- 1Centre for Nano and Material Sciences, Jain University, Jain Global Campus, Ramanagara, Bangalore, Karnataka, India
- 2Centre for Green Technology, School of Civil and Environmental Engineering, The University of Technology Sydney, Sydney, NSW, Australia
- 3Department of Chemical Engineering, School of Physics, Aristotle University of Thessaloniki, Thessaloniki, Greece
Editorial on the Research Topic
Shape and size dependent nanostructures for environmental applications
The cooperative chase to comprehend the dynamical role of functional nanostructures over the immense backdrop of environmental remediation has taken the cornerstone of the current topic “Shape and size dependent nanostructures for environmental applications”. Surmounting the conventional precincts, the issue has aimed to platform hot research, perspectives, refined reviews and mini-reviews with legitimate impact. In this context, explanatory staging of nucleation and growth dynamics of nanostructures, exploration of relative reaction selectivity, synchronous monitoring of nano-micro factors distressing the reactivity and elaborative demarcation of effectual association of anisotropic and dimensional aspects over ultimate competence, are taken as major headlines for the contents of the issue. Moreover, exploring innovative synthesis tactics, nano-micro level scientific insights, and compressive environmental applications were selectively chosen as the moral integrity of the topic. The promising lead contributing articles of the issue illustrate synergy among anisotropic and dimensional factors, ecological relevance, efficiency and sustainability of nanostructured materials in a pervasive way. The current editorial propagates through the contributions of unique research upshots further expanding the acquaintance on anisotropic and dimension-controlled materials for energy-environmental confronts paving the way for future novel research and discoveries (Figure 1).
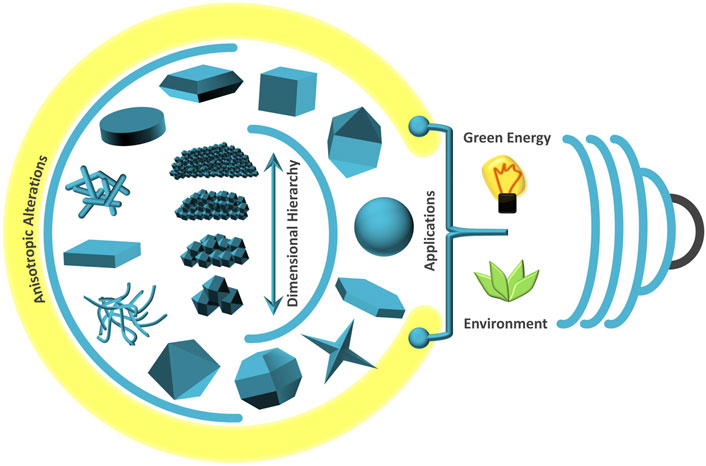
FIGURE 1. Nanostructured materials with anisotropic alterations and dimensional hierarchy for green-sustainable energy-environmental applications.
The advent of functional nanostructured materials has gained astounding anticipation as a green-sustainable elucidation of Energy-Environmental deluge repercussioned via man-made blunders. Surveying recent breakthroughs in nanostructure research unveils a multitude of applications. The employment of nanostructured materials as chemoelectric sensors, as detectors and as nanoelectronics has brought a tremendous insurrection in global environmental monitoring, advanced hazard detection and emission control processes. Furthermore, the remarkable role of nanoscale materials in global bio-environmental air-water-soil contamination treatment via adsorption, filtration, conversion and degradation of poisonous gases, radioactive wastes, organic-inorganic chemicals, elemental hazardous materials and micro-pathogens is invincible (Khin et al., 2012; Mauter and Elimelech, 2008). Furthermore, nanomaterial-based photovoltaic, photocatalytic, photoelectrocatalytic and photothermocatalytic material architecture for sustainable power generation has restructured the green energy segment (Swain et al., 2023). Advancing further, nanostructured materials-based supercapacitors and efficient battery technology have taken enormous attention in green energy storage research (Bhol et al., 2023; Mousavi et al., 2023). In this context, nanostructures have revolutionized environmental applications by offering a spectrum of opportunities to tackle pressing issues.
Such endeavour has underpinned the architecture of copious dynamic nano-micro structured materials with manoeuvred physio-physical-electrochemical attributes. Out of the assorted approaches, the catalytic application of nanostructured materials for energy-environmental remediation has evolved as a dexterous technology. The mainstay criterion of such splendid research is to engineer a functional material with elevated quantum yield, proficient conversion efficiency, and relegated disbursement. Conversely, the conventional physical-biological procedures are associated with inescapable boundaries. In this context, the meticulous fabrication of nanostructured material with controlled size and morphology is established to achieve superior catalytic efficiency. The shape and size-dependent properties of nanostructures offer a myriad of potential for tackling environmental challenges.
The morphology and dimension of nano-structured materials strongly hamper the dynamics of surface-coupled catalysis. The pivotal role of the surface/volume ratio of nanostructured particles with copious sizes towards manipulated catalysis behaviour is well evidenced (Zhou et al., 2009). Drastic alteration in size and morphology impacts the overall physicochemical and electrochemical properties. Anisotropic growth of crystals demonstrates the exposure of diverse active surfaces (Gupta et al., 2023; Baral et al., 2021), alteration in bond energy value, the conception of electron localization/delocalization centres (Madhu et al., 2023), exciton injection via lattice mismatch (Kundu et al., 2023) and alteration in active surface area (Konar et al., 2023) like parameters. Morphology disparity strictly facilitates distortion in atomic arrangement and steric constraints, thus impacting the overall chemo-absorption course (Wu et al., 2021). Desirable surface coordination of catalytically active atoms or domains substantially impacts the ultimate catalytic activity, selectivity and stability (Li and Shen, 2014).
On the other hand, selective exposure of high and low-indexed polar functional facets actively alters the facet-oriented catalytic interaction, coordinative unsaturated sites, surface oxygen mobility, surface oxygen vacancy, and acidic-basic sites responsible for diverse catalytic activity and selectivity (Baral and Parida, 2020). Nevertheless, the diverse electron mobility over selective facets alters the electro-oxidation-reduction process in a superior manner compared to traditional counterparts (Xie and Shen, 2009; Khobragade et al., 2021). Moreover, selective surface exposure with a high concentration of surface oxygen vacancy was reported to drastically convert the lattice oxygen to active oxygen and thus alter the ultimate activity (Zheng et al., 2019).
Accounting for the effectual association of catalyst dimension towards catalysis, it was precisely documented that diminutive particle size supports rapid charge transfer dynamics via minimal charge diffusion path and deteriorated resistance (Dong et al., 2018). Nevertheless, size-controlled nano-structured particles self-assembled to create the foundation to facilitate crystalline array formation and thus impact the reactivity. Drastic modification in particle size altering population of defect sites responsible for elevated catalysis over Au nanoparticles, is well authenticated by (Liang et al., 2022). The dimension of metal nanoparticles contributes to the electronic properties and plays a vital role in reactant adsorption, activation and desorption phenomena (Li et al., 2011). Disentangling physicochemical and electronic attributes of nano-structured catalysts exposes new concourse for catalyst fabrication (Wang et al., 2019).
With colossal contentment in this forum issue, we are emphasising and collectively conveying some of the hot innovations and critical review studies on the area of morphology and controlled dimension-dependent nanostructure-based catalysis. These contributions exemplify how introducing anisotropic features and controlling the catalyst dimensions can neatly expand the physicochemical attributions of nanocatalytic materials.
Plasmonic absorbance and emission are consequences of the size and dimension of the noble metals. In this regard, diverse plasmonic noble metals have been meticulously architected (Larsen et al., 2016; Bhavya et al., 2020). Advancements in developing bimetallic plasmonic alloys have also been achieved to surmount associated metal-oxidations like shortcomings (Vega et al., 2021). Combining size-controlled noble plasmonic materials with secondary shape-selective nanostructures can be a constructive avenue in catalysis as interaction and stabilization of metallic ions over specific surfaces of nanomaterials plays a vital role towards ultimate catalytic activity (Lykaki et al., 2018). In this context, the fabrication of size-controlled noble metals and successive hybridization over core catalytic material can efficiently elevate the overall activity to a demonstrable level (Gao et al., 2014; Yin et al., 2018; Herring and Montemore, 2023). Carbonaceous materials like fullerene, graphene, and carbon nanotubes with higher active surface area also grabbed the attention as efficient substrate cum support material for dimension-controlled plasmonic nano metals (Lam and Luong, 2014; Zhang et al., 2014; Baral et al., 2021). A proper assortment of confounding active surface area, elevated conductivity, and graphitic structure invent carbonaceous materials as magnificent support material for the core catalysts (Sun et al., 2013).
The desire to synthesize an improved size controlled noble bimetallic alloy and further hybrid formation with carbonaceous material and morphology triggered metal oxides pinned the foundation for the development of a noble bimetallic alloy: carbon nanotube: anisotropic metal oxide based ternary hybrid catalyst.
In a viewpoint article, Gyves et al. validated the consequential effect of modification of Au-Cu bi-metallic alloy over multiwalled carbon nanotubes (MWCNTs), and CeO2-nanobar. Nevertheless, comparative studies by intriguing CeO2–nanorod in place of CeO2-nanobar was also carried out towards electrocatalytic glycerol (sustainable material for fuel cell) oxidation analysis in alkaline media (Simões et al., 2010). A clear illustration of the effect of morphology and dimension on catalysis was well documented.
The importance of dimension-controlled nano metallic alloys is further articulated and exemplified by Mi et al. Despite scores of improvement, numerous uncertainties remain at the forefront, and organised data is limited. In accordant with this, a quality review work was reported emphasizing and summarizing the vital points like current development on Au–Cu nanostructures, controlled-meticulous fabrication strategies, emerging applications and future prospective.
In the vast dimension of catalysis, polymers have evolved as expectant active catalytic and support materials. Undoubtedly, controllable textural cum morphological parameters attributing to changeable electronic features make polymers inimitable candidates. Auxiliary porosity creation expands the dynamic surface area of polymers and hence elevates the activity (Kaur et al., 2011; Sun et al., 2015). Finely, tuning catalyst-polymer structure−property relationships can alter the dynamics of the catalytic process (Madhavan et al., 2008; Liu et al., 2012). In this context, introducing dimensional and morphological hierarchy over polymeric materials can sternly amend the absolute activity. Accounting this, the augmented efficiency of hollow-structured Prussian white (PW) polymer with an elevated surface area compared to cubic crystals is well attested by (Bu et al., 2014).
In a featured study, Bosacka et al. neatly explored the fabrication and characterization of an S,S′-thiodi-4,1-phenylene bis(thio-methacrylate)-co-divinylbenzene (DMSPS-co-DVB) nanopolymeric catalyst materials with varying DMSPS and DVB proportions. Moreover, the effectual association of synthetic strategy, morphology/dimension, and aniline absorption kinetics are well ascertained.
Moreover, the drastic accent of exhausted CO2 concentration in the open atmosphere is a terrible concern. Despite the fact that diverse techniques like photocatalytic, electrocatalytic and thermocatalytic CO2 fixation have evolved, catalytic CO2 remediation is still a sizzling Research Topic and an imperative requisite. Nevertheless, renewable CO2 offers a promising source of low-carbon-footprint fuels and chemicals (Xie et al., 2022). Accounting for the preposterous conversion efficiency associated with the CO2 condensation technique, catalytic remediation appears to be a more utilizable and viable technology as it proceeds via a catalytic reduction to multi-carbon (C2+) products like formic acid, methanol, oxidative coupled methane, oxidative dehydrogenated ethylene, and carboxylic acids, etc (Yamazaki et al., 2022; Burkart et al., 2019; Von Der Assen et al., 2013).
Considering the mere importance of catalytic CO2 utilization, Kandathil and Manoj articulated a review by briefing the diverse chemical approaches for the developments of anisotropic nanomaterials. Furthermore, the group has evidently illustrated the strategic applications of the anisotropic nanomaterials in CO2 catalytic conversion, the associated recompense, drawbacks, and future challenges.
Along with individual scientific achievement, the research topics accorded over this forum concurrently expose capsulated knowledge and assemble a new-fangled trail for future technological advancement in the associated field. Innovative material architecture, scientific interrelationship and systematic articulation of pre-established research work demonstrated a more exhaustive perception of the topic with cavernous scientific cognisance. The scope of the journal, along with the heterogeneity of embellished articles, suggests the encyclopedic spectrum of this issue.
Last and foremost, the authors and reviewers are deeply appreciated for the fine articulation and refinement of the research upshots associated with the topic “Shape and Size Dependent Nanostructures for Environmental Applications”. We anticipate that the topic will attract scientific literates and investigators nourishing them with novel research insights and will provide them with some cerebral stimulation.
Author contributions
BB: Conceptualization, Investigation, Resources, Software, Validation, Visualization, Writing–original draft, Writing–review and editing. AA: Visualization, Writing–review and editing. KS: Visualization, Writing–review and editing. AS: Resources, Supervision, Validation, Visualization, Writing–review and editing.
Funding
The author(s) declare financial support was received for the research, authorship, and/or publication of this article. JAIN University (JU/MRP/CNMS/16/2022).
Acknowledgments
BB and AS are grateful to JAIN University (JU/MRP/CNMS/16/2022) for the financial assistance, technical and moral support.
Conflict of interest
The authors declare that the research was conducted in the absence of any commercial or financial relationships that could be construed as a potential conflict of interest.
Publisher’s note
All claims expressed in this article are solely those of the authors and do not necessarily represent those of their affiliated organizations, or those of the publisher, the editors and the reviewers. Any product that may be evaluated in this article, or claim that may be made by its manufacturer, is not guaranteed or endorsed by the publisher.
References
Baral, B., Paramanik, L., and Parida, K. (2021). Functional facet isotype junction and semiconductor/r-GO minor Schottky barrier tailored In2S3@r-GO@(040/110)-BiVO4 ternary hybrid. J. Colloid Interface Sci. 585, 519–537. doi:10.1016/j.jcis.2020.10.033
Baral, B., and Parida, K. (2020). {040/110} facet isotype heterojunctions with monoclinic scheelite BiVO4. Inorg. Chem. 59 (14), 10328–10342. doi:10.1021/acs.inorgchem.0c01465
Baral, B., Sahoo, D. P., and Parida, K. (2021). Discriminatory {040}-reduction facet/Ag0 Schottky barrier coupled {040/110}-BiVO4@Ag@CoAL-LDH Z-scheme isotype heterostructure. Inorg. Chem. 60 (3), 1698–1715. doi:10.1021/acs.inorgchem.0c03210
Bhavya, M. B., Manippady, S. R., Saxena, M., B, R. P., John, N. S., Balakrishna, R. G., et al. (2020). Gold nanorods as an efficient substrate for the detection and degradation of pesticides. Langmuir 36 (26), 7332–7344. doi:10.1021/acs.langmuir.0c00809
Bhol, P., Jagdale, P. B., Jadhav, A. H., Saxena, M., and Samal, A. K. (2023). All-solid-state supercapacitors based on cobalt magnesium telluride microtubes decorated with tellurium nanotubes. ChemSusChem, e202301009. doi:10.1002/cssc.202301009
Bu, F., Hu, M., Xu, L., Meng, Q., Mao, G.-Y., Jiang, D., et al. (2014). Coordination polymers for catalysis: enhancement of catalytic activity through hierarchical structuring. Chem. Commun. 50 (62), 8543–8546. doi:10.1039/c4cc02909g
Burkart, M. D., Hazari, N., Tway, C. L., and Zeitler, E. L. (2019). Opportunities and challenges for catalysis in carbon dioxide utilization. ACS Catal. 9 (9), 7937–7956. doi:10.1021/acscatal.9b02113
Dong, C., Lian, C., Hu, S., Deng, Z., Gong, J., Li, M., et al. (2018). Size-dependent activity and selectivity of carbon dioxide photocatalytic reduction over platinum nanoparticles. Nat. Commun. 9 (1), 1252–1311. doi:10.1038/s41467-018-03666-2
Gao, C., Hu, Y., Wang, M., Chi, M., and Yin, Y. (2014). Fully alloyed Ag/Au nanospheres: combining the plasmonic property of Ag with the stability of Au. J. Am. Chem. Soc. 136 (20), 7474–7479. doi:10.1021/ja502890c
Gupta, S., Jain, P., Jagadeesan, D., and Vinod, C. P. (2023). Morphology-dependent catalysis by CO3O4 nanostructures in atmospheric pressure carbon dioxide hydrogenation. J. Phys. Chem. C 127 (27), 13055–13064. doi:10.1021/acs.jpcc.3c02857
Herring, C. J., and Montemore, M. M. (2023). Mechanistic insights into plasmonic catalysis by dynamic calculations: O2 and N2 on Au and Ag nanoparticles. Chem. Mat. 35 (4), 1586–1593. doi:10.1021/acs.chemmater.2c03061
Kaur, P., Hupp, J. T., and Nguyen, S. T. (2011). Porous organic polymers in catalysis: opportunities and challenges. ACS Catal. 1 (7), 819–835. doi:10.1021/cs200131g
Khin, M. M., Nair, A. S., Babu, V., Murugan, R., and Ramakrishna, S. A. (2012). A review on nanomaterials for environmental remediation. Energy Environ. Sci. 5 (8), 8075. doi:10.1039/c2ee21818f
Khobragade, R., Roškarič, M., Žerjav, G., Košiček, M., Zavašnik, J., Van De Velde, N., et al. (2021). Exploring the effect of morphology and surface properties of nanoshaped Pd/CeO2 catalysts on CO2 hydrogenation to methanol. Appl. Catal. A 627, 118394. doi:10.1016/j.apcata.2021.118394
Konar, S., Shaik, M. A. S., Samanta, D., Shaw, M., Basu, R., Mahto, M.Kr., et al. (2023). Carbon dots as a fluorescent probe for appraising morphology-dependent free-radical-scavenging properties of CuO nanostructures. ChemistrySelect 8 (23), e202204685. doi:10.1002/slct.202204685
Kundu, B., Shajahan, A. S., Chakraborty, B., Behera, R. K., Sarangi, M. K., Pradhan, D., et al. (2023). Probing morphology-dependent CDS/MOS2 heterostructures for photocatalytic and light-sensing applications. ACS Appl. Nano Mat. 6 (24), 23078–23089. doi:10.1021/acsanm.3c04371
Lam, E., and Luong, J. H. T. (2014). Carbon materials as catalyst supports and catalysts in the transformation of biomass to fuels and chemicals. ACS Catal. 4 (10), 3393–3410. doi:10.1021/cs5008393
Larsen, G. K., Farr, W. M., and Murph, S. E. H. (2016). Multifunctional Fe2O3–Au nanoparticles with different shapes: enhanced catalysis, photothermal effects, and magnetic recyclability. J. Phys. Chem. C 120 (28), 15162–15172. doi:10.1021/acs.jpcc.6b03733
Liang, C., Cheong, J. Y., Sitaru, G., Rosenfeldt, S., Schenk, A. S., Gekle, S., et al. (2021). Size-dependent catalytic behaviour of gold nanoparticles. Adv. Mater. Interfaces 9 (4), 2100867. doi:10.1002/admi.202100867
Li, Y., Liu, Q., and Shen, W. (2011). Morphology-dependent nanocatalysis: metal particles. Dalton Trans. 40 (22), 5811. doi:10.1039/c0dt01404d
Li, Y., and Shen, W. (2014). Morphology-dependent nanocatalysts: rod-shaped oxides. Chem. Soc. Rev. 43 (5), 1543–1574. doi:10.1039/c3cs60296f
Liu, F., Wang, Q., Sun, Q., Zhu, L., Meng, X., and Xiao, F. (2012). Transesterification catalyzed by ionic liquids on superhydrophobic mesoporous polymers: heterogeneous catalysts that are faster than homogeneous catalysts. J. Am. Chem. Soc. 134 (41), 16948–16950. doi:10.1021/ja307455w
Lykaki, M., Pachatouridou, E., Carabineiro, S. A. C., Iliopoulou, E. F., Andriopoulou, C., Kallithrakas-Kontos, N., et al. (2018). Ceria nanoparticles shape effects on the structural defects and surface chemistry: implications in CO oxidation by Cu/CeO2 catalysts. Appl. Catal. B 230, 18–28. doi:10.1016/j.apcatb.2018.02.035
Madhavan, N., Jones, C. W., and Weck, M. (2008). Rational approach to polymer-supported catalysts: synergy between catalytic reaction mechanism and polymer design. Acc. Chem. Res. 41 (9), 1153–1165. doi:10.1021/ar800081y
Madhu, R., Karmakar, A., and Kundu, S. (2023). Morphology-dependent electrocatalytic behavior of cobalt chromite toward the oxygen evolution reaction in acidic and alkaline medium. Inorg. Chem. 62 (6), 2726–2737. doi:10.1021/acs.inorgchem.2c03840
Mauter, M. S., and Elimelech, M. (2008). Environmental applications of carbon-based nanomaterials. Environ. Sci. Technol. 42 (16), 5843–5859. doi:10.1021/es8006904
Mousavi, S. M., Hashemi, S. A., Kalashgrani, M. Y., Gholami, A., Binazadeh, M., Chiang, W., et al. (2023). Recent advances in energy storage with graphene oxide-for supercapacitor technology. Sustain. Energy Fuels 7 (21), 5176–5197. doi:10.1039/d3se00867c
Simões, M., Baranton, S., and Coutanceau, C. (2010). Electro-oxidation of glycerol at Pd based nano-catalysts for an application in alkaline fuel cells for chemicals and energy cogeneration. Appl. Catal. B 93 (3–4), 354–362. doi:10.1016/j.apcatb.2009.10.008
Sun, Q., Dai, Z., Meng, X., Wang, Q., and Xiao, F. (2015). Task-specific design of porous polymer heterogeneous catalysts beyond homogeneous counterparts. ACS Catal. 5 (8), 4556–4567. doi:10.1021/acscatal.5b00757
Sun, S., Zhang, G., Gauquelin, N., Chen, N., Zhou, J., Yang, S., et al. (2013). Single-atom catalysis using Pt/graphene achieved through atomic layer deposition. Sci. Rep. 3 (1), 1775–1779. doi:10.1038/srep01775
Swain, S., Iqbal, A., Patil, S. A., Thapa, R., Saxena, M., Jadhav, A. H., et al. (2023). Octahedral Pd3Cu7 catalysts on diverse support materials for efficient hydrogen evolution: theoretical investigation and mechanistic perspective. ACS Appl. Mat. Interfaces 15 (43), 50134–50147. doi:10.1021/acsami.3c08498
Vega, L., Aleksandrov, H. A., Farris, R., Bruix, A., Illas, F., and Neyman, K. M. (2021). Chemical ordering in Pt–Au, Pt–Ag and Pt–Cu nanoparticles from density functional calculations using a topological approach. Mat. Adv. 2 (20), 6589–6602. doi:10.1039/d1ma00529d
Von Der Assen, N., Jung, J., and Bardow, A. (2013). Life-cycle assessment of carbon dioxide capture and utilization: avoiding the pitfalls. Energy Environ. Sci. 6 (9), 2721. doi:10.1039/c3ee41151f
Wang, H., Gu, X.-K., Zheng, X., Pan, H., Zhu, J., Chen, S., et al. (2019). Disentangling the size-dependent geometric and electronic effects of palladium nanocatalysts beyond selectivity. Sci. Adv. 5, eaat6413. doi:10.1126/sciadv.aat6413
Wu, D., Baaziz, W., Gu, B., Marinova, M., Hernández, W. Y., Zhou, W., et al. (2021). Surface molecular imprinting over supported metal catalysts for size-dependent selective hydrogenation reactions. Nat. Catal. 4 (7), 595–606. doi:10.1038/s41929-021-00649-3
Xie, X., and Shen, W. (2009). Morphology control of cobalt oxide nanocrystals for promoting their catalytic performance. Nanoscale 1 (1), 50–60. doi:10.1039/b9nr00155g
Xie, Y., Ou, P., Wang, X., Xu, Z., Li, Y. C., Wang, Z., et al. (2022). High carbon utilization in CO2 reduction to multi-carbon products in acidic media. Nat. Catal. 5 (6), 564–570. doi:10.1038/s41929-022-00788-1
Yamazaki, Y., Miyaji, M., and Ishitani, O. (2022). Utilization of low-concentration CO2 with molecular catalysts assisted by CO2-capturing ability of catalysts, Additives, or Reaction Media. J. Am. Chem. Soc. 144 (15), 6640–6660. doi:10.1021/jacs.2c02245
Yin, J., Wang, Y., Song, C., Zheng, L., Ma, N., Liu, X., et al. (2018). Hybrid Au–Ag nanostructures for enhanced plasmon-driven catalytic selective hydrogenation through visible light irradiation and surface-enhanced Raman scattering. J. Am. Chem. Soc. 140 (3), 864–867. doi:10.1021/jacs.7b11293
Zhang, Z., Sun, T., Chen, C., Xiao, F., Zheng, G., and Wang, S. (2014). Bifunctional nanocatalyst based on three-dimensional carbon nanotube–graphene hydrogel supported Pd nanoparticles: one-pot synthesis and its catalytic properties. ACS Appl. Mat. Interfaces 6 (23), 21035–21040. doi:10.1021/am505911h
Zheng, X., Li, Y., Zhang, L., Shen, L., Xiao, Y., Zhang, Y., et al. (2019). Insight into the effect of morphology on catalytic performance of porous CeO2 nanocrystals for H2S selective oxidation. Appl. Catal. B 252, 98–110. doi:10.1016/j.apcatb.2019.04.014
Keywords: nanostructures, dimensions, morphology, green energy, environmental applications and catalysis
Citation: Baral B, Altaee A, Simeonidis K and Samal AK (2024) Editorial: Shape and size dependent nanostructures for environmental applications. Front. Chem. 12:1362033. doi: 10.3389/fchem.2024.1362033
Received: 27 December 2023; Accepted: 08 January 2024;
Published: 22 January 2024.
Edited and reviewed by:
Sougata Ghosh, RK University, IndiaCopyright © 2024 Baral, Altaee, Simeonidis and Samal. This is an open-access article distributed under the terms of the Creative Commons Attribution License (CC BY). The use, distribution or reproduction in other forums is permitted, provided the original author(s) and the copyright owner(s) are credited and that the original publication in this journal is cited, in accordance with accepted academic practice. No use, distribution or reproduction is permitted which does not comply with these terms.
*Correspondence: Akshaya K. Samal, aksamal@gmail.com, s.akshaya@jainuniversity.ac.in