- 1Institut de Chimie Moléculaire de Reims, UMR 7312, SFR Condorcet FR CNRS 3417, Université de Reims Champagne Ardenne, Reims, France
- 2URD Agro-Biotechnologies Industrielles (ABI), CEBB, AgroParisTech, Pomacle, France
Rhamnolipids (RLs) are highly valuable molecules in the cosmetic, pharmaceutic, and agricultural sectors with outstanding biosurfactant properties. In agriculture, due to their potential to artificially stimulate the natural immune system of crops (also known as elicitation), they could represent a critical substitute to conventional pesticides. However, their current synthesis methods are complex and not aligned with green chemistry principles, posing a challenge for their industrial applications. In addition, their bioproduction is cumbersome with reproducibility issues and expensive downstream processing. This work offers a more straightforward and green access to RLs, crucial to decipher their mechanisms of action and design novel potent and eco-friendly elicitors. To achieve this, we propose an efficient seven-step synthetic pathway toward (R)-3-hydroxyfatty acid chains present in RLs, starting from cellulose-derived levoglucosenone, with Michael addition, Baeyer–Villiger oxidation, Bernet–Vasella reaction, and cross-metathesis homologation as key steps. This method allowed the production of (R)-3-hydroxyfatty acid chains and derivatives with an overall yield ranging from 24% to 36%.
Introduction
Rhamnolipids (RLs) are glycolipidic biosurfactants composed of two key moieties: L-rhamnose unit(s) as hydrophilic head and a 3-(hydroxyalkanoyloxy)-alkanoic acid (HAA) fatty acid tail (Figure 1). The variations in this core structure allow the categorization of RLs into four main subgroups, called congeners: the mono-rhamno-mono-lipidic, the mono-rhamno-di-lipidic, the di-rhamno-mono-lipidic, and the di-rhamno-di-lipidic ones (Abdel-Mawgoud et al., 2010). Within these congeners, the RLs are described with regard to the length of the HAAs, providing a wide range of molecules with various highly valuable properties for many industrial applications. Indeed, the increasing interest in these molecules has made them serious competitors to synthetic surfactants in numerous industrial sectors, such as oil recovery (Rahman et al., 2003; Costa et al., 2010), therapeutics (Magalhães and Nitschke, 2013), cosmetics (Piljac and Piljac, 1999), cleaners (Parry et al., 2013), and agriculture (Sachdev and Cameotra, 2013; Miao et al., 2015; Dashtbozorg et al., 2016), with spectacular “eco-friendly” properties (e.g., biosourced, biocompatibility, biodegradability, and biostimulant activity) (Randhawa and Rahman, 2014). In addition, RLs have shown tremendous potential for academic studies dealing with the activation of crop immunity, a novel alternative to pesticides (Schellenberger et al., 2021). They are commonly found in various bacterial species, which makes them recognizable by the plant as a pathogen signal, triggering an immune response in planta. This eliciting property, characteristic of microbe-associated molecular patterns (MAMPs) and pathogen-associated molecular patterns (PAMPs), has great potential for future crop management (Héloir et al., 2019). Nevertheless, the origin of this eliciting property is still unsettled and requires assays with analogous molecules to better unveil the chemical function responsible for these promising properties. Hence, the development of straightforward, high-yielding, and sustainable synthetic pathways toward RLs and analogs is not only a great tool for academic research purposes but also for the production of potential agrochemicals for pest control.
The chemical synthesis of RLs is challenging, and synthetic routes reported in the literature lack diversity with only a few methods and starting materials (Bauer and Rademann, 2005; Bauer et al., 2006; Coss et al., 2012; De Vleeschouwer et al., 2014; Menhour et al., 2015; Menhour et al., 2016; Palos Pacheco et al., 2017; Compton et al., 2020; Cloutier et al., 2021). The method by Bauer et al., called hydrophobically assisted switching phase (HASP) synthesis, allows the production of a wide range of rhamnolipids but requires the independent total synthesis of each HAA, making the preparation of analogs cumbersome (Scheme 1). (Bauer and Rademann, 2005; Bauer et al., 2006) In addition, although elegant, this methodology suffers from important quantities of toxic solvents (e.g., methanol and chloroform) and atom-consuming protection/deprotection sequences, although it remains the most widely used methodology (Coss et al., 2012; De Vleeschouwer et al., 2014; Palos Pacheco et al., 2017; Cloutier et al., 2021). The synthesis of (R)-3-hydroxy-alkanoic acids was also reported by Jaipuri et al. (2004). Although the overall yield was quite good (38%, six steps), synthetic steps required the use of toxic reagents and solvents, as well as a restrictive setup (i.e., cooling at 0 or −78°C and microwave activation). In 2020, Compton et al. (2020) synthesized racemic 3-hydroxy decanoic acid using Reformatsky condensation.
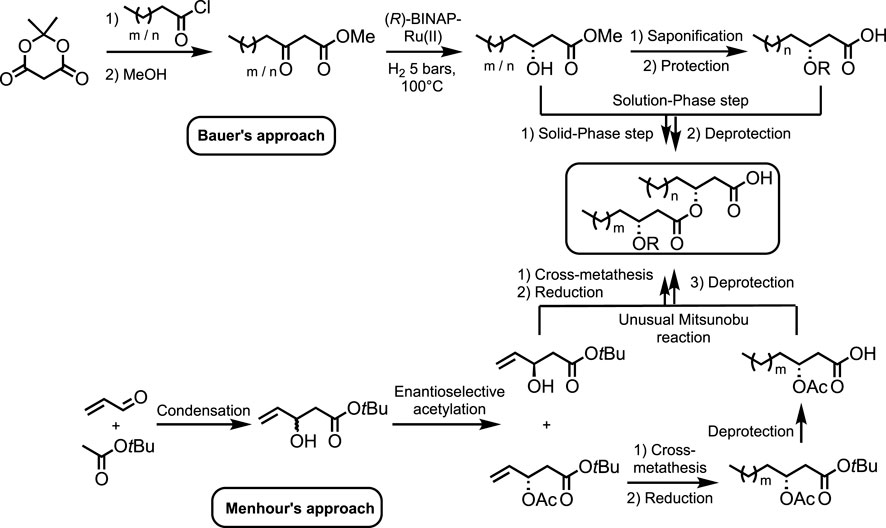
Scheme 1. Synthetic strategies developed by Bauer et al. and Menhour et al. to synthesize HAAs (Bauer et al., 2006; Menhour et al., 2015).
We have previously published articles focusing on (di-)lipidic HAAs synthesis starting from an acetoxyester (Scheme 1) (Menhour et al., 2015; Menhour et al., 2016). This compound is generated in two steps: 1) the condensation of acrolein with tert-butyl acetate leading to the racemic tert-butyl 3-hydroxypent-4-enoate and 2) the subsequent PS Amano lipase-biocatalyzed enantioselective acetylation (Dulęba et al., 2020). Using this valuable starting material and cross-metathesis, a library of HAAs has been synthesized from this unique precursor. Although an unusual Mitsunobu reaction has allowed the creation of di-lipidic structures, this synthesis requires further development to comply with the green chemistry principles. For instance, it would be more attractive if a biosourced starting material was used.
To address the aforementioned considerations, the work reported herein aims to develop a novel green synthetic pathway toward the HAA of RLs and analogs starting from levoglucosenone (LGO 1, Figure 2), a chiral biosourced synthon that can be obtained from cellulosic wastes through a catalytic aerobic fast pyrolysis approach (CFP), such as the Furacell™ process developed by Circa Group® (Court et al., 2012). LGO is recognized as a highly valuable chemical due to 1) the preservation of some natural chiral centers of cellulose and 2) the α,β-unsaturated ketone and masked aldehyde functionalities that open the way to a wide range of synthetic strategies. The proposed seven-step retrosynthetic pathway is described in Figure 2. The flexibility of this methodology will efficiently provide a library of analogs that could be involved in the HASP process and, thus, the possibility of establishing structure/activity relationships (SARs).
Advantageously, the natural chirality of LGO (chiral pool) allows obtaining 3-hydroxy-fatty acids with the desired (R)-configuration without the use of enantioselective reactions (Figure 2). Carefully chosen strategies will be explored to achieve good yields and limit protection/deprotection steps.
Results and discussion
Step 1: oxa-Michael addition
As previously reported by Diot et al., the oxa-Michael addition of water onto LGO occurred in exo with total selectivity to form hydrated levoglucosenone 2a in 75% yield (estimated with 1H NMR experiments) (Diot-néant et al., 2021). Approximately 10% of LGO remains in the crude mixture, along with 10% of a side product coming from LGO cross-coupling. Compound 2a can be purified by flash chromatography over silica gel, leading to a 62% isolated yield. Alternatively, the two by-products can also be partially removed through extraction. Indeed, these undesired components are more soluble in an organic solvent, herein ethyl acetate, than in water. In contrast, 2a, being more hydrophilic, remains mainly in the aqueous layer. Through this extraction, 2a was recovered in a 75% yield, and its purity was increased from 75% to 94% (determined by 1H NMR), which was high enough to avoid the purification step (Scheme 2).
For benzyl series b, the oxa-Michael addition of benzyl alcohol onto LGO following the conditions described by Kawai et al. gave 2b in a 94% yield (Kawai et al., 1995). As this step requires a large excess of benzyl alcohol (38 equiv.), the recyclability of benzyl alcohol was investigated to limit the ecological footprint. Interestingly, more than 98% of unreacted benzyl alcohol could be recovered through a short-path distillation (also known as molecular distillation).
The addition of ethanol to LGO was reported by Sharipov et al. (2019). However, we did not succeed in reproducing the 70% yield announced by Sharipov and co-workers using their conditions (0.5 equiv. Et3N, [LGO] = 77 mmol.L−1 in ethanol, 36 h, r.t.). To increase the yield further, a Design of Experiments (DoE) was performed on two factors: 1) concentration ranging from 0.08 to 0.5 mol.L−1, and 2) equivalence of Et3N varying between 0.5 and 2, over 72 h (Supplementary Section S3). To achieve the best HPLC yield (86%), the most diluted conditions (0.08 mol.L−1) with the highest amount of Et3N (2 equiv.) were required. Purification over silica gel was performed to fully recover 2c in high yield (86%) and purity (Scheme 2).
Step 2: Baeyer–Villiger oxidation
An organic solvent-free H2O2-mediated Baeyer–Villiger oxidation followed by an Amberlyst-catalyzed ethanolysis was performed on the 2 series (Allais et al., 2018; Bonneau et al., 2018) (Scheme 2).
In this step, 2a, 2b, or 2c and hydrogen peroxide were fully consumed, and Amberlyst beads were filtered off. Moreover, due to their low boiling point, formic acid by-products and solvents (i.e., ethanol and water) were readily removed under vacuum. Consequently, purification over silica gel was not required, and the desired 3a, 3b, and 3c were obtained in excellent yields (>99%, 93%, and 97%, respectively).
Step 3: activation (and protection)
The primary and secondary alcohols of 3a were then activated and protected by tosylation and acetylation, respectively, through a one-pot two-step procedure. A small excess of tosyl chloride was required to warrant the total conversion of 3a and limit residual reagent in the medium after extraction of 4a (77% overall yield without requiring purification over silica gel). To achieve 4d, the direct addition of acetic anhydride into the medium was performed, resulting in a global yield of 77%, meaning a quantitative acetylation of the tosylated intermediate (Scheme 2).
Activation of the primary alcohol of the lactone 3b proved to be more difficult than activation of the unprotected 3a. Indeed, the tosylation step never reached completion even with a longer reaction time (24 h instead of 2 h), higher dilution (0.5 mol.L−1 instead of 1 mol.L−1), or with the use of a co-solvent (1 mol.L−1 in Cyrene®, with 3 equiv. of pyridine). The tosylated product 4b was isolated after purification over silica gel with a maximum of 50% yield. As mechanochemical tosylation was described in the literature as an alternative sustainable methodology (Kazemi et al., 2007), an assay in a mortar led to a slight increase in conversion from 50% to 77%, as determined by 1H NMR. However, although trials performed in a ball grinder showed complete conversion of the starting material, the degradation of the desired product was observed. Thus, mesylation was attempted under solvent conditions, and TLC monitoring of the reaction revealed a total conversion of the starting material after only 2 h. After treatment, an 88% yield of the desired product 4e was isolated without the need for a specific purification step (Scheme 2). Successful activation of the primary alcohol of the lactone 3c was achieved by tosylation under the same conditions as previously described, leading to 4c in a 74% yield (Scheme 2).
Steps 4 and 5: iodination and Bernet–Vasella reaction
Tosylated 4d was then substituted by an iodine in the presence of sodium iodide, and subsequently, a Bernet–Vasella reaction was performed to provide the fully deprotected carboxylic acid with excellent yields (Bernet and Vasella, 1979) (Scheme 2). Interestingly, Wang and Nugent (2007) have previously reported a one-pot, two-step iodination and Bernet–Vasella procedure. Following their strategy, the one-pot two-step procedure led to an even higher global crude yield (e.g., 98% vs. 78% for the two steps). The Bernet–Vasella reaction was commonly performed in THF, a highly toxic solvent (Florent et al., 1987). At least one example reported using green n-propanol as the solvent (Aspinall et al., 1984). Unfortunately, compounds of the 4 series proved poorly soluble in n-propanol. Alternatively, the bio-based cyclopentyl methyl ether (CPME), a green substituent of THF, has been used successfully for the conversion of 4d. However, the final procedure used acetone as the solvent instead of CPME, as the latter proved to be a poor solvent for 4b. Excellent crude yields were achieved for 5a, 5b, and 5c (91%, 95%, and 98%, respectively). Note that the workup of the Bernet–Vasella reaction, consisting of filtration over a Celite® pad and extraction, led to the 5 series with very few impurities. Nevertheless, purification over silica gel can be performed to obtain pure 5a, 5c, and 5d in 55%, 78%, and 89% yields, respectively (Scheme 2). The highly hydrophilic 5a would require alternative purification technology than flash chromatography over silica gel.
Step 6: cross-metathesis homologation
With the different compounds in hand, the elongation of the fatty acid chain was investigated using cross-metathesis. This methodology allows the introduction of various chain lengths starting from the same precursor. However, because (R)-3-hydroxydecanoic acid has recently been reported as a very powerful elicitor, we will focus on the C-10 chain length (Schellenberger et al., 2021).
Various conditions (catalyst loading and addition of catalyst by portions or through syringe pump, in dry CPME or methylene chloride, with different concentrations) have been tested for Grubbs II-catalyzed cross-metathesis reactions between pure 5d and hept-1-ene (Scheme 3; Table 1). Grubbs II catalyst has been chosen due to its high stability to air and humidity, allowing simpler handling, in addition to its high efficacy in catalyzing the metathesis reaction. In all cases, a catalytic amount of copper(I) iodide was added to the medium as it was proved to have a beneficial effect on the yield of olefin cross-metathesis (Voigtritter et al., 2011). The continuous addition of 4.8 mol% of Grubbs II catalyst through a syringe pump has led to the best yield in 6d (62%, Table 1, entry 6), similar to what was reported by Menhour et al. (65%) (Menhour et al., 2016). Note that the starting material was not fully consumed. Unfortunately, its recovery was not possible due to the concomitant elution of a contaminating by-product. A tentative structure elucidation based on the 1H, 13C, and 2D spectra led to compound 10 (Figure 3).
Cross-metathesis between 5b and hept-1-ene was performed following the best conditions obtained for 5d (Table 1, entry 6), leading to 6b in a 47% yield (Scheme 3). Decreasing the catalyst loading by two-fold caused only a slight decrease in yield (41%), but increasing the concentration to 0.25 mol.L−1 was more influential with a drop of 12 points and 15 points in yield with Grubbs II catalyst loading of 4.8 mol% and 2.4 mol%, respectively. It is worth mentioning that the hydrogenation was not performed directly after the cross-metathesis to allow the recovery of starting material 5b. This was possible for the diluted media (i.e., 0.1 mol.L−1), and ca. 40% of the starting material was recovered without any detectable by-product, whereas in more concentrated experiments, a small amount of the same kind of impurity as for 5d was observed by 1H NMR analysis. When using 5a, contrary to what was observed from studies on 5d and 5b, reducing the amount of catalyst led to a higher yield of 6a (from 24% to 46%). After purification, 60% of the desired compound 6c and 38% of starting material 5c were recovered.
To investigate the influence of the presence of the free carboxylic acid on the cross-metathesis reaction, benzylation of the 5 series was performed, leading to the 7 series, followed by cross-metathesis with hept-1-ene (Scheme 3). Cross-metathesis of the protected ester 7a provided a higher yield (8a, 59%) than the free carboxylic acid 5a (6a, 46%). In contrast, 8d was only obtained in a 38% yield, and 53% of the starting material 7d was recovered, whereas 6d reached a 62% yield from 5d.
The cross-metathesis yields reported in this article are consistent with values reported in the literature for similar reactions (Menhour et al., 2016). The presence of a free carboxylic acid group does not seem to highly impact the reactivity of the Grubbs II catalyst, as no clear trend was observed by comparison with unprotected versus benzylated esters. Increasing the concentration from 0.1 to 0.25 mol.L−1 also has a predominantly positive effect on yields. The presence of an alcohol moiety in position 3 and a bulky group (benzyl) have a negative impact on the yields, which did not exceed 50%. In comparison, smaller substituents (i.e., acetyl and ethyl) allowed 60% yields to be achieved.
Step 7: hydrogenation
Finally, a palladium-catalyzed hydrogenation is required to achieve the synthesis of HAAs. Whereas the reduction of the double bond was completed overnight in all cases, removing the 3-O-benzyl moiety proved more challenging. However, this drawback allowed achieving the unexpected analog 9b in a 72% yield. Acetic acid was added to the ethanolic reaction medium to successfully achieve complete benzyl cleavage. All reactions proceeded with yields higher than 95%.
Compliance with green chemistry
First, LGO was chosen as the starting material not only because it is a biosourced chemical but also because it is obtained through flash-pyrolysis of cellulosic wastes through the Furacell process™, an energy-neutral approach (Circa group et al., 2023). Second, particular attention was focused on the nature of the solvents and their quantity. Indeed, no halogen-containing solvent was used, and toxic diethyl ether was replaced by CPME, which is characterized by a low formation of peroxide, a high boiling point (106°C), and renewable resource sourcing (Merck, 2019). In addition, acetone, ethyl acetate, benzyl alcohol, and ethanol can be produced from renewable feedstocks (Weizmann and Rosenfeld, 1937; Pattanaik and Mandalia, 2011; Redl et al., 2017; Liu et al., 2020; Flaiz et al., 2021; Melendez et al. 2022). Whenever possible, organic solvents were avoided (e.g., STEP 2), or the substrate concentration was as high as 1 mol.L−1 to reduce their consumption (STEPS 3–5). It is worth mentioning that, in the case of benzyl alcohol, its recyclability was successfully achieved through a short-path distillation. To further comply with the principles of green chemistry, toxic reagents were avoided whenever possible. For example, a two-step procedure (tosylation followed by iodination) was preferred to the more direct Appel reaction to avoid the use of the toxic triphenylphosphine and limit waste (i.e., triphenylphosphine oxide). Finally, the synthetic pathway described herein requires a minimum of purification steps (i.e., chromatography over silica) to access the desired compounds. Indeed, over seven steps (i.e., oxa-Michael addition, Baeyer–Villiger oxidation, activation, iodination, Bernet–Vasella reaction, cross-metathesis, and hydrogenation), only two to three purifications over silica gel were required depending on the targeted product. The key purification was systematically performed after the cross-metathesis homologation to remove by-products and recover both the desired product and the starting material.
The ecological performance of each step has been calculated using green metrics to assess their impact. Several methods exist to determine such metrics (Van Aken et al., 2006). Among them, a very simple one is the process mass intensity (PMI) ratio, which establishes a ratio between the summed masses of all products entering into a reaction (water excepted) and the mass of recovered target(s). EcoScale, another approach designed by Van Aken et al., evaluates six criteria (i.e., yield, price, safety, technical setup, temperature/time, and workup/purification) and gives penalty points deduced from a starting score of 100 (Van Aken et al., 2006). This method has been specifically designed for laboratory scale and allows ranking the reactions in three categories: 1) >75, excellent; 2) >50, acceptable; and 3) <50, inadequate. Combining these two methods led to an overview in terms of waste generation and the safety/difficulty of each reaction without performing complex calculations, as in the case of an in-depth life cycle assessment (LCA). PMI and EcoScale scores were calculated for each step of the process to evaluate which pathway was the eco-friendliest. It is important to mention that the PMI calculations did not consider treatment after the reaction due to the lack of information in the literature on the volume of solvents used during extraction and purification procedures.
For our process (Supplementary Table S6.1), all PMI ratios were significantly low (<15), except for the cross-metathesis step (between 31 and 72), particularly due to the high dilution (C = 0.1 mol.L−1). Indeed, when the concentration was increased from 0.1 to 0.25 mol.L−1 for the conversion of 7b into 8b, the PMI ratio was logically divided 2.5-fold, leading to a very acceptable value. Moreover, recyclability through distillation of CPME (bp 106°C) and unreacted hept-1-ene (bp 93°C) was not addressed here but could be considered an appreciable improvement. Whatever the synthetic strategy chosen, the average PMI ratio is less than 20, which is very good for the synthesis of a fine chemical. Generally speaking, our approach results in high to excellent EcoScale values for most steps thanks to the safety of reactants, the simplicity of treatments, the few purifications, and the good to excellent yields. One notable exception is the cross-metathesis homologation. Indeed, the moderate yields of these reactions, in addition to the use of an expensive catalyst and toxic hept-1-ene, led to inadequate reaction conditions following Van Aken’s definition. Overall, our seven-step synthetic pathway reaches acceptable conditions with average EcoScale scores above 60 (Table 2).
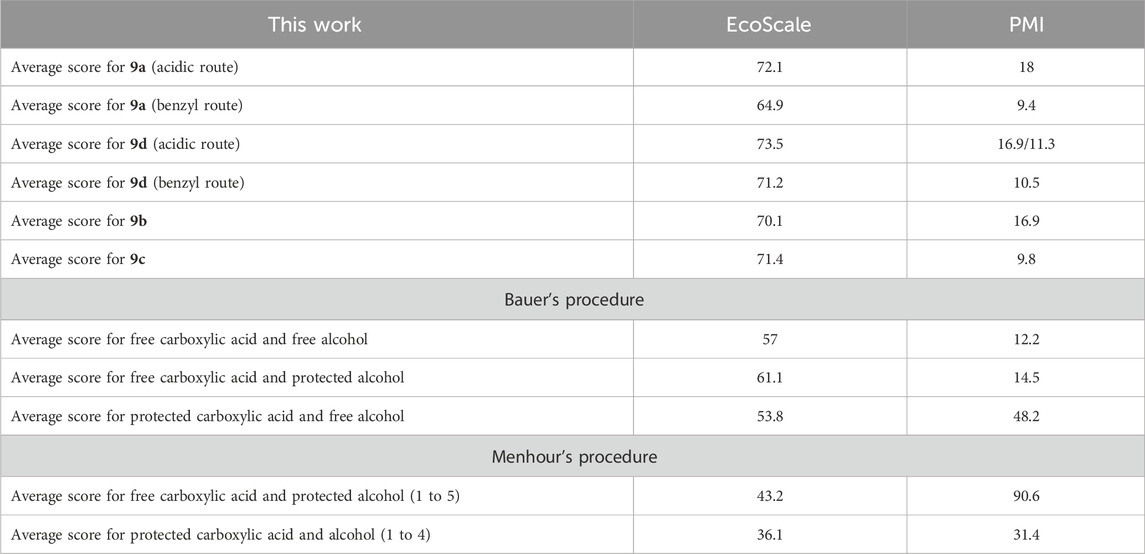
Table 2. Average PMI and EcoScale scores for the various pathways explored in this work compared with Bauer’s and Menhour’s procedures.
Surprisingly, pathways involving the benzyl protection achieved better PMI ratios than the direct route. That can be mainly explained by the increase in yield for the cross-metathesis homologation in the alcohol series (7a compared to 5a) and the high recovery of the starting material in the acetyl series (7d compared to 5d). However, the impact on the average EcoScale score is slightly negative for the acetyl derivatives; the score is more impacted for the alcohol derivatives (Table 2).
In order to assess the ecological performance of the developed process, the aforementioned EcoScale and PMI results were benchmarked against those of the already published research works.
Even though Bauer and co-workers have used toxic solvents such as chloroform, methylene chloride, or methanol (Bauer et al., 2006), their EcoScale score remains acceptable to good due to the high yields of each reaction step (>78%), few purification steps, and easy setup (except for the selective hydrogenation) and workups. However, the PMI ratio shows that this synthetic methodology generates an unreasonable amount of waste, especially during the desilylation, although the latter was carried out at a multigram scale (Table 2).
With Menhour et al.’s strategy (Supplementary Table S6.3) (Menhour et al., 2016), the PMI ratio remains acceptable as long as the reactions were performed on a “convenient” scale but drastically increases when performed on 1 mmol (cross-metathesis followed by hydrogenation), and even more on 0.1 mmol (deprotection). This shows the importance of working in concentrated conditions to limit the environmental footprint, which becomes more and more difficult when the scale is reduced. In contrast, the EcoScale score was poor for the three first steps due to toxic solvents and reagents (e.g., diethyl ether and acrolein) and low yield (i.e., enantioselective acetylation), whereas good values were achieved for the two last steps.
To summarize, considering PMI and EcoScale scores, even with more steps, our synthetic procedure remains more sustainable than the previously reported procedures. The desired HAAs were obtained with global yields ranging from 24% to 36%. The implementation of this new pathway is very easy (common setup and materials, no need for anhydrous solvent, etc.) and has been conducted on a (multi)gram scale. The synthetized HAAs could be used directly in biological assays to evaluate their impact on stimulating plant immune systems. They could also be used in the HASP developed by Bauer et al. to achieve functionalized rhamnolipids.
Data availability statement
The original contributions presented in the study are included in the article/Supplementary Material, further inquiries can be directed to the corresponding authors. In addition, NMR FID raw files can be found at DOI: 10.57745/D1G0IK.
Author contributions
EP: data curation, formal analysis, investigation, and writing–original draft. AF: conceptualization, data curation, formal analysis, investigation, methodology, writing–original draft, and writing–review and editing. M-CB: formal analysis, supervision, and writing–review and editing. SC: formal analysis, supervision, and writing–review and editing. FBi: data curation, formal analysis, and writing–review and editing. FBo: data curation, formal analysis, and writing–review and editing. AP: methodology, writing–original draft; FA: conceptualization, funding acquisition, methodology, project administration, resources, supervision, validation, visualization, writing–original draft, and writing–review and editing. AH: conceptualization, data curation, funding acquisition, methodology, project administration, resources, supervision, validation, visualization, writing–original draft, and writing–review and editing.
Funding
The authors declare that financial support was received for the research, authorship, and/or publication of this article. The authors want to acknowledge SFR Condorcet for financial support (LEGO grant). URD ABI thanks Grand Reims, Région Grand Est, and Conseil Général de la Marne for financial support.
Acknowledgments
The authors want to acknowledge Anne Wadouachi and Chloé Herrlé from LG2A, UMR CNRS 7378, UPJV for performing mechanochemistry assays.
Conflict of interest
The authors declare that the research was conducted in the absence of any commercial or financial relationships that could be construed as a potential conflict of interest.
The authors declared that they were an editorial board member of Frontiers, at the time of submission. This had no impact on the peer review process and the final decision.
Publisher’s note
All claims expressed in this article are solely those of the authors and do not necessarily represent those of their affiliated organizations, or those of the publisher, the editors, and the reviewers. Any product that may be evaluated in this article, or claim that may be made by its manufacturer, is not guaranteed or endorsed by the publisher.
Supplementary material
The Supplementary Material for this article can be found online at: https://www.frontiersin.org/articles/10.3389/fchem.2024.1362878/full#supplementary-material
References
Abdel-Mawgoud, A. M., Lépine, F., and Déziel, E. (2010). Rhamnolipids: diversity of structures, microbial origins and roles. Appl. Microbiol. Biotechnol. 86 (5), 1323–1336. doi:10.1007/s00253-010-2498-2
Allais, F., Bonneau, G., Peru, A. A. M., and Flourat, A. L. (2018). Method for converting levoglucosenone into 4-hydroxymethyl butyrolactone or 4-hydroxymethyl butenolide without using any organic solvent and catalyst. https://patents.google.com/patent/WO2018007764A1/en.
Aspinall, G. O., Chatterjee, D., and Khondo, L. (1984). The hex-5-enose degradation: zinc dust cleavage of 6-Deoxy-6-Iodo-a-D-Galactopyranosidic linkages in methylated di- and trisaccharides. Can. J. Chem. 62, 2728–2735. doi:10.1139/v84-464
Bauer, J., Brandenburg, K., Zähringer, U., and Rademann, J. (2006). Chemical synthesis of a glycolipid library by a solid-phase strategy allows elucidation of the structural specificity of immunostimulation by rhamnolipids. Chem. - A Eur. J. 12 (27), 7116–7124. doi:10.1002/chem.200600482
Bauer, J., and Rademann, J. (2005). Hydrophobically assisted switching Phase synthesis: the flexible combination of solid-phase and solution-phase reactions employed for oligosaccharide preparation. J. Am. Chem. Soc. 127 (20), 7296–7297. doi:10.1021/ja051737x
Bernet, B., and Vasella, A. (1979). Carbocyclische Verbindungen aus Monosacchariden. I. Umsetzungen in der glucosereihe. Helv. Chim. Acta 62 (204), 1990–2016. doi:10.1002/hlca.19790620629
Bonneau, G., Peru, A. A. M., Flourat, A. L., and Allais, F. (2018). Organic solvent- and catalyst-free Baeyer–Villiger oxidation of levoglucosenone and dihydrolevoglucosenone (Cyrene®): a sustainable route to (S)-γ-hydroxymethyl-α,β-butenolide and (S)-γ-hydroxymethyl-γ-butyrolactone. Green Chem. 20 (11), 2455–2458. doi:10.1039/c8gc00553b
Cloutier, M., Prévost, M.-J., Lavoie, S., Feroldi, T., Piochon, M., Groleau, M.-C., et al. (2021). Total synthesis, isolation, surfactant properties, and biological evaluation of ananatosides and related macrodilactone-containing rhamnolipids. Chem. Sci. 12 (21), 7533–7546. doi:10.1039/D1SC01146D
Compton, A. A., Deodhar, B. S., Fathi, A., and Pemberton, J. E. (2020). Optimization of a chemical synthesis for single-chain rhamnolipids. ACS Sustain Chem. Eng. 8 (24), 8918–8927. doi:10.1021/acssuschemeng.0c00733
Coss, C., Carrocci, T., Maier, R. M., Pemberton, J. E., and Polt, R. (2012). Minimally competent lewis acid catalysts: indium(III) and bismuth(III) salts produce rhamnosides (=6-Deoxymannosides) in high yield and purity. Helv. Chim. Acta 95 (12), 2652–2659. doi:10.1002/hlca.201200528
Costa, S. G. V. A. O., Nitschke, M., Lépine, F., Déziel, E., and Contiero, J. (2010). Structure, properties and applications of rhamnolipids produced by Pseudomonas aeruginosa L2-1 from cassava wastewater. Process Biochem. 45 (9), 1511–1516. doi:10.1016/j.procbio.2010.05.033
Court, G. R., Lawrence, C. H., Raverty, W. D., and Duncan, A. J. (2012). Method for converting lignocellulosic materials into useful chemicals. https://patents.google.com/patent/US20120111714A1/en.
Dashtbozorg, S. S., Kohl, J., and Ju, L.-K. (2016). Rhamnolipid adsorption in soil: factors, unique features, and considerations for use as green antizoosporic agents. J. Agric. Food Chem. 64 (17), 3330–3337. doi:10.1021/acs.jafc.6b00215
De Vleeschouwer, M., Sinnaeve, D., Van den Begin, J., Coenye, T., Martins, J. C., and Madder, A. (2014). Rapid total synthesis of cyclic lipodepsipeptides as a premise to investigate their self-assembly and biological activity. Chem. A Eur. J. 20 (25), 7766–7775. doi:10.1002/chem.201402066
Diot-néant, F., Mouterde, L. M. M., Couvreur, J., Brunois, F., Miller, S. A., and Allais, F. (2021). Green synthesis of 2-deoxy-D-ribonolactone from cellulose-derived levoglucosenone (LGO): a promising monomer for novel bio-based polyesters. Eur. Polym. J. 159 (110745), 110745–110748. doi:10.1016/j.eurpolymj.2021.110745
Dulęba, J., Siódmiak, T., and Marszałł, M. P. (2020). Amano lipase PS from burkholderia cepacia- evaluation of the effect of substrates and reaction media on the catalytic activity. Curr. Org. Chem. 24 (7), 798–807. doi:10.2174/1385272824666200408092305
Flaiz, M., Ludwig, G., Bengelsdorf, F. R., and Dürre, P. (2021). Production of the biocommodities butanol and acetone from methanol with fluorescent FAST-tagged proteins using metabolically engineered strains of eubacterium limosum. Biotechnol. Biofuels 14 (1), 117–120. doi:10.1186/s13068-021-01966-2
Florent, J. C., Ughetto-monfrin, J., and Monneret, C. (1987). Anthracyclinones. 2. Isosaccharinic acid as chiral template for the synthesis of (+)-4-demethoxy-9-deacetyl-9-hydroxymethyldaunomycinone and (-)-4-deoxy-.gamma.-rhodomycinone. J. Org. Chem. 52 (6), 1051–1056. doi:10.1021/jo00382a015
Héloir, M.-C., Adrian, M., Brulé, D., Claverie, J., Cordelier, S., Daire, X., et al. (2019). Recognition of elicitors in grapevine: from MAMP and DAMP perception to induced resistance. Front. Plant Sci. 10 (1117), 1117–17. doi:10.3389/fpls.2019.01117
Jaipuri, F. A., Jofre, M. F., Schwarz, K. A., and Pohl, N. L. (2004). Microwave-assisted cleavage of weinreb amide for carboxylate protection in the synthesis of a (R)-3-Hydroxyalkanoic acid. Tetrahedron Lett. 45 (21), 4149–4152. doi:10.1016/j.tetlet.2004.03.148
Kawai, T., Isobe, M., and Peters, S. C. (1995). Factors affecting reaction of 1, 6-anhydrohexos-2-ulose derivatives. Aust. J. Chem. 48, 115–131. doi:10.1071/CH9950115
Kazemi, F., Massah, A. R., and Javaherian, M. (2007). Chemoselective and scalable preparation of alkyl tosylates under solvent-free conditions. Tetrahedron 63 (23), 5083–5087. doi:10.1016/j.tet.2007.03.083
Liu, L., Zhu, Y., Chen, Y., Chen, H., Fan, C., Mo, Q., et al. (2020). One-pot cascade biotransformation for efficient synthesis of benzyl alcohol and its analogs. Chem. Asian J. 15 (7), 1018–1021. doi:10.1002/asia.201901680
Magalhães, L., and Nitschke, M. (2013). Antimicrobial activity of rhamnolipids against Listeria monocytogenes and their synergistic interaction with nisin. Food control 29 (1), 138–142. doi:10.1016/j.foodcont.2012.06.009
Menhour, B., Mayon, P., Plé, K., Bouquillon, S., Dorey, S., Clément, C., et al. (2015). A stereocontrolled synthesis of the hydrophobic moiety of rhamnolipids. Tetrahedron Lett. 56 (9), 1159–1161. doi:10.1016/j.tetlet.2015.01.091
Menhour, B., Obounou-Akong, F., Mayon, P., Plé, K., Bouquillon, S., Dorey, S., et al. (2016). Recycling Mitsunobu coupling: a shortcut for troublesome esterifications. Tetrahedron 72 (47), 7488–7495. doi:10.1016/j.tet.2016.09.065
Merck, (2019). The future of solvents: bio renewable. The future of solvents: bio renewable. https://www.sigmaaldrich.com/IN/en/campaigns/biorenewable-solvents.
Miao, S., Dashtbozorg, S. S., Callow, N. V., and Ju, L.-K. (2015). Rhamnolipids as platform molecules for production of potential anti-zoospore agrochemicals. J. Agric. Food Chem. 63 (13), 3367–3376. doi:10.1021/acs.jafc.5b00033
Palos Pacheco, R., Eismin, R. J., Coss, C. S., Wang, H., Maier, R. M., Polt, R., et al. (2017). Synthesis and characterization of four diastereomers of monorhamnolipids. J. Am. Chem. Soc. 139 (14), 5125–5132. doi:10.1021/jacs.7b00427
Parry, A. J., Parry, N. J., Peilow, A. C., and Stevenson, P. S. (2013). Combinaisons de Rhamonolipides et d’enzymes Pour Un Nettoyage Amélioré. https://patentimages.storage.googleapis.com/8d/c4/a6/591eecb455a317/EP2596087B1.pdf.
Pattanaik, B. N., and Mandalia, H. C. (2011). Ethyl acetate: properties, production processes and applications - a review. Int. J. Curr. Res. Rev. 3 (12), 23–40.
Piljac, T., and Piljac, G. (1999). Utilisation de Rhamnolipides comme agents cosmetiques. WO1999/043334.
Rahman, K. S. M., Rahman, T. J., Kourkoutas, Y., Petsas, I., Marchant, R., and Banat, I. M. (2003). Enhanced bioremediation of N-alkane in petroleum sludge using bacterial consortium amended with rhamnolipid and micronutrients. Bioresour. Technol. 90 (2), 159–168. doi:10.1016/S0960-8524(03)00114-7
Randhawa, K. K. S., and Rahman, P. K. S. M. (2014). Rhamnolipid biosurfactants-past, present, and future scenario of global market. Front. Microbiol. 5 (SEP), 1–7. doi:10.3389/fmicb.2014.00454
Redl, S., Sukumara, S., Ploeger, T., Wu, L., Ølshøj Jensen, T., Nielsen, A. T., et al. (2017). Thermodynamics and economic feasibility of acetone production from syngas using the thermophilic production host moorella thermoacetica. Biotechnol. Biofuels 10, 150. doi:10.1186/s13068-017-0827-8
Sachdev, D. P., and Cameotra, S. S. (2013). Biosurfactants in agriculture. Appl. Microbiol. Biotechnol. 97 (3), 1005–1016. doi:10.1007/s00253-012-4641-8
Schellenberger, R., Crouzet, J., Nickzad, A., Kutschera, A., Gerster, T., Borie, N., et al. (2021). Bacterial rhamnolipids and their 3-hydroxyalkanoate precursors activate Arabidopsis innate immunity through two independent mechanisms. Proc. Natl. Acad. Sci. 118 (39), e2101366118. doi:10.1073/pnas.2101366118
Melendez, J. R., Mátyás, B., Hena, S., Lowy, D. A., and El Salous, A. (2022). Perspectives in the production of bioethanol: A review of sustainable methods, technologies, and bioprocesses. Renew. Sust. Energ. Rev. 160, 112260. doi:10.1016/j.rser.2022.112260
Sharipov, B. T., Davydova, A. N., Faizullina, L. K., and Valeev, F. A. (2019). Preparation of the diastereomerically pure 2S-hydroxy derivative of dihydrolevoglucosenone (Cyrene). Mendeleev Commun. 29 (2), 200–202. doi:10.1016/j.mencom.2019.03.029
Van Aken, K., Strekowski, L., and Patiny, L. (2006). EcoScale, a semi-quantitative tool to select an organic preparation based on economical and ecological parameters. Beilstein J. Org. Chem. 2 (3), 3–7. doi:10.1186/1860-5397-2-3
Voigtritter, K., Ghorai, S., and Lipshutz, B. H. (2011). Rate enhanced olefin cross-metathesis reactions: the copper iodide effect. J. Org. Chem. 76, 4697–4702. doi:10.1021/jo200360s
Wang, D., and Nugent, W. A. (2007). 2-Deoxyribose as a rich source of chiral 5-carbon building blocks. J. Org. Chem. 72 (19), 7307–7312. doi:10.1021/jo0712143
Keywords: crop protection agent, cross-coupling, green chemistry, levoglucosenone, Michael addition
Citation: Petracco E, Flourat AL, Belhomme M-C, Castex S, Brunissen F, Brunois F, Peru AAM, Allais F and Haudrechy A (2024) Green synthesis of (R)-3-hydroxy-decanoic acid and analogs from levoglucosenone: a novel access to the fatty acid moiety of rhamnolipids. Front. Chem. 12:1362878. doi: 10.3389/fchem.2024.1362878
Received: 29 December 2023; Accepted: 11 March 2024;
Published: 19 April 2024.
Edited by:
Najmedin Azizi, Chemistry and Chemical Engineering Research Center, IranReviewed by:
Gleiston Dias, State University of Campinas, BrazilTanmay Pati, Rensselaer Polytechnic Institute, United States
Copyright © 2024 Petracco, Flourat, Belhomme, Castex, Brunissen, Brunois, Peru, Allais and Haudrechy. This is an open-access article distributed under the terms of the Creative Commons Attribution License (CC BY). The use, distribution or reproduction in other forums is permitted, provided the original author(s) and the copyright owner(s) are credited and that the original publication in this journal is cited, in accordance with accepted academic practice. No use, distribution or reproduction is permitted which does not comply with these terms.
*Correspondence: Amandine L. Flourat, YW1hbmRpbmUuZmxvdXJhdEBhZ3JvcGFyaXN0ZWNoLmZy; Florent Allais, ZmxvcmVudC5hbGxhaXNAYWdyb3BhcmlzdGVjaC5mcg==; Arnaud Haudrechy, YXJuYXVkLmhhdWRyZWNoeUB1bml2LXJlaW1zLmZy
†These authors have contributed equally to this work