kadnikov, D. V., and Larock, R. C. (2004). Synthesis of 2-quinolones via palladium-catalyzed carbonylative annulation of internal alkynes by N-substituted o-iodoanilines. J. Org. Chem. 69, 6772–6780. doi:10.1021/jo049149+
Kuethe, J. T., Wong, A., Qu, C., Smitrovich, J., Davies, I. W., and Hughes, D. L. (2005). Synthesis of 5-substituted-1H-indol-2-yl-1H-quinolin-2-ones: a novel class of KDR kinase inhibitors. J. Org. Chem. 70, 2555–2567. doi:10.1021/jo0480545
Leriche, C., He, X., Chang, C.-W. T., and Liu, H.-W. (2003). Reversal of the apparent regiospecificity of nad(P)H-dependent hydride transfer: the properties of the difluoromethylene group, A carbonyl mimic. J. Am. Chem. Soc. 125, 6348–6349. doi:10.1021/ja021487+
Liu, L., Zhang, Y., Zhao, W., and Li, J. (2023b). Electron donor-acceptor complex induced fused indoles with hypervalent iodine (III) reagents. Org. Lett. 25, 6251–6255. doi:10.1021/acs.orglett.3c02009
Liu, L., Zhang, Y., Zhao, W., Wen, J., Dong, C., Hu, C., et al. (2023a). Photoredox-catalyzed cascade sp2 C−H bond functionalization to construct substituted acridine with diarylamine and hypervalent iodine(III) reagents. Org. Lett. 25, 592–596. doi:10.1021/acs.orglett.2c04114
Liu, X., Xin, X., Xiang, D., Zhang, R., Kumar, S., Zhou, F., et al. (2012). Facile and efficient synthesis of quinolin-2(1H)-ones via cyclization of penta-2,4-dienamides mediated by H2SO4. Org. Biomol. Chem. 10, 5643–5644. doi:10.1039/C2OB25767J
London, G., von Wantoch Rekowski, M., Dumele, O., Schweizer, W. B., Gisselbrecht, J.-P., Boudon, C., et al. (2014). Pentalenes with novel topologies: exploiting the cascade carbopalladation reaction between alkynes and gem-dibromoolefins. Chem. Sci. 5, 965–972. doi:10.1039/C3SC52623B
Ma, X., Zhang, Q., and Zhang, W. (2023). Remote radical 1,3-1,4-1,5-1,6- and 1,7-difunctionalization reactions. Molecules 28, 3027. doi:10.3390/molecules28073027
Mai, W.-P., Sun, G.-C., Wang, J.-T., Song, G., Mao, P., Yang, L.-R., et al. (2014). Silver-catalyzed radical tandem cyclization: an approach to direct synthesis of 3-Acyl-4-arylquinolin-2(1H)-ones. J. Org. Chem. 79, 8094–8102. doi:10.1021/jo501301t
Maignan, J. R., Lichorowic, C. L., Giarrusso, J., Blake, L. D., Casandra, D., Mutka, T. S., et al. (2016). ICI 56,780 optimization: structure–activity relationship studies of 7-(2-phenoxyethoxy)-4(1H)-quinolones with antimalarial activity. J. Med. Chem. 59, 6943–6960. doi:10.1021/acs.jmedchem.6b00759
Manley, P. J., and Bilodeau, M. T. (2004). A new synthesis of naphthyridinones and quinolinones: palladium-catalyzed amidation of o-carbonyl-substituted aryl halides. Org. Lett. 6, 2433–2435. doi:10.1021/ol049165t
McQuaid, L. A., Smith, E. C. R., Lodge, D., Pralong, E., Wikel, J. H., Calligaro, D. O., et al. (1992). 3-phenyl-4-hydroxyquinolin-2(1H)-ones: potent and selective antagonists at the strychnine-insensitive Glycine site on the N-methyl-daspartate receptor complex. J. Med. Chem. 35, 3423–3425. doi:10.1021/jm00096a019
Meanwell, N. A. (2011). Synopsis of some recent tactical application of bioisosteres in drug design. J. Med. Chem. 54, 2529–2591. doi:10.1021/jm1013693
Michael, J. P. (1995). Quinoline, quinazoline, and acridone alkaloids. Nat. Prod. Rep. 12, 465–475. doi:10.1039/NP9951200465
Miliutina, M., Ejaz, S. A., Khan, S. U., Iaroshenko, V. O., Villinger, A., Iqbal, J., et al. (2017). Synthesis, alkaline phosphatase inhibition studies and molecular docking of novel derivatives of 4-quinolones. Eur. J. Med. Chem. 126, 408–420. doi:10.1016/j.ejmech.2016.11.036
Okutami, M., and Mori, Y. (2009). Conversion of bromoalkenes into alkynes by wet tetra-n-butylammonium fluoride. J. Org. Chem. 74, 442–444. doi:10.1021/jo802101a
Peifer, C., Urich, R., Schattel, V., Abadleh, M., Röttig, M., Kohlbacher, O., et al. (2008). Implications for selectivity of 3,4-diarylquinolinones as p38αMAP kinase inhibitors. Bioorg. Med. Chem. Lett. 18, 1431–1435. doi:10.1016/j.bmcl.2007.12.073
Pozharskii, A. F., Soldatenkov, A. T., and Katritzky, A. R. (1997). “Heterocycles and health,” in Heterocycles in life and society (Chichester, UK: John Wiley), 135–164.
Rogawski, M. A. (2006). Diverse mechanisms of antiepileptic drugs in the development pipeline. Epilepsy Res. 69, 273–294. doi:10.1016/j.eplepsyres.2006.02.004
Singidi, R.-R., Kutney, A.-M., Gallucci, J.-C., and RajanBabu, T.-V. (2010). Stereoselective cyclization of functionalized 1,n-diynes mediated by [X-Y] Reagents [X-Y = R3Si-SnR’3 or (R2N)2B-SnR’3]: synthesis and properties of atropisomeric 1,3-dienes. J. Am. Chem. Soc. 132, 13078–13087. doi:10.1021/ja105939v
Sliskovic, D. R., Picard, J. A., Roark, W. H., Roth, B. D., Ferguson, E., Krause, B. R., et al. (1991). Inhibitors of cholesterol biosynthesis. 4. Trans-6-[2-(substitutedquinolinyl) ethenyl/ethyl]tetrahydro-4-hydroxy-2H-pyran-2-ones, a novel series of hmg-coa reductase inhibitors. J. Med. Chem. 34, 367–373. doi:10.1021/jm00105a057
Suzuki, M., Iwasaki, H., Fujikawa, Y., Kitahara, M., Sakashita, M., and Sakoda, R. (2001). Synthesis and biological evaluations of quinoline-based HmG-CoA reductase inhibitors. Bioorg. Med. Chem. 9, 2727–2743. doi:10.1016/S0968-0896(01)00198-5
Tan, L., Zhang, Z., Gao, D., Luo, J., Tu, Z. C., Li, Z., et al. (2016). 4-Oxo-1,4-dihydroquinoline-3-carboxamide derivatives as new axl kinase inhibitors. J. Med. Chem. 59, 6807–6825. doi:10.1021/acs.jmedchem.6b00608
Tian, P., Wang, C.-Q., Cai, S.-H., Song, S., Ye, L., Feng, C., et al. (2016). F– nucleophilic-addition-induced allylic alkylation. J. Am. Chem. Soc. 138, 15869–15872. doi:10.1021/jacs.6b11205
Tsuritani, T., Yamamoto, Y., Kawasaki, M., and Mase, T. (2009). Novel approach to 3,4-dihydro-2(1H)-quinolinone derivatives via cyclopropane ring expansion. Org. Lett. 11, 1043–1045. doi:10.1021/ol802669r
Wang, A.-F., Zhou, P., Zhu, Y.-L., Hao, W.-J., Li, G., Tu, S.-J., et al. (2017). Metal-free benzannulation of 1,7-diynes towards unexpected 1-aroyl-2-naphthaldehydes and their application in fused aza-heterocyclic synthesis. Chem. Commun. 53, 3369–3372. doi:10.1039/C7CC00323D
Wang, J.-Y., Li, G., Hao, W.-J., and Jiang, B. (2023b). Catalytic benzannulation reactions of enynones for accessing heterocycle-incorporating diarylmethanes. Synlett 34, 243–248. doi:10.1055/a-1971-6187
Wang, J.-Y., Zhang, S., Tang, Y., Yan, S., and Li, G. (2023a). Copper-catalyzed annulation-trifluoromethyl functionalization of enynones. Org. Lett. 25, 2509–2514. doi:10.1021/acs.orglett.3c00679
Wang, J.-Y., Zhang, S., Yuan, Q., Li, G., and Yan, S. (2023c). Catalytic radical-triggered annulation/iododifluoromethylation of enynones for the stereospecific synthesis of 1-indenones. J. Org. Chem. 88, 8532–8541. doi:10.1021/acs.joc.3c00471
Wang, L., Xu, T., Rao, Q., Zhang, T.-S., Hao, W.-J., Tu, S.-J., et al. (2021). Photocatalytic biheterocyclization of 1,7-diynes for accessing skeletally diverse tricyclic 2-pyranones. Org. Lett. 23, 7845–7850. doi:10.1021/acs.orglett.1c02865
Wang, S.-W., Yu, J., Zhou, Q.-Y., Chen, S.-Y., Xu, Z.-H., and Tang, S. (2019). Visible-light-induced atom transfer radical addition and cyclization of perfluoroalkyl halides with 1,n-enynes. ACS Sustain. Chem. Eng. 7, 10154–10162. doi:10.1021/acssuschemeng.9b02178
Wen, J., Zhao, W., Gao, X., Ren, X., Dong, C., Wang, C., et al. (2022). Synthesis of [1,2,3]Triazolo-[1,5-a]quinoxalin-4(5H)-ones through photoredox-catalyzed [3 + 2] cyclization reactions with hypervalent iodine(III) reagents. J. Org. Chem. 87, 4415–4423. doi:10.1021/acs.joc.2c00135
Wu, B., Cui, X., Zhu, T., Wang, S., Lu, C., Wang, J., et al. (2020). Design, synthesis and anticancer activity studies of novel trimethoxyphenyl-quinoline derivatives. Chin. J. Org. Chem. 40, 978–987. doi:10.6023/cjoc201909016
Wu, D., Hao, W.-J., Rao, Q., Lu, Y., Tu, S.-J., and Jiang, B. (2021). Engaging 1,7-diynes in a photocatalytic Kharasch-type addition/1,5-(“SN”)-substitution cascade toward β-gem-dihalovinyl carbonyls. Chem. Commun. 57, 1911–1914. doi:10.1039/D0CC07880H
Yokota, M., Fujita, D., and Ichikawa, J. (2007). Activation of 1,1-Difluoro-1-alkenes with a transition-metal complex: palladium(II)-Catalyzed friedel–crafts-type cyclization of 4,4-(difluorohomoallyl)arenes. Org. Lett. 9, 4639–4642. doi:10.1021/ol702279w
Zeng, X., Xu, Y., Liu, J., and Deng, Y. (2021). Access to gem-dibromoenones enabled by carbon-centered radical addition to terminal alkynes in water solution. Org. Lett. 23, 9058–9062. doi:10.1021/acs.orglett.1c03305
Zhang, S., Chen, D., Wang, J.-Y., Yan, S., and Li, G. (2023). Four-layer folding framework: design, GAP synthesis, and aggregation-induced emission. Front. Chem. 11, 1259609. doi:10.3389/fchem.2023.1259609
Zhao, W., Zhang, Y., Yuan, S., Yu, X., Liu, L., and Li, J. (2023). Direct alkylation of quinoxalinones with boracene-based alkylborate under visible light irradiation. J. Org. Chem. 88, 6218–6226. doi:10.1021/acs.joc.3c00266
Zhao, Y., Huang, W., Zhu, L., and Hu, J. (2010). Difluoromethyl 2-pyridyl sulfone: a new gem-difluoroolefination reagent for aldehydes and ketones. Org. Lett. 12, 1444–1447. doi:10.1021/ol100090r
Zheng, J., Cai, J., Lin, J.-H., Guo, Y., and Xiao, J.-C. (2013). Synthesis and decarboxylative Wittig reaction of difluoromethylene phosphobetaine. Chem. Commun. 49, 7513–7515. doi:10.1039/C3CC44271C
Keywords: 1,7-diynes, photoinduced, Kharasch addition, annulative reactions, quinolin-2(1H)-ones
Citation: Chen D, Song Z-J, Yan S, Li G, Wang J-Y and Zhang Y (2024) Photoinduced radical tandem annulation of 1,7-diynes: an approach for divergent assembly of functionalized quinolin-2(1H)-ones. Front. Chem. 12:1371978. doi: 10.3389/fchem.2024.1371978
Received: 17 January 2024; Accepted: 26 February 2024;
Published: 26 March 2024.
Edited by:
Alexey Sukhorukov, N. D. Zelinsky Institute of Organic Chemistry (RAS), RussiaReviewed by:
Manikandan Selvaraju, University of Kansas, United StatesTanmay Pati, Rensselaer Polytechnic Institute, United States
Copyright © 2024 Chen, Song, Yan, Li, Wang and Zhang. This is an open-access article distributed under the terms of the Creative Commons Attribution License (CC BY). The use, distribution or reproduction in other forums is permitted, provided the original author(s) and the copyright owner(s) are credited and that the original publication in this journal is cited, in accordance with accepted academic practice. No use, distribution or reproduction is permitted which does not comply with these terms.
*Correspondence: Jia-Yin Wang, d2p5Y2hlbUBjY3p1LmVkdS5jbg==; Yue Zhang, enlqc0BjY3p1LmVkdS5jbg==